- 1Department of Rheumatology and Inflammation Research, University of Gothenburg, Gothenburg, Sweden
- 2Department of Microbiology and Immunology, University of Gothenburg, Gothenburg, Sweden
- 3Institute of Life Sciences, Jiangsu University, Zhenjiang, China
- 4B Cell Physiopathology Unit, Immunology Research Area, Bambino Gesù Children's Hospital IRCSS, Rome, Italy
- 5Unit of Diagnostic Immunology, Department of Laboratories, Bambino Gesù Children's Hospital IRCCS, Rome, Italy
Immunological memory ensures life-long protection against previously encountered pathogens, and in mice and humans the spleen is an important reservoir for long-lived memory B cells (MBCs). It is well-established that integrins play several crucial roles in lymphocyte survival and trafficking, but their involvement in the retention of MBCs in secondary lymphoid organs, and differences between B cell subsets in their adhesion capacity to ICAM-1 and/or VCAM-1 have not yet been confirmed. Here, we use an autoimmune mouse model, where MBCs are abundant, to show that the highest levels of LFA-1 and VLA-4 amongst B cells are found on MBCs. In vivo blockade of VLA-4 alone or in combination with LFA-1, but not LFA-1 alone, causes a release of MBCs from the spleen into the blood stream. In humans, we find that in peripheral blood, spleens, and tonsils from healthy donors the highest expression levels of the integrins LFA-1 and VLA-4 are also found on MBCs. Consistent with this, we found MBCs to have a higher capacity to adhere to ICAM-1 and VCAM-1 than naïve B cells. In patients with the autoimmune disease rheumatoid arthritis, it is the MBCs that have the highest levels of LFA-1 and VLA-4; moreover, compared with healthy donors, naïve B and MBCs of patients receiving anti-TNF medication have enhanced levels of the active form of LFA-1. Commensurate levels of the active αL subunit can be induced on B cells from healthy donors by exposure to the integrin ligands. Thus, our findings establish the selective use of the integrins LFA-1 and VLA-4 in the localization and adhesion of MBCs in both mice and humans.
Introduction
Interactions between integrins and their ligands are essential for maintaining the location of leukocytes in general, and mediate the extravasation of cells into tissues as well as their retention in peripheral lymphoid organs (1). In particular, marginal zone (MZ) B cells not only express higher levels of the LFA-1 (αLβ2) and VLA-4 (α4β1) integrins than do their neighbors the follicular (FO) B cells (2), but also depend on these receptors for their retention in the splenic marginal zone; MZ B cells retained in the spleen are released to the circulation when the interaction with ICAM-1 and VCAM-1 is blocked, both in wild-type and in autoimmune mice (2, 3). Appropriately, the LFA-1 and VLA-4 ligands, ICAM-1 and VCAM-1, are expressed in the splenic marginal sinus in mice (2), and VCAM-1 is expressed in the perifollicular and marginal zone in the human spleen (4).
Memory B cells (MBCs) are key components of immunological memory, and consequently serve as mediators of disease when they react to self-antigens in autoimmunity. In humans, unswitched MBCs (also referred to as IgM MBCs or MZ-like B cells) express high levels of the LFA-1 subunit β2 (5), and the VLA-4 integrin is involved in the activation of MBCs (6). As well as harboring MZ B cells, the spleen is an important reservoir for MBCs (7–9); although to date the mechanism of their retention there has not been identified, LFA-1 and/or VLA-4 integrins are obvious candidates for that role. Here we investigate this possibility using SLC−/− (surrogate light chain-deficient) mice, which share features with autoimmune mouse models, including spontaneous formation of germinal centers (GCs) and MBCs (3, 10–12). Furthermore, in humans we examine the expression pattern of the LFA-1 and VLA-4 integrins in B-cell subsets from secondary lymphoid organs as well as functional properties of these integrins in PB of healthy donors and patients with rheumatoid arthritis.
Materials and Methods
Mice
SLC−/− mice (13), previously backcrossed for >10 generations on the C57BL6/OlaHsd background, which lacks alpha-synuclein (14), were bred after embryo transfer onto the C57BL6/NCrl background, and intercrossed to establish SLC−/− mice with an intact alpha-synuclein locus. Mice were kept at the University of Gothenburg (EBM) SPF animal facility and bred under project license authorization (2013/81 and 2016/10). Control mice (C57Bl/6NCrl) were purchased from Charles River (Germany) and Taconic (Denmark). Female mice aged 5–6 months were used throughout.
Human Samples
Peripheral blood mononuclear cells (PBMCs), tonsils and spleens were used for the staining of integrins. The spleens were from children (6–10 years of age) undergoing surgery due to vessel malformations. The study on the splenic samples was approved by the Ethical Committee of Ospedale Pediatrico Bambino Gesù in Rome. Informed consent was obtained from the children's parents. Tonsils were collected from children (1–9 years of age) undergoing tonsillotomy. As no personal information or identity was recorded, no written consent or approval by the Human Research Ethics Committee was needed (Swedish law 2003: 460, paragraphs 4 and 13). PBMCs from patients with RA (57 ± 12 years of age) positive for both rheumatoid factor and antibodies to citrullinated proteins, except two patients that were positive only for rheumatoid factor (Supplementary Table 1), were obtained from the Sahlgrenska University Hospital (660-11, approved 2011-09-07; T996-13, approved 2013-12-12 and 334-15, approved 2015-06-11 all by the Ethical Committee of Gothenburg). In terms of the controls for PBMCs, as we did not have sufficient numbers of age- and sex-matched controls we used additional controls that were not matched. Comparing the two control groups used in our study, showed no significant differences in integrin expression. The study was performed following the guidelines of the Declaration of Helsinki.
Flow Cytometry
Single cell suspensions were stained with a cocktail of antibodies using a PBS buffer containing 3% (mouse) or 10% (human) FCS and 1 mM EDTA (see Supplementary Tables 2, 3 for a complete list of Abs recognizing mouse and human antigens) following standard techniques, and analyzed on LSRIITM, FACSVerseTM, FACSLyricTM, or LSRFortessaTM X-20 (all BD Biosciences) flow cytometers. Data were analyzed using FlowJo software version 10 (Treestar Inc.).
Treatment With Antibodies Recognizing α4 and LFA-1 Integrins
This treatment has been described previously (2, 3). Briefly, SLC−/− mice were injected on days 0, or 0 and 5, i.p. with anti-α4 (clone: M17/4; 100 μg) and/or anti-LFA-1 (clone: PS/2; 100 μg) or isotype control antibodies (BioXcell, USA), and sacrificed after 5 h or at day 14. At the time of sacrifice, blood, and spleens were collected.
