- INSERM UMR 1037 Centre de Recherche en Cancérologie de Toulouse (CRCT), ERL 5294 CNRS, Université Toulouse III Paul Sabatier, Laboratoire d'excellence Toucan, Toulouse, France
Interleukin-33 (IL-33), considered as an alarmin released upon tissue stress or damage, is a member of the IL-1 family and binds the ST2 receptor. First described as a potent initiator of type 2 immune responses through the activation of T helper 2 (TH2) cells and mast cells, IL-33 is now also known as an effective stimulator of TH1 immune cells, natural killer (NK) cells, iNKT cells, and CD8 T lymphocytes. Moreover, IL-33 was shown to play an important role in several cancers due to its pro and anti-tumorigenic functions. Currently, IL-33 is a possible inducer and prognostic marker of cancer development with a direct effect on tumor cells promoting tumorigenesis, proliferation, survival, and metastasis. IL-33 also promotes tumor growth and metastasis by remodeling the tumor microenvironment (TME) and inducing angiogenesis. IL-33 favors tumor progression through the immune system by inducing M2 macrophage polarization and tumor infiltration, and upon activation of immunosuppressive cells such as myeloid-derived suppressor cells (MDSC) or regulatory T cells. The anti-tumor functions of IL-33 also depend on infiltrated immune cells displaying TH1 responses. This review therefore summarizes the dual role of this cytokine in cancer and suggests that new proposals for IL-33-based cancer immunotherapies should be considered with caution.
Introduction
Cancer development depends on hallmarks such as self-sufficient proliferation, escape to anti-apoptotic signals, resistance to apoptosis, immune evasion, infinite replication, nurture of vascularization, and ability for invasion and metastasis (1). However, these hallmarks do not only concern the cancer cell but also the tumor microenvironment (TME) which is essential for tumorigenesis. The TME consists of fibroblasts, endothelial cells, immune cells, pericytes, and smooth muscle cells which are recruited by cancer cells as non-malignant cells but then modified to take part in tumor development (2–4). Besides cellular components, acellular components such as matrix, chemokines, and cytokines are also essential for tumor development (4). Cytokines as central mediators, favor the interaction between cells in the inflammatory tumor microenvironment (5). Amongst these cytokines, Interleukin-33 (IL-33), a member of the IL-1 superfamily of cytokines (6), is well-known now to have an important role in innate and adaptive immunity through its contribution to tissue homeostasis and responses to stress such as tumor development. IL-33 is constitutively expressed at high levels in the nucleus of human and mouse tissue lining and in various cell types including vascular endothelium (7), endothelial cells of endothelial venules (HEVs) (8, 9) and epithelial cells in barrier tissues that are exposed to the environment such as bronchial epithelial cells (10), keratinocytes, epithelial cells of the stomach, and salivary glands (7). Fibroblastic reticular cells (FRCs) in lymphoid tissues and cells of the central nervous system represent a major source of IL-33 (7, 11). IL-33 was first described in HEV as an intracellular nuclear factor with transcriptional regulatory properties (8). It was then shown that IL-33 binds a heterodimer formed by the specific ST2 receptor and a co-receptor, the IL-1 receptor accessory protein (6, 12). To exert its cytokine activity and alert the immune system, IL-33 is not secreted extracellularly like a conventional cytokine but after cell injury following cell stress or damage (11, 13–16). Full-length IL-33 is thus considered as an alarmin produced as a result of an injury to the central nervous system (15), a mechanical stress (17, 18), necroptosis (19) but also in pathological wound repair and fibrosis (20–22). The IL-33/ST2 axis is also associated with many inflammatory diseases such as asthma (23–25), rheumatoid arthritis, psoriatic arthritis or osteoarthritis (26), pulmonary fibrosis (27) or dermatitis and allergic contact dermatitis (28, 29). Many publications have summarized the important role of IL-33 in these diverse inflammatory diseases (30–34). IL-33/ST2 signaling is transduced by MyD88 and the kinase-4 associated to the ST2 receptor, which is a downstream adaptor protein, shared with other IL-1 family members and Toll-like receptors (35). Moreover, the soluble form of ST2 (sST2) produced from 3′-UTR promoter or splice variants mRNA, can be a decoy receptor for IL-33 (36–38). IL-33 was first described as a potent initiator of type 2 immune responses through the activation of many cell types, including the TH2 subset of helper cells, type 2 innate lymphoid cells (ILC2s), mast cells, basophils, eosinophils, and myeloid cells such as myeloid-derived antigen-presenting cells including macrophages and dendritic cells (DCs) (6, 35, 39–45). Furthermore, IL-33-exposed DCs or mast cells also selectively support FOXP3+ regulatory T cell (Treg cells) expansion through IL-33-induced secretion of IL-2 (40, 41) and favor TH17 cell differentiation through IL-1β and IL-6 secretion (46). IL-33 was detected in the serum of patients with TH1/TH17 mediated diseases (47, 48). However, besides this pro-inflammatory function of IL-33, its protective role in atherosclerosis, obesity, type2 diabetes and cardiac remodeling also holds an important place (16, 49–51). Moreover, IL-33 can also activate type 1 immune responses via TNF-α and IFN-γ expression by CD8 T lymphocytes, natural killer (NK) cells or iNKT cells. The latter can be stimulated by IL-33 upon its ligation to their cell surface ST2 receptors (13, 52–55). Finally, several studies have shown an important involvement of IL-33 in several types of cancer with pro or anti-tumorigenic functions depending on the immune status of the tumor. The goal of this review is to summarize the hallmarks of IL-33 in cancer, both in terms of its pro-tumorigenic function targeting resident TH2 immune cells of the TME, and as a tumor suppressor molecule activating the competent TH1 immune cells.
These properties therefore position IL-33 as a possible inducer and prognostic marker of cancer development, as reviewed here.
IL-33 as a Marker for Good or Poor Prognosis
IL-33 has been shown to be a promising biomarker in several types of cancer for tumor detection and as a predictor of prognosis and therapeutic response. Recently, IL-33 was shown to be correlated with a bad prognosis in several types of cancer, although in some cases IL-33 behaves as a tumor suppressor by inducing an immune response. In terms of bad prognosis, high levels of IL-33 were detected in the serum and tumors of patients with glioma (56), gastric cancer (57), hepatocellular carcinoma (58), uterine leiomyoma (59), lung cancer (60), colorectal cancer (61), head and neck squamous cell carcinoma (62), and breast cancer (63), when compared to corresponding healthy tissues. The Cancer Genome Atlas Pan-Cancer analysis project showed and declared that the level of IL-33 expression is altered in only 3% of ~580 tumors and that the most common genetic alteration is the deletion of the IL-33 gene (64).
Lu and collaborators detected “significantly higher IL-33 expression in glioma tissues than in normal brain tissues through immune-histochemical (IHC) analysis” (56). High IL-33 expression in glioma was correlated with shorter overall survival (OS) and progression-free survival (PFS) (56). In women, IL-33 highly promotes epithelial cell proliferation and tumorigenesis in breast cancer, since IL-33 increases Cancer Osaka Thyroid (COT) phosphorylation via ST2-COT interaction in normal epithelial and breast cancer cells. This induces the activation of MEK-ERK, JNK-cJun, and STAT3 signaling pathways, both leading to cell proliferation (65). The expression levels of IL-33 and ST2 proteins were also positively correlated with the expression of Ki-67 in epithelial ovarian cancer tumors and at the metastatic site, and negatively correlated with the patient survival time (66). High expression of IL-33, assessed by IHC staining, was associated with advanced stage clear-cell renal carcinoma and abnormally high amounts of serum IL-33 was detected in patients with hepatocellular carcinoma or gastric cancer. Hence, IL-33 is correlated with a bad prognosis in these types of cancer (57, 58, 67). sST2 was also described as a negative prognostic marker when its serum concentration was associated with OS of patients with hepatocellular carcinoma (68). Nevertheless, this soluble IL-33 receptor can also be associated with a good prognosis in colorectal cancer, as the trapping of soluble IL-33 in the TME inhibits cancer growth and metastases (69). Moreover, the level of IL-33 protein has been inversely correlated with tumor grade and size in patients with pulmonary adenocarcinoma, showing an association of low IL-33 expression level with a poor prognosis (70–72). Likewise, a genome-wide association study unveiled a correlation between high IL-33 expression and a good prognosis in patients with osteosarcoma (73). However, if IL-33 can have a pro-tumor effect by directly targeting cancer cells, the tumor suppressor functions displayed by IL-33 are indirectly promulgated by immune surveillance as we will show hereafter.
