- 1School of Medicine, Trinity Biomedical Sciences Institute, Trinity College Dublin, Dublin, Ireland
- 2National Children's Research Centre, Our Lady's Children's Hospital, Dublin, Ireland
- 3Trinity Translational Medicine Institute, St James's Hospital, Trinity College Dublin, Dublin, Ireland
Schistosomiasis is a major cause of morbidity in humans invoked by chronic infection with parasitic trematodes of the genus Schistosoma. Schistosomes have a complex life-cycle involving infections of an aquatic snail intermediate host and a definitive mammalian host. In humans, adult male and female worms lie within the vasculature. Here, they propagate and eggs are laid. These eggs must then be released from the host to continue the life cycle. Schistosoma mansoni and Schistosoma japonicum reside in the mesenteric circulation of the intestines with egg excreted in the feces. In contrast, S. haematobium are present in the venus plexus of the bladder, expelling eggs in the urine. In an impressive case of exploitation of the host immune system, this process of Schistosome “eggs-iting” the host is immune dependent. In this article, we review the formation of the egg granuloma and explore how S. mansoni eggs laid in vasculature must usurp immunity to induce regulated inflammation, to facilitate extravasation through the intestinal wall and to be expelled in the feces. We highlight the roles of immune cell populations, stromal factors, and egg secretions in the process of egg excretion to provide a comprehensive overview of the current state of knowledge regarding a vastly unexplored mechanism.
Introduction
Schistosomiasis (Bilharzia) is one of the worlds most common parasitic infections with over 200 million people requiring preventive treatment in 2016 (1, 2). While the majority of people at risk live in the endemic regions of Africa, Schistosoma species are also prevalent in the Middle East, the Caribbean, South America, and South East Asia. Autochthonous transmission of schistosomes has also been reported in Corsica, France (3, 4). Using novel, more sensitive diagnostic techniques to reveal “egg-negative/worm-positive schistosomiasis,” Colley et al. highlighted that the global prevalence of schistosomiasis may actually exceed current estimates (5). The main human pathogenic species causing intestinal schistosomiasis are Schistosoma mansoni, Schistosoma japonicum, Schistosoma mekongi, Schistosoma intercalatum, and Schistosoma guineensis. with Schistosoma haematobium causing urogenital schistosomiasis. While S. haematobium is a major cause of mortality, frequently causing renal failure, chronic morbidity is the major health concern with schistosome infection causing 3.3 million disability-adjusted life years (6). For the purpose of this review we will concentrate on the most prevalent species causing intestinal schistosomiasis, S. mansoni.
S. mansoni is well-adapted to chronically infect humans as a result of ~200,000 years of co-evolution with modern humans (7). This is reflected by the life-span of S. mansoni worms estimated to be 5.7–10.5 years in human hosts (8). Evidently, successful adaptation has established a host-parasite relation such that asymptomatic infection are present in more than 90% of individuals, however, some infected develop hepatic fibrosis, severe hepatosplenomegaly, and portal hypertension (9). Immunopathology during schistosome infection of humans is predominately caused by granulomatous inflammation around parasite eggs that are trapped in various organs. In this review, we will focus on S. mansoni and the immune-dependent process of egg granuloma formation, which facilitates the parasite egg excretion from the mammalian host and completetion of the trematodes life cycle.
Life Cycle of Schistosoma spp.
Schistosoma species have complex life-cycles involving infection of a freshwater snail intermediate host as well as a mammalian definitive host, such as humans. The S. mansoni egg stages are excreted from the human host within fecal material (or urine in case of S. haematobium). Under optimal conditions the eggs hatch in fresh water and–via asexual replication in the intermediate snail host, Biomphalaria genus for S. mansoni–thousands of free-swimming infective cercariae are released into the water. The cercariae locate a mammalian host and penetrate the skin, and then transform to the now so-called schistosomulae stage. The schistosomulae remain in the skin for several days, after which they enter the circulation via the lymphatics and venules to reach the lung 5–7 days after skin penetration. After >2 weeks, they re-enter the circulation and reach the hepatoportal circulation. Here, they remain and sexually mature into adult male or females worms after encountering a partner of the opposite sex. The monogamous pair migrate to the mesenteric veins, mate and begin egg production after ~28 days. Adult S. mansoni worms are predominantly found in the small inferior mesenteric blood vessels that surround the colon and caecum. Eggs laid by female worms are deposited onto the endothelial lining of the capillary walls. From here, the eggs are either disseminated through the blood flow into other organs or they translocate through the intestinal epithelia into the intestinal lumen. The eggs are metabolically active and highly antigenic–they evoke inflammation that leads to the formation of a granuloma around the egg necessary for the translocation through the lamina propria. Excretion of eggs within the fecal material then completes the parasites life cycle.
