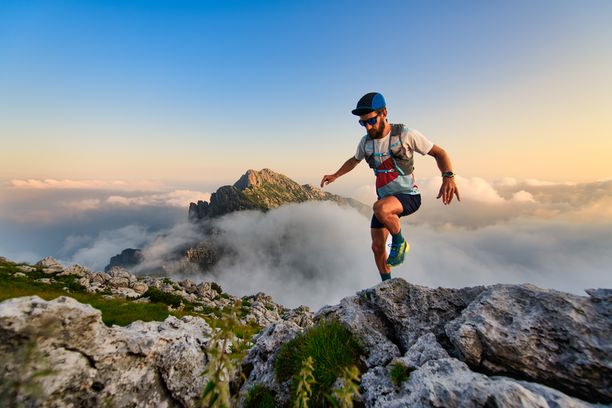
94% of researchers rate our articles as excellent or good
Learn more about the work of our research integrity team to safeguard the quality of each article we publish.
Find out more
MINI REVIEW article
Front. Immunol., 17 January 2019
Sec. Immunological Tolerance and Regulation
Volume 9 - 2018 | https://doi.org/10.3389/fimmu.2018.03179
This article is part of the Research TopicImmune Tolerance Post Allogeneic Hematopoietic Cell TransplantationView all 12 articles
Under normal conditions our intestines are inhabited by trillions of diverse microorganisms composing the intestinal microbiota, which are mostly non-pathogenic anaerobic commensal bacteria vital for the maintenance of immune homeostasis. The composition and diversity of the intestinal microbiota can be disturbed by various factors including diet, antibiotics, and exposure to intestinal pathogens. Alterations of the intestinal microbiota contributes to many diseases including graft-vs.-host disease (GVHD), a life threatening complication that occurs after allogeneic hematopoietic cell transplantation (allo-HCT) caused by an allogeneic reaction of donor T cells against recipient target tissues. Intestinal GVHD is most difficult to treat and connected to a high mortality. Due to recent advances in high-throughput sequencing technology, composition of the microbiome during allo-HCT has been characterized, and some common patterns have been identified. Metabolites produced by intestinal bacteria were shown to promote intestinal tissue homeostasis and immune tolerance post-allo-HCT. In this review, we discuss the role of the intestinal microbiota and metabolites in the context of acute GVHD. Moreover, novel therapeutic approaches that aim at protecting or regenerating intestinal cell populations will be highlighted.
Allogeneic hematopoietic cell transplantation (allo-HCT) is the only curative therapy option for most acute leukemias. Prior allo-HCT patients undergo a conditioning regimen, consisting of chemotherapy with or without radiotherapy to decrease the number of malignant cells, and prevent rejection of the transplanted hematopoietic donor cells. Subsequently, hematopoietic stem cells (HSCs) from the bone marrow or peripheral blood of an allogeneic donor are infused. In addition to the HSCs, the graft contains allogeneic donor T cells, which can on the one hand attack residual malignant cells, known as graft-vs.-leukemia (GvL) effect, but on the other hand may attack healthy host tissues, resulting in graft-vs.-host disease (GVHD). Although the understanding of the disease pathophysiology has improved significantly in the last decades, acute GVHD is still a major cause of non-relapse morbidity, and mortality post-allo-HCT (1). In particular, patients developing acute GVHD that is refractory to standard steroid therapy are difficult to treat and have a dismal prognosis with only 5–30% overall survival (2–4).
The important influence of intestinal microbiota on immune responses, including post-allo-HCT, becomes more and more recognized and the gut is one of the main targets of acute GVHD. Severe gastrointestinal (GI) GVHD remains a major issue after allo-HCT, since it is difficult to treat and involvement of the GI tract is reported in almost all fatal acute GVHD cases (5). In addition to allogeneic donor T cells, recipient-derived myeloid cells have been shown to participate in GI GVHD development. A number of publications have demonstrated that innate immune cells, including neutrophils and inflammatory monocytes, are recruited to the gut shortly after allo-HCT and contribute to GVHD tissue damage (6–9). The recruitment of neutrophils was dependent on the translocation of bacteria into the intestinal wall and was abrogated in mice housed under germ-free conditions or subjected to antibiotics-based decontamination (6, 10). While in the early phase after allo-HCT antibiotics can reduce the number of transmigrated bacteria which is beneficial, the long-term effects of antibiotics are unfavorable because they limit the microbiota diversity.
The first hint that the intestinal microbiota affect GVHD development dates back to the early 1970s, when first studies in murine models showed that GVHD was reduced and survival was prolonged in antibiotics-treated or germ-free mice undergoing allo-HCT (11–13).
Consequently, efforts were made to translate these insights into the human setting. Clinical trials investigated gut decontamination using antibiotics or laminar-airflow isolation rooms for patients undergoing allo-HCT, in order to minimize contact to microbiota (14–19). However, the study designs were heterogeneous and the outcomes showed inconsistent results. More recent trials even demonstrated that broad spectrum antibiotics increased GVHD-related mortality in allo-HCT patients and mice (20) and the lack of antibiotics that spare commensal bacteria remains a so far unresolved challenge (21). Therefore, to date no standardized protocol for prophylactic and peri-transplant antibiotic treatment has been established as standard of care across transplantation centers.
However, interest in the interplay between the microbiome and inflammatory conditions has been growing again in recent years. Major advances in modern high-throughput sequencing technology made it possible to investigate changes in the composition of the intestinal microflora after allo-HCT in depth and to analyze which bacteria might be detrimental and which may be beneficial with respect to immune tolerance post-allo-HCT.
Under physiological conditions, the intestinal microflora is highly diverse with a domination of anaerobic commensal bacteria, most importantly members of the Firmicutes and Bacteroidetes phyla (22, 23). During allo-HCT, this diversity is reduced and significant alterations in the microbial composition of the gut can be observed, indicating that the conditioning and/or transplantation induce an intestinal dysbiosis (24–26). Holler et al. demonstrated shifts in the intestinal microbiome after allo-HCT with a predominant increase in the proportion of Enterococci (24). This shift was associated with development of GI GVHD. The mean proportion of Enterococci was 21% in patients who did not develop GI GVHD as compared to 46% in those that subsequently developed GI GVHD and 74% at the time of active GVHD (24). Moreover, lower intestinal diversity has been shown to be associated with significantly worse mortality outcomes in allo-HCT patients, suggesting that the intestinal microbiota may be an important factor in the success or failure in allo-HCT (25). Looking more specifically at the composition of the microbiota of patients who died vs. patients who survived, greater abundance of γ-Proteobacteria, including Enterobacteriaceae correlated with increased mortality, whereas greater abundance of Lachnospiraceae and Actinomycetaceae was associated with favorable outcomes (25).
