- 1Biosciences and Biotechnology Postgraduate Program, Carlos Chagas Institute (ICC), Fiocruz, Curitiba, Brazil
- 2Laboratory of Immunoparasitology, Department of Pathological Sciences, State University of Londrina, Londrina, Brazil
- 3Laboratory of Biotransformation and Phytochemistry, Department of Chemistry, State University of Londrina, Universitary Hospital, Londrina, Brazil
- 4Laboratory of Molecular Virology, Carlos Chagas Institute (ICC), Fiocruz, Curitiba, Brazil
Leishmaniasis is a vector-borne neglected tropical disease that affects more than 700,000 people annually. Leishmania parasites cause the disease, and different species trigger a distinct immune response and clinical manifestations. Macrophages are the final host cells for the proliferation of Leishmania parasites, and these cells are the key to a controlled or exacerbated response that culminates in clinical manifestations. M1 and M2 are the two main macrophage phenotypes. M1 is a pro-inflammatory subtype with microbicidal properties, and M2, or alternatively activated, is an anti-inflammatory/regulatory subtype that is related to inflammation resolution and tissue repair. The present review elucidates the roles of M1 and M2 polarization in leishmaniasis and highlights the role of the salivary components of the vector and the action of the parasite in the macrophage plasticity.
Introduction
Leishmaniasis is a broad term that is used for a group of vector-borne diseases caused by species of protozoan parasites of the Leishmania genus of which 18 spp. are pathogenic to humans (1). The disease presents in five main clinical forms: visceral leishmaniasis (VL, or kala-azar), cutaneous leishmaniasis (CL), mucocutaneous leishmaniasis (MCL), diffuse cutaneous leishmaniasis (DCL) and post-kala-azar dermal leishmaniasis (PKDL) (2). All types of leishmaniases are transmitted to an animal or human reservoir through the bite of female infected phlebotomine sand flies, which infect a range of 70 animal species, including humans, rodents, and canids in their transmission cycle (3).
The World Health Organization (WHO) classifies leishmaniasis as a neglected tropical disease because it is directly linked to economically disadvantaged populations in tropical regions (2). A total of 700,000 to one million cases of leishmaniasis occur annually in 102 countries, areas or territories worldwide, with 20,000–30,000 deaths (3).
The high prevalence of this disease is directly influenced by the success of long host–parasite coevolutionary process in which parasites Leishmania have the ability to manipulate the vertebrate immune system in their favor, through the synthesis of parasites molecules, but also by vector saliva molecules, which are injected into the blood-feeding site during transmission (4).
The Leishmania parasites exhibit a biological digenetic life cycle with variable morphology that alternates between two main distinct developmental stages: the free-living flagellated promastigote form found in the midgut of phlebotomine sandfly vectors and the obligate intracellular aflagellated amastigotes in phagolysosomal vesicles of the vertebrate phagocytic cells, mainly into macrophages (5, 6).
During the blood feeding of the infected sandfly, which inoculates the host with metacyclic promastigotes and a large portion of the salivary content of the insect. Phlebotomine saliva is composed of pharmacologically active components with anti-hemostatic, chemotactic and immunomodulatory properties, that directly influence the parasite infection process modulating the local immune response (7). At the site of the bite occurs a rapid and intense neutrophil infiltration after inoculation, followed by monocytes/macrophages (8, 9).
Neutrophils primarily phagocytize most (80–90%) of the parasites and produce chemokines and cytokines that recruit and activate different cell types to regulate the development of the adaptive immune response during Leishmania sp. infection (8). Neutrophils are important components of the initial immune response against Leishmania parasites, even though there are currently contradictory findings on their role in the Leishmania infection.
Although the effective participation of neutrophils in the elimination of the parasite has been reported for L. braziliensis, L. amazonensis (10–15) and Leishmania donovani (16), collectively, most of these studies reported that the leishmanicidal action of neutrophils is clearly insufficient to control the establishment of infection and the development of the disease [reviewed in (17)].
Subversion of neutrophil killing functions by Leishmania is a strategy that allows parasite spreading in the host with a consequent infection evolution, transforming the primary protective role of neutrophils into a deleterious one. Neutrophils do not eliminate the parasite but act as “Trojan horses,” becoming late apoptotic and rapidly internalized by macrophages and dendritic cells, increasing the infectivity and persistence of the parasite (18, 19).
Macrophages play a dual role in Leishmania infection. These cells are responsible for the destruction of internalized parasites but also provide a safe place for Leishmania replication. Therefore, macrophages are key to disease progression and the success or failure of the infection depends on the interplay between infecting Leishmania species and the type and magnitude of the host's immune response. Both of these factors are closely related to the clinical forms of leishmaniasis (20, 21).
Macrophages are normally at rest as naïve macrophages (M0), but the microenvironment in which these cells are found provides different signals that activate them and lead to the development of functionally distinct macrophage's phenotype, toward “classically activated” (M1) or “alternatively activated” (M2) with different disease outcomes (22, 23). Therefore, the activation of M1 macrophages by Th1 lymphocyte subpopulation, which produces various cytokines, primarily interferon gamma (IFN-γ) and tumor necrosis factor-alpha (TNF-α) is crucial for the elimination of this intracellular pathogen via the triggering of an oxidative burst. The host cells increase the production of reactive oxygen species (ROS), including superoxide, hydrogen peroxide, and hydroxyl radicals, and nitric oxide (NO), which exhibit high microbicidal capacity (20, 22).
In contrast, the activation of Th2 lymphocytes, which produce IL-4 and IL-13 cytokines, induces the M2 profile caractherized by polyamine biosynthesis via activation of the enzyme arginase (arg) and production of urea and L-ornithine, which are beneficial for Leishmania intramacrophage growth favoring parasite survival in the infected macrophages and disease progression (22, 24).
Different Leishmania species trigger distinct immune responses (25). These responses are far beyond the classical Th1/Th2 paradigm (26) and increase the interest in understanding the role of M1 and M2 macrophages in the context of different Leishmania species infection. The immunomodulatory influence of the saliva of different leishmaniasis vectors should also be considered in the differential recruitment/activation of macrophages subtypes.
It is known how important macrophages are in the resistance or susceptibility to Leishmania infection, therefore we reviewed the impact of macrophage plasticity and M1 and M2 phenotypes on infection outcome. We also consider the role of vector saliva, which is a well-established immunomodulatory element in the Leishmania infection, in macrophage plasticity and phenotype.
M1 and M2 Macrophages: an Overview
Macrophages are phagocytic cells that are found in several tissues. In innate immunity, macrophages are responsible primarily for the control of pathogens and in adaptive immunity, this cell participates in the recognition, processing, and presentation of antigens to T cells (27). Macrophages interact with T and B cells via intercellular contact and the production of molecules and mediators to participate in the inflammatory response and tissue repair (27).
Two main macrophages phenotypes are known, M1 and M2 (28). The M1, or the classically activated macrophage, is a pro-inflammatory subtype that exhibits microbicidal properties (29). The M2, or the alternatively activated macrophage, is an anti-inflammatory/regulatory subtype that plays a role in the resolution of inflammation and tissue repair (30). The polarization of macrophages into M1 and M2 phenotypes is dependent on the signals provided by the microenvironment (28, 30, 31).
The designations M1 and M2 originated from Th1 and Th2 cytokine patterns and are associated with the change in macrophage phenotypes (32). Macrophage subtypes also differ in the production of cytokines, chemokines and other mediators and the expression of receptors, surface molecules, and transcription factors, which can act as specific markers to aid in the identification and function of these cells (27, 29, 32–34).
M1 macrophages are characterized by a high production of pro-inflammatory cytokines (TNF-α, IL-1β, IL-6, IL-12, IL-18, IL-23, and Type 1 IFN), high phagocytosis rate, and the production of reactive oxygen and nitrogen species and act to control intracellular pathogens. This macrophage subtype plays a role in tumor control, and it may be involved in autoimmune diseases and tissue damage (7, 27, 34–39).
M1 macrophages constitute the first line of defense against intracellular pathogens and induce the development of the Th1 response via IL-12 secretion (40, 41). The polarization of M1 macrophages may be primarily due to the presence of lipopolysaccharide (LPS), IFN-γ or TNF-α. Granulocyte-macrophage colony-stimulating factor (GM-CSF) may also result in the differentiation and maintenance of the M1 phenotype (41, 42). Ruan et al. (43) demonstrated that complement system activation is also related to M1 polarization.
M1 polarization activates transcription factors, such as AP1, STATs, NFκBp65 and IRFs, which lead to the expression of pro-inflammatory genes, costimulatory molecules and chemokines to attract various immune cells (Table 1) (7, 27, 34–39). IRF4 and IRF5 are involved in the polarization of M1 and M2 macrophages, but the role of IRFs in M2 polarization is not completely clear (44).