Acute clinical symptoms may include the development of a light rash, commonly referred to as “swimmers itch.” “Katayama fever” is characterized by fever, fatigue, and dry cough–among other symptoms–and may occur 2–12 weeks after infection resulting from a systemic reaction against the migrating schistosomulae. During chronic stages of infection, half to two thirds of the eggs deposited in mesenteric venules are swept away in the circulation to multiple organs, with the majority ending up in the liver (10). In the liver, granulomatous inflammation around eggs and the subsequent fibrosis lead to the major pathologies associated with schistosomiasis mansoni. Fibrosis in the liver portal tract often leads to obstructive portal lesions and portal hypertension, and may result in gastrointestinal bleeding, hepatic encephalopathy and liver failure. Interestingly, despite the constant translocation of eggs from the vasculature into the intestinal lumen, cases of S. mansoni-associated sepsis are rare, reinforcing the highly adapted process of egg excretion (11).
Schistosome Animal Models are Required to Study The egg Excretion Process
Human studies are generally undertaken in endemic settings and commonly are based on observations before and after drug treatment for schistosomiasis. While analysis of human material (blood, tissue, stool) are definitely required to translate findings from animal experiments to the human system (12), these samples come with some caveats: heterogenic background, medical history, co-infections, environmental factors, etc. Perhaps these may be overcome someday by the growing computational power and the use of high throughput “omics.” More intriguingly, the ongoing studies involving controlled human infection with S. mansoni will provide new insight to most aspects of infection of humans (13). However, to formally address the egg excretion process in humans the deliberate experimental chronic infection with mixed sex cercariae, resulting in egg producing male and female worm infections and egg associated tissue immunopathology leading to morbidity and the risk of mortality, may pose ethical concerns. Alternatively, longitudinal studies in endemic areas are logistically demanding as they would require colonoscopy to access the intestinal epithelium.
Animal models have greatly advanced our understanding of the pathophysiology of schistosome infection. While chimpanzees and baboons are the most faithful models recapitulating all features of human schistosomiasis including peri-portal fibrosis and intestinal lesions (14–18), today the most frequently used species is the mouse, although not all findings are translatable. This suitability of mice as a model, must be considered in the context that S. mansoni may have adapted some 125,000 years ago to humans from the rodent trematode S. rodhaini (19). While the mouse shows differences in hepatic fibrosis and pathology, which is more associated with the granulomatous response to trapped parasite eggs in the liver and intestine (20), the development of granulomas adequately reflects human disease. As the process of egg excretion is dependent on the immune-dependent formation of granulomatous inflammation mice are a well-suited model to mechanistically study egg translocation, which is facilitated by the availability of reagents and transgenic mice ultimately guiding the studies on infected humans. In this review, we will rely mostly on data generated in mice as a model of S. mansoni infection and immunopathology.
Differences in Granulomatous Inflammation of Liver and Intestine
Immune Response to S. mansoni
Following infection, schistosomulae migrate through the host body and a type 1 immune dominated response is elicited, persisting for ~5 weeks. This response is characterized by increased release of Interleukin (IL)-12 and interferon (IFN)-γ and is mainly targeted at worm antigens. However, as the parasite matures and starts to produce eggs (~5–6 weeks postinfection), a shift toward a type 2-biased immune response occurs. Consequently, IFN-γ production decreases, while CD4+ T helper (Th) 2 cell polarization is induced. The switch from type 1 to type 2 is elicited by the eggs released by adult female worms. Indeed, whole S. mansoni eggs or their soluble egg antigens (SEA) potently induce type 2 responses when injected into naïve mice (21). Importantly, a defect in switching the type of immune response leads to aberrant intestinal inflammation and fatal disease (22, 23). The protective type 2 immune response is characterized by expansion of Th2 cells, eosinophils, and basophils, increased production of IL-4, IL-5, and IL-13, an isotype switch toward IgG1 and IgE, as well as polarization of macrophages toward the M2 phenotype (24, 25). During the chronic phase of infection (>3 months), the magnitude of the Th2 response decreases, coincident with a reduction in granulomatous inflammation around eggs, and regulatory T and B cells emerge leading to a state of immune hyporesponsiveness.
