Corrigendum: Inonotus sanghuang Polyphenols Attenuate Inflammatory Response Via Modulating the Crosstalk Between Macrophages and Adipocytes
- 1Institute of Infection and Immunity of Huaihe Hospital, Henan University, Kaifeng, China
- 2School of Physical Education, Henan University, Kaifeng, China
- 3College of Biology Science and Engineering, Hebei University of Economics and Business, Shijiazhuang, Hebei, China
Aims: Obesity is characterized as a chronic state of low-grade inflammation with progressive immune cell infiltration into adipose tissue. Adipose tissue macrophages play a critical role in the establishment of chronic inflammatory states and metabolic dysfunctions. Inonotus (I.) sanghuang and its extract polyphenols exhibit anti-carcinogenesis, anti-inflammatory, and anti-oxidant activities. However, the action of I. sanghuang polyphenols in obesity-related inflammation has not been reported. The aim of this study was to explore the anti-inflammatory action of polyphenols from I. sanghuang extract (ISE) in macrophages and the interaction between macrophages and adipocytes.
Materials and Methods: RAW264.7 macrophages were stimulated with LPS or conditioned medium of hypertrophied 3T3-L1 adipocytes or cocultured with differentiated adipocytes in the presence of different doses of ISE. The inflammatory cytokines were evaluated by ELISA, the MAPK, NF-κB, and IL-6/STAT3 signals were determined by immunoblotting, and the migrated function of macrophages was determined by migration assay.
Results: ISE suppressed the inflammatory mediators including NO, TNF-α, IL-6, and MCP-1 induced by either LPS or conditioned medium derived from 3T3-L1 adipocytes. ISE also decreased the production of these inflammatory mediators in cocultures of 3T3-L1 adipocytes and RAW264.7 macrophages. Furthermore, ISE blocked RAW264.7 macrophages migration toward 3T3-L1 adipocytes in cocultures. Finally, this effect of ISE might be mediated via inhibiting ERK, p38, and STAT3 activation.
Conclusions: Our findings indicate the possibility that ISE suppresses the interaction between macrophages and adipocytes, attenuates chronic inflammation in adipose tissue and improves obesity-related insulin resistance and complication, suggesting that ISE might be a valuable medicinal food effective in improving insulin resistance and metabolic syndrome.
Introduction
Obesity is a medical condition in which excess body fat has accumulated to the extent that it may have a negative effect on health. Obesity prevalence has doubled since 1980 and been continuously increasing. In 2015, high BMI accounted for 4.0 million deaths globally, nearly 40% of which occurred among non-obese people (1).
Obesity is a chronic disease, and studies (2) have shown that obesity is not only related to the occurrence of a variety of chronic diseases, but also a risk factor for these chronic non-communicable diseases such as type 2 diabetes mellitus (T2DM), hypertension, coronary heart disease, and strokes (3). Not only does obesity affect health, but also the complications it causes have become some of the main disease burdens worldwide. However, the metabolic response to obesity is diverse; a growing amount of evidence suggests that there is a considerable proportion of obese individuals that lack obesity-associated diseases and are metabolically healthy (4). Inflammation is one of the causes that distinguish metabolically healthy from metabolically unhealthy obesity. Obesity-related inflammation is mainly caused by cytokines secreted from adipose tissue macrophages (ATMs) (5). Inflammation that ATMs drive links obesity to insulin resistance, which is a central mechanism in obesity-associated diseases such as T2DM and metabolic syndrome (6). With progressive obesity, ATMs are crucial mediators of meta-inflammation, insulin resistance, metabolic dysfunction, and have other bad influences on adipocyte function (7).
ATMs are distributed between adipocytes and along vascular structures in adipose tissue, and they secrete anti-inflammatory mediators such as IL-10 and catecholamine which regulate adipocyte lipid metabolism. Resident macrophages in tissue show prodigious heterogeneity in their activities and functions, primarily reflecting their local metabolic and immune microenvironment (8). There is substantial evidence that ATMs exhibit the phenotypic change from M2 or “alternatively activated” (anti-inflammatory) macrophages to M1 or “classically activated” (pro-inflammatory) macrophages polarization during the course of obesity, thereby accelerating adipose tissue inflammation (9). M1 macrophages secrete pro-inflammatory cytokines including TNF-α, IL-6, and IL-1β, that act as main effectors of impaired adipocyte function and inflammatory signals. On the one hand, the pro-inflammatory factors derived from M1 macrophages like TNF-α act on the receptors in hypertrophied adipocytes, thereby inducing pro-inflammatory cytokine production such as monocyte chemotactic protein-1 (MCP-1) and adipocyte lipolysis through nuclear factor-κB (NF-κB)-dependent and -independent [possibly members of mitogen-activated protein kinases (MAPK)-dependent] mechanisms, respectively (10). A series of processes described above promote the decomposition of adipocytes and the continuous production of free fatty acids (FFA). Dysregulation of adipocytokines and overproduction of FFA will result in ectopic lipid accumulation and lipotoxicity such as endothelial dysfunction (atherosclerosis), insulin resistance (diabetes), and non-alcoholic steatohepatitis (11). On the other hand, saturated fatty acids emerged from adipocytes activate Toll-like receptor 4 (TLR4) signaling in macrophages and promote the release of inflammatory factors (12). A deficiency of TLR4 could protect against obesity-induced M1 polarization and adipose tissue inflammation (12). This finding indicates that the vicious cycle established among adipocytes and ATMs augments chronic inflammatory changes (13) and the inhibition of chronic inflammation is a significant target in the treatment of insulin resistance and metabolic syndrome.
Mushrooms are widely grown in nature, and many of them have been traditionally used as medicinal foods in Asian countries (14, 15). Mushroom Phellinus linteus (“Sanghuang” in Chinese) is a popular medicinal polypore used throughout China, Japan, and Korea (16) and plays a physiological function in resistive effects of oxidize, germ, and tumor, as well as reducing the blood sugar and lipemia and improving immunity (17–19). More importantly, no apparent adversities after its consumption have been reported. While 15 sanghuang mushroom species have been found in the world, only some of them were found to display anti-inflammatory, antioxidant and anti-carcinogenic activities (16, 19, 20). Inonotus sanghuang (I. sanghuang) is one species of sanghuang mushroom and a white-rot fungus in the family of hymenochaetaceae. Ethanol extract of I. sanghuang mycelia produced from liquid fermentation scavenged DPPH and hydroxyl radicals has been shown to have anti-oxidant activity due to the existing phytochemicals (polyphenolics), such as rutin, eriodictyol, naringenin, and sakuranetin (21). Our recent in vitro study has shown that the anti-oxidant, anti-proliferative, and anti-microbial activities have been found in I. Sanghuang extract (polyphenols) from another Sanghuang species, wild I. Sanghuang from the Aershan Region of Inner Mongolia (Inner Mongolia, China) (22). However, there are no studies exploring the anti-inflammatory and immunomodulating properties of wild I. Sanghuang from the Aershan Region of Inner Mongolia and its use for the prevention/treatment of inflammation-related diseases.
In the present study, we investigated the anti-inflammatory property of I. sanghuang extract (ISE) on RAW264.7 macrophages and then explored the effect of ISE on the crosstalk between RAW264.7 macrophages and 3T3-L1 adipocytes using in vitro cell coculture models.
Materials and Methods
Chemicals
I. sanghuang was collected from the Aershan Region of Inner Mongolia (Inner Mongolia, China) and I. sanghuang extract (ISE) was prepared as described previously (22). ISE polyphenols from ethyl acetate fraction of I. sanghuang powder was prepared and have been characterized as described previously to identify 6 compounds, namely, rutin, quercetin, quercitrin, icarisid II, isorhamnetin and chlorogenic acid, which have been suggested to have potent anti-oxidant, anti-proliferative and anti-microbial activities (22). Thus, we use ethyl acetate fraction (EAF) as I. sanghuang extract (ISE) for this study. Concentrated ISE was stored at −20°C until further use. A stock solution of ISE dissolved in DMSO at 10 mg/mL was stored at −80°C and diluted with culture medium to the appropriate working concentrations immediately prior to use.