IL-33 as a Pro-tumorigenic Cytokine Through Actions on Cancer Cells and TME
As described above, IL-33 is considered as a prognostic biomarker when expressed in tumors. This intratumoral IL-33 is expressed by cancer cells as well as by other cell components of the TME. For instance, in patients with head and neck squamous cell carcinoma and oral squamous cell carcinoma, intratumoral IL-33 has been shown to be expressed in cancer-associated fibroblasts (CAF) (62, 74). This infiltrating IL-33 has a direct pro-tumorigenic effect on cancer cells and indirect effects on cellular components of the TME.
In the first case, Wang and collaborators showed that the IL-33/ST2 pathway up-regulated membrane glucose transporter 1 in non-small-cell lung cancer cells, enhancing their glucose uptake and glycolysis, thus favoring in vitro outgrowth of human lung cancer and its metastasis in a mouse model (60). By in vitro and in vivo experiments, IL-33 was also shown to be able to promote growth, invasion and migration of gastric cancer and colorectal cancer cells due to the autocrine secretion of several metalloproteases (MMP3, MMP9, MMP2), IL-6 and CXCR4 via the ST2-ERK1/2 pathway (61, 75). Moreover, IL-33 directly targets colon cancer cells and breast cancer cells via JNK-cJun activation, which promotes cell proliferation and therefore tumor growth (65, 76).
The impact of IL-33 on the TME encompasses angiogenesis, matrix remodeling and cytokine/growth factor production by non-epithelial cell components. The IL-33/ST2 signaling pathway, favoring pro-angiogenic VEGF expression in tumor cells and reducing tumor necrosis, is highly involved in mammary tumor growth (77). Concerning matrix modeling, human subepithelial myofibroblasts stimulated in vitro with IL-33 induced the expression of extracellular matrix components and growth factors associated with intestinal tumor progression (78). IL-33-stimulated cancer cells produce cytokines, and TME infiltrating immune cells are also involved in the expression of IL-6 in response to IL-33/ST2 signaling. Likewise, IL-33 stimulates the secretion of cytokines and growth factors in bone marrow myeloid and non-hematopoietic cells, resulting in myeloproliferation of neoplasms (79, 80). Indeed, suppression of IL-33 or high expression of sST2 suppresses IL-33-induced angiogenesis, TH2 responses, macrophage infiltration and M2 macrophage polarization. This negatively regulates tumor growth and metastatic spread of colorectal cancer, for instance through the modification of the TME (69). IL-33 in the TME recruits macrophages and stimulates their production of PGE2, and in turn, macrophage-derived PGE2 stimulates colon tumor development (76). The recruitment of macrophages in the TME might account for the stimulation of CCL2 expression by IL-33-stimulated cancer cells that express ST2, such as human colon cancer cells (81). After recruitment, macrophages are directly induced by IL-33 to be polarized in M2 tumor associated macrophages (TAM) in the TME. Such TAMs are then able to produce IL-10, VEGF, IL-6, and MMP9 which promote proliferation and invasiveness of cancer cells (82–84). Yang and collaborators showed that TAM are recruited by IL-33 in the TME, and IL-33-stimulated TAM can increase intravasation of tumor cells into the circulation at the early stages of metastasis (85). Even in the brain, IL-33 in the TME induces growth of glioma cells and facilitates microglia/macrophage infiltration (86). IL-33-stimulated macrophages are also activated to produce G-CSF, which in turn, boost myeloid-derived suppressor cells (MDSC) from the pro-tumoral TME (87). Indeed, MDSC contribute to tumor-mediated immune escape by suppressing antitumor immune responses. IL-33 released in tumor tissues in breast and colorectal cancer mouse models and in breast cancer patients, has been shown to facilitate MDSC expansion, recruitment and survival in the TME. This role could be due to the induction of an autocrine secretion of GM-CSF (88–90). Interestingly, another study showed that IL-33 does not affect the number of MDSC but can significantly reduce the differentiation of lineage-negative bone marrow progenitor cells into granulocytic MDSC in tumor-bearing mice. Moreover in the same study, IL-33-treated MDSC were shown to be less immunosuppressive, with a reduced capacity to inhibit T cell proliferation and IFN-γ production, production of reactive oxygen species and their capacity to induce Treg differentiation and expansion (91). IL-33 has a direct effect on Treg cells expressing surface ST2. Indeed, these lymphocytes are constitutively abundant in the intestine and able to prevent dysregulated inflammatory responses to self and environmental stimuli. IL-33 is constitutively expressed in epithelial cells at barrier sites. High levels of IL-33 were also observed in inflamed lesions of patients with inflammatory bowel disease, supporting its role in disease pathogenesis (92, 93). In inflammatory conditions, IL-33 signaling in Treg cells enhances transforming growth factor (TGF)-β1-mediated differentiation. Alternatively, IL-33 may provide a signal necessary for inducing their accumulation and maintenance in inflamed tissues (94). Local accumulation of Treg cells has been described in intestinal tumors preventing tumor clearance in mouse models and in patients. This role may be associated with a reduction of E-cadherin expression, increased β-catenin signaling and IL-33 production by malignant and injured epithelial cells (95). In tumors with low levels of infiltrating Treg cells, administration of IL-33 accelerates tumor growth and occurrence of liver and lung metastasis in breast cancer mouse models, and these models display an intratumoral accumulation of MDSC and Treg cells, as compared to untreated mice (90). Moreover, IL-33 blockade, in addition to abrogating the polarization of TAM, reduces the accumulation of Treg cells in lung tumors of human lung preclinical mouse models (82). However, as inflammation contributes to tumorigenesis, the accumulation of Treg in inflammatory zones must contain inflammation and therefore tumorigenesis. Treg may promote or inhibit tumor development depending on the context, revealing the complex relationship between inflammation, and cancer development. Furthermore, mast cells which also express ST2 receptors and respond to cell injury via IL-33 released from necrotic cells, can secrete leukotrienes and cytokines to initiate pro-inflammatory responses (96). In a colorectal cancer mouse model, IL-33 deficiency reduced mast cell accumulation in tumors. This deficiency further inhibited the expression of mast cell-derived proteases and cytokines that promote polyposis (78, 96–98). Generally, mast cells accumulate in inflamed gut and in colorectal tumors, and their presence is correlated with a poor prognosis and low overall survival (99, 100). In skin cancers, dermal mast cells are able to respond to UVB-induced IL-33 by releasing IL-10 to protect skin homeostasis after excessive UVB exposure. However, IL-10 may contribute to skin cancer development, as IL-10-deficient mice do not develop skin tumors upon UVB exposure (101, 102).