Adhesion Assay
CD19+ B cells were purified from PBMCs by negative immunomagnetic sorting (Dynabeads® Untouched™ Human B Cells Kit, Invitrogen, Life Technologies). After washing, cells were resuspended in RPMI with 10% FCS at a concentration of 5 x 105 cells/ml. High absorbance plates (Immulon 4 HBX, Extra High Binding, 96 wells, Flat-bottom, VWR) were coated with the ligands (VCAM-1 5 μg/ml; ICAM-1 10 μg/ml) at 37°C for 2 h in 50 μl carbonate buffer. The plate was decanted and then incubated with 200 μl PBS with 1% BSA (1 g BSA in 100 ml PBS) for 1 h at 37°C to block non-specific adhesion. The plate was washed once with RPMI before use. 5 x 104 cells (in 100 μl RPMI with 10% FCS) were added to the wells and incubated for 30 min at 37°C. Non-adherent cells were removed by gentle washing. Subsequently, adherent cells were removed by incubating for 15 min on ice in RPMI with 5 mM EDTA. Both adherent and non-adherent cells were washed once and then stained for flow cytometry.
Pre-treatment of PBMCs With ICAM-1 and VCAM-1 and Staining for Active Conformation of αL Integrin Subunit
PBMCs from HDs and patients with RA under anti-TNF medication were pre-treated with ICAM-1 (20 μg/ml) and VCAM-1 (10 μg/ml) at 37°C for 1 h in RPMI with 10% FCS. Thereafter the cells were stained for the active form of αL with the standard panel of antibodies (Supplementary Table 3).
Statistics
Outliers were removed by using the ROUT method with Q set to 1%. Shapiro-Wilk test was used to evaluate normality. The statistical significance of differences between tissues and treatments was determined using tests as appropriate: an unpaired or paired two-tailed t-test or ordinary one-way ANOVA with Tukey's multiple comparisons, using Graph-Pad Prism version 7 (La Jolla, CA, USA). Significance levels are represented on figures by asterisks: *p < 0.05; **p < 0.01; ***p < 0.001; ****p < 0.0001.
Results
Sustained Treatment With Anti-integrin Antibodies Depletes MBCs in the Spleen
The integrins of interest in this study are LFA-1 and VLA-4, and their ligands ICAM-1 and VCAM-1 (Figure 1A). Starting with a population of mature B cells identified as CD19+CD93−CD43−GL7− lymphocytes, MBCs were defined as CD80+CD73+/−PDL2+/− based on the differential expression of the CD80, CD73, and PDL2 surface markers (15), (Supplementary Figure 1; Figure 1B). These are to be described in more detail elsewhere (Aranburu et. al. in preparation); here it suffices to note that the MBCs in SLC−/− mice contain mainly IgM-expressing cells (Figure 1C).
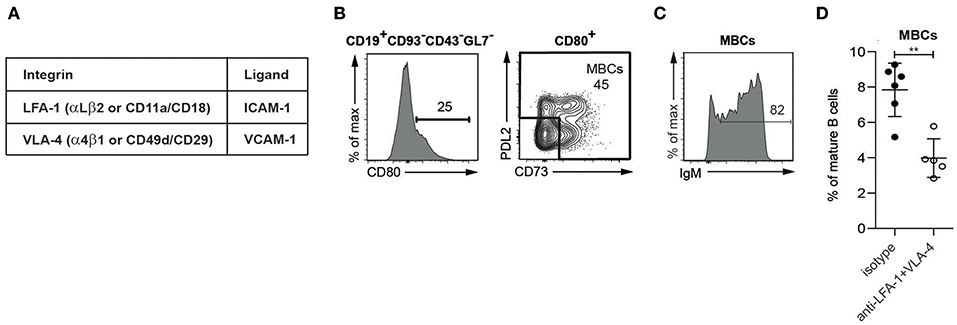
Figure 1. MBCs present in the spleen of SLC−/− mice are dependent on integrins for their retention (A). The integrins (subunits) of interest and their ligands (B–D). Flow cytometric analysis of spleen from SLC−/− mice (B) Gating strategy for MBCs (C) Percentage of IgM-expressing cells in MBCs (D) Percentages of MBCs isolated from spleens of SLC−/− mice treated for 2 weeks with anti-LFA-1 and anti-α4. n = 6 (treated), n = 5 (isotype control); error bars show mean +/–SD; data are representative of two independent experiments. An unpaired two-tailed Student t-test was used (**p < 0.01).
To determine whether the adhesion of mouse MBCs in the spleen depends on integrins, we treated SLC−/− mice with antibodies against LFA-1 and VLA-4. After a 2-week period, the presence of MBCs was significantly reduced (Figure 1D), showing that MBCs rely on the interaction with ICAM-1 and VCAM-1 for their retention in the spleen.
Acute Treatment With anti-VLA-4 Antibodies Induces the Release of MBCs Into PB
To investigate whether the observed integrin-mediated loss of MBCs from the spleen resulted in their accumulation in the circulation, we started by looking at the number of leukocytes in the PB of SLC−/− mice soon (5 h) after the injection of the blocking antibodies. Compared to the injection of control antibodies, leukocyte number more than doubled after the injection of antibodies against both LFA-1 and VLA-4 together, but did not alter significantly when each antibody was used alone (Figure 2A). This is to be contrasted with the situation for the MBCs, where the anti-VLA-4 acted selectively, increasing their release into the blood (Supplementary Figure 2; Figure 2B). On the other hand, the number of MZ B cells was selectively increased by anti-LFA-1 treatment, and blocking with both antibodies increased numbers of MBCs as well as MZ B cells at least 3-fold (Figures 2B,C). Comparison of the proportions of MBCs and MZ B cells as well as their ratios in the blood (Figure 2D) shows the selectivity of anti-VLA-4 treatment for MBC release, whereas MZ B cells release was more dependent on anti-LFA-1 and double-blocking. Flow cytometric analysis of spleen B cell populations 5 h after antibody treatment confirmed the loss of MZ B cells relative to MBCs due to treatment with both antibodies (Figure 2E). These data show that the two integrins of interest have additive effects on the retention of both MZ B cells and MBCs, but that the selectively important partners are LFA-1 for MZ B cells and VLA-4 for MBCs.