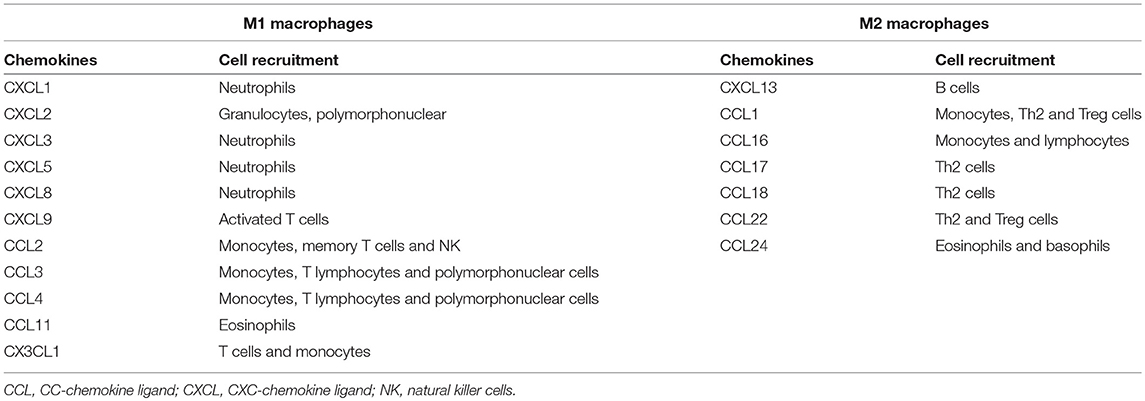
Table 1. Chemokines differentially produced by M1 and M2 macrophages and their role in cell recruitment.
The alternatively activated macrophages, or M2, exhibit an anti-inflammatory/immunoregulatory phenotype that is related to tissue remodeling and repair, resistance to some parasites and the promotion of tumor growth (30, 45, 46). M2 was initially characterized by the expression of mannose receptor (CD206), but a range of markers and mediators produced by these cells were described, including important chemokines for the recruitment of different cells (Table 1) (37, 45, 47).
Different stimuli, such as IL-4/IL-13, IL-10, TGF-β, M-CSF, vitamin D3, and immunocomplexes, induce M2 macrophages (48, 49). Other cytokines, such as IL-21 and IL-33, may also act on macrophage polarization and maintenance to an M2 phenotype (50–52). Li et al. (52) demonstrated that IL-21 reduced the expression of CD86, iNOS, TLR-4, and IL-6 and TNF-α production via STAT3 phosphorylation. IL-33 amplifies IL-13-induced M2 polarization (50, 51).
The classification of M2 macrophages was recently expanded and subdivides these cells into four subtypes, M2a, M2b, M2c, and M2d, according to stimulus and function (23, 45). M2a macrophages are polarized by macrophage colony-stimulating factor (M-CSF) and IL-4 or IL-13. This subtype is characterized by arg-1, IL-10, and SOCS3 expression and produces CCL24, CCL17, and CCL22, which are responsible for the recruitment of eosinophils, basophils and Th2 cells. These cells are involved in allergic reactions, parasite death and encapsulation, the promotion of fibrogenesis, tissue repair and cell proliferation (53–55).
The M2b phenotype is induced by the combination of immunocomplexes with IL-1βRa/TLR ligands, apoptotic cells or LPS. These cells secrete a large amount of IL-10 and inflammatory mediators, such as TNF-α and IL-6, and express iNOS (30, 53). M2b macrophages secrete the CCL1 chemokine, which results in the infiltration of eosinophils, Th2 lymphocytes and regulatory T cells (Tregs) (56). Therefore, M2b macrophages act as an immunoregulator and trigger activation of the Th2 response (23, 53).
M2c macrophage polarization results from IL-10, TGF-β and glucocorticoids (38, 57, 58). This subtype produces IL-10, TGF-β, CXCL13, CCL16, and CCL18, which leads to the down-regulation of pro-inflammatory cytokines and an increase in the recruitment of eosinophils and naïve T cells. M2c cells express high levels of arg-1, CD163, CD206, scavenger receptors, TLR1, TLR8, FPR1, CCR2, and CCR5, and this subtype is involved in tissue regeneration and angiogenesis (53–55, 59).
The M2d macrophage phenotype is involved in the inhibition of the immune response and the promotion of angiogenesis (60). These cells are induced by IL-6, toll-like receptor (TLR) ligands and adenosine A2A receptor agonists (60, 61). Stimulation with adenosine may result in the polarization of M1 macrophages to M2d (53, 61). This phenotype expresses high levels of IL-10, VEGF, and iNOS and secretes CCL5, CXCL10, and CXCL6 and low levels of IL-12 and TNF-α (53, 60–63).
Signals of the microenvironment are of great importance of the change in the polarization state of macrophages, and the programming from one phenotype to another is closely related to the activation of specific transcription factors and microRNAs (miRNAs) (64, 65). miRNAs are small molecules of non-coding RNAs which can act on gene expression at the post-transcriptional level (64), regulating some important transcription factors in the M1 and M2 phenotypes (66, 67).
Li et al. (67) reviewed the role of various miRNAs which participate in the regulation and polarization of macrophages in murine and human models. The miRNAs miRNA-9, miRNA-146a, miRNA-146b, miRNA-124, miRNA-181a, miRNA let-7c, miRNA-93, and miRNA-210 act to suppress the M1 and promote M2 phenotype. On the other hand, miRNA-27a, miRNA-130a, miRNA-130b, miRNA-155, miRNA-21, miRNA-19a-3p, miRNA-23a, miRNA-125a, miRNA-125b, miRNA-26a, miRNA-26b, and miRNA-720 act on transcription factors involved in the promotion of M1 phenotype and M2 suppression [reviewed in (67)].
Although we discussed above on the macrophage polarization of M0 to M1 or M2, it is known that macrophages have high plasticity and can be repolarized or reprogrammed under specific stimuli, in other words, M1 macrophages can differentiate into M2, and vice versa (40, 68). M2 macrophage may be repolarized more quickly than M1 macrophages, and this repolarization can occur through exposure of TLR ligands such as LPS and/or IFN-γ, or by expression of miRNAs such as miR-155 (69, 70). Van den Bossche et al. (71) showed that exposure of M1 macrophages to IL-4 is not able to reprogram the macrophages to M2 (71). However, the miRNAs can suppress the M1 phenotype and promote the polarization to M2 as commented above (67).
Interaction of Vector Saliva With Immune Cells
Among over 800 species of phlebotomines recorded, 98 are proven or suspected vectors of human leishmaniases; these include 42 Phlebotomus species in the Old World and 56 Lutzomyia species in the New World (all: Diptera: Psychodidae) (72). It is known that through the insect bite, vector saliva plays an important role in the establishment of Leishmania infection by increasing the infectivity of the parasite and modulating the host immune response (73, 74). Arthropod saliva contains anti-inflammatory, chemotactic and anti-hemostatic components that influence the course of parasite transmission to the host (7, 75, 76).
Most studies of the role of vector saliva in disease course were performed prior to the establishment of the M1 and M2 macrophage concept. Therefore, these works do not use this nomenclature. However, they investigated molecules that are involved in the plastic response of macrophages. These molecules are discussed below.
Some groups produced extracts, homogenates, sonicates and salivary lysates to elucidate the function of vector saliva in Leishmania transmission and infection (Table 2). We review the literature and assemble the results of different groups to provide an overview of the function of saliva.
Saliva contains molecules that induce a long-lasting erythema, which facilitates the obtaining of blood from capillaries in the host tissue (79). Vector saliva may facilitate cell recruitment via the promotion of vasodilation (79, 80, 92). The recruited cells include neutrophils, eosinophils and macrophages. Neutrophils may be attracted by the presence of several mediators, such as LTB4, and the role of these cells as a “Trojan horse” favors the establishment of infection (80, 92). The role of eosinophils is controversial, and whether these cells promote or suppress the infection is not well known (80, 95).
Some studies observed that vector saliva increased macrophages recruitment to the site of infection because of the modulation of chemotactic factors, such as CCL2/MCP-1, CCR2 and PGE2 (80, 92). Although some studies have shown the participation of lipid mediators such as PGE2 and LTB4 in cell migration (73, 92), the role of these lipid mediators acting in the polarization or recruitment of a specific profile of macrophages is uncertain, differing between cell types and models studied (28, 96–98).
Vector saliva plays an important immunomodulatory role and favors the M2 profile in different manners. Vector saliva induces IL-10 to promote a regulatory response (81, 89, 90), and it is related to the activation of a Th2 response via the increase in IL-4 and IL-6 synthesis (85, 87) Rohousov et al. (88). Besides that, the salivary components reducing the M1 related parameters, such as the pro-inflammatory cytokines IFN-γ and IL-12, iNOS and nitric oxide (NO) (85, 86, 88, 90).
However, some studies showed the action of saliva inducing M1 parameters. C57BL/6 mice immunized with plasmids encoding salivary proteins developed a Th1 response, resulting in protection against L. major infection, demonstrating that saliva may provide a protective effect and conferring characteristics for the development of a vaccine against Leishmania (90, 99). In a clinical study, was observed that individuals from an endemic area exposed to P. duboscqi bite presented high serum levels of INF-γ and decrease of IL-13, IL-5, directing a Th1 profile. Nonetheless, when PBMC of those individuals were exposed to P. duboscqi saliva, the most presented a mixed Th1/Th2 response, without a specific polarized profile (82).
In addition to complexes containing salivary components, the biological activity of pharmacological compounds isolated from vector saliva was examined. Adenosine and adenosine monophosphatase are active compounds found in P. papatasi saliva, and these factors inhibit the function of dendritic cells via a PGE2/IL-10-dependent mechanism to promote a tolerogenic profile that is characterized by the induction of regulatory T cells, which is also related to M2 polarization (73).