Granuloma formation around eggs trapped within hepatic and intestinal tissue is a hallmark of schistosome infection and the major cause of pathology in infected hosts. However, the egg granuloma functions for both the host and parasite (26): (a) intestinal granulomatous inflammation facilitates the translocation process for the egg into the gastrointestinal lumen, (b) it ameliorates bacterial translocation from the intestine into the circulation of the host, (c) it protects host tissues from exaggerated immune responses against the antigenic eggs, and (d) it ultimately benefits the adult parasites to keep the host intact.
Intestinal Granulomas
Female S. mansoni worms deposit their eggs close to the vasculature surrounding the intestines and exploit the host protective mechanism to facilitate egg transgression in order to get them transported through the endothelium and intestinal wall into the gut lumen. It has been estimated that S. mansoni eggs are viable for ~3 weeks indicating that eggs complete the transition through the intestinal wall well within that time frame but in no less than 6 days (27). Therefore, the intestinal granulomas will have characteristics of what is seen in early stage liver granulomas (Figure 1). Moreover, histological analysis has shown that colon granuloma composition differs from liver granulomas harboring more macrophages but less eosinophils, T and B cells (28). However, to date, a comprehensive in-depth characterization of the composition of the intestinal granuloma remains to be published. Due to the longevity of infection and constant egg deposition, assessing the temporal order of events during granuloma formation in the intestine is challenging. Recently, a novel approach has been published, in which S. mansoni eggs are surgically implanted into the sub-serosal tissue of small intestine or colon of mice (29), facilitating the direct and kinetic analysis of egg priming of the immune system in the intestine and within the local draining mesenteric lymph nodes.
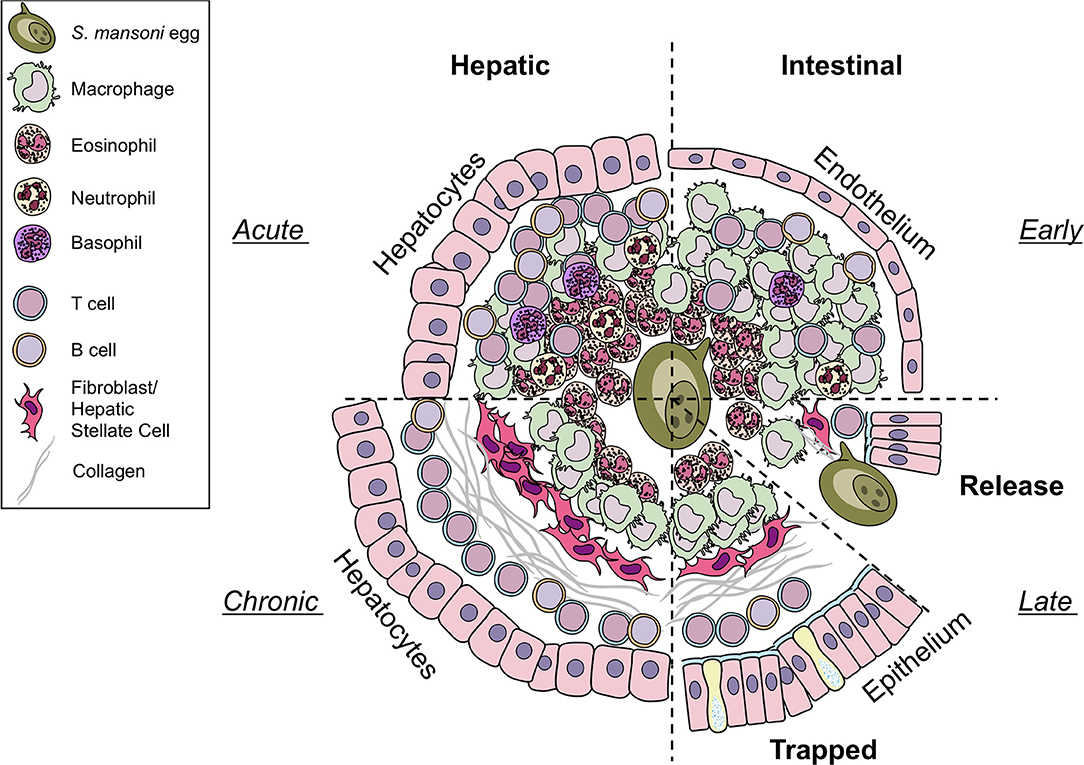
Figure 1. Differences in hepatic and intestinal granuloma composition. Cellular granuloma composition in the liver (left) and the intestine (right). While early (upper half) granulomas may appear similar, intestinal granulomas harbor less eosinophils, T cells, and B cells than hepatic granulomas, more macrophages are present. Only few neutrophils and basophils can be observed in both sites. During later stages (lower half) eggs in the liver become trapped and fibrosis develops. In contrast, eggs deposited in the gut must be released to the intestinal lumen by yet to define mechanisms. However, they may also become trapped and may resemble chronic liver granulomas in shape and collagen content. Illustrations modified from Servier Medical Art, licensed under a Creative Common Attribution 3.0 Unported License.
