- 1Department of Microbiology and Immunology, University of North Carolina, Chapel Hill, NC, United States
- 2UNC HIV Cure Center, University of North Carolina, Chapel Hill, NC, United States
Antiretroviral therapy (ART) has transformed HIV from a fatal disease to a chronic condition. In recent years there has been considerable interest in strategies to enable HIV-infected individuals to cease ART without viral rebound, either by purging all cells infected harboring replication-competent virus (HIV eradication), or by boosting immune responses to allow durable suppression of virus without rebound (HIV remission). Both of these approaches may need to harness HIV-specific CD8+ T cells to eliminate infected cells and/or prevent viral spread. In untreated infection, both HIV-specific and total CD8+ T cells are dysfunctional. Here, we review our current understanding of both global and HIV-specific CD8+ T cell immunity in HIV-infected individuals with durably suppressed viral load under ART, and its implications for HIV cure, eradication or remission. Overall, the literature indicates significant normalization of global T cell parameters, including CD4/8 ratio, activation status, and telomere length. Global characteristics of CD8+ T cells from HIV+ART+ individuals align more closely with those of HIV-seronegative individuals than of viremic HIV-infected individuals. However, markers of senescence remain elevated, leading to the hypothesis that immune aging is accelerated in HIV-infected individuals on ART. This phenomenon could have implications for attempts to prime de novo, or boost existing HIV-specific CD8+ T cell responses. A major challenge for both HIV cure and remission strategies is to elicit HIV-specific CD8+ T cell responses superior to that elicited by natural infection in terms of response kinetics, magnitude, breadth, viral suppressive capacity, and tissue localization. Addressing these issues will be critical to the success of HIV cure and remission attempts.
CD8+ T Cells in the Post-ART Era
The long-term goal of HIV cure is to enable HIV-infected individuals to cease life-long antiretroviral therapy (ART) through the development of strategies to eradicate cells latently infected with HIV. Studies in which patients with little to no measurable HIV reservoir (due to very early ART treatment) rebounded following ART removal suggest that HIV reactivation may originate from a few or even a single replication competent provirus (1, 2). Total eradication of the HIV reservoir, and therefore true HIV cure, while no doubt the ideal, will therefore be challenging to achieve. Consequently, many groups are pursuing strategies to induce durable ART-free remission without HIV rebound. Both in vivo and in vitro studies support a role for CD8+ T cells in HIV eradication and durable remission approaches (3–6). CD8+ T cells are highly efficient killers of virus-infected cells; however, HIV-specific CD8+ T cells induced by natural infection fail to suppress viral replication after cessation of ART (Figure 1, top), suggesting that a successful HIV cure or durable remission strategy may require the priming of de novo HIV-specific responses and/or qualitative shifts in CD8+ T cell function. To date, CD8+ T cell HIV immunotherapies have been broadly unsuccessful. Failure has been attributed not only to poor population-level immunogenicity but also ongoing immune dysfunction in HIV+ART+ individuals.
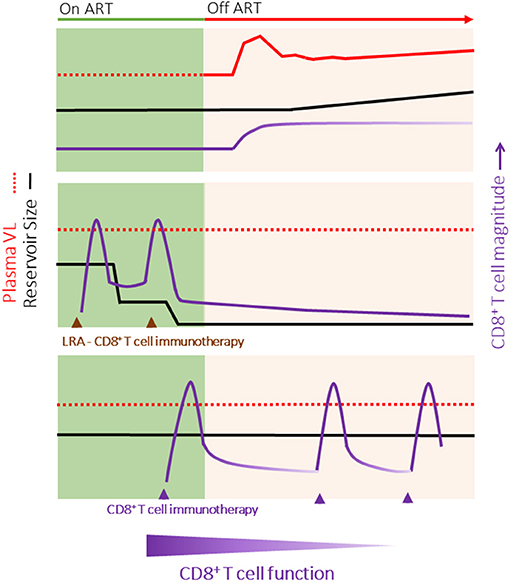
Figure 1. HIV Cure Strategies may require different properties of CD8+ T cells. (Top) Outline of typical HIV rebound (red line) following the cessation of ART. Although the magnitude of the HIV-specific CD8+ T cell response increases, there is a progressive loss of function with time off ART. (Middle) HIV Eradication of the replication competent reservoir (black line) combining latency reversal agents and immunotherapies to boost or redirect CD8+ T cells (purple line) to rapidly eliminate all cells infected with HIV. Following viral clearance, the magnitude of the HIV-specific CD8+ T cell response would decline, but a small population of functional memory cells would persist long-term. (Bottom) Durable ART-free remission in which the CD8+ T cell host immune response limits HIV rebound without decreasing the size of the HIV reservoir. This strategy may require intermittent boosting of the CD8+ T cell response (for example, through immunization) to combat a potential decline in the magnitude and function of HIV-specific CD8+ T cell responses over time. It is likely that different functional properties of CD8+ T cells will be required for HIV eradication (e.g., rapid killing, penetration of tissue reservoirs) vs. HIV remission (e.g., memory maintenance). Note, HIV eradication and remission strategies may be combined.
A new generation of HIV therapeutic vaccines have been developed that exhibit greater immunogenicity and efficacy in pre-clinical testing (7, 8). In addition, therapies such as bispecific biologics work by harnessing all CD8+ T cells, and therefore promise to be scalable to a large, genetically diverse population (9–11). Success with all of these strategies still however relies on the quality and function of CD8+ T cells. Here, we review the global function of CD8+ T cells under ART, comparing CD8+ T cell characteristics between HIV+ART+, HIV seronegative individuals (HIV-), and untreated HIV+ infected individuals grouped into elite controllers (EC), viremic controllers (VC) and typical progressors (TP). We also summarize literature comparing HIV-specific CD8+ T cells in treated and untreated HIV infection.
Overall, CD8+ T cells undergo substantial restoration of function following prolonged ART suppression, including in individuals treated in chronic/advanced infection. The phenotype and functional profile of total CD8+ T cells in HIV+ART+ individuals more closely resembles that of HIV seronegative (HIV−) than of HIV seropositive (HIV+) individuals, including HIV controllers. This supports the continued testing of CD8+ T cell immunotherapies for HIV cure. However, CD8+ T cells, including HIV-specific CD8+ T cells, in HIV+ART+ individuals resemble the phenotypic and functional profile of CD8+ T cells in older HIV− individuals. We postulate that the “immunosenescent” phenotype of CD8+ T cells in HIV+ART+ individuals has differential implications for CD8+ T cell immunotherapies targeted at HIV eradication vs. durable remission strategies.
Total CD8+ T Cells Under ART
Untreated HIV infection causes progressive CD8+ T cell dysfunction, skewing T cell differentiation and limiting CD8+ T cell proliferation, cytokine production and lytic function (12–17). In untreated infection, sustained HIV viremia is a major driver of CD8+ T cell dysregulation. In individuals in whom viremia is lower, broader T cell function is observed (14, 18, 19). EC and VC, who typically control viremia in acute/early HIV infection, consistently exhibit a broader range of CD8+ T cell cytokine production and higher lytic function than typical progressors (TP) (Table 1). Similarly, CD8+ T cells from individuals who initiate ART in early infection mostly exhibit broader function than those treated in chronic infection (16, 64, 65). Early virus control also limits other drivers of CD8+ T cell dysfunction, including CD4+ depletion and lymph node fibrosis (66–68).
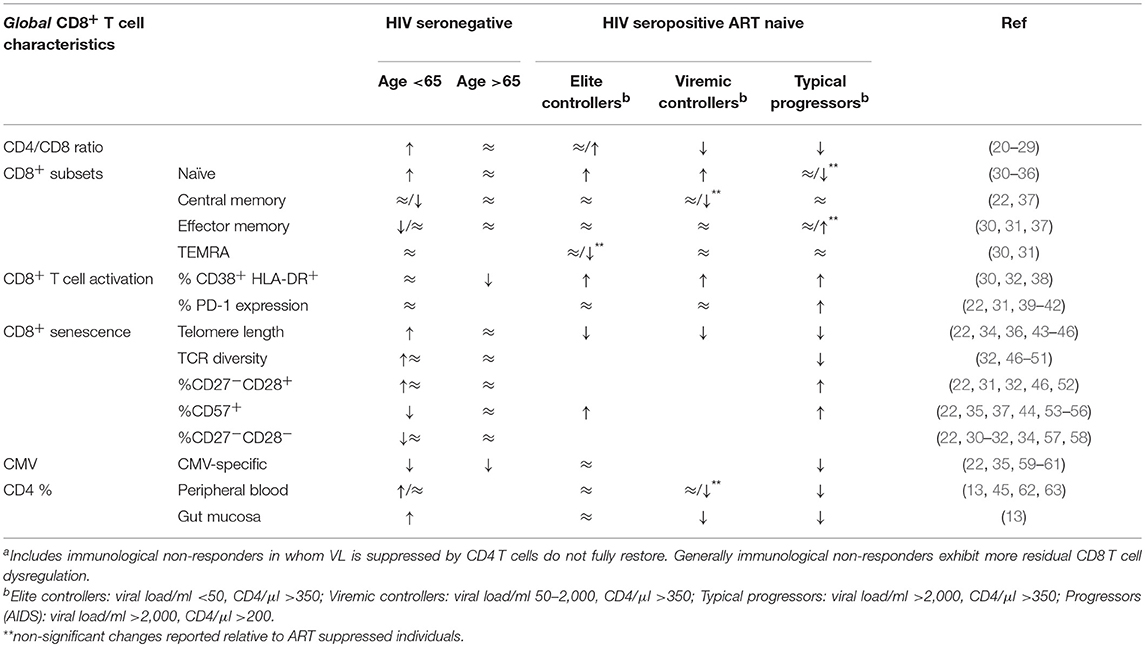
Table 1. Total T cell characteristics in HIV seronegative and HIV seropositive participants relative to HIV seropositive, durably ART suppressed individualsa (↑, higher relative to ART treated; ↓, lower relative to ART treated; ≈, comparable to ART treated).
Under ART, low-level viremia (~1 copy/mL plasma) is detectable in most individuals and likely contributes to the long-term detection of HIV-specific CD8+ T cells, and the CD8+ T cell phenotype and dysfunction observed (69). The other driver/s of residual CD8+ T cell dysregulation are increasingly difficult to assess because of the changing demographics of HIV+ART+ individuals. Age impacts immune function, and because over 50% of HIV+ART+ individuals in the U.S. are over the age of 50, age is a confounder in studies of CD8+ T cell function in HIV+ART+ individuals (70).
The primary effect of aging on the immune system is the process of immunosenescence. Features of immunosenescence in healthy individuals include low CD4/CD8 T cell ratio, decreased telomere lengths which limits mitosis, and an increase in total, senescent, terminally differentiated and activated CD8+ T cells (71–73). This latter phenotype limits CD8+ T cell proliferative and functional capacity. Immunosenescence is also associated with co-morbidities such as elevated risk of cardiovascular disease, cancer, fragility and tissue damage resulting from dysregulated inflammation (74). The incidence of these co-morbidities is also increased in HIV+ART+ individuals (47).
CD4/CD8 Ratio Under ART
Untreated HIV infection is characterized by an inverted, low CD4/CD8 ratio (75), that results from both ongoing CD4+ T cell depletion and the persistent elevation of peripheral CD8+ T cells. While ART consistently improves patient CD4/CD8 ratio, irrespective of pre-ART CD4+ counts (76), CD8+ T cell absolute counts in untreated infection remain relatively stable post-ART (75). The net result is that ART generally fails to fully normalize the CD4/CD8 ratio to levels measured in age-matched HIV seronegative individuals (20–25, 77) (Table 1), and the consistently low CD4/CD8 ratio observed in ART treated individuals is strongly associated with a number of immunological abnormalities (20, 21, 77). Risk of co-morbidities and mortality are further increased in immunological non-responders in whom ART controls virus load but CD4+ T cell recovery is poor (78–80).
HCMV seropositivity, which is higher in HIV-infected individuals (>80%) than the broader population (81), has been consistently implicated as a driver of the elevated global CD8+ T cell counts observed in HIV-infected individuals both off and on ART (82). HCMV seropositivity also increases with age, and HCMV serostatus is independently associated with elevated non-AIDS co-morbidities (81, 83). HCMV induces a potent CD8+ T cell response that increases in magnitude over time, a phenomenon termed “memory inflation” (84), and HCMV-specific CD8+ T cell responses can account for as much as 50% of all antigen-specific CD8+ T cells in elderly individuals [reviewed in (85)]. A recent study showed that HCMV+HIV+ individuals had higher absolute CD8+ T cell counts than individuals who were either HCMV+ or HIV+ alone (86), but it is unclear whether these higher levels resulted only from increased frequencies of HCMV-specific CD8+ T cells (22, 59). While HCMV-specific CD8+ T cell responses do not themselves exhibit functional impairment in HIV+ART+ individuals (22), HCMV infection may indirectly impact CD8+ T cell immunoscenescence through ongoing production of proinflammatory cytokines and by limiting T cell receptor diversity across the broader CD8+ T cell population (22, 59).
