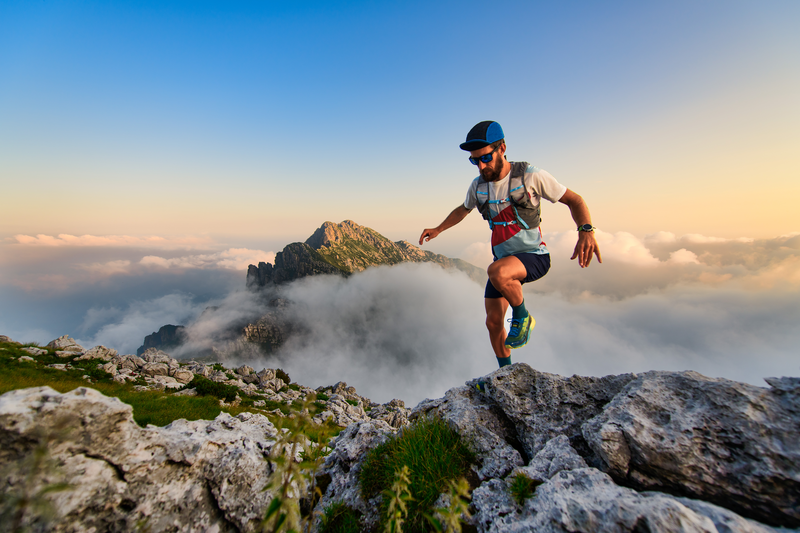
94% of researchers rate our articles as excellent or good
Learn more about the work of our research integrity team to safeguard the quality of each article we publish.
Find out more
REVIEW article
Front. Immunol. , 12 February 2019
Sec. Microbial Immunology
Volume 10 - 2019 | https://doi.org/10.3389/fimmu.2019.00229
This article is part of the Research Topic Immunity to Malaria and Vaccine Strategies View all 35 articles
IL-10 produced by CD4+ T cells suppresses inflammation by inhibiting T cell functions and the upstream activities of antigen presenting cells (APCs). IL-10 was first identified in Th2 cells, but has since been described in IFNγ-producing Tbet+ Th1, FoxP3+ CD4+ regulatory T (Treg) and IL-17-producing CD4+ T (Th17) cells, as well as many innate and innate-like immune cell populations. IL-10 production by Th1 cells has emerged as an important mechanism to dampen inflammation in the face of intractable infection, including in African children with malaria. However, although these type I regulatory T (Tr1) cells protect tissue from inflammation, they may also promote disease by suppressing Th1 cell-mediated immunity, thereby allowing infection to persist. IL-10 produced by other immune cells during malaria can also influence disease outcome, but the full impact of this IL-10 production is still unclear. Together, the actions of this potent anti-inflammatory cytokine along with other immunoregulatory mechanisms that emerge following Plasmodium infection represent a potential hurdle for the development of immunity against malaria, whether naturally acquired or vaccine-induced. Recent advances in understanding how IL-10 production is initiated and regulated have revealed new opportunities for manipulating IL-10 for therapeutic advantage. In this review, we will summarize our current knowledge about IL-10 production during malaria and discuss its impact on disease outcome. We will highlight recent advances in our understanding about how IL-10 production by specific immune cell subsets is regulated and consider how this knowledge may be used in drug delivery and vaccination strategies to help eliminate malaria.
Malaria remains a major public health problem in tropical and sub-tropical regions of the world despite substantial efforts to reduce associated morbidity and mortality. There are still around 250 million cases and 500,000 deaths annually, with young children in sub-Saharan Africa being most affected (1). A major unmet medical need for malaria is an effective vaccine. No vaccine tested to date in malaria endemic areas has performed as well as when tested in healthy volunteers, including RTS,S/AS01 (2–4).
CD4+ T cells play critical roles in coordinating immune responses during infection by differentiating into functional subsets best suited to control pathogen growth (5). Diseases caused by intracellular protozoan parasites, such as Plasmodium species, require the generation of IFNγ-producing, Tbet+ CD4+ (Th1) cells to promote antigen capture and presentation by dendritic cells (DCs) and macrophages, as well as stimulate phagocytic cells to kill captured or resident pathogens (6). However, the inflammatory cytokines produced by Th1 cells can also damage tissues. In addition, recent data suggests that Th1 cell development may also influence the development of T follicular helper (Tfh) cells, another important CD4+ T cell subset in malaria needed for the expansion of antigen-specific B cell populations and the production anti-parasitic antibody (7, 8). Hence, a better understanding about the development of CD4+ T cell responses during malaria is needed to improve strategies aimed at improving anti-parasitic immunity.
The development of a robust host immune response is essential to eliminate parasites that cause malaria and protect against re-infection. Concurrently, these responses need to be tightly regulated to avoid immune-mediated damage to host tissue. This requires the establishment of immunoregulatory networks which ultimately determine the magnitude of immune response following infection. However, if these networks over-power anti-parasitic immunity too early, parasites can persist and cause associated disease. Many molecules and cell types contribute to these immunoregulatory networks, including anti-inflammatory cytokines such as interleukine-10 (IL-10) and transforming growth factor (TGF)β, immune check point molecules such as PD-1, CTLA-4, and LAG-3, as well as CD4+ FoxP3+ regulatory T (Treg) cells. However, our understanding about how immunoregulatory networks develop following Plasmodium infection and are maintained after resolution of infection is still incomplete.
One possible explanation for the failure of RTS,S/AS01 vaccine is the early imprinting of potent, pathogen-specific immunoregulatory networks in children following first exposure to malaria that prevents the generation of robust, vaccine-induced anti-parasitic immunity (9). Hence, targeting these networks may be critical step needed for malaria vaccines to stimulate long-lasting, anti-parasitic immunity in disease-endemic areas.
IL-10 has emerged as an important regulatory molecule in malaria that protects tissues by preventing excessive inflammation (10). It suppresses inflammation not only by directly dampening pro-inflammatory cytokine and/or chemokine production, but also by down-regulating the expression of MHC-II and co-stimulatory molecules on antigen presenting cells (APCs) and increasing expression of immune checkpoint molecules (11–13). IL-10 is secreted by many different cells, including B cells, Th1, Th2, Th17, and Treg cells, as well as innate immune cells such as macrophages and DCs (14). More recently, IL-10-producing Th1 (type 1 regulatory; Tr1) cells were found to develop relatively quickly in healthy volunteers participating in controlled human malaria infection (CHMI) studies and children living in malaria-endemic areas (15–18). Results from both pre-clinical malaria models and human studies show that IL-10 not only protects against severe disease, but also inhibits protective anti-parasitic immunity. In this review, we will discuss the role of IL-10 during the blood stage of experimental and human malaria, as well as describe the cellular sources of IL-10 and how the production of this potent anti-inflammatory cytokine is regulated. We will also examine how IL-10 mediated immune response may be manipulated to improve vaccine efficacy and/or current drug treatment regimes.
