- 1Division of Nephrology, Department of Internal Medicine, University of Groningen, University Medical Center Groningen, Groningen, Netherlands
- 2Department of Obstetrics and Gynecology, Martini Hospital, Groningen, Netherlands
- 3Department of Nephrology, University of Leiden, Leiden University Medical Center, Leiden, Netherlands
- 4Department of Immunology, Oslo University Hospital and University of Oslo, Oslo, Norway
- 5Research Laboratory, Bodø Hospital, and K.G. Jebsen TREC, University of Tromsø, Tromsø, Norway
- 6Centre of Molecular Inflammation Research, Norwegian University of Science and Technology, Trondheim, Norway
- 7Immunorheumatology Research Laboratory, Istituto Auxologico Italiano, IRCCS, Milan, Italy
- 8Department of Hygiene, Microbiology and Public Health, Medical University of Innsbruck, Innsbruck, Austria
- 9Department of Rheumatology, Leiden University Medical Center, Leiden, Netherlands
- 10Department of Immunohematology and Blood Transfusion, Leiden University Medical Center, Leiden, Netherlands
- 11Department of Laboratory Medicine, Section of Microbiology, Immunology and Glycobiology, Lund University, Lund, Sweden
- 12Department of Medical Microbiology and Immunology, St. Antonius Hospital, Nieuwegein, Netherlands
Introduction: The complement system is essential for an adequate immune response. Much attention has been given to the role of complement in disease. However, to better understand complement in pathology, it is crucial to first analyze this system under different physiological conditions. The aim of the present study was therefore to investigate the inter-individual variation in complement activity and the influences of age and sex.
Methods: Complement levels and functional activity were determined in 120 healthy volunteers, 60 women, 60 men, age range 20–69 year. Serum functional activity of the classical pathway (CP), lectin pathway activated by mannan (MBL-LP) and alternative pathway (AP) was measured in sera, using deposition of C5b-9 as readout. In addition, levels of C1q, MBL, MASP-1, MASP-2, ficolin-2, ficolin-3, C2, C4, C3, C5, C6, C7, C8, C9, factor B, factor D, properdin, C1-inhibitor and C4b-binding protein, were determined. Age- and sex-related differences were evaluated.
Results: Significantly lower AP activity was found in females compared to males. Further analysis of the AP revealed lower C3 and properdin levels in females, while factor D concentrations were higher. MBL-LP activity was not influenced by sex, but MBL and ficolin-3 levels were significantly lower in females compared to males. There were no significant differences in CP activity or CP components between females and males, nevertheless females had significantly lower levels of the terminal components. The CP and AP activity was significantly higher in the elderly, in contrast to MBL-LP activity. Moreover, C1-inhibitor, C5, C8, and C9 increased with age in contrast to a decrease of factor D and C3 levels. In-depth analysis of the functional activity assays revealed that MBL-LP activity was predominantly dependent on MBL and MASP-2 concentration, whereas CP activity relied on C2, C1-inhibitor and C5 levels. AP activity was strongly and directly associated with levels of C3, factor B and C5.
Conclusion: This study demonstrated significant sex and age-related differences in complement levels and functionality in the healthy population. Therefore, age and sex analysis should be taken into consideration when discussing complement-related pathologies and subsequent complement-targeted therapies.
Introduction
The complement system, a major component of innate immunity, plays a crucial role in the immune response. In health, maintaining a balance between activation and inhibition of the complement system is key to preserve tissue homeostasis and to enable immune surveillance (1, 2). However, an overactive system can cause autoimmune and inflammatory diseases, whereas an inactive complement system results in an increased risk for infection. Several elements can disrupt this delicate balance and with age these effects are exacerbated. Complement deficiencies or dysfunctions have been shown to be associated with diseases such as atypical hemolytic uremic syndrome (aHUS), age-related macular degeneration (AMD), paroxysmal nocturnal hemoglobinuria (PNH), systemic lupus erythematosus (SLE), C3 glomerulopathy (C3G), and other kidney diseases (3, 4). Furthermore, complement activation contributes to several diseases and pathological conditions such as renal replacement therapy (5–7), cancer (8), hypersensitivity reactions (9, 10), and neurological conditions (11).
The complement system is activated via an enzymatic cascade reaction and has three different activation pathways, namely the classical pathway (CP), the lectin pathway (LP), and the alternative pathway (AP). Complement activity for each of these pathways depends on the expression and function of a large number of complement proteins. The CP is mainly initiated by immune complexes binding to C1q leading to activation of C1r and C1s, but can also be activated in an antibody independent manner like C1q binding to C-reactive protein. The LP is initiated by the binding of mannose-binding lectin (MBL), ficolins, or collectins to sugars or acetylated compounds resulting in the activation of the MBL-associated serine protease (MASP)-1 and MASP-2. Recently it has also been shown that the LP can be activated by naturally occurring antibodies, underscoring the overlap and cross-talk between the pathways (12). LP and CP activation both lead to the cleavage of C4 and C2, and subsequently to the generation of C4b2a, the C3-convertase. Furthermore, C1-inhibitor (C1-INH) regulates the activity of the recognition complexes, while C4b-binding protein (C4bp) functions as a cofactor for factor I-mediated cleavage of C4b. Initiation of the AP occurs via spontaneous hydrolysis of C3 into C3(H2O) or by the binding of C3b to altered surfaces. Factor D cleaves factor B when the latter is complexed with C3b, creating the C3-convertase of the AP, C3bBb. In addition, this C3-convertase is stabilized by properdin, the only positive regulator of the complement system. Regardless of the initial pathway, complement activation can lead to the initiation of the terminal pathway (TP) and thereby the generation of C5-convertase, which cleaves C5 in C5a, a powerful anaphylatoxin, and C5b. Next, C5b binds C6 which then attaches to a surface and interacts with C7, C8, and C9 to form the membrane attack complex (MAC/C5b-9) (13, 14). If there is no surface present, C5b6 will bind to C7, C8 and C9 together with the control proteins vitronectin and clusterin in the fluid-phase and thereby the soluble form of the terminal complement complex, sC5b-9, is formed. To prevent unintended complement activation the system is kept under control by a variety of regulators. The major regulators of the AP are the plasma proteins factor H and factor I. Factor H inhibits complement activation by accelerating the decay of the C3bBb convertase of the AP and by providing cofactor activity for factor I-mediated cleavage of C3b.
Both age and sex are known to influence and significantly impact the immune system (15). Females and males have distinct innate and adaptive immune responses (16). Moreover, they also differ in their immunological responses to self and foreign-antigens (17). Sex-based immunological differences can be found in various species (18). In general, the immune response seems to be stronger in females than in males (15). These immunological sex differences are thought to arise from discrepancies in hormones, genetic factors and environmental mediators. Notably, these sex-related differences in the immune system could give insight into the epidemiology and etiology of autoimmune and infectious diseases (15). Likewise, aging is associated with a progressive decline in immunity. The impact of aging on adaptive immunity is well accepted (19). However, less certainty exists on the effect of aging on innate immunity (20). Impaired function of neutrophils and macrophages as well as reduced interaction between dendritic cells and T cells, suggest also a decline in innate immune function (21). As a result of impaired immune function, the ability of elderly to respond to microorganisms is diminished and the number of infectious disease is increased (22). Also the increased incidence of autoimmune diseases might be related to altered immunity in elderly (23).
In the current era of personalized medicine combined with the recent success of complement inhibitors in clinical trials, it is essential to identify the influence of sex and age on the complement system. Given that complement therapeutics are an effective treatment for complement-mediated diseases, individuals will need different doses in order to efficiently block the complement system depending on the concentrations and functional activity of the complement components. Factors influencing the levels could be e.g., sex and age. Thus, the aim of the present study was to explore the effect of age and sex on the complement system in a healthy Caucasian population by performing functional and quantitative complement analyses. The current study also enables us to better understand the complement system in different physiological conditions.