CD8+ T Cell Subsets Under ART
CD8+ T cell differentiation and maturation is skewed during HIV infection (Table 1) (30, 31, 87). Naïve (CD45RA+ CD27+) CD8+ T cells are depleted from early infection, more so in TP than EC and VC (30, 87). ART partially restores naïve CD8+ T cells relative to TP, but frequencies are more comparable to those of older HIV- than age-matched healthy individuals. Differences in terminally differentiated (CD45RA+CD27−), central memory (CD45RA−CD27+) and effector memory (CD45RA−CD27−) subsets between ART treated and untreated HIV-infected individuals, although less striking, have also been reported (30, 31, 87). The failure to fully restore the CD8+ naïve compartment combined with elevated total CD8+ T cell frequencies described above suggests that, similar to observations in older people, induction of de novo CD8+ T cell responses in HIV+ART+ individuals may be more limited.
There have been few reports of induction of novel HIV-specific CD8+ T cell responses following therapeutic vaccination of ART+HIV+ individuals (88), though this in part may arise from the limited immunogenicity of first generation T cell vaccines. Whether it will be possible to induce potent de novo HIV-specific T cell responses in HIV+ART+ individuals, equally important for both HIV eradication and remission approaches, is a key question in the current era of CD8+ T cell immunotherapy.
Immune Activation Under ART
Uncontrolled HIV replication is characterized by elevated frequencies of CD4+ and CD8+ T cells expressing activation markers such as CD38 and HLA-DR (89, 90). The importance of immune activation in HIV pathogenesis is underscored by the observation that in untreated infection, T cell activation predicts disease progression independently of viral load (91–93). In individuals with durably suppressed viral load on ART, peripheral T cell activation is substantially reduced relative to untreated individuals, including EC (30, 32, 38). However, in many cases, T cell activation is not fully normalized relative to HIV-uninfected individuals (22, 94, 95). Residual T cell activation appears to be particularly prevalent in immunologic non-responders (96, 97).
As introduced earlier, low-level viremia is observed in most HIV+ART+ individuals (98, 99). These levels are lower than observed in EC, who exhibit higher T cell activation (100). Activation levels are higher again in VC and TP (30, 32, 38). Other drivers of elevated T cell activation may be an ongoing consequence of immune dysregulation prior to the initiation of ART. These include lymphoid fibrosis, the depletion of regulatory T cells (Tregs) with anti-inflammatory activities, and loss of gut barrier integrity leading to translocation of bacterial products such as LPS that could trigger inflammatory responses (69, 101–103). This has sparked interest in whether it might be possible to normalize immune activation through early initiation of ART (often defined as ART within 6 months of infection), perhaps by minimizing the size of the viral reservoir and preserving regulatory T cells, the integrity of lymphoid architecture, and the gut epithelial barrier. Research in this area has produced conflicting results, indicating that the effect of early ART on T cell activation may depend on how early after infection ART is initiated, how long after ART initiation activation is measured, and other, as yet undefined, factors (104–108).
Residual T cell activation may have implications for HIV cure. Activated CD4+ T cells more readily support productive HIV replication, potentially rendering them more vulnerable to infection in any HIV cure attempt involving analytic treatment interruption (ATI) (109). Activation-induced cell death of uninfected (or abortively infected) “bystander” CD4+ T cells could also contribute to CD4+ depletion during ART treatment interruption (110, 111). Conversely, activated CD4+ T cells harboring replication-competent but latent virus may be more amenable to latency reversal. Notably, some putative latency-reversing agents activate T cells in vitro (112–114).
CD8+ T Cell Function in Lymphoid Tissues Under ART
Most research on CD8+ T cell function under ART conducted to date has been performed on peripheral blood mononuclear cells (PBMC). While the blood is the easiest compartment to sample, most HIV replication occurs in other tissues, particularly mucosal and lymphoid tissue (115–117). These tissues harbor the bulk of HIV RNA+ cells in HIV+ART+ individuals (118). Polyfunctional HIV-specific tissue resident memory (TRM) CD8+ T cells are found at higher frequencies in the gastrointestinal tract of EC compared with individuals on ART, suggesting that in EC these cells may play an important role in in vivo viral suppression (119). Current available data indicate that in both HIV+ and HIV− individuals, memory CD8+ T cells in the lymph nodes and rectal mucosa express less perforin and granzyme B and are less efficient killers of target cells than their counterparts in the peripheral blood (120–122). This less cytotoxic phenotype may be related to the function of lymphoid tissue as predominantly a site of lymphocyte priming and maintenance. Furthermore, de novo perforin production is lower in ex-vivo stimulated HIV-specific CD8+ T cells from EC compared with typical progressors, suggesting that perforin production may not be the major control mechanism in the gut, and that cytokine production in lymphoid tissues may be a more useful correlate of virologic suppression (13, 121).
In secondary lymphoid tissues (LT), HIV replication is concentrated within CD4+ T cells in the B cell follicles (123). This may be a consequence of the partial exclusion of HIV-specific CD8+ T cells from follicles (123–126). HIV replication is also associated with LT fibrosis, which strongly correlates with the depletion of naïve CD4+ T cells, and is inversely correlated with the extent of immune reconstitution upon the initiation of ART (66). LT fibrosis, caused through the deposition of collagen by T regulatory cells, disrupts LT architecture, resulting in T cells with less access to antigen and IL-7, which is critical for T cell maturation and maintenance (66, 127). LT fibrosis is elevated in all HIV+ groups (HIV+ EC, VC, TP, and HIV+ART+, both immunologic responders and non- responders), compared to HIV− individuals (128). To date, the data suggest that LT fibrosis does not reverse with ART; however, early initiation of ART may limit the viral replication-dependent inflammation that drives fibrosis, improving immune reconstitution (66, 128). These additional barriers, need to be considered for HIV cure or remission strategies. Strategies to redirect CD8+ T cells to immune-privileged sites, such as the follicles, and/or strategies to limit LT fibrosis or reverse collagen deposition, such as IL-7 therapy are being investigated (129, 130).
CD8+ T Cell Senescence Under ART
Telomere integrity is critical for mitotic division and cell survival. The shortening of telomeres is a hallmark of decreased cell proliferation and can activate pathways resulting in apoptosis or cellular senescence (22, 131–133). Compared to HIV− individuals, the telomeres of CD8+ T cells in both HIV-infected ART naïve or treated individuals are significantly shorter, indicating a history of increased cell divisions (22, 43, 134). Though multiple studies suggest that T cell telomere length is partially restored (relative to untreated infection) following ART, ongoing proliferation defects in ART treated individuals have been confirmed (Table 1) (112, 135–137). Similar to alterations in T cell differentiation state, telomere length in T cells is more consistent with that of older seronegative individuals (47).
CD8+ T cells with shortened telomeres exhibit a combination of CD28−, CD57+, or CD27− phenotypes (138–141). An increased frequency of CD28−CD57+ CD8+ T cells is observed in HIV infection, where elevated CD57+ expression has been associated with reduced proliferative capacity of CD8+ T cells (22, 53, 142). Initiation of ART in early infection is able to largely normalize the proportion of CD8+ CD28−, CD57+, and CD27− T cells, though not to the levels seen in age-matched HIV-seronegative individuals (142). Expression of senescence markers in HIV+ ART+ individuals again resembles that of older seronegative individuals.
A highly diverse T cell repertoire is generally associated with an effective immune system and efficient control of chronic viral infections. HIV infection is associated with qualitative TCR repertoire changes, including disruption of the TCR variable region, Vβ, with CD8+ T cells affected to a greater extent than CD4+ T cells (143, 144). Compared to HIV− individuals, HIV+ treatment-naïve individuals exhibit a significant decrease in whole-blood TCR diversity (145). However, the TCR repertoire diversity of naïve and memory/effector sub-populations is comparable between HIV+ and HIV− individuals. This suggests that the decrease in TCR repertoire diversity in the blood results from the expansion of more differentiated T cell populations with lower TCR diversity (145). Most (48, 49, 146), but not all (147), studies have observed that ART is unable to fully reconstitute the TCR repertoire in CD8+ T cells to the diversity seen in seronegative individuals. Whether the diminished TCR diversity in HIV+ART+ individuals will impact curative strategies is unclear as co-factors separate from TCR diversity also contribute to CD8+ T cell function (148).
Summary
Collectively, the current available literature suggests that durable viral suppression under ART is associated with partial normalization of the frequency, activation, differentiation, senescence, and diversity of global CD8+ T cells. The overall, global CD8+ T cell profile in HIV+ART+ individuals is more similar to HIV seronegative than HIV seropositive individuals, but when HIV seronegatives are stratified by age, data consistently indicate that CD8+ T cells in HIV+ART+ individuals show an aging phenotype.
Since existing HIV-specific CD8+ T cells have, by definition, failed to control viral replication in individuals on ART, a successful HIV cure or remission strategy may require the priming of de novo HIV-specific responses by vaccination. Reduced TCR diversity, lower frequencies of circulating naïve CD8+ T cells, and accumulation of terminally differentiated and senescent cells could hinder this approach by reducing the pool of CD8+ T cells available for priming. Further studies are required to definitively elucidate whether early ART or other interventions could preserve CD8+ T cell diversity, stemness, and self-renewal capacity.
HIV-Specific CD8+ T Cells Under ART
HIV infection induces a robust HIV-specific CD8+ T cell response. In untreated infection, the HIV-specific CD8+ T cell response is highly dynamic. CD8+ T cells exert selection pressure on HIV, resulting in the emergence of non-synonymous mutations in and around T cell epitopes (149–151). These mutations can result in HIV-infected cells either failing to present the viral epitope or, if presented evading recognition by CD8+ T cells (17, 151–154). This process, termed “escape” is first observed within weeks of transmission and continues through infection (149–151). Virus escape results in an ongoing shift in the HIV-specific CD8+ T cell response pattern, with new CD8+ T cell responses constantly emerging (154). However, HIV infection is also characterized by a progressive loss of HIV-specific CD8+ T cell immune function, specifically the loss of the capacity to simultaneously produce antiviral cytokines and release lytic molecules following antigenic stimulation (14, 16, 16). HIV-specific CD8+ T cells also exhibit shorter telomeres and poorer proliferative capacity (32, 137). This increasing loss of function in CD8+ T cells is associated with upregulation of immune checkpoint markers such as PD-1, CD160, Tim-3, and TIGIT, typically referred to as an “exhausted” phenotype (39). By contrast, HIV-specific CD8+ T cells in EC that largely control HIV viremia early in acute-early infection exhibit broader CD8+ T cell function, robust proliferation and lower immune activation (16). Here we consider the magnitude, breadth and functional phenotype of CD8+ T cells in ART treated individuals compared to TP, EC and VC.
HIV-Specific CD8+ T Cell Magnitude and Breadth
T cell magnitude can be measured by either the absolute frequency (multimer staining to detect all HIV-specific cells, whether functional or not) or by assessing the frequency of functional cells (ELISpot, intracellular cytokine staining, CSFE proliferation etc). Magnitude is an important measure of T cell potency. In HIV infection, the functional magnitude of T cells, measured by IFN-γ ELISpot, is correlated to the time to viral escape, although associations between the overall frequency of HIV-specific CD8+ T cells using functional assays and viral load have not been consistently observed (65, 155, 156). Viremia is a primary driver of the magnitude of the HIV-specific T cell response, an observation underscored by the differences in HIV-specific T cell magnitude between groups of HIV infected individuals, including ART treated individuals (155, 157, 158). In a study examining the total summed magnitude of CD8+ T cell responses against the entire HIV proteome from PBMCs, as measured by ex vivo IFNγ ELISpot, the summed magnitude of CD8+ T cells in untreated chronic infection is at least 2-fold greater than the summed magnitude of CD8+ T cells from treated infection (Table 2) (155). EC, who control HIV better than TP and have low viral load, exhibit significantly lower T cell responses than TP. In turn, EC, who exhibit higher viremia than ART+HIV+, have higher magnitude T cell responses (155, 157, 158). Even so, the HIV-specific T cell response in ART treated individuals remains detectable over time, with responses detectable ex vivo after years of durable suppression (155).
