- 1Department of Animal Biology, School of Life Science, University of Hyderabad, Hyderabad, India
- 2Division of Emerging and Transfusion Transmitted Diseases, Center for Biologics Evaluation and Research, Food and Drug Administration, Silver Spring, MD, United States
Leptin, a pleiotropic protein has long been recognized to play an important role in the regulation of energy homeostasis, metabolism, neuroendocrine function, and other physiological functions through its effects on the central nervous system (CNS) and peripheral tissues. Leptin is secreted by adipose tissue and encoded by the obese (ob) gene. Leptin acts as a central mediator which regulates immunity as well as nutrition. Importantly, leptin can modulate both innate and adaptive immune responses. Leptin deficiency/resistance is associated with dysregulation of cytokine production, increased susceptibility toward infectious diseases, autoimmune disorders, malnutrition and inflammatory responses. Malnutrition induces a state of immunodeficiency and an inclination to death from communicable diseases. Infectious diseases are the disease of poor who invariably suffer from malnutrition that could result from reduced serum leptin levels. Thus, leptin has been placed at the center of many interrelated functions in various pathogenic conditions, such as bacterial, viruses and parasitic infections. We review herein, the recent advances on the role of leptin in malnutrition in pathogenesis of infectious diseases with a particular emphasis on parasitic diseases such as Leishmaniasis, Trypanosomiasis, Amoebiasis, and Malaria.
Introduction
Leptin is a hormone derived from adipocytes in response to the nutritional status, and it signals to the central nervous system (CNS) and peripheral organs (1). The circulating plasma leptin concentrations are mostly influenced by the total body fat mass index, metabolic hormones, and gender. Women have higher concentrations of circulating leptin compared to men (2). The central function of leptin is metabolic homeostasis that can be attained by the delivery of information about the total body fat mass to the hypothalamus that in turn alters the CNS function and regulates glucocorticoids, insulin hormone and food intake & energy balance (3, 4). Concurrently, leptin is also a critical regulator of immunity and functions as a pro-inflammatory cytokine-like interleukin (IL)-1, IL-6, IL-8, IL-18, and tumor necrosis factor-α (TNF-α), and its deficiency increases susceptibility to infectious (5–8).
Leptin was identified as the gene defect responsible for the obesity syndrome in Leptin-deficient (ob/ob) mice and reported as the product of Ob gene (9, 10). Leptin is a 16 kDa α-helix type protein like the long-chain helical cytokine family such as IL-6, IL-2, IL-12, leukocyte inhibitory factor (LIF), Granulocyte-colony stimulating factor (G-CSF), Ciliary neurotrophic factor (CNTF), and Oncostatin M (11, 12). Most of the biological functions of leptin are exerted through leptin receptor (Ob-R) signaling via the Janus kinase/signal transducer and activator of transcription (JAK/STAT) pathway (13, 14). In general, leptin enhances the immune response via activating antigen presenting cells (APCs), Th1 cells function and proliferation, and mediating the secretion of the pro-inflammatory cytokines, such as TNF-α, IL-2, or IL-6 (7, 15, 16). Leptin-deficient (ob/ob; double knockout obese gene) and leptin receptor-deficient (db/db; double knockout obese receptor gene) mice display marked reduction in the size & thymic atrophy and exhibit defective immune responses (17, 18). Similarly, reduced leptin levels in starved and malnourished individuals is further associated with alterations of the immune response and thymic atrophy. However, these conditions can be reversed by leptin administration (19–22).
Over the past decade, the role leptin in infectious diseases was extensively explored. It has been reported that leptin deficiency is correlated with starvation or nutritional deprivation/malnutrition (23). Malnutrition affects both innate & acquired immunity of the host (24) thereby increasing the incidences of infections and mortality (25). The immune dysfunction in malnutrition or restricted calorie intake reduced the memory T cells, total CD4+ and CD8+ T cell numbers compared to well-nourished infected controls (26–29). Interestingly, leptin has a crucial role in mediating phagocytosis, T cell number, function, and metabolism in both obesity and malnutrition. The systemic circulating leptin deficiency in malnutrition is also correlated with several other bacterial, viral and parasitic infections such as tuberculosis (30), pneumonia (31), sepsis (32), colitis (33), viral infection (34, 35) leishmaniasis (36), trypanosomiasis (37), amoebiasis (38), and malaria (39) due to defective cytokine production (40–45). Hence, nutritional status is critically essential for immune cell function in both malnutrition and infection. Understanding how leptin is altered in malnutrition and infection will lead to better insight of and treatment for diseases where nutritional status determines clinical outcome. Furthermore, there has been increasing evidence that leptin is involved in the pathogenesis of various infectious diseases. In the present review, we will discuss the emerging role of leptin in different infectious diseases and will further highlight how malnutrition or starvation could play a role.
Leptin and Immunity
Leptin is a pleiotropic molecule, which can function as a hormone as well as cytokine (adipokine). Almost all immune cells such as neutrophils, monocytes, lymphocytes express leptin receptor and it belongs to the family of class-I cytokine receptors (46–48). Leptin regulates angiogenesis, hematopoiesis, innate & adaptive immunity and induces the Th1 response by increasing IFN-γ, IL-2, and TNF-α production, subsequently leading to the activation of monocyte/macrophages and prevents the apoptosis of various immune cells by delaying the cleavage of Bid and Bax (49–55).
In innate immunity, leptin enhances the activity and function of neutrophils by the release of oxygen free radicals, increased CD11b expression and intercellular adhesion molecule-1 (ICAM-1), which leads to migration of immune cells at the sites of inflammation (56–58). Leptin activates the monocytes and dendrite cells (DCs) that in turn leads to the production of pro-inflammatory cytokines such as TNF-α, IL-6 along with IL-12, a key cytokine that facilitates the shifting of T-cells toward the Th1 phenotype (59–62). Leptin also promotes DCs survival by triggering the activation of nuclear factor-kappa B (NF-kappa B) and up-regulates B-cell lymphoma 2 (Bcl-2) and B-cell lymphoma-extra-large (Bcl-xL) gene expression via the PI3K-Akt signaling pathway (62). Moreover, upon leptin stimulation, DCs also exhibit increased production of multiple cytokines including IL-1, IL-6, IL-12, TNF-α, MIP-1α and induces the expression of surface molecules, such as CD1a, CD80, CD83, or CD86 (63, 64). Indirectly, leptin leads to the activation of natural killer (NK) cells upon modulation of IL-1, IL-6, and TNF-α via monocytes and macrophages (61) resulting an increased IL-12 and reduced IL-15 expression in NK cells (65, 66).
In adaptive immunity, leptin induces the maturation and survival of thymic T-cells by reducing their rate of apoptosis through inhibition of FAS-directed apoptosis pathway (16, 67). Eventually, leptin has anti-apoptotic effects on mature T-cells, by up-regulating the expression of Bcl-xL (68), T-box transcription factor (T-bet) (69), and synergizes with other cytokines in lymphocyte proliferation and activation possibly via signal transducer and activator of transcription 3 (STAT3) signaling (70, 71). Leptin deficiency in both mouse and human results in severe immune defects characterized by decrease in total lymphocytes, CD4+ helper T cell number, increased thymocyte apoptosis, and a skewing away from the Th1 toward Th2 phenotype thereby resulting in increased susceptibility to intracellular infections (16, 17, 72–74). Leptin mediates T-cells polarization by inducing the cell-mediated immune response through the secretion of IL-2, IL-12, TNF-α, and IFN-γ from Th1 cells and suppresses the production of IL-10 and IL-4 from Th2 cells (13, 75–78). In facts, thymocytes treated with leptin induces CD4+CD8+ cell differentiation mainly to CD4+ mature thymocytes (79). Additionally, leptin also activates human B cells to secrete cytokines, such as IL-6, IL-10, and TNF-α, through the activation of JAK2/STAT3 and p38MAPK/ERK1/2 signaling pathways (80, 81). Leptin-STAT3 signaling also influences the production of C-X-C chemokine receptor type 3 (CXCR3) and C-C chemokine receptor type 5 (CCR5) ligands, which are preferentially expressed on Th1 cells and enhances pro-inflammatory cytokines such as IL-1β, TNF-α, and IL-6 in serum (39, 82, 83). In conclusion, leptin acts as a Th1 cytokine and regulates all immune cells through leptin receptor and affects innate & adaptive immune responses together facilitating a shift toward Th1 response. The brief role of leptin in innate & adaptive immune response was summarized in Figure 1.
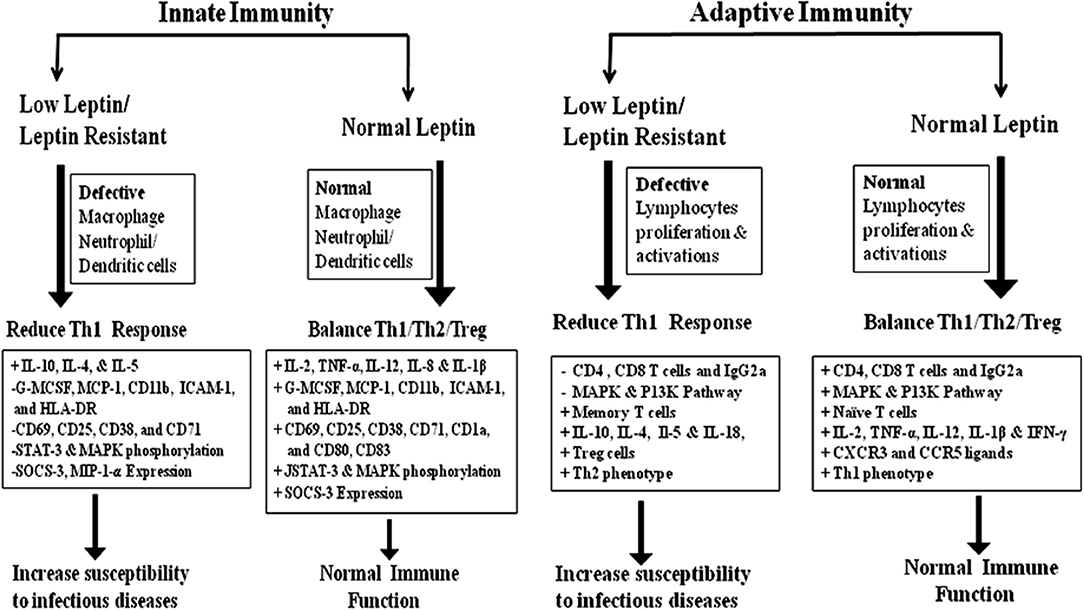
Figure 1. The role of leptin in Innate and adaptive immunity: innate immunity; Leptin plays a crucial role in the activation and proliferation of macrophage, neutrophil, and dendritic cells through the up and down regulation of various cytokine/chemokines. Adaptive immunity; Leptin-induced the activation and proliferation of total lymphocytes (T and B cells), regulatory T cells and Naive T cells through the up and down regulation of pro-inflammatory and anti-inflammatory cytokines. The Innate and adaptive immune response induced by leptin signaling through the phosphorylation of MAKP/STAT-3/P13K pathways (15–18, 49–55).
Leptin in Malnutrition
Nutritional deficiency impaired phagocyte function, cell-mediated immunity, cytokine production, antibody response and the complement system (24, 25, 84) and predisposed to death from infectious diseases (85). Concurrently, malnutrition is the most common cause of secondary immunodeficiency worldwide associated with protein-energy malnutrition (PEM) (86, 87), a nutritional deficiency in which individuals suffer from protein but not calorific malnutrition (88). Strikingly, PEM causes a drastic reduction in body fat mass and decreases the circulating concentration of leptin, which, in turn, impairs the generation of proinflammatory mediators [IFN-γ, TNF-α and (NO) nitric oxide] (89, 90), and increases the incidence of infectious diseases (72, 83). Malnutrition is a primary risk factor for many infectious diseases. From recent research, it seems that malnutrition is a predictor of tuberculosis disease and is associated with worse outcomes. Active tuberculosis is correlated with weight loss, cachexia, and low serum concentrations of leptin which in turn suppresses the lymphocyte stimulation and Th1 cytokines such as IL-2, IFN-γ, and TNF-α secretion (91–96). PEM significantly reduced the lymphocyte stimulation as well as secretion of the Th1 cytokines such as IL-2, IFN-γ, and TNF-α, in M. tuberculosis-infected guinea pig (92, 97). Furthermore, PEM also diminishes leptin concentrations and increases serum levels of stress hormones, i.e., glucocorticoids which impairs macrophage functions by limiting NF-kB translocation into the nucleus (96). Macrophages from experimental PEM mice are less sensitive to lipopolysaccharides (LPS) due to decreased NF-kB translocation resulting in impairment of active phagocytosis, cytokines response and reactive oxygen intermediates (ROIs) productions (98–101).