Materials and Methods
Serum Samples
Serum samples were obtained from a population of 120 healthy Caucasian individuals from Norway, registered as blood donors. From each sex, 12 samples were obtained per age decade between 20 and 70. Sixty females with a mean age of 44.7 years (range: 20–69 years) and 60 males with a mean age of 45.1 years (range: 20–65 years) were included. Serum samples obtained were directly aliquoted and stored at −80°C.
Assessment of Pathway Activity in Normal Human Serum Samples
The complement kit (Complement System Screen Wieslab®, Eurodiagnostica, Malmö, Sweden) for assessment of CP, LP, and AP activity was used according to the manufacturer's instructions (24, 25). In brief, strips of wells for CP were coated with IgM, strips for MBL-LP were coated with mannan and strips for AP were coated with LPS. Sera were diluted 1/101 for the CP and MBL-LP assays and 1/18 for the AP assay in specific buffers to ensure that activation of only the actual pathway occurred, and were incubated for 1 h at 37°C. After washing, alkaline phosphatase-conjugated anti-human C5b-9 was added before incubation at room temperature for 30 min. Additional washing was performed, substrate was added and the wells were incubated for 30 min. Finally, absorbance values were read at 450 nm. In each assay standard positive and negative control sera, provided in the kit as lyophilized material and reconstituted with distilled water, were assessed. The positive standard serum was a pool of 5 sera from healthy individuals and the negative control consisted of sera heat-inactivated at 56°C for 20 min. Complement activity was calculated using the following formula: activity = 100% x (mean A450 (sample)–mean A450 (negative control) / (mean A450 (standard serum) - mean A450 (negative control). Samples as well as standard serum and negative control serum were tested in duplicate.
Serum Complement Concentrations
Assessment of MBL concentrations was performed using a commercial MBL ELISA obtained from BIOPORTO Diagnostics A/S (Hellerup, Denmark) and performed according to the manufacturer's instructions. C1q, C4, C3, and C1-inhibitor concentrations were assessed using nephelometry on a BN-Prospec, with validated diagnostic protocols provided by the manufacturer (Siemens Healthcare Diagnostics, Marburg, Germany), calibrated using a complement standard serum provided by the manufacturer. C2 and factor B were measured using rocket-immunoelectrophoresis as described by Sjöholm et al. (26–28). Properdin and Factor D were assessed by electroimmunoassay as previously described (29, 30). Concentrations of C2, factor B, properdin and factor D were expressed in arbitrary units (% of pooled human serum).
MASP-1, MASP-2, Ficolin-2, and Ficolin-3 levels were determined using in-house ELISAs. For all in house ELISA's (Leiden University Medical Center, Leiden, the Netherlands) a standard protocol was used. In brief, Nunc Maxisorb plates (Nunc, Roskilde, Denmark) were coated using coating buffer (100 mM Na2CO3/NaHCO3, pH 9.6), for 16 h at room temperature. After each step, plates were washed three times with PBS containing 0.05% Tween 20. Residual binding sites were blocked by incubation with PBS containing 1% BSA. Unless otherwise indicated, all subsequent steps were incubated in PBS containing 0.05% Tween 20 and 1% BSA, for 1 h at 37°C. Detection antibodies were conjugated to digoxigenin (Dig) using Dig-3-O-methylcarbonyl-ε-aminocaproic acid-N-hydroxysuccinimide ester (from Boehringer Mannheim, Mannheim, Germany), followed by detection using HRP-conjugated rabbit anti-Dig Abs (Fab, from Boehringer Mannheim). Enzyme activity of HRP was detected using 2,2′-azino-bis(3-ethylbenzthiazoline-6-sulfonic acid) (Sigma) and absorption was measured at 415 nm.
In more detail, for the detection of MASP-1 and MASP-2, polyclonal antibodies were raised in rabbits against the recombinant protease domain of MASP-1 and MASP-2, respectively, kindly provided by Dr. Peter Gál (31). However, based on the specificity of the anti-MASP-1 antibody it is likely that the results from this ELISA represent the sum of MASP-1 and MASP-3 levels. ELISA plates were coated with rabbit IgG anti-MASP-1 and rabbit IgG anti-MASP-2, respectively. Patient samples were incubated in GVB/NaCl/EDTA (Veronal-buffered saline containing 0.05 % Tween-20, 0.1 % gelatine, 0.5M NaCl, 10 mM EDTA; pH 7.5), followed by detection using the same antibodies, which were conjugated as described above. Results were expressed in arbitrary units per ml using a standard line of serially diluted pooled normal human serum for calibration. For detection of ficolin-2, a similar protocol was followed using mouse monoclonal antibody GN5. Results were expressed in pg/ml as described previously (32). Both GN5 and 4H5 were kindly provided by dr. T. Fujita, Department of Immunology, Fukushima Medical University, Fukushima, Japan). For detection of ficolin-3, the standard ELISA protocol was followed and the mouse monoclonal antibody 4H5 was used for both coating and detection. Results were expressed in arbitrary units/ml using pooled human serum for calibration.
C4bp, C5, C8, and C9 were measured by ELISA as previously described (33–35). C6 and C7 were measured using an in-house ELISA assay as previously described (36). In brief, wells were coated with an in house mouse monoclonal antibody against C6 (WU 6-4, Hycult, Uden, NL) and with an in house polyclonal antibody against C7. For the detection, respective biotinylated polyclonal antibodies were used. For the set-up of the ELISA, purified C6 or C7 (Cytotech, San Diego, CA, USA) were used to calibrate human serum as a standard.
The standards used in the different assays were not calibrated to the official European complement standard.
Statistics
Statistical analysis was performed using IBM SPSS 22.0 (IBM Corporation, Chicago, IL, USA). Concentrations of complement proteins and pathway activity were presented as median with interquartile range [IQR]. Differences between sexes were assessed with the Mann Whitney U test. Correlation between age and complement proteins or pathway activity was evaluated using the Spearman Rank correlation coefficient (r). Univariate and subsequent multivariate linear regression analysis were performed to identify independent determinants of complement pathway activity. Multivariate analysis models were constructed using backward selection (Pout > 0.05) including complement proteins that were associated significantly (P < 0.05) with complement pathway activity in univariate analysis. P < 0.05 were considered statistically significant.
Results
Differences Between Males and Females in Complement Pathway Activity and Components
CP, MBL-LP, and AP activity were determined by deposition of C5b-9 in serum samples from 60 males and 60 females. CP and MBL-LP activity were similar in both sexes (Figure 1). However, AP activity was significantly lower in females compared to males. The median AP activity for females was 69.5% compared with 81.0% for males (P < 0.001, Figure 1).
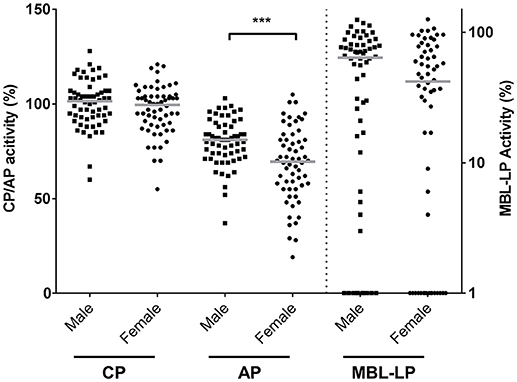
Figure 1. Complement pathway activity according to sex. The activity of the classical pathway (CP), alternative pathway (AP), and MBL-induced lectin pathway (MBL-LP) was measured in 120 Caucasian healthy subjects, of which 60 males and 60 females. The solid lines indicate the median values in each group. The differences between males and females was assessed by the Mann Whitney test (***P < 0.001). CP/AP activity is referred to the left Y-axis in linear scale whereas MBL-LP activity is referred to the right Y-axis in a logarithm scale.