Hepatic Granulomas
The formation of granulomas around eggs disseminated into the liver is better understood (Figure 1). In contrast to intestinal granulomas, the liver granuloma cannot be shed and becomes fibrotic over time. Recently, a histological analysis of granulomas revealed that the majority of productive, collagen rich granulomas during natural and experimental S. mansoni infection develop in the liver, probably as eggs are trapped in the hepatic tissue as opposed to intestinal granulomas, which appear more organized and with fewer, circumferal collagen fibers (30). Interestingly, hepatic granuloma size decreases from weeks 8 to 20 post-infection, at which point it stabilizes for at least another 32 weeks (10). This down modulation of egg granuloma size reflects the hyporesponsive state of immunity that develops as the infection progresses to chronicity. While normal lobular liver architecture is retained, angiogenesis occurs and contributes both to the genesis of schistosomal portal fibrosis and to fibrotic degradation (31). Indeed, experimentally infected and treated mice showed significant remodeling of hepatic schistosomal lesions over time. A notable feature of the granuloma surrounding the the liver is its protective function to encapsulate hepatotoxic secretions from the egg (26)–in particular omega-1 (ω1) (32).
The Egg-Immune Interface
It is important to highlight that the egg is a live and biologically active organism that proactively interacts with the host to manipulate immunity and achieve its successful excretion from the host. The shell of the egg itself, aided by factors released from maturing eggs, likely contributes to the initial attachment of the egg to the endothelium. This activating process facilitates granuloma formation and ultimately egg excretion. Indeed, these egg secretions (ES) are the active interface between the egg and the host (33, 34). The egg shell and its ES bear a number of molecules with potential immunomodulatory (IM) activity. The two major secreted egg IM are described in more detail here as they may play an excretion-promoting role in the intestinal granuloma.
Alpha-1 (α1)/IPSE/smCKBP
Alpha-1 (α1) is a dimeric glycoprotein that was first described by Dunne and Doenhoff (35, 36). Haas and colleagues identified that native α1 induced IL-4 release from basophils and cloned the recombinant molecule; subsequently termed IL-4-inducing principle of S. mansoni eggs (IPSE) (37, 38). Furthermore, IPSE has a nuclear localization signal in the C-terminus that conveys “infiltrin” activity, an ability to infiltrate through the cell membrane and cytoplasm and translocate to the nucleus (39, 40). Importantly, IPSE/α1 further contributes to the enlargement of hepatic granulomas (41). In many pathogens, host subversion strategies utilize immunomodulators to interact with and manipulate chemokines and alter local cellular recruitment and activation–termed chemokine binding proteins (CKBP) (42). A screen of homogenates or ES from the S. mansoni life cycle stages that infect the mammalian hosts identified a CKBP (SmCKBP), within only SEA (~10 μg per mg), that was enriched in ES (~150 mg per mg). SmCKBP selectively bound certain chemokines and when recombinant SmCKBP was administered to mice, it blocked chemokine activity and inflammation (43). SmCKBP has an active role in granuloma formation around live eggs and is released from the maturing egg. This has been evidenced in vitro by circumoval precipitation and in vivo by detection within hepatic as well as intestinal granulomas (43) (Figures 2A,B). The vastly different biological properties of α1/IPSE/smCKBP–inducing release of IL-4, binding chemokines, infiltrin activity, and enlargement of granulomas–highlight the dynamism and duality of functions for helminth IM. Indeed, the immunomodulating activity of the molecule extends beyond helminths, with recombinant forms shown to suppress inflammation and pathology in models of skin inflammation as well-bladder disease (43, 45).