The balance between host pro- and anti-inflammatory immune responses plays a critical role in determining the outcome of Plasmodium infection. A weak pro-inflammatory response may result in uncontrolled replication of parasites, while an excessive pro-inflammatory response may cause tissue damage, such as occurs in severe malaria syndromes, including cerebral malaria and multi-organ failure. Studies from mice have identified a clear role of IL-10 in controlling inflammatory responses and preventing tissue damage (14). IL-10-deficient mice infected with P. chaubadi chaubadi AS displayed exacerbated disease pathology, including hypoglycemia, hypothermia, and a loss in body weight, along with enhanced pro-inflammatory cytokine (IFN-γ, TNF-α, and IL-12) production (19). The excessive pro-inflammatory conditions in P. chabaudi AS-infected IL-10-deficient mice were also thought to cause parasite sequestration to the brain, associated with cerebral edema and hemorrhages (20). IL-10 has also been reported to play a protective role in experimental cerebral malaria (ECM) caused by P. berghei ANKA (21). In this model, decreased expression of IL-10 mRNA in spleen and brain tissue was associated with susceptibility to ECM. Sequestration of parasitized RBC (pRBC) in brain was mediated by ICAM-1 expressed by endothelial cells, and IL-10 inhibited expression of ICAM-I on these cells, thus providing a potential mechanism for the prevention of pathology associated with ECM. In a lethal P. yoelii infection in mice, production of IL-10 and TGF-β were thought to inhibit pro-inflammatory responses, and this was correlated with high parasitemia and severe anemia (22, 23). IL-10 has also been reported to promote hyper-parasitemia in mice infected with P. chabaudi adami (24). Therefore, data from mouse models of malaria indicates that IL-10 is required to protect host tissue from inflammation, but by doing so, can also promote growth of parasites and associated disease manifestations.
In a prospective longitudinal study conducted in a malaria endemic area, IL10 gene polymorphisms associated with high IL-10 production were found to increase the risk of developing clinical malaria in young children (25). High levels of circulating IL-10 have been reported in patients with mild, severe and cerebral malaria (26, 27). Similar to studies in pre-clinical models, African children with severe anemia had lower plasma IL-10 levels than patients with moderate anemia or cerebral malaria, suggesting that IL-10 plays an important role in preventing severe anemia (28). However, a case control study in an African population with mild or severe malaria showed that both IL-10 and TNF-α were elevated in severe malaria and positively correlated with parasitemia (29). In another study with children living in a holo-endemic area of Western Kenya, higher ratios of plasma IL-10 to TNF levels were strongly associated with protection against severe malaria anemia, providing evidence that IL-10 may be protective by inhibiting TNF activity (30). This was supported by data from pre-clinical malaria models that have shown over-expression of TNF can suppress haematopoiesis in the bone marrow and promote RBC destruction (31), while IL-10 is thought to enhance hematopoietic activity (32). High levels of plasma TNF have also been associated with anemia and high-density P. falciparum infection in Zairian children (33), as well as being associated with other severe malaria complications such as renal failure (34). Thus, IL-10 appears to play a critical role in regulating the pathogenic effects of TNF during malaria, but in performing this important role, IL-10 may promote high-density infections that can result in other complications of malaria, including accumulation of pRBC in tissue that can cause hypoxia and direct damage to the vasculature.
IL-10 suppressed IL-12 production by monocytes (35), which was required for the development of protective immunity against malaria and skewing the cytokine production pattern toward a pro-inflammatory response (36, 37). A study in patients with severe malaria anemia living in a holo-endemic region of western Kenya showed that ingestion of Plasmodium-derived pigment (hemozoin; [PfHz]) by monocytes, suppressed IL-12 production in an IL-10-dependent manner (38). IFNγ signaling is critical for the development of hematopoietic progenitor subsets during acute experimental malaria (39), and given the important role for IL-12 in IFNγ production, reduced levels of IL-12 would likely impact hematopoiesis during malaria.
IL-10 can also augment antibody production and B cell maturation (37, 40). In experimental malaria caused by P. yoelli infection of C57BL/6 mice, B cell intrinsic IL-10 signaling enhanced germinal center (GC) B cell responses by limiting IFNγ activity and subsequent Tbet expression by these cells, thereby promoting antibody production and parasite clearance (41). Hence, IL-10 may be beneficial for the development of humoral immunity, but detrimental for cell-mediated immune responses during malaria.
IL-10 production was initially identified in Th2 cells (42), but has since been shown to be produced by many immune cells, including Th1 cells (43, 44), Treg cells (45), IL-17-producing CD4+ T (Th17) cells (46), Tfh cells (47), CD8+ T cells (48), B cells (49), including regulatory B cells (50), NK cells (51), and γδ T cells (52, 53). Additionally, innate immune cells such as macrophages and DCs (12) have also been shown to produce IL-10. In both lethal and non-lethal mouse models caused by P. yoelii infection, the major source of IL-10 were FoxP3-negative CD4+ T cells that didn't produce Th1, Th2, or Th17-associated cytokines, and these cells not only prevented hepatic immunopathology but also suppressed the effector T cell response, preventing parasite clearance (54). These regulatory cells are amongst several specialized CD4+ T cell sub-populations which emerge from the thymus as conventional CD4+ T cells and acquire regulatory functions in the periphery following exposure to inflammatory conditions (55–57). The most well-studied of these subsets are IL-10-producing Th1 (Tr1) cells that have been identified in many infectious diseases, including visceral leishmaniasis (58), tuberculosis (59), and human immunodeficiency virus (60). Importantly, they have also be identified as an important immunoregulatory cell population in African children with P. falciparum malaria (16–18, 61), as well as in healthy volunteers participating in CHMI studies (15). A high frequency of antigen-specific Tr1 cells were also found in neonates whose mothers had active placental malaria during pregnancy, suggesting that these cells might be able to influence anti-parasitic immunity from very early in life (62). Hence, the rapid generation of Tr1 cells during malaria may play a critical role in determining the outcome of infection. Furthermore, because these cells are likely to be generated prior to vaccination in malaria endemic areas, they are also likely to impact the efficacy of malaria vaccines, and their presence and function may be an important factor contributing to the failure of these vaccines to date.
γδ T cells play several different roles in host defense against Plasmodium infection (63), including their rapid expansion upon exposure to P. falciparum antigen in malaria naïve individuals (64). However, studies in children with chronic malaria exposure showed that the Vδ2+ subset of γδ T cells declined in number and switched from a predominant pro-inflammatory response to an anti-inflammatory response that was postulated to contribute to clinical tolerance during malaria (52). Another recent study in a malaria endemic area found a subset of γδ T cells from uncomplicated malaria patients expressing Vδ9 T cell receptor that expanded and produced IFNγ and IL-10 when cultured in presence of P. falciparum antigen (53). Given that these γδ T cells produce IL-10 and can provide help to B cells for antibody production (65), they may play a role in the acquisition of natural immunity against malaria.
NK cells have also been reported to produce IL-10 during many systemic infections (66, 67). A recent study in mice infected with P. berghei ANKA showed that systemic inflammation during ECM stimulated NK cell IL-10 production but was induced too late to prevent inflammation-mediated disease pathology (51). However, in the same study, treatment of mice with recombinant IL-15 complexed with antibody to extend cytokine half-life and target NK cell induced IL-10 production which protected against disease without affecting the parasite burden.