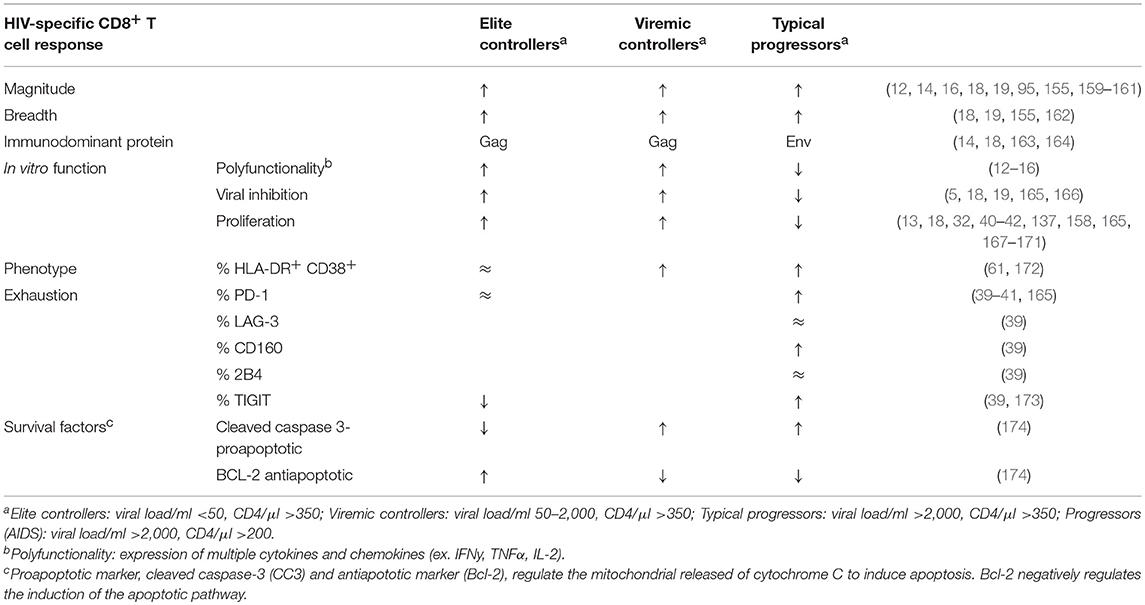
Table 2. HIV-specific CD8+ T cell responses in HIV seropositive, treatment naïve individuals relative to HIV seropositive durably ART suppressed individuals. (↑, higher relative to ART treated; ↓, lower relative to ART treated; ≈, comparable to ART treated).
The magnitude of the total HIV-specific CD8+ T cell responses positively correlates with the number of reactive epitopes targeted, that is the breadth of response (155). Similar to magnitude, the breadth of response is decreased during ART treatment, and timing of ART initiation affects the breadth of CD8+ T cell response (155). Treatment during acute infection results in breadth of response being significantly lower and more narrowly directed than individuals with untreated or intermittently treated HIV infection (Table 2) (155, 175).
HIV-Specific CD8+ T Cell Targeting of HIV
The specificity of the CD8+ T cell response may also be critical in HIV control. Associations between the targeting of HIV-specific CD8+ T cell responses and virus set point have been observed. In cohort studies of individuals in South Africa with chronic clade C HIV untreated infection, viral load was inversely correlated with the breadth of Gag-specific T cell responses, and was directly correlated with the breadth of Env-specific responses (163). Similar observations were observed in chronic, HIV-clade B untreated infection, where individuals with lower viral loads more extensively target HIV-Gag (176, 177). Work by Mothe and colleagues extended these previous findings (178). T cell responses against the full HIV proteome were mapped in 950 participants and a set of T cell epitopes were identified as “protective,” based on cohort-level associations with lower viral load (178). The protective epitopes occurred in regions of Gag, Pol, and Vif, while non-protective epitopes that were associated with higher virus loads occurred in regions of Env, Nef, Vpr, and Pol (178).
HIV sequence variability at the population-level can be quantified using a measurement called entropy (179). High entropy epitopes are more variable at the population level, whereas low entropy epitopes are more conserved. The “protective” epitopes described by Mothe et al. typically exhibited lower entropy relative to non-protective epitopes (178). Lower entropy regions are less likely to accommodate escape mutations without inducing a fitness cost (17, 154, 180). For example, Gag is subject to more stringent sequence constraints than Env, making it less likely for HIV to accommodate a mutation in this region. More generally, eliciting CD8+ T cell responses against the most highly-conserved regions of HIV may be a good strategy for immunotherapies, as escape mutations in these regions can result in fitness cost for HIV.
Virus escape mutations were identified in HIV provirus over 25 years ago (181). More recently, virus escape was also confirmed in the replication-competent reservoir in durably ART suppressed individuals, highlighting the challenge of pre-existing virus escape to T cell immunotherapy strategies, whether for HIV eradication or durable remission (182). Further studies are needed to quantify the level and extent of pre-existing virus escape in the HIV reservoir.
HIV-Specific CD8+ T Cell Function Under ART
In chronic untreated infection, HIV-specific CD8+ T cells progressively lose the capacity to proliferate and secrete cytokines and cytotoxic effectors, though even in late-stage infection some function is retained (183–185) (Table 2). ART significantly normalizes HIV CD8+ T cell functions (186). However, multiple studies have reported that HIV-specific CD8+ T cells from individuals durably suppressed on ART do not exhibit the same breadth of function as HIV-specific CD8+ T cells from EC (40, 165). Relative to EC, CD8+ T cells from HIV+ART+ individuals display reduced proliferative capacity and cytokine and lytic molecule production following stimulation with HIV antigens (13, 137, 158, 165, 167, 170). Even more pertinently, HIV-specific CD8+ T cells from ART-suppressed individuals have reduced capacity to eliminate both productively infected and latently infected CD4+ T cells compared with CD8+ T cells from EC (5, 165, 166). Although these observations were likely to be influenced by increased frequencies of HIV-specific CD8+ T cells in EC or stronger targeting of protective, low entropy T cell epitopes (13, 165), together they indicate that HIV-specific CD8 T cells in HIV+ART+ individuals while broadly functional, are not optimal.
HIV-Specific CD8+ T Cell Exhaustion
In untreated HIV infection, progressive functional impairment of CD8+ T cells is accompanied by the upregulation of “immune checkpoint markers” such as PD-1, CD160, 2B4, LAG-3, and TIGIT, (with negligible expression of TIM3 and CTLA-4), which can inhibit signaling downstream of the TCR on HIV-specific CD8+ T cells, and in chronic viral infections, promote apoptosis (39, 171, 187, 188). Checkpoint marker upregulation in dysfunctional CD8+ T cells is a signature of T cell exhaustion. As exhaustion is partly driven by chronic antigen exposure, it would be expected that viral suppression under ART would be associated with at least partial recovery of HIV-specific CD8+ T cell function, and this does appear to be the case for multiple markers when HIV+ART+ are compared to TP (Table 2)(41, 186, 189). Literature are limited on whether checkpoint inhibitor levels remain elevated relative to the HIV seronegative population; however, Tauriainen et al. report that HIV-specific CD8+ TIGIThi cells were associated with lower function in durably treated participants and co-expressed other exhaustion markers, suggesting some ongoing T cell exhaustion in durably suppressed individuals (173).
Antibody blocking of checkpoint inhibitors, with most focus on the PD-1/PDL-1 pathway, can increase CD8+ T cell function and, remarkably, in some cancer patients has afforded complete clinical response (190). Clinical data are more limited in HIV-infected individuals. A small clinical study found evidence that low level anti-PD-1 treatment increase CD8+ T cell functionality in a subset of durably ART-suppressed HIV seropositive participants, and more recently CTLA-4 blockade was reported to be well tolerated in HIV-infected individuals (191, 192). In SIV-infected macaques, α-PD-1 antibody given 10 days prior to ART initiation increased antiviral CD8+ T cell function and produced more rapid suppression and CD4+ T cell reconstitution following ART initiation (193). We anticipate that results from several ongoing clinical and non-human primate studies will be reported over coming years.
Summary
The initiation of ART, particularly during acute infection, is associated with a decrease in HIV-specific CD8+ T cell response magnitude and breadth. A HIV cure or remission strategy may require redirecting CD8+ T cells to the more highly conserved regions of HIV, as escape mutations in these regions can result in a fitness cost for the virus. When designing immunotherapies intended to elicit CD8+ T cells capable of clearing reactivated latent cells, increasing the frequency and redirecting CD8+ T cells may not be enough, and specifically, the implications of sub-optimal HIV-specific CD8+ T cell function should be considered.
Immunotherapy in Cure
CD8+ T Cell Vaccines and Therapies for HIV Cure
In most people, the CD8+ T cell response to HIV is inadequate to prevent virus rebound. As detailed above inadequate CD8+ T cell magnitude and breadth, pre-existing escape in the HIV reservoir, insufficient restoration of CD8+ T cell function following ART and ongoing exclusion of CD8+ T cells from virus reservoirs such as B cell follicles likely all contribute to the failure of CD8+ T cell immunity to control HIV rebound. However, a recent study observed that dual bNAb treatment in the first weeks of macaque simian/human immunodeficiency virus (SHIV) challenge in rhesus macaques resulted in lower persistent viremia (194). SHIV rebound was observed following CD8-antibody depletion in controller animals. In most animals virus control was regained following restoration of CD8+ T cells (194). While this study design cannot be easily translated into clinical practice, the observations provide proof-of-principle that CD8+ T cell immunity can be augmented, resulting in improved long-term virus control.
CD8+ T cell vaccine therapies against HIV aim to stimulate pre-existing and/or generate de novo HIV-specific CD8+ T cell responses to suppress viral replication through the clearance of HIV-infected cells. To date, therapeutic vaccine regimens, which range from recombinant DNA and viral vectors to dendritic cell vaccines (195, 196), have only shown a modest effect, and a minimal delay in virus rebound following ATI, which may be due to limited immunogenicity of the vaccine [reviewed in (197)]. The limited efficacy of current therapeutic vaccines may also be due to HIV escape or lack of restoration of CD8+ T cell function. However, the newest generation of vaccines involve heterologous vector prime-boost regimens, which have shown enhanced immunity compared to homologous regimens, as well as conserved immunogenic designs which may help to overcome population level immunogenicity and virus escape (7, 198). Adoptive transfer of in vitro expanded autologous and allogeneic HIV-specific CD8+ T cells is also being pursued (199, 200). This approach has been successful against some cancers (6, 201, 202) and to date, HIV-CD8+ T cell therapy has been safe in HIV+ART+ individuals (203).
Other approaches under active investigation seek to harness bulk (rather than antigen-specific) CD8+ T cells for HIV clearance. These approaches include the use of chimeric antigen receptors, Dual-Affinity Re-Targeting protein (DARTS) and T cell receptor (TCR)-targeting system with an anti-CD3 effector function (ImmTAVs) in which CD8+ T cells are transduced with either a HIV-specific T cell receptor, a HIV-specific binding antibody, HIV-specific antibodies, or T cell receptors ligated to a CD3 effector molecule (9, 10, 204). Several molecules are in Phase I testing or progressing to Phase I testing.
These approaches could all be combined with approaches to block checkpoint inhibitors and enhance CD8+ T cell function of CD8+ T cell responses or combined with approaches targeting other arms of the immune response such as bNAb therapy. In combination with antiretroviral drugs, CD8+ T cell therapies, bNAbs, blockade of regulatory pathways and the harnessing of other immune cells may offer new therapeutic approaches in a near future.
Integration of CD8+ Therapies to HIV Cure
HIV eradication requires reactivation and clearance of latently infected cells that evade ART because they are long-lived and quiescent (205) and/or undergo homeostatic proliferation without reactivation of the integrated provirus (206). Multiple groups are focusing on developing small molecule or immune-based approaches to reactivate HIV latently infected cells. To date, latency reversing agents have successfully increased cell associated HIV RNA in resting CD4+ T cells and induced viral blipping in vivo (207–209). However, these studies, supported by in vitro work (5), suggest that reactivation is transient, with cells rapidly returning to a state of quiescence, and no change in the size of the HIV reservoir was observed. This suggests that CD8+ T cells and or other immune effectors have a limited window to clear reactivated cells. The implication for HIV eradication strategies is that latency reversal and immunotherapy should occur in concert; that is CD8+ T cell immunotherapy should produce the “best” CD8+ T cell response around the time of maximal latency reversal. For eradication strategies, CD8+ T cell clearance in short well-timed bursts may be sufficient (Figure 1, middle).
The desired outcome of HIV cure attempts contrasts with HIV remission strategies that do not seek to eradicate the reservoir but rather control and limit HIV rebound in the long term. Here, T cell based immunotherapy would need to afford sustained CD8+ T cell surveillance of stochastic HIV reactivation, perhaps over years (Figure 1, bottom). HIV eradication vs. HIV remission strategies therefore may require qualitatively and quantitatively different CD8+ T cell responses.
In conclusion, ART at least partially restores lytic function and virus inhibitory capacity of CD8+ T cells. This suggests that therapeutic vaccination can drive expansion of HIV-specific CD8+ T cell responses, at least in the short-term (210, 211). These intact functions may be sufficient for HIV eradication approaches that are shorter term and rely more on rapid expansion and lytic function of CD8+ T cells. However, accelerated aging phenotype of CD8+ T cells could be a greater limitation to durable remission approaches. In this case, ongoing proliferation defects, cellular activation and exhaustion may over time, limit long-term efficacy of immunotherapies. For optimal and sustained efficacy, T cell boosting regimens may need to be incorporated in HIV remission strategies as well as adjunct therapies aimed at reversing or limiting CD8+ T cell immunosenescence in the ART suppressed population.
Author Contributions
JAW, GC, and NG all participated in the conception, drafting, and composition of this manuscript.
Conflict of Interest Statement
The authors declare that the research was conducted in the absence of any commercial or financial relationships that could be construed as a potential conflict of interest.
Acknowledgments
NG is an investigator in the UNC HIV Cure Center. This work was supported by the National Institute of Health (NIH) awards: U01 AI131310 and 1R24AG044325.