Cell Cultures
The RAW264.7 macrophage cell line was provided by J. Jin (Zhejiang University, Hangzhou, China) and maintained in DMEM supplemented with penicillin (100 U/mL)-streptomycin (100 μg/mL) and 10% heat-inactivated fetal bovine serum in a humidified 5% CO2 atmosphere at 37°C. The method of 3T3-L1 adipocyte differentiation was performed as described previously (23). Briefly, 3T3-L1 preadipocytes (Saierbio Inc., Tianjin, China) were cultured in 24-well plates (2.5 × 105 cells/well) in DMEM with 10% calf serum, 100 U/mL penicillin, and 100 μg/mL streptomycin at 37°C under a humidified 5% CO2 atmosphere. Two days after the preadipocytes post confluency, use 3-Isobutyl-1-Methylxanthine (IBMX, 0.5 mM), dexamethasone (1.0 μM) and insulin (10 μg/mL) in 10% FBS/DMEM to stimulate the cells. Twelve to twenty days later, a large amount of red lipid droplets were observed by oil red O staining and the cells were used as hypertrophied 3T3-L1 adipocytes (Supplemental Figure 1). Adipocytes and macrophages were cocultured in a contact system as previously described (23). Briefly, RAW264.7 macrophages (2.5 × 105 cells/well) were plated into dishes with serum-starved and hypertrophied 3T3-L1 adipocytes. The coculture was incubated in serum-free DMEM for 24 h. RAW264.7 macrophages and 3T3-L1 adipocytes were cultured separately under the same conditions for contrast. Different concentrations of ISE were administrated meanwhile as that of coculture. The supernatants were collected and stored at −20°C until further measurements. At the same time, RAW264.7 macrophages were seeded in 24-well plates (2.5 × 105 cells/well) and treated with either 1 μg/mL LPS or 3T3-L1 adipocyte conditioned medium (L1CM) for 30 min or 24 h with different concentrations of ISE. The hypertrophied 3T3-L1 adipocytes were cultured in serum-free medium for 12 h, and then collected the supernatants and designated as the L1CM and stored at −20°C until use. As for the effect of ISE on the viability of RAW264.7 macrophages, RAW264.7 were transferred into 96-well plates at 1 × 104 cells/well and incubated with ISE (final concentrations: 0.5, 1.0, 2.0, and 4.0 μg/mL) for 24 h. The wells were incubated in the absence of ISE as the control group and CCK-8 (Dojindo, Kumamoto, Japan) was added to each well for cell staining for another 4 h according to the manufacturer's instruction. The cell viability was calculated as a percentage of control, which was considered as 100%, according to the formula: (A sample-A blank)/(A control-A blank) × 100. Plates were read at OD450 in a microplate reader (BIO-TEK Instruments, Winooski, VT, USA).
Measurement of MCP-1, TNF-α, IL-6, and NO
Cell-free supernatants were collected to determine the MCP-1 (Biolegend, San Jose, CA), TNF-α, and IL-6 (both from eBioscience, San Diego, CA) using ELISA kit assay. In addition, nitrite as the end-point of NO generation from activated macrophages was measured by determining NO2-concentration in the culture supernatant. Briefly, add 100 μL of Griess reagent (1% sulfanilamide and 0.1% N-[1-naphthyl]-ethylenediamine dihydrochloride in 5% phosphoric acid) into 100 μL samples of medium. The concentration of NO2 was calculated by extrapolating a NaNO2 standard curve.
Immunoblotting
After being pretreated with ISE, RAW264.7 macrophages were stimulated with LPS or L1CM for 30 min, and then harvested in RIPA lysis buffer containing 50 mM Tris-HCl (pH 7.4), 150 mM NaCl, 1% NP40, 1 × protease inhibitor cocktail (Roche Applied Science, Indianapolis, IN), and 1 × phosphatase inhibitor cocktail (Sigma-Aldrich). Proteins were resolved in 7.5% acrylamide gels and then transferred to nitrocellulose membranes. The membrane was blocked with 5% non-fat milk in Tris-buffered saline before being incubated, respectively with specific primary antibodies for the following proteins: IκB-α (1:1000), phosphor-p44/p42 (Thr202/Tyr204) (p-ERK) (1:1000), phosphor-p38 (Thr202/Tyr204) (p-p38) (1:1000), and Phospho-SAPK/JNK (Thr183/Tyr185) (p-JNK) (1:1000), phosphor-STAT3 (p-STAT3) (1:1000), ERK (1:1000), JNK (1:1000), p38 (1:1000), STAT3 (1:1000) (all from Cell Signaling Technologies, Danvers, MA), and β-actin (1:5000, Sigma-Aldrich). The membranes were next incubated with horseradish peroxides (HRP)-conjugated secondary antibodies followed by exposure to enhanced chemiluminescent reagents (Millipore, Burlington, MA).
Macrophage Migration Assay
Migration assays were performed using Transwell inserts with a 5 μm membrane pore size (Corning, NY). The L1CM from fully differentiated 3T3-L1 adipocytes was transferred to 24 well plates containing inserts. The RAW264.7 macrophages were pre-incubated with or without ISE for 1 h. Then, the RAW264.7 macrophages were seeded onto the inserts at 5 × 104 cells/well. After migrating for 4 h at 37°C, any non-migrated cells were wiped off with a cotton swab, and the cells adhering to the underside of the membrane were fixed with 4% paraformaldehyde for 20 min. The membranes were then washed with PBS. The number of migrated cells in twelve random microscopy fields per membrane was counted at 400 × magnification.
Statistical Analysis
All data were presented as the means ± SD. The statistical significance of any difference in each parameter among treatment was evaluated by one-way analysis of variance (ANOVA) followed by Tukey's test using Prism 6.0 software. Significance level was set at P < 0.05.
Results
ISE Inhibited the Production of Inflammation Mediators Released by LPS-Activated RAW264.7 Macrophages
To investigate whether ISE affects the production of pro-inflammatory mediators from macrophages in obesity-related environments, RAW264.7 cells were treated with different doses of ISE in the presence or absence of LPS. We first determined the effect of ISE at different doses on cell viability. ISE at 4.0 μg/mL, not 0.5, 1.0 and 2.0 μg/mL, used according to the anti-oxidant activity of ISE as previously described (22), had the toxicity on cell viability (Figure 1). Thus, we used ISE concentrations at 0.5, 1.0, and 2.0 μg/mL in the subsequent experiments to determine the working mechanisms of ISE.
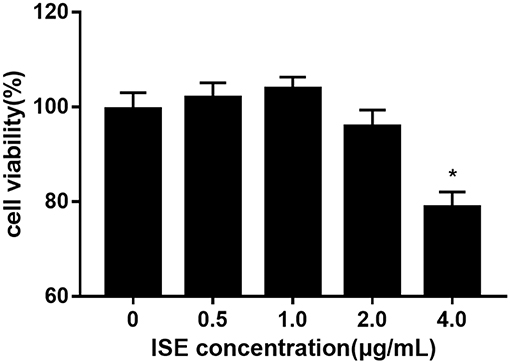
Figure 1. Cell viability of RAW264.7 macrophages pretreated with different concentrations of ISE. RAW264.7 macrophages were incubated with ISE in the indicated concentrations for 24 h and cell viability was determined using CCK-8 kit as described in “Materials and Methods” section. The values are means ± SD, n = 6, *P < 0.01.
Next we determined the effect of ISE on inflammation mediators produced by LPS-activated RAW264.7 macrophages, which are M1 macrophages induced by LPS or pro-inflammatory mediators and produce significant amounts of pro-inflammatory cytokines, such as TNF-α, IL-6, and NO (24, 25). ISE administration decreased NO production in a dose-dependent manner (IC50 = 0.52 μg/mL) (Figure 2A). In addition, the secretion of TNF-α (IC50 = 2.22 μg/mL) (Figure 2B), and IL-6 (IC50 = 0.43 μg/mL) (Figure 2C) were inhibited dose-dependently by ISE administration in LPS-activated macrophages. However, ISE only reduced the MCP-1 secretion (IC50 = 2.24 μg/mL) at 2.0 μg/mL (Figure 2D).