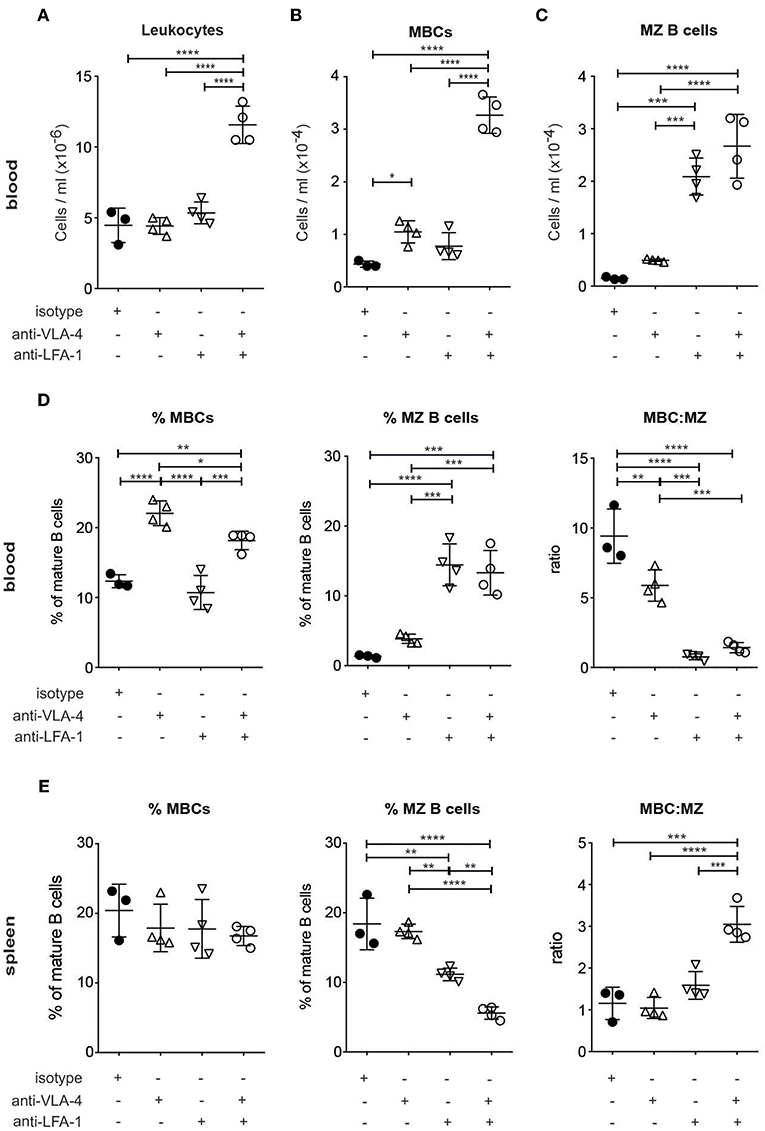
Figure 2. MBCs are acutely dependent on VLA-4 for their retention in the spleen with an additive requirement of LFA-1 (A–D). Flow cytometric analysis of PB taken from SLC−/− mice after 5 h treatment with anti-LFA-1 and/or anti-α4 antibodies (A) Numbers of leukocytes (B) Numbers of MBCs (C) Numbers of MZ B cells (D) %MBCs as proportions of mature B cells (left panel), % of MZ B cells as proportions of mature B cells (middle panel) and ratio of MBC:MZ expressed as proportions of mature B cells (right panel) (E). Flow cytometric analysis of spleen cells: %MBCs as proportions of mature B cells (left panel), % of MZ B cells as proportions of mature B cells (middle panel) and ratio of MBC:MZ expressed as proportions of mature B cells (right panel). n = 4 (treated) and n = 3 (isotype control); error bars show mean +/–SD; data are representative of two independent experiments. One-way ANOVA with Tukey's multiple comparisons was used (*p < 0.05, **p < 0.01, ***p < 0.001, ****p < 0.0001).
Elevated Integrin Expression on MBCs in SLC−/− Mice
Given our observations on their functional effects, we set out to determine the appearance of the four integrin subunits studied with respect to the various mature B cell subsets in SLC−/− mice. Compared to FO B cells, MZ B cells and MBCs expressed at least twice the levels of the LFA-1 integrins (Figure 3). For the VLA-4 subunits, however, it was the MZ B cell subset that expressed the lowest levels, and MBCs by comparison demonstrated several-fold higher expression. These data sit well with our observations on the selective release of MBCs and MZ B cells into the PB, and single out the MBCs as distinct from the naïve B cells (FO and MZ) in expressing high levels of all four integrin subunits, a feature that is likely to be pertinent to the autoimmune status of the SLC−/− mice.
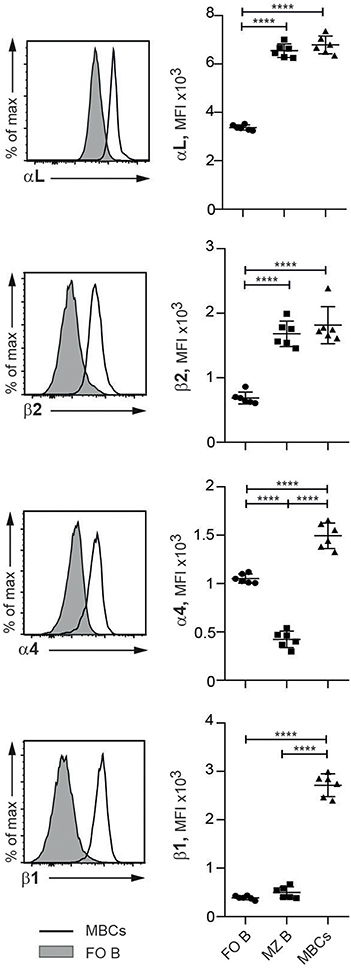
Figure 3. MBCs express higher levels of LFA-1 and VLA-4 than FO and MZ B cells in SLC−/− mice. Flow cytometric analysis of spleen cells from SLC−/− mice. Histograms show the expression of integrins on FO B and MBCs and graphs show the MFIs of integrin subunits αL, β2, α4, and β1 expressed on FO B, MZ B and MBCs. n = 6, data are from three independent experiments; error bars show mean +/–SD. One-way ANOVA with Tukey's multiple comparisons was used (****p < 0.0001).
Integrin Expression Is Elevated on Human MBCs in Peripheral Blood and Secondary Lymphoid Organs
Looking for relevance of our data from SLC−/− mice to human immunity, we investigated the expression patterns of the integrin subunits of interest in human tissues: PBMC, tonsil and spleen (for gating strategy see Supplementary Figure 3). In all three tissues, MBCs (unswitched and switched) expressed higher levels of all four integrin subunits of interest than did their naïve counterparts; these elevations were statistically significant in all cases except for β2 (naïve vs. switched MBCs) and α4 (naïve vs. unswitched MBC) in the spleen (Figures 4A,B,D). Further, both VLA-4 subunits were significantly more highly expressed on switched than on unswitched MBCs from PBMCs and tonsils (Figures 4A,B). Gating on GC B and plasma cells in the tonsils, we also noticed that the levels of all four integrin subunits were higher on the latter (Figure 4C). Thus, in humans as in mice, the integrins of interest here are expressed at higher levels on MBCs than on naïve B cells.