Serum leptin concentration decreases as malnutrition becomes more pronounced and thus serves as a biomarker of poor nutritional status in chronic cirrhosis due to viral hepatitis and candidiasis due to Candida albicans (102–105). Moreover, during fasting or starvation, leptin levels also fall disproportionately due to the decrease in adipose tissue fat mass (106, 107). The decrease in leptin level during starvation rendered wild-type mice susceptible to LPS and TNF-α induced lethality whereas leptin treatment restores those changes despite ongoing starvation, suggesting that the lack of leptin plays a role in the immune dysfunction during starvation (18, 108). Exogenous leptin administration modulates T cell responses in mice and prevents starvation-induced immune suppression on the development of a delay type of hypersensitivity (DTH) response and protects from starvation-induced lymphoid atrophy in mice (12, 15, 68, 109).
Taken together, Leptin is a protein hormone secreted by adipocytes, regulating body fat and food intake through neuroendocrine-signaling system. Hence, it is possible to speculate that leptin might act in the brain to directly regulate metabolic response along with peripheral immune function thereby contributing to better outcomes in various infectious diseases compared with states of relative or total leptin deficiency. Importantly, recent studies reported that serum leptin level may be used as a promising diagnostic or prognostic marker for critical illness sepsis that is triggered by an infective agent such as bacteria, viruses, fungi, or parasites (110). Therefore, from here onwards, the review focuses on the role of leptin in various infectious diseases.
Leptin and Bacterial Infections
Leptin-deficient mice and mice rendered leptin-deficient by fasting exhibit impaired pulmonary bacterial clearance (Table 1) and enhanced lethality during pulmonary tuberculosis, bacterial pneumonia, and sepsis (30–32). The patients with pulmonary tuberculosis (PTB) have decreased serum leptin levels, and an increase in adiponectin may serve as a reliable biomarker for predicting the development and progression of PTB pathogenesis (111, 145, 146). Mycobacterium infection in ob/ob mice was hampered to produce organized granulomatous response and defective in CD4+ and CD8+ T cells functions, IFN-γ and DTH responses (30). The mechanisms underlying the defective leukocyte effector function in cells from leptin-deficient mice were associated with a reduction in leukotriene (LT) synthesis in alveolar macrophage (AMs), reduced complement receptor (CR3) expression and decreased H2O2 synthesis in neutrophils (PMNs) infected with Klebsiella pneumoniae (112–114). Restoring the level of circulating leptin to physiological levels in fasted and ob/ob mice significantly improved the survival and pulmonary bacterial clearance, reduced bacteraemia, reconstituted alveolar macrophage phagocytosis and increased H2O2 production in the PMNs resulting in increased killing of S. pneumonia in vitro (31, 115). Leptin binding to leptin receptor activates multiple intracellular signaling pathways, including STAT3, STAT5, and ERK1/2. STAT3 activates transcription of suppressor of cytokine signaling (SOCS)-3, a protein that inhibits JAK2 and STAT3 signaling during prolonged stimulation of the Leptin receptor long isoform (Lep-Rb) (147, 148). Lep-Rb mediated phosphorylation of Tyr1077 activates STAT5 signaling pathway (149). Phosphorylation of Tyr985 in leptin receptor recruits binding partners SH2-containing tyrosine phosphatase (SHP-2) and growth factor binding 2 (GRB2) which activate extracellular signal-regulated kinase 1 and 2 (ERK 1/2) signaling (147, 150). A mutation of the Try985 with L985 residue in the leptin receptor exhibited increased mortality and impaired pulmonary bacterial clearance following an intratracheal challenge with K. pneumoniae due to the disruption of ERK-dependent activation (151).
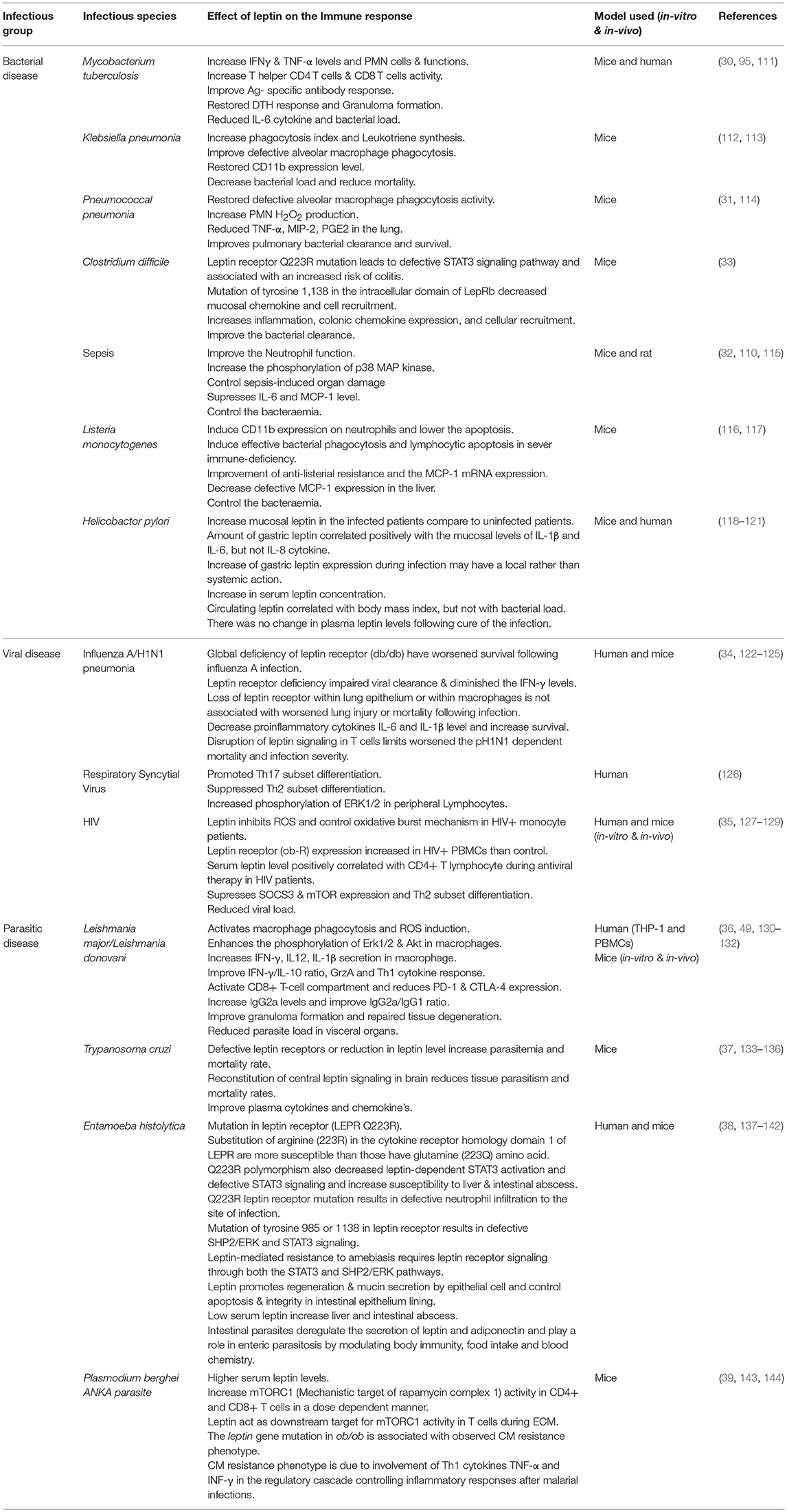
Table 1. The distinctive immune responses in various infectious diseases upon leptin treatment were summarized.
Moreover, Leptin-dependent neutrophilic phagocytosis of L. monocytogenes was more potent than Escherichia coli due to the presence of apoptotic factor Listeriolysin O, which is absent in E. coli (116). Exogenous leptin restored the anti-listeria resistance and monocyte chemoattractant protein-1 (MCP-1) and MIP-2 production in leptin-deficient mice (117, 152). Clostridium difficile colitis is a primary causative agent of nosocomial infection in humans and murine. The defective STAT3 signaling pathway leads to susceptibility to infectious colitis and bacterial peritonitis (33, 153) and leptin treatment restored the protective mucosal immune response in C. difficile colitis by the STAT3 inflammatory pathway (33).
In contrast to above finding, disruption of leptin receptor-mediated STAT3 signaling pathway improved AMs phagocytosis and host defense against P. pneumonia in (Leprs1138/s1138) s/s mice following an intratracheal challenge with S. pneumonia (154). These effects are mediated by an intracellular signaling pathway that is dependent on ERK1/2 activation in AMs resulting an increased in LT synthesis, which enhanced the phagocytosis in cells from s/s mice (154). Mice infected with Helicobacter pylori-induced pro-inflammatory cytokine response and enhanced the leptin secretion from gastric mucosa which may be playing a role in weight gain after eradication of H pylori infection (118–120) suggesting that leptin has a local effect rather than systemic action in patients with gastritis (121, 155). These findings reveal the existence of a relevant neuroendocrine control of leptin in systemic immune defense in various bacterial diseases thereby highlighting the possible therapeutic potential of leptin analogous to control infectious diseases.
Leptin and Viral Infections
Mice deficient in the leptin receptor or malnourished leads to impaired viral clearance (Table 1), diminished lung IFNγ level and reduced survival during influenza-A pneumonia infections (156). The mice lacking functional leptin receptor in T cells (LepRT−/−) limits pH1N1 influenza mortality and infection severity in obese mice suggesting that leptin signaling in T cells may be a critical mediator of pH1N1 severity in obese mice (122, 123). Moreover, Leptin resistant obese mice or decreased leptin level in obese individuals may increase the susceptibility to influenza virus infection by suppressing the memory T-cell function and IFN-α, IFN-β, and IFN-γ mRNA expression which leads to an increase in viral titer and infiltration (124). Furthermore, mice lacking functional leptin receptor in tissue- specific lung epithelial and macrophage cells have improved viral clearance and reduced lung injury following influenza-A infection suggesting that leptin signaling is also associated with non-myeloid cells such as natural killer cells and T cells (125). Leptin significantly upregulated the Th17 subset but suppressed Th2 subset differentiation possibly via regulating ERK1/2 phosphorylation in human bronchial epithelial cells (hBECs) infected with the respiratory syncytial virus (RSV) (126). Human immunodeficiency virus (HIV)-infected patients have an exaggerated expression of leptin receptor on their blood mononuclear cells while low leptin levels in their serum leads to an immune deficiency in these patients (127, 128). Importantly, leptin therapy, a novel strategy now in clinical trials, and its beneficial positive role in HIV patients in correction of metabolic complications related to HIV-associated lipodystrophy syndrome (HALS) has been reported recently (129). Leptin also diminishes the oxidative status of monocytes suggesting that leptin can alter the redox status of monocytes, which leads to immunological alterations in HIV infection (35). Therefore, taken together these findings reveal that leptin could control the systemic immune defense failure in viral specific immune cells dysfunction and further suggests the possible healing potential for leptin analogs in infectious disease.
Leptin and Parasitic Infections
Parasitic infections contribute significantly to the burden of communicable diseases worldwide. Reportedly, much of infections and mortality from parasitic illnesses are restricted mainly in developing countries (157). Malnutrition or loss of appetite is a common characteristic of many infectious diseases including parasitic infections which result in reduced serum leptin levels (158). Since, leptin has been reported to induce pro-inflammatory cytokines & chemokines, neutrophil chemotaxis, NK cell cytotoxicity, and T cell functions, therefore its deficiency leads to an increase in susceptibility to infectious diseases (64, 65, 159, 160). Furthermore, leptin exerts central effects on hypothalamic-pituitary function and disruption of these effects have been implicated into severe parasitic diseases due to immune dysfunction in host (35–38).