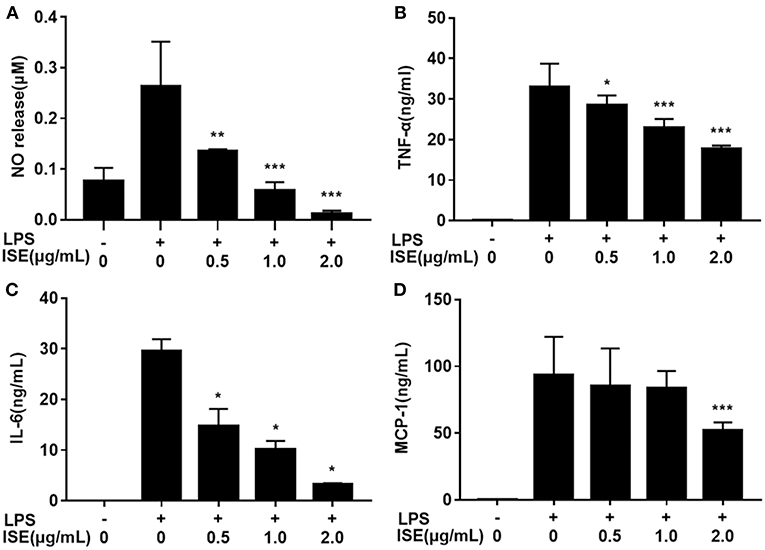
Figure 2. Effects of ISE on RAW264.7 macrophage activation by LPS. RAW264.7 macrophages were pretreated with different concentrations of ISE for 1 h and then were incubated with LPS at 1.0 μg/mL for 24 h. Cell-free supernatants were collected to determine the concentrations of NO (A), TNF-α (B), IL-6 (C), and MCP-1 (D) as described in “Materials and Methods” section. The values are means ± SD of 6 samples. Statistical comparisons were made with each vehicle control. *P < 0.05, **P < 0.01, ***P < 0.001.
ISE Reduced the Level of Inflammation Mediators Released by RAW264.7 Macrophage Stimulated by 3T3-L1 Adipocyte Conditioned Medium
To investigate whether ISE affected the secretion of pro-inflammatory cytokines from macrophages in obesity-related environments, RAW264.7 macrophages were co-treated with ISE and L1CM to induce an obesity-related inflammatory reaction. Pro-inflammatory mediators NO (Figure 3A), TNF-α (Figure 3B), IL-6 (Figure 3C), and MCP-1 (Figure 3D) production apparently increased in L1CM-activated models (IC50 = 0.84, 0.82, and 1.40 μg/mL, respectively). In the model with L1CM, the suppressive property of ISE was observed as NO, TNF-α, and IL-6 in a dose-dependent manner. In accordance with MCP-1 released by LPS-activated macrophages, only ISE at 2.0 μg/mL decreased MCP-1 production (IC50 = 1.62 μg/mL).
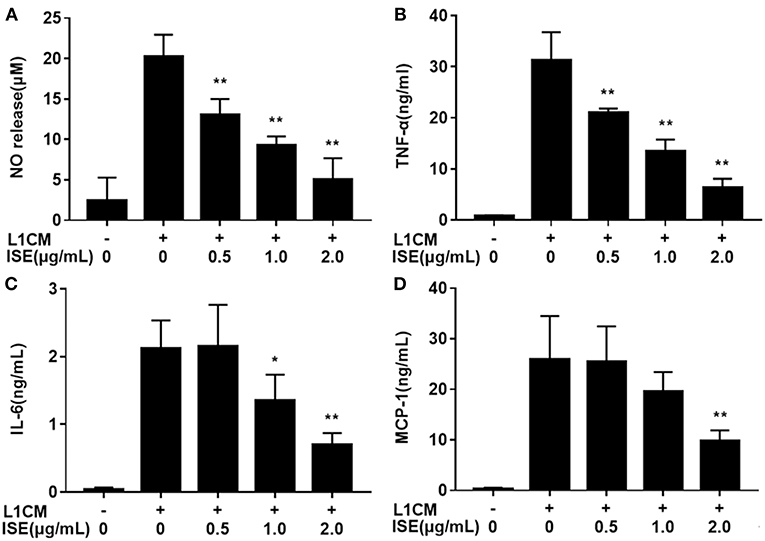
Figure 3. Effects of ISE on inflammation in RAW264.7 macrophages activated by conditioned medium of hypertrophied 3T3-L1 adipocytes. RAW264.7 macrophages were pretreated with different concentrations of ISE for 1 h and then were incubated with conditioned medium of hypertrophied 3T3-L1 adipocytes (L1CM) for 24 h. Cell-free supernatants were collected to determine the concentrations of NO (A), TNF-α (B), IL-6 (C), and MCP-1 (D) as described in “Materials and Methods” section. The values are means ± SD of 6 samples. Statistical comparisons were made with each vehicle control. *P < 0.05, **P < 0.01.
ISE Administration Decreased the Pro-Inflammatory Cytokine Level in Cocultures of 3T3-L1 Adipocytes and RAW264.7 Macrophages
We next examined whether ISE treatment could suppress inflammatory changes in a coculture system composed of 3T3-L1 adipocytes and RAW264.7 macrophages. In this model, 3T3-L1 adipocytes or RAW264.7 macrophages were cultured separately and treated with ISE by the same method that we used in the coculture system. As shown in Figure 4, ISE treatment dose-dependently suppressed the production of NO (IC50 = 1.55 μg/mL) (Figure 4A), TNF-α (IC50 = 0.80 μg/mL) (Figure 4B) and IL-6 (Figure 4C) (IC50 = 2.30 μg/mL), but did not affect MCP-1 (Figure 4D) in the coculture system. These data indicate that ISE treatment has the ability to block the release of inflammatory mediators in the coculture system.
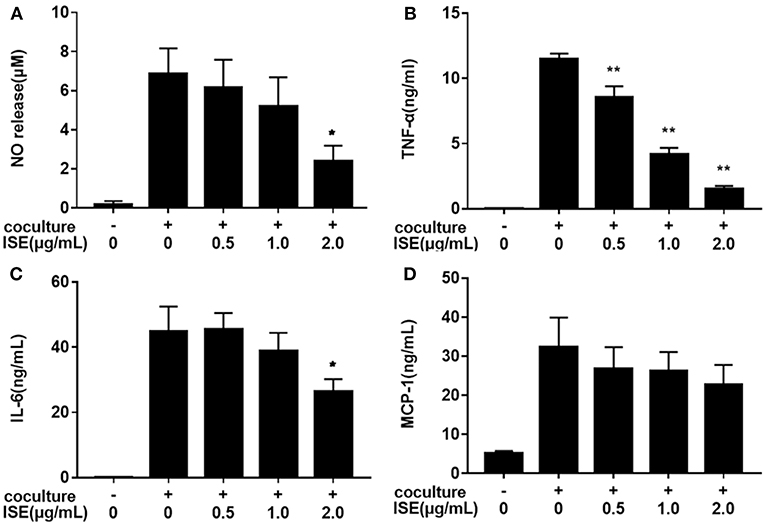
Figure 4. Effects of ISE on inflammation in RAW264.7 macrophages cocultured with differentiated 3T3-L1 adipocytes. RAW264.7 macrophages were pretreated with different concentrations of ISE for 1 h and then were incubated with differentiated 3T3-L1 adipocytes for 24 h. Cell-free supernatants were collected to determine the concentrations of NO (A), TNF-α (B), IL-6 (C), and MCP-1 (D) as described in “Materials and Methods” section. The values are means ± SD of 6 samples. Statistical comparisons were made with each vehicle control. *P < 0.05, **P < 0.01.
The Anti-inflammatory Effects of ISE Were Caused by Inhibition of MAPK and STAT Activity
TLR4 is the main pathway for stimulating inflammatory responses. To investigate the molecular mechanism of the anti-inflammatory effects of ISE, we examined TLR4 signaling pathway in RAW264.7 macrophages.