In accordance, the levels of C3 and properdin were also significantly lower in women in comparison with men. Median C3 concentrations in females were 1.37 mg/mL [1.19–1.59] compared to 1.51 mg/mL [1.34–1.76] in males (P = 0.001, Figure 2A), whereas median levels of properdin in females were 107% [91–128] vs. 120% [98–138] in males (P = 0.03, Figure 2B). Conversely, serum concentration of factor D showed an opposite pattern (P < 0.001, Figure 2C), since levels were significantly higher in females (140%, IQR: 115%−200%) than in males (100%, IQR: 82%−137%). Males had higher levels of serum MBL in relation to female subjects. Median MBL concentrations were 533 ng/mL [142–1,076] and 843 ng/mL [289–1,646] in females and males, respectively (P = 0.03, Figure 2D). Likewise, female subjects also had significantly lower levels of ficolin-3 compared to male subjects, with a median of 830 AU/mL [676–1,034] and 1042 AU/mL [883–1,192] in females and males, respectively (P = 0.001, Figure 2E).
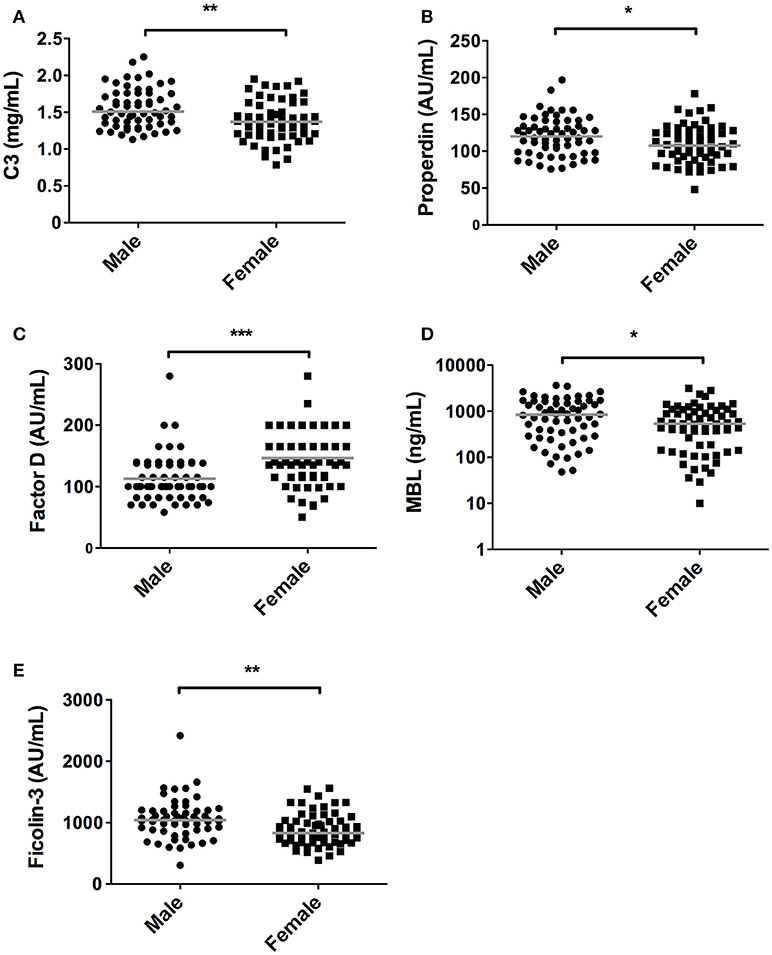
Figure 2. Differences in complement components between sexes. In 120 subjects, consisting of 60 males and 60 females complement levels of (A) C3, (B) Properdin, (C) Factor D, (D) MBL, (E) Ficolin-3 were measured. The solid lines indicate the median values in each group. The differences between males and females was assessed by the Mann Whitney test (*P < 0.05, **P < 0.01, ***P < 0.001).
The rest of the components measured did not differ between females and males (Table 1). In line with CP activity, serum levels of C1q, C4, C2, C1-INH, and C4bp did not differ between the sexes (Table 1). Furthermore, despite the fact that MBL-LP activity was not significantly different between the sexes, a trend was still seen for lower activity in females compared to males. Additionally, 17% of the males were MBL deficient (0% MBL-LP activity) whereas in females this was 23%. The threshold of MBL concentration to result in zero activity was 130 ng/mL. The other components from the LP such as MASP-1, MASP-2 and Ficolin-2 did not differ between both sexes (Table 1).
Quantification of the terminal complex components revealed that all these components, except C6, were significantly lower in female subjects compared to male subjects (P < 0.05, Figure 3). The presented data show that in women, levels were 53, 15, 59, and 14% lower, for C5, C7, C8, and C9, respectively. For C8, concentrations above 50 μg/ml were exclusively observed in males (65% of male subjects had concentrations of C8 above 50 μg/ml).
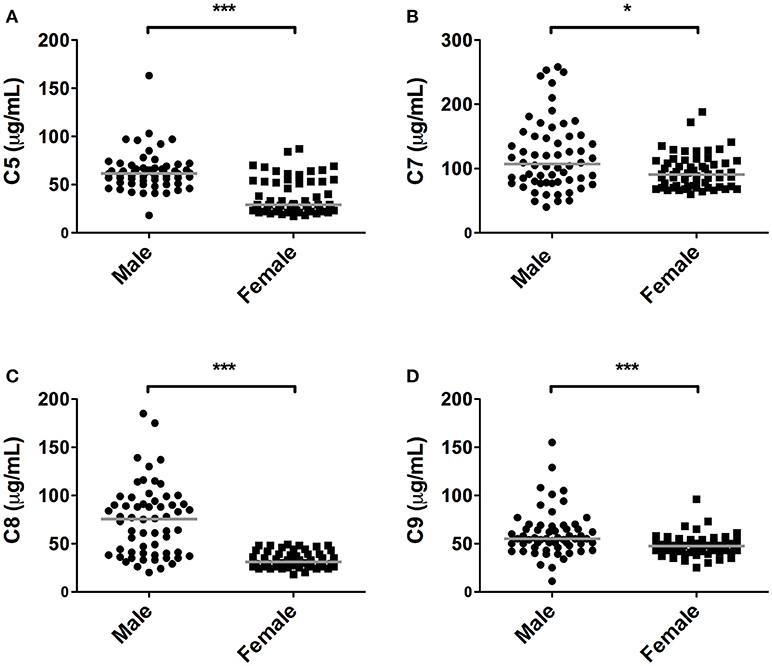
Figure 3. Differences in terminal pathway components between sexes. In 120 subjects, consisting of 60 males and 60 females, complement levels of (A) C5, (B) C7, (C) C8, and (D) C9 were measured. The solid lines indicate the median values in each group. The differences between males and females was assessed by the Mann Whitney test (*P < 0.05, ***P < 0.001).
Age-Related Changes in Complement Pathway Activity and Components
Next, we determined the effect of age on the complement system by correlating complement components and activity with age in 120 healthy individuals with a mean age of 45 years ± 13.5, ranging from 20 to 69 years old. Linear regression analysis demonstrated a significant age-related effect for CP and AP activity, but not for the MBL-LP activity (data not shown). In accordance, age significantly correlated with CP activity (r = 0.42, P < 0.001, Figure 4A) and AP activity (r = 0.30, P < 0.001, Figure 4B), but not with MBL-LP activity (Figure 4C).