Maxadilan (Max) is a vasodilator peptide isolated from the saliva of arthropod vectors, and it reduces CD80 expression, which is responsible for T cell activation, and CCR7, which is involved in the development of adaptive immunity. Max increases CD86 expression on a subpopulation of dendritic cells, which leads to a preferential Th2 type response. Max also promotes an increased production of IL-6, IL-10, and TGF-β, and reduction of the Th1 cytokines, IL-1β, IL-12p70, TNF-α, and IFN-γ (87, 93). Max treatment of L. major-infected peritoneal exudate cells increased parasite load because of Th2 polarization and decreased NO production (94). These results suggest that Max acts on M2 polarization, as demonstrated previously for total saliva.
Parasites also play an important role in vector saliva modulation because these pathogens secrete promastigote secretory gel (PSG) in the insect gut. The vector regurgitates PSG with the other salivary components at the moment of blood-feeding (77). Leishmania mexicana-PSG regurgitated by L. longipalpis exacerbates skin infection via an increase in the recruitment of macrophages to the site of infection (77). PSG also increases parasite load in vitro and in vivo via increasing arginase activity (77).
In more detail, Giraud et al. (78) demonstrated that PSG exacerbates the inflammatory phase of the early wound response (high levels of cytokines IL-1β, IL-6, IL-10, TNFα and chemokines CCL2, CCL3, CCL4, CXCL2), to induce insulin-like growth factor 1 (IGF1)-signaling and later IGF1-dependent expression of arg-1 in macrophages. As a result, the M2 macrophages promote the effective infection of the parasites in a PGS-dependent manner (78).
In this way, it is noteworthy that some works show that high levels of pro-inflammatory cytokines are induced by saliva components, leading to a Th1 profile, that can act in a host protective way. However, the most of studies inferred that the different salivary components are allied to the immunomodulatory capacity of the parasite, and these components are essential tools in the success of the infection, via the down-regulation of a pro-inflammatory response and reduction of macrophages M1, which are fundamental to parasite elimination and disease resolution. The saliva also up-regulates Th2-standard cytokines and regulatory molecules, which act in M2 polarization, facilitate the promastigote infection and increase the survival and proliferation of intracellular amastigotes (Figure 1).
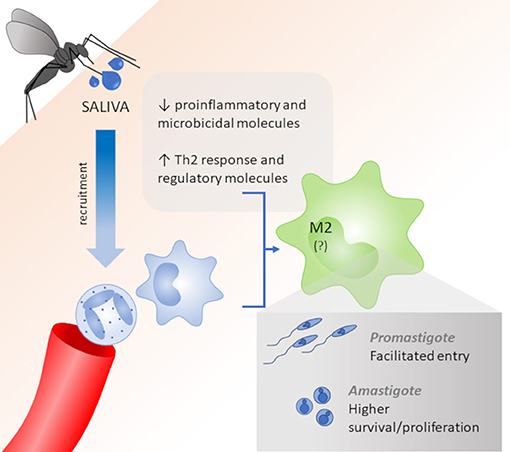
Figure 1. Role of saliva vectors on macrophage polarization. Vector saliva induces the recruitment of neutrophils and macrophages and acts as an immunomodulator to reduce pro-inflammatory and microbicidal molecules and improve Th2 cytokines and regulatory molecules, which lead to a M2 polarization. M2 macrophages allow for the facilitated entry of Leishmania promastigotes and higher survival/proliferation of intracellular amastigotes.
M1 and M2 Macrophages in Leishmania Infection
The role of macrophage subsets in Leishmania infection was not investigated thoroughly. However, the fundamental role of these cells in the development of the lesion support an improved understanding of the M1 and M2 profiles as an important tool in the pathogenesis of leishmaniasis. In vitro and in vivo studies of the host response to Leishmania (Table 3) and therapeutic strategies for modulating key molecules that control cellular activity were performed. We discuss the studies of species that cause the visceral and cutaneous forms of the disease.
M1 and M2 Macrophages in Cutaneous Leishmaniasis (CL)
CL is the most common form of leishmaniasis, with an estimated 600,000 to 1 million new cases worldwide annually. CL causes skin lesions that leave life-long scars and serious disability, and it has become a serious public health problem (3). The primary etiological agents include the Leishmania tropica and L. major species, in the Old World, and the L. mexicana species complex (e.g., L. amazonensis), and the subgenus Viannia, as the L. (V.) braziliensis species complex, in the New World (115).
Patients with CL have higher plasma levels of arg-1, TGF-β and PGE2 (116), as wells as, increased arginine in lesions (117), suggesting that M2 macrophages might play a role in the pathogenesis of the disease. In this sense, an in vitro study demonstrated that only M2 macrophages allow for L. major and L. amazonensis growth (114). These authors demonstrated that lipophosphoglycan (LPG) and glycoprotein GP63 of the parasites acted on M2 macrophages and suppressed non-coding RNAs, which left these cells permissive to infection (114). Lee et al. (118) also demonstrated that a non-healing strain of L. major efficiently interacted with M2 macrophages (CD206hi), which phagocytized the parasite in vitro and in vivo. However, a strain that produced self-healing lesions was less phagocytosed by M2 macrophages. The authors stated that the preferential infection of M2 cells played a crucial role in the severity of the cutaneous disease.
The role of peroxisome proliferator-activated receptors (PPARs) was investigated in infected macrophages from mouse strains resistant and susceptible to Leishmania infection. PPARs are ligand-activated transcription factors that are expressed in macrophages, and regulate the expression of certain genes related to the inflammatory response (119). Odegaard et al. (120) demonstrated that PPARγ-knockout mice have delayed in disease progress, with less footpad swelling and reduced parasitic burden. Importantly, genes preferentially expressed in alternatively activated macrophages, such as arg-1, Mrc1, and Clec7a, were also decreased in the tissue of PPARγ-knockout mice. These data strongly suggest that PPARγ is required for acquisition and maintenance of the M2 macrophages in L. major model (120). Besides that, Gallardo-Soler et al. (121) showed that PPARγ and -δ ligands promote intracellular L. major amastigote growth in infected macrophages, and this effect is dependent on both PPAR expression and arg-1 activity, namely suggesting that PPAR ligands promote amastigote growth in M2 macrophages in an arginase-dependent manner (121).
On the other hand, PPAR expression induced the activation of the murine macrophage cell line J774A.1 via polarization toward an M1 profile, with high production of pro-inflammatory mediators (TNF-α, IL-1β, IL-6, TLR4, and ROS), and an increase in microbicide activity against L. mexicana (113).
The transmembrane activator and calcium modulator and cyclophilin ligand interactor (TACI) is a key molecule for plasma cell maintenance. This receptor is in the TNF family, and it is required in infections where protection depends on antibody response. Analysis of macrophage phenotype revealed that macrophages adapted the M2 phenotype in the absence of TACI. The levels of M2 markers, IL-4Rα and CD206, were significantly higher in TACI-knockout macrophages than wild-type cells. TACI-knockout mice were unable to control L. major infection in vitro, which confirms their M2 phenotype, and intradermal inoculation of Leishmania resulted in a more severe manifestation of the disease than in the resistant C57BL/6 strain. The transfer of WT macrophages to TACI-knockout mice significantly reduced the disease severity (110).
One fundamental role of TNF was the induction of M1 differentiation and blockade of M2 polarization in the livers of L. major-infected mice (100). These authors also described the role of IL-6, which did not interfere with the macrophage phenotype alone, but it was highly expressed in M2 macrophages. The authors suggested that a balance between TNF and IL-6 mediated macrophage polarization in L. major infection (100).
Vellozo et al. (122) also demonstrated that the resistance of C57BL/6 mice to Leishmania infection was because of their ability to mature macrophages in the peritoneum from M0 to M1. Susceptible mice (BALB/c) exhibit immature peritoneal macrophages and succumb to infection. However, both mouse strains are resistant to L. braziliensis infection because in both strains convert M0 to M1 macrophages in this model, despite the incomplete M1 maturation and lower iNOS expression and NO production in BALB/c mice.
The co-culture of mesenchymal stem cells and L. major-infected macrophages also induced an event suggestive of M1 polarization, with the induction of inflammatory cytokines and reduction of IL-10 levels (108). This strategy provides new hope for stem cell therapy in the control of L. major (108).
SLPI (secretory leukocyte protease inhibitor) is a potent serine protease inhibitor that exhibits antimicrobial and anti-inflammatory functions. The role of SLPI in L. major infected-macrophage polarization was investigated (112). SPLI-knockout macrophages produced high levels of iNOS and IFN-γ but failed to contain cutaneous L. major infection. This study highlights that a very strong M1 response is detrimental in L. major models and causes tissue injury because of exacerbated inflammation. This study suggests that a balance of M1 and M2 macrophage responses influences the outcome of innate host defense against intracellular parasites, and SLPI is critical for the coordination of this balance and the resistance to chronic leishmaniasis (112).
One study of PKDL demonstrated that monocytes from patients exhibited decreased expression of TLR-2/4 and an attenuated generation of reactive oxidative/nitrosative species (109). Patients also exhibited an increased expression of classical M2 markers (arg-1, PPARγ and CD206) in monocytes and lesional macrophages, which indicated the M2 polarization of macrophages. These subsets appeared to sustain disease chronicity, which is a hallmark of Indian PKDL (109).