TLR4 signaling stimulated by LPS or L1CM results in the activation of NF-κB and MAPK family including ERK, JNK, and p38 MAPK, which drive the upregulation of pro-inflammatory genes (26, 27). To characterize the mechanism underlying the suppression of pro-inflammatory mediators by ISE, we used Western blotting to measure the phosphorylation of ERK, JNK, p38, and the degradation of I-κB-α. Stimulation with LPS or L1CM increased the phosphorylation of ERK (Figures 5A, 6A), JNK (Figures 5B, 6B), p38 (Figures 5C, 6C), and enhanced the degradation of I-κB-α (Figures 5D, 6D). While ERK and p38 activation were significantly suppressed by ISE, the phosphorylation of JNK was not impacted by ISE in LPS- or L1CM- activated macrophages. Furthermore, ISE treatment could not prevent the degradation of I-κB-α, which suggests that NF-κB signals may not be involved in the inhibitory effects of ISE on pro-inflammatory mediator release.
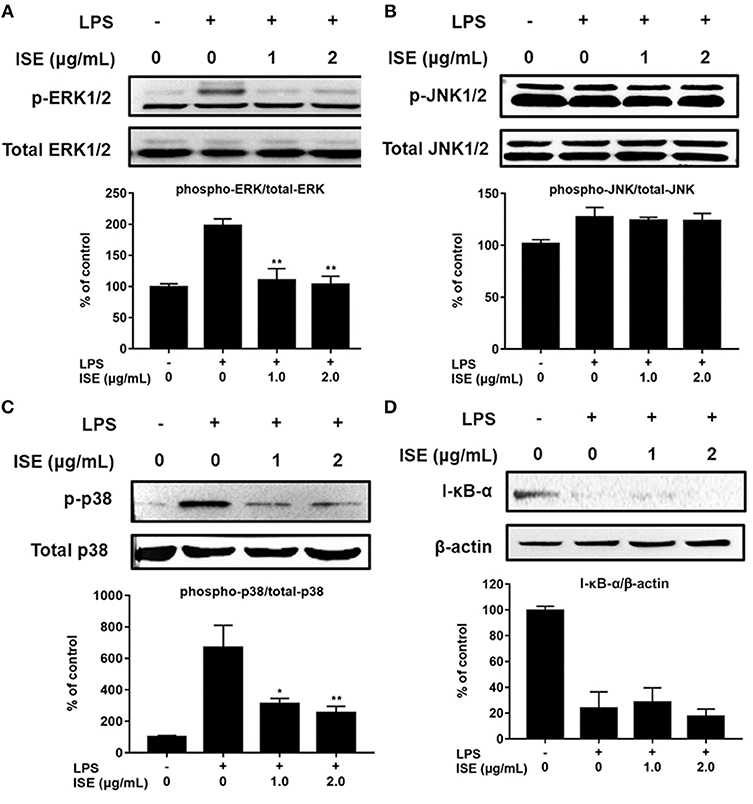
Figure 5. Effects of ISE on LPS-induced MAPK and NF-κB signals. RAW264.7 macrophages were pretreated with different concentrations of ISE for 1 h and then stimulated by 1.0 μg/mL LPS for 30 min. Total cell lysates were extracted, and then western blotting using specific antibodies was used for the detection of phosphorylated and total forms of three MAPK molecules, ERK (A), JNK (B), p38 (C), and I-κB-α (D). The value of a control was set at 100%, and the relative value was presented as fold induction to that of the control, which was normalized to total MAPK or β-actin. The values are means ± SD, n = 3. Statistical comparisons were made with each vehicle. *P < 0.01, **P < 0.001.
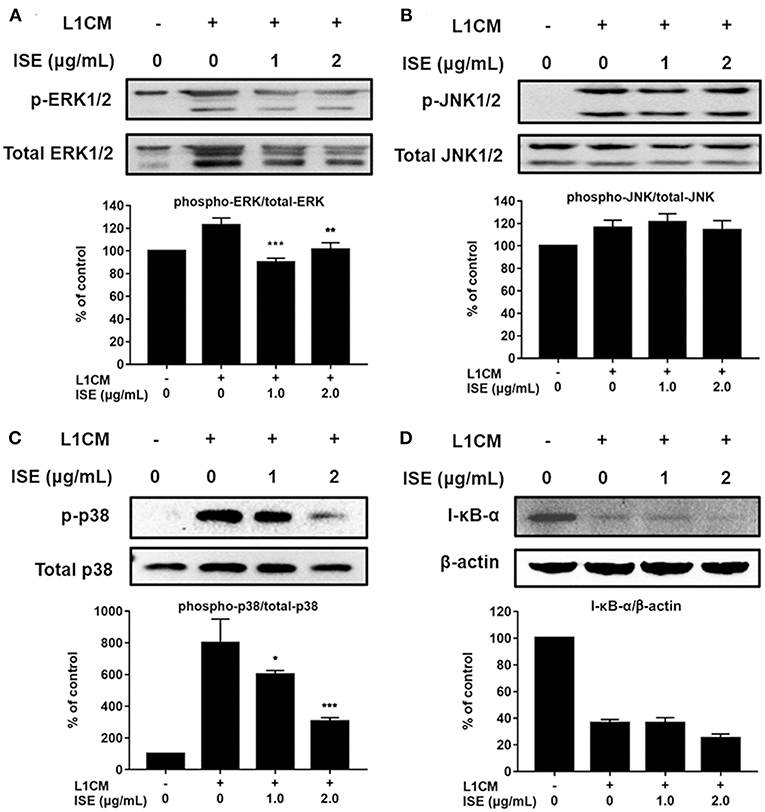
Figure 6. Effects of ISE on 3T3L1 CM-induced MAP kinase and NF-κB signals. RAW264.7 macrophages were pretreated with different concentrations of ISE for 1 h and then stimulated by 3T3-L1 CM (L1CM) for 30 min. Total cell lysates were extracted to detect the levels of phosphorylated ERK (A), JNK (B), p38 (C), and I-κB-α (D). The value of a control was set at 100%, and the relative value was presented as fold induction to that of the control, which was normalized to total ERK, JNK, p38 or β-actin. The values are means ± SD, n = 3. Statistical comparisons were made with each vehicle controls. *P < 0.05, **P < 0.01, ***P < 0.001.
STAT signals are also important transcriptional activators that regulate cytokine transcription (28). Inflammatory cytokine IL-6 is one of the active mediators of STAT3, which transmits signals through its receptor IL-6 and activates Janus kinase, which in turn activates the STAT family, including the phosphorylation and activation of STAT3 (29). To further explore the effect of ISE on the decrease of pro-inflammatory mediators through JAK/STAT3 signaling pathway in the obesity-related environment, we measured the protein expression level of p-STAT3 and STAT3 in LPS (Figure 7A) and L1CM (Figure 7B), respectively. Data showed that the phosphorylation of STAT3 was markedly suppressed by ISE in LPS- and L1CM- activated models.
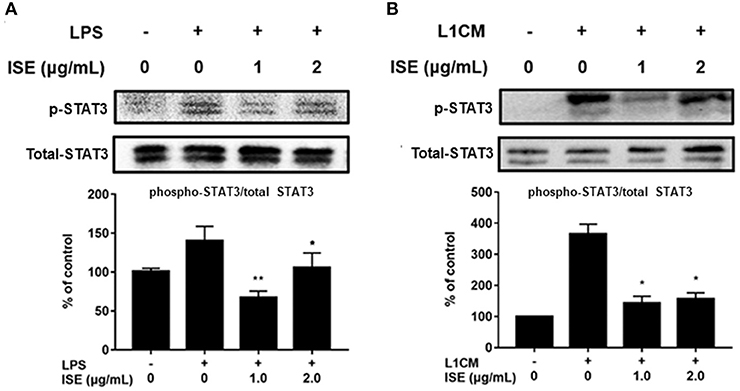
Figure 7. Effects of ISE on STAT3 signal. RAW264.7 macrophages were pretreated with different concentrations of ISE for 1 h and then stimulated by 1.0 μg/mL LPS or 3T3-L1 CM for 30 min. Total cell lysates were extracted, and then western blotting using specific antibodies was used to determine the expression of p-STAT3 and STAT3 in LPS (A) and 3T3-L1 CM (L1CM) stimulation (B), respectively. The value of a control was set at 100%, and the relative value was presented as fold induction to that of the control, which was normalized to total STAT3. Statistical comparisons were made with each vehicle controls. The values are means ± SD, n = 3. *P < 0.05, **P < 0.01.