Direct or Indirect Effects of IL-33 as a Tumor Suppressor
Alongside its pro-tumorigenic role, IL-33 can also behave as a tumor suppressor. Only one study has shown a direct anti-tumor effect of this cytokine with the in vitro inhibition of proliferation and induction of apoptosis of MIA PaCa-2, a pancreatic cancer cell line (103). However, its anti-tumor functions were largely associated with the activation of immune effector cells able to lead to tumor clearance. All immune cells express the ST2 receptor and are able to respond to IL-33 stimulation. IL-33 has a significant role in cancer immune-surveillance in primary prostate and lung tumors, which can be lost during the metastatic transition inducing immune escape. The correlation between IL-33 and HLA expression in human tumors using RNA-sequencing data of resected prostate tumors was recently shown. The down-regulation of IL-33 during the metastatic process ultimately decreases the functionality of HLA-I and reduces immune-surveillance favoring tumor development (104). In a multivariable analysis, the infiltration of human hepatocellular carcinomas (HCC) by cells expressing IL-33 and by CD8+ T cells was associated with prolonged patient survival. These results led to propose an HCC immune score identifying high- vs. low-risk patients with different gene expression profiles (105). Injection of IL-33 into established murine melanoma or acute myeloid leukemia models inhibits tumor growth in a CD8+ T cell-dependent manner prolonging the survival of mice. In the first model, the reduction of tumor growth delay was correlated with intratumoral accumulation of CD8+ T cells, and a decrease in the number of immunosuppressive myeloid cells (106). In the second model, the anti-leukemia activity was associated with increased expansion and IFN-γ production of leukemia-reactive CD8+ T cells (107). Moreover, the correlation between decreased IFN-γ secretion and colon cancer aggressiveness, suggests that IL-33 signaling defects may impair the generation of IFN-γ-mediated immunity (108). In soft tissue sarcoma, higher transcriptional levels of IL-33 were also associated with a good prognosis. The expression of IL-33 has also been negatively correlated with the expression of chemokines, such as TGF-β, recruiting Treg and MDSC, and positively correlated with the expression of chemokines that recruit CD8+ T cells which promote anti-tumor immune responses especially through INF-γ production (109).
It has been shown that IFN-γ-producing cells present in tumors associated with an IL-33 antitumor effect, were CD8+ T cells and NK cells. Indeed, IL-33 expression in several cancers affects the number of CD8+ T cells and NK cells in tumor tissues and the production of IFN-γ/TNF-α, thereby favoring tumor eradication through tumor cell cytolysis (110, 111). This was also shown with the reduction of tumor metastasis in B16 melanoma and Lewis lung carcinoma metastatic models thanks to the transgenic expression of IL-33. In these transgenic mice models, tumor infiltration and CD8+ T lymphocyte and NK cell cytotoxicity was significantly increased compared to non-transgenic mice. Moreover, treatment with recombinant IL-33 increased CD8+ T lymphocyte and NK cell cytotoxicity in vitro (112). CD8+ T cells are also indirectly stimulated by IL-33 through DC. DC maturation is promoted by IL-33 which increases their cross presentation ability particularly during the anti-leukemia or anti-melanoma immune response (107, 113). As mentioned in the introduction, IL-33-activated DC are also able to promote the differentiation of TH17 cells which play an important role in cancer development. TH17 are T helper lymphocytes secreting IL-17 and other inflammatory cytokines, but can also display immunosuppressive activities, therefore mediating context dependent pro- or anti-tumor responses (114, 115). However, there are no published studies mentioning a direct relationship between TH17 cells and IL-33 in cancers. TH17 cells expressing the ST2 receptor were found to accumulate in the small intestine in bowel diseases where intestinal epithelial cells are the providing source of IL-33, we can therefore stipulate that these cells could play a role in digestive cancers. TH17 cells could have an anti-tumor function with the production of pro-inflammatory cytokines (116) or a pro-tumor role when they can acquire a regulatory phenotype with immunosuppressive properties upon IL-33 activation (117). Furthermore, DC can also drive TH9 cell dependent anti-tumor responses through the expression of Ox40L when activated by IL-33 and stimulated by dectin-1 signaling (118–120).
Finally, the ILC2s which support type 2 immune responses by producing IL-5 and IL-13 in response to IL-33 could also have an antitumor function. Indeed, their tissue-repair function can induce cholangiocarcinoma and liver metastasis (121). ILC2s can also be mobilized from the lung and other tissues thanks to IL-33, to penetrate tumors, mediate immune-surveillance with DC, and promote adaptive cytolytic T cell responses and attraction (122, 123).
Concluding Remarks
IL-33 therefore appears as a pro-tumorigenic cytokine that can also limit tumor growth through the activation of antitumor immunity. These opposing roles in tumorigenesis, as shown in this review, greatly depend on the IL-33/ST2 signaling in different immune cells. IL-33 is able to promote inflammatory events which contribute to tumorigenesis whilst activating anti-tumor immune responses. The different events promoted by IL-33 activation of various immune cells which can be found in the TME are summarized in the Figure 1. Depending on the tumor context, IL-33 produced in the TME can activate diverse immune cells which are able to promote a pro-tumor effect such as TAM, MDSC, fibroblasts, mast cells, Treg and DC, or to prevent tumor development such as NK cells, CD8+ T cells, iNKT, ILC2, TH9, and TH17. All these cell types produce specific cytokines, chemokines, and other molecules. These conclusions are supported by Wasmer and Krebs' review who demonstrated the multiple functions of IL-33 in different cancer types (124). As many cytokines with immunomodulatory properties, IL-33 has been considered for anticancer immunotherapies. However, knowing its dual role, therapeutic manipulation of this cytokine should be considered with caution. The majority of the studies mentioned propose cancer immunotherapy strategies based on exogenous IL-33 administration. These IL-33 adjuvanted vaccines aim at activating the immune cells involved in the immune response (106, 107, 125–128). IL-33 could also indirectly activate effector T cells. For instance, a replicating viral vector system used in cancer immunotherapy which delivers tumor-associated antigens to DC for efficient cytotoxic T cells priming, depends on IL-33 signaling (129). IL-33 could likewise increase T cell activation to promote graft- vs.-leukemia (GVL) reactions while decreasing fatal graft-vs.-host- disease (GVHD) (130). Possibly however, ST2 blockade might preserve GVL activity by blocking Treg controlling GVHD (131). Indeed, considering the immunosuppressive pro-tumorigenic role of IL-33, others have proposed to block IL-33 as a novel anticancer strategy (62, 65, 69, 76, 82, 88). In the future, IL-33 targeting in cancer immunotherapies should be considered with caution, especially taking into account the intricate dual role of this cytokine in cancer as shown in this review.
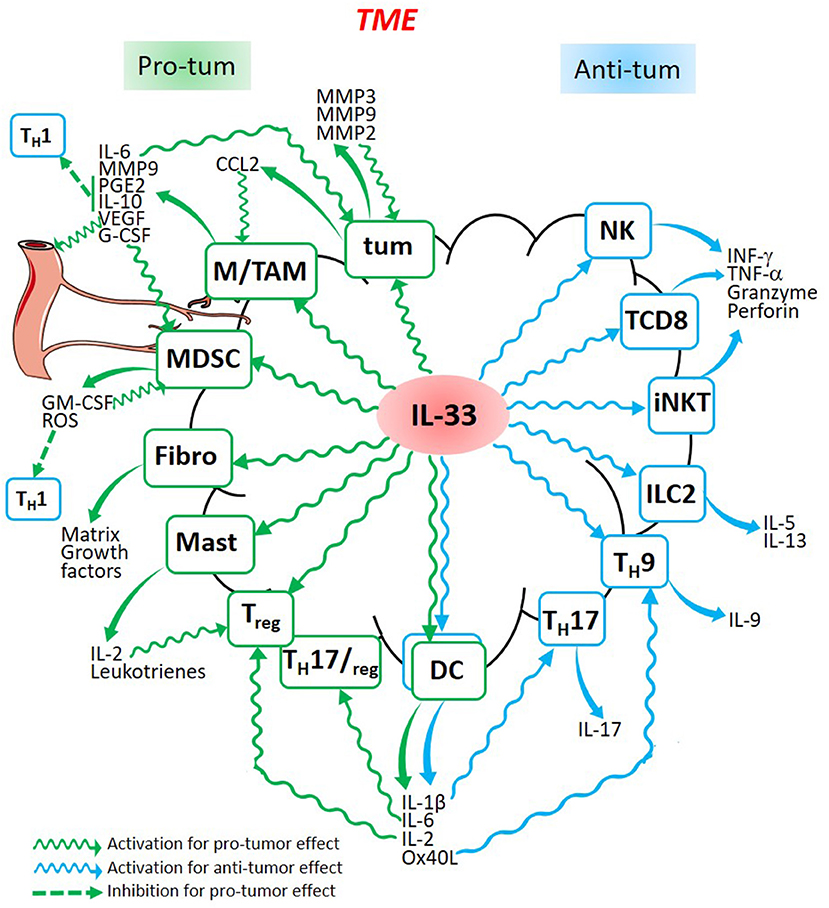
Figure 1. Dual role of Il-33 in cancer. IL-33 released in the TME is able to stimulate cancer cells (tum), fibroblasts (Fibro), and different immune cells (Macrophages, TAM, MDSC, mast cells, Treg, Dendritic Cells, TH17, TH9, ILC2, iNKT, CD8+ T cells, and Natural Killer cells) which are activated to produce molecules involved in pro-tumor (green) or anti-tumor (blue) processes leading to the development or to the regression of the tumor. Some cytokines produced by pro-tumor cells such as MDSC or TAM, are also able to produce cytokines which inhibit anti-tumor cells such as all the TH1 cells.