Since those first innovative studies, a lot of work has been done to investigate how the intestinal microbiota affects immune tolerance post-allo-HCT. A list of preclinical and clinical studies that have analyzed the role of specific bacteria during GVHD pathogenesis can be found in Table 1 and has been reviewed in detail elsewhere (37, 38).
Table 1. Summary of studies investigating how microbiota changes affect GVHD (structured by phylum).
In the following, we will highlight the most recent of these findings as well as the latest clinical trials aiming to reduce GVHD by manipulating the intestinal microbiota.
Following up on previous studies showing post-transplant monodomination of the gut microbiome with Enterococcus spp. in a smaller number of allo-HCT patients (24, 39), these findings were recently confirmed in a large cohort derived from three different centers (28). Monodomination with Enterococcus was significantly associated with severe acute GVHD. Moreover, oral administration of Enterococcus faecalis following transplantation significantly aggravated acute GVHD in a murine MHC-mismatched model, indicating a causative role for Enterococcus spp. in the pathogenesis of acute GVHD (28).
Another study found a significant depletion of anti-inflammatory Clostridia spp. (AIC) preceding the development of GVHD in pediatric allo-HCT patients (35). Treatment with anti-anaerobic antibiotics and subsequent depletion of AIC was associated with increased GVHD. These clinical observations were also validated in a murine GVHD model, where clindamycin depleted Clostridia and exacerbated disease, whereas oral AIC supplementation attenuated GVHD (35).
In a very recent monocentric study comprising 275 patients, the cumulative incidence of acute GVHD, in particular in the gut, was significantly increased in allo-HCT recipients treated with 4th generation cephalosporins peritransplant (40). A retrospective analysis of 857 allo-HCT recipients by Shono et al. demonstrated that treatment with the broadband antibiotics imipenem-cilastatin or piperacillin-tazobactam was associated with increased GVHD-related mortality at 5 years (20). However, in contrast with the aforementioned study (40), GVHD-related mortality was not correlated with cefepime or aztreonam therapy. Moreover, imipenem-cilastatin treatment caused destruction of the colonic mucus layer in mice and expansion of Akkermansia muciniphila, which is a commensal bacterium with mucus-degrading capabilities (41, 42). These data suggest that mucus degradation might aggravate murine GVHD (20).
Based on these recent advances in the field, several clinical trials are currently aiming to reduce GVHD by manipulating the intestinal microbiota. Fecal microbiota transplantation (FMT), also known as stool transplantation, has been used to successfully treat recurrent Clostridium difficile infection in non-HCT patients (43). Very recently, first promising data from studies investigating FMT in allo-HCT patients were published (44, 45). A pilot study used FMT from healthy donors to treat eight patients with steroid refractory acute GI GVHD, who have a dismal prognosis (NCT03148743). Following FMT, bacterial diversity and proportion of beneficial bacteria, such as Bacteroides, increased and clinical symptoms relieved (44). Another recent pilot trial assessed the effect of third-party oral FMT early after allogeneic HCT (NCT02733744). First studies showed that FMT was feasible and associated with improvements in microbiome diversity in transplant recipients (45). Further studies assessing the safety and efficacy of FMT for GVHD prophylaxis are ongoing (NCT03214289, NCT03359980, NCT03549676, NCT03492502).
Microbial metabolites comprise a multitude of different intermediate products and end products of intestinal microbiota metabolism with various functions, yet their role in GVHD pathophysiology is merely starting to be discovered. Very recent publications revealed that one of the mechanisms, how intestinal bacteria can influence immune tolerance post-allo-HCT, is the production of metabolites (Figure 1). Table 2 summarizes studies that have investigated the role of microbial metabolites in epithelial regeneration.
Figure 1. Microbial metabolites regulating gastrointestinal GVHD. IECs are damaged by the cytotoxic conditioning regimen as well as by GI GVHD, leading to disruption of the intestinal barrier. DAMPs released by the dying IECs as well as translocating bacteria and PAMPs activate host APCs via TLRs and the NLRP3 inflammasome, resulting in pro-inflammatory cytokine release, donor T cell activation, and GVHD. Microbial metabolites derived from intestinal microbiota can regulate IEC damage and mitigate GVHD. SCFAs mediate IEC protection via at least two different mechanisms. Firstly, binding of SCFAs to the G-protein-coupled receptor GPR43 on IECs leads to ERK phosphorylation and subsequent NLRP3 inflammasome activation, which promotes IEC integrity and repair by increasing IL-18 secretion. Secondly, the SCFA butyrate acts as a histone deacetylase inhibitor, thereby increasing expression of many different target genes, including anti-apoptotic BCL-B and the junctional protein JAM. This results in decreased IEC apoptosis and increased junctional integrity and hence IEC protection. MAIT cells located in the lamina propria respond to riboflavin metabolite antigens presented on the MHC class I-like molecule MR-1 to secrete large amounts of IL-17A, which enhances intestinal barrier integrity. Indoles and indole derivatives act via type I IFN signaling to protect and repair the mucosal barrier from damage and ameliorate GVHD. The exact molecular mechanisms and involved proteins remain to be elucidated. APC, antigen-presenting cell; DAMP, danger-associated molecular pattern; GI, gastrointestinal; GVHD, graft-vs.-host disease; IEC, intestinal epithelial cell; IFN, interferon; JAM, junctional adhesion molecule; MAIT cell, mucosal-associated invariant T cell; PAMP, pathogen-associated molecular pattern; SCFA, short chain fatty acid; siTCR, semiinvariant T cell receptor; TLR, Toll-like receptor; UA, uric acid.
Table 2. Summary of studies investigating how microbial metabolites affect intestinal epithelial regeneration.