Two studies showed the development of new drugs addressed the M1/M2 plasticity (101, 104). The first study demonstrated that high antimony dilutions, a homeopathic medicine, potentiated the L. amazonensis-induced reduction of pro-inflammatory cytokine (IL-6, IL-12, and IFN-γ) and chemokine (CCL-2 and CCL-4) production in RAW cells. The treatment also induced an increase of the parasites internalization, but there was a reduction of the acid vacuoles, which implied in less elimination of the amastigote forms. The authors suggest that this phenomenon is related to the M2 polarization and regulation of the chronic inflammation events (104).
The second study used crotoxin, which is the main component of Crotalus durissus terrificus venom, to treat peritoneal BALB/c mouse macrophages infected with L. amazonensis (101). The host cells exhibited an increase in nitric oxide, IL-6 and TNF-α production, that converged into an M1 activation profile, as suggested by their morphological changes (larger spreading), inducing leishmanicidal activity against intracellular parasites, which may be associated with a better prognosis for CL (101).
Siewe et al. (107) proposed a mathematical model that states that Leishmania parasite takes advantage of the immune system and invades M1 and M2 macrophages. Simulations of the model demonstrated that the number of M2 macrophages constantly increased and M1 macrophages constantly decreased over the course of the infection, but the sum of the number of M1 and M2 cells reached a steady state, which was approximately the same as the healthy state of the host. The ratio of Leishmania parasites to macrophages depends homogeneously on their ratio at the time of the initial infection (107).
M1 and M2 Macrophages in Visceral Leishmaniasis (VL)
Visceral leishmaniasis is the most aggressive form of the disease, with high rates of mortality (3). Visceral leishmaniasis is generally caused by L. donovani and L. infantum chagasi species and affects internal organs, such as spleen, liver, and bone marrow (115, 123). It has been shown that blood arginase levels in VL patients are considerably increased (124), while NO levels are decreased (125). In this sense, a recent study demonstrated that monocytes/macrophages of VL patients present reduced oxidative burst and antigen presentation, with a M2 regulatory phenotype, characterized by high CD163, IL-10 and CXCL14 levels (126).
In the same direction, Silva et al. (102) demonstrated that the CD163s molecule was associated with the M2 macrophage phenotype, and it may be used as a biomarker of the clinical parameters of human VL severity. L. donovani also promotes the output of monocytes with a regulatory phenotype from the bone marrow that function as safe targets for the parasite (127). These data are consistent with analyses of dogs with VL, that demonstrated a higher number of M2-polarized macrophages compared to healthy dogs (106). The authors concluded that the predominance of the M2 phenotype (high CD163 labeling) in VL dogs favored the multiplication of L. infantum in the skin, spleen and lymph nodes (106).
Similarly, Chan et al. (128) suggest that the regulation of the macrophage phenotype is induced by the parasite, since PPAR expression is induced by parasitic infection. The authors showed that L. donovani activation of PPARγ promotes survival, whereas blockade of PPARγ facilitates removal of the parasite. Thus, Leishmania parasites harness PPARγ to sustain the M2 phenotype and increase infectivity of visceral disease (128).
A recent study has also shown the role of mammalian target of rapamycin (mTOR) in M2 macrophage polarization for Leishmania survival (129). The authors observed that L. donovani-infection activated host mTOR pathway, resulting in reduced expression of M1 macrophage markers (ROS, NO, iNOS, NOX-1, IL-12, IL-1β, and TNF-α), and increased expression of M2 macrophage markers (arg-1, IL-10, TGF-β, CD206, and CD163), favoring the Leishmania survival inside macrophages. Thus, they concluded that mTOR plays a crucial role in M2 macrophage polarization and parasite persistence in L. donovani infection (129).
These results correlated with studies performed in L. donovani-infected Syrian hamsters, in which the spleen environment was inflammatory with a high production of types I and II IFN. However, IFN-γ did not direct M1 macrophage polarization in this model, which allowed for parasite growth and the expression of counter-regulatory molecules, such as arg-1 (103).
A different group demonstrated that macrophages cultured from the lymph nodes of VL dogs exhibited low arg-1 activity and high NO and PGE2 production compared to uninfected dogs (105). These authors suggested that M1 macrophages were participating in the immune response in the lymph nodes of these animals (105). Attenuated L. donovani induced innate immunity via the classical activation of macrophages (M1), with upregulation of IL-1β, TNF-α, IL-12 and downregulation of IL-10, YM1, Arg-1, and MRC-1 genes, which led to the generation of protective Th1 responses in BALB/c mice (111).
This controversy may be explained by the fact that different patterns of gene expression are found in macrophages at different time points (130). A recent study demonstrated that the early response against L. donovani infection was distinguished by the increase in of Th1 markers and M1-macrophage activation molecules (IFN-γ, Stat1, Cxcl9, Cxcl10, Ccr5, Cxcr3, Xcl1, and Ccl3). However, this activation was not protective because the parasitic burden increased over time. There was no marked overlap of macrophage phenotypes at intermediate times of infection, and the overexpression of these Th1/M1 markers was restored later in the chronic phase without parasitic burden control (130).
Together, these results demonstrate that there are no “good guys” and “bad guys” in the polarization of macrophages following infection by Leishmania parasites. Therefore, a balance between the potent microbicidal response of M1 macrophages and the potential regulation by M2 macrophages may be key to the success of overcoming leishmaniasis (Figure 2).
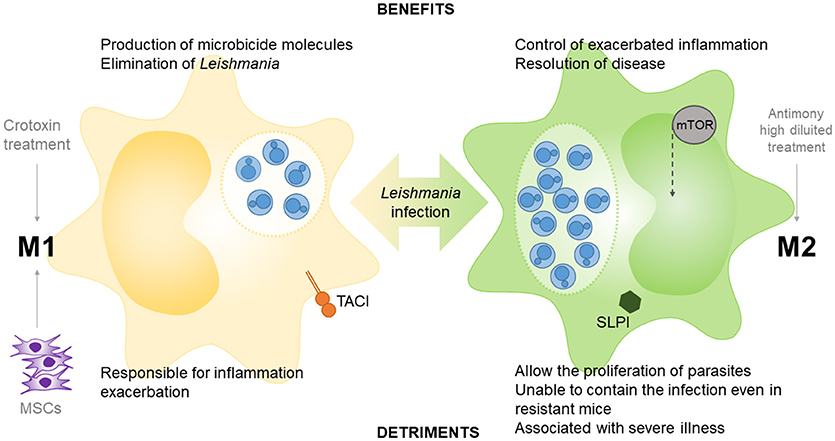
Figure 2. Role of M1 and M2 macrophages in Leishmania infection. TACI (transmembrane activator and calcium modulator and cyclophilin ligand interactor), peroxisome proliferator-activated receptors (PPARs) and mesenchymal stem cells (MSCs) participate in M1 polarization in macrophage-leishmania studies, as well as the crotoxin treatment. The epithelial and myeloid-derived serine protease inhibitor (SLPI), mammalian target of rapamycin (m-TOR) and the treatment with high diluted antimony participate in M2 polarization in leishmania models.
Conclusion
The collected works suggest that vector saliva plays an immunomodulatory effect on macrophages, which leads to the polarization of macrophages to an M2 phenotype. Therefore, vector saliva may contribute to an increase in the infectivity and persistence of the parasite during the initial periods of infection. Different Leishmania species exhibit virulence factors that can subvert the microbicidal mechanisms of the host to favor its proliferation because of the M2 polarization. However, M1 macrophages act during later periods of the infection and trigger an exacerbated immune response that leads to a worsening of the lesions, despite the role of these cells as powerful microbicidal agents. Therefore, a balance between the initial microbicidal response (M1) followed by a restorative response (M2) at later periods of time would provide the utmost benefit for the host. Further studies are necessary to fully elucidate the balance between these two main macrophages populations in the control of Leishmania infection.
Author Contributions
FT-P study design, literature review, data collection and manuscript writing. BB, JA, MG, AC, and MM-S literature review, data collection and manuscript writing. IC-C and JB text correction and organization. WP study design, text correction and organization.
Funding
This work was conducted during scholarships supported by CAPES (Coordenação de Aperfeiçoamento de Pessoal de Nível Superior).
Conflict of Interest Statement
The authors declare that the research was conducted in the absence of any commercial or financial relationships that could be construed as a potential conflict of interest.
Abbreviations
Arg, arginase; CL, cutaneous leishmaniasis; IL, interleukin; IFNγ, interferon gamma; IGF1, insulin-like growth factor 1; iNOS, inducible nitric oxide synthase; LPS, lipopolysaccharide; Max, maxadilan; MCL, mucocutaneous leishmaniasis; mTOR, mammalian target of rapamycin; NO, nitric oxide; PKDL, post-kala-azar dermal leishmaniasis; PPAR, proliferator-activated receptors; PSG, promastigote secretory gel; ROS, reactive oxygen species; SGE, salivary gland extract; SGH, salivary gland homogenate; SGL, salivary gland lysate; TACI, transmembrane activator and calcium modulator and cyclophilin ligand interactor; TGFβ, transforming growth factor beta; Th, T helper; TLR, toll like receptor; TNFα, tumor necrosis factor alpha; Treg, T regulatory; and VL, visceral leishmaniasis.