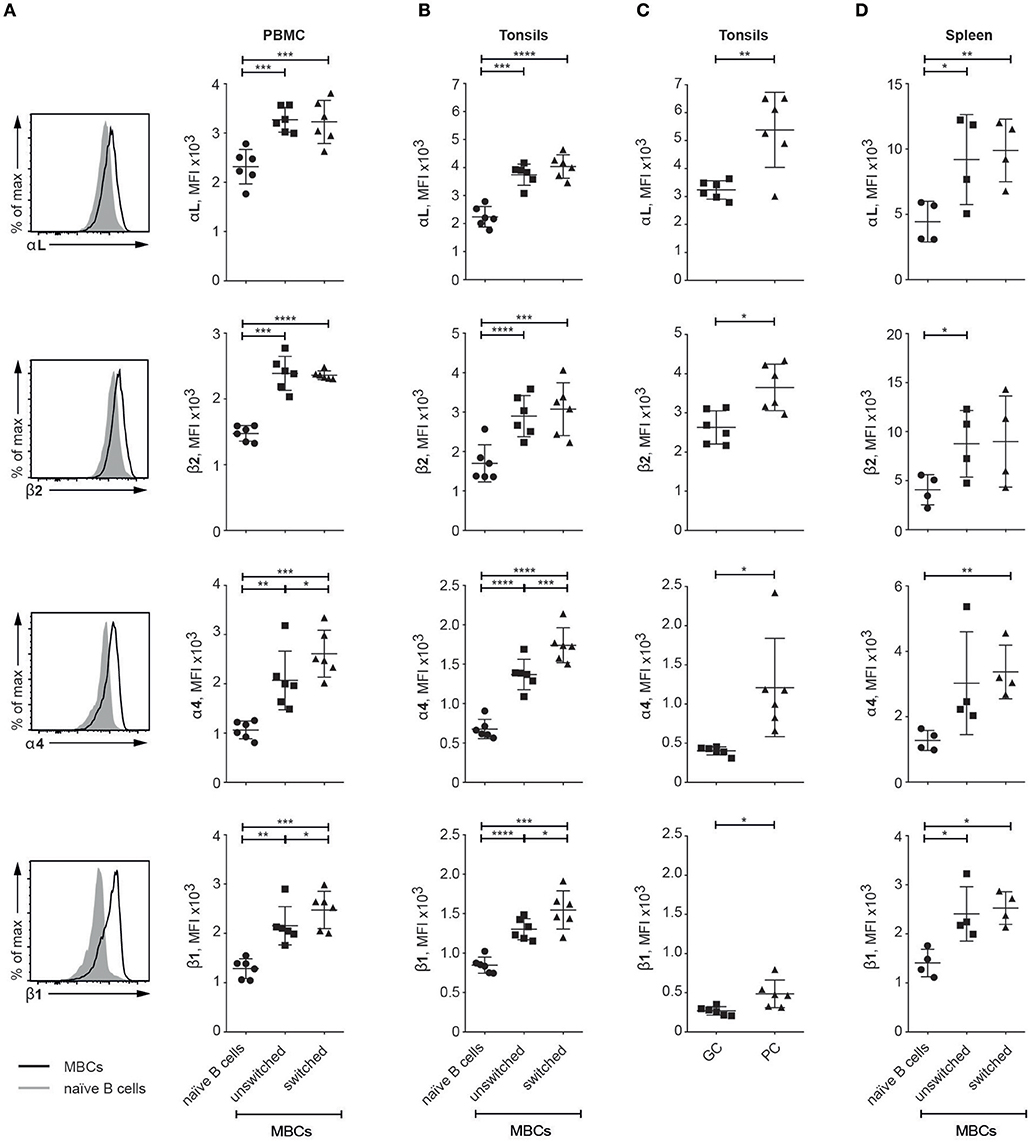
Figure 4. Human MBCs express higher levels of the integrins of interest than naïve B cells (A–D). Flow cytometric analysis of human tissues; graphs compare naïve B cells with unswitched and switched MBC populations as well as GC B cells and plasma cells (PCs) (in C) for their levels of expression of the αL, β2, α4, and β1 integrin subunits (A) PBMC Histograms compare integrin expression levels between naïve B and all MBCs (B) Tonsil (C) Tonsil GC B cell and PCs (D) Spleen. Data are from at least two independent experiments; error bars show mean +/– SD. An unpaired two-tailed Student t-test was used (*p < 0.05, **p < 0.01, ***p < 0.001, ****p < 0.0001).
Greater Adhesion Capacity to ICAM-1 and VCAM-1 in Human MBCs Than in Naïve B Cells
To determine whether the enhanced expression of LFA-1 and VLA-4 integrins on human MBCs compared to naïve B cells was related to adhesion capacity, we performed an ex vivo adhesion assay (2) on human B cell subsets. A higher proportion of the naïve B cells were found in the non-adherent fraction (before EDTA) whereas the unswitched and switched MBCs showed the opposite pattern (Figure 5), thus demonstrating a higher adhesion capacity to ICAM-1 and VCAM-1 than the naïve B cells. The ex vivo demonstration of MBC adhesion to the integrin ligands is complementary support of the in vivo data from our mouse model.
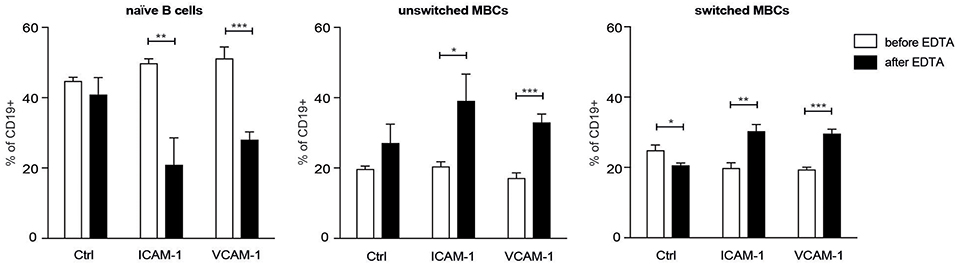
Figure 5. Human unswitched and switched MBCs show a higher adhesion capacity to ICAM-1 and VCAM-1 than do naïve B cells. Flow cytometric analysis of CD19+ B cells from PB of HDs tested for adhesion to the integrins of interest. Shown are the proportions of naïve B cells, unswitched MBCs, and switched MBCs harvested before and after treatment with EDTA. n = 3; data are representative of two independent experiments. An unpaired two-tailed Student t-test was used (*p < 0.05, **p < 0.01, ***p < 0.001).