Indole and indole derivatives are produced by commensal bacteria using the enzyme tryptophanase and are known to enhance epithelial barrier integrity and to attenuate inflammation (57). Several reports have shown that in the GI tract, bacterial-, and diet-derived indoles engage the aryl hydrocarbon receptor (AhR) and thereby expand innate lymphoid cells (ILC3) and their production of IL-22 (47, 58–60). Specifically, the tryptophan metabolite indole-3-aldehyde produced by intestinal microbiota induces AhR-dependent Il22 transcription and mucosal immune homeostasis (47). The important role of IL-22 in the protection of intestinal tissue is further discussed below. Very recently, indole and indole derivatives either produced by administered E. coli strains or administered exogenously have been shown to strongly reduce GVHD severity, damage of the intestinal epithelium, and transepithelial bacterial translocation, while the GvL effect was not compromised. Mechanistically it was demonstrated that the effects of indole administration were mediated through an increased type I interferon response in an IL-22 independent fashion (48).
Another study focusing on microbiota-derived metabolites could show that the short-chain fatty acid butyrate was significantly reduced in murine intestinal tissue post-allo-HCT resulting in diminished histone acetylation in intestinal epithelial cells (IECs) (34). Daily intragastric administration of butyrate improved IEC junctional integrity and reduced clinical scores and mortality in different murine GVHD models (34). Moreover, butyrate-producing bacteria in the gut were shown to be associated with increased resistance against respiratory viral infection in allo-HCT patients, indicating a favorable role of these bacteria for both immune regulation and prevention of infection after allo-HCT (61). Interestingly, a clinical trial analyzing the role of oral potato-based starch, which is able to increase microbial butyrate production (62), in GVHD prevention is currently recruiting (NCT02763033).
Focusing more on the receptors of bacterial metabolites, the group of Dr. Reddy recently revealed an important regulatory role for GPR43, a G-protein-coupled receptor on IECs recognizing the microbiota-derived short-chain fatty acids, butyrate, and propionate, during GVHD development. Sensing of the microbial metabolites by GPR43 reduced GVHD severity and mortality in murine models by activating the NLRP3 inflammasome in recipient non-hematopoietic cells via ERK phosphorylation. Importantly, GPR43 did not seem to play a role for host and donor hematopoietic cells, since absence of GPR43 did not lead to systemic activation of donor T cells or inflammation (50). While previous reports have already demonstrated a critical role of NLRP3 inflammasome activation in host APCs for the full manifestation of GVHD (63), this study shows that it has the opposite effect in non-hematopoietic IECs. Hence, these data shed new light on the function of NLRP3 in the pathogenesis of GVHD, implicating that the NLRP3 inflammasome can either mitigate or exacerbate GVHD, depending on the involved cell type.
In a recently published study, Varelias et al. demonstrated that recipient mucosal-associated invariant T (MAIT) cells are present in acute GVHD target organs in murine models, including the intestinal lamina propria (56). These cells express semiinvariant T cell receptor repertoires recognizing and responding to microbial riboflavin metabolites. During acute GVHD, MAIT cells generated large amounts of IL-17A, enhanced intestinal barrier function and reduced GI GVHD severity (56). Given the known ability of IL-17 to maintain epithelial cell barrier function (64, 65), the authors conclude that riboflavin metabolite sensing MAIT cells regulate acute GVHD at least partially via IL-17A secretion.
Severe GI GVHD remains a major issue after allo-HCT, since it is difficult to treat and involvement of the GI tract is reported in almost all fatal acute GVHD cases (5). Therefore, new therapy approaches aiming for tissue regeneration in GVHD target organs, as opposed to systemic immunosuppression, are promising.
Intestinal stem cells (ISCs) represent important players for both physiological renewal of intestinal cells and tissue regeneration after injury. In a murine model, it was shown that ISCs are damaged during the conditioning regimen and the development of GVHD (66). This could be rescued by treatment with the Wnt agonist R-spondin1 (R-Spo1), which protected against ISC damage after allo-HCT, thereby ameliorating GVHD. In a subsequent study the authors extended their findings, demonstrating that R-Spo1 induces differentiation of ISCs into Paneth cells (67). Paneth cells are known to produce anti-microbial peptides, most importantly alpha defensins, which shape the intestinal antimicrobial flora by mostly targeting non-commensals. Targeting and destruction of Paneth cells during GVHD has already been reported (29, 68, 69). Consistently, by inducing ISC differentiation into Paneth cells, R-Spo1 treatment augmented secretion of alpha defensins, and prevented GVHD-mediated dysbiosis, restoring intestinal homeostasis. The authors suggest that R-Spo1 could inhibit the growth of pathogenic non-commensals, while protecting favorable symbiotic bacteria (67). These findings represent a potential novel strategy to physiologically shape the intestinal microbiome.
IL-22 is a member of the IL-10 cytokine family that plays opposing roles in different autoimmune diseases, being able to drive or regulate disease (70–73). Similarly, both protective and pro-inflammatory roles for IL-22 in GVHD models have been described. On the one hand, IL-22 was recently shown to exacerbate acute GVHD by reducing regulatory T cells in a parent-to-F1 mouse model (74) and to promote cutaneous chronic GVHD (75).
In contrast, Hanash et al. demonstrated that recipient innate lymphoid cell (ILC)-derived IL-22 was able to enhance regeneration of IL-22R-expressing ISCs in a more acute major mismatch GVHD model (76). Host deficiency of IL-22 caused increased epithelial damage, loss of ISCs, and loss of epithelial integrity, resulting in GVHD exacerbation. In a subsequent study, the Hanash group further elucidated the underlying mechanisms, showing that IL-22 increased the growth of murine and human organoids by inducing STAT3 phosphorylation in Lgr5+ ISCs (77). Moreover, the authors used two different in vivo treatment approaches and showed the feasibility of both recombinant IL-22 and a recombinant human IL-22-dimer/Fc-fusion protein (F-652) for the treatment of murine GVHD. Based on the results from these preclinical studies, a Phase IIa clinical trial investigating F-652 in combination with systemic corticosteroids in patients with grade II-IV lower GI acute GVHD is currently recruiting (NCT0240665).
The described conflicting data of both a regulatory and inflammatory role for IL-22 in GVHD might result from many factors differing between the studies, including kinetics of the model, source of IL-22, presence or absence of other cytokines and pathophysiological differences between acute and chronic GVHD models.