Author Contributions
All authors listed have made a substantial, direct and intellectual contribution to the work, and approved it for publication.
Conflict of Interest Statement
The authors declare that the research was conducted in the absence of any commercial or financial relationships that could be construed as a potential conflict of interest.
Acknowledgments
This work was supported by institutional grants from the Institut National de la Santé et de la Recherche Médicale (INSERM), the Université Toulouse 3, the Centre National de la Recherche Scientifique (CNRS) and the Laboratoire d'Excellence TOUCAN.
References
1. Hanahan D, Weinberg RA. Hallmarks of cancer: the next generation. Cell (2011) 144:646–74. doi: 10.1016/j.cell.2011.02.013
2. Hanahan D, Coussens LM. Accessories to the crime: functions of cells recruited to the tumor microenvironment. Cancer Cell (2012) 21:309–22. doi: 10.1016/j.ccr.2012.02.022
3. Grivennikov SI, Greten FR, Karin M. Immunity, inflammation, and cancer. Cell (2010) 140:883–99. doi: 10.1016/j.cell.2010.01.025
4. Leibovici J, Itzhaki O, Huszar M, Sinai J. The tumor microenvironment: part 1. Immunotherapy (2011) 3:1367–84. doi: 10.2217/imt.11.111
5. Candido J, Hagemann T. Cancer-Related Inflammation. J Clin Immunol. (2013) 33:79–84. doi: 10.1007/s10875-012-9847-0
6. Schmitz J, Owyang A, Oldham E, Song Y, Murphy E, McClanahan TK, et al. IL-33, an interleukin-1-like cytokine that signals via the IL-1 receptor-related protein ST2 and induces T helper type 2-associated cytokines. Immunity (2005) 23:479–90. doi: 10.1016/j.immuni.2005.09.015
7. Moussion C, Ortega N, Girard J-P. The IL-1-like cytokine IL-33 is constitutively expressed in the nucleus of endothelial cells and epithelial cells in vivo: a novel “alarmin”? PLoS ONE (2008) 3:e3331. doi: 10.1371/journal.pone.0003331
8. Carriere V, Roussel L, Ortega N, Lacorre D-A, Americh L, Aguilar L, et al. IL-33, the IL-1-like cytokine ligand for ST2 receptor, is a chromatin-associated nuclear factor in vivo. Proc Natl Acad Sci USA. (2007) 104:282–7. doi: 10.1073/pnas.0606854104
9. Baekkevold ES, Roussigné M, Yamanaka T, Johansen F-E, Jahnsen FL, Amalric F, et al. Molecular characterization of NF-HEV, a nuclear factor preferentially expressed in human high endothelial venules. Am J Pathol. (2003) 163:69–79. doi: 10.1016/S0002-9440(10)63631-0
10. Hardman CS, Panova V, McKenzie ANJ. IL-33 citrine reporter mice reveal the temporal and spatial expression of IL-33 during allergic lung inflammation. Eur J Immunol. (2013) 43:488–98. doi: 10.1002/eji.201242863
11. Pichery M, Mirey E, Mercier P, Lefrancais E, Dujardin A, Ortega N, et al. Endogenous IL-33 is highly expressed in mouse epithelial barrier tissues, lymphoid organs, brain, embryos, and inflamed tissues: in situ analysis using a novel Il-33-LacZ gene trap reporter strain. J Immunol. (2012) 188:3488–95. doi: 10.4049/jimmunol.1101977
12. Lingel A, Weiss TM, Niebuhr M, Pan B, Appleton BA, Wiesmann C, et al. Structure of IL-33 and its interaction with the ST2 and IL-1RAcP receptors–insight into heterotrimeric IL-1 signaling complexes. Structure (2009) 17:1398–410. doi: 10.1016/j.str.2009.08.009
13. Bonilla WV, Fröhlich A, Senn K, Kallert S, Fernandez M, Johnson S, et al. The alarmin interleukin-33 drives protective antiviral CD8+ T cell responses. Science (2012) 335:984–9. doi: 10.1126/science.1215418
14. Cayrol C, Girard J-P. The IL-1-like cytokine IL-33 is inactivated after maturation by caspase-1. Proc Natl Acad Sci USA. (2009) 106:9021–6. doi: 10.1073/pnas.0812690106
15. Gadani SP, Walsh JT, Smirnov I, Zheng J, Kipnis J. The glia-derived alarmin IL-33 orchestrates the immune response and promotes recovery following CNS injury. Neuron (2015) 85:703–9. doi: 10.1016/j.neuron.2015.01.013
16. Miller AM. Role of IL-33 in inflammation and disease. J Inflamm. (2011) 8:22. doi: 10.1186/1476-9255-8-22
17. Chen WY, Hong J, Gannon J, Kakkar R, Lee RT. Myocardial pressure overload induces systemic inflammation through endothelial cell IL-33. Proc Natl Acad Sci USA. (2015) 112:7249–54. doi: 10.1073/pnas.1424236112
18. Kakkar R, Hei H, Dobner S, Lee RT. Interleukin 33 as a mechanically responsive cytokine secreted by living cells. J Biol Chem. (2012) 287:6941–8. doi: 10.1074/jbc.M111.298703
19. Rickard JA, O'Donnell JA, Evans JM, Lalaoui N, Poh AR, Rogers T, et al. RIPK1 regulates RIPK3-MLKL-driven systemic inflammation and emergency hematopoiesis. Cell (2014) 157:1175–88. doi: 10.1016/j.cell.2014.04.019
20. McHedlidze T, Waldner M, Zopf S, Walker J, Rankin AL, Schuchmann M, et al. Interleukin-33-dependent innate lymphoid cells mediate hepatic fibrosis. Immunity (2013) 39:357–71. doi: 10.1016/j.immuni.2013.07.018
21. Molofsky AB, Van Gool F, Liang H-E, Van Dyken SJ, Nussbaum JC, Lee J, et al. Interleukin-33 and interferon-γ counter-regulate group 2 innate lymphoid cell activation during immune perturbation. Immunity (2015) 43:161–74. doi: 10.1016/j.immuni.2015.05.019
22. Liew FY, Girard J-P, Turnquist HR. Interleukin-33 in health and disease. Nat Rev Immunol. (2016) 16:676–89. doi: 10.1038/nri.2016.95
23. Lloyd CM. IL-33 family members and asthma - bridging innate and adaptive immune responses. Curr Opin Immunol. (2010) 22:800–6. doi: 10.1016/j.coi.2010.10.006
24. Mizutani N, Nabe T, Yoshino S. IL-17A promotes the exacerbation of IL-33-induced airway hyperresponsiveness by enhancing neutrophilic inflammation via CXCR2 signaling in mice. J Immunol. (2014) 192:1372–84. doi: 10.4049/jimmunol.1301538
25. Cayrol C, Duval A, Schmitt P, Roga S, Camus M, Stella A, et al. Environmental allergens induce allergic inflammation through proteolytic maturation of IL-33. Nat Immunol. (2018) 19:375–85. doi: 10.1038/s41590-018-0067-5
26. Talabot-Ayer D, McKee T, Gindre P, Bas S, Baeten DL, Gabay C, et al. Distinct serum and synovial fluid interleukin (IL)-33 levels in rheumatoid arthritis, psoriatic arthritis and osteoarthritis. Joint Bone Spine (2012) 79:32–37. doi: 10.1016/j.jbspin.2011.02.011