Tr1 cells are an important immunoregulatory CD4+ T cell subset that not only prevent immune pathology during Plasmodium infection but can also promote establishment of infection by suppressing Th1 cell-mediated anti-parasitic immunity. The manipulation of this regulatory T cell subset is being considered for a wide range of immunotherapeutic applications, including in organ transplantation, rheumatoid arthritis, colitis, and cancer (68–71). Therefore, a better understanding about how IL-10 production is initiated and maintained by Tr1 cells is required for the development of new therapeutic approaches targeting this cell population. Tr1 cells are Tbet+ Foxp3− CD4+ T cells that are most likely derived from Th1 cells, and acquire an ability to co-produce IL-10 and IFNγ under inflammatory conditions (72). IL-27 has been identified as a critical regulator of T cell IL-10 production in humans and mice (46, 73–76). Generally, IL-27 produced by APC's, such as DC's and macrophages, can induce expression of the transcription factors c-Maf and aryl hydrocarbon receptor (Ahr) via STAT1 and STAT3-dependent mechanisms, which in turn, stimulated IL-21 production by CD4+ T cells which acted in an autocrine manner to expand IL-10-producing Tr1 cells (77–79) (Figure 1). In mouse models of malaria, a critical role for IL-27 signaling in the regulation of pro-inflammatory Th1 cell responses and suppression of immune-mediated pathology has been reported (80–83). A study with P. chaubadi AS infection showed that although Treg cells produced IL-10 during infection, Tr1 cells were the important source of IL-10 for protection against severe immune-mediated pathology, and that although the generation of these Tr1 cells was dependent on IL-27 signaling, it was independent of IL-21 (84). Similarly, another study with mice infected with P. yoelli confirmed that Tr1 cell generation was dependent on IL-27 receptor signaling during blood stage malaria (85). Thus, IL-27 is a critical regulator of IL-10 producing Tr1 cells in mouse models of malaria. The involvement of IL-27 in Tr1 cell generation in human malaria has not been fully established, although patients with severe malaria were reported to have reduced IL-27 plasma levels, compared to uncomplicated malaria patients and endemic controls, suggesting a potential role in controlling pathology (86). Additionally, many of the intracellular signaling pathways described above and below (Figure 1) have yet to be confirmed in samples from experimental malaria or malaria patients. This is clearly an important gap in our knowledge. It should also be noted that Tr1 cells are likely to represent a heterogenous cell population (87), and cytokine production and responsiveness may be regulated distinctly, depending on local immune conditions.
Figure 1. Key regulators of the Th1/Tr1 cell axis in mouse malaria models. Following blood-stage infection with Plasmodium parasites, dendritic cells (DC's) recognize parasite molecules and produce IL-12 to drive the expansion of antigen-specific CD4+ T cells into IFNγ-producing Th1 cells. Soon after, type I interferon (IFN) and IL-27 production is initiated by macrophages and DC's, presumably in response to activation of pattern recognition receptors by parasite molecules. Type I IFNs promote the development of IL-10-producing Th1 (Tr1) cells by T cell-independent and T cell-dependent activities (dashed red line), depending on the Plasmodium species. IL-27 stimulates STAT1 and STAT3-dependent transcription of c-Maf and aryl hydrocarbon receptor (AhR) in Th1 cells, which then drive IL-10 and IL-21 gene transcription. T cell receptor signaling of Th1 cells promotes expression of the transcription factor Erg2, which in turn, induces Prdm1 (encoding Blimp1) transcription and IL-10-production by Th1 cells in a STAT3-dependent manner. IL-21 acts as an autocrine growth factor for IL-10-producing Th1 (Tr1) cells. IL-10 produced by these cells can suppress the activity of Th1 cells and phagocytes, as well as the antigen presenting capacity of DC's and macrophages. However, the Bhlhe40 transcription factor can also be upregulated in Th1 cells to block IL-10 gene transcription and promote IFNγ production and IL-12 receptor β (IL-12Rβ) chain expression, which both re-enforce Th1 cell development and activity. Thus, Bhlhe40 has an important role in determining the balance between Th1 and Tr1 cell development. The small red ellipsoids in DC's and macrophages represent captured parasites and associated antigens. The question marks (?) indicate pathways not yet validated in Plasmodium infections.
More recently, type I interferons (IFNs) have emerged as important regulators of IL-10 production by Tr1 cells. Type I IFNs comprise a large family of cytokines that includes several types of IFNα and two types of IFNβ proteins which all signal through the common IFNα receptor (IFNAR) that consists of IFNAR1 and IFNAR2 chains which signal via STAT-1 and STAT-2 (88, 89) to mediate diverse functions during many infections (90). Polymorphisms in the IFNAR1 gene have been associated with increased risk of severe malaria in The Gambia (91, 92), while a whole-brain transcriptional analysis in genetically resistant and susceptible inbred mice infected with P. berghei ANKA identified type I IFN-dependent transcriptional program associated with the pathogenesis of severe malaria ECM (93). Type I IFNs suppress CD4+ T cell-dependent parasite control during experimental blood-stage malaria by modulating the function of CD8α− cDC following P. berghei ANKA infection, rather than acting directly on CD4+ T cells (94, 95). Another study in mice infected with P. yoelli YM showed that type I IFNs produced by plasmacytoid DCs (pDCs) activated conventional DCs (cDCs) and macrophages for generating B and T cell responses which are required for controlling parasitemia and mortality during late phase of infection (96). However, another study in mice infected with P. yoelli showed that type I IFNs directly promoted the expansion of Tr1 cells (97) (Figure 1). Significantly, in CHMI studies, type I IFNs produced by several different cell sources were found to be important regulators of developing anti-parasitic immunity. Type I IFNs not only suppressed innate immune cell function and parasitic-specific CD4+ T cell IFNγ production, but also promoted the development of parasitic-specific Tr1 cells (9). Thus, IL-27 is a major mediator of Tr1 cell development in mouse models of malaria, but to date, the main driver of Tr1 cell generation identified in humans infected with P. falciparum are type I IFNs. Whether these results point to separate pathways for Tr1 cell generation in humans and mice is still not clear, but if so, this has significant ramifications for developing strategies to modulate Tr1 cells for clinical advantage.
TNF is a potent pro-inflammatory cytokine that has been implicated in malaria pathogenesis. As mentioned above, the ratio of plasma IL-10 to TNF plays an important role in determining whether children with malaria develop anemia. It is often assumed that the relationship between these two cytokines is characterized by IL-10 dampening the pro-inflammatory activity of TNF. However, TNF can also promote IL-10 production, as demonstrated by TNF playing a major role in lipopolysaccharide-induced IL-10 secretion by human monocytes (98). Similarly, TGFβ was reported to play a protective role against severe malaria in mice (99), and TGFβ can drive IL-10 production by several different CD4+ T cell subsets, including Treg, Th17 and other FoxP3-negative cells (100). Therefore, modulation of IL-10 production in malaria may be achieved by targeting the activities of upstream activating cytokines, such as IL-27, IL-21, type I IFNs, TNF, or TGFβ. However, as indicated previously, the precise roles for these cytokines in clinical malaria still needs to be fully elucidated before these strategies can be developed and implemented.
As discussed earlier, IL-10 can be produced by most CD4+ T cell subsets in various inflammatory settings, but whether IL-10 production is mediated by common transcription factors or cell lineage-specific transcription factors is unclear. Many transcription factors, including c-Maf, have been shown to modulate IL10 gene expression in vitro (77, 101, 102). The ligand-activated transcription factor Aryl hydrocarbon Receptor (AhR) has also been shown to promote the development of Tr1 cells in humans (102). During IL-27-mediated Tr1 cell differentiation, AhR physically associates with c-Maf and trans-activates the IL10 and IL21 promoters (78) (Figure 1). A recent study in mice infected with P. chabaudi AS showed that c-Maf regulates T cell IL-10 production and T cell-specific c-Maf-deficiency was associated with greater acute-phase pathology, compared to control mice, but had little effect on blood parasitemia, similar to the phenotype observed in IL-10-deficient mice (103). Thus, although c-Maf-dependent T cell IL-10 production protected against the detrimental impact of inflammation, it had a minimal effect of the development of anti-parasitic immunity in this non-lethal, mouse malaria model.
Another transcriptional regulator, B lymphocyte induced maturation protein (BLIMP)1, has been shown to play an important role in IL-10 production by Treg cells (104). BLIMP1 is induced by IL-12 in a STAT4-dependent manner and was shown to control IL-10 expression by Tr1 cells in mice infected with Toxoplasma gondii (105). In chronic lymphocytic choriomeningitis viral infection, as well as in central nervous system-related autoimmunity, BLIMP1 was identified as a critical regulator of IL-10 production by Tr1 cells (106, 107). IL-27-dependent production of the early growth response gene 2 (Egr2), a transcription factor required for T cell anergy induction, was also required for IL-10 production by Tr1 cells in a BLIMP1-dependent manner (108, 109). BLIMP1-mediated IL-10 production by Tr1 cells was recently reported in experimental malaria (97), and BLIMP1-dependent IL-10 production by Tr1 cells protected against IFNγ-dependent, TNF-mediated splenic tissue damage, but also limited the control of P. chabaudi AS blood parasitemia (110).