References
1. Steverding D. The history of leishmaniasis. Parasit Vectors (2017) 10:82. doi: 10.1186/s13071-017-2028-5
2. World Health Organization Leishmaniasis in high-burden countries: an epidemiological update based on data reported in 2014. Relev Epidemiol Hebd. (2016) 91:287–96.
3. World Health Organization (2018). Leishmaniasis. Available online at: http://www.who.int/news-room/fact-sheets/detail/leishmaniasis
4. Lestinova T, Rohousova I, Sima M, de Oliveira CI, Volf P. Insights into the sand fly saliva: blood-feeding and immune interactions between sand flies, hosts, and Leishmania. PLoS Negl Trop Dis. (2017) 11:e0005600. doi: 10.1371/journal.pntd.0005600
5. Liu D, Uzonna JE. The early interaction of Leishmania with macrophages and dendritic cells and its influence on the host immune response. Front Cell Infect Microbiol. (2012) 2:83. doi: 10.3389/fcimb.2012.00083
6. Serafim TD, Coutinho-Abreu IV, Oliveira F, Meneses C, Kamhawi S, Valenzuela JG. Sequential blood meals promote Leishmania replication and reverse metacyclogenesis augmenting vector infectivity. Nat Microbiol. (2018) 3:548–55. doi: 10.1038/s41564-018-0125-7
7. Scorza BM, Carvalho EM, Wilson ME. Cutaneous manifestations of human and murine Leishmaniasis. Int J Mol Sci. (2017) 18:1296. doi: 10.3390/ijms18061296
8. Peters NC, Egen JG, Secundino N, Debrabant A, Kimblin N, Kamhawi S, et al. In Vivo imaging reveals an essential role for neutrophils in leishmaniasis transmitted by sand flies. Science (80-).(2008) 321:970–4. doi: 10.1126/science.1159194
9. Hurrell BP, Schuster S, Grün E, Coutaz M, Williams RA, Held W, et al. Rapid sequestration of leishmania mexicana by neutrophils contributes to the development of chronic lesion. PLoS Pathog. (2015) 11:e1004929. doi: 10.1371/journal.ppat.1004929
10. Carlsen ED, Jie Z, Liang Y, Henard CA, Hay C, Sun J, et al. Interactions between neutrophils and leishmania braziliensis amastigotes facilitate cell activation and parasite clearance. J Innate Immun. (2015) 7:354–63. doi: 10.1159/000373923
11. Falcão SAC, Weinkopff T, Hurrell BP, Celes FS, Curvelo RP, Prates DB, et al. Exposure to leishmania braziliensis triggers neutrophil activation and apoptosis. PLoS Negl Trop Dis. (2015) 9:e0003601. doi: 10.1371/journal.pntd.0003601
12. Guimarães-Costa AB, Nascimento MTC, Froment GS, Soares RPP, Morgado FN, Conceição-Silva F, et al. Leishmania amazonensis promastigotes induce and are killed by neutrophil extracellular traps. Proc Natl Acad Sci USA. (2009) 106:6748–53. doi: 10.1073/pnas.0900226106
13. Novais FO, Santiago RC, Bafica A, Khouri R, Afonso L, Borges VM, et al. Neutrophils and macrophages cooperate in host resistance against leishmania braziliensis infection. J Immunol. (2009) 183:8088–98. doi: 10.4049/jimmunol.0803720
14. Rochael NC, Guimarães-Costa AB, Nascimento MTC, DeSouza-Vieira TS, Oliveira MP, Garcia e Souza LF, et al. Classical ROS-dependent and early/rapid ROS-independent release of neutrophil extracellular traps triggered by leishmania parasites. Sci Rep. (2016) 5:18302. doi: 10.1038/srep18302
15. Tavares NM, Araújo-Santos T, Afonso L, Nogueira PM, Lopes UG, Soares RP, et al. Understanding the mechanisms controlling Leishmania amazonensis infection in vitro: the role of LTB4 derived from human neutrophils. J Infect Dis. (2014) 210:656–66. doi: 10.1093/infdis/jiu158
16. McFarlane E, Perez C, Charmoy M, Allenbach C, Carter KC, Alexander J, et al. Neutrophils contribute to development of a protective immune response during onset of infection with Leishmania donovani. Infect Immun. (2008) 76:532–41. doi: 10.1128/IAI.01388-07
17. Regli I, Passelli K, Hurrell B, Tacchini-Cottier F. Survival mechanisms used by some leishmania species to escape neutrophil killing. Front Immunol. (2017) 8:1558. doi: 10.3389/fimmu.2017.01558
18. Ribeiro-Gomes FL, Peters NC, Debrabant A, Sacks DL. Efficient capture of infected neutrophils by dendritic cells in the skin inhibits the early anti-leishmania response. PLoS Pathog. (2012) 8:e1002536. doi: 10.1371/journal.ppat.1002536
19. van Zandbergen G, Klinger M, Mueller A, Dannenberg S, Gebert A, Solbach W, et al. Cutting edge: neutrophil granulocyte serves as a vector for Leishmania entry into macrophages. J Immunol. (2004) 173, 6521–5. doi: 10.4049/jimmunol.173.11.6521
20. Scott P, Novais FO. Cutaneous leishmaniasis: immune responses in protection and pathogenesis. Nat Rev Immunol. (2016) 16:581–92. doi: 10.1038/nri.2016.72
21. Srivastava S, Shankar P, Mishra J, Singh S. Possibilities and challenges for developing a successful vaccine for leishmaniasis. Parasit Vectors (2016) 9:277. doi: 10.1186/s13071-016-1553-y
22. Arango Duque G, Descoteaux A. Macrophage cytokines: involvement in immunity and infectious diseases. Front Immunol. (2014) 5:491. doi: 10.3389/fimmu.2014.00491
23. Zanluqui NG, Wowk PF, Pinge-Filho P. Macrophage polarization in chagas disease. J Clin Cell Immunol. (2015) 6:1–6. doi: 10.4172/2155-9899.1000317
24. Muxel SM, Aoki JI, Fernandes JCR, Laranjeira-Silva MF, Zampieri RA, Acuña SM, et al. Arginine and polyamines fate in leishmania infection. Front Microbiol. (2017) 8:2682. doi: 10.3389/fmicb.2017.02682
25. Mendonça SCF. Differences in immune responses against Leishmania induced by infection and by immunization with killed parasite antigen: implications for vaccine discovery. Parasit Vectors (2016) 9:492. doi: 10.1186/s13071-016-1777-x
26. Sacks D, Noben-Trauth N. The immunology of susceptibility and resistance to leishmania major in mice. Nat Rev Immunol. (2002) 2:845–58. doi: 10.1038/nri933
27. Murray PJ, Wynn TA. Protective and pathogenic functions of macrophage subsets. Nat Rev Immunol. (2011) 11:723–37. doi: 10.1038/nri3073
28. Parisi L, Gini E, Baci D, Tremolati M, Fanuli M, Bassani B, et al. Macrophage polarization in chronic inflammatory diseases: killers or builders? J Immunol Res. (2018) 2018:1–25. doi: 10.1155/2018/8917804
29. Covarrubias A, Byles V, Horng T. ROS sets the stage for macrophage differentiation. Cell Res. (2013) 23:984–5. doi: 10.1038/cr.2013.88
30. Anderson CF, Mosser DM. A novel phenotype for an activated macrophage: the type 2 activated macrophage. J Leukoc Biol. (2002) 72:101–6. doi: 10.1189/jlb.72.1.101
31. Bashir S, Sharma Y, Elahi A, Khan F. Macrophage polarization: the link between inflammation and related diseases. Inflamm Res. (2016) 65:1–11. doi: 10.1007/s00011-015-0874-1
32. Muraille E, Leo O, Moser M. TH1/TH2 paradigm extended: macrophage polarization as an unappreciated pathogen-driven escape mechanism? Front Immunol. (2014) 5:603. doi: 10.3389/fimmu.2014.00603
33. Murray PJ, Allen JE, Biswas SK, Fisher EA, Gilroy DW, Goerdt S, et al. Macrophage activation and polarization: nomenclature and experimental guidelines. Immunity (2014) 41:14–20. doi: 10.1016/j.immuni.2014.06.008
34. Sica A, Mantovani A. Macrophage plasticity and polarization: in vivo veritas. J Clin Invest. (2012) 122:787–95. doi: 10.1172/JCI59643
35. Jablonski KA, Amici SA, Webb LM, Ruiz-Rosado J, de D, Popovich PG, Partida-Sanchez S, et al. Novel markers to delineate murine M1 and M2 macrophages. PLoS ONE (2015) 10:e0145342. doi: 10.1371/journal.pone.0145342
36. Lehtonen A, Ahlfors H, Veckman V, Miettinen M, Lahesmaa R, Julkunen I. Gene expression profiling during differentiation of human monocytes to macrophages or dendritic cells. J Leukoc Biol. (2007) 82:710–20. doi: 10.1189/jlb.0307194
37. Mantovani A, Sica A, Sozzani S, Allavena P, Vecchi A, Locati M. The chemokine system in diverse forms of macrophage activation and polarization. Trends Immunol. (2004) 25:677–86. doi: 10.1016/j.it,.2004.09.015