27. Tajima S, Bando M, Ohno S, Sugiyama Y, Oshikawa K, Tominaga S, et al. ST2 gene induced by type 2 helper T cell (Th2) and proinflammatory cytokine stimuli may modulate lung injury and fibrosis. Exp Lung Res. (2007) 33:81–97. doi: 10.1080/01902140701198583
28. Cevikbas F, Steinhoff M. IL-33: a novel danger signal system in atopic dermatitis. J Invest Dermatol. (2012) 132:1326–9. doi: 10.1038/jid.2012.66
29. Hueber AJ, Alves-Filho JC, Asquith DL, Michels C, Millar NL, Reilly JH, et al. IL-33 induces skin inflammation with mast cell and neutrophil activation. Eur J Immunol. (2011) 41:2229–37. doi: 10.1002/eji.201041360
30. Gupta RK, Gupta K, Dwivedi PD. Pathophysiology of IL-33 and IL-17 in allergic disorders. Cytokine Growth Factor Rev. (2017) 38:22–36. doi: 10.1016/j.cytogfr.2017.09.005
31. Griesenauer B, Paczesny S. The ST2/IL-33 axis in immune cells during inflammatory diseases. Front Immunol. (2017) 8:475. doi: 10.3389/fimmu.2017.00475
32. Garlanda C, Dinarello CA, Mantovani A. The interleukin-1 family: back to the future. Immunity (2013) 39:1003–18. doi: 10.1016/j.immuni.2013.11.010
33. Braun H, Afonina IS, Mueller C, Beyaert R. Dichotomous function of IL-33 in health and disease: from biology to clinical implications. Biochem Pharmacol. (2018) 148:238–52. doi: 10.1016/j.bcp.2018.01.010
34. Di Salvo E, Ventura-Spagnolo E, Casciaro M, Navarra M, Gangemi S. IL-33/IL-31 axis: a potential inflammatory pathway. Mediators Inflamm. (2018) 2018:3858032. doi: 10.1155/2018/3858032
35. Espinassous Q, Garcia-de-Paco E, Garcia-Verdugo I, Synguelakis M, von Aulock S, Sallenave J-M, et al. IL-33 enhances lipopolysaccharide-induced inflammatory cytokine production from mouse macrophages by regulating lipopolysaccharide receptor complex. J Immunol. (2009) 183:1446–55. doi: 10.4049/jimmunol.0803067
36. Hayakawa H, Hayakawa M, Kume A, Tominaga S. Soluble ST2 blocks interleukin-33 signaling in allergic airway inflammation. J Biol Chem. (2007) 282:26369–80. doi: 10.1074/jbc.M704916200
37. Iwahana H, Yanagisawa K, Ito-Kosaka A, Kuroiwa K, Tago K, Komatsu N, et al. Different promoter usage and multiple transcription initiation sites of the interleukin-1 receptor-related human ST2 gene in UT-7 and TM12 cells. Eur J Biochem. (1999) 264:397–406.
38. Bergers G, Reikerstorfer A, Braselmann S, Graninger P, Busslinger M. Alternative promoter usage of the Fos-responsive gene Fit-1 generates mRNA isoforms coding for either secreted or membrane-bound proteins related to the IL-1 receptor. EMBO J. (1994) 13:1176–88.
39. Ali S, Huber M, Kollewe C, Bischoff SC, Falk W, Martin MU. IL-1 receptor accessory protein is essential for IL-33-induced activation of T lymphocytes and mast cells. Proc Natl Acad Sci USA. (2007) 104:18660–5. doi: 10.1073/pnas.0705939104
40. Pecaric-Petkovic T, Didichenko SA, Kaempfer S, Spiegl N, Dahinden CA. Human basophils and eosinophils are the direct target leukocytes of the novel IL-1 family member IL-33. Blood (2009) 113:1526–34. doi: 10.1182/blood-2008-05-157818
41. Neill DR, Wong SH, Bellosi A, Flynn RJ, Daly M, Langford TKA, et al. Nuocytes represent a new innate effector leukocyte that mediates type-2 immunity. Nature (2010) 464:1367–70. doi: 10.1038/nature08900
42. Chang Y-J, Kim HY, Albacker LA, Baumgarth N, McKenzie ANJ, Smith DE, et al. Innate lymphoid cells mediate influenza-induced airway hyper-reactivity independently of adaptive immunity. Nat Immunol. (2011) 12:631–8. doi: 10.1038/ni.2045
43. Kurowska-Stolarska M, Stolarski B, Kewin P, Murphy G, Corrigan CJ, Ying S, et al. IL-33 amplifies the polarization of alternatively activated macrophages that contribute to airway inflammation. J Immunol. (2009) 183:6469–77. doi: 10.4049/jimmunol.0901575
44. Turnquist HR, Sumpter TL, Tsung A, Zahorchak AF, Nakao A, Nau GJ, et al. IL-1beta-driven ST2L expression promotes maturation resistance in rapamycin-conditioned dendritic cells. J Immunol. (2008) 181:62–72. doi: 10.4049/jimmunol.181.1.62
45. Rank MA, Kobayashi T, Kozaki H, Bartemes KR, Squillace DL, Kita H. IL-33-activated dendritic cells induce an atypical TH2-type response. J Allergy Clin Immunol. (2009) 123:1047–54. doi: 10.1016/j.jaci.2009.02.026
46. Park SH, Kim MS, Lim HX, Cho D, Kim TS. IL-33-matured dendritic cells promote Th17 cell responses via IL-1β and IL-6. Cytokine (2017) 99:106–13. doi: 10.1016/j.cyto.2017.07.022
47. Vaccaro M, Cicero F, Mannucci C, Calapai G, Spatari G, Barbuzza O, et al. IL-33 circulating serum levels are increased in patients with non-segmental generalized vitiligo. Arch Dermatol Res. (2016) 308:527–30. doi: 10.1007/s00403-016-1675-2
48. Liang M, Liwen Z, Yun Z, Yanbo D, Jianping C. Serum levels of IL-33 and correlation with IL-4, IL-17A, and hypergammaglobulinemia in patients with autoimmune hepatitis. Mediators Inflamm. (2018) 2018:7964654. doi: 10.1155/2018/7964654
49. Theoharides TC, Petra AI, Taracanova A, Panagiotidou S, Conti P. Targeting IL-33 in autoimmunity and inflammation. J Pharmacol Exp Ther. (2015) 354:24–31. doi: 10.1124/jpet.114.222505
50. Han JM, Wu D, Denroche HC, Yao Y, Verchere CB, Levings MK. IL-33 Reverses an obesity-induced deficit in visceral adipose tissue ST2+ T regulatory cells and ameliorates adipose tissue inflammation and insulin resistance. J Immunol. (2015) 194:4777–83. doi: 10.4049/jimmunol.1500020
51. Hasnain SZ, Borg DJ, Harcourt BE, Tong H, Sheng YH, Ng CP, et al. Glycemic control in diabetes is restored by therapeutic manipulation of cytokines that regulate beta cell stress. Nat Med. (2014) 20:1417–26. doi: 10.1038/nm.3705
52. Baumann C, Bonilla WV, Fröhlich A, Helmstetter C, Peine M, Hegazy AN, et al. T-bet- and STAT4-dependent IL-33 receptor expression directly promotes antiviral Th1 cell responses. Proc Natl Acad Sci USA. (2015) 112:4056–61. doi: 10.1073/pnas.1418549112