Moreover, very little is known about the role of leptin in pathogenesis of parasitic infections. There is a need for studying the role of leptin in controlling parasitic infections since preponderance of such infections is associated with malnutrition which goes hand in hand in developing countries. As a first step, we have highlighted some of these studies to generate interest in initiating such studies (Table 1).
Leishmaniasis
Leishmaniasis is a vector-borne protozoan disease caused by Leishmania parasites. Leishmaniasis is commonly prevalent in tropical and subtropical regions of the world with different immunopathology and varying degrees of morbidity and mortality. Among which, Visceral Leushmaniasis (VL) is the deadliest form of the diseases, marked by uncontrolled parasitemia in the spleen, liver, and bone marrow (161, 162).
VL is an endemic disease found mostly in economically poor societies, who invariably suffer from malnutrition. Malnutrition is characterized by the lower serum leptin level which in turn adversely alters the development of innate and adaptive immune responses during VL in both mice and children living in endemic areas (163–165). Hence, malnutrition and low serum leptin level are playing a critical role in Leishmania infection and its pathogenesis. Leptin improved cytokine production and phagocytosis of Leishmania donovani by murine and human macrophages by increasing the phagolysosome formation and oxidative killing of the parasite via intracellular reactive oxygen species (ROS) generation (36). Leptin in combination with miltefosine, the conventional antileishmanial drug, augments the protective immunity in mouse macrophage during L. donovani infection in vitro (130). Similarly, it enhances the host protective Th1 cytokine responses in THP-1, and human PMBCs derived macrophages by inducing Erk1/2 and Akt phosphorylation, which is usually dephosphorylated in L. donovani infection (36). It can also maintain the protective environment against L. donovani infection through the classical macrophage activation (36). Recently we demonstrated that leptin induces the innate immune response in bone marrow-derived antigen presenting dendritic cells, and causes heightened nitric oxide, proinflammatory cytokines (IFN-γ, IL-12, and IL1β) in the splenocytes stimulated with soluble Leishmania antigen. Besides this, leptin-induced IFN-γ production from both CD4+ and CD8+ T cells compared with untreated infected normal mice, indicates that leptin-induced heightened Th1 response (131). Alternatively, leptin deficient ob/ob mice had higher splenic and liver parasite burden compared with the normal infected mice. Nevertheless, leptin treatment of ob/ob mice failed to reduce the splenic parasite burden and host-protective cytokine response. Moreover, in contrast to DCs from a normal mouse, ob/ob mouse-derived DCs showed limitation in the initiation of innate immune response during Leishmania infection that could not be restored by leptin treatment suggesting that leptin signaling was differentially regulated in ob/ob mice compared with normal mice fed with healthy diet (131, 166). Interestingly, very recently we demonstrated that leptin also induces a protective CD8+ T-cell dependent immune response in malnutrition coupled with L. donovani infection through up-regulation of Granzyme A (GrzA) and down-regulation of cytotoxic T-lymphocyte-associated protein 4 (CTLA-4) and Programmed death-1 (PD-1) markers (132). The PD-1 ligand plays a significant role in CD8+ T cell exhaustion during the chronic infections of various infectious diseases including VL (167). It is worth mentioning here that in contrast to the above reports an increased expression or activity of leptin, has been reported in blood samples of dogs with canine leishmaniasis (CanL) and suggested possible use of leptin as a biomarker for CanL (168). In conclusion, leptin treatment may improve parasite clearance in malnourished VL condition through restoration of normal immune cell function via leptin signaling.
Trypanosomiasis
Chagas disease is caused by a protozoan parasite Trypanosoma cruzi. The parasite is transmitted to humans and other hosts mainly by feces of infected blood-feeding triatomines, blood transfusion, or by ingestion of contaminated food (169). Chagas disease remains a serious health problem in Central and South America and a major cause of morbidity and mortality (135, 170). T. cruzi uses adipocytes as a reservoir for chronic infection and displays a pro-inflammatory phenotype by upregulating cytokines such as IL-1β, IFN-γ, TNF-α, and chemokines such as CCL2, CXCL10, and CCL5 along with innate immune receptors such as Toll-like receptor (TLR)-2 and 9 (171–173). Other pathways, such as ERK and PI3K pathways were also activated upon T. cruzi infection (174, 175). Additionally, Adipose tissue profoundly expressed Peroxisome proliferator-activated receptor (PPAR-γ) along with adiponectin, which exerts an anti-inflammatory effect. The levels of PPAR-γ were also decreased in T. cruzi infected cells, which leads to the reduced secretion of adiponectin and increased inflammatory reactions (176).
Since parasite infects many organs including adipose tissue which is a source to a variety of adipokines, including leptin and could have significant role in pathogenesis of Trypanosomiasis (133, 134). Mice infected with T. cruzi showed significant reduction in leptin levels, possibly due to adipocyte involvement in disease progression (133, 135). It was also reported that chemically induced diabetic mice and genetically susceptible db/db diabetic mice with defective leptin receptors had higher parasitemia and mortality after T. cruzi infection, which suggests that the dysregulation of host metabolism may be beneficial to parasitic survival in the host (136). Moreover, mice with defective leptin receptor are metabolically challenged and upon infection with T. cruzi suffer high mortality (37). In NSE-Rb db/db mice, a genetically modified db/db mouse, central leptin signaling is reconstituted only in the brain which is sufficient to correct the metabolic defects and when infected with T. cruzi showed reduced parasitemia, mortality rates, and tissue parasitism as compared to normal db/db mice. The plasma levels of several cytokines and chemokines were also significantly increased in infected db/db mice compared with NSE-Rb db/db mice (37). In summary, the normalization of the metabolic dysfunction in NSE-Rb db/db mice through the restoration of leptin receptor signaling in brain reconstitute the normal immune response against T. cruzi infection, but not peripheral restoration, highlighting that leptin may play a role as a central regulator for both metabolic function and immune response.
Amoebiasis
Amoebiasis is the disease caused by an enteric protozoan parasite Entamoeba histolytica. Infection results from ingestion of the parasite cyst from feces-contaminated food or water (177). E. histolytica primarily lives in the intestinal mucosa and mainly restricted in colon infection causing devastating dysentery, colitis, and liver abscess by producing tissue damages (178, 179) while many deaths are associated with extraintestinal invasive disease (180, 181). Amoebiasis occurs when trophozoites disrupt the mucosal barrier and penetrate the underlying tissue and break down extracellular matrix, destroy cells, and phagocytose cellular debris (182). Studies on amoebic liver abscess (ALA) carried out in Indian subcontinent suggest that malnutrition is associated with ALA outcome (183, 184).
Moreover, malnutrition and serum leptin levels are directly proportional to the pathogenesis of amoebiasis, and low serum leptin plays a critical role in E. histolytica- associated diarrheal illness and extent of liver injury (137, 185). The mucosal immune response can be suppressed by a mutation in leptin receptor and defective STAT3 signaling pathways, resulting in susceptibility to intestinal abscess due to E. histolytica infections (38, 138). The mechanism of mucosal immune suppression depends on homozygous allelic mutation in leptin receptor Q223R (rs1137101) that ablates STAT3 signaling, results in decreased mucosal chemokine's LIF, CXCL9, CXCL10, CCL3, and CCL4 secretion (68, 69). A mutation or polymorphism in leptin receptor at 233 (from glutamine to arginine) is liable to enhance 4 times more susceptibility to E. histolytica infection in children irrespective of nutritional status (139). The leptin-deficient (ob/ob) and LepRb-deficient (db/db) mice were highly susceptible to infection with E. histolytica, whereas wild-type C57BL/6 mice were resistant. Moreover, mice either homozygous or heterozygous for the 223R allele of leptin receptor were significantly more prone to amoebic infection. Both types of mice ceca were shown to have profound epithelial denudation because of trophozoites invasion. Leptin signaling in the intestinal epithelium and downstream STAT3 and SHP2 (Src homology phosphatase 2) signaling was required for protection in the murine model of amoebic colitis (140). Leptin-mediated specific activation of STAT3 and ERK or Akt signaling pathways in gut mucosal epithelial cells offers more resistance against amoebiasis caused by E. histolytica infection (141, 142). In conclusion, leptin may control the amoebic infections by the activation of leptin signaling pathway in gut mucosal epithelial cells via up-regulation of various signaling pathway.
Malaria
Malaria is caused by Plasmodium species, an intracellular parasite transmitted by the bite of an infected female Anopheles mosquito. It is endemic in most of the tropical countries such as sub-tropical regions of Asia, Africa, South and Central America (186). Plasmodium falciparum is the most virulent form of the human malaria parasites and responsible for 90% of malaria-related morbidity and mortality (187). During a mosquito bite, sporozoites are injected into host's skin, enter the bloodstream and reach to the liver. Parasites differentiate and replicate inside hepatocytes, and then released as merozoites into the bloodstream, which subsequently invades red blood cells (RBCs) (186).
Inflection of diet can affect the outcome of parasitic diseases either through the effects on parasite growth and development, or via the host immune response, or both. Leptin is a cytokine predominantly secreted by adipocytes that increases in proportion to total body fat mass, and upon exposure to pro-inflammatory cytokines, it inhibits both appetite and adiposity in malaria infection (39). Moreover, serum leptin levels were approximately five-fold higher in Plasmodium berghei-infected mice than in non-infected controls (188). Leptin-deficient mice infected with P. berghei ANKA were shown to be resistant to the development of cerebral malaria whereas the normal mice developed signs of cerebral malaria. Dietary restriction prevented severe experimental cerebral malaria (ECM) symptoms and death in mice through modulation of leptin levels and mechanistic target of rapamycin complex 1 (mTORC1) activity in T cells (143). Pharmacological inhibition of either leptin signaling with a mutant peptide, or downstream mTORC1 signaling with rapamycin, blocked ECM symptoms and reduced mortality (39). Furthermore, leptin exerts central effects on hypothalamic-pituitary function and these outcomes might affect the severity of malaria disease. Disturbance in hypothalamic-pituitary-adrenal axis during P. falciparum infection have been involved in the pathogenic mechanism of severe malaria (144). Importantly, the level of leptin in serum of malaria patients has been recently reported to be used as prognostic markers of treatment outcomes and pathogenesis of malaria patients (144). In conclusion, leptin could play an important role to control the immuno-compromised malarial infections by the activation of immune cells through leptin signaling pathway.
Conclusion and Summary
Many studies have been conducted in recent past to understand the role of leptin in immune modulation such as activation of phagocytosis, cytokine polarization and cell-mediated immunity in infectious diseases. Both obesity and malnutrition are pandemics associated with immune-deficiencies that lead to increased vulnerability to infectious disease. Interestingly, both obesity and malnutrition are related to aberrant leptin levels, obesity due to chronically elevated leptin levels, whereas malnutrition results in significantly diminished leptin levels. Emerging data from animal models and human indicates that immune dysfunction underlies the etiology of malnutrition and reduced immune-mediated protection from infections, which interplay between nutrition, leptin levels and immune responses (86, 189, 190). Malnutrition is characterized by immune suppression and increased risk of mortality from infectious diseases (191). The immune dysfunction is not only a consequence of inadequate diet but also contributes in various mechanisms, including the energy homeostasis, metabolism, the role of leptin and it signals to the hypothalamic-pituitary-adrenal axis and peripheral organs. Thus, it is likely that the CNS plays a critical role in malnutrition associated immune deficiency. Protein-energy malnutrition reduces leptin concentrations which impairs macrophage functions, ability to engulf pathogens and to produce proinflammatory cytokines (25, 27). Importantly, leptin has a crucial role in mediating innate and adaptive immune response which are significantly affected by nutritional status and play a vital role in the immune adaptation in both malnutrition and infection. Moreover, many infectious diseases directly or indirectly are linked to malnutrition which compromises the innate and adaptive immunity of host and increased susceptibility to infectious disease.