ISE Blocked the LICM-Stimulated Migration of RAW264.7 Macrophages
To examine the potential of ISE to limit the motility of macrophages, RAW264.7 macrophages were preincubated with different concentrations of ISE ahead of L1CM incubation and compared with cells incubated with L1CM alone. As shown in Figure 8, L1CM induced an obvious migration of macrophages. While this process was suppressed by ISE.
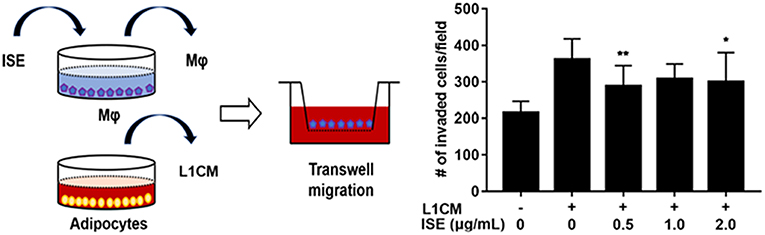
Figure 8. Effects of ISE on macrophage motility. (Left) Procedures of the macrophage migration experiments. RAW 264.7 macrophages were treated with vehicle or ISE (0.5, 1.0, 2.0 μg/mL) for 1 h and the detached cells were used for migration assay in the presence of DMEM or L1CM. (Right) Migrated RAW264.7 macrophages were quantified as described in “Materials and Methods” section. The values are means ± SD, n = 12. *P < 0.05, **P < 0.01. Mφ, RAW264.7 macrophages.
Discussion
I. sanghuang, as a popular medicinal polypore used throughout Southeast Asia, was reported diffusely for its effects of anti-oxidant, anti-tumor, anti-microbial, lipid-lowering effect (30). Evidence has shown that various ingredients of I. sanghuang, such as polysaccharides (18, 31), had anti-inflammatory activity. However, the anti-inflammatory activity of polyphenols extracted from I. sanghuang has not been reported. The present study showed that ISE polyphenols directly inhibits pro-inflammatory cytokines NO, TNF-α, and IL-6 released by RAW264.7 macrophages activated by LPS, L1CM, and cocultured with differentiated 3T3-L1 adipocytes. These effects might be mediated via suppressing p-ERK- and p-p38- MAPK and STAT3 signaling pathways (Figure 9). These data indicate that ISE polyphenols have the ability to interfere the crosstalk between macrophages and adipocytes and inhibit obesity-induced adipose inflammation.
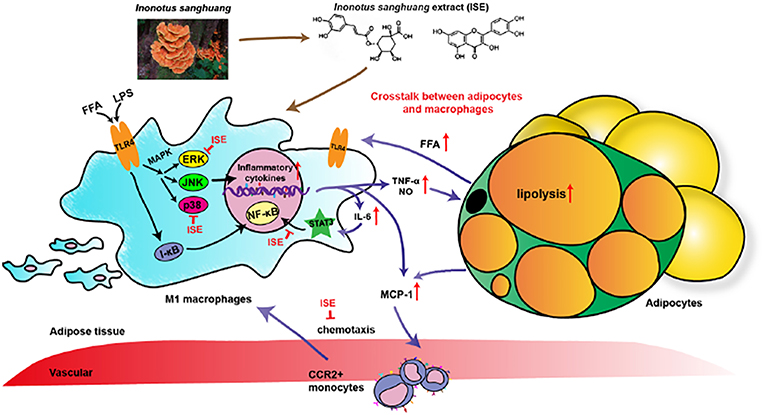
Figure 9. Schematic models of molecular targets affected by ISE to attenuate inflammatory signaling pathways. On the one hand, LPS- and free fatty acid (FFA)-induced inflammatory responses are regulated by both NF-κB and MAPK signaling pathways. In activated macrophages, ISE decreases pro-inflammatory cytokine production via inhibiting the ERK and p38 MAPK pathways, not NF-κB signals. On the other hand, ISE markedly suppresses the phosphorylation of STAT3 to reduce cytokines transcription. Subsequently, the lipolysis of adipocytes is suppressed and proinflammatory M1 macrophages are less recruited via the MCP-1-CCR2 pathway. And the decrease of inflammatory cytokine IL-6 further restrains the IL-6/STAT3 activation and the inflammatory mediator production. In a word, treatment with ISE reduces the levels of these pro-inflammatory mediators, thereby disrupting the crosstalk between macrophages and adipocytes in a coculture. ISE apparently exerted anti-inflammatory ability, possibly diminishing the obesity-induced inflammatory diseases.
It's beyond dispute that obesity-induced adipose inflammation by paracrine interactions between adipocytes and adipose-infiltrating macrophages plays a causative role in insulin resistance and metabolic dysfunction in obesity and is characterized by abnormal secretion of pro-inflammatory cytokines in white adipose tissue (32). On the one hand, adipose tissue promotes macrophages to secrete inflammatory mediators by releasing FFA and to accumulate with chemotaxis in adipose tissue by releasing MCP-1. On the other hand, inflammatory cytokines from ATMs could accelerate the secretion of FFA from adipose tissue. In other words, macrophages markedly infiltrate into obese adipose tissue and establish an inflammatory paracrine loop with adipocytes. From what has been discussed above, it's expected to reduce the chronic low-grade inflammatory response in obesity by cutting off the interaction between macrophages and adipocytes mediated by reducing the secretion of pro-inflammatory mediators.
In vitro, coculture of highly differentiated 3T3-L1 adipocytes and RAW264.7 macrophages has been used as the model of adipose inflammation in which pro-inflammatory cytokine genes and proteins such as MCP-1, IL-6, and TNF-α are significantly upregulated (33). Several polyphenols have been reported to be able to reduce secretion of pro-inflammatory indicators in the coculture model (34, 35). However, ISE polyphenols have not been tested. Additionally, TLR4, the receptor of LPS, which could activate the NF-κB and MAPK signaling pathways, is expressed in various cells, including adipocytes and macrophages (36, 37). It can be activated by LPS or endogenous saturated FFA and initiate potent downstream inflammatory responses in obese adipose tissue (12). Furthermore, this is supported by a study showing that TLR4 deficiency can prevent insulin resistance in lipid-infused male mice (37). Thus, inhibition of TLR4 signaling has been shown as an attractive therapeutic strategy for treatment of obesity-induced insulin resistance and adipocytes mediated chronic inflammation.
In this study, ISE suppressed production of pro-inflammatory mediators in LPS- or L1CM-activated RAW264.7 macrophages and a coculture system with 3T3-L1 adipocytes and RAW264.7 macrophages. We found that ISE dose-dependently may directly suppress production of NO, TNF-α, IL-6, and MCP-1 (IC50 = 0.52, 2.22, 0.43, and 2.24 μg/mL, respectively) in the single culture of RAW264.7 macrophages activated by LPS. LPS from gram-negative intestinal microbiota is an early factor recruiting a signals through TLR4 to induce secretion of pro-inflammatory cytokines and then triggering metabolic diseases induced by a high-fat diet (38, 39). Our and these data suggest that ISE may have beneficial effects on obesity-related diseases. Current data supported that the production of pro-inflammatory mediators NO, TNF-α, IL-6, and MCP-1 (IC50 = 0.84, 0.82, 1.40, and 1.62 μg/mL, respectively) could be significantly suppressed by ISE in L1CM-activated RAW264.7 macrophages in a dose-dependent manner. It has been reported that FFA secreted from the L1CM which is derived from hypertrophied adipocytes is the key factor to enhance the expression of TNF-α in ATMs (40). Thus, these data indicate that 3T3-L1 CM could activate RAW264.7 macrophages to induce inflammatory response possibly via adipocytes secreting FFA and other cytokines. Moreover, our data suggest that ISE might be able to reduce pro-inflammatory mediators NO, TNF-α, and IL-6 (IC50 = 1.55, 0.80, and 2.30 μg/mL, respectively) and then promote inflammatory changes in obese adipose tissue. However, whether it is true needs to be investigated. In addition, ISE treatment failed to promote changes in MCP-1 in the coculture system. It's well known that MCP-1 plays an important role in both potently recruiting macrophages into adipose tissue and determining a more inflammatory M1 macrophage phenotype (41), suggesting that MCP-1 is secreted by 3T3-L1 adipocytes from the most part rather than by RAW264.7 macrophages. Thus, we speculate that anti-inflammatory effects of ISE might have more impact on the activated macrophages in adipose tissue. Finally, we noted that among different activated macrophages, the mean IC50 values of ISE toward inflammatory mediators NO, TNF-α and IL-6 was at least 2.0 μg/mL less than the other mediator MCP-1, suggesting that the inhibitory effects of ISE on the interaction between adipocytes and macrophages might mainly be caused by suppressing pro-inflammatory mediators.