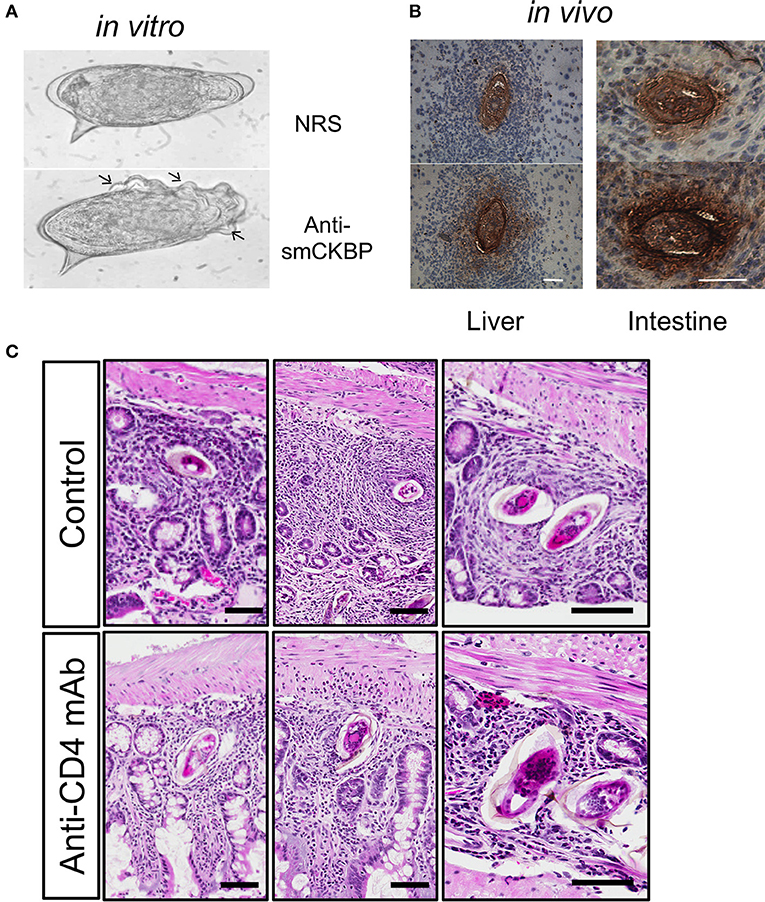
Figure 2. Release of smCKBP from S.mansoni live eggs under in vitro conditions and detection in vivo adjacent to eggs within granulomas in the liver and intestine of infected mice. (A) Live eggs were cultured in vitro with anti-smCKBP rabbit sera or normal rabbit sera (NRS), with SmCKBP-antibody precipitate formation (arrows) when cultured with anti-smCKBP sera. (B) Immohistochemistry detection of smCKBP (brown stain) within the granulomas surrounding eggs in liver or intestines of infected mice. Bar, 50 μm. 2015 Smith et al. Originally published in The Journal of Experimental Medicine. https://doi.org/10.1084/jem.20050955. (C) Smaller granulomas forming around eggs within intestines of S. mansoni-infected mice treated with anti-CD4 mAb compared to control mice, as described (44). H&E-stained sections. Bar, 100 μm.
Omega-1 (ω1)
The initial identification of Omega-1, a hepatotoxic egg glycoprotein, is also attributed to Dunne and Doenhoff (32, 36). ω1 possesses both T2 RNase activity (46) and potent Th2 inducing activity (47, 48). The ability of ω1 to prime dendritic cells to elicit Th2 cell expansion is mTOR-independent. Rather, it is dependent on ω1 RNase activity in addition to its native glycosylation, enabling the molecule to bind to the mannose receptor on dendritic cells and be internalized (49, 50). It is noteworthy that both α1 and ω1 within SEA are glycoproteins, with many functional activities of such egg molecules being glycan dependent, requiring interactions with selected C-type lectin receptors on immune cells (51–54).