53. Yang Q, Li G, Zhu Y, Liu L, Chen E, Turnquist H, et al. IL-33 synergizes with TCR and IL-12 signaling to promote the effector function of CD8+ T cells. Eur J Immunol. (2011) 41:3351–60. doi: 10.1002/eji.201141629
54. Smithgall MD, Comeau MR, Yoon B-RP, Kaufman D, Armitage R, Smith DE. IL-33 amplifies both Th1- and Th2-type responses through its activity on human basophils, allergen-reactive Th2 cells, iNKT and NK cells. Int Immunol. (2008) 20:1019–30. doi: 10.1093/intimm/dxn060
55. Bourgeois E, Van LP, Samson M, Diem S, Barra A, Roga S, et al. The pro-Th2 cytokine IL-33 directly interacts with invariant NKT and NK cells to induce IFN-gamma production. Eur J Immunol. (2009) 39:1046–55. doi: 10.1002/eji.200838575
56. Zhang J, Wang P, Ji W, Ding Y, Lu X. Overexpression of interleukin-33 is associated with poor prognosis of patients with glioma. Int J Neurosci. (2017) 127:210–7. doi: 10.1080/00207454.2016.1175441
57. Sun P, Ben Q, Tu S, Dong W, Qi X, Wu Y. Serum interleukin-33 levels in patients with gastric cancer. Dig Dis Sci. (2011) 56:3596–601. doi: 10.1007/s10620-011-1760-5
58. Zhang P, Liu X-K, Chu Z, Ye JC, Li K-L, Zhuang W-L, et al. Detection of interleukin-33 in serum and carcinoma tissue from patients with hepatocellular carcinoma and its clinical implications. J Int Med Res. (2012) 40:1654–61. doi: 10.1177/030006051204000504
59. Santulli P, Even M, Chouzenoux S, Millischer AE, Borghese B, de Ziegler D, et al. Profibrotic interleukin-33 is correlated with uterine leiomyoma tumour burden. Hum Reprod. (2013) 28:2126–33. doi: 10.1093/humrep/det238
60. Wang C, Chen Z, Bu X, Han Y, Shan S, Ren T, et al. IL-33 signaling fuels outgrowth and metastasis of human lung cancer. Biochem Biophys Res Commun. (2016) 479:461–8. doi: 10.1016/j.bbrc.2016.09.081
61. Liu X, Zhu L, Lu X, Bian H, Wu X, Yang W, et al. IL-33/ST2 pathway contributes to metastasis of human colorectal cancer. Biochem Biophys Res Commun. (2014) 453:486–92. doi: 10.1016/j.bbrc.2014.09.106
62. Chen S-F, Nieh S, Jao S-W, Wu M-Z, Liu C-L, Chang Y-C, et al. The paracrine effect of cancer-associated fibroblast-induced interleukin-33 regulates the invasiveness of head and neck squamous cell carcinoma. J Pathol. (2013) 231:180–9. doi: 10.1002/path.4226
63. Liu J, Shen J-X, Hu J-L, Huang W-H, Zhang G-J. Significance of interleukin-33 and its related cytokines in patients with breast cancers. Front Immunol. (2014) 5:141. doi: 10.3389/fimmu.2014.00141
64. Cancer Genome Atlas Research Network, Weinstein JN, Collisson EA, Mills GB, Shaw KRM, Ozenberger BA, et al. The Cancer genome atlas pan-cancer analysis project. Nat Genet. (2013) 45:1113–20. doi: 10.1038/ng.2764
65. Kim JY, Lim S-C, Kim G, Yun HJ, Ahn S-G, Choi HS. Interleukin-33/ST2 axis promotes epithelial cell transformation and breast tumorigenesis via upregulation of COT activity. Oncogene (2015) 34:4928–38. doi: 10.1038/onc.2014.418
66. Tong X, Barbour M, Hou K, Gao C, Cao S, Zheng J, et al. Interleukin-33 predicts poor prognosis and promotes ovarian cancer cell growth and metastasis through regulating ERK and JNK signaling pathways. Mol Oncol. (2016) 10:113–25. doi: 10.1016/j.molonc.2015.06.004
67. Wang Z, Xu L, Chang Y, Zhou L, Fu H, Zhang W, et al. IL-33 is associated with unfavorable postoperative survival of patients with clear-cell renal cell carcinoma. Tumour Biol. (2016) 37:11127–34. doi: 10.1007/s13277-016-4879-3
68. Bergis D, Kassis V, Ranglack A, Koeberle V, Piiper A, Kronenberger B, et al. High serum levels of the interleukin-33 receptor soluble ST2 as a negative prognostic factor in hepatocellular carcinoma. Transl Oncol. (2013) 6:311–8. doi: 10.1593/tlo.12418
69. Akimoto M, Maruyama R, Takamaru H, Ochiya T, Takenaga K. Soluble IL-33 receptor sST2 inhibits colorectal cancer malignant growth by modifying the tumour microenvironment. Nat Commun. (2016) 7:13589. doi: 10.1038/ncomms13589
70. Yang M, Feng Y, Yue C, Xu B, Chen L, Jiang J, et al. Lower expression level of IL-33 is associated with poor prognosis of pulmonary adenocarcinoma. PLoS ONE (2018) 13:e0193428. doi: 10.1371/journal.pone.0193428
71. Kim MS, Kim E, Heo J-S, Bae D-J, Lee J-UW, Lee T-H, et al. Circulating IL-33 level is associated with the progression of lung cancer. Lung Cancer (2015) 90:346–51. doi: 10.1016/j.lungcan.2015.08.011
72. Hsu Y-L, Hung J-Y, Lee Y-L, Chen F-W, Chang K-F, Chang W-A, Tsai Y-M, Chong I-W, Kuo P-L. Identification of novel gene expression signature in lung adenocarcinoma by using next-generation sequencing data and bioinformatics analysis. Oncotarget (2017) 8:104831–54. doi: 10.18632/oncotarget.21022
73. Koster R, Panagiotou OA, Wheeler WA, Karlins E, Gastier-Foster JM, Caminada de Toledo SR, et al. Genome-wide association study identifies the GLDC/IL33 locus associated with survival of osteosarcoma patients. Int J Cancer (2018) 142:1594–601. doi: 10.1002/ijc.31195
74. Ding L, Ren J, Zhang D, Li Y, Huang X, Hu Q, et al. A novel stromal lncRNA signature reprograms fibroblasts to promote the growth of oral squamous cell carcinoma via LncRNA-CAF/interleukin-33. Carcinogenesis (2018) 39:397–406. doi: 10.1093/carcin/bgy006
75. Yu X-X, Hu Z, Shen X, Dong L-Y, Zhou W-Z, Hu W-H. IL-33 promotes gastric cancer cell invasion and migration via ST2-ERK1/2 pathway. Dig Dis Sci. (2015) 60:1265–72. doi: 10.1007/s10620-014-3463-1
76. Fang M, Li Y, Huang K, Qi S, Zhang J, Zgodzinski W, et al. IL33 promotes colon cancer cell stemness via JNK activation and macrophage recruitment. Cancer Res. (2017) 77:2735–45. doi: 10.1158/0008-5472.CAN-16-1602
77. Milosavljevic MZ, Jovanovic IP, Pejnovic NN, Mitrovic SLJ, Arsenijevic NN, Simovic Markovic BJ, et al. Deletion of IL-33R attenuates VEGF expression and enhances necrosis in mammary carcinoma. Oncotarget (2016) 7:18106–15. doi: 10.18632/oncotarget.7635
78. Maywald RL, Doerner SK, Pastorelli L, De Salvo C, Benton SM, Dawson EP, et al. IL-33 activates tumor stroma to promote intestinal polyposis. Proc Natl Acad Sci USA. (2015) 112:E2487–96. doi: 10.1073/pnas.1422445112