More importantly the mechanism of leptin signaling in various infectious diseases is depends on SOCS3 expression as describes in NF-κB dependent pathway. The SOCS3 expression attenuates the macrophage's response to IFN-γ at both proximal level activation and downstream expression. Hence, taken together above-mentioned observations indicate that the potential role of leptin signaling in various pathogens has been summarized in a schematic diagram (Figure 2) (192–205). Therefore, understanding the link between nutrition, leptin, and immune dysfunction in murine and human infectious diseases will inform targeted interventions for a vulnerable population with undernutrition, which is a crucial need for new approaches to reduce global mortality from infectious diseases. Present review provides a rationale for future studies to explore role of leptin as therapeutics to host immune dysfunction in infectious diseases during malnutrition.
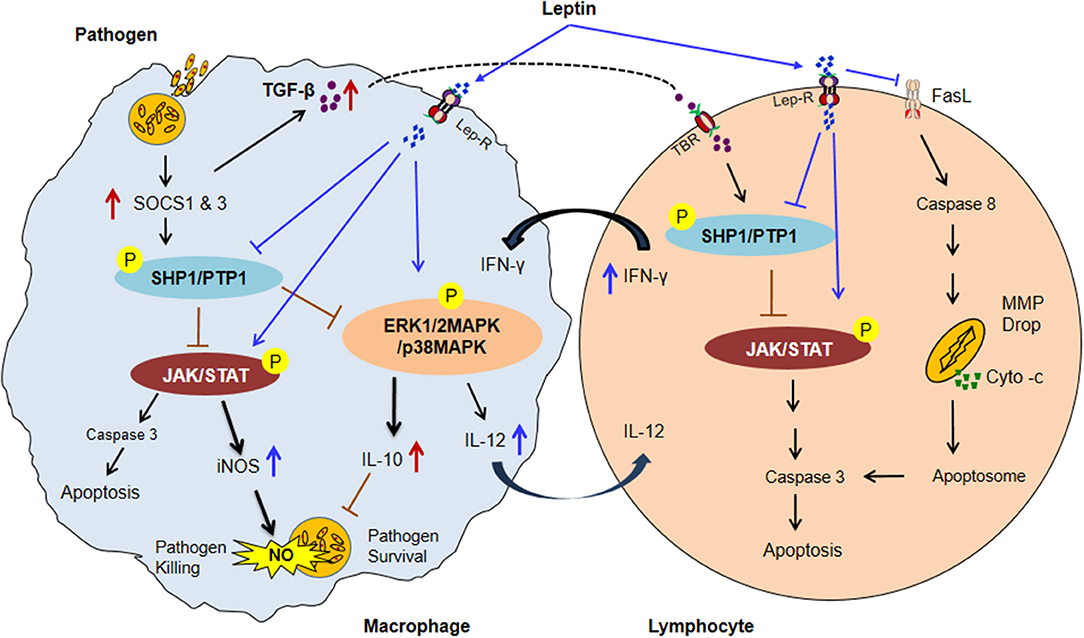
Figure 2. The possible model of Leptin and Immune response in malnutrition coupled infectious diseases. In malnutrition, low adipocyte mass causes a reduction of serum leptin level resultant impairment of normal macrophages and lymphocytes activities. Infected macrophages induce the SOCS1 & 3 proteins expression subsequently upregulates ROS scavenging enzyme Thioredoxin which leads to activates SHP1/PTPase molecules. SHP1/PTP1 negatively regulates the JAK/STAT, and MAP-Kinase pathways thus inhibiting IFN–inducible macrophage functions (increased IL-10 and TGF-β level and decreased the IL-12 cytokines in infected macrophage). IL-10 suppresses the NO activity and improves the parasite survival. TGF-β activates SHP1/PTPase activity in lymphocytes through TGF-β receptor (TBR) which leads to lymphocytes apoptosis. In contrast, Leptin treatment inactivated SHP1/PTPase directed pathways and reversed the macrophage activities by up-regulating the pro-inflammatory cytokines (IFNγ, TNF-α, and IL-12) secretion and NO expression. IL-12 cytokine released from activated macrophage upon leptin treatment inhibits the SHP1/PTPase dependent T lymphocytes apoptosis by activation of JAK/STAT pathway. Moreover, Leptin directly inhibits the FasL-dependent T lymphocytes apoptosis by the inhibition of the caspase 8 activity. Caspase-8 then promotes mitochondrial outer membrane permeabilization (MOMP) by diminishing the inhibitory effect of various antiapoptotic and proapoptotic molecules. MOMP results in cytochrome-c release from the mitochondria, enabling activation of a supramolecular complex, the apoptosome that activates caspase-3 to undertake apoptotic cell death (Suppressors of cytokine signaling: SOCS1 & 3; Protein tyrosine phosphatases: SHP1/PTP1, Mitochondrial membrane potential drop: MMP drop, and P: phosphorylation) (192–206).
Author Contributions
RM conceived and conducted literature reviews, figure, and table construction and contributed to the writing of the manuscript. PB, RD, and HN critically edited and reviewed the complete manuscript, tables and figures. All authors approved the final manuscript for submission.
Conflict of Interest Statement
The authors declare that the research was conducted in the absence of any commercial or financial relationships that could be construed as a potential conflict of interest.
Acknowledgments
We sincerely thank University Grant Commission (UGC), DST-SERB, DST-FIST, DST-Purse and University of Hyderabad for providing the lab infrastructure and subscription of many scientific journal and books to compile the review. FDA authors' contributions represent their best judgment and do not bind or obligate the FDA.
References
1. La Cava A, Matarese G. The weight of leptin in autoimmunity. Nat Rev Immunol. (2004) 4:371–9. doi: 10.1038/nri1350
2. Grinspoon S, Gulick T, Askari H, Landt M, Lee K, Anderson E, et al. Serum leptin levels in women with anorexia nervosa. J Clin Endocrinol Metab. (1996) 81:3861–3.
3. Mantzoros CS, Magkos F, Brinkoetter M, Sienkiewicz E, Dardeno TA, Kim SY, et al. Leptin in human physiology and pathophysiology. Am J Physiol Endocrinol Metab. (2011) 301:E567–84. doi: 10.1152/ajpendo.00315.2011
4. Dardeno TA, Chou SH, Moon HS, Chamberland JP, Fiorenza CG, Mantzoros CS. Leptin in human physiology and therapeutics. Front Neuroendocrinol. (2010) 31:377–93. doi: 10.1016/j.yfrne.2010.06.002
5. Faggioni R, Jones-Carson J, Reed DA, Dinarello CA, Feingold KR, Grunfeld C, et al. Leptin-deficient (ob/ob) mice are protected from T cell-mediated hepatotoxicity: role of tumor necrosis factor α and IL-18. Proc Natl Acad Sci USA. (2000) 97:2367–72. doi: 10.1073/pnas.040561297
6. Procaccini C, Jirillo E, Matarese G. Leptin as an immunomodulator. Mol Aspects Med. (2012) 33:35–45. doi: 10.1016/j.mam.2011.10.012
7. Fernandez-Riejos P, Najib S, Santos-Alvarez J, Martín-Romero C, Pérez-Pérez A, González-Yanes C, et al. Role of leptin in the activation of immune cells. Media Inflamm. (2010) 2010:568343. doi: 10.1155/2010/568343
8. Sharma A, Satyam A, Sharma JB. Leptin, IL-10 and inflammatory markers (TNF-alpha, IL-6 and IL-8) in pre-eclamptic, normotensive pregnant and healthy non-pregnant women. Am J Reprod Immunol. (2007) 58:21–30. doi: 10.1111/j.1600-0897.2007.00486.x
9. Zhang Y, Proenca R, Maffei M, Barone M, Leopold L, Friedman JM. Positional cloning of the mouse obese gene and its human homologue. Nature (1994) 372:425–32. doi: 10.1038/372425a0
10. Coleman DL. Obese and diabetes: two mutant genes causing diabetes-obesity syndromes in mice. Diabetologia (1978) 14:141–8. doi: 10.1007/BF00429772
11. Madej T, Boguski MS, Bryant SH, Threading analysis suggests that the obese gene product may be a helical cytokine. FEBS Lett. (1995) 373:13–8. doi: 10.1016/0014-5793(95)00977-H
12. Tartaglia LA, Dembski M, Weng X, Deng N, Culpepper J, Devos R, et al. Identification and expression cloning of a leptin receptor, OB-R. Cell (1995) 83:1263–71. doi: 10.1016/0092-8674(95)90151-5
13. Licinio J, Mantzoros C, Negrão AB, Cizza G, Wong ML, Bongiorno PB, et al. Human leptin levels are pulsatile and inversely related to pituitary-adrenal function. Nat Med. (1997) 3:575–9. doi: 10.1038/nm0597-575
14. Mansour E, Pereira FG, Araujo EP, Amaral MEC, Morari J, Ferraroni NR, et al. Leptin inhibits apoptosis in thymus through a janus kinase-2-independent, insulin receptor substrate-1/phosphatidylinositol-3 kinase-dependent pathway. Endocrinology (2006) 147:5470–9. doi: 10.1210/en.2006-0223
15. Martin-Romero C, Santos-Alvarez J, Governa R, Sanchez-Margalet V. Human leptin enhances activation and proliferation of human circulating T lymphocytes. Cell Immunol. (2000) 199:15–24. doi: 10.1006/cimm.1999.1594
16. Matarese G, Moschos S, Mantzoros CS. Leptin in immunology. J Immunol. (2005) 174:3137–42. doi: 10.4049/jimmunol.174.6.3137
17. Lord GM, Matarese G, Howard JK, Baker RJ, Bloom SR, Lechler RI. Leptin modulates the T-cell immune response and reverses starvation-induced immunosuppression. Nature (1998) 394:897–901. doi: 10.1038/29795
18. Howard JK, Lord GM, Mataresse G, Vendetti S, Ghatei MA, Ritter MA, et al. Leptin protects mice from starvation-induced lymphoid atrophy and increases thymic cellularity in ob/ob mice. J Clin Invest. (1999) 104:1051–9. doi: 10.1172/JCI6762
19. Saucillo DC, Gerriets VA, Sheng J, Rathmell JC, Maciver NJ. Leptin metabolically licenses T cells for activation to link nutrition and immunity. J Immunol. (2014) 192:136–44. doi: 10.4049/jimmunol.1301158
20. Faggioni R, Feingold KR, Grunfeld C. Leptin regulation of the immune response and the immunodeficiency of malnutrition. FASEB J. (2001) 15:2565–71. doi: 10.1096/fj.01-0431rev
21. Paz-Filho GJ, Delibasi T, Erol HK, Wong ML, Licinio J. Cellular immunity before and after leptin replacement therapy. J Pediatr Endocrinol Metab. (2009) 22:1069–74. doi: 10.1515/JPEM.2009.22.11.1069
22. Farooqi IS, Matarese G, Lord GM. Beneficial effects of leptin on obesity, T cell hyporesponsiveness, and neuroendocrine/metabolic dysfunction of human congenital leptin deficiency. J Clin Invest. (2002) 110:1093–103. doi: 10.1172/JCI0215693
23. Moore S, Morgan G, Collinson A, Swain J, O'Connell M, Prentice A. Leptin, malnutrition, and immune response in rural Gambian children. Arch Dis Child. (2002) 87:192–7. doi: 10.1136/adc.87.3.192
24. Woodward B. Protein, calories, and immune defenses. Nutr Rev. (1998) 56:S84–92. doi: 10.1111/j.1753-4887.1998.tb01649.x
25. Schaible UE, Kaufmann SH. Malnutrition and infection: complex mechanisms and global impacts. PLoS Med. (2007) 4:e115. doi: 10.1371/journal.pmed.0040115
26. Najera O, Gonzalez C, Toledo G, López L, Cortés E, Betancourt M, et al. CD45RA and CD45RO isoforms in infected malnourished and infected well-nourished children. Clin Exp Immunol. (2001) 126:461–5. doi: 10.1046/j.1365-2249.2001.01694.x
27. Iyer SS, Chatraw JH, Tan WG, Wherry EJ, Becker TC, Ahmed R, et al. Protein-energy malnutrition impairs homeostatic proliferation of memory CD8 T cells. J Immunol. (2012) 188:77–84. doi: 10.4049/jimmunol.1004027