Previous studies have shown that some species of sanghuang exert anti-inflammatory effects through inhibiting the signals of NF-κB and MAPKs (e.g., ERK and p38) (42–44). In addition, polyphenols have been demonstrated to suppress inflammatory responses by blocking the ERK and NF-κB pathways in microglia and inhibit production of pro-inflammatory cytokines TNF-α, IL-1β, and IL-6 (45). Current study found that ISE could down-regulate MAPKs, not NF-κB signaling triggered by L1CM-activated macrophages. Notably, activation of ERK and p38, not JNK, was inhibited by ISE, and these two are considered to be the major mechanism that accounts for the suppression of pro-inflammatory mediator secretion by ISE.
Polyphenols are the secondary metabolites of mushrooms in the genus Inonotus. In the current study, 6 polyphenols such as quercetin, rutin, quercitrin, icarisid II, isorhamnetin and chlorogenic acid are contained in ISE which could exert anti-oxidant, anti-proliferative, and anti-microbial activities in vitro (22). Quercetin has been shown to be able to inhibit the MAPK signals in adipocytes, macrophages, lipid accumulation and obesity-induced inflammation in the animal models (46). Although rutin has been reported to have no effect on lipid accumulation in 3T3-L1 adipocytes, it can attenuate obesity through activating brown fat and then increasing energy expenditure (47). Furthermore, quercitrin (48), Icaritin II (49), isorhamnetin (50), and chlorogenic acid (51) have also been reported to have anti-inflammatory activities in macrophages or animal models. These reports and current data indicate that the attenuation of ISE on inflammatory response in macrophages or crosstalk between macrophages and adipocytes be mediated, at least in part by I. sanghuang polyphenols. However, which compound is involved in this effect needs to be investigated.
In this study, we also demonstrated that ISE inhibited STAT3 phosphorylation, as one of the pathways to suppress production of pro-inflammatory mediators in activated macrophages. Polyphenols could reduce production of inflammatory factors via suppressing IL-6/STAT3 signaling pathway (52, 53). In addition, polyphenols treatment could prevent the pro-inflammatory effect on macrophages by potentially inhibiting STAT3 activation (54). Since ISE treatment could inhibit IL-6 released by macrophages activated by LPS or L1CM, we speculate that ISE might have the ability to affect IL-6/STAT3 signals. As expected, the activation of STAT3 was markedly inhibited by ISE. Therefore, our data suggest that the anti-inflammatory effect of ISE may be mediated, not only by inhibiting Erk and p38 MAPK signaling, but also by affecting the reduction of IL-6 and inhibiting IL-6/STAT3 signaling pathway. In addition, whether ISE administration has benefits in obesity-related diseases and the underlying mechanism need to be investigated.
As described above, activated macrophages infiltrating into adipose tissue are critical source of pro-inflammatory mediators, which subsequently cause systemic insulin resistance and obesity-related complications. Therefore, preventing the infiltration of macrophages into adipose tissue could ameliorate obesity-related inflammation and provide a therapeutic target for obesity-related complications. However, the mechanisms that initiate macrophages recruitment to adipose tissue remain not fully elucidated, but it presumably involves increased secretion of chemotactic factors, in particular chemokine MCP-1 secreted from adipocytes (55). This study gives rise to the question of how ISE prevents macrophages migration, while we couldn't supply an immediate answer to this important question at present. Our data showed that ISE didn't inhibit secretion of MCP-1 from cocultures; in other words, ISE presumably inhibits macrophage migration by acting on macrophages rather than adipocytes. Previous studies have demonstrated that MCP-1 induces macrophage migration largely via MAPKs signaling (56). In addition, LPS increases macrophage motility (57), suggesting the possibility that ISE could impair the ability of macrophages to migrate in response to MCP-1 by inhibiting TLR4 activity. Researchers have also found that polyphenols from tea could significantly suppress proliferation, migration, and invasion in melanoma by TLR4 inhibition (58). Moreover, macrophages treated with butein (a polyphenol of vegetal origin) exhibited a reduced ability to migrate toward 3T3-L1 CM (59). According to the above, this study implies that ISE at higher concentrations has the potential to block ATMs recruitment in the adipose tissue by TLR4 or any other signaling pathways inhibition, which needs to be clarified further.
In conclusion, we demonstrated that ISE has the ability to inhibit the crosstalk between adipocytes and macrophages, mediated by attenuating inflammatory responses produced by macrophages (Figure 9). Our results suggest that ISE polyphenols may be valuable as a functional medicinal food in terms of ameliorating chronic inflammation in obese adipose tissue and improving obesity-related insulin resistance and complications.
Author Contributions
JW, MZ, WP, and YZ contributed to the design of the study. MZ, YX, XS, and KL, performed the experiments. MZ analyzed the data. MZ and JW wrote or critically revised the manuscript. All authors read and approved the final manuscript.
Conflict of Interest Statement
The authors declare that the research was conducted in the absence of any commercial or financial relationships that could be construed as a potential conflict of interest.
Acknowledgments
This work was supported by the National Natural Science Foundation of China (81402677), the Natural Science Foundation of Henan Province (182300410325), the Projects of Henan Science and Technology Development (172102410036, 162300410116, and 172102310151), the Science Research program for Colleges and Universities of Hebei Province (ZD2018067), and the Key Project of Henan Education Committee (16A320037).