Recombinant ω1 has been shown to have therapeutic activity in reducing the incidence and development of diabetes in NOD mice (55), modulating inflammasome-dependent IL-1β release (56) and inducing FoxP3 expression in CD4+ cell to drive Treg development (57, 58). Regarding metabolic effects, ω1 binds to mouse and human adipocytes and, in mouse models of diet-induced obesity, was shown to induce release of IL-33 resulting in weight loss (59). Further studies are required to investigate whether ω1-induced IL-33-release is responsible for DC modulation and Th2/Treg induction. These immunomodulatory effects may play an important role in regulating optimal granuloma formation and transition through the lamina propria.
Mechanism of “Eggs-Cretion”
While the S. mansoni egg excretion process still remains ill-defined to date, it can be broadly divided into four stages (Figure 3):
I Egg release into the bloodstream and attachment to the endothelium
II Immune-dependent granuloma formation
III Transition between endothelium and epithelium
IV Release into the intestinal lumen
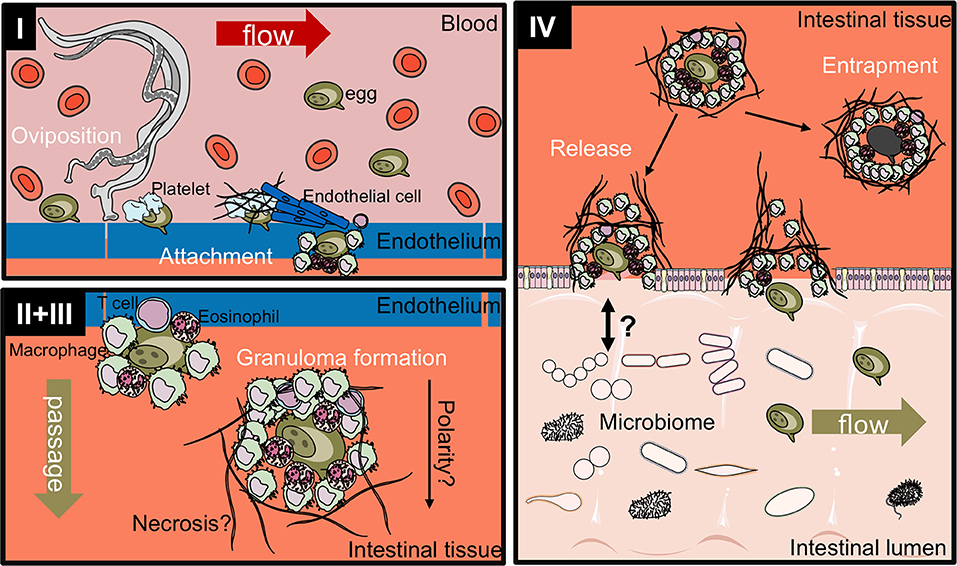
Figure 3. Proposed four-stage process of intestinal egg excretion. I: Adult schistosomes deposit eggs into the vasculature close to the lamina propria. Platelets and fibrinogen adhere to the eggs and activate the endothelium. Endothelial cells actively grow over the egg supporting its extravasation. Eggs that do not cross the endothelial border are disseminated by the blood flow and become trapped mostly in the liver portal system. II + III: Immune cells, such as macrophages, T cells and eosinophils start to encapsulate the egg. Granuloma formation occurs around the egg and together with other processes, such as fibrinolysis, egg secretions-induced necrosis, leads to the passage of the egg toward the intestinal lumen. IV: Entrapped eggs become fibrotic and calcified. Interaction with the microbiome, epithelial cell death and remodeling may lead to the active release of eggs, which are then released to the environment with host feces. Illustrations modified from Servier Medical Art, licensed under a Creative Common Attribution 3.0 Unported License.
Stage I. Egg Release Into the Bloodstream and Attachment to the Endothelium Is Triggered by Adult Worm and Egg Secretions
Within the mesenteric vasculature, adult male and female schistosomes reside. Here, they move against the blood toward the endothelium facing the intestinal epithelium as a response to a nutrient gradient. Female S. mansoni worms release roughly 300 eggs per day–equalling approx. one egg every 5 min–a rate that stays constant for at least 1 year (10). Although female worms flex backwards during oviposition (thereby releasing the egg in close proximity to the endothelium), active penetration of eggs through the endothelium is unlikely (34). The metabolic enzymes enolase (phosphopyruvate hydratase) and glyceraldehyde-3-P-dehydrogenase (GAPDH) have been identified as parts of the eggshell and act as surface receptors to bind plasminogen (60, 61) increasing the fibrinolytic activity of the egg. Further, lack of enolase has been shown to decrease binding of other pathogens to the endothelium (62, 63). Although both enolase and GAPDH are present in exosome-like vesicles (ELVs) produced by adult S. mansoni worms (61), it is not known if ELVs are also released from the egg stage. The release of such metabolic enzymes within the surrounding egg milieu induces a localized metabolic niche that modulates local cell functions to achieve egg excretion.