28. Chatraw JH, Wherry EJ, Ahmed R, Kapasi ZF. Diminished primary CD8 T cell response to viral infection during protein-energy malnutrition in mice is due to changes in microenvironment and low numbers of viral-specific CD8 T cell precursors. J Nutr. (2008) 138:806–12. doi: 10.1093/jn/138.4.806
29. Nájera O, González C, Toledo G, López L, Ortiz R. Flow cytometry study of lymphocyte subsets in malnourished and well-nourished children with bacterial infections. Clin Diagn Lab Immunol. (2004) 11:577–80. doi: 10.1128/CDLI.11.3.577-580.2004
30. Wieland CW, Florquin S, Chan ED, Leemans JC, Weijer S, Verbon A, et al. Pulmonary Mycobacterium tuberculosis infection in leptin-deficient ob/ob mice. Int Immunol. (2005) 17:1399–408. doi: 10.1093/intimm/dxh317
31. Hsu A, Aronoff DM, Phipps J, Goel D, Mancuso P. Leptin improves pulmonary bacterial clearance and survival in ob/ob mice during Pneumococcal pneumonia. Clin Exp Immunol. (2007) 150:332–9. doi: 10.1111/j.1365-2249.2007.03491.x
32. Tschöp J, Nogueiras R, Haas-Lockie S, Kasten KR, Castañeda TR, Huber N, et al. CNS leptin action modulates immune response and survival in sepsis. J Neurosci. (2010) 30:6036–47. doi: 10.1523/JNEUROSCI.4875-09.2010
33. Madan R, Guo X, Naylor C, Buonomo EL, Mackay D, Noor Z, et al. Role of leptin-mediated colonic inflammation in defense against Clostridium difficile Colitis. Infect Immun. (2014) 82:341–9. doi: 10.1128/IAI.00972-13
34. Zhang AJ, To KK, Li C, Lau CC, Poon VK, Chan CC, et al. Leptin mediates the pathogenesis of severe 2009 pandemic influenza a (h1n1) infection associated with cytokine dysregulation in mice with diet-induced obesity. J Infect Dis. (2013) 207:1270–80. doi: 10.1093/infdis/jit031
35. Sánchez-Pozo C, Rodriguez-baño J, Domínguez-Castellano A, Muniain MA, Goberna R, Sánchez-Margalet V. Leptin stimulates the oxidative burst in control monocytes but attenuates the oxidative burst in monocytes from HIV-infected patients. Clin Exp Immunol. (2003) 134:464–9. doi: 10.1111/j.1365-2249.2003.02321.x
36. Dayakar A, Chandrasekaran S, Veronica J, Maurya R. Leptin induces the phagocytosis and protective immune response in Leishmania donovani infected THP-1 cell line and human PBMCs. Exp Parasitol. (2016) 160:54–9. doi: 10.1016/j.exppara.2015.12.002
37. Nagajyothi F, Zhao D, Machado FS, Weiss LM, Schwartz GJ, Desruisseaux MS, et al. Crucial role of the central leptin receptor in murine Trypanosoma cruzi infection. J Infect Dis. (2010) 202:1104–13. doi: 10.1086/656189
38. Vedantama G, Viswanathan VK. Leptin signaling protects the gut from Entamoeba histolytica infection. Gut Microb. (2012) 3:2–3. doi: 10.4161/gmic.19424
39. Pulido-Mendez M, De Sanctis J, Rodriguez-Acosta A. Leptin and leptin receptors during malaria infection in mice. Folia Parasitol. (2002) 49:249–51. doi: 10.14411/fp.2002.046
40. Chan J, Tian Y, Tanaka KE, Tsang MS, Yu K, Salgame P, et al. Effects of protein-calorie malnutrition on tuberculosis in mice. Proc Natl Acad Sci USA. (1996) 93:14857–61. doi: 10.1073/pnas.93.25.14857
41. Pena-Cruz V, Reiss CS, McIntosh K. Sendai virus infection of mice with protein malnutrition. J Virol. (1989) 63:3541–4.
42. Ritz BW, Aktan I, Nogusa S, Gardner EM. Energy restriction impairs natural killer cell function and increases the severity of influenza infection in young adult male C57BL/6 mice. J Nutr. (2008) 138:2269–75. doi: 10.3945/jn.108.093633
43. Goldberg EL, Romero-Aleshire MJ, Renkema KR, Ventevogel MS, Chew WM, Uhrlaub JL, et al. Lifespan-extending caloric restriction or mTOR inhibition impair adaptive immunity of old mice by distinct mechanisms. Aging Cell (2015) 14:130–8. doi: 10.1111/acel.12280
44. Kristan DM. Chronic calorie restriction increases susceptibility of laboratory mice (Mus musculus) to a primary intestinal parasite infection. Aging Cell (2007) 6:817–25. doi: 10.1111/j.1474-9726.2007.00345.x
45. Rodríguez L, Graniel J, Ortiz R. Effect of leptin on activation and cytokine synthesis in peripheral blood lymphocytes of malnourished infected children. Clin Exp Immunol. (2007) 148:478–85. doi: 10.1111/j.1365-2249.2007.03361.x
46. Procaccini C, Lourenco EV, Matarese G, La Cava A. Leptin signaling: a key pathway in immune responses. Curr Signal Transduct Ther. (2009) 4:22–30. doi: 10.2174/157436209787048711
47. Fantuzzi G. Three questions about leptin and immunity. Brain Behav Immun. (2009) 23:405–10. doi: 10.1016/j.bbi.2008.10.007
48. Sánchez-Margalet V, Martín-Romero C, Santos-Alvarez J, Goberna R, Najib S, Gonzalez-Yanes C. Role of leptin as an immunomodulator of blood mononuclear cells: mechanisms of action. Clin Exp Immunol. (2003) 133:11–9. doi: 10.1046/j.1365-2249.2003.02190.x
49. Gainsford T, Willson TA, Metcalf D. Leptin can induce proliferation, differentiation, and functional activation of hemopoietic cells. Proc Natl Acad Sci USA. (1996) 93:14564–8. doi: 10.1073/pnas.93.25.14564
50. Raso GM, Pacilio M, Esposito E, Coppola A, Di Carlo R, Meli R. Leptin potentiates IFN-gamma induced expression of nitric oxide synthase and cyclo-oxygenase-2 in murine macrophage J774A. 1. Br J Pharmacol. (2002) 137:799–804. doi: 10.1038/sj.bjp.0704903
51. Santos-Alvarez J, Goberna R, Sanchez-Margalet V. Human leptin stimulates proliferation and activation of human circulating monocytes. Cell Immunol. (1999) 194:6–11. doi: 10.1006/cimm.1999.1490
52. Bennett BD, Solar GP, Yuan JQ, Mathias J, Thomas GR, Matthews W. A role for leptin and its cognate receptor in hematopoiesis. Curr Biol. (1996) 6:1170–80. doi: 10.1016/S0960-9822(02)70684-2
53. Najib S, Sanchez-Margalet V. Human leptin promotes survival of human circulating blood monocytes prone to apoptosis by activation of p42/44 MAPK pathway. Cell Immunol. (2002) 220:43–149. doi: 10.1016/S0008-8749(03)00027-3
54. Loffreda S, Yang SQ, Lin HZ, Karp CL, Brengman ML, Wang DJ, et al. Leptin regulates proinflammatory immune responses. FASEB J. (1998) 12:57–65. doi: 10.1096/fasebj.12.1.57
55. Oral EA, Javor ED, Ding L, Uzel G, Cochran EK, Young JR, et al. Leptin replacement therapy modulates circulating lymphocyte subsets and cytokine responsiveness in severe lipodystrophy. J Clin Endocrinol Metab. (2006) 91:621–8. doi: 10.1210/jc.2005-1220
56. Bruno A, Conus S, Schmid I, Simon H-U. Apoptotic pathways are inhibited by leptin receptor activation in neutrophils. J Immunol. (2005) 174:8090–6. doi: 10.4049/jimmunol.174.12.8090
57. Zarkesh-Esfahani H, Pockley AG, Wu Z, Hellewell PG, Weetman AP, Ross RJ, et al. Leptin indirectly activates human Neutrophils via induction of TNF-α. J Immunol. (2004) 172:1809–14. doi: 10.4049/jimmunol.172.3.1809
58. Brake DK, Smith EB, Mersmann H, Smith CW, Robker RL. ICAM-1 expression in adipose tissue: effects of diet-induced obesity in mice. Am J Physiol Cell Physiol. (2006) 291:C1232–9. doi: 10.1152/ajpcell.00008.2006
59. Kiguchi N, Maeda T, Kobayashi Y, Fukazawa Y, Kishioka S. Leptin enhances CC-chemokine ligand expression in cultured murine macrophage. Biochem Biophys Res Commun. (2009) 384:311–5. doi: 10.1016/j.bbrc.2009.04.121
60. Gruen ML, Hao M, Piston DW, Hasty AH. Leptin requires canonical migratory signaling pathways for induction of monocyte and macrophage chemotaxis. Am J Physiol Cell Physiol. (2007) 293:C1481–8. doi: 10.1152/ajpcell.00062.2007
61. Rafail S, Ritis K, Schaefer K, Kourtzelis I, Speletas M, Doumas M, et al. Leptin induces the expression of functional tissue factor in human neutrophils and peripheral blood mononuclear cells through JAK2-dependent mechanisms and TNF-α involvement. Thromb Res. (2008) 122:366–75. doi: 10.1016/j.thromres.2007.12.018
62. Mattioli B, Straface E, Quaranta MG, Giordani L, Viora M. Leptin promotes differentiation and survival of human dendritic cells and licenses them for Th1 priming. J Immunol. (2005) 174:6820–8. doi: 10.4049/jimmunol.174.11.6820
63. Mattioli B, Giordani L, Quaranta MG, Viora M. Leptin exerts an anti-apoptotic effect on human dendritic cells via the PI3K-Akt signalling pathway. FEBS Lett. (2009) 583:1102–6. doi: 10.1016/j.febslet.2009.02.029
64. Lam QL, Liu S, Cao X, Lu L. Involvement of leptin signalling in the survival and maturation of bone marrow-derived dendritic cells. Eur J Immunol. (2006) 36:3118–30. doi: 10.1002/eji.200636602
65. Tian Z, Sun R, Wei H, Gao B. Impaired natural killer (NK) cell activity in leptin receptor deficient mice: leptin as a critical regulator in NK cell development and activation. Biochem Biophys Res Commun. (2002) 298:297–302. doi: 10.1016/S0006-291X(02)02462-2
66. Zhao Y, Sun R, You L, Gao C, Tian Z. Expression of leptin receptors and response to leptin stimulation of human natural killer cell lines. Biochem Biophys Res Commun. (2003) 300:247–52. doi: 10.1016/S0006-291X(02)02838-3
67. Velloso LA, Savino W, Mansour E. Leptin action in the thymus. Ann N Y Acad Sci. (2009) 1153:29–34. doi: 10.1111/j.1749-6632.2008.03973.x
68. Da Silva SV, Salama C, Renovato-Martins M, Helal-Neto E, Citelli M, Savino W. Increased leptin response and inhibition of apoptosis in thymocytes of young rats offspring from protein deprived dams during lactation. PLoS ONE (2013) 8:e64220. doi: 10.1371/journal.pone.0064220
69. Merrick JC, Edelson BT, Bhardwaj V, Swanson PE, Unanue ER. Lymphocyte apoptosis during early phase of Listeria infection in mice. Am J Pathol. (1997) 151:785–92.