Supplementary Material
The Supplementary Material for this article can be found online at: https://www.frontiersin.org/articles/10.3389/fimmu.2019.00286/full#supplementary-material
References
1. Afshin A, Forouzanfar M, Reitsma M, Sur P, Estep K, Lee A, et al. Health effects of overweight and obesity in 195 countries over 25 years. New Engl J Med. (2017) 377:13–27. doi: 10.1056/NEJMoa1614362
2. Winter J, Wuppermann A. Do they know what is at risk? health risk perception among the obese. Health Econ. (2014) 23:564–85. doi: 10.1002/hec.2933
3. Tchernof A, Despres JP. Pathophysiology of human visceral obesity: an update. Physiol Rev. (2013) 93:359–404. doi: 10.1152/physrev.00033.2011
4. Phillips CM. Metabolically healthy obesity: definitions, determinants and clinical implications. Rev Endocr Metab Disord. (2013) 14:219–27. doi: 10.1007/s11154-013-9252-x
5. Engin A. The pathogenesis of obesity-associated adipose tissue inflammation. Adv Exp Med Biol. (2017) 960:221–45. doi: 10.1007/978-3-319-48382-5_9
6. Xu H, Barnes GT, Yang Q, Tan G, Yang D, Chou CJ, et al. Chronic inflammation in fat plays a crucial role in the development of obesity-related insulin resistance. J Clin Invest. (2003) 112:1821–30. doi: 10.1172/JCI200319451
7. Russo L, Lumeng CN. Properties and functions of adipose tissue macrophages in obesity. Immunology (2018) 155:407–17. doi: 10.1111/imm.13002
8. Gordon S, Taylor PR. Monocyte and macrophage heterogeneity. Nat Rev Immunol. (2005) 5:953–64. doi: 10.1038/nri1733
9. Lumeng CN, DelProposto JB, Westcott DJ, Saltiel AR. Phenotypic switching of adipose tissue macrophages with obesity is generated by spatiotemporal differences in macrophage subtypes. Diabetes (2008) 57:3239–46. doi: 10.2337/db08-0872
10. Tsukumo DM, Carvalho-Filho MA, Carvalheira JB, Prada PO, Hirabara SM, Schenka AA, et al. Loss-of-function mutation in toll-like receptor 4 prevents diet-induced obesity and insulin resistance. Diabetes (2007) 56:1986–98. doi: 10.2337/db06-1595
11. Ota T. Chemokine systems link obesity to insulin resistance. Diabetes Metab J. (2013) 37:165–72. doi: 10.4093/dmj.2013.37.3.165
12. Nguyen MT, Favelyukis S, Nguyen AK, Reichart D, Scott PA, Jenn A, et al. A subpopulation of macrophages infiltrates hypertrophic adipose tissue and is activated by free fatty acids via toll-like receptors 2 and 4 and jnk-dependent pathways. J Biol Chem. (2007) 282:35279–92. doi: 10.1074/jbc.M706762200
13. Suganami T, Tanaka M, Ogawa Y. Adipose tissue inflammation and ectopic lipid accumulation. Endocr J. (2012) 59:849–57. doi: 10.1507/endocrj.EJ12-0271
14. Wang Y-Z, Zhang J, Zhao Y-L, Li T, Shen T, Li J-Q, et al. Mycology, cultivation, traditional uses, phytochemistry and pharmacology of wolfiporia cocos (schwein.) ryvarden et gilb.: a review. J Ethnopharmacol. (2013) 147:265–76. doi: 10.1016/j.jep.2013.03.027
15. Zhao Y-Y. Traditional uses, phytochemistry, pharmacology, pharmacokinetics and quality control of polyporus umbellatus (pers.) fries: a review. J Ethnopharmacol. (2013) 149:35–48. doi: 10.1016/j.jep.2013.06.031
16. Han J-G, Hyun M-W, Kim CS, Jo JW, Cho J-H, Lee K-H, et al. Species identity of phellinus linteus (sanghuang) extensively used as a medicinal mushroom in Korea. J Microbiol. (2016) 54:290–95. doi: 10.1007/s12275-016-5520-2
17. Xu X, Li J, Hu Y. Polysaccharides from inonotus obliquus sclerotia and cultured mycelia stimulate cytokine production of human peripheral blood mononuclear cells in vitro and their chemical characterization. Int Immunopharmacol. (2014) 21:269–78. doi: 10.1016/j.intimp.2014.05.015
18. Friedman M. Mushroom polysaccharides: chemistry and antiobesity, antidiabetes, anticancer, and antibiotic properties in cells, rodents, and humans. Foods (2016) 5:80. doi: 10.3390/foods5040080
19. Chen H, Tian T, Miao H, Zhao Y-Y. Traditional uses, fermentation, phytochemistry and pharmacology of phellinus linteus: a review. Fitoterapia (2016) 113:6–26. doi: 10.1016/j.fitote.2016.06.009
20. Hu H, Zhang Z, Lei Z, Yang Y, Sugiura N. Comparative study of antioxidant activity and antiproliferative effect of hot water and ethanol extracts from the mushroom inonotus obliquus. J Biosci Bioengg. (2009) 107:42–8. doi: 10.1016/j.jbiosc.2008.09.004
21. Yeh CH, Yang JJ, Yang ML, Li YC, Kuan YH. Rutin decreases lipopolysaccharide-induced acute lung injury via inhibition of oxidative stress and the mapk-Nf-Kappab pathway. Free Radic Biol Med. (2014) 69:249–57. doi: 10.1016/j.freeradbiomed.2014.01.028
22. Liu K, Xiao X, Wang J, Chen CYO, Hu H. Polyphenolic composition and antioxidant, antiproliferative, and antimicrobial activities of mushroom inonotus sanghuang. LWT Food Sci Tech. (2017) 82:154–61. doi: 10.1016/j.lwt.2017.04.041
23. Lin S, Hirai S, Goto T, Sakamoto T, Takahashi N, Yano M, et al. Auraptene suppresses inflammatory responses in activated raw264 macrophages by inhibiting P38 mitogen-activated protein kinase activation. Mol Nutr Food Res. (2013) 57:1135–44. doi: 10.1002/mnfr.201200611
24. Lee DH, Ghiasi H. Roles of M1 and M2 macrophages in herpes simplex virus 1 infectivity. J Virol. (2017) 91:e00578–17. doi: 10.1128/JVI.00578-17
25. Gordon S, Martinez FO. Alternative activation of macrophages: mechanism and functions. Immunity (2010) 32:593–604. doi: 10.1016/j.immuni.2010.05.007
26. Rossol M, Heine H, Meusch U, Quandt D, Klein C, Sweet MJ, et al. Lps-induced cytokine production in human monocytes and macrophages. Crit Rev Immunol. (2011) 31:379–446. doi: 10.1615/CritRevImmunol.v31.i5.20
27. Könner AC, Brüning JC. Toll-like receptors: linking inflammation to metabolism. Trends Endocrin Met. (2011) 22:16–23. doi: 10.1016/j.tem.2010.08.007
28. Liu Z, Gan L, Zhou Z, Jin W, Sun C. Socs3 promotes inflammation and apoptosis via inhibiting Jak2/Stat3 signaling pathway in 3t3-L1 adipocyte. Immunobiology (2015) 220:947–53. doi: 10.1016/j.imbio.2015.02.004
29. Ju A, Cho YC, Cho S. Methanol extracts of Xanthium sibiricum roots inhibit inflammatory responses via the inhibition of nuclear factor-kappab (Nf-Kappab) and signal transducer and activator of transcription 3 (Stat3) in murine macrophages. J Ethnopharmacol. (2015) 174:74–81. doi: 10.1016/j.jep.2015.07.038
30. Ma L, Chen H, Dong P, Lu X. Anti-inflammatory and anticancer activities of extracts and compounds from the mushroom inonotus obliquus. Food Chem. (2013) 139:503–8. doi: 10.1016/j.foodchem.2013.01.030
31. Fan L, Ding S, Ai L, Deng K. Antitumor and immunomodulatory activity of water-soluble polysaccharide from inonotus obliquus. Carbohydr Polym. (2012) 90:870–4. doi: 10.1016/j.carbpol.2012.06.013
32. Nishimura S, Manabe I, Nagasaki M, Seo K, Yamashita H, Hosoya Y, et al. In vivo imaging in mice reveals local cell dynamics and inflammation in obese adipose tissue. J Clin Invest. (2008) 118:710–21. doi: 10.1172/JCI33328
33. Mori K, Ouchi K, Hirasawa N. The anti-inflammatory effects of lion's mane culinary-medicinal mushroom, hericium erinaceus(higher basidiomycetes) in a coculture system of 3t3-L1 adipocytes and raw264 macrophages. Int J Med Mushrooms (2015) 17:609–18. doi: 10.1615/IntJMedMushrooms.v17.i7.10