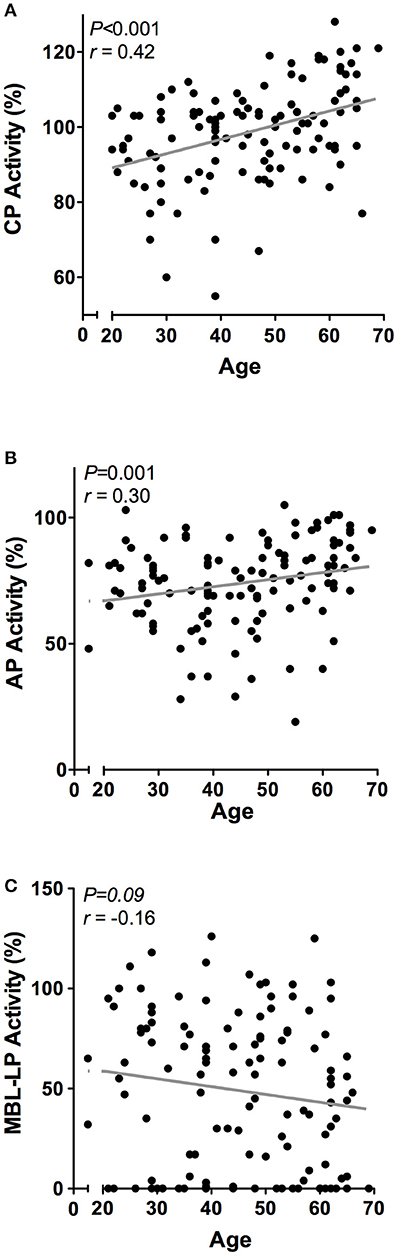
Figure 4. Correlations between complement pathway activity and age. In 120 healthy subjects age was correlated to the activity of the (A) classical pathway (CP), (B) alternative pathway (AP) and (C) MBL-induced lectin pathway (MBL-LP). These correlations were evaluated using the Spearman Rank correlation coefficient. P < 0.05 were considered to be statistically significant.
However, when the twenty-five oldest individuals (mean age 62 ± 2.3 years) were compared to the twenty-five youngest individuals (mean age 26 ± 3.3 years) significant differences were observed in all pathways: higher CP activity (106 vs. 91%, P < 0.001), lower MBL-LP activity (35 vs. 60%, P = 0.01) and higher AP activity (83 vs. 74%, P = 0.01). Subsequently, we analyzed the effect of age on the concentration of the different complement components and factors. Regardless of statistics, a correlation coefficient below 0.3 was not perceived as clinically relevant (37). A significant effect of age was observed for C1-INH, factor D, C5, C8, and C9 levels (Figure 5). C1-INH showed an age-related rise (Figure 5A, r = 0.30, P = 0.001), whereas factor D (Figure 5B, r = −0.32, P = 0.001) levels decreased with age. Consistent with the age-related increase in CP and AP activity, levels of C5 (Figure 5C, r = 0.40, P < 0.001), C8 (Figure 5D, r = 0.43, P < 0.001) and C9 (Figure 5E, r = 0.31, P = 0.001) positively correlated with age. The comparison between the oldest and youngest twenty-five individuals showed that C5, C8, and C9 levels increased by 47% (P < 0.001), 60% (P < 0.001), and 24% (P = 0.001), respectively, with age. Finally, if we correct for sex, the impact of age on CP and AP activity remains significant. However, the age-related effect on AP is predominantly found in female subjects (females: r = 0.35, P = 0.05), whereas the effect of age on CP activity was predominantly found in male subjects (males: r = 0.61, P < 0.001).
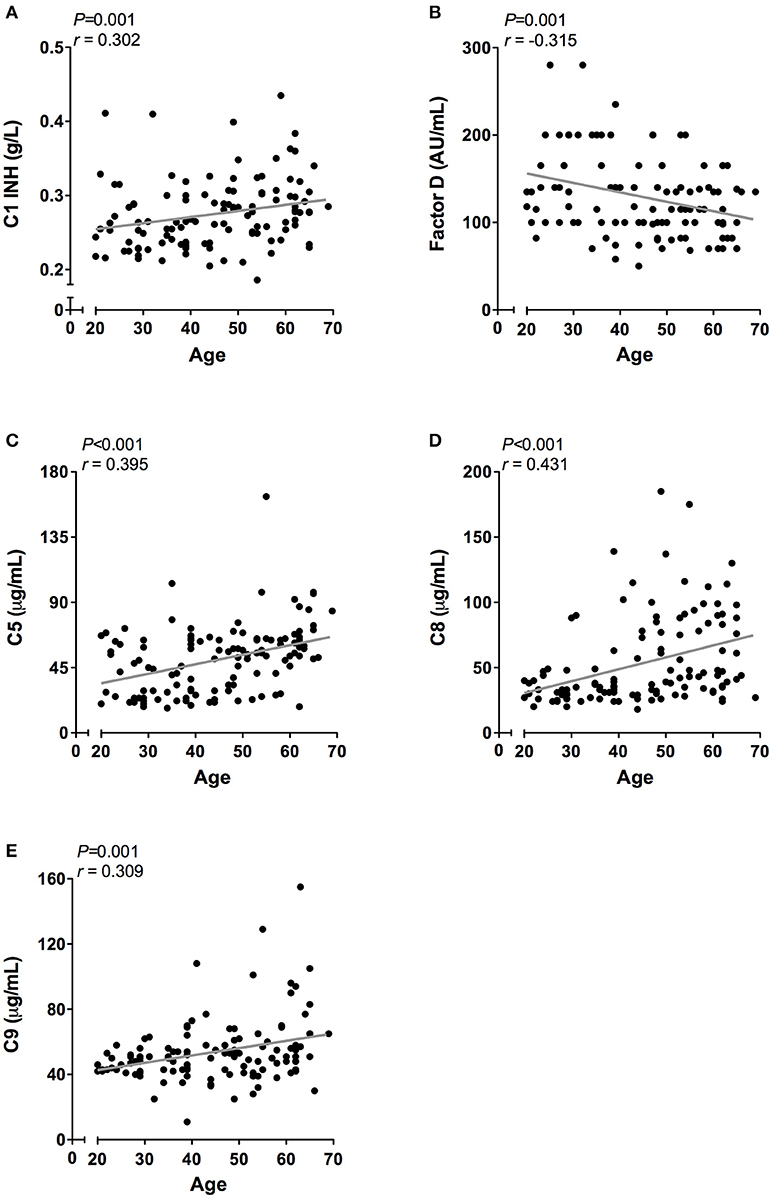
Figure 5. Correlation of complement proteins with age. In 120 healthy subjects age was correlated to the concentration of (A) C1-INH, (B) Factor D, (C) C5. (D) C8, and (E) C9. These correlations were evaluated using the Spearman Rank correlation coefficient. P < 0.05 were considered to be statistically significant.
The Relationship Between Complement Components and Pathway Activity
To better understand the determinants of complement activity of the different pathways, we related individual complement components with their respective complement pathway (Tables 2–4). CP activity correlated significantly with C2 levels (Table 2, r = 0.51, P < 0.001), whereas MBL-LP activity significantly correlated with MBL concentrations (Figure 6, Table 3, r = 0.89, P < 0.001). Furthermore, for AP activity significant correlations were seen with properdin (Table 4, r = 0.35, P < 0.001), factor B (Table 4, r = 0.51 P < 0.001) and C3 (Table 4, r = 0.42, P < 0.001). In addition, we correlated terminal pathway components with complement activity of the different pathways (Table 5). CP activity correlated significantly with C5 (Table 5, r = 0.39, P < 0.001) and C9 (Table 2, r = 0.42, P < 0.001), while AP activity correlated with C5 (Table 5, r = 0.56, P < 0.001), C8 (Table 5, r = 0.43, P < 0.001) and C9 (Table 5, r = 0.38, P < 0.001). MBL-LP activity did not correlate with any of the terminal pathway components (Table 5). However, besides the correlation between individual complement components with their respective complement pathway, there were also correlations between different complement components with each other. The latter could form a possible confounder for the pathway analysis. Subsequently, we performed a multivariate linear regression analysis and found that levels of C2, C1-INH, and C5 were independent determinants of CP activity (model R2 = 0.46, Table 6), whereas MBL and MASP-2 were independent determinants of MBL-LP activity (model R2 = 0.66, Table 6). Moreover, the multivariate linear regression analysis demonstrated that only C3, factor B and C5 were independent determinants of AP activity (model R2 = 0.46, Table 6).