70. Papathanassoglou E, El-Haschimi K, Li XC, Matarese G, Strom T, Mantzoros C. Leptin receptor expression and signaling in lymphocytes: kinetics during lymphocyte activation, role in lymphocyte survival, and response to high-fat diet in mice. J Immunol. (2006) 176:7745–52. doi: 10.4049/jimmunol.176.12.7745
71. Fujita Y, Murakami M, Ogawa Y, Masuzaki H, Tanaka M, Ozaki S, et al. Leptin inhibits stress-induced apoptosis of T lymphocytes. Clin Ex Immunol. (2002) 128:21–6. doi: 10.1046/j.1365-2249.2002.01797.x
72. Lord GM, Matarese G, Howard JK, Bloom SR, Lechler RI. Leptin inhibits the anti-CD3-driven proliferation of peripheral blood T cells but enhances the production of proinflammatory cytokines. J Leukoc Biol. (2002) 72:330–8. doi: 10.1189/jlb.72.2.330
73. Farooqi IS, Wangensteen T, Collins S, Kimber W, Matarese G, Keogh JM, et al. Clinical and molecular genetic spectrum of congenital deficiency of the leptin receptor. N Engl J Med. (2007) 356:237–47. doi: 10.1056/NEJMoa063988
74. Ozata M, Ozdemir IC, Licinio J. Human leptin deficiency caused by a missense mutation: multiple endocrine defects, decreased sympathetic tone, and immune system dysfunction indicates new targets for leptin action, greater central than peripheral resistance to the effects of leptin, and spontaneous correction of leptin-mediated defects. J Clin Endocrinol Metab. (1999) 84:3686–95. doi: 10.1210/jcem.84.10.5999
75. Chandra RK. Cell-mediated immunity in genetically obese C57BL/6J (ob/ob) mice. Am J Clin Nutr. (1980) 33:13–6. doi: 10.1093/ajcn/33.1.13
76. Banks AS, Davis SM, Bates SH, Myers MG Jr. Activation of downstream signals by the long form of the leptin receptor. J Biol Chem. (2000) 275:14563–72. doi: 10.1074/jbc.275.19.14563
77. Dunn SL, Bjornholm M, Bates SH, Chen Z, Seifert M, Myers MG Jr, et al. Feedback inhibition of leptin receptor/Jak2 signaling via Tyr1138 of the leptin receptor and suppressor of cytokine signaling 3. Mol Endocrinol. (2005) 19:925–38. doi: 10.1210/me.2004-0353
78. Kim SY, Lim JH, Choi SW, Kim M, Kim ST, Kim MS, et al. Preferential effects of leptin on CD4 T cells in central and peripheral immune system are critically linked to the expression of leptin receptor. Biochem Biophys Res Commun. (2010) 394:562–8. doi: 10.1016/j.bbrc.2010.03.019
79. De Rosa V, Procaccini C, Calì G, Pirozzi G, Fontana S, Zappacosta S, et al. A key role of leptin in the control of regulatory T cell proliferation. Immunity (2007) 26:241–55. doi: 10.1016/j.immuni.2007.01.011
80. Sanchez-Margalet V, Martin-Romero C. Human leptin signaling in human peripheral blood mononuclear cells: activation of the JAK-STAT pathway. Cell Immunol. (2001) 211:30–6. doi: 10.1006/cimm.2001.1815
81. Agrawal S, Gollapudi S, Su H, Gupta S. Leptin activates human B cells to secrete TNF-alpha, IL-6, and IL-10 via JAK2/STAT3 and p38MAPK/ERK1/2 signaling pathway. J Clin Immunol. (2011) 31:472–8. doi: 10.1007/s10875-010-9507-1
82. Groom JR, Luster AD. CXCR3 in T cell function. Exp Cell Res. (2011) 315:620–31. doi: 10.1016/j.yexcr.2010.12.017
83. Biesiada G, Czepiel J, Ptak-Belowska A, Targosz A, Krzysiek-Maczka G, Strzalka M, et al. Expression and release of leptin and proinflammatory cytokines in patients with ulcerative colitis and infectious diarrhea. J Physiol Pharmacol. (2012) 63:471–81.
84. Chandra RK. Nutrition, immunity and infection: from basic knowledge of dietary manipulation of immune responses to practical application of ameliorating suffering and improving survival. Proc Natl Acad Sci USA. (1996) 93:14304–7. doi: 10.1073/pnas.93.25.14304
85. Matarese G. Leptin and the immune system: how nutritional status influences the immune response. Eur Cytokine Netw. (2000) 11:7–14.
86. Gerriets VA, MacIver NJ. Role of T cells in malnutrition and obesity. Front Immunol. (2014) 5:379. doi: 10.3389/fimmu.2014.00379
87. Chandra R. Nutrition, and immunology: from the clinic to cellular biology and back again. Proc Nutr Soc. (1999) 58:681–3. doi: 10.1017/S0029665199000890
88. Cederholm T, Arner P, Palmblad J. Low circulating leptin levels in protein-energy malnourished chronically ill elderly patients. J Intern Med. (1997) 242:377–82. doi: 10.1046/j.1365-2796.1997.00216.x
89. Soliman AT, Zalabany El, Salama MM, Ansari BM. Serum leptin concentrations during protein-energy malnutrition: correlation with growth parameters and endocrine function. Metabolism (2000) 49:819–25. doi: 10.1053/meta.2000.6745
90. Blackburn GL. Pasteur's quadrant and malnutrition. Nature (2001) 409:397–401. doi: 10.1038/35053187
91. Dai G, McMurray DN. Altered cytokine production and impaired antimycobacterial immunity in protein-malnourished guinea pigs. Infect Immun. (1998) 66:3562–8.
92. Cegielski JP, McMurray DN. The relationship between malnutrition and tuberculosis: evidence from studies in humans and experimental animals. Int J Tuberc Lung Dis. (2004) 8:286–98.
93. Cakir B, Yonem A, Guler S, Odabasi E, Demirbas B, Gürsoy G, et al. Relation of leptin and tumor necrosis factor alpha to body weight changes in patients with pulmonary tuberculosis. Horm Res. (1999) 52:279–83.
94. Schwenk A, Hodgson L, Rayner CF, Griffin GE, Macallan DC. Leptin and energy metabolism in pulmonary tuberculosis. Am J Clin Nutr. (2003) 77:392–8. doi: 10.1093/ajcn/77.2.392
95. van Crevel R, Karyadi E, Netea MG, Verhoef H, Nelwan RH, West CE, et al. Decreased plasma leptin concentrations in tuberculosis patients are associated with wasting and inflammation. J Clin Endocrinol Metab. (2002) 87:758–63. doi: 10.1210/jcem.87.2.8228
96. van Lettow M, van der Meer JW, West CE, van Crevel R, Semba RD. Interleukin- 6 and human immunodeficiency virus load, but not plasma leptin concentration, predict anorexia and wasting in adults with pulmonary tuberculosis in Malawi. J Clin Endocrinol Metab. (2005) 90:4771–6. doi: 10.1210/jc.2004-2539
97. Hoang T, Agger EM, Cassidy JP, Christensen JP, Andersen P. Protein energy malnutrition during vaccination has limited influence on vaccine efficacy but abolishes immunity if administered during Mycobacterium tuberculosis infection. Infect Immun. (2015) 83:2118–26. doi: 10.1128/IAI.03030-14
98. Auphan N, Didonato JA, Helmberg A, Rosette C, Karin M. Immunoregulatory genes and immunosuppression by glucocorticoids. Arch Toxicol Suppl. (1997) 19:87–95. doi: 10.1007/978-3-642-60682-3_8
99. McCarter MD, Naama HA, Shou J, Kwi LX, Evoy DA, Calvano SE, et al. Altered macrophage intracellular signaling induced by protein-calorie malnutrition. Cell Immunol. (1998) 183:131–6. doi: 10.1006/cimm.1998.1241
100. Redmond HP, Gallagher HJ, Shou J, Daly JM. Antigen presentation in protein-energy malnutrition. Cell Immunol. (1995) 163:80–7. doi: 10.1006/cimm.1995.1101
101. Redmond HP, Leon P, Lieberman MD, Hofmann K, Shou J, Reynolds JV, et al. Impaired macrophage function in severe protein-energy malnutrition. Arch Surg. (1991) 126:192–6. doi: 10.1001/archsurg.1991.01410260080011
102. Palacio A, Lopez M, Perez-Bravo F, Monkeberg F, Schlesinger L. Leptin levels are associated with immune response in malnourished infants. J Clin Endocrinol Metab. (2002) 87:3040–6. doi: 10.1210/jcem.87.7.8636
103. Bartz S, Mody A, Hornik C, Bain J, Muehlbauer M, Kiyimba T, et al. Severe acute malnutrition in childhood: hormonal and metabolic status at presentation, response to treatment, and predictors of mortality. J Clin Endocrinol Metab. (2014) 99:2128–37. doi: 10.1210/jc.2013-4018
104. Testa R, Franceschini R, Giannini E, Cataldi A, Botta F, Fasoli A, et al. Serum leptin levels in patients with viral chronic hepatitis or liver cirrhosis. J Hepatol. (2000) 33:33–7. doi: 10.1016/S0168-8278(00)80156-7
105. Redmond HP, Shou J, Kelly CJ, Leon P, Daly JM. Protein-calorie malnutrition impairs host defense against Candida albicans. J Surg Res. (1991) 50:552–9. doi: 10.1016/0022-4804(91)90040-S
106. Cason J, Ainley CC, Wolstencroft RA, Norton KR, Thompson RP. Cell-mediated immunity in anorexia nervosa. Clin Exp Immunol. (1986) 64:370–5.
107. Ahima RS, Prabakaran D, Mantzoros C, Qu D, Lowell B, Maratos-Flier E, et al. Role of leptin in the neuroendocrine response to fasting. Nature (1996) 382:250–2. doi: 10.1038/382250a0
108. Faggioni R, Moser A, Feingold KR, Grunfeld C. Reduced leptin levels in starvation increase susceptibility to endotoxic shock. Am J Pathol. (2000) 156:1781–7. doi: 10.1016/S0002-9440(10)65049-3
109. Faggioni R, Fantuzzi G, Gabay C, Moser A, Dinarello CA, Feingold KR, et al. Leptin deficiency enhances sensitivity to endotoxin-induced lethality. Am J Physiol. (1999) 276:R136–42.
110. Chen M, Wang B, Xu Y, Deng Z, Xue H, Wang L, et al. Diagnostic value of serum leptin and a promising novel diagnostic model for sepsis. Exp Therapeutic Med. (2014) 7:881–6. doi: 10.3892/etm.2014.1506
111. Ye M, Bian L-F. Association of serum leptin levels and pulmonary tuberculosis: a meta-analysis. J Thorac Dis. (2018)10:1027–36. doi: 10.21037/jtd.2018.01.70
112. Mancuso P, Gottschalk A, Phare SM, Peters-Golden M, Lukacs NW, Huffnagle GB. Leptin-deficient mice exhibit impaired host defense in Gram-negative pneumonia. J Immunol. (2002) 168:4018–24. doi: 10.4049/jimmunol.168.8.4018
113. Moore SI, Huffnagle GB, Chen GH, White ES, Mancuso P. Leptin modulates neutrophil phagocytosis of Klebsiella pneumoniae. Infect Immun. (2003) 71:4182–5. doi: 10.1128/IAI.71.7.4182-4185.2003
114. Mancuso P, Huffnagle GB, Olszewski MA, Phipps J, Peters-Golden M. Leptin corrects host defense defects after acute starvation in murine Pneumococcal pneumonia. Am J Respir Crit Care Med. (2006) 173:212–8. doi: 10.1164/rccm.200506-909OC
115. Koca C, Kavakli HS, Alici O. Immunomodulatory role of leptin treatment in experimental sepsis caused by gram negative bacteria. Turk J Med Sci. (2011) 41:251–8. doi: 10.3906/sag-1009-1109
116. Morshedi A, Zarkesh-Esfahani HS, Behjati M. Effect of leptin on neutrophils phagocytosis and lymphocytes apoptosis challenge by Listeria monocytogenes and Escherichia coli. Jundishapur J Microbiol. (2013) 6:e6389. doi: 10.5812/jjm.6389
117. Ikejima S, Sasaki S, Sashinami H, Mori F, Ogawa Y, Nakamura T, et al. Impairment of host resistance to Listeria monocytogenes infection in the liver of db/db and ob/ob mice. Diabetes (2005) 54:182–9. doi: 10.2337/diabetes.54.1.182
118. Nishi Y, Isomoto H, Uotani S, Wen CY, Shikuwa S, Ohnita K, et al. Enhanced production of leptin in gastric fundic mucosa with Helicobacter pylori infection. World J Gastroenterol. (2005) 11:695–9. doi: 10.3748/wjg.v11.i5.695
119. Khosravi Y, Seow SW, Amoyo AA, Chiow KH, Tan TL, Wong WY, et al. Helicobacter pylori infection can affect energy modulating hormones and body weight in germ free mice. Sci Rep. (2015) 5:8731. doi: 10.1038/srep08731
120. Azuma T, Suto H, Ito Y, Ohtani M, Dojo M, Kuriyama M, et al. Gastric leptin and Helicobacter pylori infection. Gut (2001) 49:324–9. doi: 10.1136/gut.49.3.324
121. Khudhur S, Jameel D, Karhoot J, AL-Khafaji J, Mohsin J. Increase serum leptin level in Helicobacter pylori infection in Iraqi gastritis patients. Al-Kindy Col Med J. (2011) 7:74–7.