Higher Than Normal LFA-1 Expression on MBCs in Patients With RA
To test our observations on autoimmune mice in humans, we chose to investigate patients with RA, following studies by others in which antibody blockade of ICAM-1 in such patients was reported to have beneficial effects on various clinical parameters, although no data on the effects on B cells were published (16). As for the MBCs from healthy donors, we found that the MBCs in peripheral blood from patients with RA expressed higher levels of all four integrin subunits of interest than the naïve B cells (Figure 6A). Additionally, and as observed in PBMCs and tonsils in healthy donors, both VLA-4 subunits (α4 and β1) appeared at significantly higher levels on switched than unswitched MBCs.
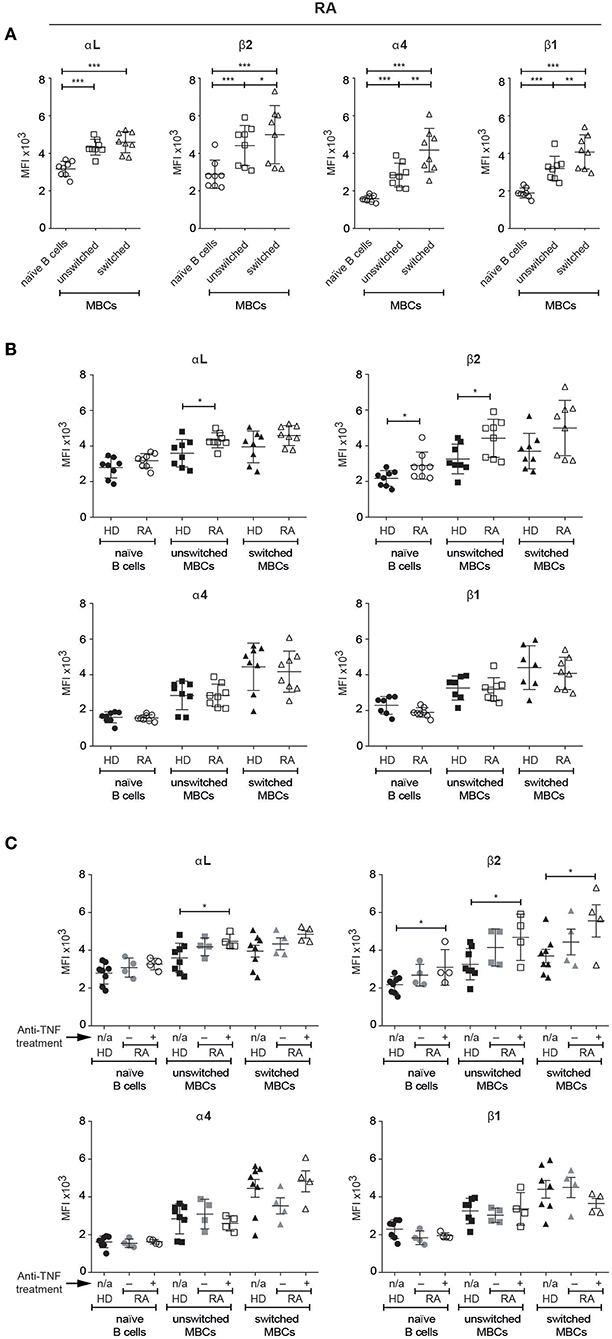
Figure 6. Human unswitched MBCs from patients with RA show an upregulation of LFA-1 subunits. Flow cytometric analysis comparing the expression of αL, β2, α4, and β1 integrin subunits amongst selected B cell populations in PBMCs (A) Patients with RA (B) Comparison between HDs and patients with RA treated with anti-TNF medication or not (C) Comparison between HDs and patients with RA with or w/o anti-TNF medication. Data are pooled from two independent experiments. Error bars show mean +/– SD. An unpaired two-tailed Student t-test was used (*p < 0.05, **p < 0.01, ***p < 0.001).
Comparing B cells in peripheral blood from healthy donors and patients with RA revealed some significant elevations of LFA-1 subunits expression in the RA samples: unswitched MBCs had higher levels of both αL and β2, and naïve B cells had a higher level of β2 than their counterparts from HDs (Figure 6B). As the patients appeared to split into two groups, we looked for an association between the number of swollen/tender joints and integrin expression, but found none (data not shown). In contrast however, dividing the patients into those that receive anti-TNF treatment and those that do not revealed significant differences in LFA-1 expression (Figure 6C). Those treated with anti-TNF had higher expression than HDs of the αL subunit on unswitched MBCs, and of the β2 subunit on all three B cell populations identified.
The Expression of the Active Form of αL can be Induced by Pre-treatment With ICAM-1 and VCAM-1
The antibody that was used to stain for β2 integrin expression recognizes the active form of this subunit (17). We decided, therefore, to investigate also the expression levels of the active form of αL using an antibody known to recognize this form, selecting RA patients treated with anti-TNF medication. Our analysis showed that expression of the active form of αL was elevated relative to HDs on naïve, unswitched and switched MBCs from patients with RA, amongst which active αL expression was invariant across the B cell populations (Figure 7A). Thus, circulating naïve B cells as well as unswitched and switched MBCs in RA patients on anti-TNF medication express the active form of both subunits of the LFA-1 integrin.