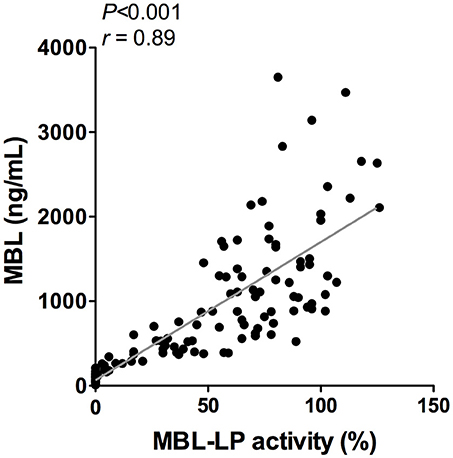
Figure 6. Correlation between MBL-LP activity and MBL levels. In 120 healthy subjects MBL levels were correlated to the activity of the MBL-LP (%). This correlation was evaluated using the Spearman Rank correlation coefficient. A p < 0.05 was considered to be statistically significant.
Discussion
In the current study, we demonstrate in a healthy Caucasian population that sex and age significantly impact the complement system. Sex-related analysis revealed that females have lower AP activity and lower AP and LP complement components, with an exception for factor D. In addition, females showed significantly lower levels of C3 and terminal pathway components. These results demonstrate that females have significantly lower complement activity and levels of complement components compared to males. Furthermore, age-related analysis showed that aging is associated with an enhanced functional activity of the CP and the AP. Correspondingly, terminal pathway components levels increased with age. Lastly, we analyzed the determinants of functional complement pathway activity, by relating individual complement components with their respective complement pathway. In healthy individuals, CP activity was determined by C2, C1-INH, and C5, MBL-LP activity by MBL and AP activity by C3, factor B and C5. These results support the relevance of age- and sex-matched control cohorts in studies related to the complement field, including the assessment of reference values used in clinical laboratory diagnostics. Moreover, these results suggest that age and sex should be taken into account in complement-related pathology as well as in complement-targeted therapies.
For the majority of complement components the liver is the predominant source of production, and production of complement proteins can be regulated by an acute phase response (38). Other main production sites includes peripheral blood mononuclear cells which are responsible for the production of C1q, properdin and C7, adipocytes which produce factor D and the lungs where ficolin-3 is mainly produced (39, 40). In fact, most tissues and inflammatory cells are able to produce various complement proteins, e.g., upon stimulation with cytokines (40). Additionally, genetic environmental and lifestyle factors such as obesity and smoking also influence complement levels (41–43). Multiple complement deficiencies have been described and associated with pathology (44). In addition, single-nucleotide polymorphisms (SNP) can strongly affect the concentration and/or function of various complement proteins, as have been well-documented for e.g., MBL (45, 46). Yet, limited studies have investigated the influence of sex and age on the complement system (47–49).
The immune system varies between males and females and differences in innate and adaptive immunity have already been demonstrated. At least two factors are known to explain the influences of sexual dimorphism in immunity: genetics (e.g., the X chromosome) and hormonal differences (50). Remarkably, the X chromosome contains genes that encode for several proteins related to immune response such as toll-like receptors and interleukins. Moreover, properdin is encoded on the short arm of the X chromosome (51). However, in our study, females had lower properdin levels than males, demonstrating once more that genetic factors do not solely determine the concentration of the components. In addition, sex hormones are known to influence innate and adaptive immunity. The majority of the cells from innate and adaptive immunity express estrogen receptors (52). However, data on the influence of sex on the complement system remains limited. A study by Roach et al. demonstrated that in children (age range 1–19 years) there were differences in several complement components between boys and girls (49). However, the differences found in this study were age-dependent. Troldborg et al. studied complement proteins restricted to the lectin pathway and showed comparable results to ours, showing that female have lower complement levels than males (53). Previously, an animal study demonstrated the influence of sex hormones on the complement system by injecting estrogen and testosterone in healthy and castrated mice from both sexes (54). Mice treated with testosterone showed increased late acting complement activity while mice treated with estrogen showed diminished activity (48). In accordance, a recent animal study demonstrated lower complement functionality in female mice due to lower levels of terminal complement components (47). This has been a reason for our group to perform complement-related animals experiments solely in male rodents (55, 56). Altogether, these results in rodents are in line with our findings that females have lower terminal complement components and lower functional activity. In our study, only Factor D was significantly higher in woman. A possible explanation could be a higher amount of adipose tissue in woman than in men, resulting in enhanced production. Unfortunately, factor H and factor I were not determined in our cohort. Previous studies did not find significant differences between sexes in factor H levels in adults (57). In addition, very recent work did not observe an effect of sex or age in factor H levels in children (54). However, since we observed a lower AP functional activity in females, a possible explanation for this difference could be higher levels of factor H and/or factor I. Nonetheless, the clinical consequences of these differences remain to be elucidated. Yet, some clues already indicate that sex could form a possible confounder in complement-mediated diseases. For instance, in a cohort of healthy people, low MBL levels were associated with cardiovascular disease, however this association was seen only in men and not in women (58). Moreover, in a study of AMD, distinct alterations were shown between the sexes in levels of AP components (59). Thus, more studies are needed to clarify the significance of sexual dimorphism on the complement system. In the future, these shortcomings could be addressed by studying the complement system in transsexual subjects undergoing hormonal replacement therapy. Nevertheless, an important question that remains is: should we treat women and men equally when it comes to complement therapeutics?
In the early phase of life, innate immunity plays a fundamental role since adaptive immunity is still under development, whereas during adolescence this is reversed (60). Immunity undergoes severe deterioration with age (15, 60). Previous studies showed that both for innate and adaptive immunity, the function reduces with age (22, 61). Whether this decline in innate immune function is also true for the complement system was still unknown. In a Japanese healthy cohort, C3 levels varied according to different age ranges, however not in a continuous way (62). Certain LP components were also investigated in a large population and showed MBL levels are reduced in adults when compared to children (63) Previously, in a cohort of centenarians, MBL levels were reported to be reduced compared to the general population (64). However, this reduction was based on a higher prevalence of mbl2 gene mutations, suggesting a beneficial role of intermediate levels of MBL for longevity. In our study, we found that age had a minor effect on MBL-LP activity, but significantly enhanced the activity of CP and AP. Accordingly, in an earlier study in healthy subjects aged between 20 and 69 years old, increased CH50 activity during aging was observed and associated with increased levels of individual components of the CP (65). Moreover, in line with the enhanced functional activity of CP and AP, the levels of terminal pathway components C5, C8, and C9 also raised with age. However, an important limitation of our results is that our study design is cross-sectional and not longitudinal. Accordingly, our study is unable to discriminate between true age-related changes or better survival due to evolutionary advantages. Possible explanations for the aging immune system could be epigenetic changes, metabolic changes, changes in protein production and degradation and accumulation of senescence cells. Finally, the increased levels of terminal pathway components with age could be a mechanism to compensate for the impaired clearance of pathogens and apoptotic cells due to lower cellular immunity (66).
The current findings help to become conscious of changes in complement function during physiological conditions. Furthermore, the data obtained provide insight in the complex teamwork of all the different complement proteins to achieve the end goal, i.e., the production of the membrane attack complex upon complement activation. To further study the contribution of each individual component to their respective pathway(s) we first correlated individual complement components with their complement pathway function. However, since complement components also correlated with each other, this could cause a potential confounder. Thus, we next performed multivariate regression analysis, to correct for other components and confounders. In this analysis, MBL and MASP-2 levels were independent determinants of MBL-P activity. For the CP activity, C2 and C5 levels were the strongest determinants. In accordance, C2 has previously been described as the rate-limiting factor in the CP activation (67). In addition, C1-INH was also an independent determinant of CP activity, however with a negative value, confirming that C1-INH acts as a negative regulator of CP activation (68, 69). Furthermore, AP activity was dependent on C3, factor B and C5. Factor D has previously been described as the rate-limiting step of the AP, however the current study does not confirm these results (67, 70). Our studies confirm the key role of C5 as a rate limiting step for activation of the terminal pathway of complement, both via the CP and via the AP.