A recent study nicely demonstrated the role of the Paneth cell derived protein Regenerating islet-derived protein 3 alpha (REG3α) to regulate GI acute GVHD (78). REG3α blood levels were strongly upregulated in patients developing severe GI GVHD, which had been shown before (79), and increased blood levels of REG3α in patients with GVHD inversely correlated with Paneth cell numbers. In murine models, the authors demonstrated that absence of REG3γ, which is the mouse homolog of REG3α, exacerbated GVHD without altering the microbial composition in the intestine (78). IL-22 is a known regulator of REG3γ expression (80, 81). In agreement with this, IL-22 administration restored REG3γ production and intestinal epithelial integrity by preventing ISC and Paneth cell apoptosis, resulting in amelioration of GVHD. In Reg3γ deficient mice this protection was completely abrogated, emphasizing the important role of REG3γ for gastrointestinal crypt homeostasis (78).
Since host genetics can shape the microbiome, single nucleotide polymorphisms (SNPs) in the genes encoding two of the most abundant Paneth cell antimicrobial peptides REG3α and HD5 were studied (82). Interestingly, SNPs in the gene for HD5 modulated the risk for acute GVHD severity in allo-HCT recipients, potentially by affecting intestinal expression of HD5, which leads to dysbiosis. In contrast, REG3A SNPs were not associated with acute GVHD severity in this retrospective study including 350 patients (82).
Keratinocyte growth factor (KGF) is a member of the fibroblast growth factor family, regulating differentiation, and proliferation of epithelial cells, including IECs, as well as intestinal stem cells (83, 84). Almost 20 years ago, KGF was already shown to ameliorate GVHD-induced tissue damage in murine models, while preserving GvL effects (85, 86). Based on these preclinical findings two placebo-controlled randomized clinical trials evaluated the efficacy of human recombinant KGF (palifermin) to decrease acute GVHD. Severity of mucositis was reduced by palifermin in a subgroup of patients conditioned with cyclophosphamide and fractionated total-body irradiation in one trial (87). However, in contrast to the findings in murine models, GVHD incidence, severity, and survival were not significantly improved by KGF as compared to placebo in both trials (87, 88). Consistently, a more recent meta-analysis of several clinical studies found no statistically significant difference in oral mucositis and acute GVHD severity in palifermin treated patients compared with those receiving placebo (89).
In the last 10 years it has become evident that a loss of diversity of the intestinal microbiota flora due to reduced food intake, chemotherapy-related damage, and antibiotics promotes the development of GVHD, which changed the dogma that antibiotics-based decontamination of the intestinal tract improves outcome post-allo-HCT. With the discovery that indoles and butyrate promote intestinal homeostasis the connection between antibiotics treatment and unfavorable outcome post-allo-HCT could be explained. Beyond bacterial species that were connected to GVHD, recent studies have also identified fungi and viruses that occurred more frequently in patients with severe GVHD. Based on this improved understanding on how the microbiome and the intestinal tract interact, novel strategies have been developed such as FMT that hold promise to overcome acute GVHD in a majority of patients. Ongoing clinical studies will provide important information on the role of FMT and regenerative strategies.
NK and RZ collected literature, discussed the studies, and wrote the manuscript.
The authors declare that the research was conducted in the absence of any commercial or financial relationships that could be construed as a potential conflict of interest.
We apologize to those investigators whose work could not be cited due to space restrictions.
1. D'Souza, Fretham C. Current Uses and Outcomes of Hematopoietic Cell Transplantation (HCT): CIBMTR Summary Slides (2017). Available online at: http://www.cibmtr.org
2. Zeiser R, Socie G, Blazar BR. Pathogenesis of acute graft-versus-host disease: from intestinal microbiota alterations to donor T cell activation. Br j haematol. (2016) 175:191–207. doi: 10.1111/bjh.14295
3. Martin PJ, Rizzo JD, Wingard JR, Ballen K, Curtin PT, Cutler C, et al. First- and second-line systemic treatment of acute graft-versus-host disease: recommendations of the american society of blood and marrow transplantation. Biol blood marrow transplant. (2012) 18:1150–63. doi: 10.1016/j.bbmt.2012.04.005
4. Westin JR, Saliba RM, De Lima M, Alousi A, Hosing C, Qazilbash MH, et al. Steroid-refractory acute GVHD: predictors and outcomes. Adv hematol. (2011) 2011:601953. doi: 10.1155/2011/601953
5. Castilla-Llorente C, Martin PJ, McDonald GB, Storer BE, Appelbaum FR, Deeg HJ, et al. Prognostic factors and outcomes of severe gastrointestinal GVHD after allogeneic hematopoietic cell transplantation. Bone marrow transplantat. (2014) 49:966–71. doi: 10.1038/bmt.2014.69