More recently, basic helix-loop-helix family member e40 (Bhlhe40) has been identified as a negative transcriptional regulator of IL-10 production during Mycobacterium tuberculosis (111) and T. gondii infection (112). Interestingly, Bhlhe40 regulated IL-10 production in both T cells and DCs during M. tuberculosis infection by binding directly to the Il10 gene promoter in both cell populations (111). In the case of T. gondii infection, Bhlhe40 promoted Tbet-dependent IFNγ production by CD4+ T cells, while also suppressing IL-10 production in this cell population (112). Given the many similar mechanisms of T cell IL-10 generation between these two infectious diseases and malaria, it will be important to establish the role of this transcription factor in IL-10 production during Plasmodium infections. Another basic helix-loop-helix family member Twist-1 also regulates IL-10 production (113), although little is currently known about its role in infectious diseases settings.
Therefore, key transcriptional regulators of IL-10 production have been identified with potentially important roles in malaria (Figure 1), although as mentioned previously, many still need to be validated with samples from pre-clinical models of malaria or malaria patients. However, this area of research is still at an early stage and it will be necessary to carefully elucidate the specific cell populations in which these transcription factors operate, the range of genes they regulate and whether their modulation can change IL-10 activity to improve anti-parasitic immunity without causing tissue damage.
IL-10 plays a critical role in the immunoregulatory networks that protect tissue from infection-mediated inflammation during malaria, and there is convincing mechanistic evidence form pre-clinical malaria models and associative data from malaria patient samples showing key roles for IL-10 is preventing several severe manifestations of malaria, including the development of anemia and damage to organs. These actions of IL-10 are like a double-edged sword, cutting both ways, as they can both suppress important anti-parasitic immune responses, and in particular, the functions of Th1 cell responses, but also protect the host from tissue damage. Furthermore, as mentioned above, IL-10 may also promote anti-parasitic antibody production by B cells. Therefore, given these beneficial and detrimental roles for IL-10 during malaria, it is critically important that we improve our understanding about how IL-10 production is regulated and the specific roles for IL-10 produced by different cell populations. We know that parasite-specific Tr1 cells develop early in children living in malaria endemic areas, as well as in healthy volunteers participating in CHMI studies with P. falciparum. However, given the important roles these cells play in preventing disease pathology, as outlined above, modulation of their activity to improve vaccine efficacy or the development of immunity after anti-parasitic drug treatment may be dangerous. However, if modulation of IL-10 production by specific cell populations or subsets can be achieved, leaving in place mechanisms to protect tissue from inflammatory mediators, then clinical benefits may be achieved. This will require the identification of unique cellular signaling pathways that regulate IL-10 production in different cell populations. In addition, strategies to target specific cell signaling pathways will need to be developed.
IL-10 is a critical immunoregulatory molecule with both positive and negative roles during malaria. Blocking IL-10 activity may promote anti-parasitic immunity by enhancing APC functions and associated T cell activity. However, this will likely result in concomitant tissue pathology and related disease. Therefore, a more selective strategy for IL-10 modulation will be needed. As we identify the cell subsets producing this cytokine, and learn more about upstream regulators, the cell signaling pathways, transcription factors and post-translational modifications controlling IL-10 production, we may be able to manipulate IL-10 activity to improve anti-parasitic immunity in response to vaccination or drug treatment. Although IL-10 is a promising target for immune modulation, it is not the only such target for trying to improve outcomes in malaria. Immune checkpoint molecules, other anti-inflammatory cytokines and alternative host-directed therapies have also been identified (9, 57, 114). Ideally, different approaches should be tested in parallel to establish the safest and best approach to take. However, robust pre-clinical and clinical models will be required, as well as appropriate resourcing. The development of effective, long-lasting immunity to malaria through vaccination or drug-mediated strategies is an important priority, and our increasing knowledge should help make this possible.
RK and CE researched the work and wrote the paper. SN prepared the figure. All authors reviewed and edited the manuscript.
This work was made possible through Queensland State Government funding. It was supported by grants and fellowships from the National Health and Medical Research Council of Australia, as well as an Australian Post-graduate Awards through Griffith University and an INSPIRE Fellowship to RK provided by the Indian government Department of Science and Technology (DST).
The authors declare that the research was conducted in the absence of any commercial or financial relationships that could be construed as a potential conflict of interest.
We thank staff in the QIMR Berghofer Infection and Immunity laboratory for helpful discussions and comments on the manuscript.
2. Polhemus ME, Remich SA, Ogutu BR, Waitumbi JN, Otieno L, Apollo S, et al. Evaluation of RTS, S/AS02A and RTS, S/AS01B in adults in a high malaria transmission area. PLoS ONE (2009) 4:e6465. doi: 10.1371/journal.pone.0006465
3. Ockenhouse CF, Regules J, Tosh D, Cowden J, Kathcart A, Cummings J, et al. Ad35. CS. 01-RTS, S/AS01 heterologous prime boost vaccine efficacy against sporozoite challenge in healthy malaria-naive adults. PLoS ONE (2015) 10:e0131571. doi: 10.1371/journal.pone.0131571
4. Kester KE, Cummings JF, Ofori-Anyinam O, Ockenhouse CF, Krzych U, Moris P, et al. Randomized, double-blind, phase 2a trial of falciparum malaria vaccines RTS, S/AS01B and RTS, S/AS02A in malaria-naive adults: safety, efficacy, and immunologic associates of protection. J Infect Dis. (2009) 200:337–46. doi: 10.1086/600120
5. Sallusto F. Heterogeneity of human CD4+ T cells against microbes. Ann Rev Immunol. (2016) 34:317–34. doi: 10.1146/annurev-immunol-032414-112056
6. Tubo NJ, Jenkins MK. CD4+ T cells: guardians of the phagosome. Clin Microbiol Rev. (2014) 27:200–13. doi: 10.1128/CMR.00097-13
7. Obeng-Adjei N, Portugal S, Tran TM, Yazew TB, Skinner J, Li S, et al. Circulating Th1-cell-type Tfh cells that exhibit impaired B cell help are preferentially activated during acute malaria in children. Cell Rep. (2015) 13:425–39. doi: 10.1016/j.celrep.2015.09.004
8. Ryg-Cornejo V, Ioannidis LJ, Ly A, Chiu CY, Tellier J, Hill DL, et al. Severe malaria infections impair germinal center responses by inhibiting T follicular helper cell differentiation. Cell Rep. (2016)14:68–81. doi: 10.1016/j.celrep.2015.12.006
9. Montes De Oca M, Good MF, McCarthy JS, Engwerda CR. The impact of established immunoregulatory networks on vaccine efficacy and the development of immunity to malaria. J Immunol. (2016) 197:4518–26. doi: 10.4049/jimmunol.1600619
10. Couper KN, Blount DG, Riley EM. IL-10: the master regulator of immunity to infection. J Immunol. (2008) 180:5771–7. doi: 10.4049/jimmunol.180.9.5771
11. Fiorentino DF, Zlotnik A, Vieira P, Mosmann TR, Howard M, Moore KW, O'Garra A. IL-10 acts on the antigen-presenting cell to inhibit cytokine production by Th1 cells. J Immunol. (1991) 146:3444–51.