Expression of complement genes and complement function is at least partially genetically determined. In the present study, the function of the MBL pathway was strongly determined by the concentration of MBL, of which the expression of functional molecules is largely genetically controlled by a number of SNP in the promoter and coding region (45, 71). Our data also show that the concentration of factor B is positively correlated to the concentrations of C4 (r = 0.39) and C2 (r = 0.42), suggesting co-regulation of the genes involved, which are located in close proximity to each other in the MHC class III region at chromosome 6 (72). Similarly, expression of C5 and C8 is strongly correlated, suggesting co-regulation of expression of genes for C5 and C8-gamma, located at chromosome 9q33.
The present study focused on complement function and individual component concentration. It was out of the scope to investigate complement activation products reflecting basic ongoing in vivo complement activation. In contrast to differences found in native component concentrations, we have previously shown that a number of complement activation products did not differ between female and male blood donors indicating that the degree of physiologic activation not necessarily is reflected by the different individual component concentrations (73). Furthermore, the concentration of activation products did not differ between the age groups divided in decades from 20 to 70 years (unpublished data). Others have shown that there is an individual diurnal variation of complement components and anaphylatoxins dependent of sleep (74).
We acknowledge that the present study has limitations. Although our cohort includes a variety of subjects in different age ranges, one limitation is the absence of age below and above blood donor acceptance (i.e., 20 and 69 years). Additionally, we did not have genetic and lifestyle background from the subjects of the study, which could add valuable information. However, all donors were accepted as blood donors according to the health criteria for donating blood at the hospital, implying that they represent the health part of the population. Furthermore, the complement system comprises over more than 50 proteins and we were therefore unable to determine all complement proteins. Concerning LP, the current study only measured functional activity of MBL and not from the other initiators such as ficolins and collectins. Finally, the standards used in the different assays were not calibrated to the official European complement standard. However, this would not change our conclusions, just the absolute values. On the other hand, strengths include the number of complement proteins determined, the combination of quantitative and functional analysis, the population size and the in-depth performed statistical analysis.
In conclusion, there are important sex- and age-related differences in the complement system. These changes should be taken into account when studying complement-related diseases. Furthermore, complement therapies that are now reaching the clinical phase should be tested for the different sexes and age ranges since different complement profiles might affect the efficiency of a therapy.
Ethics Statement
The study was approved by the local ethical committee of the North-Norwegian Health Authority. The Blood donors were recruited from the Bloodbank and signed a written informed consent before blood donation.
Author Contributions
Research idea and study design by MS, AR, MD, CvK; data acquisition by MS, AR, TM, FT, RW, LAT and LeT; data analysis/interpretation by MG, FP, MS, and AR. Statistical analysis by FP, MG; FP, MG, and MS wrote the manuscript. All authors were involved in editing the final manuscript. All authors read and approved the final manuscript.
Conflict of Interest Statement
The authors declare that the research was conducted in the absence of any commercial or financial relationships that could be construed as a potential conflict of interest.
Acknowledgments
The authors like to thank the members of the EU consortium Complement in disease for helpful comments and discussions, Dr. M.W. Turner, Dr. J. Wieslander, Dr. T. Fujita, and Dr. Peter Gál for valuable reagents used in the present studies. The authors thank the department of Clinical Chemistry, LUMC, Leiden, The Netherlands for their contribution in nephelometric measurements. Part of this work was supported by a grant from the European Union (QLGT-CT2001-01039) and by the Dutch Kidney Foundation (PC 95, C98-1763).
Abbreviations
aHUS, Atypical haemolytic uremic syndrome; AMD, Age-related macular degeneration; AP, Alternative pathway; C1-INH, C1-inhibitor; C4bp, C4b-binding protein; C3G, C3 glomerulopathy; CP, Classical pathway; IQR, Interquartile range; LP, Lectin pathway; MAC, Membrane attack complex; MASP, MBL-associated serine protease; MBL, Mannan-binding lectin; MBL-LP, MBL-induced lectin pathway; PNH, Paroxysmal nocturnal hemoglobinuria; SLE, Systemic lupus erythematosus; SNP, Single-nucleotide polymorphism; TP, Terminal pathway.
References
1. Ricklin D, Hajishengallis G, Yang K, Lambris JD. Complement: a key system for immune surveillance and homeostasis. Nat Immunol. (2010) 11:785–97. doi: 10.1038/ni.1923
2. Schmidt CQ, Lambris JD, Ricklin D. Protection of host cells by complement regulators. Immunol Rev. (2016) 274:152–71. doi: 10.1111/imr.12475
3. Ricklin D, Reis ES, Lambris JD. Complement in disease: a defence system turning offensive. Nat Rev Nephrol. (2016) 12:383–401. doi: 10.1038/nrneph.2016.70
4. Gaya da Costa M, Poppelaars F, Berger SP, Daha MR, Seelen MA. The lectin pathway in renal disease: old concept and new insights. Nephrol Dial Transplant. (2018) doi: 10.1093/ndt/gfy073. [Epub ahead of print].
5. Poppelaars F, Seelen MA. Complement-mediated inflammation and injury in brain dead organ donors. Mol Immunol. (2017) 84:77–83. doi: 10.1016/j.molimm.2016.11.004
6. Poppelaars F, Faria B, Gaya da Costa M, Franssen CFM, van Son WJ, Berger SP, et al. The complement system in dialysis: a forgotten story? Front Immunol. (2018) 9:71. doi: 10.3389/fimmu.2018.00071
7. Jager NM, Poppelaars F, Daha MR, Seelen MA. Complement in renal transplantation: the road to translation. Mol Immunol. (2017) 89:22–35. doi: 10.1016/j.molimm.2017.05.014
8. Reis ES, Mastellos DC, Ricklin D, Mantovani A, Lambris JD. Complement in cancer: untangling an intricate relationship. Nat Rev Immunol. (2017) 18:5–18. doi: 10.1038/nri.2017.97
9. Hempel JC, Poppelaars F, Gaya Da Costa M, Franssen CF, De Vlaam TP, Daha MR, et al. Distinct in vitro complement activation by various intravenous iron preparations. Am J Nephrol. (2017) 45:49–59. doi: 10.1159/000451060
10. Szebeni J. Complement activation-related pseudoallergy: a stress reaction in blood triggered by nanomedicines and biologicals. Mol Immunol. (2014) 61:163–73. doi: 10.1016/j.molimm.2014.06.038
11. Brennan FH, Lee JD, Ruitenberg MJ, Woodruff TM. Therapeutic targeting of complement to modify disease course and improve outcomes in neurological conditions. Semin Immunol. (2016) 28:292–308. doi: 10.1016/j.smim.2016.03.015