6. Schwab L, Goroncy L, Palaniyandi S, Gautam S, Triantafyllopoulou A, Mocsai A, et al. Neutrophil granulocytes recruited upon translocation of intestinal bacteria enhance graft-versus-host disease via tissue damage. Nat med. (2014) 20:648–54. doi: 10.1038/nm.3517
7. Socié G, Mary J-Y, Lemann M, Daneshpouy M, Guardiola P, Meignin V, et al. Prognostic value of apoptotic cells and infiltrating neutrophils in graft-versus-host disease of the gastrointestinal tract in humans: TNF and fas expression. Blood (2004) 103:50–7. doi: 10.1182/blood-2003-03-0909
8. Giroux M, Delisle JS, Gauthier SD, Heinonen KM, Hinsinger J, Houde B, et al. SMAD3 prevents graft-versus-host disease by restraining Th1 differentiation and granulocyte-mediated tissue damage. Blood (2011) 117:1734–44. doi: 10.1182/blood-2010-05-287649
9. Klämbt V, Wohlfeil SA, Schwab L, Hülsdünker J, Ayata K, Apostolova P, et al. A novel function for P2Y2 in myeloid recipient–derived cells during graft-versus-host disease. J Immunol. (2015) 195:5795–804. doi: 10.4049/jimmunol.1501357
10. Hulsdunker J, Ottmuller KJ, Neeff HP, Koyama M, Gao Z, Thomas OS, et al. Neutrophils provide cellular communication between ileum and mesenteric lymph nodes at graft-versus-host disease onset. Blood (2018) 131:1858–69. doi: 10.1182/blood-2017-10-812891
11. Jones JM, Wilson R, Bealmear PM. Mortality and gross pathology of secondary disease in germfree mouse radiation chimeras. Radiat Res. (1971) 45:577–88. doi: 10.2307/3573066
12. van Bekkum DW, Knaan S. Role of bacterial microflora in development of intestinal lesions from graft-versus-host reaction. J Natl Cancer Inst. (1977) 58:787–90. doi: 10.1093/jnci/58.3.787
13. van Bekkum DW, Roodenburg J, Heidt PJ, van der Waaij D. Mitigation of secondary disease of allogeneic mouse radiation chimeras by modification of the intestinal microflora. J Natl Cancer Inst. (1974) 52:401–4. doi: 10.1093/jnci/52.2.401
14. Buckner CD, Clift RA, Sanders JE, Meyers JD, Counts GW, Farewell VT, et al. Protective environment for marrow transplant recipients: a prospective study. Ann Intern Med. (1978) 89:893–901. doi: 10.7326/0003-4819-89-6-893
15. Petersen FB, Buckner CD, Clift RA, Nelson N, Counts GW, Meyers JD, et al. Prevention of nosocomial infections in marrow transplant patients: a prospective randomized comparison of systemic antibiotics versus granulocyte transfusions. Infect Control (1986) 7:586–92. doi: 10.1017/S0195941700065437
16. Petersen FB, Buckner CD, Clift RA, Lee S, Nelson N, Counts GW, et al. Laminar air flow isolation and decontamination: a prospective randomized study of the effects of prophylactic systemic antibiotics in bone marrow transplant patients. Infection (1986) 14:115–21. doi: 10.1007/BF01643474
17. Storb R, Prentice RL, Buckner CD, Clift RA, Appelbaum F, Deeg J, et al. Graft-versus-host disease and survival in patients with aplastic anemia treated by marrow grafts from HLA-identical siblings. beneficial effect of a protective environment. N Engl j med. (1983) 308:302–7. doi: 10.1056/NEJM198302103080602
18. Schmeiser T, Kurrle E, Arnold R, Krieger D, Heit W, Heimpel H. Antimicrobial prophylaxis in neutropenic patients after bone marrow transplantation. Infection (1988) 16:19–24. doi: 10.1007/BF01646924
19. Beelen DW, Haralambie E, Brandt H, Linzenmeier G, Muller KD, Quabeck K, et al. Evidence that sustained growth suppression of intestinal anaerobic bacteria reduces the risk of acute graft-versus-host disease after sibling marrow transplantation. Blood (1992) 80:2668–76.
20. Shono Y, Docampo MD, Peled JU, Perobelli SM, Velardi E, Tsai JJ, et al. Increased GVHD-related mortality with broad-spectrum antibiotic use after allogeneic hematopoietic stem cell transplantation in human patients and mice. Sci transl med. (2016) 8:339ra71. doi: 10.1126/scitranslmed.aaf2311
21. Weber D, Hiergeist A, Weber M, Dettmer K, Wolff D, Hahn J, et al. Detrimental effect of broad-spectrum antibiotics on intestinal microbiome diversity in patients after allogeneic stem cell transplantation: lack of commensal sparing antibiotics. Clin Infect Dis. (2018). doi: 10.1093/cid/ciy711. [Epub ahead of print].
22. Eckburg PB, Bik EM, Bernstein CN, Purdom E, Dethlefsen L, Sargent M, et al. Diversity of the human intestinal microbial flora. Science (2005) 308:1635–8. doi: 10.1126/science.1110591
23. Backhed F, Ley RE, Sonnenburg JL, Peterson DA, Gordon JI. Host-bacterial mutualism in the human intestine. Science (2005) 307:1915–20. doi: 10.1126/science.1104816
24. Holler E, Butzhammer P, Schmid K, Hundsrucker C, Koestler J, Peter K, et al. Metagenomic analysis of the stool microbiome in patients receiving allogeneic stem cell transplantation: loss of diversity is associated with use of systemic antibiotics and more pronounced in gastrointestinal graft-versus-host disease. Biol blood marrow transplant. (2014) 20:640–5. doi: 10.1016/j.bbmt.2014.01.030
25. Taur Y, Jenq RR, Perales MA, Littmann ER, Morjaria S, Ling L, et al. The effects of intestinal tract bacterial diversity on mortality following allogeneic hematopoietic stem cell transplantation. Blood (2014) 124:1174–82. doi: 10.1182/blood-2014-02-554725
26. Chiusolo P, Metafuni E, Paroni Sterbini F, Giammarco S, Masucci L, Leone G, et al. Gut microbiome changes after stem cell transplantation. Blood (2015) 126:1953.