12. Moore KW, de Waal Malefyt R, Coffman RL, O'Garra A. Interleukin-10 and the interleukin-10 receptor. Ann Rev Immunol. (2001) 19:683–765. doi: 10.1146/annurev.immunol.19.1.683
13. Mege L-J, Meghari S, Honstettre A, Capo C, Raoult D. The two faces of interleukin 10 in human infectious diseases. Lancet Infect Dis. (2006) 6:557–69. doi: 10.1016/S1473-3099(06)70577-1
14. A.do Rosario PF, Langhorne J. T cell-derived IL-10 and its impact on the regulation of host responses during malaria. Int J Parasitol. (2012) 42:549–55. doi: 10.1016/j.ijpara.2012.03.010
15. Montes de Oca M, Kumar R, Rivera F, Amante F, Sheel M, Faleiro R, et al. Type I interferons regulate immune responses in humans with blood-stage Plasmodium falciparum infection. Cell Rep. (2016) 17:399–412. doi: 10.1016/j.celrep.2016.09.015
16. Walther M, Jeffries D, Finney OC, Njie M, Ebonyi A, Deininger S, et al. Distinct roles for FOXP3+ and FOXP3– CD4+ T cells in regulating cellular immunity to uncomplicated and severe Plasmodium falciparum malaria. PLoS Pathog. (2009) 5:e1000364. doi: 10.1371/journal.ppat.1000364
17. Jagannathan P, Eccles-James I, Bowen K, Nankya F, Auma A, Wamala S, et al. IFNγ/IL-10 co-producing cells dominate the CD4 response to malaria in highly exposed children. PLoS Pathog. (2014) 10:e1003864. doi: 10.1371/journal.ppat.1003864
18. Portugal S, Moebius J, Skinner J, Doumbo S, Doumtabe D, Kone Y, et al. Exposure-dependent control of malaria-induced inflammation in children. PLoS Pathog. (2014) 10:e1004079. doi: 10.1371/journal.ppat.1004079
19. Li C, Corraliza I, Langhorne J. A defect in interleukin-10 leads to enhanced malarial disease in Plasmodium chabaudi chabaudi infection in mice. Infection Immunity (1999) 67:4435–42.
20. Sanni LA, Jarra W, Li C, Langhorne J. Cerebral edema and cerebral hemorrhages in interleukin-10-deficient mice infected with Plasmodium chabaudi. Infect Immun. (2004) 72:3054–8. doi: 10.1128/IAI.72.5.3054-3058.2004
21. Kossodo S, Monso C, Juillard P, Velu T, Goldman M, Grau GE. Interleukin-10 modulates susceptibility in experimental cerebral malaria. Immunology (1997) 91:536–40. doi: 10.1046/j.1365-2567.1997.00290.x
22. Omer FM, de Souza JB, Riley EM. Differential induction of TGF-β regulates proinflammatory cytokine production and determines the outcome of lethal and nonlethal Plasmodium yoelii infections. J Immunol. (2003) 171:5430–36. doi: 10.4049/jimmunol.171.10.5430
23. Kobayashi F, Ishida H, Matsui T, Tsuji M. Effects of in vivo administration of anti-IL-10 or anti-IFN-γ monoclonal antibody on the host defense mechanism against Plasmodium yoelii yoelii infection. J Vet Med Sci. (2000) 62:583–7. doi: 10.1292/jvms.62.583
24. Weidanz WP, Batchelder JM, Flaherty P, LaFleur G, Wong C, Van der Heyde H. Plasmodium chabaudi adami: use of the B-cell-deficient mouse to define possible mechanisms modulating parasitemia of chronic malaria. Exp Parasitol. (2005) 111:97–104. doi: 10.1016/j.exppara.2005.06.006
25. Zhang G, Manaca MN, McNamara-Smith M, Mayor A, Nhabomba A, Berthoud TK, et al. IL-10 polymorphisms are associated with IL-10 production and clinical malaria in young children. Infect Immun. (2012) IAI:00261–12. doi: 10.1128/IAI.00261-12.
26. Peyron F, Burdin N, Ringwald P, Vuillez J, Rousset F, Banchereau J. High levels of circulating IL-10 in human malaria. Clin Exp Immunol. (1994) 95:300–3. doi: 10.1111/j.1365-2249.1994.tb06527.x
27. Lyke K, Burges R, Cissoko Y, Sangare L, Dao M, Diarra I, et al. Serum levels of the proinflammatory cytokines interleukin-1 beta (IL-1β), IL-6, IL-8, IL-10, tumor necrosis factor alpha, and IL-12 (p70) in Malian children with severe Plasmodium falciparum malaria and matched uncomplicated malaria or healthy controls. Infect Immun. (2004) 72:5630–7. doi: 10.1128/IAI.72.10.5630-5637.2004
28. Kurtzhals JA, Adabayeri V, Goka BQ, Akanmori BD, Oliver-Commey JO, Nkrumah FK, et al. Low plasma concentrations of interleukin 10 in severe malarial anaemia compared with cerebral and uncomplicated malaria. Lancet (1998) 351:1768–72. doi: 10.1016/S0140-6736(97)09439-7
29. Luty AJ, Perkins DJ, Lell B, Schmidt-Ott R, Lehman LG, Luckner D, et al. Low interleukin-12 activity in severe Plasmodium falciparum malaria. Infect Immun. (2000) 68:3909–15. doi: 10.1128/IAI.68.7.3909-3915.2000
30. Othoro C, Lal AA, Nahlen B, Koech D, Orago AS, Udhayakumar V. A low interleukin-10 tumor necrosis factor-α ratio is associated with malaria anemia in children residing in a holoendemic malaria region in western Kenya. J Infect Dis. (1999) 179:279–82. doi: 10.1086/314548
31. Taverne J, Sheikh N, De Souza J, Playfair J, Probert L, Kollias G. Anaemia and resistance to malaria in transgenic mice expressing human tumour necrosis factor. Immunology (1994) 82:397.
32. Van PV, Falla N, R.Van DH, Dasch J. Interleukin-10 stimulates hematopoiesis in murine osteogenic stroma. Clin Orthop Related Res. (1995) 313:103–14.
33. Shaffer N, Grau GE, Hedberg K, Davachi F, Lyamba B, Hightower AW, et al. Tumor necrosis factor and severe malaria. J Infect Dis. (1991) 163:96–101. doi: 10.1093/infdis/163.1.96
34. Day NP, Hien TT, Schollaardt T, Loc PP, Chuong LV, Chau TT, et al. The prognostic and pathophysiologic role of pro- and antiinflammatory cytokines in severe malaria. J Infect Dis. (1999) 180:1288–97. doi: 10.1086/315016
35. D'Andrea A, Rengaraju M, Valiante NM, Chehimi J, Kubin M, Aste M, et al. Production of natural killer cell stimulatory factor (interleukin 12) by peripheral blood mononuclear cells. J Exp Med. (1992) 176:1387–98. doi: 10.1084/jem.176.5.1387
36. Crutcher JM, Stevenson MM, Sedegah M, Hoffman SL. Interleukin-12 and malaria. Res Immunol. (1995) 146:552–9. doi: 10.1016/0923-2494(96)83031-8
37. Xu X, Sumita K, Feng C, Xiong X, Shen H, Maruyama S, et al. Down-regulation of IL-12 p40 gene in Plasmodium berghei-infected mice. J Immunol. (2001) 167:235–41. doi: 10.4049/jimmunol.167.1.235
38. Keller CC, Yamo O, Ouma C, Ong'echa JM, Ounah D, Hittner JB, et al. Acquisition of hemozoin by monocytes down-regulates interleukin-12 p40 (IL-12p40) transcripts and circulating IL-12p70 through an IL-10-dependent mechanism: in vivo and in vitro findings in severe malarial anemia. Infect Immun. (2006) 74:5249–60. doi: 10.1128/IAI.00843-06
39. Belyaev NN, Brown DE, Diaz AI, Rae A, Jarra W, Thompson J, et al. Induction of an IL7-R(+)c-Kit(hi) myelolymphoid progenitor critically dependent on IFN-gamma signaling during acute malaria. Nat Immunol. (2010) 11:477–85. doi: 10.1038/ni.1869
40. Heine G, Drozdenko G, Grun JR, Chang HD, Radbruch A, Worm M. Autocrine IL-10 promotes human B-cell differentiation into IgM- or IgG-secreting plasmablasts. Eur J Immunol. (2014) 44:1615–21. doi: 10.1002/eji.201343822
41. Guthmiller JJ, Graham AC, Zander RA, Pope RL, Butler NS. Cutting edge: IL-10 is essential for the generation of germinal center B cell responses and anti-plasmodium humoral immunity. J Immunol. (2017) 198:617–22. doi: 10.4049/jimmunol.1601762
42. Fiorentino DF, Bond MW, Mosmann T. Two types of mouse T helper cell. IV. Th2 clones secrete a factor that inhibits cytokine production by Th1 clones. J Exp Med. (1989) 170:2081–95.