12. Zhang M, Alicot EM, Chiu I, Li J, Verna N, Vorup-Jensen T, et al. Identification of the target self-antigens in reperfusion injury. J Exp Med. (2006) 203:141–152. doi: 10.1084/jem.20050390
13. Hajishengallis G, Reis ES, Mastellos DC, Ricklin D, Lambris JD. Novel mechanisms and functions of complement. Nat Immunol. (2017) 18:1288–98. doi: 10.1038/ni.3858
14. Mackay IR, Rosen FS, Walport MJ. Complement. N Engl J Med. (2001) 344:1058–66. doi: 10.1056/NEJM200104053441406
15. Giefing-Kröll C, Berger P, Lepperdinger G, Grubeck-Loebenstein B. How sex and age affect immune responses, susceptibility to infections, and response to vaccination. Aging Cell (2015) 14:309–21. doi: 10.1111/acel.12326
16. Roved J, Westerdahl H, Hasselquist D. Sex differences in immune responses: hormonal effects, antagonistic selection, and evolutionary consequences. Horm Behav. (2017) 88:95–105. doi: 10.1016/j.yhbeh.2016.11.017
17. Fairweather D, Frisancho-Kiss S, Rose NR. Sex differences in autoimmune disease from a pathological perspective. Am J Pathol. (2008) 173:600–9. doi: 10.2353/ajpath.2008.071008
18. Klein SL, Flanagan KL. Sex differences in immune responses. Nat Rev Immunol. (2016) 16:626–38. doi: 10.1038/nri.2016.90
19. Weng N-P. Aging of the immune system: how much can the adaptive immune system adapt? Immunity (2006) 24:495–9. doi: 10.1016/j.immuni.2006.05.001
20. Shaw AC, Goldstein DR, Montgomery RR. Age-dependent dysregulation of innate immunity. Nat Rev Immunol. (2013) 13:875–87. doi: 10.1038/nri3547
21. Dorshkind K, Montecino-Rodriguez E, Signer RAJ. The ageing immune system: is it ever too old to become young again? Nat Rev Immunol. (2009) 9:57–62. doi: 10.1038/nri2471
22. Shaw AC, Joshi S, Greenwood H, Panda A, Lord JM. Aging of the innate immune system. Curr Opin Immunol (2010) 22:507–513. doi: 10.1016/j.coi.2010.05.003
23. Gubbels Bupp MR, Potluri T, Fink AL, Klein SL. The confluence of sex hormones and aging on immunity. Front Immunol. (2018) 9:1269. doi: 10.3389/fimmu.2018.01269
24. Seelen MA, Roos A, Wieslander J, Mollnes TE, Sjöholm AG, Wurzner R, et al. Functional analysis of the classical, alternative, and MBL pathways of the complement system: standardization and validation of a simple ELISA. J Immunol Methods (2005) 296:187–98. doi: 10.1016/j.jim.2004.11.016
25. Roos A, Bouwman LH, Munoz J, Zuiverloon T, Faber-Krol MC, Fallaux-van den Houten FC, et al. Functional characterization of the lectin pathway of complement in human serum. Mol Immunol. (2003) 39:655–68. doi: 10.1016/S0161-5890(02)00254-7
26. Sjöholm AG. Complement components in normal serum and plasma quantitated by electroimmunoassay. Scand J Immunol. (1975) 4:25–30. doi: 10.1111/j.1365-3083.1975.tb02596.x
27. Prellner K, Sjöholm AG, Truedsson L. Concentrations of C1q, factor B, factor D and properdin in healthy children, and the age-related presence of circulating C1r-C1s complexes. Acta Paediatr Scand. (1987) 76:939–43. doi: 10.1111/j.1651-2227.1987.tb17268.x
28. Ekdahl K, Truedsson L, Sjöholm AG, Braconier JH. Complement analysis in adult patients with a history of bacteremic pneumococcal infections or recurrent pneumonia. Scand J Infect Dis. (1995) 27:111–7. doi: 10.3109/00365549509018989
29. Sturfelt G, Truedsson L. Complement factor D concentrations in normal sera: comparison of immunochemical and functional determinations. Acta Pathol Microbiol Immunol Scand C (1983) 91:383–9.
30. Fredrikson GN, Gullstrand B, Westberg J, Sjoholm AG, Uhlen M, Truedsson1 ' L. Expression of properdin in complete and incomplete deficiency: normal in vitro synthesis by monocytes in two cases with properdin deficiency type II due to distinct mutations. J Clin Immunol. (1998) 18:272–82.
31. Ambrus G, Gál P, Kojima M, Szilágyi K, Balczer J, Antal J, et al. Natural substrates and inhibitors of mannan-binding lectin-associated serine protease-1 and−2: a study on recombinant catalytic fragments. J Immunol. (2003) 170:1374–82. doi: 10.4049/jimmunol.170.3.1374
32. van de Geijn FE, Roos A, de Man YA, Laman JD, de Groot CJM, Daha MR, et al. Mannose-binding lectin levels during pregnancy: a longitudinal study. Hum. Reprod. (2007) 22:362–71. doi: 10.1093/humrep/del392
33. Zadura AF, Theander E, Blom AM, Trouw LA. Complement inhibitor C4b-binding protein in primary sjögren's syndrome and its association with other disease markers. Scand. J. Immunol. (2009) 69:374–380. doi: 10.1111/j.1365-3083.2009.02229.x
34. Bulla R, Bossi F, Agostinis C, Radillo O, Colombo F, De Seta F, et al. Complement production by trophoblast cells at the feto-maternal interface. J Reprod Immunol. (2009) 82:119–2. doi: 10.1016/j.jri.2009.06.124
35. Langeggen H, Pausa M, Johnson E, Casarsa C, Tedesco F. The endothelium is an extrahepatic site of synthesis of the seventh component of the complement system. Clin Exp Immunol. (2000) 121:69–76. doi: 10.1046/j.1365-2249.2000.01238.x
36. Würzner R, Orren A, Potter P, Morgan BP, Ponard D, Späth P, et al. Functionally active complement proteins C6 and C7 detected in C6- and C7-deficient individuals. Clin Exp Immunol. (1991) 83:430–7. doi: 10.1111/j.1365-2249.1991.tb05656.x
37. Mukaka MM. Statistics corner: a guide to appropriate use of correlation coefficient in medical research. Malawi Med. J. (2012) 24:69–71.
38. Colten HR, Strunk RC. Synthesis of complement components in liver and at extrahepatic sites. In: Complement in Health and Disease (1993) 127–158. Available online at: http://www.springerlink.com/index/10.1007/978-94-011-2214-6_4
39. Morgan BP, Gasque P. Extrahepatic Complement Biosynthesis: Where, When and Why? Available online at: https://www.ncbi.nlm.nih.gov/pmc/articles/PMC1904545/pdf/cei0107-0001.pdf
40. Lubbers R, van Essen MF, van Kooten C, Trouw LA. Production of complement components by cells of the immune system. Clin Exp Immunol. (2017) 188:183–94. doi: 10.1111/cei.12952
41. Nimgaonkar VL, Prasad KM, Chowdari KV, Severance EG, Yolken RH. The complement system: a gateway to gene-environment interactions in schizophrenia pathogenesis. Mol Psychiatry (2017) 22:1554–61. doi: 10.1038/mp.2017.151
43. Botto M, Kirschfink M, Macor P, Pickering MC, Würzner R, Tedesco F. Complement in human diseases: lessons from complement deficiencies. Mol Immunol. (2009) 46:2774–83. doi: 10.1016/j.molimm.2009.04.029
44. de Cordoba SR, Tortajada A, Harris CL, Morgan BP. Complement dysregulation and disease: from genes and proteins to diagnostics and drugs. Immunobiology (2012) 217:1034–46. doi: 10.1016/j.imbio.2012.07.021
45. Garred P, Larsen F, Seyfarth J, Fujita R, Madsen HO. Mannose-binding lectin and its genetic variants. Genes Immun. (2006) 7:85–94. doi: 10.1038/sj.gene.6364283
46. Heurich M, Martínez-Barricarte R, Francis NJ, Roberts DL, Rodríguez de Córdoba S, Morgan BP, et al. Common polymorphisms in C3, factor B, and factor H collaborate to determine systemic complement activity and disease risk. Proc Natl Acad Sci USA. (2011) 108:8761–6. doi: 10.1073/pnas.1019338108