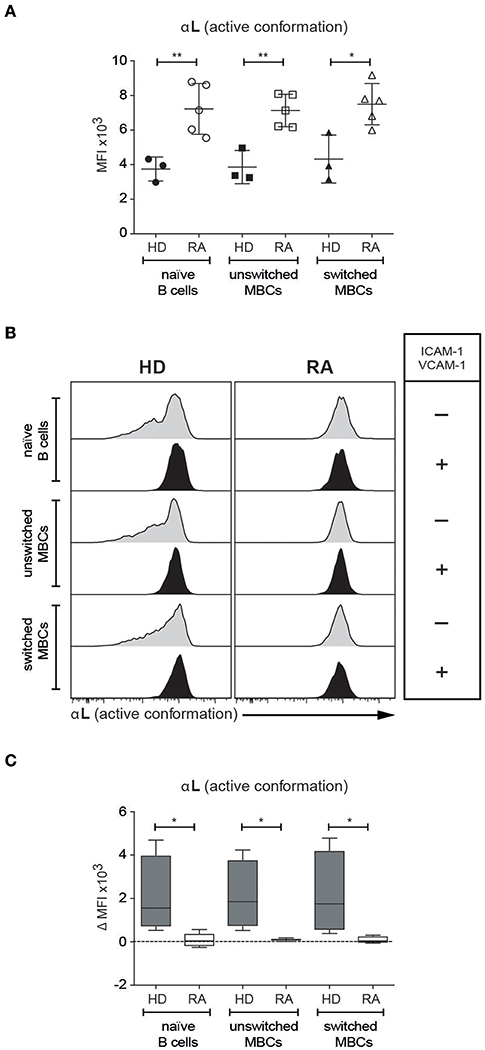
Figure 7. Human naïve, unswitched and switched MBCs from patients with RA show an upregulation of the active form of αL (A). Comparison based on flow cytometric analysis of the expression of the active form of αL integrin subunit amongst selected B cell populations in PBMCs from HD and patients with RA under treatment with anti-TNF medication (B,C). Staining for the active form of αL on PBMCs from HDs and patients with RA under treatment with anti-TNF medication, following pre-treatment with ICAM-1 and VCAM-1 (B) Expression of αL before and after treatment with ICAM-1 and VCAM-1 (C). ΔMFI of αL due to treatment with ICAM-1 and VCAM-1. Data are pooled from two independent experiments. Error bars show mean +/- SD. Unpaired (A) or paired (B,C) two-tailed Student t-tests were used (*p < 0.05, **p < 0.01).
It has previously been shown that patients with RA have higher levels of circulating ICAM-1 (18). To see whether the higher expression levels of the active form of αL seen in patients with RA could be induced in healthy donors in the presence of the ligand, we pre-treated PBMCs from both HDs and patients with ICAM-1 and VCAM-1. In consequence, the level of αL expression on naïve, unswitched and switched MBCs from HDs increased, matching those of their counterparts from the RA patient group (Figures 7B,C), which remained unaffected by the pre-treatment. Thus, the presence of ICAM-1 and VCAM-1 can trigger an increase in the activated form of αL expressed on the surface of PB B cells in healthy donors, to levels already expressed on the B cells of patients with RA.
Discussion
Immunological memory depends on the retention of long-lived lymphocytes primed against specific antigens; as a consequence of this requirement, secure retention of memory cells in appropriate locations is essential. It has already been shown that VLA-4 and LFA-1 are crucial for the retention of MZ B cells in the murine spleen (2, 3). These molecules are therefore likely candidates for maintaining a functional MBC population in secondary lymphoid tissues, and as regulators of B cell trafficking. Here, we provide evidence that they are indeed responsible, via their enhanced expression on MBCs, for the selective retention of B cell memory at sites where ICAM-1 and VCAM-1 occur. Our blocking experiments demonstrate that both LFA-1 and VLA-4 have a role to play, but that their differential expression on MBCs relative to MZ B cells provides for selective placement of the subsets in lymphoid tissues, VLA-4 exerting the stronger pull for MBCs, and LFA-1 being the dominant influence on MZ B cells.
Unswitched human MBCs are known to be located in the splenic marginal zone, whereas switched MBCs are found in the perifollicular zone (19, 20); our results predict that a significant measure of their positional specificity is mediated by ligation to VCAM-1 expressed in these zones (4), and by inference also ICAM-1. For the unswitched MBCs, which depend on the spleen for their generation and survival (8, 21, 22), this receptor-ligand pairing may even be of developmental importance. The enhancement of adhesion capacity of both unswitched and switched MBCs over naïve B cells that we demonstrate is likely to reflect the differential importance for the two subsets of staying in one place.
B cells are one of the key players in RA, as is evident in the beneficial effects of B-cell depletion therapy (23). They are also prominent in a mouse model of RA, collagen-induced arthritis, in which the incidence of arthritis is substantially reduced in the absence of the LFA-1 ligand, ICAM-1 (24). It has been known for some time that ICAM-1 and VCAM-1 are expressed in the synovium and on chondrocytes of patients with RA (25–28), and for not quite so long that MBCs can be found in RA synovium (29). These previous observations are extended by our findings that levels of the active conformations of the LFA-1 subunits are elevated on the B cells of patients with RA on anti-TNF medication, and that the presence of ICAM-1 and VCAM-1 can induce similarly elevated levels of the active αL subunit on B cells from healthy donors. The implication is that the adhesion capacity of B cells, especially MBCs, may be a mediating factor in the development of sites of inflammation in RA. Our data would predict that the beneficial effects of blocking ICAM-1 in some patients with RA (16) might be due, at least in part, to the dual outcomes of reduction in the ICAM-1 induced elevation of active subunit expression, and prevention of MBCs expressing the active form of LFA-1 from adhering to the synovial tissue.
Integrins expressed on B cells, and in particular MBCs, have been found to be relevant to autoimmune conditions other than RA. In multiple sclerosis, interference with the integrin-mediated trafficking of leukocytes to the brain using a blocking antibody to the VLA-4 subunit α4 (natalizumab) results in B cells being trapped in the circulation (30). In systemic lupus erythematosus it has recently been shown that a MBC population expresses a transcriptional profile that includes increased levels of the adhesion molecules αL and β1 (31). Simple blocking of integrin molecules as a therapy for such autoimmune states carries the risk of side effects such as infection (32), and efforts are already being directed toward more specific inhibition; for example, inhibitors targeting gut-specific integrins are now under evaluation for Ulcerative Colitis (33).
Collectively, our results demonstrate that LFA-1 and VLA-4 are more highly expressed on MBCs in both mice and human than on the respective circulating naïve B cells, and MBCs in humans adhere better to ICAM-1 and VCAM-1 than do naïve B cells. Murine MBCs are retained in the spleen by both LFA-1 and VLA-4, but VLA-4 holds the dominant influence via higher expression levels. These data constitute significant steps toward the deciphering of the mechanisms behind lymphocyte retention, release, and trafficking. This understanding could reopen the use of a more specific integrin blockade to address several autoimmune diseases; blocking MBCs from entry into as well as forcing their release into the circulation from the spleen and inflammatory sites could break the positive feedback loop that nourishes autoimmune pathology.
Data Availability
All datasets generated for this study are included in the manuscript and/or the supplementary files.