Indeed, human enolase has been shown to activate pulmonary endothelial cells and to increase surface expression of the cellular adhesion molecule ICAM-1 (64). It has been reported that endothelial cells actively migrate over freshly deposited eggs in vitro (65), a process enhanced in the presence of sera. Furthermore, eggs can bind to and activate platelets, which may subsequently activate endothelial cells (66). This process also seems to contribute to extravasation, with thrombocytopenia impairing egg excretion (67). Interestingly, schistosome eggs also bind other host plasma proteins, including von-Willebrand factor, which may assist in the initial attachment to the damaged or activated endothelium (68). Enolase, present in the egg shell, can also act as a plasminogen receptor and induce plasminogen activation and plasmin generation (69). Plasmin can subsequently contribute to blood clot lysis (important for adult worms) and may induce monocyte recruitment (70).
The initial recruitment of immune cells to the site of granuloma formation is dependent on cellular adhesion molecules, such as ICAM-1. ICAM-1 is upregulated by SEA and high expression can be observed within hepatic granulomas (71). Here, it is essential for granuloma formation, especially during early acute stages (72–74). Ileal and colonic granulomas also showed high expression of ICAM-1 as well as LFA-1 and VLA-4 in acute and chronic infection (75). In ICAM-1-deficient mice, VCAM-1 was upregulated in hepatic granulomas, whereas no expression of ICAM-2 or PeCAM was observed (71). Interestingly, close correlation has been identified between soluble ICAM-1 and fecal egg counts from infected patients feces (73) where it was proposed to function as a negative regulator by inhibiting leukocyte recruitment and downmodulation of granuloma formation.
It has been suggested that the presence of the characteristic lateral spine of the eggs may facilitate attachment to the endothelium, perhaps causing cell damage to elicit a danger signal and while intestinal peristalsis may also contribute to the extravasation process, its actual function remains unclear. However, a study published in 2013 showed that the location of egg deposition within the intestinal tract may contribute to efficient egress of eggs (76). S. mansoni eggs are significantly more abundant in areas with Peyer's patches, where egg secretions cause loss of cellularity facilitating transition. Indeed, in support of this, mice without Peyer's patches excrete fewer eggs (76).
Stage II. Immune-Dependent Granuloma Formation Is Driving Egg Excretion
The main mechanism that facilitates egg excretion is the formation of the granuloma around the egg. As most findings concerning granulomatous inflammation have been found in hepatic granulomas, the following events have to be treated with caution as experimental data for the formation of intestinal granulomas is largely missing.
It has been noted earlier that the process of S. mansoni egg excretion is an exquisite, immune-dependent process (Figure 3) as illustrated by significant reduction in fecal egg excretion in T cell- (44, 77, 78) and nude mice (79). Severe combined immunodeficient mice were almost incapable of passing parasite eggs in the first weeks of oviposition (80, 81). Indeed, S. mansoni-infected HIV+ patients with acquired immunodeficiency syndrome had fewer eggs in their feces than HIV seronegative patients with the same levels of S. mansoni infection (82, 83), which correlated with CD4 T cell counts after anti-retroviral therapy (84). However, the role for CD4+ cells in schistosome egg excretion of humans was not evident in studies on other HIV+ patient cohorts (85, 86).
Strikingly, mice deficient in the Th2-associated cytokines IL-4 and IL-13 passed almost no eggs in their feces (23). IL-4-deficient mice display significantly impaired granuloma formation and increased mortality associated with intestinal pathology (22). This is also observed in mice with a combined deficiency in IL-4 and IL-13 (23) which also develop fatal endotoxemia. Furthermore, IL-4-deficient mice show a significant accumulation of eggs in the intestinal wall, a finding supportive of the function of the granuloma in facilitating egg excretion (23). Similarly, mice with specific deficiency in IL-4 and IL-13 within T cells succumb to acute S. mansoni infection (25). Also, IL-4Rα−/− mice may develop fatal hemorrhaging following S. mansoni indicative of a protective role of the granuloma from exacerbated immunopathology in the intestine (87). IL-4Rα-signaling involves both IL-4- and IL-13-mediated receptor engagement and the particular role of IL-13 was revealed to drive hepatic fibrosis (23, 88).