43. Meyaard L, Hovenkamp E, Otto SA, Miedema F. IL-12-induced IL-10 production by human T cells as a negative feedback for IL-12-induced immune responses. J Immunol. (1996) 156:2776–82.
44. Groux H, O'garra A, Bigler M, Rouleau M, Antonenko S, De Vries JE, et al. A CD4+ T-cell subset inhibits antigen-specific T-cell responses and prevents colitis. Nature (1997) 389:737. doi: 10.1038/39614
45. Rubtsov YP, Rasmussen JP, Chi EY, Fontenot J, Castelli L, Ye X, et al. Regulatory T cell-derived interleukin-10 limits inflammation at environmental interfaces. Immunity (2008) 28:546–58. doi: 10.1016/j.immuni.2008.02.017
46. Stumhofer JS, Silver JS, Laurence A, Porrett PM, Harris TH, Turka LA, et al. Interleukins 27 and 6 induce STAT3-mediated T cell production of interleukin 10. Nat Immunol. (2007) 8:1363. doi: 10.1038/ni1537
47. Laidlaw BJ, Lu Y, Amezquita RA, Weinstein JS, Vander Heiden JA, Gupta NT, et al. Interleukin-10 from CD4+ follicular regulatory T cells promotes the germinal center response. Sci Immunol. (2017) 2:eaan4767. doi: 10.1126/sciimmunol.aan4767
48. Sun J, Madan R, Karp CL, Braciale TJ. Effector T cells control lung inflammation during acute influenza virus infection by producing IL-10. Nat Med. (2009) 15:277. doi: 10.1038/nm.1929
49. Madan R, Demircik F, Surianarayanan S, Allen JL, Divanovic S, Trompette A, et al. Nonredundant roles for B cell-derived IL-10 in immune counter-regulation. J Immunol. (2009) 183:2312–20. doi: 10.4049/jimmunol.0900185
50. Liu Y, Chen Y, Li Z, Han Y, Sun Y, Wang Q, et al. Role of IL-10-producing regulatory B cells in control of cerebral malaria in Plasmodium berghei infected mice. Eur J Immunol. (2013) 43:2907–18. doi: 10.1002/eji.201343512
51. Burrack KS, Huggins MA, Taras E, Dougherty P, Henzler CM, Yang R, et al. Interleukin-15 complex treatment protects mice from cerebral malaria by inducing interleukin-10-producing natural killer cells. Immunity (2018) 48:760–72 e4. doi: 10.1016/j.immuni.2018.03.012
52. Jagannathan P, Kim CC, Greenhouse B, Nankya F, Bowen K, Eccles-James I, et al. Loss and dysfunction of Vdelta2(+) gammadelta T cells are associated with clinical tolerance to malaria. Sci Transl Med. (2014) 6:251ra117. doi: 10.1126/scitranslmed.3009793
53. Taniguchi T, Md Mannoor K, Nonaka D, Toma H, Li C, Narita M, et al. A unique subset of gammadelta T cells expands and produces IL-10 in patients with naturally acquired immunity against Falciparum malaria. Front Microbiol. (2017) 8:1288. doi: 10.3389/fmicb.2017.01288
54. Couper KN, Blount DG, Wilson MS, Hafalla JC, Belkaid Y, Kamanaka M, et al. IL-10 from CD4+ CD25– Foxp3– CD127– adaptive regulatory T cells modulates parasite clearance and pathology during malaria infection. PLoS Pathog. (2008) 4:e1000004. doi: 10.1371/journal.ppat.1000004
55. O'Garra A, Vieira PL, Vieira P, Goldfeld AE. IL-10–producing and naturally occurring CD4+ Tregs: limiting collateral damage. J Clin Invest. (2004) 114:1372–8. doi: 10.1172/JCI23215
56. Belkaid Y, Rouse BT. Natural regulatory T cells in infectious disease. Nat Immunol. (2005) 6:353. doi: 10.1038/ni1181
57. Engwerda CR, Ng SS, Bunn PT. The regulation of CD4+ T cell responses during protozoan infections. Front Immunol. (2014) 5:498. doi: 10.3389/fimmu.2014.00498
58. Nylen S, Maurya R, Eidsmo L, Manandhar KD, Sundar S, Sacks D. Splenic accumulation of IL-10 mRNA in T cells distinct from CD4+CD25+ (Foxp3) regulatory T cells in human visceral leishmaniasis. J Exp Med. (2007) 204:805–17. doi: 10.1084/jem.20061141
59. Kumar NP, Moideen K, Banurekha VV, Nair D, Sridhar R, Nutman TB, et al. IL-27 and TGFbeta mediated expansion of Th1 and adaptive regulatory T cells expressing IL-10 correlates with bacterial burden and disease severity in pulmonary tuberculosis. Immun Inflamm Dis. (2015) 3:289–99. doi: 10.1002/iid3.68
60. Chevalier MF, Didier C, Petitjean G, Karmochkine M, Girard PM, Barre-Sinoussi F, et al. Phenotype alterations in regulatory T-cell subsets in primary HIV infection and identification of Tr1-like cells as the main interleukin 10-producing CD4+ T cells. J Infect Dis. (2015) 211:769–79. doi: 10.1093/infdis/jiu549
61. Boyle MJ, Jagannathan P, Bowen K, McIntyre TI, Vance HM, Farrington LA, et al. The development of Plasmodium falciparum-specific il10 cD4 T cells and protection from malaria in children in an area of high malaria transmission. Front Immunol. (2017) 8:1329. doi: 10.3389/fimmu.2017.01329
62. Brustoski K, Möller U, Kramer M, Petelski A, Brenner S, Palmer DR, et al. IFN-γ and IL-10 mediate parasite-specific immune responses of cord blood cells induced by pregnancy-associated Plasmodium falciparum malaria. J Immunol. (2005) 174:1738–45. doi: 10.4049/jimmunol.174.3.1738
63. Deroost K, Langhorne J. Gamma/delta T cells and their role in protection against malaria. Front Immunol. (2018) 9:2973. doi: 10.3389/fimmu.2018.02973
64. Behr C, Dubois P. Preferential expansion of V gamma 9 V delta 2 T cells following stimulation of peripheral blood lymphocytes with extracts of Plasmodium falciparum. Int Immunol. (1992) 4:361–6.