79. Mertz KD, Mager LF, Wasmer M-H, Thiesler T, Koelzer VH, Ruzzante G, et al. The IL-33/ST2 pathway contributes to intestinal tumorigenesis in humans and mice. Oncoimmunology (2016) 5:e1062966. doi: 10.1080/2162402X.2015.1062966
80. Mager LF, Riether C, Schürch CM, Banz Y, Wasmer M-H, Stuber R, et al. IL-33 signaling contributes to the pathogenesis of myeloproliferative neoplasms. J Clin Invest. (2015) 125:2579–91. doi: 10.1172/JCI77347
81. O'Donnell C, Mahmoud A, Keane J, Murphy C, White D, Carey S, et al. An antitumorigenic role for the IL-33 receptor, ST2L, in colon cancer. Br J Cancer (2016) 114:37–43. doi: 10.1038/bjc.2015.433
82. Wang K, Shan S, Yang Z, Gu X, Wang Y, Wang C, et al. IL-33 blockade suppresses tumor growth of human lung cancer through direct and indirect pathways in a preclinical model. Oncotarget (2017) 8:68571–82. doi: 10.18632/oncotarget.19786
83. Wan S, Zhao E, Kryczek I, Vatan L, Sadovskaya A, Ludema G, et al. Tumor-associated macrophages produce interleukin 6 and signal via STAT3 to promote expansion of human hepatocellular carcinoma stem cells. Gastroenterology (2014) 147:1393–404. doi: 10.1053/j.gastro.2014.08.039
84. Ariyoshi W, Okinaga T, Chaweewannakorn W, Akifusa S, Nisihara T. Mechanisms involved in enhancement of matrix metalloproteinase-9 expression in macrophages by interleukin-33. J Cell Physiol. (2017) 232:3481–95. doi: 10.1002/jcp.25809
85. Yang Y, Andersson P, Hosaka K, Zhang Y, Cao R, Iwamoto H, et al. The PDGF-BB-SOX7 axis-modulated IL-33 in pericytes and stromal cells promotes metastasis through tumour-associated macrophages. Nat Commun. (2016) 7:11385. doi: 10.1038/ncomms11385
86. Fang K-M, Yang C-S, Lin TC, Chan TC, Tzeng S-F. Induced interleukin-33 expression enhances the tumorigenic activity of rat glioma cells. Neuro-Oncol (2014) 16:552–66. doi: 10.1093/neuonc/not234
87. Huang J-R, Tsai Y-C, Chang YJ, Wu JC, Hung JT, Lin K-H, et al. α-Galactosylceramide but not phenyl-glycolipids induced NKT cell anergy and IL-33-mediated myeloid-derived suppressor cell accumulation via upregulation of egr2/3. J Immunol. (2014) 192:1972–81. doi: 10.4049/jimmunol.1302623
88. Zhang Y, Davis C, Shah S, Hughes D, Ryan JC, Altomare D, et al. IL-33 promotes growth and liver metastasis of colorectal cancer in mice by remodeling the tumor microenvironment and inducing angiogenesis. Mol Carcinog. (2017) 56:272–87. doi: 10.1002/mc.22491
89. Xiao P, Wan X, Cui B, Liu Y, Qiu C, Rong J, et al. Interleukin 33 in tumor microenvironment is crucial for the accumulation and function of myeloid-derived suppressor cells. Oncoimmunology (2016) 5:e1063772. doi: 10.1080/2162402X.2015.1063772
90. Jovanovic IP, Pejnovic NN, Radosavljevic GD, Pantic JM, Milovanovic MZ, Arsenijevic NN, et al. Interleukin-33/ST2 axis promotes breast cancer growth and metastases by facilitating intratumoral accumulation of immunosuppressive and innate lymphoid cells. Int J Cancer (2014) 134:1669–82. doi: 10.1002/ijc.28481
91. Lim HX, Choi S, Cho D, Kim TS. IL-33 inhibits the differentiation and immunosuppressive activity of granulocytic myeloid-derived suppressor cells in tumor-bearing mice. Immunol Cell Biol. (2017) 95:99–107. doi: 10.1038/icb.2016.72
92. Beltrán CJ, Núñez LE, Díaz-Jiménez D, Farfan N, Candia E, Heine C, et al. Characterization of the novel ST2/IL-33 system in patients with inflammatory bowel disease. Inflamm Bowel Dis. (2010) 16:1097–107. doi: 10.1002/ibd.21175
93. Pastorelli L, Garg RR, Hoang SB, Spina L, Mattioli B, Scarpa M, et al. Epithelial-derived IL-33 and its receptor ST2 are dysregulated in ulcerative colitis and in experimental Th1/Th2 driven enteritis. Proc Natl Acad Sci USA. (2010) 107:8017–22. doi: 10.1073/pnas.0912678107
94. Schiering C, Krausgruber T, Chomka A, Fröhlich A, Adelmann K, Wohlfert EA, et al. The alarmin IL-33 promotes regulatory T-cell function in the intestine. Nature (2014) 513:564–8. doi: 10.1038/nature13577
95. Meinicke H, Bremser A, Brack M, Akeus P, Pearson C, Bullers S, et al. Tumour-associated changes in intestinal epithelial cells cause local accumulation of KLRG1+ GATA3+ regulatory T cells in mice. Immunology (2017) 152:74–88. doi: 10.1111/imm.12750
96. Enoksson M, Lyberg K, Möller-Westerberg C, Fallon PG, Nilsson G, Lunderius-Andersson C. Mast cells as sensors of cell injury through IL-33 recognition. J Immunol. (2011) 186:2523–2528. doi: 10.4049/jimmunol.1003383
97. Rigoni A, Bongiovanni L, Burocchi A, Sangaletti S, Danelli L, Guarnotta C, et al. Mast cells infiltrating inflamed or transformed gut alternatively sustain mucosal healing or tumor growth. Cancer Res. (2015) 75:3760–70. doi: 10.1158/0008-5472.CAN-14-3767
98. Moulin D, Donzé O, Talabot-Ayer D, Mézin F, Palmer G, Gabay C. Interleukin (IL)-33 induces the release of pro-inflammatory mediators by mast cells. Cytokine (2007) 40:216–25. doi: 10.1016/j.cyto.2007.09.013
99. Gulubova M, Vlaykova T. Prognostic significance of mast cell number and microvascular density for the survival of patients with primary colorectal cancer. J Gastroenterol Hepatol. (2009) 24:1265–75. doi: 10.1111/j.1440-1746.2007.05009.x
100. Malfettone A, Silvestris N, Saponaro C, Ranieri G, Russo A, Caruso S, et al. High density of tryptase-positive mast cells in human colorectal cancer: a poor prognostic factor related to protease-activated receptor 2 expression. J Cell Mol Med. (2013) 17:1025–37. doi: 10.1111/jcmm.12073
101. Byrne SN, Beaugie C, O'Sullivan C, Leighton S, Halliday GM. The immune-modulating cytokine and endogenous alarmin interleukin-33 is upregulated in skin exposed to inflammatory UVB radiation. Am J Pathol. (2011) 179:211–22. doi: 10.1016/j.ajpath.2011.03.010
102. Loser K, Apelt J, Voskort M, Mohaupt M, Balkow S, Schwarz T, et al. IL-10 controls ultraviolet-induced carcinogenesis in mice. J Immunol. (2007) 179:365–71.