27. Heimesaat MM, Nogai A, Bereswill S, Plickert R, Fischer A, Loddenkemper C, et al. MyD88/TLR9 mediated immunopathology and gut microbiota dynamics in a novel murine model of intestinal graft-versus-host disease. Gut (2010) 59:1079–87. doi: 10.1136/gut.2009.197434
28. Stein-Thoeringer C, Peled JU, Lazrak A, Slingerland AE, Shono Y, Staffas A, et al. Domination of the gut microbiota with Enterococcus species early after allogeneic bone marrow transplantation is an important contributor to the development of acute graft-versus-host disease (GHVD) in mouse and man. Biol Blood Marrow Transplant. (2018) 24:S40–1. doi: 10.1016/j.bbmt.2017.12.594
29. Jenq RR, Ubeda C, Taur Y, Menezes CC, Khanin R, Dudakov JA, et al. Regulation of intestinal inflammation by microbiota following allogeneic bone marrow transplantation. J exp med. (2012) 209:903–11. doi: 10.1084/jem.20112408
30. Gerbitz A, Schultz M, Wilke A, Linde HJ, Scholmerich J, Andreesen R, et al. Probiotic effects on experimental graft-versus-host disease: let them eat yogurt. Blood (2004) 103:4365–7. doi: 10.1182/blood-2003-11-3769
31. Gorshein E, Wei C, Ambrosy S, Budney S, Vivas J, Shenkerman A, et al. Lactobacillus rhamnosus GG probiotic enteric regimen does not appreciably alter the gut microbiome or provide protection against GVHD after allogeneic hematopoietic stem cell transplantation. Clin transplant. (2017) 31:e12947. doi: 10.1111/ctr.12947
32. Ladas EJ, Bhatia M, Chen L, Sandler E, Petrovic A, Berman DM, et al. The safety and feasibility of probiotics in children and adolescents undergoing hematopoietic cell transplantation. Bone marrow transplant. (2016) 51:262–6. doi: 10.1038/bmt.2015.275
33. Jenq RR, Taur Y, Devlin SM, Ponce DM, Goldberg JD, Ahr KF, et al. Intestinal blautia is associated with reduced death from graft-versus-host disease. Biol blood marrow transpl. (2015) 21:1373–83. doi: 10.1016/j.bbmt.2015.04.016
34. Mathewson ND, Jenq R, Mathew AV, Koenigsknecht M, Hanash A, Toubai T, et al. Gut microbiome-derived metabolites modulate intestinal epithelial cell damage and mitigate graft-versus-host disease. Nat immunol. (2016) 17:505–13. doi: 10.1038/ni.3400
35. Simms-Waldrip TR, Sunkersett G, Coughlin LA, Savani MR, Arana C, Kim J, et al. Antibiotic-induced depletion of anti-inflammatory clostridia is associated with the development of graft-versus-host disease in pediatric stem cell transplantation patients. Biol blood marrow transplant. (2017) 23:820–9. doi: 10.1016/j.bbmt.2017.02.004
36. Ubeda C, Bucci V, Caballero S, Djukovic A, Toussaint NC, Equinda M, et al. Intestinal microbiota containing barnesiella species cures vancomycin-resistant enterococcus faecium colonization. Infect Immun. (2013) 81:965–73. doi: 10.1128/IAI.01197-12
37. Andermann TM, Peled JU, Ho C, Reddy P, Riches M, Storb R, et al. The microbiome and hematopoietic cell transplantation: past, present, and future. Biol blood marrow transplant. (2018) 24:1322–40. doi: 10.1016/j.bbmt.2018.02.009
38. Shono Y, van den Brink MRM. Gut microbiota injury in allogeneic haematopoietic stem cell transplantation. Nat rev Cancer (2018) 18:283–95. doi: 10.1038/nrc.2018.10
39. Taur Y, Xavier JB, Lipuma L, Ubeda C, Goldberg J, Gobourne A, et al. Intestinal domination and the risk of bacteremia in patients undergoing allogeneic hematopoietic stem cell transplantation. Clin Infect Dis. (2012) 55:905–14. doi: 10.1093/cid/cis580
40. Nishi K, Kanda J, Hishizawa M, Kitano T, Kondo T, Yamashita K, et al. Impact of the use and type of antibiotics on acute graft-versus-host disease. Biol blood marrow transplant. (2018) 24:2178–83. doi: 10.1016/j.bbmt.2018.06.031
41. Derrien M, van Passel MW, van de Bovenkamp JH, Schipper RG, de Vos WM, Dekker J. Mucin-bacterial interactions in the human oral cavity and digestive tract. Gut Microbes (2010) 1:254–68. doi: 10.4161/gmic.1.4.12778
42. Derrien M, Van Baarlen P, Hooiveld G, Norin E, Muller M, de Vos WM. Modulation of mucosal immune response, tolerance, and proliferation in mice colonized by the mucin-degrader akkermansia muciniphila. Front Microbiol. (2011) 2:166. doi: 10.3389/fmicb.2011.00166
43. Gupta S, Allen-Vercoe E, Petrof EO. Fecal microbiota transplantation: in perspective. Therap Adv Gastroenterol. (2016) 9:229–39. doi: 10.1177/1756283X15607414
44. Qi X, Li X, Zhao Y, Wu X, Chen F, Ma X, et al. Treating steroid refractory intestinal acute graft-vs.-host disease with fecal microbiota transplantation: a pilot study. Front immunol. (2018) 9:2195. doi: 10.3389/fimmu.2018.02195
45. DeFilipp Z, Peled JU, Li S, Mahabamunuge J, Dagher Z, Slingerland AE, et al. Third-party fecal microbiota transplantation following allo-HCT reconstitutes microbiome diversity. Blood Adv. (2018) 2:745–53. doi: 10.1182/bloodadvances.2018017731
46. Li Y, Innocentin S, Withers DR, Roberts NA, Gallagher AR, Grigorieva EF, et al. Exogenous stimuli maintain intraepithelial lymphocytes via aryl hydrocarbon receptor activation. Cell (2011) 147:629–40. doi: 10.1016/j.cell.2011.09.025
47. Zelante T, Iannitti RG, Cunha C, De Luca A, Giovannini G, Pieraccini G, et al. Tryptophan catabolites from microbiota engage aryl hydrocarbon receptor and balance mucosal reactivity via interleukin-22. Immunity (2013) 39:372–85. doi: 10.1016/j.immuni.2013.08.003
48. Swimm A, Giver CR, DeFilipp Z, Rangaraju S, Sharma A, Ulezko Antonova A, et al. Indoles derived from intestinal microbiota act via type i interferon signaling to limit graft-versus-host-disease. Blood (2018) 32:2506–19. doi: 10.1182/blood-2018-03-838193
49. Donohoe DR, Garge N, Zhang X, Sun W, O'Connell TM, Bunger MK, et al. The microbiome and butyrate regulate energy metabolism and autophagy in the mammalian colon. Cell Metab. (2011) 13:517–26. doi: 10.1016/j.cmet.2011.02.018
50. Fujiwara H, Docampo MD, Riwes M, Peltier D, Toubai T, Henig I, et al. Microbial metabolite sensor GPR43 controls severity of experimental GVHD. Nat commun. (2018) 9:3674. doi: 10.1038/s41467-018-06048-w
51. Willemsen LE, Koetsier MA, van Deventer SJ, van Tol EA. Short chain fatty acids stimulate epithelial mucin 2 expression through differential effects on prostaglandin E(1) and E(2) production by intestinal myofibroblasts. Gut (2003) 52:1442–7. doi: 10.1136/gut.52.10.1442
52. Bilotta AJ, Ma C, Huang X, Yang W, Chen L, Yao S, et al. Microbiota metabolites SCFA promote intestinal epithelial repair and wound healing through promoting epithelial cell production of milk fat globule-EGF factor 8. J Immunol. (2018) 200(Suppl. 1):53.17.