122. Milner J, Meyers M, Sheridan P, Mancuso P, Beck M. The role of T cell leptin signaling in 2009 pandemic H1N1 influenza infection mortality in obese mice (VIR2P.1013). J Immunol. (2014) 192(Suppl. 1):75.2.
123. Milner JJ, Rebeles J, Dhungana S, Stewart DA, Sumner SC, Meyers MH, et al. Obesity increases mortality and modulates the lung metabolome during pandemic H1N1 influenza virus infection in mice. J Immunol. (2015) 194:4846–59. doi: 10.4049/jimmunol.1402295
124. Hur SJ, Kim DH, Chun SC, Lee SK. Effect of adenovirus and influenza virus infection on obesity. Life Sci. (2013) 93:531–5. doi: 10.1016/j.lfs.2013.08.016
125. Radigan KA, Morales-Nebreda L, Soberanes S, Nicholson T, Nigdelioglu R, Cho T, et al. Impaired clearance of influenza a virus in obese, leptin receptor-deficient mice is independent of leptin signaling in the lung epithelium and macrophages. PLoS ONE (2014) 9:e108138. doi: 10.1371/journal.pone.0108138
126. Qin L, Tan YR, Hu CP, Liu XA, He RX. Leptin is over-secreted by respiratory syncytial virus-infected bronchial epithelial cells and regulates th2 and th17 cell differentiation. Int Arch Allergy Immunol. (2015) 167:65–71. doi: 10.1159/000436966
127. Sánchez-Margalet V, Martín-Romero C, González-Yanes C, Goberna R, Rodríguez-Baños J, Muniain MA. Leptin receptor expression is induced in activated mononuclear cells in vitro and in vivo in HIV-infected patients. Clin Exp Immunol. (2002)129:119–24. doi: 10.1046/j.1365-2249.2002.01900.x
128. Azzoni L, Crowther NJ, Firnhaber C, Foulkes AS, Yin X, Glencross D, et al. Association between HIV replication and serum leptin levels: an observational study of a cohort of HIV-1-infected South African women. J Int AIDS Soc. (2010) 13:33. doi: 10.1186/1758-2652-13-33
129. Sinha U, Sinharay K, Sengupta N, Mukhopadhyay P. Benefits of leptin therapy in HIV patients. Ind J Endocrinol Metab. (2012) 16(Suppl. 3):S637–43. doi: 10.4103/2230-8210.105583
130. Shivahare R, Ali W, Vishwakarma P, Natu SM, Puri SK, Gupta S. Leptin augments protective immune responses in murine macrophages and enhances the potential of miltefosine against experimental visceral leishmaniasis. Acta Trop. (2015) 150:35–41. doi: 10.1016/j.actatropica.2015.06.024
131. Maurya R, Bhattacharya P, Ismail N, Dagur PK, Joshi AB, Razdan K, et al. Differential role of leptin as an immunomodulator in controlling visceral leishmaniasis in normal and leptin-deficient mice. Am J Trop Med Hyg. (2016) 95:109–19. doi: 10.4269/ajtmh.15-0804
132. Dayakar A, Chandrasekaran S, Veronica J, Bharadwaja V, Maurya R. Leptin regulates Granzyme-A, PD-1 and CTLA-4 expression in T cell to control visceral leishmaniasis in BALB/c Mice. Sci Rep. (2017) 7:14664. doi: 10.1038/s41598-017-15288-7
133. Combs TP Nagajyothi Mukherjee S, de Almeida CJ, Jelicks, LA, Schubert, W, et al. The adipocyte as an important target cell for Trypanosoma cruzi infection. J Biol Chem. (2005) 280:24085–94. doi: 10.1074/jbc.M412802200
134. Gutierrez FR, Guedes PM, Gazzinelli RT, Silva JS. The role of parasite persistence in the pathogenesis of Chagas heart disease. Parasite Immunol. (2009) 31:673–85. doi: 10.1111/j.1365-3024.2009.01108.x
135. Matos Ferreira AV, Segatto M, Menezes Z, Macedo AM, Gelape C, de Oliveira Andrade L, et al. Evidence for Trypanosoma cruzi in adipose tissue in human chronic Chagas disease. Microb Infect. (2011) 13:1002–5. doi: 10.1016/j.micinf.2011.06.002
136. Tanowitz HB, Amole B, Hewlett D, Wittner M. Trypanosoma cruzi infection in diabetic mice. Trans R Soc Tropic Med Hyg. (1988) 82:90–3. doi: 10.1016/0035-9203(88)90272-6
137. Alam F, Salam A, Mahmood I, Kabir M, Chowdhury S, Haque R. Amebic liver abscess is associated with malnutrition and low serum leptin level. J Infect Dis Ther. (2016) 4:298. doi: 10.4172/2332-0877.1000298
138. Duggal P, Guo X, Haque R, Peterson KM, Ricklefs S, Mondal D, et al. A mutation in the leptin receptor is associated with Entamoeba histolytica infection in children. J Clin Invest. (2011) 121:1191–8. doi: 10.1172/JCI45294
139. Naylor C, Burgess S, Madan R, Buonomo E, Razzaq K, Ralston K et al. Leptin receptor mutation results in defective neutrophil recruitment to the colon during Entamoeba histolytica infection. MBio (2014) 5:e02046–14. doi: 10.1128/mBio.02046-14
140. Guo X, Roberts MR, Becker SM, Podd B, Zhang Y, Chua SC Jr, et al. Leptin signaling in intestinal epithelium mediates resistance to enteric infection by Entamoeba histolytica. Mucosal Immunol. (2011) 4:294–303. doi: 10.1038/mi.2010.76
141. Marie CS, Verkerke HP, Paul SN, Mackey AJ, Petri WA Jr. Leptin protects host cells from Entamoeba histolytica cytotoxicity by a STAT-3 dependent mechanism. Infect Immun. (2012) 226:2123–30.
142. Mackey-Lawrence NM, Petri WA, Jr. Leptin and mucosal immunity. Mucosal Immunol. (2012) 5:472–9. doi: 10.1038/mi.2012.40
143. Mejia P, Treviño-Villarreal JH, Hine C, Harputlugil E, Lang S, Calay E, et al. Dietary restriction protects against experimental cerebral malaria via leptin modulation and T cell mTORC1 suppression. Nat Commun. (2015) 6:6050. doi: 10.1038/ncomms7050
144. Al-Fadhli MA, Saraya MA, Qasem JA. Evaluation of leptin, interleukin-1 beta and tumor necrosis factor alpha in the serum of malaria patients as prognostic markers of treatment outcome. Asian Pac J Trop Biomed. (2014) 4:441–5. doi: 10.12980/APJTB.4.201414B11
145. Elnemr GM, Elnashar MA, Elmargoushy NM, Elnashar NA, Elnashar MA. Adiponectin levels as a marker of inflammation in pulmonary tuberculosis. Egypt J Hosp Med. (2015) 59:208–14. doi: 10.12816/0012177
146. Neeraj Indora N, Sharma K, Karunanand B, Sudan DPS, Shah AK. Assessment of metabolic (Adiponectin, Leptin) and inflammatory markers (TNF-α, hs-CRP & IFN-γ) in pulmonary tuberculosis: prospective Study. Int J Clin Biochem Res. (2017) 4:216–9. doi: 10.18231/2394-6377.2017.0051
147. Shirshev SV, Orlova EG. Molecular mechanisms of regulation of the functional activity of mononuclear phagocytes by leptin. Biochemistry (2005) 70:841–7. doi: 10.1007/s10541-005-0193-1
148. Bates SH, Stearns WH, Dundon TA, Schubert M, Tso AW, Wang Y, et al. STAT3 signalling is required for leptin regulation of energy balance but not reproduction. Nature (2003) 421:856–9. doi: 10.1038/nature01388
149. Gong Y, Ishida-Takahashi R, Villanueva EC, Fingar DC, Münzberg H, Myers MG Jr. The long form of the leptin receptor regulates STAT5 and ribosomal protein S6 via alternate mechanisms. J Biol Chem. (2007) 282:31019–27. doi: 10.1074/jbc.M702838200
150. Bjørbaek C, Buchholz RM, Davis SM, Bates SH, Pierroz DD, Gu H, et al. Divergent roles of SHP-2 in ERK activation by leptin receptors. J Biol Chem. (2001) 276:4747–55. doi: 10.1074/jbc.M007439200
151. Mancuso P, Myers MG, Goel D, Serezani CH, O'Brien E, Goldberg J, et al. Ablation of leptin receptor-mediated ERK activation impairs host defense against gram-negative pneumonia. J Immunol. (2012) 189:867–75. doi: 10.4049/jimmunol.1200465
152. Caldefie-Chezet F, Poulin A, Tridon A, Sion B, Vasson MP. Leptin: a potential regulator of polymorphonuclear neutrophil bactericidal action? J Leukoc Biol. (2001) 69:414–8.
153. Moshyedi AK, Josephs MD, Abdalla EK, Mackay SLD, Edwards CK, Copeland EM, et al. Increased leptin expression in mice with bacterial peritonitis is partially regulated by tumor necrosis factor alpha. Infect Immun. (1998) 66:1800–2.
154. Mancuso P, Peters-Golden M, Goel D. Disruption of leptin receptor-stat3 signaling enhances leukotriene production and pulmonary host defense against Pneumococcal pneumonia. J Immunol. (2011) 186:1081–90. doi: 10.4049/jimmunol.1001470
155. Breideri M, Miehlike S, Glasou A, Orban Z, Stolte M, Ehninger G, et al. Leptin and its receptor in normal human gastric mucosa and in H pylori-associated gastritis. Scand J Gastroenterol. (1999) 34:954–61. doi: 10.1080/003655299750025039
156. Taylor AK, Cao W, Vora KP, De La Cruz J, Shieh WJ, Zaki SR, et al. Protein-energy malnutrition decreases immunity and increases susceptibility to influenza infection in mice. J Infect Dis. (2013) 207:501–10. doi: 10.1093/infdis/jis527
157. Ortega YR, Eberhard ML, Kris H. Protozoan diseases: cryptosporidiosis, giardiasis and other intestinal protozoan diseases. International Encyclopedia of Public Health. Oxford: Academic Press (2008) p. 354–66.
158. Lõhmus M, Moalem S, Björklund M. Leptin, a tool of parasites? Biol Lett. (2012) 8:849–52. doi: 10.1098/rsbl.2012.0385
159. Caldefie-Chezet F, Poulin A, Vasson MP. Leptin regulates functional capacities of polymorphonuclear neutrophils. Free Radic Res. (2003) 37:809–14. doi: 10.1080/1071576031000097526
160. Naylor C, Petri WA Jr. Leptin regulation of immune responses. Trends Mol Med. (2016) 22:88–98. doi: 10.1016/j.molmed.2015.12.001
161. WHO. Control of the leishmaniasis: report of a meeting of the WHO Expert Committee on the Control of Leishmaniases, Geneva, March 22–26, 2010. World Health Organ Tech Rep Ser. (2010) 949:1–186.