103. Fang Y, Zhao L, Xiao H, Cook KM, Bai Q, Herrick EJ, et al. IL-33 acts as a foe to MIA PaCa-2 pancreatic cancer. Med Oncol. (2017) 34:23. doi: 10.1007/s12032-016-0880-3
104. Saranchova I, Han J, Huang H, Fenninger F, Choi KB, Munro L, et al. Discovery of a metastatic immune escape mechanism initiated by the loss of expression of the tumour biomarker interleukin-33. Sci Rep. (2016) 6:30555. doi: 10.1038/srep30555
105. Brunner SM, Rubner C, Kesselring R, Martin M, Griesshammer E, Ruemmele P, et al. Tumor-infiltrating, interleukin-33-producing effector-memory CD8(+) T cells in resected hepatocellular carcinoma prolong patient survival. Hepatology (2015) 61:1957–67. doi: 10.1002/hep.27728
106. Lucarini V, Ziccheddu G, Macchia I, La Sorsa V, Peschiaroli F, Buccione C, et al. IL-33 restricts tumor growth and inhibits pulmonary metastasis in melanoma-bearing mice through eosinophils. Oncoimmunology (2017) 6:e1317420. doi: 10.1080/2162402X.2017.1317420
107. Qin L, Dominguez D, Chen S, Fan J, Long A, Zhang M, et al. Exogenous IL-33 overcomes T cell tolerance in murine acute myeloid leukemia. Oncotarget (2016) 7:61069–80. doi: 10.18632/oncotarget.11179
108. Eissmann MF, Dijkstra C, Wouters MA, Baloyan D, Mouradov D, Nguyen PM, et al. Interleukin 33 signaling restrains sporadic colon cancer in an interferon-γ-dependent manner. Cancer Immunol Res. (2018) 6:409–21. doi: 10.1158/2326-6066.CIR-17-0218
109. Chen H, Chen Y, Liu H, Que Y, Zhang X, Zheng F. Integrated expression profiles analysis reveals correlations between the IL-33/ST2 Axis and CD8+ T cells, regulatory T cells, and myeloid-derived suppressor cells in soft tissue sarcoma. Front Immunol. (2018) 9:1179. doi: 10.3389/fimmu.2018.01179
110. Jovanovic I, Radosavljevic G, Mitrovic M, Juranic VL, McKenzie ANJ, Arsenijevic N, et al. ST2 deletion enhances innate and acquired immunity to murine mammary carcinoma. Eur J Immunol. (2011) 41:1902–12. doi: 10.1002/eji.201141417
111. Gao X, Wang X, Yang Q, Zhao X, Wen W, Li G, et al. Tumoral expression of IL-33 inhibits tumor growth and modifies the tumor microenvironment through CD8+ T and NK cells. J Immunol. (2015) 194:438–45. doi: 10.4049/jimmunol.1401344
112. Gao K, Li X, Zhang L, Bai L, Dong W, Gao K, et al. Transgenic expression of IL-33 activates CD8(+) T cells and NK cells and inhibits tumor growth and metastasis in mice. Cancer Lett. (2013) 335:463–71. doi: 10.1016/j.canlet.2013.03.002
113. Dominguez D, Ye C, Geng Z, Chen S, Fan J, Qin L, et al. Exogenous IL-33 restores dendritic cell activation and maturation in established cancer. J Immunol. (2017) 198:1365–75. doi: 10.4049/jimmunol.1501399
114. Asadzadeh Z, Mohammadi H, Safarzadeh E, Hemmatzadeh M, Mahdian-Shakib A, Jadidi-Niaragh F, et al. The paradox of Th17 cell functions in tumor immunity. Cell Immunol. (2017) 322:15–25. doi: 10.1016/j.cellimm.2017.10.015
115. Song Y, Yang JM. Role of interleukin (IL)-17 and T-helper (Th)17 cells in cancer. Biochem Biophys Res Commun. (2017) 493:1–8. doi: 10.1016/j.bbrc.2017.08.109
116. Kempski J, Brockmann L, Gagliani N, Huber S. TH17 cell and epithelial cell crosstalk during inflammatory bowel disease and carcinogenesis. Front Immunol. (2017) 8:1373. doi: 10.3389/fimmu.2017.01373
117. Pascual-Reguant A, Bayat Sarmadi J, Baumann C, Noster R, Cirera-Salinas D, Curato C, et al. TH17 cells express ST2 and are controlled by the alarmin IL-33 in the small intestine. Mucosal Immunol. (2017) 10:1431–42. doi: 10.1038/mi.2017.5
118. de Kleer IM, Kool M, de Bruijn MJW, Willart M, van Moorleghem J, Schuijs MJ, et al. Perinatal activation of the interleukin-33 pathway promotes type 2 immunity in the developing lung. Immunity (2016) 45:1285–98. doi: 10.1016/j.immuni.2016.10.031
119. Zhao Y, Chu X, Chen J, Wang Y, Gao S, Jiang Y, et al. Dectin-1-activated dendritic cells trigger potent antitumour immunity through the induction of Th9 cells. Nat Commun. (2016) 7:12368. doi: 10.1038/ncomms12368
120. Chen J, Zhao Y, Jiang Y, Gao S, Wang Y, Wang D, et al. Interleukin-33 contributes to the induction of Th9 cells and antitumor efficacy by dectin-1-activated dendritic cells. Front Immunol. (2018) 9:1787. doi: 10.3389/fimmu.2018.01787
121. Li J, Razumilava N, Gores GJ, Walters S, Mizuochi T, Mourya R, et al. Biliary repair and carcinogenesis are mediated by IL-33-dependent cholangiocyte proliferation. J Clin Invest. (2014) 124:3241–51. doi: 10.1172/JCI73742
122. Saranchova I, Han J, Zaman R, Arora H, Huang H, Fenninger F, et al. Type 2 innate lymphocytes actuate immunity against tumours and limit cancer metastasis. Sci Rep. (2018) 8:2924. doi: 10.1038/s41598-018-20608-6
123. Kim J, Kim W, Moon UJ, Kim HJ, Choi H-J, Sin J-I, et al. Intratumorally establishing type 2 innate lymphoid cells blocks tumor growth. J Immunol. (2016) 196:2410–23. doi: 10.4049/jimmunol.1501730
124. Wasmer M-H, Krebs P. The role of IL-33-dependent inflammation in the tumor microenvironment. Front Immunol. (2016) 7:682. doi: 10.3389/fimmu.2016.00682
125. Villarreal DO, Wise MC, Walters JN, Reuschel EL, Choi MJ, Obeng-Adjei N, Yet al. Alarmin IL-33 acts as an immunoadjuvant to enhance antigen-specific tumor immunity. Cancer Res. (2014) 74:1789–800. doi: 10.1158/0008-5472.CAN-13-2729
126. Villarreal DO, Weiner DB. IL-33 isoforms: their future as vaccine adjuvants? Expert Rev Vaccines (2015) 14:489–92. doi: 10.1586/14760584.2015.1011135
127. Duault C, Franchini DM, Familliades J, Cayrol C, Roga S, Girard J-P, et al. TCRVγ9 γδ T cell response to IL-33: A CD4 T cell-dependent mechanism. J Immunol. (2016) 196:493–502. doi: 10.4049/jimmunol.1500260
128. Duault C, Betous D, Bezombes C, Roga S, Cayrol C, Girard J-P, et al. IL-33-expanded human Vγ9Vδ2 T cells have anti-lymphoma effect in a mouse tumor model. Eur J Immunol. (2017) 47:2137–41. doi: 10.1002/eji.201747093
129. Kallert SM, Darbre S, Bonilla WV, Kreutzfeldt M, Page N, Müller P, et al. Replicating viral vector platform exploits alarmin signals for potent CD8+ T cell-mediated tumour immunotherapy. Nat Commun. (2017) 8: 15327. doi: 10.1038/ncomms15327
130. Ramadan A, Griesenauer B, Adom D, Kapur R, Hanenberg H, Liu C, et al. Specifically differentiated T cell subset promotes tumor immunity over fatal immunity. J Exp Med. (2017) 214:3577–96. doi: 10.1084/jem.20170041
Keywords: interleukin-33, immunity, cancer, immunosuppression, microenvironment
Citation: Fournié J-J and Poupot M (2018) The Pro-tumorigenic IL-33 Involved in Antitumor Immunity: A Yin and Yang Cytokine. Front. Immunol. 9:2506. doi: 10.3389/fimmu.2018.02506
Received: 28 May 2018; Accepted: 10 October 2018;
Published: 26 October 2018.
Edited by:
Hui-Rong Jiang, University of Strathclyde, United KingdomReviewed by:
Paola Italiani, Consiglio Nazionale Delle Ricerche (CNR), ItalyKesley Attridge, Aston University, United Kingdom
Copyright © 2018 Fournié and Poupot. This is an open-access article distributed under the terms of the Creative Commons Attribution License (CC BY). The use, distribution or reproduction in other forums is permitted, provided the original author(s) and the copyright owner(s) are credited and that the original publication in this journal is cited, in accordance with accepted academic practice. No use, distribution or reproduction is permitted which does not comply with these terms.
*Correspondence: Mary Poupot, bWFyeS5wb3Vwb3RAaW5zZXJtLmZy