47. Kotimaa J, Klar-Mohammad N, Gueler F, Schilders G, Jansen A, Rutjes H, et al. Sex matters: systemic complement activity of female C57BL/6J and BALB/cJ mice is limited by serum terminal pathway components. Mol Immunol. (2016) 76:13–21. doi: 10.1016/j.molimm.2016.06.004
48. Weintraub RM, Churchill WH, Crisler C, Rapp HJ, Borsos T. Mouse complement: influence of sex hormones on its activity. Science (1966) 152:783–5. doi: 10.1126/science.152.3723.783
49. Roach B, Kim Y, Jerome E, Michael AF. Influence of age and sex on serum complement components in children. Arch Pediatr Adolesc Med. (1981) 135:918. doi: 10.1001/archpedi.1981.02130340030011
50. Libert C, Dejager L, Pinheiro I. The X chromosome in immune functions: when a chromosome makes the difference. Nat Rev Immunol. (2010) 10:594–604. doi: 10.1038/nri2815
51. Coleman MP, Murray JC, Willard HF, Nolan KF, Reid KB, Blake DJ, et al. Genetic and physical mapping around the properdin P gene. Genomics (1991) 11:991–6. doi: 10.1016/0888-7543(91)90024-9
52. Jaillon S, Berthenet K, Garlanda C. Sexual dimorphism in innate immunity. Clin Rev Allergy Immunol. (2017) 1–14. doi: 10.1007/s12016-017-8648-x
53. Troldborg A, Hansen A, Hansen SWK, Jensenius JC, Stengaard-Pedersen K, Thiel S. Lectin complement pathway proteins in healthy individuals. Clin. Exp. Immunol. (2017) 188:138–47. doi: 10.1111/cei.12909
54. van Beek AE, Kamp A, Kruithof S, Nieuwenhuys EJ, Wouters D, Jongerius I, et al. Reference intervals of factor H and Factor H-related proteins in healthy children. Front Immunol. (2018) 9:1727. doi: 10.3389/fimmu.2018.01727
55. Poppelaars F, van Werkhoven MB, Kotimaa J, Veldhuis ZJ, Ausema A, Broeren SGM, et al. Critical role for complement receptor C5aR2 in the pathogenesis of renal ischemia-reperfusion injury. FASEB J. (2017) 31:3193–204. doi: 10.1096/fj.201601218R
56. Poppelaars F, Jager NM, Kotimaa J, Leuvenink HGD, Daha MR, van Kooten C, et al. C1-inhibitor treatment decreases renal injury in an established brain-dead rat model. Transplantation (2018) 102:79–87. doi: 10.1097/TP.0000000000001895
57. de Paula PF, Barbosa JE, Junior PR, Ferriani VPL, Latorre MR, Nudelman V, et al. Ontogeny of complement regulatory proteins - concentrations of factor h, factor I, c4b-binding protein, properdin and vitronectin in healthy children of different ages and in adults. Scand J Immunol. (2003) 58:572–7. doi: 10.1046/j.1365-3083.2003.01326.x
58. Keller TT, Van Leuven SI, Meuwese MC, Wareham NJ, Luben R, Stroes ES, et al. Serum levels of mannose-binding lectin and the risk of future coronary artery disease in apparently healthy men and women. Arterioscler Thromb Vasc Biol. (2006) 26:2345–50. doi: 10.1161/01.ATV.0000240517.69201.77
59. Silva AS, Teixeira AG, Bavia L, Lin F, Velletri R, Belfort R, et al. Plasma levels of complement proteins from the alternative pathway in patients with age-related macular degeneration are independent of Complement Factor H Tyr402His polymorphism. Mol Vis. (2012) 18:2288–99.
60. Simon AK, Hollander GA, McMichael A. Evolution of the immune system in humans from infancy to old age. Proc R Soc London B Biol Sci. (2015) 282:3085. doi: 10.1098/rspb.2014.3085
61. Panda A, Arjona A, Sapey E, Bai F, Fikrig E, Montgomery RR, et al. Human innate immunosenescence: causes and consequences for immunity in old age. Trends Immunol. (2009) 30:325–33. doi: 10.1016/j.it.2009.05.004
62. Yonemasu K, Kitajima H, Tanabe S, Ochi T, Shinkai H. Effect of age on C1q and C3 levels in human serum and their presence in colostrum. Immunology (1978) 35:523–30.
63. Sallenbach S, Thiel S, Aebi C, Otth M, Bigler S, Jensenius JC, et al. Serum concentrations of lectin-pathway components in healthy neonates, children and adults: mannan-binding lectin (MBL), M-, L-, and H-ficolin, and MBL-associated serine protease-2 (MASP-2). Pediatr Allergy Immunol. (2011) 22:424–30. doi: 10.1111/j.1399-3038.2010.01104.x
64. Tomaiuolo R, Ruocco A, Salapete C, Carru C, Baggio G, Franceschi C, et al. Activity of mannose-binding lectin in centenarians. Aging Cell (2012) 11:394–400. doi: 10.1111/j.1474-9726.2012.00793.x
65. Nagaki K, Hiramatsu S, Inai S, Sasaki A. The effect of aging on complement activity (CH50) and complement protein levels. J Clin Lab Immunol. (1980) 3:45–50.
66. Cole DS, Morgan BP. Beyond lysis: how complement influences cell fate. Clin Sci. (2003) 104:455–466. doi: 10.1042/CS20020362
67. Nielsen HE, Larsen SO, Vikingsdottir T. Rate-limiting components and reaction steps in complement-mediated haemolysis. APMIS (1992) 100:1053–60. doi: 10.1111/j.1699-0463.1992.tb04040.x
68. Poppelaars F, Damman J, de Vrij EL, Burgerhof JGM, Saye J, Daha MR, et al. New insight into the effects of heparinoids on complement inhibition by C1-inhibitor. Clin Exp Immunol. (2016) 184:378–88. doi: 10.1111/cei.12777
69. Nielsen EW, Waage C, Fure H, Brekke OL, Sfyroera G, Lambris JD, et al. Effect of supraphysiologic levels of C1-inhibitor on the classical, lectin and alternative pathways of complement. Mol Immunol. (2007) 44:1819–26. doi: 10.1016/j.molimm.2006.10.003
70. Volanakis JE, Barnum SR, Giddens M, Galla JH. Renal filtration and catabolism of complement protein D. N Engl J Med. (1985) 312:395–9. doi: 10.1056/NEJM198502143120702
71. Roos A, Dieltjes P, Vossen RH, Daha MR, de Knijff P. Detection of three single nucleotide polymorphisms in the gene encoding mannose-binding lectin in a single pyrosequencing reaction. J Immunol Methods (2006) 309:108–14. doi: 10.1016/j.jim.2005.11.017
72. Colten HR. Expression of the MHC class III genes. Philos Trans R Soc Lond B Biol Sci. (1984) 306:355–66. doi: 10.1098/rstb.1984.0096
73. Bergseth G, Ludviksen JK, Kirschfink M, Giclas PC, Nilsson B, Mollnes TE. An international serum standard for application in assays to detect human complement activation products. Mol Immunol. (2013) 56:232–9. doi: 10.1016/j.molimm.2013.05.221
Keywords: complement, health, sex and age, innate imunity, gender
Citation: Gaya da Costa M, Poppelaars F, van Kooten C, Mollnes TE, Tedesco F, Würzner R, Trouw LA, Truedsson L, Daha MR, Roos A and Seelen MA (2018) Age and Sex-Associated Changes of Complement Activity and Complement Levels in a Healthy Caucasian Population. Front. Immunol. 9:2664. doi: 10.3389/fimmu.2018.02664
Received: 30 June 2018; Accepted: 29 October 2018;
Published: 20 November 2018.
Edited by:
Uday Kishore, Brunel University London, United KingdomReviewed by:
Teizo Fujita, Fukushima Medical University, JapanRobert Braidwood Sim, University of Oxford, United Kingdom
Kenneth Reid, University of Oxford, United Kingdom
Copyright © 2018 Gaya da Costa, Poppelaars, van Kooten, Mollnes, Tedesco, Würzner, Trouw, Truedsson, Daha, Roos and Seelen. This is an open-access article distributed under the terms of the Creative Commons Attribution License (CC BY). The use, distribution or reproduction in other forums is permitted, provided the original author(s) and the copyright owner(s) are credited and that the original publication in this journal is cited, in accordance with accepted academic practice. No use, distribution or reproduction is permitted which does not comply with these terms.
*Correspondence: Marc A. Seelen, m.seelen@umcg.nl
†These authors have contributed equally to this work