65. Caccamo N, Todaro M, La Manna MP, Sireci G, Stassi G, Dieli F. IL-21 regulates the differentiation of a human gammadelta T cell subset equipped with B cell helper activity. PLoS ONE (2012) 7:e41940. doi: 10.1371/journal.pone.0041940
66. Maroof A, Beattie L, Zubairi S, Svensson M, Stager S, Kaye PM. Posttranscriptional regulation of II10 gene expression allows natural killer cells to express immunoregulatory function. Immunity (2008) 29:295–305. doi: 10.1016/j.immuni.2008.06.012
67. Tarrio ML, Lee SH, Fragoso MF, Sun HW, Kanno Y, O'Shea JJ, et al. Proliferation conditions promote intrinsic changes in NK cells for an IL-10 response. J Immunol. (2014) 193:354–63. doi: 10.4049/jimmunol.1302999
68. Mfarrej B, Tresoldi E, Stabilini A, Paganelli A, Caldara R, Secchi A, et al. Generation of donor-specific Tr1 cells to be used after kidney transplantation and definition of the timing of their in vivo infusion in the presence of immunosuppression. J Transl Med. (2017) 15:40. doi: 10.1186/s12967-017-1133-8
69. Roncarolo MG, Gregori S, Bacchetta R, Battaglia M. Tr1 cells and the counter-regulation of immunity: natural mechanisms and therapeutic applications. Curr Top Microbiol Immunol. (2014) 380:39–68. doi: 10.1007/978-3-662-43492-5_3
70. Bacchetta R, Lucarelli B, Sartirana C, Gregori S, Stanghellini L, Teresa M, et al. Immunological outcome in haploidentical-HSC transplanted patients treated with IL-10-anergized donor T cells. Front Immunol. (2014) 5:16. doi: 10.3389/fimmu.2014.00016
71. Desreumaux P, Foussat A, Allez M, Beaugerie L, Hébuterne X, Bouhnik Y, et al. Safety and efficacy of antigen-specific regulatory T-cell therapy for patients with refractory Crohn's disease. Gastroenterology (2012) 143:1207–17. e2. doi: 10.1053/j.gastro.2012.07.116
72. Jankovic D, Kullberg MC, Feng CG, Goldszmid RS, Collazo CM, Wilson M, et al. Conventional T-bet+ Foxp3– Th1 cells are the major source of host-protective regulatory IL-10 during intracellular protozoan infection. J Exp Med. (2007) 204:273–83. doi: 10.1084/jem.20062175
73. Murugaiyan G, Mittal A, Lopez-Diego R, Maier LM, Anderson DE, Weiner HL. IL-27 is a key regulator of IL-10 and IL-17 production by human CD4+ T cells. J Immunol. (2009)183:2435–43. doi: 10.4049/jimmunol.0900568
74. Awasthi A, Carrier Y, Peron JP, Bettelli E, Kamanaka M, Flavell RA, et al. A dominant function for interleukin 27 in generating interleukin 10–producing anti-inflammatory T cells. Nat Immunol. (2007) 8:1380. doi: 10.1038/ni1541
75. Ansari NA, Kumar R, Gautam S, Nylén S, Singh OP, Sundar S, et al. IL-27 and IL-21 are associated with T cell IL-10 responses in human visceral leishmaniasis. J Immunol. (2011) 186:3977–85. doi: 10.4049/jimmunol.1003588
76. Anderson CF, Stumhofer JS, Hunter CA, Sacks D. IL-27 regulates IL-10 and IL-17 from CD4+ cells in nonhealing Leishmania major infection. J Immunol. (2009) 183:4619–27. doi: 10.4049/jimmunol.0804024
77. Pot C, Jin H, Awasthi A, Liu SM, Lai CY, Madan R, et al. Cutting edge: IL-27 induces the transcription factor c-Maf, cytokine IL-21, and the costimulatory receptor ICOS that coordinately act together to promote differentiation of IL-10-producing Tr1 cells. J Immunol. (2009) 183:797–801. doi: 10.4049/jimmunol.0901233
78. Apetoh L, Quintana FJ, Pot C, Joller N, Xiao S, Kumar D, et al. The aryl hydrocarbon receptor interacts with c-Maf to promote the differentiation of type 1 regulatory T cells induced by IL-27. Nat Immunol. (2010) 11:854. doi: 10.1038/ni.1912
79. Spolski R, Kim H-P, Zhu W, Levy DE, Leonard WJ. IL-21 mediates suppressive effects via its induction of IL-10. J Immunol. (2009) 182:2859–67. doi: 10.4049/jimmunol.0802978
80. Villegas-Mendez A, de Souza JB, Lavelle SW, Findlay EG, Shaw TN, van Rooijen N, et al. IL-27 receptor signalling restricts the formation of pathogenic, terminally differentiated Th1 cells during malaria infection by repressing IL-12 dependent signals. PLoS Pathog. (2013) 9:e1003293. doi: 10.1371/journal.ppat.1003293
81. Findlay EG, Villegas-Mendez A, O'Regan N, de Souza JB, Grady LM, Saris CJ, et al. IL-27R signalling regulates memory CD4+ T cell populations and suppresses rapid inflammatory responses during secondary malaria infection. Infect Immun. (2013) IAI:01091–13. doi: 10.1128/IAI.01091-13
82. Kimura D, Miyakoda M, Kimura K, Honma K, Hara H, Yoshida H, et al. Interleukin-27-producing CD4+ T cells regulate protective immunity during malaria parasite infection. Immunity (2016) 44:672–82. doi: 10.1016/j.immuni.2016.02.011
83. Findlay EG, Greig R, Stumhofer JS, Hafalla JC, de Souza JB, Saris CJ, et al. Essential role for IL-27 receptor signaling in prevention of Th1-mediated immunopathology during malaria infection. J Immunol. (2010) 185:2482–92 doi: 10.4049/jimmunol.0904019
84. do Rosário APF, Lamb T, Spence P, Stephens R, Lang A, Roers A, et al. IL-27 promotes IL-10 production by effector Th1 CD4+ T cells: a critical mechanism for protection from severe immunopathology during malaria infection. J Immunol. (2011) 188:1178–90. doi: 10.4049/jimmunol.1102755
85. Villegas-Mendez A, Shaw TN, Inkson CA, Strangward P, de Souza JB, Couper KN. Parasite-specific CD4+ IFN-γ+ IL-10+ T cells distribute within both lymphoid and non-lymphoid compartments and are controlled systemically by IL-27 and ICOS during blood-stage malaria infection. Infect Immun. (2015) IAI:01100–15. doi: 10.1128/IAI.01100-15
86. Ayimba E, Hegewald J, Segbena A, Gantin R, Lechner C, Agosssou A, et al. Proinflammatory and regulatory cytokines and chemokines in infants with uncomplicated and severe Plasmodium falciparum malaria. Clin Exp Immunol. (2011) 166:218–26. doi: 10.1111/j.1365-2249.2011.04474.x
87. Brockmann L, Soukou S, Steglich B, Czarnewski P, Zhao L, Wende S, et al. Molecular and functional heterogeneity of IL-10-producing CD4(+) T cells. Nat Commun. (2018) 9:5457. doi: 10.1038/s41467-018-07581-4
88. Ivashkiv LB, Donlin LT. Regulation of type I interferon responses. Nat Rev Immunol. (2014) 14:36–49. doi: 10.1038/nri3581
89. Pestka S, Krause CD, Walter MR. Interferons, interferon-like cytokines, and their receptors. Immunol. Rev. (2004) 202:8–32. doi: 10.1111/j.0105-2896.2004.00204.x
90. McNab F, Mayer-Barber K, Sher A, Wack A, O'garra A. Type I interferons in infectious disease. Nat Rev Immunol. (2015) 15:87. doi: 10.1038/nri3787
91. Aucan C, Walley A, Hennig B, Fitness J, Frodsham A, Zhang L, et al. Interferon-alpha receptor-1 (IFNAR1) variants are associated with protection against cerebral malaria in the Gambia. Genes Immun. (2003) 4:275. doi: 10.1038/sj.gene.6363962
92. Khor CC, Vannberg FO, Chapman SJ, Walley A, Aucan C, Loke H, et al. Positive replication and linkage disequilibrium mapping of the chromosome 21q22. 1 malaria susceptibility locus. Genes Immun. (2007) 8:570. doi: 10.1038/sj.gene.6364417
93. Lovegrove FE, Gharib SA, Patel SN, Hawkes CA, Kain KC, Liles WC. Expression microarray analysis implicates apoptosis and interferon-responsive mechanisms in susceptibility to experimental cerebral malaria. Am J Pathol. (2007) 171:1894–903. doi: 10.2353/ajpath.2007.070630
94. Haque A, Best SE, De Oca MM, James KR, Ammerdorffer A, Edwards CL, et al. Type I IFN signaling in CD8–DCs impairs Th1-dependent malaria immunity. J Clin Invest. (2014) 124:2483–96. doi: 10.1172/JCI70698
95. Haque A, Best SE, Ammerdorffer A, Desbarrieres L, de Oca MM, Amante FH, et al. Type I interferons suppress CD4+ T-cell-dependent parasite control during blood-stage Plasmodium infection. Eur J Immunol. (2011) 41:2688–98. doi: 10.1002/eji.201141539
96. Yu X, Cai B, Wang M, Tan P, Ding X, Wu J, et al. Cross-regulation of two type I interferon signaling pathways in plasmacytoid dendritic cells controls anti-malaria immunity and host mortality. Immunity (2016) 45:1093–107. doi: 10.1016/j.immuni.2016.10.001
97. Zander RA, Guthmiller JJ, Graham AC, Pope RL, Burke BE, Carr DJ, Butler NS. Type I interferons induce T regulatory 1 responses and restrict humoral immunity during experimental malaria. PLoS Pathog. (2016) 12:e1005945. doi: 10.1371/journal.ppat.1005945
98. Wanidworanun C, Strober W. Predominant role of tumor necrosis factor-alpha in human monocyte IL-10 synthesis. J Immunol. (1993) 151:6853–61.