References
1. Luzuriaga K, Gay H, Ziemniak C, Sanborn KB, Somasundaran M, Rainwater-Lovett K, et al. Viremic relapse after HIV-1 remission in a perinatally infected child. N Engl J Med. (2015) 372:786–8. doi: 10.1056/NEJMc1413931
2. Henrich TJ, Hatano H, Bacon O, Hogan LE, Rutishauser R, Hill A, et al. HIV-1 persistence following extremely early initiation of antiretroviral therapy (ART) during acute HIV-1 infection: An observational study. PLoS Med. (2017) 14:e1002417. doi: 10.1371/journal.pmed.1002417
3. Pandrea I, Gaufin T, Gautam R, Kristoff J, Mandell D, Montefiori D, et al. Functional cure of SIVagm infection in rhesus macaques results in complete recovery of CD4+ T cells and is reverted by CD8+ cell depletion. PLoS Pathog. (2011) 7:e1002170. doi: 10.1371/journal.ppat.1002170
4. Smith NM, Mlcochova P, Watters SA, Aasa-Chapman MM, Rabin N, Moore S, et al. Proof-of-principle for immune control of global HIV-1 reactivation in vivo. Clin Infect Dis. (2015) 61:120–8. doi: 10.1093/cid/civ219
5. Shan L, Deng K, Shroff NS, Durand CM, Rabi SA, Yang HC, et al. Stimulation of HIV-1-specific cytolytic T lymphocytes facilitates elimination of latent viral reservoir after virus reactivation. Immunity (2012) 36:491–501. doi: 10.1016/j.immuni.2012.01.014
6. Sung JA, Lam S, Garrido C, Archin N, Rooney CM, Bollard CM, et al. Expanded cytotoxic T-cell lymphocytes target the latent HIV reservoir. J Infect Dis. (2015) 212:258–63. doi: 10.1093/infdis/jiv022
7. Ondondo B, Murakoshi H, Clutton G, Abdul-Jawad S, Wee EG, Gatanaga H, et al. Novel conserved-region T-cell mosaic vaccine with high global HIV-1 coverage is recognized by protective responses in untreated infection. Mol Ther. (2016) 24:832–42. doi: 10.1038/mt.2016.3
8. Borducchi EN, Cabral C, Stephenson KE, Liu J, Abbink P, Ng'ang'a D, et al. Ad26/MVA therapeutic vaccination with TLR7 stimulation in SIV-infected rhesus monkeys. Nature (2016) 540:284–7. doi: 10.1038/nature20583
9. Sung JA, Pickeral J, Liu L, Stanfield-Oakley SA, Lam CY, Garrido C, et al. Dual-affinity re-targeting proteins direct T cell-mediated cytolysis of latently HIV-infected cells. J Clin Invest. (2015) 125:4077–90. doi: 10.1172/JCI82314
10. Yang H, Buisson S, Bossi G, Wallace Z, Hancock G, So C, et al. Elimination of latently HIV-infected cells from antiretroviral therapy-suppressed subjects by engineered immune-mobilizing T-cell receptors. Mol Ther. (2016) 24:1913–25. doi: 10.1038/mt.2016.114
11. Riley JL, Montaner LJ. Cell-mediated immunity to target the persistent human immunodeficiency virus reservoir. J Infect Dis. (2017) 215:S160–71. doi: 10.1093/infdis/jix002
12. Jensen SS, Fomsgaard A, Larsen TK, Tingstedt JL, Gerstoft J, Kronborg G, et al. Initiation of Antiretroviral Therapy (ART) at different stages of HIV-1 disease is not associated with the proportion of exhausted CD8+ T Cells. PLoS ONE (2015) 10:e0139573. doi: 10.1371/journal.pone.0139573
13. Ferre AL, Hunt PW, Critchfield JW, Young DH, Morris MM, Garcia JC, et al. Mucosal immune responses to HIV-1 in elite controllers: a potential correlate of immune control. Blood (2009) 113:3978–89. doi: 10.1182/blood-2008-10-182709
14. Hersperger AR, Pereyra F, Nason M, Demers K, Sheth P, Shin LY, et al. Perforin expression directly ex vivo by HIV-specific CD8+ T-cells is a correlate of HIV elite control. PLoS Pathog. (2010) 6:e1000917. doi: 10.1371/journal.ppat.1000917
15. Buggert M, Frederiksen J, Noyan K, Svard J, Barqasho B, Sonnerborg A, et al. Multiparametric bioinformatics distinguish the CD4/CD8 ratio as a suitable laboratory predictor of combined T cell pathogenesis in HIV infection. J Immunol. (2014) 192:2099–108. doi: 10.4049/jimmunol.1302596
16. Betts MR, Nason MC, West SM, De Rosa SC, Migueles SA, Abraham J, et al. HIV nonprogressors preferentially maintain highly functional HIV-specific CD8+ T cells. Blood (2006) 107:4781–9. doi: 10.1182/blood-2005-12-4818
17. Ferrari G, Korber B, Goonetilleke N, Liu MK, Turnbull EL, Salazar-Gonzalez JF, et al. Relationship between functional profile of HIV-1 specific CD8 T cells and epitope variability with the selection of escape mutants in acute HIV-1 infection. PLoS Pathog. (2011) 7:e1001273. doi: 10.1371/journal.ppat.1001273
18. Koofhethile CK, Ndhlovu ZM, Thobakgale-Tshabalala C, Prado JG, Ismail N, Mncube Z, et al. CD8+ T cell breadth and ex vivo virus inhibition capacity distinguish between viremic controllers with and without protective HLA class I alleles. J Virol. (2016) 90:6818–31. doi: 10.1128/JVI.00276-16
19. Sá Ez-Cirió, Lacabaratz C, Lambotte O, Versmisse P, Urrutia A, Boufassa F, et al. HIV controllers exhibit potent CD8 T cell capacity to suppress HIV infection ex vivo and peculiar cytotoxic T lymphocyte activation phenotype. Proc Natl Acad Sci USA. (2007) 104:6776–81. doi: 10.1073/pnas.0611244104
20. Rajasuriar R, Wright E, Lewin SR. Impact of antiretroviral therapy (ART) timing on chronic immune activation/inflammation and end-organ damage. Curr Opin HIV AIDS (2015) 10:35–42. doi: 10.1097/COH.0000000000000118
21. Serrano-Villar S, Sainz T, Lee SA, Hunt PW, Sinclair E, Shacklett BL, et al. HIV-infected individuals with low CD4/CD8 ratio despite effective antiretroviral therapy exhibit altered T cell subsets, heightened CD8+ T cell activation, and increased risk of non-AIDS morbidity and mortality. PLoS Pathog. (2014) 10:e1004078. doi: 10.1371/journal.ppat.1004078
22. Cobos Jiménez V, Wit FW, Joerink M, Maurer I, Harskamp AM, Schouten J, et al. T-cell activation independently associates with immune senescence in HIV-infected recipients of long-term antiretroviral treatment. J Infect Dis. (2016) 214:216–25. doi: 10.1093/infdis/jiw146
23. Hocqueloux L, Avettand-Fènoël V, Jacquot S, Prazuck T, Legac E, Mélard A, et al. Long-term antiretroviral therapy initiated during primary HIV-1 infection is key to achieving both low HIV reservoirs and normal T cell counts. J Antimicrob Chemother. (2013) 68:1169–78. doi: 10.1093/jac/dks533
24. Groves KC, Bibby DF, Clark DA, Isaksen A, Deayton JR, Anderson J, et al. Disease progression in HIV-1-infected viremic controllers. J Acquir Immune Defic Syndr. (2012) 61:407–16. doi: 10.1097/QAI.0b013e318269c414
25. Pastor L, Urrea V, Carrillo J, Parker E, Fuente-Soro L, Jairoce C, et al. Dynamics of CD4 and CD8 T-cell subsets and inflammatory biomarkers during early and chronic HIV infection in Mozambican adults. Front Immunol. (2018) 8:1925. doi: 10.3389/fimmu.2017.01925
26. Blankson JN, Siliciano RF Elite suppression of HIV-1 replication. Immunity (2008) 29:845–7. doi: 10.1016/j.immuni.2008.12.002
27. Mutoh Y, Nishijima T, Inaba Y, Tanaka N, Kikuchi Y, Gatanaga H, et al. Incomplete recovery of CD4 cell count, CD4 percentage, and CD4/CD8 ratio in patients with human immunodeficiency virus infection and suppressed viremia during long-term antiretroviral therapy. Clin Infect Dis. (2018) 67:927–33. doi: 10.1093/cid/ciy176
28. Helleberg M, Kronborg G, Ullum H, Ryder LP, Obel N, Gerstoft J. Course and clinical significance of CD8 + T-cell counts in a large cohort of HIV-infected individuals. J Infect Dis. (2015) 211:1726–34. doi: 10.1093/infdis/jiu669
29. Bansal A, Sterrett S, Erdmann N, Westfall AO, Dionne-Odom J, Overton ET, et al. Normal T-cell activation in elite controllers with preserved CD4+ T-cell counts. AIDS (2015) 29:2245–54. doi: 10.1097/QAD.0000000000000860
30. López M, Soriano V, Peris-Pertusa A, Rallón N, Restrepo C, Benito JM. Elite controllers display higher activation on central memory CD8 T cells than HIV patients successfully on HAART. AIDS Res Hum Retroviruses (2011) 27:157–65. doi: 10.1089/aid.2010.0107
31. Tavenier J, Langkilde A, Haupt TH, Henriksen JH, Jensen FK, Petersen J, et al. Immunosenescence of the CD8+ T cell compartment is associated with HIV-infection, but only weakly reflects age-related processes of adipose tissue, metabolism, and muscle in antiretroviral therapy-treated HIV-infected patients and controls. BMC Immunol. (2015) 16:72. doi: 10.1186/s12865-015-0136-6
32. Desai S, Landay A. Early immune senescence in HIV disease. Curr HIV AIDS Rep. (2010) 7:4–10. doi: 10.1007/s11904-009-0038-4
33. Nixon DE, Landay AL. Biomarkers of immune dysfunction in HIV. Curr Opin HIV AIDS (2010) 5:498–503. doi: 10.1097/COH.0b013e32833ed6f4
34. Rickabaugh TM, Jamieson BD. A challenge for the future: aging and HIV infection. Immunol Res. (2010) 48:59–71. doi: 10.1007/s12026-010-8167-9
35. Appay V, Ne Fastenackels S, Katlama C, Ait-Mohand H, Schneider L, Guihot A, et al. Old age and anti-cytomegalovirus immunity are associated with altered T-cell reconstitution in HIV-1-infected patients. AIDS (2011) 25:1813–22. doi: 10.1097/QAD.0b013e32834640e6
36. Gaardbo JC, Hartling HJ, Ronit A, Thorsteinsson K, Madsen HO, Springborg K, et al. Different immunological phenotypes associated with preserved CD4+ T cell counts in HIV-infected controllers and viremic long term non-progressors. PLoS ONE (2013) 8:e63744. doi: 10.1371/journal.pone.0063744
37. Ferrando-Martinez S, Ruiz-Mateos E, Concepcion Romero-Sanchez M, Angeles Munoz-Fernandez M, Viciana P, Genebat M, et al. HIV infection-related premature immunosenescence: high rates of immune exhaustion after short time of infection. Curr HIV Res. (2011) 9:289–94. doi: 10.2174/157016211797636008
38. Hua S, Lécuroux C, Sáez-Cirión A, Pancino G, Girault I, Versmisse P, et al. Potential role for HIV-specific CD38–/HLA-DR+ CD8+ T cells in viral suppression and cytotoxicity in HIV controllers. PLoS ONE (2014) 9:e101920. doi: 10.1371/journal.pone.0101920
39. Yamamoto T, Price DA, Casazza JP, Ferrari G, Nason M, Chattopadhyay PK, et al. Surface expression patterns of negative regulatory molecules identify determinants of virus-specific CD8+ T-cell exhaustion in HIV infection. Blood (2011) 117:4805–15. doi: 10.1182/blood-2010-11-317297
40. Peretz Y, He Z, Shi Y, Yassine-Diab B, Goulet JP, Bordi R, et al. CD160 and PD-1 co-expression on HIV-specific CD8 T cells defines a subset with advanced dysfunction. PLoS Pathog. (2012) 8:e1002840. doi: 10.1371/journal.ppat.1002840
41. Trautmann L, Janbazian L, Chomont N, Said EA, Gimmig S, Bessette B, et al. Upregulation of PD-1 expression on HIV-specific CD8+ T cells leads to reversible immune dysfunction. Nat Med. (2006) 12:1198–202. doi: 10.1038/nm1482
42. Cockerham LR, Jain V, Sinclair E, Glidden DV, Hartogenesis W, Hatano H, et al. Programmed death-1 expression on CD4+ and CD8+ T cells in treated and untreated HIV disease. AIDS (2014) 28:1749–58. doi: 10.1097/QAD.0000000000000314
43. Lichterfeld M, Mou D, Cung TDH, Williams KL, Waring MT, Huang J, et al. Telomerase activity ofHIV-1–specific CD8≦ T cells: constitutive up-regulation in controllers and selective increase by blockade ofPD ligand 1 in progressors. Blood (2008) 112:3679–87. doi: 10.1182/blood-2008-01-135442
44. Appay V, Almeida J, Sauce D, Autran B, Papagno L. Accelerated immune senescence and HIV-1 infection. Exp Gerontol. (2007) 42:432–7. doi: 10.1016/j.exger.2006.12.003
45. Deeks SG. HIV Infection, inflammation, immunosenescence, and aging. Ann Rev Med. (2011) 62:141–55. doi: 10.1146/annurev-med-042909-093756
46. van Baarle D, Tsegaye A, Miedema F, Akbar A. Significance of senescence for virus-specific memory T cell responses: rapid ageing during chronic stimulation of the immune system. Immunol Lett. (2005) 97:19–29. doi: 10.1016/j.imlet.2004.10.003
47. Deeks SG, Phillips AN. HIV infection, antiretroviral treatment, ageing, and non-AIDS related morbidity. BMJ (2009) 338:a3172. doi: 10.1136/bmj.a3172
48. Gorochov G, Neumann AU, Kereveur A, Parizot C, Li T, Katlama C, et al. Perturbation of CD4+ and CD8+ T-cell repertoires during progression to AIDS and regulation of the CD4+ repertoire during antiviral therapy. Nat Med. (1998) 4:215–21. doi: 10.1038/nm0298-215