34. Reyes-Farias M, Vasquez K, Ovalle-Marin A, Fuentes F, Parra C, Quitral V, et al. Chilean native fruit extracts inhibit inflammation linked to the pathogenic interaction between adipocytes and macrophages. J Med Food. (2015) 18:601–8. doi: 10.1089/jmf.2014.0031
35. Sakurai T, Kitadate K, Nishioka H, Fujii H, Kizaki T, Kondoh Y, et al. Oligomerized grape seed polyphenols attenuate inflammatory changes due to antioxidative properties in coculture of adipocytes and macrophages. J Nutr Biochem. (2010) 21:47–54. doi: 10.1016/j.jnutbio.2008.10.003
36. Suganami T, Tanimoto-Koyama K, Nishida J, Itoh M, Yuan X, Mizuarai S, et al. Role of the toll-like receptor 4/Nf-Kappab pathway in saturated fatty acid-induced inflammatory changes in the interaction between adipocytes and macrophages. Arterioscler Thromb Vasc Biol. (2007) 27:84–91. doi: 10.1161/01.ATV.0000251608.09329.9a
37. Shi H, Kokoeva MV, Inouye K, Tzameli I, Yin H, Flier JS. Tlr4 links innate immunity and fatty acid–induced insulin resistance. J Clin invest. (2006) 116:3015–25. doi: 10.1172/JCI28898
38. Menon D, Coll R, O'Neill LAJ, Board PG. Gsto1–1 modulates metabolism in macrophages activated through the Lps and Tlr4 pathway. J Cell Sci. (2015) 128:1982–90. doi: 10.1242/jcs.167858
39. Cani PD, Amar J, Iglesias MA, Poggi M, Knauf C, Bastelica D, et al. Metabolic endotoxemia initiates obesity and insulin resistance. Diabetes (2007) 56:1761–72. doi: 10.2337/db06-1491
40. Suganami T, Nishida J, Ogawa Y. A paracrine loop between adipocytes and macrophages aggravates inflammatory changes: role of free fatty acids and tumor necrosis factor alpha. Arterioscler Thromb Vasc Biol. (2005) 25:2062–8. doi: 10.1161/01.ATV.0000183883.72263.13
41. Gögebakan Ö, Kruse M, Nauck M, Osterhoff MA, Seltmann A-C, Pfeiffer AFH. Gip increases adipose tissue expression and blood levels of Mcp-1 in humans and links high energy diets to inflammation a randomised trial. Diabetologia (2015) 58:1759–68. doi: 10.1007/s00125-015-3618-4
42. Sun Y, Zhong S, Yu J, Zhu J, Ji D, Hu G, et al. The aqueous extract of phellinus igniarius (Sh) ameliorates dextran sodium sulfate-induced colitis in C57bl/6 mice. PLoS ONE (2018) 13:e0205007. doi: 10.1371/journal.pone.0205007
43. Lin W-C, Deng J-S, Huang S-S, Wu S-H, Chen C-C, Lin W-R, et al. Anti-inflammatory activity of sanghuangporus sanghuang mycelium. Int J Mol Sci. (2017) 18:347. doi: 10.3390/ijms18020347
44. Yan GH, Choi YH. Phellinus linteus extract exerts anti asthmatic effects by suppressing Nf-kb and P38 mapk activity in an ova-induced mouse model of asthma. Immune Net. (2014) 14:107–15. doi: 10.4110/in.2014.14.2.107
45. Andrade PB, Valentao P. Insights into natural products in inflammation. Int J Mol Sci. (2018) 19:644. doi: 10.3390/ijms19030644
46. Seo MJ, Lee YJ, Hwang JH, Kim KJ, Lee BY. The inhibitory effects of quercetin on obesity and obesity-induced inflammation by regulation of mapk signaling. J Nutr Biochem. (2015) 26:1308–16. doi: 10.1016/j.jnutbio.2015.06.005
47. Jeon HJ, Seo MJ, Choi HS, Lee OH, Lee BY. Gelidium elegans, an edible red seaweed, and hesperidin inhibit lipid accumulation and production of reactive oxygen species and reactive nitrogen species in 3t3-L1 and Raw264.7 cells. Phytother Res. (2014) 28:1701–9. doi: 10.1002/ptr.5186
48. Li W, Li H, Zhang M, Wang M, Zhong Y, Wu H, et al. Quercitrin ameliorates the development of systemic lupus erythematosus-like disease in a chronic graft-versus-host murine model. Am J Physiol Renal Physiol. (2016) 311:F217–26. doi: 10.1152/ajprenal.00249.2015
49. Liu Y-Q, Yang Q-X, Cheng M-C, Xiao H-B. Synergistic inhibitory effect of icariside ii with icaritin from herba epimedii on pre-osteoclastic raw264.7 cell growth. Phytomedicine (2014) 21:1633–37. doi: 10.1016/j.phymed.2014.07.016
50. Li Y, Chi G, Shen B, Tian Y, Feng HJI. Isorhamnetin ameliorates Lps induced inflammatory response through downregulation of Nf-kb signaling. Inflammation (2016) 39:1291–301. doi: 10.1007/s10753-016-0361-z
51. Cheng D, Zhang X, Tang J, Kong Y, Wang X, Wang S. Chlorogenic acid protects against aluminum toxicity via Mapk/Akt signaling pathway in murine raw264.7 macrophages. J Inorg Biochem. (2019) 190:113–20. doi: 10.1016/j.jinorgbio.2018.11.001
52. Andujar I, Recio MC, Giner RM, Cienfuegos-Jovellanos E, Laghi S, Muguerza B, et al. Inhibition of ulcerative colitis in mice after oral administration of a polyphenol-enriched cocoa extract is mediated by the inhibition of Stat1 and Stat3 phosphorylation in colon cells. J Agric Food Chem. (2011) 59:6474–83. doi: 10.1021/jf2008925
53. Pandurangan AK, Mohebali N, Esa NM, Looi CY, Ismail S, Saadatdoust Z. Gallic acid suppresses inflammation in dextran sodium sulfate-induced colitis in mice: possible mechanisms. Int Immunopharmacol. (2015) 28:1034–43. doi: 10.1016/j.intimp.2015.08.019
54. Capiralla H, Vingtdeux V, Zhao H, Sankowski R, Al-Abed Y, Davies P, et al. Resveratrol mitigates lipopolysaccharide- and abeta-mediated microglial inflammation by inhibiting the Tlr4/Nf-Kappab/stat signaling cascade. J Neurochem. (2012) 120:461–72. doi: 10.1111/j.1471-4159.2011.07594.x
55. Eguchi A, Mulya A, Lazic M, Radhakrishnan D, Berk MP, Povero D, et al. Microparticles release by adipocytes act as “find-me” signals to promote macrophage migration. PLOS ONE (2015) 10:e0123110. doi: 10.1371/journal.pone.0123110
56. Huang C, Jacobson K, Schaller MD. Map kinases and cell migration. J Cell Sci. (2004) 117:4619–28. doi: 10.1242/jcs.01481
57. Maa MC, Chang MY, Li J, Li YY, Hsieh MY, Yang CJ, et al. The Inos/Src/Fak axis is critical in toll-like receptor-mediated cell motility in macrophages. Biochimica et Biophysica Acta (2011) 1813:136–47. doi: 10.1016/j.bbamcr.2010.09.004
58. Chen X, Chang L, Qu Y, Liang J, Jin W, Xia X. Tea polyphenols inhibit the proliferation, migration, and invasion of melanoma cells through the down-regulation of Tlr4. Int J Immunopathol Pharmacol. (2018) 32:394632017739531. doi: 10.1177/0394632017739531
Keywords: Inonotus sanghuang, polyphenols, inflammation, obesity, NF-κB signaling, MAPK signaling
Citation: Zhang M, Xie Y, Su X, Liu K, Zhang Y, Pang W and Wang J (2019) Inonotus sanghuang Polyphenols Attenuate Inflammatory Response Via Modulating the Crosstalk Between Macrophages and Adipocytes. Front. Immunol. 10:286. doi: 10.3389/fimmu.2019.00286
Received: 02 December 2018; Accepted: 04 February 2019;
Published: 26 February 2019.
Edited by:
Thea Magrone, University of Bari, ItalyReviewed by:
Ying-Yong Zhao, Northwest University, ChinaYaqing Qie, Mayo Clinic Florida, United States
Hemavathy Harikrishnan, National University of Malaysia, Malaysia
Vishwa Deepak, University of Pennsylvania, United States
Copyright © 2019 Zhang, Xie, Su, Liu, Zhang, Pang and Wang. This is an open-access article distributed under the terms of the Creative Commons Attribution License (CC BY). The use, distribution or reproduction in other forums is permitted, provided the original author(s) and the copyright owner(s) are credited and that the original publication in this journal is cited, in accordance with accepted academic practice. No use, distribution or reproduction is permitted which does not comply with these terms.
*Correspondence: Junpeng Wang, jpwangchina@henu.edu.cn
†These authors share senior authorship