38. Mosser DM, Edwards JP. Exploring the full spectrum of macrophage activation. Nat Rev Immunol. (2008) 8:958–69. doi: 10.1038/nri2448
39. Szanto A, Balint BL, Nagy ZS, Barta E, Dezso B, Pap A, et al. STAT6 transcription factor is a facilitator of the nuclear receptor PPARγ-regulated gene expression in macrophages and dendritic cells. Immunity (2010) 33:699–712. doi: 10.1016/j.immuni.2010.11.009
40. Wang N, Liang H, Zen K. Molecular mechanisms that influence the macrophage m1-m2 polarization balance. Front Immunol. (2014) 5:614. doi: 10.3389/fimmu.2014.00614
41. Xu W, Zhao X, Daha MR, van Kooten C. Reversible differentiation of pro- and anti-inflammatory macrophages. Mol Immunol. (2013) 53:179–86. doi: 10.1016/j.molimm.2012.07.005
42. Finnin M, Hamilton JA, Moss ST. Characterization of a CSF-induced proliferating subpopulation of human peripheral blood monocytes by surface marker expression and cytokine production. J Leukoc Biol. (1999) 66:953–60. doi: 10.1002/jlb.66.6.953
43. Ruan C-C, Ge Q, Li Y, Li X-D, Chen D-R, Ji K-D, et al. Complement-mediated macrophage polarization in perivascular adipose tissue contributes to vascular injury in deoxycorticosterone acetate–salt micesignificance. Arterioscler Thromb Vasc Biol. (2015) 35:598–606. doi: 10.1161/ATVBAHA.114.304927
44. Chistiakov DA, Myasoedova VA, Revin VV, Orekhov AN, Bobryshev YV. The impact of interferon-regulatory factors to macrophage differentiation and polarization into M1 and M2. Immunobiology (2018) 223:101–11. doi: 10.1016/j.imbio.2017.10.005
45. Gordon S. Alternative activation of macrophages. Nat Rev Immunol. (2003) 3:23–35. doi: 10.1038/nri978
46. Gordon S, Martinez FO. Alternative activation of macrophages: mechanism and functions. Immunity (2010) 32:593–604. doi: 10.1016/j.immuni.2010.05.007
47. Martinez FO, Helming L, Gordon S. Alternative activation of macrophages: an immunologic functional perspective. Annu Rev Immunol. (2009) 27:451–83. doi: 10.1146/annurev.immunol.021908.132532
48. Pesce J, Kaviratne M, Ramalingam TR, Thompson RW, Urban JF, Cheever AW, et al. The IL-21 receptor augments Th2 effector function and alternative macrophage activation. J Clin Invest. (2006) 116:2044–55. doi: 10.1172/JCI27727
49. Biswas SK, Mantovani A. Macrophage plasticity and interaction with lymphocyte subsets: cancer as a paradigm. Nat Immunol. (2010) 11:889–96. doi: 10.1038/ni.1937
50. Hazlett LD, McClellan SA, Barrett RP, Huang X, Zhang Y, Wu M, et al. IL-33 shifts macrophage polarization, promoting resistance against Pseudomonas aeruginosa Keratitis. Investig Opthalmol Vis Sci. (2010) 51:1524–32. doi: 10.1167/iovs.09-3983
51. Kurowska-Stolarska M, Stolarski B, Kewin P, Murphy G, Corrigan CJ, Ying S, et al. IL-33 amplifies the polarization of alternatively activated macrophages that contribute to airway inflammation. J Immunol. (2009) 183:6469–77. doi: 10.4049/jimmunol.0901575
52. Li S, Wang W, Fu S, Wang J, Liu H, Xie S, et al. IL-21 modulates release of proinflammatory cytokines in LPS-stimulated macrophages through distinct signaling pathways. Mediators Inflamm. (2013) 2013:548073. doi: 10.1155/2013/548073
53. Fraternale A, Brundu S, Magnani M. Polarization and Repolarization of Macrophages. J Clin Cell Immunol. (2015) 6:319. doi: 10.4172/2155-9899.1000319.
54. Lu J, Cao Q, Zheng D, Sun Y, Wang C, Yu X, et al. Discrete functions of M 2a and M 2c macrophage subsets determine their relative efficacy in treating chronic kidney disease. Kidney Int. (2013) 84:745–55. doi: 10.1038/ki.2013.135
55. Lucey DR, Clerici M, Shearer GM. Type 1 and type 2 cytokine dysregulation in human infectious, neoplastic, and inflammatory diseases. Clin Microbiol Rev. (1996) 9:532–62.
56. Asai A, Nakamura K, Kobayashi M, Herndon DN, Suzuki F. CCL1 released from M2b macrophages is essentially required for the maintenance of their properties. J Leukoc Biol. (2012) 92:859–67. doi: 10.1189/jlb.0212107
57. Ley K, Miller YI, Hedrick CC. Monocyte and macrophage dynamics during atherogenesis. Arterioscler Thromb Vasc Biol. (2011) 31:1506–16. doi: 10.1161/ATVBAHA.110.221127
58. Wolfs I, Donners M, de Winther M. Differentiation factors and cytokines in the atherosclerotic plaque micro-environment as a trigger for macrophage polarisation. Thromb Haemost. (2011) 106:763–71. doi: 10.1160/TH11-05-0320
59. Park-Min K-H, Antoniv TT, Ivashkiv LB. Regulation of macrophage phenotype by long-term exposure to IL-10. Immunobiology (2005) 210:77–86. doi: 10.1016/j.imbio.2005.05.002
60. Wang Q, Ni H, Lan L, Wei X, Xiang R, Wang Y. Fra-1 protooncogene regulates IL-6 expression in macrophages and promotes the generation of M2d macrophages. Cell Res. (2010) 20:701–12. doi: 10.1038/cr.2010.52
61. Ferrante CJ, Pinhal-Enfield G, Elson G, Cronstein BN, Hasko G, Outram S, et al. The adenosine-dependent angiogenic switch of macrophages to an M2-like phenotype is independent of interleukin-4 receptor alpha (IL-4Rα) signaling. Inflammation (2013) 36:921–31. doi: 10.1007/s10753-013-9621-3
62. Csóka B, Selmeczy Z, Koscsó B, Németh ZH, Pacher P, Murray PJ, et al. Adenosine promotes alternative macrophage activation via A 2A and A 2B receptors. FASEB J. (2012) 26:376–86. doi: 10.1096/fj.11-190934
63. Lawrence T, Natoli G. Transcriptional regulation of macrophage polarization: enabling diversity with identity. Nat Rev Immunol. (2011) 11:750–61. doi: 10.1038/nri3088
64. Self-Fordham JB, Naqvi AR, Uttamani JR, Kulkarni V, Nares S. MicroRNA: dynamic regulators of macrophage polarization and plasticity. Front Immunol. (2017) 8:1062. doi: 10.3389/fimmu.2017.01062
65. Terry RL, Miller SD. Molecular control of monocyte development. Cell Immunol. (2014) 291:16–21. doi: 10.1016/j.cellimm.2014.02.008
66. Graff JW, Dickson AM, Clay G, McCaffrey AP, Wilson ME. Identifying functional MicroRNAs in macrophages with polarized phenotypes. J Biol Chem. (2012) 287:21816–25. doi: 10.1074/jbc.M111.327031
67. Li H, Jiang T, Li M-Q, Zheng X-L, Zhao G-J. Transcriptional regulation of macrophages polarization by MicroRNAs. Front Immunol. (2018) 9:1175. doi: 10.3389/fimmu.2018.01175
68. Davis MJ, Tsang TM, Qiu Y, Dayrit JK, Freij JB, Huffnagle GB, et al. Macrophage M1/M2 polarization dynamically adapts to changes in cytokine microenvironments in Cryptococcus neoformans infection. MBio (2013) 4:e00264–e00213. doi: 10.1128/mBio.00264-13
69. Mylonas KJ, Nair MG, Prieto-Lafuente L, Paape D, Allen JE. Alternatively activated macrophages elicited by helminth infection can be reprogrammed to enable microbial killing. J Immunol. (2009) 182:3084–94. doi: 10.4049/jimmunol.0803463
70. Stout RD, Jiang C, Matta B, Tietzel I, Watkins SK, Suttles J. Macrophages sequentially change their functional phenotype in response to changes in microenvironmental influences. J Immunol. (2005) 175:342–9. doi: 10.4049/jimmunol.175.1.342
71. Van den Bossche J, Baardman J, Otto NA, van der Velden S, Neele AE, van den Berg SM, et al. Mitochondrial dysfunction prevents repolarization of inflammatory macrophages. Cell Rep. (2016) 17:684–96. doi: 10.1016/j.celrep.2016.09.008
72. Maroli M, Feliciangeli MD, Bichaud L, Charrel RN, Gradoni L. Phlebotomine sandflies and the spreading of leishmaniases and other diseases of public health concern. Med Vet Entomol. (2013) 27:123–47. doi: 10.1111/j.1365-2915.2012.01034.x
73. Carregaro V, Ribeiro JM, Valenzuela JG, Souza-júnior DL. Nucleosides present on phlebotomine saliva induce immunossuppression and promote the infection establishment. PLoS Negl Trop Dis. (2015) 9:e0003600. doi: 10.1371/journal.pntd.0003600
74. Rouhousová I, Volf P. Sand fly saliva: effects on host immune response and Leishmania transmission. Folia Parasitol (Praha). (2006) 53:161–71. doi: 10.14411/fp.2006.022