49. Giovannetti A, Pierdominici M, Mazzetta F, Salemi S, Marziali M, Kuonen D, et al. T cell responses to highly active antiretroviral therapy defined by chemokine receptors expression, cytokine production, T cell receptor repertoire and anti-HIV T-lymphocyte activity. Clin Exp Immunol. (2001) 124:21–31. doi: 10.1046/j.1365-2249.2001.01502.x
50. Heather JM, Best K, Oakes T, Gray ER, Roe JK, Thomas N, et al. Dynamic perturbations of the T-cell receptor repertoire in chronic HIV infection and following antiretroviral therapy. Front Immunol. (2015) 6:644. doi: 10.3389/fimmu.2015.00644
51. Rebai N, Pantaleo G, Demarest JF, Ciurli C, Soudeyns H, Adelsberger JW, et al. Analysis of the T-cell receptor beta-chain variable-region (V beta) repertoire in monozygotic twins discordant for human immunodeficiency virus: evidence for perturbations of specific V beta segments in CD4+ T cells of the virus-positive twins. Proc Natl Acad Sci USA. (1994) 91:1529–33. doi: 10.1073/pnas.91.4.1529
52. Robert Kalayjian C, Landay A, Richard Pollard B, Dennis Taub D, Barry Gross H, Isaac Francis R, et al. Age-related immune dysfunction in health and in Human Immunodeficiency Virus (HIV) disease: association of age and HIV infection with naive CD8 + cell depletion, reduced expression of CD28 on CD8 + cells, and reduced thymic volumes. J Infect Dis. (2003) 187:1924–33. doi: 10.1086/375372
53. Lee SA, Sinclair E, Hatano H, Hsue PY, Epling L, Hecht FM, et al. Impact of HIV on CD8+ T cell CD57 expression is distinct from that of CMV and aging. PLoS ONE (2014) 9:e89444. doi: 10.1371/journal.pone.0089444
54. Brenchley JM, Karandikar NJ, Betts MR, Ambrozak DR, Hill BJ, Crotty LE, et al. Expression ofCD57 defines replicative senescence and antigen-induced apoptotic death ofCD8+ T cells. Blood (2003) 101:2711–20. doi: 10.1182/blood-2002-07-2103
55. Almeida JR, Price DA, Papagno L, Arkoub ZA, Sauce D, Bornstein E, et al. Superior control of HIV-1 replication by CD8+ T cells is reflected by their avidity, polyfunctionality, and clonal turnover. J Exp Med. (2007) 204:2473–85. doi: 10.1084/jem.20070784
56. Lee JK, Stewart-Jones G, Dong T, Harlos K, Di Gleria K, Dorrell L, et al. T cell cross-reactivity and conformational changes during TCR engagement. J Exp Med. (2004) 200:1455–66. doi: 10.1084/jem.20041251
57. Appay V, Sauce D. Assessing immune aging in HIV-infected patients. Virulence (2017) 8:529–38. doi: 10.1080/21505594.2016.1195536
58. Vivar N, Ruffin N, Sammicheli S, Hejdeman B, Rethi B, Chiodi F. Survival and proliferation of CD28- T cells during HIV-1 infection relate to the amplitude of viral replication. J Infect Dis. (2011) 203:1658–67. doi: 10.1093/infdis/jir156
59. Naeger DM, Martin JN, Sinclair E, Hunt PW, Bangsberg DR, Hecht F, et al. Cytomegalovirus-specific T cells persist at very high levels during long-term antiretroviral treatment of HIV disease. PLoS ONE (2010) 5:e8886. doi: 10.1371/journal.pone.0008886
60. Gómez-Mora E, García E, Urrea V, Massanella M, Puig J, Negredo E, et al. Preserved immune functionality and high CMV-specific T-cell responses in HIV-infected individuals with poor CD4+ T-cell immune recovery. Sci Rep. (2017) 7:11711. doi: 10.1038/s41598-017-12013-2
61. Owen RE, Heitman JW, Hirschkorn DF, Lanteri MC, Biswas HH, Martin JN, et al. HIV + elite controllers have low HIV-specific T cell activation yet maintain strong, polyfunctional T cell responses The NIAID Center for HIV/AIDS Vaccine Immunology. AIDS (2010) 15:1095–105. doi: 10.1097/QAD.0b013e3283377a1e
62. Mehandru S, Poles MA, Tenner-Racz K, Horowitz A, Hurley A, Hogan C, et al. Primary HIV-1 infection is associated with preferential depletion of CD4+ T lymphocytes from effector sites in the gastrointestinal tract. J Exp Med. (2004) 200:761–70. doi: 10.1084/jem.20041196
63. Vrisekoop NR, van Gent AB, de Boer SA, Otto JCC, Borleffs R, Steingrover JM, et al. Restoration of the CD4 T Cell Compartment after Long-Term Highly Active Antiretroviral Therapy without Phenotypical Signs of Accelerated Immunological Aging. J Immunol. (2008) 181:1573–81. doi: 10.4049/jimmunol.181.2.1573
64. Betts MR, Casazza JP, Koup RA. Monitoring HIV-specific CD8+ T cell responses by intracellular cytokine production. Immunol Lett. (2001) 79:117–25. doi: 10.1016/S0165-2478(01)00273-5
65. Betts MR, Ambrozak DR, Douek DC, Bonhoeffer S, Brenchley JM, Casazza JP, et al. Analysis of total human immunodeficiency virus (HIV)-specific CD4(+) and CD8(+) T-cell responses: relationship to viral load in untreated HIV infection. J Virol. (2001) 75:11983–91. doi: 10.1128/JVI.75.24.11983-11991.2001
66. Zeng M, Smith AJ, Wietgrefe SW, Southern PJ, Schacker TW, Reilly CS, et al. Cumulative mechanisms of lymphoid tissue fibrosis and T cell depletion in HIV-1 and SIV infections. J Clin Invest. (2011) 121:998–1008. doi: 10.1172/JCI45157
67. Zeng M, Southern PJ, Reilly CS, Beilman GJ, Chipman JG, Schacker TW, et al. Lymphoid tissue damage in HIV-1 infection depletes naive T cells and limits T cell reconstitution after antiretroviral therapy. PLoS Pathog. (2012) 8:e1002437. doi: 10.1371/journal.ppat.1002437
68. Cheret A, Bacchus-Souffan C, Avettand-Fenoel V, Melard A, Nembot G, Blanc C, et al. Combined ART started during acute HIV infection protects central memory CD4+ T cells and can induce remission. J Antimicrob Chemother. (2015) 70:2108–20. doi: 10.1093/jac/dkv084
69. Maldarelli F, Palmer S, King MS, Wiegand A, Polis MA, Mican J, et al. ART suppresses plasma HIV-1 RNA to a stable set point predicted by pretherapy viremia. PLoS Pathog. (2007) 3:e46. doi: 10.1371/journal.ppat.0030046
70. Wing EJ. The aging population with HIV infection. Trans Am Clin Climatol Assoc. (2017) 128:131–44. doi: 10.1016/j.ijid.2016.10.004
71. Rickabaugh TM, Baxter RM, Sehl M, Sinsheimer JS, Hultin PM, Hultin LE, et al. Acceleration of age-associated methylation patterns in HIV-1-infected adults. PLoS ONE (2015) 10:e0119201. doi: 10.1371/journal.pone.0119201
72. Deng Y, Jing Y, Campbell AE, Gravenstein S. Age-related impaired type 1 T cell responses to influenza: reduced activation ex vivo, decreased expansion in CTL culture in vitro, and blunted response to influenza vaccination in vivo in the elderly. J Immunol. (2004) 172:3437–46. doi: 10.4049/jimmunol.172.6.3437
73. Czesnikiewicz-Guzik M, Lee WW, Cui D, Hiruma Y, Lamar DL, Yang ZZ, et al. T cell subset-specific susceptibility to aging. Clin Immunol. (2008) 127:107–18. doi: 10.1016/j.clim.2007.12.002
74. Andrew Gross M, Philipp Jaeger A, Jason Kreisberg F, Licon K, Kristen Jepsen L, Khosroheidari M, et al. Methylome-wide analysis of chronic HIV infection reveals five-year increase in biological age and epigenetic targeting of HLA. Mol Cell (2016) 62:157–68. doi: 10.1016/j.molcel.2016.03.019
75. Cao W, Mehraj V, Trottier B, Baril JG, Leblanc R, Lebouche B, et al. Early initiation rather than prolonged duration of antiretroviral therapy in HIV infection contributes to the normalization of CD8 T-cell counts. Clin Infect Dis. (2016) 62:250–7. doi: 10.1093/cid/civ809
76. Okulicz JF, Grandits GA, Weintrob AC, Landrum ML, Ganesan A, Crum-Cianflone NF, et al. CD4 T cell count reconstitution in HIV controllers after highly active antiretroviral therapy. Clin Infect Dis. (2010) 50:1187–91. doi: 10.1086/651421
77. Mussini C, Lorenzini P, Cozzi-Lepri A, Lapadula G, Marchetti G, Nicastri E, et al. CD4/CD8 ratio normalisation and non-AIDS-related events in individuals with HIV who achieve viral load suppression with antiretroviral therapy: an observational cohort study. Lancet HIV (2015) 2:e98–106. doi: 10.1016/S2352-3018(15)00006-5
78. Antiretroviral Therapy Cohort Collaboration. Life expectancy of individuals on combination antiretroviral therapy in high-income countries: a collaborative analysis of 14 cohort studies. Lancet (2008) 372:293–9. doi: 10.1016/S0140-6736(08)61113-7
79. Piketty C, Weiss L, Thomas F, Mohamed AS, Belec L, Kazatchkine MD. Long-term clinical outcome of human immunodeficiency virus-infected patients with discordant immunologic and virologic responses to a protease inhibitor-containing regimen. J Infect Dis. (2001) 183:1328–35. doi: 10.1086/319861
80. Engsig FN, Gerstoft J, Kronborg G, Larsen CS, Pedersen G, Roge B, et al. Long-term mortality in HIV patients virally suppressed for more than three years with incomplete CD4 recovery: a cohort study. BMC Infect Dis. (2010) 10:318. doi: 10.1186/1471-2334-10-318
81. Lichtner M, Cicconi P, Vita S, Cozzi-Lepri A, Galli M, Lo Caputo S, et al. Cytomegalovirus coinfection is associated with an increased risk of severe non-AIDS-defining events in a large cohort of HIV-infected patients. J Infect Dis. (2015) 211:178–86. doi: 10.1093/infdis/jiu417
82. Olsson J, Wikby A, Johansson B, Lofgren S, Nilsson BO, Ferguson FG. Age-related change in peripheral blood T-lymphocyte subpopulations and cytomegalovirus infection in the very old: the Swedish longitudinal OCTO immune study. Mech Ageing Dev. (2000) 121:187–201. doi: 10.1016/S0047-6374(00)00210-4
83. Staras SA, Dollard SC, Radford KW, Flanders WD, Pass RF, Cannon MJ. Seroprevalence of cytomegalovirus infection in the United States, 1988-1994. Clin Infect Dis. (2006) 43:1143–51. doi: 10.1086/508173
84. Karrer U, Sierro S, Wagner M, Oxenius A, Hengel H, Koszinowski UH, et al. Memory inflation: continuous accumulation of antiviral CD8+ T cells over time. J Immunol. (2003) 170:2022–9. doi: 10.4049/jimmunol.170.4.2022
85. Klenerman P, Oxenius A. T cell responses to cytomegalovirus. Nat Rev Immunol. (2016) 16:367–77. doi: 10.1038/nri.2016.38
86. Freeman ML, Mudd JC, Shive CL, Younes SA, Panigrahi S, Sieg SF, et al. CD8 T-cell expansion and inflammation linked to CMV coinfection in ART-treated HIV infection. Clin Infect Dis. (2016) 62:392–6. doi: 10.1093/cid/civ840
87. Roederer M, Dubs JG, Anderson MT, Raju PA, Herzenberg LA, Herzenberg LA. CD8 naive T cell counts decrease progressively in HIV-infected adults. J Clin Invest. (1995) 95:2061–6. doi: 10.1172/JCI117892
88. Woolfrey AE, Malhotra U, Harrington RD, McNevin J, Manley TJ, Riddell SR, et al. Generation of HIV-1-specific CD8+ cell responses following allogeneic hematopoietic cell transplantation. Blood (2008) 112:3484–7. doi: 10.1182/blood-2008-05-157511
89. Kestens L, Vanham G, Gigase P, Young G, Hannet I, Vanlangendonck F, et al. Expression of activation antigens, HLA-DR and CD38, on CD8 lymphocytes during HIV-1 infection. AIDS (1992) 6:793–7. doi: 10.1097/00002030-199208000-00004
90. Kestens L, Vanham G, Vereecken C, Vandenbruaene M, Vercauteren G, Colebunders RL, et al. Selective increase of activation antigens HLA-DR and CD38 on CD4+ CD45RO+ T lymphocytes during HIV-1 infection. Clin Exp Immunol. (1994) 95:436–41. doi: 10.1111/j.1365-2249.1994.tb07015.x
91. Deeks SG, Kitchen CM, Liu L, Guo H, Gascon R, Narvaez AB, et al. Immune activation set point during early HIV infection predicts subsequent CD4+ T-cell changes independent of viral load. Blood (2004) 104:942–7. doi: 10.1182/blood-2003-09-3333
92. Giorgi JV, Hultin LE, McKeating JA, Johnson TD, Owens B, Jacobson LP, et al. Shorter survival in advanced human immunodeficiency virus type 1 infection is more closely associated with T lymphocyte activation than with plasma virus burden or virus chemokine coreceptor usage. J Infect Dis. (1999) 179:859–70. doi: 10.1086/314660
93. Liu Z, Cumberland WG, Hultin LE, Kaplan AH, Detels R, Giorgi JV. CD8+ T-lymphocyte activation in HIV-1 disease reflects an aspect of pathogenesis distinct from viral burden and immunodeficiency. J Acquir Immune Defic Syndr Hum Retrovirol. (1998) 18:332–40.