99. Omer FM, Riley EM. Transforming growth factor β production is inversely correlated with severity of murine malaria infection. J Exp Med. (1998) 188:39–48.
100. Saraiva M, O'garra A. The regulation of IL-10 production by immune cells. Nat Rev Immunol. (2010) 10:170. doi: 10.1038/nri2711
101. Xu J, Yang Y, Qiu G, Lal G, Wu Z, Levy DE, et al. c-Maf regulates IL-10 expression during Th17 polarization. J Immunol. (2009) 182:6226–36. doi: 10.4049/jimmunol.0900123
102. Gandhi R, Kumar D, Burns EJ, Nadeau M, Dake B, Laroni A, et al. Activation of the aryl hydrocarbon receptor induces human type 1 regulatory T cell–like and Foxp3+ regulatory T cells. Nature Immunol. (2010) 11:846. doi: 10.1038/ni.1915
103. Gabryšová L, Alvarez-Martinez M, Luisier R, Cox LS, Sodenkamp J, Hosking C, et al. c-Maf controls immune responses by regulating disease-specific gene networks and repressing IL-2 in CD4+ T cells. Nat Immunol. (2018) 19:497. doi: 10.1038/s41590-018-0083-5
104. Cretney E, Xin A, Shi W, Minnich M, Masson F, Miasari M, et al. The transcription factors Blimp-1 and IRF4 jointly control the differentiation and function of effector regulatory T cells. Nat Immunol. (2011) 12:304. doi: 10.1038/ni.2006
105. Neumann C, Heinrich F, Neumann K, Junghans V, Mashreghi MF, Ahlers J, et al. Role of Blimp-1 in programing Th effector cells into IL-10 producers. J Exp Med. (2014) 211:1807–19. doi: 10.1084/jem.20131548
106. Heinemann C, Heink S, Petermann F, Vasanthakumar A, Rothhammer V, Doorduijn E, et al. IL-27 and IL-12 oppose pro-inflammatory IL-23 in CD4+ T cells by inducing Blimp1. Nat Commun. (2014) 5:3770. doi: 10.1038/ncomms4770
107. Parish IA, Marshall HD, Staron MM, Lang PA, Brüstle A, Chen JH, et al. Chronic viral infection promotes sustained Th1-derived immunoregulatory IL-10 via BLIMP-1. J Clin Invest. (2014) 124:3455–468. doi: 10.1172/JCI66108
108. Iwasaki Y, Fujio K, Okamura T, Yanai A, Sumitomo S, Shoda H, et al. Egr-2 transcription factor is required for Blimp-1-mediated IL-10 production in IL-27-stimulated CD4+ T cells. Eur J Immunol. (2013) 43:1063–73. doi: 10.1002/eji.201242942
109. Okamura T, Fujio K, Shibuya M, Sumitomo S, Shoda H, Sakaguchi S, et al. CD4+ CD25– LAG3+ regulatory T cells controlled by the transcription factor Egr-2. Proc Nat Acad Sci USA. 106 (2009) 13974–79. doi: 10.1073/pnas.0906872106
110. Montes de Oca M, Kumar R, de Labastida Rivera F, Amante FH, Sheel M, Faleiro RJ, et al. Blimp-1-dependent IL-10 production by Tr1 cells regulates TNF-mediated tissue pathology. PLoS Pathog. (2016) 12:e1005398. doi: 10.1371/journal.ppat.1005398
111. Huynh JP, Lin CC, Kimmey JM, Jarjour NN, Schwarzkopf EA, Bradstreet TR, et al. Bhlhe40 is an essential repressor of IL-10 during Mycobacterium tuberculosis infection. J Exp Med. (2018) 215:1823–38 doi: 10.1084/jem.20171704
112. Yu F, Sharma S, Jankovic D, Gurram RK, Su P, Hu G, et al. The transcription factor Bhlhe40 is a switch of inflammatory versus antiinflammatory Th1 cell fate determination. J Exp Med. (2018) 215:1813–21. doi: 10.1084/jem.20170155
113. Zheng S, Hedl M, Abraham C. Twist1 and Twist2 contribute to cytokine downregulation following chronic NOD2 stimulation of human macrophages through the coordinated regulation of transcriptional repressors and activators. J Immunol. (2015) 195:217–26. doi: 10.4049/jimmunol.1402808
Keywords: IL-10, malaria, protozoan, T cells, Inflammation
Citation: Kumar R, Ng S and Engwerda C (2019) The Role of IL-10 in Malaria: A Double Edged Sword. Front. Immunol. 10:229. doi: 10.3389/fimmu.2019.00229
Received: 31 August 2018; Accepted: 28 January 2019;
Published: 12 February 2019.
Edited by:
Noah Butler, The University of Iowa, United StatesReviewed by:
Tracey Lamb, University of Utah, United StatesCopyright © 2019 Kumar, Ng and Engwerda. This is an open-access article distributed under the terms of the Creative Commons Attribution License (CC BY). The use, distribution or reproduction in other forums is permitted, provided the original author(s) and the copyright owner(s) are credited and that the original publication in this journal is cited, in accordance with accepted academic practice. No use, distribution or reproduction is permitted which does not comply with these terms.
*Correspondence: Rajiv Kumar, cmFqaXYwODJAeWFob28uY29t
Christian Engwerda, Y2hyaXN0aWFuLmVuZ3dlcmRhQHFpbXIuZWR1LmF1
Disclaimer: All claims expressed in this article are solely those of the authors and do not necessarily represent those of their affiliated organizations, or those of the publisher, the editors and the reviewers. Any product that may be evaluated in this article or claim that may be made by its manufacturer is not guaranteed or endorsed by the publisher.
Research integrity at Frontiers
Learn more about the work of our research integrity team to safeguard the quality of each article we publish.