Ethics Statement
This study was carried out in accordance with the recommendations of the regional ethical review board of Gothenburg and of the local ethical committe of Bambino Gesu Children's Hospital, Rome with written informed consent from all subjects. All subjects gave written informed consent in accordance with the Declaration of Helsinki. The protocol was approved by the regional ethical review board of Gothenburg and by the local ethical committee of Bambino Gesu Children's Hospital, Rome. This study was carried out in accordance with the recommendations of the regional animal ethics committee in Gothenburg, Sweden. The protocol was approved by the regional animal ethics committee in Gothenburg, Sweden.
Author Contributions
AC, NG, OG, and I-LM designed the experiments. AC, CF, DC, IG, KT, LSO, NG, OG, RC, TF, and YW carried out or contributed essential reagents and materials for the experiments. AA and SC contributed substantially to the discussions. AC, I-LM, NG, and OG wrote the manuscript with contributions from the co-authors.
Funding
This work was supported by the Swedish Science Research Council (2018-03128), the Swedish Cancer Foundation (CAN 2016/481), the Swedish Childhood Cancer Fund (PR2018-0170), AFA, ALF: the regional agreement on medical training and clinical research, King Gustav V. Stiftelse, Ingabritt, and Arne Lundbergs Forskningsstiftelse, Swedish Medical Society, Swedish Rheumatism Association (R-758881), Stiftelsen Lars Hiertas minne, Lundgrens Stiftelse, Amlövs Stiftelser, Adlerbertska stiftelsen, The Royal Society of Arts and Sciences in Gothenburg, and Sigurd och Elsa Golje's minne.
Conflict of Interest Statement
The authors declare that the research was conducted in the absence of any commercial or financial relationships that could be construed as a potential conflict of interest.
Acknowledgments
We thank Ms. Linda Löfbom for help with injections.
Supplementary Material
The Supplementary Material for this article can be found online at: https://www.frontiersin.org/articles/10.3389/fimmu.2019.00534/full#supplementary-material
Abbreviations
Ab, antibody; FO, Follicular; HD, Healthy Donor; ICAM-1, Intercellular Adhesion Molecule 1; LFA-1, Lymphocyte function-associated antigen 1; MBC, Memory B Cell; MZ, Marginal Zone; PBMC, Peripheral Blood Mononuclear Cell; RA, Rheumatoid arthritis; SLC, Surrogate light chain; VCAM-1, Vascular cell adhesion protein 1; VLA-4, Very late antigen-4.
References
1. Kinashi T. Overview of integrin signaling in the immune system. Methods Mol Biol. (2012) 757:261–78. doi: 10.1007/978-1-61779-166-6_17
2. Lu TT, Cyster JG. Integrin-mediated long-term B cell retention in the splenic marginal zone. Science. (2002) 297:409–12. doi: 10.1126/science.1071632297/5580/409
3. Ren W, Grimsholm O, Bernardi AI, Hook N, Stern A, Cavallini N, et al. Surrogate light chain is required for central and peripheral B-cell tolerance and inhibits anti-DNA antibody production by marginal zone B cells. Eur J Immunol. (2015) 45:1228–37. doi: 10.1002/eji.201444917
4. Steiniger B, Barth P, Hellinger A. The perifollicular and marginal zones of the human splenic white pulp: do fibroblasts guide lymphocyte immigration? Am J Pathol. (2001) 159:501–12. doi: 10.1016/S0002-9440(10)61722-1
5. Descatoire M, Weller S, Irtan S, Sarnacki S, Feuillard J, Storck S, et al. Identification of a human splenic marginal zone B cell precursor with NOTCH2-dependent differentiation properties. J Exp Med. (2014) 211:987–1000. doi: 10.1084/jem.20132203
6. Silvy A, Altevogt P, Mondiere P, Bella C, Defrance T. A role for the VLA-4 integrin in the activation of human memory B cells. Eur J Immunol. (1997) 27:2757–64. doi: 10.1002/eji.1830271103
7. Di Sabatino A, Carsetti R, Corazza GR. Post-splenectomy and hyposplenic states. Lancet. (2011) 378:86–97. doi: 10.1016/S0140-6736(10)61493-6
8. Kruetzmann S, Rosado MM, Weber H, Germing U, Tournilhac O, Peter HH, et al. Human immunoglobulin M memory B cells controlling Streptococcus pneumoniae infections are generated in the spleen. J Exp Med. (2003) 197:939–45. doi: 10.1084/jem.20022020
9. Mamani-Matsuda M, Cosma A, Weller S, Faili A, Staib C, Garcon L, et al. The human spleen is a major reservoir for long-lived vaccinia virus-specific memory B cells. Blood. (2008) 111:4653–9. doi: 10.1182/blood-2007-11-123844
10. Aranburu A, Hook N, Gerasimcik N, Corleis B, Ren W, Camponeschi A, et al. Age-associated B cells expanded in autoimmune mice are memory cells sharing H-CDR3-selected repertoires. Eur J Immunol. (2018) 48:509–21. doi: 10.1002/eji.201747127
11. Grimsholm O, Ren W, Bernardi AI, Chen H, Park G, Camponeschi A, et al. Absence of surrogate light chain results in spontaneous autoreactive germinal centers expanding VH81X-expressing B cells. Nat Commun. (2015) 6:7077. doi: 10.1038/ncomms8077
12. Keenan RA, De Riva A, Corleis B, Hepburn L, Licence S, Winkler TH, et al. Censoring of autoreactive B cell development by the pre-B cell receptor. Science. (2008) 321:696–9. doi: 10.1126/science.1157533
13. Shimizu T, Mundt C, Licence S, Melchers F, Martensson IL. VpreB1/VpreB2/lambda 5 triple-deficient mice show impaired B cell development but functional allelic exclusion of the IgH locus. J Immunol. (2002) 168:6286–93. doi: 10.4049/jimmunol.168.12.6286
14. Specht CG, Schoepfer R. Deletion of the alpha-synuclein locus in a subpopulation of C57BL/6J inbred mice. BMC Neurosci. (2001) 2:11. doi: 10.1186/1471-2202-2-11
15. Anderson SM, Tomayko MM, Ahuja A, Haberman AM, Shlomchik MJ. New markers for murine memory B cells that define mutated and unmutated subsets. J Exp Med. (2007) 204:2103–14. doi: 10.1084/jem.20062571
16. Kavanaugh AF, Davis LS, Nichols LA, Norris SH, Rothlein R, Scharschmidt LA, et al. Treatment of refractory rheumatoid arthritis with a monoclonal antibody to intercellular adhesion molecule 1. Arthritis Rheum. (1994) 37:992–9.