94. Almeida M, Cordero M, Almeida J, Orfao A. Relationship between CD38 expression on peripheral blood T-cells and monocytes, and response to antiretroviral therapy: a one-year longitudinal study of a cohort of chronically infected ART-naive HIV-1+ patients. Cytometry B Clin Cytom. (2007) 72:22–33. doi: 10.1002/cyto.b.20144
95. Conrad JA, Ramalingam RK, Duncan CB, Smith RM, Wei J, Barnett L, et al. Antiretroviral therapy reduces the magnitude and T cell receptor repertoire diversity of HIV-specific T cell responses without changing T cell clonotype dominance. J Virol. (2012) 86:4213–21. doi: 10.1128/JVI.06000-11
96. Hunt PW, Martin JN, Sinclair E, Bredt B, Hagos E, Lampiris H, et al. T cell activation is associated with lower CD4+ T cell gains in human immunodeficiency virus-infected patients with sustained viral suppression during antiretroviral therapy. J Infect Dis. (2003) 187:1534–43. doi: 10.1086/374786
97. Lederman MM, Calabrese L, Funderburg NT, Clagett B, Medvik K, Bonilla H, et al. Immunologic failure despite suppressive antiretroviral therapy is related to activation and turnover of memory CD4 cells. J Infect Dis. (2011) 204:1217–26. doi: 10.1093/infdis/jir507
98. McMahon D, Jones J, Wiegand A, Gange SJ, Kearney M, Palmer S, et al. Short-course raltegravir intensification does not reduce persistent low-level viremia in patients with HIV-1 suppression during receipt of combination antiretroviral therapy. Clin Infect Dis. (2010) 50:912–9. doi: 10.1086/650749
99. Palmer S, Maldarelli F, Wiegand A, Bernstein B, Hanna GJ, Brun SC, et al. Low-level viremia persists for at least 7 years in patients on suppressive antiretroviral therapy. Proc Natl Acad Sci USA. (2008) 105:3879–84. doi: 10.1073/pnas.0800050105
100. Chun TW, Shawn Justement J, Murray D, Kim CJ, Blazkova J, Hallahan CW, et al. Effect of antiretroviral therapy on HIV reservoirs in elite controllers. J Infect Dis. (2013) 208:1443–7. doi: 10.1093/infdis/jit306
101. Brenchley JM, Price DA, Schacker TW, Asher TE, Silvestri G, Rao S, et al. Microbial translocation is a cause of systemic immune activation in chronic HIV infection. Nat Med. (2006) 12:1365–71. doi: 10.1038/nm1511
102. Eggena MP, Barugahare B, Jones N, Okello M, Mutalya S, Kityo C, et al. Depletion of regulatory T cells in HIV infection is associated with immune activation. J Immunol. (2005) 174:4407–14. doi: 10.4049/jimmunol.174.7.4407
103. Schacker TW, Nguyen PL, Beilman GJ, Wolinsky S, Larson M, Reilly C, et al. Collagen deposition in HIV-1 infected lymphatic tissues and T cell homeostasis. J Clin Invest. (2002) 110:1133–9. doi: 10.1172/JCI0216413
104. Amu S, Lantto Graham R, Bekele Y, Nasi A, Bengtsson C, Rethi B, et al. Dysfunctional phenotypes of CD4+ and CD8+ T cells are comparable in patients initiating ART during early or chronic HIV-1 infection. Medicine (2016) 95:e3738. doi: 10.1097/MD.0000000000003738
105. Crowell TA, Fletcher JL, Sereti I, Pinyakorn S, Dewar R, Krebs SJ, et al. Initiation of antiretroviral therapy before detection of colonic infiltration by HIV reduces viral reservoirs, inflammation and immune activation. J Int AIDS Soc. (2016) 19:21163. doi: 10.7448/IAS.19.1.21163
106. Hey-Cunningham WJ, Murray JM, Natarajan V, Amin J, Moore CL, Emery S, et al. Early antiretroviral therapy with raltegravir generates sustained reductions in HIV reservoirs but not lower T-cell activation levels. AIDS (2015) 29:911–9. doi: 10.1097/QAD.0000000000000625
107. Jain V, Hartogensis W, Bacchetti P, Hunt PW, Hatano H, Sinclair E, et al. Antiretroviral therapy initiated within 6 months of HIV infection is associated with lower T-cell activation and smaller HIV reservoir size. J Infect Dis. (2013) 208:1202–11. doi: 10.1093/infdis/jit311
108. Vinikoor MJ, Cope A, Gay CL, Ferrari G, McGee KS, Kuruc JD, et al. Antiretroviral therapy initiated during acute HIV infection fails to prevent persistent T-cell activation. J Acquir Immune Defic Syndr. (2013) 62:505–8. doi: 10.1097/QAI.0b013e318285cd33
109. Biancotto A, Iglehart SJ, Vanpouille C, Condack CE, Lisco A, Ruecker E, et al. HIV-1 induced activation of CD4+ T cells creates new targets for HIV-1 infection in human lymphoid tissue ex vivo. Blood (2008) 111:699–704. doi: 10.1182/blood-2007-05-088435
110. Finkel TH, Tudor-Williams G, Banda NK, Cotton MF, Curiel T, Monks C, et al. Apoptosis occurs predominantly in bystander cells and not in productively infected cells of HIV- and SIV-infected lymph nodes. Nat Med. (1995) 1:129–34. doi: 10.1038/nm0295-129
111. Doitsh G, Galloway NL, Geng X, Yang Z, Monroe KM, Zepeda O, et al. Cell death by pyroptosis drives CD4 T-cell depletion in HIV-1 infection. Nature (2014) 505:509–14. doi: 10.1038/nature12940
112. Clutton G, Xu Y, Baldoni PL, Mollan KR, Kirchherr J, Newhard W, et al. The differential short- and long-term effects of HIV-1 latency-reversing agents on T cell function. Sci Rep. (2016) 6:30749. doi: 10.1038/srep30749
113. Laird GM, Bullen CK, Rosenbloom DI, Martin AR, Hill AL, Durand CM, et al. Ex vivo analysis identifies effective HIV-1 latency-reversing drug combinations. J Clin Invest. (2015) 125:1901–12. doi: 10.1172/JCI80142
114. Walker-Sperling VE, Pohlmeyer CW, Tarwater PM, Blankson JN. The effect of latency reversal agents on primary CD8+ T cells: implications for shock and kill strategies for human immunodeficiency virus eradication. EBioMedicine (2016) 8:217–29. doi: 10.1016/j.ebiom.2016.04.019
115. Pantaleo G, Graziosi C, Butini L, Pizzo PA, Schnittman SM, Kotler DP, et al. Lymphoid organs function as major reservoirs for human immunodeficiency virus. Proc Natl Acad Sci USA. (1991) 88:9838–42. doi: 10.1073/pnas.88.21.9838
116. Embretson J, Zupancic M, Ribas JL, Burke A, Racz P, Tenner-Racz K, et al. Massive covert infection of helper T lymphocytes and macrophages by HIV during the incubation period of AIDS. Nature (1993) 362:359–62. doi: 10.1038/362359a0
117. Brenchley JM, Schacker TW, Ruff LE, Price DA, Taylor JH, Beilman GJ, et al. CD4+ T cell depletion during all stages of HIV disease occurs predominantly in the gastrointestinal tract. J Exp Med. (2004) 200:749–59. doi: 10.1084/jem.20040874
118. Banga R, Procopio FA, Noto A, Pollakis G, Cavassini M, Ohmiti K, et al. PD-1(+) and follicular helper T cells are responsible for persistent HIV-1 transcription in treated aviremic individuals. Nat Med. (2016) 22:754–61. doi: 10.1038/nm.4113
119. Kiniry BE, Li S, Ganesh A, Hunt PW, Somsouk M, Skinner PJ, et al. Detection of HIV-1-specific gastrointestinal tissue resident CD8(+) T-cells in chronic infection. Mucosal Immunol. (2018) 11:909–20. doi: 10.1038/mi.2017.96
120. Kiniry BE, Hunt PW, Hecht FM, Somsouk M, Deeks SG, Shacklett BL. Differential expression of CD8(+) T cell cytotoxic effector molecules in blood and gastrointestinal mucosa in HIV-1 infection. J Immunol. (2018) 200:1876–88. doi: 10.4049/jimmunol.1701532
121. Kiniry BE, Ganesh A, Critchfield JW, Hunt PW, Hecht FM, Somsouk M, et al. Predominance of weakly cytotoxic, T-bet(Low)Eomes(Neg) CD8(+) T-cells in human gastrointestinal mucosa: implications for HIV infection. Mucosal Immunol. (2017) 10:1008–20. doi: 10.1038/mi.2016.100
122. Reuter MA, Del Rio Estrada PM, Buggert M, Petrovas C, Ferrando-Martinez S, Nguyen S, et al. HIV-specific CD8(+) T cells exhibit reduced and differentially regulated cytolytic activity in lymphoid tissue. Cell Rep. (2017) 21:3458–70. doi: 10.1016/j.celrep.2017.11.075
123. Kohler SL, Pham MN, Folkvord JM, Arends T, Miller SM, Miles B, et al. Germinal center T follicular helper cells are highly permissive to HIV-1 and alter their phenotype during virus replication. J Immunol. (2016) 196:2711–22. doi: 10.4049/jimmunol.1502174
124. Connick E, Mattila T, Folkvord JM, Schlichtemeier R, Meditz AL, Graham MR, et al. CTL fail to accumulate at sites of HIV-1 replication in lymphoid tissue. J Immunol. (2007) 178:6975–83. doi: 10.4049/jimmunol.178.11.6975
125. Connick E, Folkvord JM, Lind KT, Rakasz EG, Miles B, Wilson NA, et al. Compartmentalization of simian immunodeficiency virus replication within secondary lymphoid tissues of rhesus macaques is linked to disease stage and inversely related to localization of virus-specific CTL. J Immunol. (2014) 193:5613–25. doi: 10.4049/jimmunol.1401161
126. Fukazawa Y, Lum R, Okoye AA, Park H, Matsuda K, Bae JY, et al. B cell follicle sanctuary permits persistent productive simian immunodeficiency virus infection in elite controllers. Nat Med. (2015) 21:132–9. doi: 10.1038/nm.3781
127. Tan JK, O'Neill HC. Maturation requirements for dendritic cells in T cell stimulation leading to tolerance versus immunity. J Leukocyte Biol. (2005) 78:319–24. doi: 10.1189/jlb.1104664
128. Sanchez JL, Hunt PW, Reilly CS, Hatano H, Beilman GJ, Khoruts A, et al. Lymphoid fibrosis occurs in long-term nonprogressors and persists with antiretroviral therapy but may be reversible with curative interventions. J Infect Dis. (2015) 211:1068–75. doi: 10.1093/infdis/jiu586
129. Cockerham LR, Yukl SA, Harvill K, Somsouk M, Joshi SK, Sinclair E, et al. A randomized controlled trial of lisinopril to decrease lymphoid fibrosis in antiretroviral-treated, HIV-infected individuals. Pathog Immun. (2017) 2:310–34. doi: 10.20411/pai.v2i3.207
130. Fletcher AL, Acton SE, Knoblich K. Lymph node fibroblastic reticular cells in health and disease. Nat Rev Immunol. (2015) 15:350–61. doi: 10.1038/nri3846
131. Campisi J, d'Adda di Fagagna F. Cellular senescence: when bad things happen to good cells. Nat Rev Mol Cell Biol. (2007) 8:729–40. doi: 10.1038/nrm2233
132. Rufer N, Migliaccio M, Antonchuk J, Humphries RK, Roosnek E, Lansdorp PM, et al. Transfer of the human telomerase reverse transcriptase (TERT) gene into T lymphocytes results in extension of replicative potential. Blood (2001) 98:597–603. doi: 10.1182/blood.V98.3.597
133. Sahin E, DePinho RA. Linking functional decline of telomeres, mitochondria and stem cells during ageing. Nature (2010) 464:520–8. doi: 10.1038/nature08982
134. Le Priol Y, Puthier D, Lécureuil C, Combadière C, Debré P, Nguyen C, et al. High cytotoxic and specific migratory potencies of senescent CD8+ CD57+ cells in HIV-infected and uninfected individuals. J. Immunol. (2006) 177:5145–54. doi: 10.4049/jimmunol.177.8.5145