75. Kamhawi S. The biological and immunomodulatory properties of sand fly saliva and its role in the establishment of Leishmania infections. Microbes Infect. (2000) 2:1765–73. doi: 10.1016/S1286-4579(00)01331-9
76. Soares RPP, Turco SJ. Lutzomyia longipalpis (Diptera: psychodidae: phlebotominae): a review. An Acad Bras Cienc. (2003) 75:301–30. doi: 10.1590/S0001-37652003000300005
77. Rogers M, Kropf P, Choi B, Dillon R, Podinovskaia M, Bates P, et al. Proteophosophoglycans regurgitated by leishmania-infected sand flies target the L-arginine metabolism of host macrophages to promote parasite survival. PLoS Pathog. (2009) 5:e1000555. doi: 10.1371/journal.ppat.1000555
78. Giraud E, Lestinova T, Derrick T, Martin O, Dillon RJ, Volf P, et al. Leishmania proteophosphoglycans regurgitated from infected sand flies accelerate dermal wound repair and exacerbate leishmaniasis via insulin-like growth factor 1-dependent signalling. PLoS Pathog. (2018) 14:e1006794. doi: 10.1371/journal.ppat.1006794
79. Ribeiro JMC, Rossignol PA, Spielman A. Blood-finding strategy of a capillary-feeding sandfly, lutzomyia longipalpis. Comp Biochem Physiol. (1986) 83:683–6. doi: 10.1016/0300-9629(86)90709-7
80. Teixeira CR, Teixeira MJ, Regis BB, Santos CS, Andrade BB, Silva JS, et al. Saliva from lutzomyia longipalpis induces CC chemokine ligand 2/monocyte chemoattractant protein-1 expression and macrophage recruitment. J Immunol. (2005) 175:8346–53. doi: 10.4049/jimmunol.175.12.8346
81. Pushpanjali AKT, Purkait B, Jamal F, Singh MK, Ahmed G, Bimal S, et al. Direct evidence for role of anti-saliva antibodies against salivary gland homogenate of P. argentipes in modulation of protective Th1-immune response against Leishmania donovani. Cytokine (2016) 86:79–85. doi: 10.1016/j.cyto.2016.07.017
82. Oliveira F, Traoré B, Gomes R, Faye O, Gilmore DC, Keita S, et al. Delayed-type hypersensitivity to sand fly saliva in humans from a leishmaniasis-endemic area of mali is TH1-mediated and persists to midlife. J Invest Dermatol. (2013) 133:452–9. doi: 10.1038/jid.2012.315
83. Theodos CM, Ribeiro JM, Titus RG. Analysis of enhancing effect of sand fly saliva on Leishmania infection in mice. Infect Immun. (1991) 59:1592–8.
84. Titus RG, Ribeiro JM. Salivary gland lysates from the sand fly Lutzomyia longipalpis enhance Leishmania infectivity. Science (1988) 239:1306–8. doi: 10.1126/science.3344436
85. Mbow ML, Bleyenberg JA, Hall LR, Titus RG. Phlebotomus papatasi S and fly salivary gland lysate down-regulates a Th1, but up-regulates a Th2, response in mice infected with leishmania major. J Immunol. (1998) 161:5571–7.
86. Hall LR, Titus RG. Sand fly vector saliva selectively modulates macrophage functions that inhibit killing of Leishmania major and nitric oxide production. J Immunol. (1995) 155:3501–6.
87. Rogers KA, Titus RG. Immunomodulatory effects of Maxadilan and Phlebotomus papatasi sand fly salivary gland lysates on human primary in vitro immune responses. Parasite Immunol. (2003) 25:127–34. doi: 10.1046/j.1365-3024.2003.00623.x
88. Rohousová I, Volf P, Lipoldová M. Modulation of murine cellular immune response and cytokine production by salivary gland lysate of three sand fly species. Parasite Immunol. (2005) 704:469–73. doi: 10.1111/j.1365-3024.2005.00787.x
89. Norsworthy NB, Sun J, Elnaiem D, Lanzaro G, Soong L. Sand fly saliva enhances leishmania amazonensis infection by modulating interleukin-10 production. Infect Immun. (2004) 72:1240–7. doi: 10.1128/IAI.72.3.1240-1247.2004
90. Abdeladhim M, Ben Ahmed M, Marzouki S, Belhadj Hmida N, Boussoffara T, Belhaj Hamida N, et al. Human cellular immune response to the saliva of phlebotomus papatasi is mediated by IL-10-producing CD8+ T cells and Th1-polarized CD4+ lymphocytes. PLoS Negl Trop Dis. (2011) 5:e1345. doi: 10.1371/journal.pntd.0001345
91. Belkaid Y, Kamhawi S, Modi G, Valenzuela J, Noben-Trauth N, Rowton E, et al. Development of a natural model of cutaneous leishmaniasis: powerful effects of vector saliva and saliva preexposure on the long-term outcome of Leishmania major infection in the mouse ear dermis. J Exp Med. (1998) 188:1941–53. doi: 10.1084/jem.188.10.1941
92. Araújo-Santos T, Prates DB, Andrade BB, Nascimento DO, Clarêncio J, Entringer PF, et al. Lutzomyia longipalpis saliva triggers lipid body formation and prostaglandin E2 production in murine macrophages. PLoS ONE Neglected Trop Dis. (2010) 4:e873. doi: 10.1371/journal.pntd.0000873
93. Wheat WH, Pauken KE, Morris RV, Titus RG. Lutzomyia longipalpis salivary peptide maxadilan alters murine dendritic cell expression of CD80/86, CCR7 and cytokine secretion and reprograms dendritic cell-mediated cytokine release from cultures containing allogeneic T cells. J Immunol. (2008) 180:8286–98. doi: 10.4049/jimmunol.180.12.8286
94. Brodie TM, Smith MC, Morris RV, Titus RG. Immunomodulatory effects of the lutzomyia longipalpis salivary gland protein maxadilan on mouse macrophages. Infect Immun. (2007) 75:2359–65. doi: 10.1128/IAI.01812-06
95. Rodríguez NE, Wilson ME. Eosinophils and mast cells in leishmaniasis. Immunol Res. (2014) 59:129–41. doi: 10.1007/s12026-014-8536-x
96. Hosono K, Isonaka R, Kawakami T, Narumiya S, Majima M. Signaling of prostaglandin E receptors, EP3 and EP4 facilitates wound healing and lymphangiogenesis with enhanced recruitment of M2 macrophages in mice. PLoS ONE (2016) 11:e0162532. doi: 10.1371/journal.pone.0162532
97. Kim Y-G, Udayanga KGS, Totsuka N, Weinberg JB, Núñez G, Shibuya A. Gut dysbiosis promotes M2 macrophage polarization and allergic airway inflammation via fungi-induced PGE2. Cell Host Microbe (2014) 15:95–102. doi: 10.1016/j.chom.2013.12.010
98. Wang Z, Brandt S, Medeiros A, Wang S, Wu H, Dent A, et al. MicroRNA 21 is a homeostatic regulator of macrophage polarization and prevents prostaglandin E2-mediated M2 generation. PLoS ONE (2015) 10:e0115855. doi: 10.1371/journal.pone.0115855
99. Xu X, Oliveira F, Chang BW, Collin N, Gomes R, Teixeira C, et al. Structure and function of a "yellow" protein from saliva of the sand fly Lutzomyia longipalpis that confers protective immunity against Leishmania major infection. J Biol Chem. (2011) 286:32383–93. doi: 10.1074/jbc.M111.268904
100. Hu S, Marshall C, Darby J, Wei W, Lyons AB, Körner H. Absence of tumor necrosis factor supports alternative activation of macrophages in the liver after infection with leishmania major. Front Immunol. (2018) 9:1. doi: 10.3389/fimmu.2018.00001
101. Farias LHS, Rodrigues APD, Coêlho EC, Santos MF, Sampaio SC, Silva EO. Crotoxin stimulates an M1 activation profile in murine macrophages during Leishmania amazonensis infection. Parasitology (2017) 144:1458–67. doi: 10.1017/S0031182017000944
102. Silva RLL, Santos MB, Almeida PLS, Barros TS, Magalhães L, Cazzaniga RA, et al. sCD163 levels as a biomarker of disease severity in leprosy and visceral leishmaniasis. PLoS Negl Trop Dis. (2017) 11:e0005486. doi: 10.1371/journal.pntd.0005486
103. Kong F, Saldarriaga OA, Spratt H, Osorio EY, Travi BL, Luxon BA, et al. Transcriptional profiling in experimental visceral leishmaniasis reveals a broad splenic inflammatory environment that conditions macrophages toward a disease-promoting phenotype. PLoS Pathog. (2017) 13:e1006165. doi: 10.1371/journal.ppat.1006165
104. de Santana FR, Dalboni LC, Nascimento KF, Konno FT, Alvares-Saraiva AM, Correia MSF, et al. High dilutions of antimony modulate cytokines production and macrophage – Leishmania (L.) amazonensis interaction in vitro. Cytokine (2017) 92:33–47. doi: 10.1016/j.cyto.2017.01.004