17. Byron A, Humphries JD, Askari JA, Craig SE, Mould AP, Humphries MJ. Anti-integrin monoclonal antibodies. J Cell Sci. (2009) 122:4009–11. doi: 10.1242/jcs.056770
18. Cush JJ, Rothlein R, Lindsley HB, Mainolfi EA, Lipsky PE. Increased levels of circulating intercellular adhesion molecule 1 in the sera of patients with rheumatoid arthritis. Arthritis Rheum. (1993) 36:1098–102.
19. Dunn-Walters DK, Isaacson PG, Spencer J. Analysis of mutations in immunoglobulin heavy chain variable region genes of microdissected marginal zone (MGZ) B cells suggests that the MGZ of human spleen is a reservoir of memory B cells. J Exp Med. (1995) 182:559–66.
20. Steiniger B, Timphus EM, Jacob R, Barth PJ. CD27+ B cells in human lymphatic organs: re-evaluating the splenic marginal zone. Immunology. (2005) 116:429–42. doi: 10.1111/j.1365-2567.2005.02242.x
21. Chiu SN, Shao PL, Wang JK, Hsu HW, Lin MT, Chang LY, et al. Low immunoglobulin M memory B-cell percentage in patients with heterotaxy syndrome correlates with the risk of severe bacterial infection. Pediatr Res. (2016) 79:271–7. doi: 10.1038/pr.2015.221
22. Piano Mortari E, Baban A, Cantarutti N, Bocci C, Adorisio R, Carsetti R. Heterotaxy syndrome with and without spleen: different infection risk and management. J Allergy Clin Immunol. (2017) 139:1981–84. doi: 10.1016/j.jaci.2016.10.014
23. Dorner T, Lipsky PE. B cells: depletion or functional modulation in rheumatic diseases. Curr Opin Rheumatol. (2014) 26:228–36. doi: 10.1097/BOR.0000000000000000
24. Bullard DC, Hurley LA, Lorenzo I, Sly LM, Beaudet AL, Staite ND. Reduced susceptibility to collagen-induced arthritis in mice deficient in intercellular adhesion molecule-1. J Immunol. (1996) 157:3153–8.
25. Davies ME, Sharma H, Pigott R. ICAM-1 expression on chondrocytes in rheumatoid arthritis: induction by synovial cytokines. Mediators Inflamm. (1992) 1:71–4. doi: 10.1155/S0962935192000140
26. el-Gabalawy H, Gallatin M, Vazeux R, Peterman G, Wilkins J. Expression of ICAM-R (ICAM-3), a novel counter-receptor for LFA-1, in rheumatoid and non-rheumatoid synovium. comparison with other adhesion molecules. Arthritis Rheum. (1994) 37:846–54.
27. Koch AE, Burrows JC, Haines GK, Carlos TM, Harlan JM, Leibovich SJ. Immunolocalization of endothelial and leukocyte adhesion molecules in human rheumatoid and osteoarthritic synovial tissues. Lab Invest. (1991) 64:313–20.
28. Szekanecz Z, Haines GK, Lin TR, Harlow LA, Goerdt S, Rayan G, et al. Differential distribution of intercellular adhesion molecules (ICAM-1, ICAM-2, and ICAM-3) and the MS-1 antigen in normal and diseased human synovia. their possible pathogenetic and clinical significance in rheumatoid arthritis. Arthritis Rheum. (1994) 37:221–31.
29. Souto-Carneiro MM, Mahadevan V, Takada K, Fritsch-Stork R, Nanki T, Brown M, et al. Alterations in peripheral blood memory B cells in patients with active rheumatoid arthritis are dependent on the action of tumour necrosis factor. Arthritis Res Ther. (2009) 11:R84. doi: 10.1186/ar2718
30. Saraste M, Penttila TL, Airas L. Natalizumab treatment leads to an increase in circulating CXCR3-expressing B cells. Neurol Neuroimmunol Neuroinflamm. (2016) 3:e292. doi: 10.1212/NXI.0000000000000292
31. Wang S, Wang J, Kumar V, Karnell JL, Naiman B, Gross PS, et al. IL-21 drives expansion and plasma cell differentiation of autoreactive CD11c(hi)T-bet(+) B cells in SLE. Nat Commun. (2018) 9:1758. doi: 10.1038/s41467-018-03750-7
32. Bloomgren G, Richman S, Hotermans C, Subramanyam M, Goelz S, Natarajan A, et al. risk of natalizumab-associated progressive multifocal leukoencephalopathy. N Engl J Med. (2012) 366:1870–80. doi: 10.1056/NEJMoa1107829
33. Baker KF, Isaacs JD. Novel therapies for immune-mediated inflammatory diseases: what can we learn from their use in rheumatoid arthritis, spondyloarthritis, systemic lupus erythematosus, psoriasis, crohn's disease and ulcerative colitis? Ann Rheum Dis. (2018) 77:175–87. doi: 10.1136/annrheumdis-2017-211555
Keywords: integrins, LFA-1, VLA-4, memory B cells, adhesion, spleen, autoimmunity
Citation: Camponeschi A, Gerasimcik N, Wang Y, Fredriksson T, Chen D, Farroni C, Thorarinsdottir K, Sjökvist Ottsjö L, Aranburu A, Cardell S, Carsetti R, Gjertsson I, Mårtensson I-L and Grimsholm O (2019) Dissecting Integrin Expression and Function on Memory B Cells in Mice and Humans in Autoimmunity. Front. Immunol. 10:534. doi: 10.3389/fimmu.2019.00534
Received: 30 October 2018; Accepted: 27 February 2019;
Published: 21 March 2019.
Edited by:
Ali Ellebedy, Washington University in St. Louis, United StatesReviewed by:
Alfred Hyoungju Kim, Washington University School of Medicine in St. Louis, United StatesScott A. Jenks, Emory University, United States
Copyright © 2019 Camponeschi, Gerasimcik, Wang, Fredriksson, Chen, Farroni, Thorarinsdottir, Sjökvist Ottsjö, Aranburu, Cardell, Carsetti, Gjertsson, Mårtensson and Grimsholm. This is an open-access article distributed under the terms of the Creative Commons Attribution License (CC BY). The use, distribution or reproduction in other forums is permitted, provided the original author(s) and the copyright owner(s) are credited and that the original publication in this journal is cited, in accordance with accepted academic practice. No use, distribution or reproduction is permitted which does not comply with these terms.
*Correspondence: Inga-Lill Mårtensson, bGlsbC5tYXJ0ZW5zc29uQHJoZXVtYS5ndS5zZQ==
†These authors have contributed equally to this work
‡Present Address: Natalija Gerasimcik, Division of Periodontology, Department of Dental Medicine, Karolinska Institute, Huddinge, Sweden