53. Fukuda S, Toh H, Hase K, Oshima K, Nakanishi Y, Yoshimura K, et al. Bifidobacteria can protect from enteropathogenic infection through production of acetate. Nature (2011) 469:543–7. doi: 10.1038/nature09646
54. Inagaki T, Moschetta A, Lee YK, Peng L, Zhao G, Downes M, et al. Regulation of antibacterial defense in the small intestine by the nuclear bile acid receptor. Proc Natl Acad Sci USA. (2006) 103:3920–5. doi: 10.1073/pnas.0509592103
55. Liu L, Guo X, Rao JN, Zou T, Xiao L, Yu T, et al. Polyamines regulate E-cadherin transcription through c-Myc modulating intestinal epithelial barrier function. Am J Physiol Cell Physiol. (2009) 296:C801–10. doi: 10.1152/ajpcell.00620.2008
56. Varelias A, Bunting MD, Ormerod KL, Koyama M, Olver SD, Straube J, et al. Recipient mucosal-associated invariant T cells control GVHD within the colon. J clin invest. (2018) 128:1919–36. doi: 10.1172/JCI91646
57. Bansal T, Alaniz RC, Wood TK, Jayaraman A. The bacterial signal indole increases epithelial-cell tight-junction resistance and attenuates indicators of inflammation. Proc Natl Acad Sci USA. (2010) 107:228–33. doi: 10.1073/pnas.0906112107
58. Lee JS, Cella M, McDonald KG, Garlanda C, Kennedy GD, Nukaya M, et al. AHR drives the development of gut ILC22 cells and postnatal lymphoid tissues via pathways dependent on and independent of notch. Nat immunol. (2011) 13:144–51. doi: 10.1038/ni.2187
59. Qiu J, Heller JJ, Guo X, Chen ZM, Fish K, Fu YX, et al. The aryl hydrocarbon receptor regulates gut immunity through modulation of innate lymphoid cells. Immunity (2012) 36:92–104. doi: 10.1016/j.immuni.2011.11.011
60. Kiss EA, Vonarbourg C, Kopfmann S, Hobeika E, Finke D, Esser C, et al. Natural aryl hydrocarbon receptor ligands control organogenesis of intestinal lymphoid follicles. Science (2011) 334:1561–5. doi: 10.1126/science.1214914
61. Haak BW, Littmann ER, Chaubard JL, Pickard AJ, Fontana E, Adhi F, et al. Impact of gut colonization with butyrate-producing microbiota on respiratory viral infection following allo-HCT. Blood (2018) 131:2978–86. doi: 10.1182/blood-2018-01-828996
62. Venkataraman A, Sieber JR, Schmidt AW, Waldron C, Theis KR, Schmidt TM. Variable responses of human microbiomes to dietary supplementation with resistant starch. Microbiome (2016) 4:33. doi: 10.1186/s40168-016-0178-x
63. Jankovic D, Ganesan J, Bscheider M, Stickel N, Weber FC, Guarda G, et al. The Nlrp3 inflammasome regulates acute graft-versus-host disease. J exp med. (2013) 210:1899–910. doi: 10.1084/jem.20130084
64. Song X, Dai D, He X, Zhu S, Yao Y, Gao H, et al. Growth factor FGF2 cooperates with interleukin-17 to repair intestinal epithelial damage. Immunity (2015) 43:488–501. doi: 10.1016/j.immuni.2015.06.024
65. Lee JS, Tato CM, Joyce-Shaikh B, Gulen MF, Cayatte C, Chen Y, et al. Interleukin-23-independent IL-17 production regulates intestinal epithelial permeability. Immunity (2015) 43:727–38. doi: 10.1016/j.immuni.2015.09.003
66. Takashima S, Kadowaki M, Aoyama K, Koyama M, Oshima T, Tomizuka K, et al. The Wnt agonist R-spondin1 regulates systemic graft-versus-host disease by protecting intestinal stem cells. J exp med. (2011) 208:285–94. doi: 10.1084/jem.20101559
67. Hayase E, Hashimoto D, Nakamura K, Noizat C, Ogasawara R, Takahashi S, et al. R-spondin1 expands paneth cells and prevents dysbiosis induced by graft-versus-host disease. J exp med. (2017) 214:3507–18. doi: 10.1084/jem.20170418
68. Eriguchi Y, Takashima S, Oka H, Shimoji S, Nakamura K, Uryu H, et al. Graft-versus-host disease disrupts intestinal microbial ecology by inhibiting paneth cell production of alpha-defensins. Blood (2012) 120:223–31. doi: 10.1182/blood-2011-12-401166
69. Levine JE, Huber E, Hammer ST, Harris AC, Greenson JK, Braun TM, et al. Low paneth cell numbers at onset of gastrointestinal graft-versus-host disease identify patients at high risk for nonrelapse mortality. Blood (2013) 122:1505–9. doi: 10.1182/blood-2013-02-485813
70. Kagami S, Rizzo HL, Lee JJ, Koguchi Y, Blauvelt A. Circulating Th17, Th22, and Th1 cells are increased in psoriasis. J invest dermatol. (2010) 130:1373–83. doi: 10.1038/jid.2009.399
71. Kim KW, Kim HR, Park JY, Park JS, Oh HJ, Woo YJ, et al. Interleukin-22 promotes osteoclastogenesis in rheumatoid arthritis through induction of RANKL in human synovial fibroblasts. Arthritis rheum. (2012) 64:1015–23. doi: 10.1002/art.33446
72. Sugimoto K, Ogawa A, Mizoguchi E, Shimomura Y, Andoh A, Bhan AK, et al. IL-22 ameliorates intestinal inflammation in a mouse model of ulcerative colitis. J clin invest. (2008) 118:534–44. doi: 10.1172/JCI33194
73. Zenewicz LA, Yancopoulos GD, Valenzuela DM, Murphy AJ, Stevens S, Flavell RA. Innate and adaptive interleukin-22 protects mice from inflammatory bowel disease. Immunity (2008) 29:947–57. doi: 10.1016/j.immuni.2008.11.003
74. Wu J, Gu J, Zhou S, Lu H, Lu Y, Lu L, et al. Anti-IL-22 antibody attenuates acute graft-versus-host disease via increasing Foxp3(+) T cell through modulation of CD11b(+) cell function. J Immunol Res. (2018) 2018:1605341. doi: 10.1155/2018/1605341