105. Venturin GL, Chiku VM, Silva KLO, de Almeida BFM, de Lima VMF. M1 polarization and the effect of PGE 2 on TNF-α production by lymph node cells from dogs with visceral leishmaniasis. Parasit Immunol. (2016) 38:698–704. doi: 10.1111/pim.12353
106. Moreira PRR, Fernando FS, Montassier HJ, André MR, de Oliveira Vasconcelos R. Polarized M2 macrophages in dogs with visceral leishmaniasis. Vet Parasitol. (2016) 226:69–73. doi: 10.1016/j.vetpar.2016.06.032
107. Siewe N, Yakubu A-A, Satoskar AR, Friedman A. Immune response to infection by Leishmania : a mathematical model. Math Biosci. (2016) 276:28–43. doi: 10.1016/j.mbs.2016.02.015
108. Dameshghi S, Zavaran-Hosseini A, Soudi S, Shirazi FJ, Nojehdehi S, Hashemi SM. Mesenchymal stem cells alter macrophage immune responses to Leishmania major infection in both susceptible and resistance mice. Immunol Lett. (2016) 170:15–26. doi: 10.1016/j.imlet.2015.12.002
109. Mukhopadhyay D, Mukherjee S, Roy S, Dalton JE, Kundu S, Sarkar A, et al. M2 Polarization of monocytes-macrophages is a hallmark of indian post Kala-Azar dermal leishmaniasis. PLoS Negl Trop Dis. (2015) 9:e0004145. doi: 10.1371/journal.pntd.0004145
110. Allman WR, Dey R, Liu L, Siddiqui S, Coleman AS, Bhattacharya P, et al. TACI deficiency leads to alternatively activated macrophage phenotype and susceptibility to Leishmania infection. Proc Natl Acad Sci USA. (2015) 112:E4094–103. doi: 10.1073/pnas.1421580112
111. Bhattacharya P, Dey R, Dagur PK, Kruhlak M, Ismail N, Debrabant A, et al. Genetically modified live attenuated leishmania donovani parasites induce innate immunity through classical activation of macrophages that direct the Th1 response in mice. Infect Immun. (2015) 83:3800–15. doi: 10.1128/IAI.00184-15
112. McCartney-Francis N, Jin W, Belkaid Y, McGrady G, Wahl SM. Aberrant host defense against Leishmania major in the absence of SLPI. J Leukoc Biol. (2014) 96:917–29. doi: 10.1189/jlb.4A0612-295RR
113. Díaz-Gandarilla JA, Osorio-Trujillo C, Hernández-Ramírez VI, Talamás-Rohana P. PPAR activation induces M1 macrophage polarization via cPLA 2 -COX-2 inhibition, activating ROS production against Leishmania mexicana. Biomed Res Int. (2013) 2013:1–13. doi: 10.1155/2013/215283
114. Farrow AL, Rana T, Mittal MK, Misra S, Chaudhuri G. Leishmania-induced repression of selected non-coding RNA genes containing B-box element at their promoters in alternatively polarized M2 macrophages. Mol Cell Biochem. (2011) 350:47–57. doi: 10.1007/s11010-010-0681-5
116. França-Costa J, Weyenbergh J, Van Boaventura VS, Luz NF, Malta-Santos H, Cezar M, et al. Arginase, I., polyamine, and prostaglandin E2 pathways suppress the inflammatory response and contribute to diffuse cutaneous leishmaniasis. J Infect Dis. (2014) 211:426–35. doi: 10.1093/infdis/jiu455
117. Abebe T, Hailu A, Woldeyes M, Mekonen W, Bilcha K, Cloke T, et al. Local increase of arginase activity in lesions of patients with cutaneous leishmaniasis in ethiopia. PLoS Negl. Trop. Dis. (2012) 6:e1684. doi: 10.1371/journal.pntd.0001684
118. Lee SH, Charmoy M, Romano A, Paun A, Chaves MM, Cope FO, et al. Mannose receptor high, M2 dermal macrophages mediate nonhealing Leishmania major infection in a Th1 immune environment. J Exp Med. (2018) 215:357–75. doi: 10.1084/jem.20171389
119. Rigamonti E, Chinetti-Gbaguidi G, Staels B. Regulation of macrophage functions by PPAR-alpha, PPAR-gamma, and LXRs in mice and men. Arterioscler Thromb Vasc Biol. (2008) 28:1050–9. doi: 10.1161/ATVBAHA.107.158998
120. Odegaard JI, Ricardo-Gonzalez RR, Goforth MH, Morel CR, Subramanian V, Mukundan L, et al. Macrophage-specific PPARγ controls alternative activation and improves insulin resistance. Nature (2007) 447:1116–20. doi: 10.1038/nature05894
121. Gallardo-Soler A, Gómez-Nieto C, Campo ML, Marathe C, Tontonoz P, Castrillo A, et al. Arginase I induction by modified lipoproteins in macrophages: a peroxisome proliferator-activated receptor-gamma/delta-mediated effect that links lipid metabolism and immunity. Mol Endocrinol. (2008) 22:1394–402. doi: 10.1210/me.2007-0525
122. Vellozo NS, Pereira-Marques ST, Cabral-Piccin MP, Filardy AA, Ribeiro-Gomes FL, Rigoni TS, et al. All-trans retinoic acid promotes an M1- to M2-phenotype shift and inhibits macrophage-mediated immunity to leishmania major. Front Immunol. (2017) 8:1560. doi: 10.3389/fimmu.2017.01560
123. de Freitas EO, Leoratti FM, de S, Freire-de-Lima CG, Morrot A, Feijó DF. The contribution of immune evasive mechanisms to parasite persistence in visceral leishmaniasis. Front Immunol. (2016) 7:153. doi: 10.3389/fimmu.2016.00153
124. Abebe T, Takele Y, Weldegebreal T, Cloke T, Closs E, Corset C, et al. Arginase activity - a marker of disease status in patients with visceral leishmaniasis in ethiopia. PLoS Negl Trop Dis. (2013) 7:e2134. doi: 10.1371/journal.pntd.0002134
125. Sarkar A, Saha P, Mandal G, Mukhopadhyay D, Roy S, Singh SK, et al. Monitoring of intracellular nitric oxide in leishmaniasis: its applicability in patients with visceral leishmaniasis. Cytom Part A (2011) 79A:35–45. doi: 10.1002/cyto.a.21001
126. Roy S, Mukhopadhyay D, Mukherjee S, Moulik S, Chatterji S, Brahme N, et al. An IL-10 dominant polarization of monocytes is a feature of indian visceral leishmaniasis. Parasite Immunol. (2018) 40:e12535. doi: 10.1111/pim.12535
127. Hammami A, Abidin BM, Charpentier T, Fabié A, Duguay A-P, Heinonen KM, et al. HIF-1α is a key regulator in potentiating suppressor activity and limiting the microbicidal capacity of MDSC-like cells during visceral leishmaniasis. PLoS Pathog. (2017) 13:e1006616. doi: 10.1371/journal.ppat.1006616
128. Chan MM, Adapala N, Chen C. Peroxisome proliferator-activated receptor-γ-mediated polarization of macrophages in leishmania infection. PPAR Res. (2012) 2012:796235. doi: 10.1155/2012/796235
129. Kumar A, Das S, Mandal A, Verma S, Abhishek K, Kumar A, et al. Leishmania infection activates host mTOR for its survival by M2 macrophage polarization. Parasit Immunol. (2018) 40:e12586. doi: 10.1111/pim.12586
130. Ontoria E, Hernández-Santana YE, González-García AC, López MC, Valladares B, Carmelo E. Transcriptional profiling of immune-related genes in leishmania infantum-infected mice: identification of potential biomarkers of infection and progression of disease. Front Cell Infect Microbiol. (2018) 8:197. doi: 10.3389/fcimb.2018.00197
Keywords: classical macrophage, non-classical macrophage, vector saliva, Leishmania, immunomodulation, chemokine
Citation: Tomiotto-Pellissier F, Bortoleti BTdS, Assolini JP, Gonçalves MD, Carloto ACM, Miranda-Sapla MM, Conchon-Costa I, Bordignon J and Pavanelli WR (2018) Macrophage Polarization in Leishmaniasis: Broadening Horizons. Front. Immunol. 9:2529. doi: 10.3389/fimmu.2018.02529
Received: 08 August 2018; Accepted: 15 October 2018;
Published: 31 October 2018.
Edited by:
Wanderley De Souza, Universidade Federal do Rio de Janeiro, BrazilReviewed by:
Jaqueline França-Costa, Universidade Federal da Bahia, BrazilMirian Nacagami Sotto, Universidade de São Paulo, Brazil
Copyright © 2018 Tomiotto-Pellissier, Bortoleti, Assolini, Gonçalves, Carloto, Miranda-Sapla, Conchon-Costa, Bordignon and Pavanelli. This is an open-access article distributed under the terms of the Creative Commons Attribution License (CC BY). The use, distribution or reproduction in other forums is permitted, provided the original author(s) and the copyright owner(s) are credited and that the original publication in this journal is cited, in accordance with accepted academic practice. No use, distribution or reproduction is permitted which does not comply with these terms.
*Correspondence: Juliano Bordignon, bordignonjuliano@gmail.com
Wander Rogério Pavanelli, wanderpavanelli@yahoo.com.br