162. Desjeux P. Leishmaniasis: current situation and new perspectives. Comp Immunol Microbiol Infect Dis. (2004). 27:305–18. doi: 10.1016/j.cimid.2004.03.004
163. Pearson RD, Cox G, Jeronimo SM, Castracane J, Drew JS, Evans T, et al. Visceral leishmaniasis: a model for infection-induced cachexia. Am J Trop Med Hyg. (1992) 47(1 Pt 2):8–15. doi: 10.4269/ajtmh.1992.47.8
164. Anstead GM, Chandrasekar B, Zhao W, Yang J, Perez LE, Melby PC. Lalnutrition alters the innate immune response and increases early visceralization following Leishmania donovani infection. Infect Immun. (2001) 69:4709–18. doi: 10.1128/IAI.69.8.4709-4718.2001
165. Ibrahim MK, Barnes JL, Anstead GM, Jimenez F, Travi BL, Peniche AG, et al. The malnutrition-related increase in early visceralization of Leishmania donovani is associated with a reduced number of lymph node phagocytes and altered conduit system flow. PLoS Negl Tropic Dis. (2013) 7:e2329. doi: 10.1371/journal.pntd.0002329
166. Macia L, Delacre M, Abboud G, Ouk TS, Delanoye A, Verwaerde C, et al. Impairment of dendritic cell functionality and steady-state number in obese mice. J Immunol. (2006) 177:5997. doi: 10.4049/jimmunol.177.9.5997
167. Jin HT, Anderson AC, Tan WG, West EE, Ha SJ, Araki K, et al. Cooperation of Tim-3 and PD-1 in CD8 T-cell exhaustion during chronic viral infection. Proc Natl Acad Sci USA. (2010) 107:14733–8. doi: 10.1073/pnas.1009731107
168. Di Loria A, Squillacioti C, De Luca A, Veneziano V, Mirabella N, Guccione J, et al. Increased leptin mRNA expression in the blood of dogs naturally infected by Leishmania infantum. Vet J. (2014) 202:634–6. doi: 10.1016/j.tvjl.2014.10.010
170. Machado FS, Mukherjee S, Weiss LM, Tanowitz HB, Ashton AW. Bioactive lipids in Trypanosoma cruzi infection. Adv Parasitol. (2011) 76:1–31. doi: 10.1016/B978-0-12-385895-5.00001-3
171. Miao Q, Ndao M. Trypanosoma cruzi infection and host lipid metabolism. Mediators Inflamm. (2014) 2014:902038. doi: 10.1155/2014/902038
172. Nagajyothi F, Desruisseaux MS, Thiruvur N, Weiss LM, Braunstein VL, Albanese C, et al. Trypanosoma cruzi infection of cultured adipocytes results in an inflammatory phenotype. Obesity (2008) 16:1992–7. doi: 10.1038/oby.2008.331
173. Rodrigues CM, Valadares HM, Francisco AF, Arantes JM, Campos CF, Teixeira-Carvalho A, et al. Coinfection with different Trypanosoma cruzi strains interferes with the host immune response to infection. PLoS Negl Trop Dis. (2010) 4:e846. doi: 10.1371/journal.pntd.0000846
174. Mukherjee S, Huang H, Petkova SB, Albanese C, Pestell RG, Braunstein VL, et al. Trypanosoma cruizi infection activates extracellular signal-regulated kinase in cultured endothelial and smooth muscle cells. Infect Immun. (2004) 72:5274–82. doi: 10.1128/IAI.72.9.5274-5282.2004
175. Wilkowsky SE, Barbieri MA, Stahl P, Isola ELD. Trypanosoma cruzi: phosphatidylinositol 3-kinase and protein kinase B activation is associated with parasite invasion. Exp Cell Res. (2001) 264:211–8. doi: 10.1006/excr.2000.5123
176. Hovsepian E, Penas F, Mirkin GA, Goren NB. Role of PPARs in Trypanosoma cruzi infection: implications for chagas disease therapy. PPAR Res. (2011) 2012:528435. doi: 10.1155/2012/528435
178. Baxt LA, Singh U. New insights into Entamoeba histolytica pathogenesis. Curr Opin Infect Dis. (2008) 21:489–94. doi: 10.1097/QCO.0b013e32830ce75f
179. Mortimer L, Chadee K. The immunopathogenesis of Entamoeba histolytica. Exp Parasitol. (2010) 126:366–80. doi: 10.1016/j.exppara.2010.03.005
180. Petri WA Jr, Mondal D, Peterson KM, Duggal P, Haque R. Association of malnutrition with amebiasis. Nutr Rev. (2009) 67 (Suppl. 2):S207–15. doi: 10.1111/j.1753-4887.2009.00242.x
181. Ali IK, Clark CG, Petri WA Jr. Molecular epidemiology of amebiasis. Infect Genet Evol. (2008) 8:698–707. doi: 10.1016/j.meegid.2008.05.004
182. Haque R, Mondal D, Duggal P, Kabir M, Roy S, Farr BM, et al. Entamoeba histolytica infection in children and protection from subsequent amebiasis. Infect Immun. (2006) 74:904–9. doi: 10.1128/IAI.74.2.904-909.2006
183. Blessman J, Van LP, Tanich E. Epidemiology of amebiasis in a region of high incidence of amebic liver abscess in central Vietnam. Am J Trop Med Hyg. (2002) 66:578–83. doi: 10.4269/ajtmh.2002.66.578
184. Siddiqui MA, Ekram ARSM, Islam QT, Hoque MA, Masum QAAI. Clinico-pathological profile of liver abscess in a teaching hospital. Teachers Assoc J Rajshahi (2008) 21:46–8.
185. Mondal D, Haque R, Sack RB, Kirkpatrick BD, Petri WA. Attribution of malnutrition to cause-specific diarrheal illness: evidence from a prospective study of preschool children in Mirpur, Dhaka, Bangladesh. Am J Trop Med Hyg. (2009) 80:824–6. doi: 10.4269/ajtmh.2009.80.824
187. Bousema T, Drakeley C. Epidemiology and infectivity of Plasmodium falciparum and Plasmodium vivax gametocytes in relation to malaria control and elimination. Clin Microbiol Rev. (2011) 24:377–410. doi: 10.1128/CMR.00051-10
188. Robert V, Bourgouin C, Depoix D, Thouvenot C, Lombard MN, Grellier P. Malaria and obesity: obese mice are resistant to cerebral malaria. Malaria J. (2008) 7:81. doi: 10.1186/1475-2875-7-81
189. Finlay D, Cantrell DA. Metabolism, migration and memory in cytotoxic T cells. Nat Rev Immunol. (2011) 11:109–17. doi: 10.1038/nri2888
190. Hughes SM, Amadi B, Mwiya M, Nkamba H, Tomkins A, Goldblatt D. Dendritic cell anergy results from endotoxemia in severe malnutrition. J Immunol. (2009) 183:2818–26. doi: 10.4049/jimmunol.0803518
191. Bourke CD, Berkley JA, Prendergast AJ. Immune dysfunction as a cause and consequence of malnutrition. Trends Immunol. (2016) 37:386–98. doi: 10.1016/j.it.2016.04.003
193. Vernooy JHJ, Ubags NDJ, Brusselle GG, Tavernier J, Suratt BT, Joos GF, et al. Leptin as regulator of pulmonary immune responses: involvement in respiratory diseases. Pulmon Pharmacol Therapeut. (2013) 26:464–72. doi: 10.1016/j.pupt.2013.03.016
194. Duncan SA, Baganizi DR, Sahu R, Singh SR, Dennis VA. SOCS proteins as regulators of inflammatory responses induced by bacterial infections: a review. Front Microbiol. (2017) 8:2431. doi: 10.3389/fmicb.2017.02431
195. Paracha RZ, Ahmad J, Ali A, Hussain R, Niazi U, Tareen SHK, et al. Formal modelling of toll like receptor 4 and JAK/STAT signalling pathways: insight into the roles of SOCS-1, interferon-β and proinflammatory cytokines in sepsis. PLoS ONE (2014) 9:e108466. doi: 10.1371/journal.pone.0108466
196. Akhtar LN, Qin H, Muldowney MT, Yanagisawa LL, Kutsch O, Clements J E, et al. Suppressor of cytokine signaling 3 inhibits antiviral IFN- β signaling to enhance HIV-1 replication in macrophages. J Immunol. (2010) 185:2393–404. doi: 10.4049/jimmunol.0903563
197. Wei H, Wang S, Chen Q, Chen Y, Chi X, Zhang L, et al. Suppression of interferon lambda signaling by SOCS-1 results in their excessive production during influenza virus infection. PLoS Pathog. (2014) 10:e1003845. doi: 10.1371/journal.ppat.1003845
198. Michaud F, Coulombe F, Gaudreault E, Paquet-Bouchard C, Rola- Pleszczynski M, Gosselin J. Epstein-Barr virus interferes with the amplification of IFN-α secretion by activating suppressor of cytokine signaling 3 in primary human monocytes. PLoS ONE (2010) 5:e11908. doi: 10.1371/journal.pone.0011908
199. Srivastav S, Basu Ball W, Gupta P, Giri J, Ukil A, Das PK. Leishmania donovani prevents oxidative burst-mediated apoptosis of host macrophages through selective induction of suppressors of cytokine signaling (SOCS) proteins. J Biol Chem. (2014) 289:1092–105. doi: 10.1074/jbc.M113.496323
200. Banerjee R, Kumar S, Sen A, Mookerjee A, Roy S, Pal S, et al. TGF-β-regulated tyrosine phosphatases induce lymphocyte apoptosis in Leishmania donovani-infected hamsters. Immunol Cell Biol. (2011) 89:466–74. doi: 10.1038/icb.2010.108
201. Bertholet S, Dickensheets H L, Sheikh F, Gam AA, Donnelly R P, Kenney RT. Leishmania donovani-induced expression of suppressor of cytokine signaling 3 in human macrophages: a novel mechanism for intracellular parasite suppression of activation. Infect Immun. (2003) 71:2095–101. doi: 10.1128/IAI.71.4.2095-2101.2003
202. Blanchette J, Racette N, Faure R, Siminovitch KA, Olivier M. Leishmania-induced increases in activation of macrophage SHP-1 tyrosine phosphatase are associated with impaired IFN-gamma-triggered JAK2 activation. Eur J Immunol. (1999) 29:3737–44. doi: 10.1002/(SICI)1521-4141(199911)29:11 < 3737::AID-IMMU3737>3.0.CO;2-S
203. Forget G, Gregory DJ, Whitcombe LA, Olivier M. Role of host protein tyrosine phosphatase SHP-1 in Leishmania donovani-induced inhibition of nitric oxide production. Infect Immun. (2006) 74:6272–79. doi: 10.1128/IAI.00853-05
204. Alti D, Sambamurthy C, Kalangi SK. Emergence of leptin in infection and immunity: scope and challenges in vaccines formulation. Front Cell Infect Microbiol. (2018) 8:147. doi: 10.3389/fcimb.2018.00147
205. Stahl P, Schwarz RT, Debierre-Grockiego F, Meyer T. Trypanosoma cruzi parasites fight for control of the JAK-STAT pathway by disarming their host. JAKSTAT (2015) 3:e1012964. doi: 10.1080/21623996.2015.1012964
Keywords: leptin, leishmaniasis, trypanosomiasis, amoebiasis, malaria, bacteria, virus, malnutrition
Citation: Maurya R, Bhattacharya P, Dey R and Nakhasi HL (2018) Leptin Functions in Infectious Diseases. Front. Immunol. 9:2741. doi: 10.3389/fimmu.2018.02741
Received: 20 March 2018; Accepted: 07 November 2018;
Published: 26 November 2018.
Edited by:
Xun Suo, China Agricultural University, ChinaReviewed by:
Theo Araújo-Santos, Universidade Federal do Oeste da Bahia, BrazilAbhigyan Satyam, Harvard Medical School, United States
Copyright © 2018 Maurya, Bhattacharya, Dey and Nakhasi. This is an open-access article distributed under the terms of the Creative Commons Attribution License (CC BY). The use, distribution or reproduction in other forums is permitted, provided the original author(s) and the copyright owner(s) are credited and that the original publication in this journal is cited, in accordance with accepted academic practice. No use, distribution or reproduction is permitted which does not comply with these terms.
*Correspondence: Radheshyam Maurya, rmusl@uohyd.ernet.in; radhemaurya@rediifmail.com