75. Gartlan KH, Bommiasamy H, Paz K, Wilkinson AN, Owen M, Reichenbach DK, et al. A critical role for donor-derived IL-22 in cutaneous chronic GVHD. Am J Transplant. (2018) 18:810–20. doi: 10.1111/ajt.14513
76. Hanash AM, Dudakov JA, Hua G, O'Connor MH, Young LF, Singer NV, et al. Interleukin-22 protects intestinal stem cells from immune-mediated tissue damage and regulates sensitivity to graft versus host disease. Immunity (2012) 37:339–50. doi: 10.1016/j.immuni.2012.05.028
77. Lindemans CA, Calafiore M, Mertelsmann AM, O'Connor MH, Dudakov JA, Jenq RR, et al. Interleukin-22 promotes intestinal-stem-cell-mediated epithelial regeneration. Nature (2015) 528:560–4. doi: 10.1038/nature16460
78. Zhao D, Kim YH, Jeong S, Greenson JK, Chaudhry MS, Hoepting M, et al. Survival signal REG3alpha prevents crypt apoptosis to control acute gastrointestinal graft-versus-host disease. J clin invest. (2018) 28:4970–9. doi: 10.1172/JCI99261
79. Ferrara JL, Harris AC, Greenson JK, Braun TM, Holler E, Teshima T, et al. Regenerating islet-derived 3-alpha is a biomarker of gastrointestinal graft-versus-host disease. Blood (2011) 118:6702–8. doi: 10.1182/blood-2011-08-375006
80. Zheng Y, Valdez PA, Danilenko DM, Hu Y, Sa SM, Gong Q, et al. Interleukin-22 mediates early host defense against attaching and effacing bacterial pathogens. Nat med. (2008) 14:282–9. doi: 10.1038/nm1720
81. Sanos SL, Bui VL, Mortha A, Oberle K, Heners C, Johner C, et al. RORgammat and commensal microflora are required for the differentiation of mucosal interleukin 22-producing NKp46+ cells. Nat immunol. (2009) 10:83–91. doi: 10.1038/ni.1684
82. Rashidi A, Shanley R, Yohe SL, Thyagarajan B, Curtsinger J, Anasetti C, et al. Recipient single nucleotide polymorphisms in paneth cell antimicrobial peptide genes and acute graft-versus-host disease: analysis of BMT CTN-0201 and−0901 samples. Br j haematol. (2018) 182:887–94. doi: 10.1111/bjh.15492
83. Housley RM, Morris CF, Boyle W, Ring B, Biltz R, Tarpley JE, et al. Keratinocyte growth factor induces proliferation of hepatocytes and epithelial cells throughout the rat gastrointestinal tract. J clin invest. (1994) 94:1764–77. doi: 10.1172/JCI117524
84. Khan WB, Shui C, Ning S, Knox SJ. Enhancement of murine intestinal stem cell survival after irradiation by keratinocyte growth factor. Radiat Res. (1997) 148:248–53. doi: 10.2307/3579609
85. Panoskaltsis-Mortari A, Lacey DL, Vallera DA, Blazar BR. Keratinocyte growth factor administered before conditioning ameliorates graft-versus-host disease after allogeneic bone marrow transplantation in mice. Blood (1998) 92:3960–7.
86. Krijanovski OI, Hill GR, Cooke KR, Teshima T, Crawford JM, Brinson YS, et al. Keratinocyte growth factor separates graft-versus-leukemia effects from graft-versus-host disease. Blood (1999) 94:825–31.
87. Blazar BR, Weisdorf DJ, Defor T, Goldman A, Braun T, Silver S, et al. Phase 1/2 randomized, placebo-control trial of palifermin to prevent graft-versus-host disease (GVHD) after allogeneic hematopoietic stem cell transplantation (HSCT). Blood (2006) 108:3216–22. doi: 10.1182/blood-2006-04-017780
88. Jagasia MH, Abonour R, Long GD, Bolwell BJ, Laport GG, Shore TB, et al. Palifermin for the reduction of acute GVHD: a randomized, double-blind, placebo-controlled trial. Bone marrow transplant. (2012) 47:1350–5. doi: 10.1038/bmt.2011.261
89. Mozaffari HR, Payandeh M, Ramezani M, Sadeghi M, Mahmoudiahmadabadi M, Sharifi R. Efficacy of palifermin on oral mucositis and acute GVHD after hematopoietic stem cell transplantation (HSCT) in hematology malignancy patients: a meta-analysis of trials. Contemp Oncol. (2017) 21:299–305. doi: 10.5114/wo.2017.72400
Keywords: GVHD, allo-HCT, microbiota, intestinal inflammation, microbial metabolites, Paneth cells
Citation: Köhler N and Zeiser R (2019) Intestinal Microbiota Influence Immune Tolerance Post Allogeneic Hematopoietic Cell Transplantation and Intestinal GVHD. Front. Immunol. 9:3179. doi: 10.3389/fimmu.2018.03179
Received: 26 October 2018; Accepted: 27 December 2018;
Published: 17 January 2019.
Edited by:
Everett Meyer, Stanford University, United StatesReviewed by:
Teresa Zelante, University of Perugia, ItalyCopyright © 2019 Köhler and Zeiser. This is an open-access article distributed under the terms of the Creative Commons Attribution License (CC BY). The use, distribution or reproduction in other forums is permitted, provided the original author(s) and the copyright owner(s) are credited and that the original publication in this journal is cited, in accordance with accepted academic practice. No use, distribution or reproduction is permitted which does not comply with these terms.
*Correspondence: Robert Zeiser, cm9iZXJ0LnplaXNlckB1bmlrbGluaWstZnJlaWJ1cmcuZGU=
Disclaimer: All claims expressed in this article are solely those of the authors and do not necessarily represent those of their affiliated organizations, or those of the publisher, the editors and the reviewers. Any product that may be evaluated in this article or claim that may be made by its manufacturer is not guaranteed or endorsed by the publisher.
Research integrity at Frontiers
Learn more about the work of our research integrity team to safeguard the quality of each article we publish.