135. Kaushal S, Landay AL, Lederman MM, Connick E, Spritzler J, Kuritzkes DR, et al. Increases in T cell telomere length in HIV infection after antiretroviral combination therapy for HIV-1 infection implicate distinct population dynamics in CD4+ and CD8+ T cells. Clin Immunol. (1999) 92:14–24. doi: 10.1006/clim.1999.4726
136. H.Côté CF, A.Hsieh YY. Leukocyte telomere length in HIV infection: a marker of persistent immune aging or transient immune reconstitution? J Infect Dis. (2018). 218:1521–2. doi: 10.1093/infdis/jiy365
137. Migueles SA, Weeks KA, Nou E, Berkley AM, Rood JE, Osborne CM, et al. Defective human immunodeficiency virus-specific CD8+ T-cell polyfunctionality, proliferation, and cytotoxicity are not restored by antiretroviral therapy. J Virol. (2009) 83:11876–89. doi: 10.1128/JVI.01153-09
138. Larbi A, Fulop T. From “truly naive” to “exhausted senescent” T cells: when markers predict functionality. Cytometry A (2014) 85:25–35. doi: 10.1002/cyto.a.22351
139. Verma K, Ogonek J, Varanasi PR, Luther S, Bunting I, Thomay K, et al. Human CD8+ CD57- TEMRA cells: too young to be called “old.” PLoS ONE (2017) 12:e0177405. doi: 10.1371/journal.pone.0177405
140. Chou JP, Ramirez CM, Ryba DM, Koduri MP, Effros RB, Prostaglandin E2 promotes features of replicative senescence in chronically activated human CD8+ T cells. PLoS ONE (2014) 9:e99432. doi: 10.1371/journal.pone.0099432
141. Bellon M, Nicot C. Telomere dynamics in immune senescence and exhaustion triggered by chronic viral infection. Viruses (2017) 9:E289. doi: 10.3390/v9100289
142. Lee SA, Sinclair E, Jain V, Huang Y, Epling L, Van Natta M, et al. Low proportions of CD28– CD8+ T cells expressing CD57 can be reversed by early ART initiation and predict mortality in treated HIV infection. J Infect Dis. (2014) 210:374–82. doi: 10.1093/infdis/jiu109
143. Connors M, Kovacs JA, Krevat S, Gea-Banacloche JC, Sneller MC, Flanigan M, et al. HIV infection induces changes in CD4+ T-cell phenotype and depletions within the CD4+ T-cell repertoire that are not immediately restored by antiviral or immune-based therapies. Nat Med. (1997) 3:533–40. doi: 10.1038/nm0597-533
144. Yin L, Kou ZC, Rodriguez C, Hou W, Goodenow MM, Sleasman JW. Antiretroviral therapy restores diversity in the T-cell receptor Vbeta repertoire of CD4 T-cell subpopulations among human immunodeficiency virus type 1-infected children and adolescents. Clin Vaccine Immunol. (2009) 16:1293–301. doi: 10.1128/CVI.00074-09
145. Baum PD, Young JJ, Schmidt D, Zhang Q, Hoh R, Busch M, et al. Blood T-cell receptor diversity decreases during the course of HIV infection, but the potential for a diverse repertoire persists. Blood (2012) 119:3469–77. doi: 10.1182/blood-2011-11-395384
146. Hebbeler AM, Propp N, Cairo C, Li H, Cummings JS, Jacobson LP, et al. Failure to restore the Vgamma2-Jgamma1.2 repertoire in HIV-infected men receiving highly active antiretroviral therapy (HAART). Clin Immunol. (2008) 128:349–57. doi: 10.1016/j.clim.2008.04.008
147. González-Serna A, Abad-Fernández M, Soriano-Sarabia N, Leal M, Vallejo A. CD8 TCR β chain repertoire expansions and deletions are related with immunologic markers in HIV-1-infected patients during treatment interruption. J Clin Virol. (2013) 58:703–9. doi: 10.1016/j.jcv.2013.10.017
148. Joglekar AV, Liu Z, Weber JK, Ouyang Y, Jeppson JD, Noh WJ, et al. T cell receptors for the HIV KK10 epitope from patients with differential immunologic control are functionally indistinguishable. Proc Natl Acad Sci USA. (2018) 115:1877–82. doi: 10.1073/pnas.1718659115
149. Koup RA, Safrit JT, Cao Y, Andrews CA, McLeod G, Borkowsky W, et al. Temporal association of cellular immune responses with the initial control of viremia in primary human immunodeficiency virus type 1 syndrome. J Virol. (1994) 68:4650–5.
150. Price DA, Goulder PJ, Klenerman P, Sewell AK, Easterbrook PJ, Troop M, et al. Positive selection of HIV-1 cytotoxic T lymphocyte escape variants during primary infection. Proc Natl Acad Sci USA. (1997) 94:1890–5. doi: 10.1073/pnas.94.5.1890
151. Goonetilleke N, Liu MK, Salazar-Gonzalez JF, Ferrari G, Giorgi E, Ganusov VV, et al. The first T cell response to transmitted/founder virus contributes to the control of acute viremia in HIV-1 infection. J Exp Med. (2009) 206:1253–72. doi: 10.1084/jem.20090365
152. Brackenridge S, Evans EJ, Toebes M, Goonetilleke N, Liu MK, di Gleria K, et al. An early HIV mutation within an HLA-B*57-restricted T cell epitope abrogates binding to the killer inhibitory receptor 3DL1. J Virol. (2011) 85:5415–22. doi: 10.1128/JVI.00238-11
153. Ferrari G, Pollara J, Kozink D, Harms T, Drinker M, Freel S, et al. An HIV-1 gp120 envelope human monoclonal antibody that recognizes a C1 conformational epitope mediates potent antibody-dependent cellular cytotoxicity (ADCC) activity and defines a common ADCC epitope in human HIV-1 serum. J Virol. (2011) 85:7029–36. doi: 10.1128/JVI.00171-11
154. Ganusov VV, Goonetilleke N, Liu MK, Ferrari G, Shaw GM, McMichael AJ, et al. Fitness costs and diversity of the cytotoxic T lymphocyte (CTL) response determine the rate of CTL escape during acute and chronic phases of HIV infection. J Virol. (2011) 85:10518–28. doi: 10.1128/JVI.00655-11
155. Addo MM, Yu XG, Rathod A, Cohen D, Eldridge RL, Strick D, et al. Comprehensive epitope analysis of human immunodeficiency virus type 1 (HIV-1)-specific T-cell responses directed against the entire expressed HIV-1 genome demonstrate broadly directed responses, but no correlation to viral load. J Virol. (2003) 77:2081–92. doi: 10.1128/JVI.77.3.2081-2092.2003
156. Edwards BH, Bansal A, Sabbaj S, Bakari J, Mulligan MJ, Goepfert PA. Magnitude of functional CD8+ T-cell responses to the gag protein of human immunodeficiency virus type 1 correlates inversely with viral load in plasma. J Virol. (2002) 76:2298–305. doi: 10.1128/jvi.76.5.2298-2305.2002
157. Dalod M, Dupuis M, Deschemin JC, Sicard D, Salmon D, Delfraissy JF, et al. Broad, intense anti-human immunodeficiency virus (HIV) ex vivo CD8(+) responses in HIV type 1-infected patients: comparison with anti-Epstein-Barr virus responses and changes during antiretroviral therapy. J Virol. (1999) 73:7108–16.
158. Hersperger AR, Martin JN, Shin LY, Sheth PM, Kovacs CM, Cosma GL, et al. Increased HIV-specific CD8 T-cell cytotoxic potential in HIV elite controllers is associated with T-bet expression. Blood (2011) 117:3799–808. doi: 10.1182/blood-2010-12-322727
159. Ogg GS, Jin X, Bonhoeffer S, Moss P, Nowak MA, Monard S, et al. Decay kinetics of human immunodeficiency virus-specific effector cytotoxic T lymphocytes after combination antiretroviral therapy. J Virol. (1999) 73:797–800.
160. Casazza JP, Betts MR, Picker LJ, Koup RA. Decay kinetics of human immunodeficiency virus-specific CD8+ T cells in peripheral blood after initiation of highly active antiretroviral therapy. J Virol. (2001) 75:6508–16. doi: 10.1128/JVI.75.14.6508-6516.2001
161. Lacabaratz-Porret C, Urrutia A, Doisne JM, Goujard C, Deveau C, Dalod M, et al. Impact of antiretroviral therapy and changes in virus load on human immunodeficiency virus (HIV)–specific T cell responses in primary HIV infection. J Infect Dis. (2003) 187:748–57. doi: 10.1086/368333
162. Ferre AL, Lemongello D, Hunt PW, Morris MM, Garcia JC, Pollard RB, et al. Immunodominant HIV-specific CD8+ T-cell responses are common to blood and gastrointestinal mucosa, and Gag-specific responses dominate in rectal mucosa of HIV controllers. J Virol. (2010) 84:10354–65. doi: 10.1128/JVI.00803-10
163. Kiepiela P, Ngumbela K, Thobakgale C, Ramduth D, Honeyborne I, Moodley E, et al. CD8+ T-cell responses to different HIV proteins have discordant associations with viral load. Nat Med. (2007) 13:46–53. doi: 10.1038/nm1520
164. Riou C, Burgers WA, Mlisana K, Koup RA, Roederer M, Abdool Karim SS, et al. Differential impact of magnitude, polyfunctional capacity, and specificity of HIV-specific CD8+ T cell responses on HIV set point. J Virol. (2014) 88:1819–24. doi: 10.1128/JVI.02968-13
165. Migueles SA, Osborne CM, Royce C, Compton AA, Joshi RP, Weeks KA, et al. Lytic granule loading of CD8+ T cells is required for HIV-infected cell elimination associated with immune control. Immunity (2008) 29:1009–21. doi: 10.1016/j.immuni.2008.10.010
166. Saez-Cirion A, Sinet M, Shin SY, Urrutia A, Versmisse P, Lacabaratz C, et al. Heterogeneity in HIV suppression by CD8 T cells from HIV controllers: association with Gag-specific CD8 T cell responses. J Immunol. (2009) 182:7828–37. doi: 10.4049/jimmunol.0803928
167. Ndhlovu ZM, Chibnik LB, Proudfoot J, Vine S, McMullen A, Cesa K, et al. High-dimensional immunomonitoring models of HIV-1-specific CD8 T-cell responses accurately identify subjects achieving spontaneous viral control. Blood (2013) 121:801–11. doi: 10.1182/blood-2012-06-436295
168. Cao W, Mehraj V, Kaufmann DE, Li T, Routy JP Elevation and persistence of CD8 T-cells in HIV infection: the Achilles heel in the ART era. J Int AIDS Soc. (2016) 19:20697. doi: 10.7448/IAS.19.1.20697
169. Betts MR, Brenchley JM, Price DA, De Rosa SC, Douek DC, Roederer M, et al. Sensitive and viable identification of antigen-specific CD8+ T cells by a flow cytometric assay for degranulation. J Immunol Methods (2003) 281:65–78. doi: 10.1016/S0022-1759(03)00265-5
170. Migueles SA, Laborico AC, Shupert WL, Sabbaghian MS, Rabin R, Hallahan CW, et al. HIV-specific CD8+ T cell proliferation is coupled to perforin expression and is maintained in nonprogressors. Nat Immunol. (2002) 3:1061–8. doi: 10.1038/ni845
171. Day CL, Kaufmann DE, Kiepiela P, Brown JA, Moodley ES, Reddy S, et al. PD-1 expression on HIV-specific T cells is associated with T-cell exhaustion and disease progression. Nature (2006) 443:350–4. doi: 10.1038/nature05115
172. Hunt PW, Brenchley J, Sinclair E, McCune JM, Roland M, Page-Shafer K, et al. Relationship between T cell activation and CD4+ T cell count in HIV-seropositive individuals with undetectable plasma HIV RNA levels in the absence of therapy. J Infect Dis. (2008) 197:126–33. doi: 10.1086/524143.
173. Tauriainen J, Scharf L, Frederiksen J, Naji A, Ljunggren HG, Sonnerborg A, et al. Perturbed CD8(+) T cell TIGIT/CD226/PVR axis despite early initiation of antiretroviral treatment in HIV infected individuals. Sci Rep. (2017) 7:40354. doi: 10.1038/srep40354
174. Yan J, Sabbaj S, Bansal A, Amatya N, Shacka JJ, Goepfert PA, et al. HIV-specific CD8+ T cells from elite controllers are primed for survival. J Virol. (2013) 87:5170–81. doi: 10.1128/JVI.02379-12
175. Ananworanich J, Sacdalan CP, Pinyakorn S, Chomont N, de Souza M, Luekasemsuk T, et al. Virological and immunological characteristics of HIV-infected individuals at the earliest stage of infection. J Virus Eradicat. (2016) 2:43–8.
176. Julg B, Williams KL, Reddy S, Bishop K, Qi Y, Carrington M, et al. Enhanced anti-HIV functional activity associated with Gag-specific CD8 T-cell responses. J Virol. (2010) 84:5540–9. doi: 10.1128/JVI.02031-09
177. Chen H, Piechocka-Trocha A, Miura T, Brockman MA, Julg BD, Baker BM, et al. Differential neutralization of human immunodeficiency virus (HIV) replication in autologous CD4 T cells by HIV-specific cytotoxic T lymphocytes. J Virol. (2009) 83:3138–49. doi: 10.1128/JVI.02073-08
178. Mothe B, Llano A, Ibarrondo J, Daniels M, Miranda C, Zamarreño J, et al. Definition of the viral targets of protective HIV-1-specific T cell responses. J Trans Med. (2011) 9:208. doi: 10.1186/1479-5876-9-208
179. Shannon CE. A mathematical theory of communication. Bell Syst Tech J. (1948) 27:379–423. doi: 10.1002/j.1538-7305.1948.tb01338.x
180. Liu MK, Hawkins N, Ritchie AJ, Ganusov VV, Whale V, Brackenridge S, et al. Vertical T cell immunodominance and epitope entropy determine HIV-1 escape. J Clin Invest. (2013) 123:380–93. doi: 10.1172/JCI65330
181. Phillips RE, Rowland-Jones S, Nixon DF, Gotch FM, Edwards JP, Ogunlesi AO, et al. Human immunodeficiency virus genetic variation that can escape cytotoxic T cell recognition. Nature (1991) 354:453–9. doi: 10.1038/354453a0
182. Deng K, Pertea M, Rongvaux A, Wang L, Durand CM, Ghiaur G, et al. Broad CTL response is required to clear latent HIV-1 due to dominance of escape mutations. Nature (2015) 517:381–5. doi: 10.1038/nature14053
183. Appay V, Nixon DF, Donahoe SM, Gillespie GM, Dong T, King A, et al. HIV-specific CD8(+) T cells produce antiviral cytokines but are impaired in cytolytic function. J Exp Med. (2000) 192:63–75. doi: 10.1084/jem.192.1.63
184. Shankar P, Russo M, Harnisch B, Patterson M, Skolnik P, Lieberman J. Impaired function of circulating HIV-specific CD8(+) T cells in chronic human immunodeficiency virus infection. Blood (2000) 96:3094–101.
185. Kostense S, Vandenberghe K, Joling J, Van Baarle D, Nanlohy N, Manting E, et al. Persistent numbers of tetramer+ CD8(+) T cells, but loss of interferon-gamma+ HIV-specific T cells during progression to AIDS. Blood (2002) 99:2505–11. doi: 10.1182/blood.V99.7.2505
186. Rehr M, Cahenzli J, Haas A, Price DA, Gostick E, Huber M, et al. Emergence of polyfunctional CD8+ T cells after prolonged suppression of human immunodeficiency virus replication by antiretroviral therapy. J Virol. (2008) 82:3391–404. doi: 10.1128/JVI.02383-07
187. Jones RB, Ndhlovu LC, Barbour JD, Sheth PM, Jha AR, Long BR, et al. Tim-3 expression defines a novel population of dysfunctional T cells with highly elevated frequencies in progressive HIV-1 infection. J Exp Med. (2008) 205:2763–79. doi: 10.1084/jem.20081398
188. Tian X, Zhang A, Qiu C, Wang W, Yang Y, Qiu C, et al. The upregulation of LAG-3 on T cells defines a subpopulation with functional exhaustion and correlates with disease progression in HIV-infected subjects. J Immunol. (2015) 194:3873–82. doi: 10.4049/jimmunol.1402176
189. Rutishauser RL, Hartogensis W, Deguit CD, Krone M, Hoh R, Hecht FM, et al. Early and delayed antiretroviral therapy results in comparable reductions in CD8(+) T cell exhaustion marker expression. AIDS Res Hum Retroviruses (2017) 33:658–67. doi: 10.1089/aid.2016.0324
190. Leavy O. Immunotherapy: a triple blow for cancer. Nat Rev Cancer (2015) 15:258–9. doi: 10.1038/nrc3946
191. Gay CL, Bosch RJ, Ritz J, Hataye JM, Aga E, Tressler RL, et al. Clinical trial of the anti-PD-L1 antibody BMS-936559 in HIV-1 infected participants on suppressive antiretroviral therapy. J Infect Dis. (2017) 215:1725–33. doi: 10.1093/infdis/jix191
192. Colston E, Grasela D, Gardiner D, Bucy RP, Vakkalagadda B, Korman AJ, et al. An open-label, multiple ascending dose study of the anti-CTLA-4 antibody ipilimumab in viremic HIV patients. PLoS ONE (2018) 13:e0198158. doi: 10.1371/journal.pone.0198158
193. Mylvaganam GH, Chea LS, Tharp GK, Hicks S, Velu V, Iyer SS, et al. Combination anti-PD-1 and antiretroviral therapy provides therapeutic benefit against SIV. JCI Insight (2018). doi: 10.1172/jci.insight.122940. [Epub ahead of print].
194. Nishimura Y, Gautam R, Chun TW, Sadjadpour R, Foulds KE, Shingai M, et al. Early antibody therapy can induce long-lasting immunity to SHIV. Nature (2017) 543:559–63. doi: 10.1038/nature21435.
195. Ngu LN, Nji NN, Ambada G, Ngoh AA, Njambe Priso GD, Tchadji JC, et al. Dendritic cell targeted HIV-1 gag protein vaccine provides help to a recombinant Newcastle disease virus vectored vaccine including mobilization of protective CD8(+) T cells. Immun Inflamm Dis. (2018) 6:163–75. doi: 10.1002/iid3.209
196. Gray GE, Laher F, Lazarus E, Ensoli B, Corey L. Approaches to preventative and therapeutic HIV vaccines. Curr Opin Virol. (2016) 17:104–9. doi: 10.1016/j.coviro.2016.02.010
197. Pantaleo G, Levy Y. Therapeutic vaccines and immunological intervention in HIV infection: a paradigm change. Curr Opin HIV AIDS (2016) 11:576–84. doi: 10.1097/COH.0000000000000324
198. Borthwick N, Ahmed T, Ondondo B, Hayes P, Rose A, Ebrahimsa U, et al. Vaccine-elicited human T cells recognizing conserved protein regions inhibit HIV-1. Mol Ther. (2014) 22:464–75. doi: 10.1038/mt.2013.248
199. Lam S, Sung J, Cruz C, Castillo-Caro P, Ngo M, Garrido C, et al. Broadly-specific cytotoxic T cells targeting multiple HIV antigens are expanded from HIV+ patients: implications for immunotherapy. Mol Ther. (2015) 23:387–95. doi: 10.1038/mt.2014.207
200. Patel S, Lam S, Cruz CR, Wright K, Cochran C, Ambinder RF, et al. Functionally active HIV-specific T cells that target Gag and Nef can be expanded from virus-naive donors and target a range of viral epitopes: implications for a cure strategy after allogeneic hematopoietic stem cell transplantation. Biol Blood Marrow Transplant. (2016) 22:536–41. doi: 10.1016/j.bbmt.2015.12.007
201. Bollard CM, Heslop HE. T cells for viral infections after allogeneic hematopoietic stem cell transplant. Blood (2016) 127:3331–40. doi: 10.1182/blood-2016-01-628982
202. Bollard CM, Gottschalk S, Torrano V, Diouf O, Ku S, Hazrat Y, et al. Sustained complete responses in patients with lymphoma receiving autologous cytotoxic T lymphocytes targeting epstein-barr virus latent membrane proteins. J Clin Oncol. (2014) 32:798–808. doi: 10.1200/JCO.2013.51.5304
203. Sung JA, Patel S, Clohosey ML, Roesch L, Tripic T, Kuruc JD, et al. HIV-specific, ex vivo expanded T cell therapy: feasibility, safety, and efficacy in ART-suppressed HIV-infected individuals. Mol Ther. (2018) 26:2496–506. doi: 10.1016/j.ymthe.2018.08.015
204. Tan MP, Gerry AB, Brewer JE, Melchiori L, Bridgeman JS, Bennett AD, et al. T cell receptor binding affinity governs the functional profile of cancer-specific CD8+ T cells. Clin Exp Immunol. (2015) 180:255–70. doi: 10.1111/cei.12570
205. Siliciano JD, Siliciano RF. A long-term latent reservoir for HIV-1: discovery and clinical implications. J Antimicrob Chemother. (2004) 54:6–9. doi: 10.1093/jac/dkh292
206. Chomont N, El-Far M, Ancuta P, Trautmann L, Procopio FA, Yassine-Diab B, et al. HIV reservoir size and persistence are driven by T cell survival and homeostatic proliferation. Nat Med. (2009) 15:893–900. doi: 10.1038/nm.1972
207. Archin NM, Liberty AL, Kashuba AD, Choudhary SK, Kuruc JD, Crooks AM, et al. Administration of vorinostat disrupts HIV-1 latency in patients on antiretroviral therapy. Nature (2012) 487:482–5. doi: 10.1038/nature11286
208. Elliott JH, Wightman F, Solomon A, Ghneim K, Ahlers J, Cameron MJ, et al. Activation of HIV transcription with short-course vorinostat in HIV-infected patients on suppressive antiretroviral therapy. PLoS Pathog. (2014) 10:e1004473. doi: 10.1371/journal.ppat.1004473
209. Sogaard OS, Graversen ME, Leth S, Olesen R, Brinkmann CR, Nissen SK, et al. The depsipeptide romidepsin reverses HIV-1 latency in vivo. PLoS Pathog. (2015) 11:e1005142. doi: 10.1371/journal.ppat.1005142
210. Mothe B, Climent N, Plana M, Rosas M, Jimenez JL, Munoz-Fernandez MA, et al. Safety and immunogenicity of a modified vaccinia Ankara-based HIV-1 vaccine (MVA-B) in HIV-1-infected patients alone or in combination with a drug to reactivate latent HIV-1. J Antimicrob Chemother. (2015) 70:1833–42. doi: 10.1093/jac/dkv046
211. Achenbach CJ, Assoumou L, Deeks SG, Wilkin TJ, Berzins B, Casazza JP, et al. Effect of therapeutic intensification followed by HIV DNA prime and rAd5 boost vaccination on HIV-specific immunity and HIV reservoir (EraMune 02): a multicentre randomised clinical trial. Lancet HIV (2015) 2:e82–91. doi: 10.1016/S2352-3018(15)00026-0
Keywords: HIV, CD8 T cell, antiretroviral therapy (ART), HIV cure strategies, aging, immunosenescence, vaccines
Citation: Warren JA, Clutton G and Goonetilleke N (2019) Harnessing CD8+ T Cells Under HIV Antiretroviral Therapy. Front. Immunol. 10:291. doi: 10.3389/fimmu.2019.00291
Received: 01 October 2018; Accepted: 04 February 2019;
Published: 26 February 2019.
Edited by:
John Frater, University of Oxford, United KingdomReviewed by:
Vijayakumar Velu, Emory University, United StatesCristian Apetrei, University of Pittsburgh, United States
Copyright © 2019 Warren, Clutton and Goonetilleke. This is an open-access article distributed under the terms of the Creative Commons Attribution License (CC BY). The use, distribution or reproduction in other forums is permitted, provided the original author(s) and the copyright owner(s) are credited and that the original publication in this journal is cited, in accordance with accepted academic practice. No use, distribution or reproduction is permitted which does not comply with these terms.
*Correspondence: Nilu Goonetilleke, bmlsdV9nb29uZXRpbGxla2VAbWVkLnVuYy5lZHU=