While there has been a focus on T cells, other immune cells also contribute to both granuloma formation and the excretion of eggs. Indeed, eosinophils are a major constituent of intestinal granulomas (89). Although their precise role remains unclear, it has been reported that eosinophils may promote egg excretion (90). However, studies using anti-IL-5 antibody-mediated eosinophil blockade found normal numbers of eggs passed in the feces (91, 92), with no marked alterations in hepatic granuloma formation noted in transgenic mouse models of eosinophil deficiency (93). Another possible function of granuloma eosinophils may be the destruction of miracidia within the surrounded (trapped) egg (94), in part explaining the higher abundance of eosinophils in hepatic granulomas (28, 30) and the occurrence of eosinophil pyroptosis in vivo (95).
Basophils can be directly activated by IPSE/α1 to release IL-4 (38) and are present in intestinal granulomas (96). Additionally, a new molecular mechanism of action has been revealed whereby IPSE, a member of the βγ-crystallin superfamily, can bind IgE through the IPSEs crystallin fold thereby activating basophils independently of formal IgE cross-linking (97). Although basophils have been shown to drive Th2 polarization in other helminth infection models (98, 99), whether or not they contribute to intestinal granuloma formation and egg excretion is unclear to this date (25, 100).
While neutrophils are found to acquire a pro-inflammatory phenotype during S. japonicum infection (101), they do not seem to participate in the granuloma formation in S. mansoni infection (87), which may be in part due the presence of the kunitz-type protease inhibitor SmKI-1, capable of inhibiting neutrophil function (102).
The more recently described group of innate lymphoid cells (ILC) have gained a lot of attention as they are capable of initiating type 2 immune responses against certain helminths and during allergic responses (group 2), maintaining intestinal homeostasis (group 3), and enhancing type 1 responses via IFN-γ release [group 1; reviewed in (103, 104)]. To date, only limited data is available on the contribution of ILC, and in particular ILC2, during S. mansoni infection toward granuloma formation and egg excretion. In a pulmonary model of schistosome egg injection we have shown that ILC2 can contribute to pulmonary fibrosis (105). Because ILC2 are abundant in the intestine and they can directly interact with CD4 T cells to enhance polarization toward Th2, they may play non-redundant roles in the excretion process by instructing T cell responses. ILC2 are mainly activated by the alarmin cytokines IL-33, IL-25, and TSLP to produce IL-5 and IL-13. While individual ablation of each of these cytokines did not impair hepatic granuloma formation in chronic schistosomiasis, the combinatorial targeting of all three cytokines led to reduced hepatic fibrosis, impaired eosinophil recruitment and fewer numbers of IL-13-producing ILC2 (106). Thus, while the impact on early intestinal granuloma formation and egg excretion remains obscure, the marked effect of alarmins is highly likely to impact on intestinal granuloma formation. Omega-1 released from eggs within the intestines may–in addition to cytotoxic effects–increase the release of IL-33 and therefore the activation of ILC2. Additionally, ILC3 might exert similar functions as they have been shown to be involved in the maintenance of tolerance toward commensal microbiota through the interaction with CD4 T cells in the gut (107). Interestingly, altered numbers of ILC2 have been detected in the circulation of S. haematobium-infected patients (108). However, further investigation is required to fully elucidate the role of ILC populations in schistosoma infection and granulomatous inflammation.
Dendritic cells (DCs) are capable of processing and presenting schistosome egg antigens (109) and depletion of DCs during an ongoing S. mansoni infection severely disrupts the generation of the Th2-polarized immune response (110). While hepatic conventional DCs act in an immunogenic, rather than tolerogenic, capacity, their function in the intestine may be different (111). Using an injection model for the direct delivery of schistosome eggs into the subserosal intestinal tissue revealed that IRF4-expressing CD11b+ DCs promote Th2 responses in the intestine (29). It further has been shown that Omega-1 is the major component conditioning DCs for Th2 polarization (48).
Macrophages are the most abundant cell population in intestinal granulomas (28). During S. mansoni infection of immunocompetent mice macrophages acquire a protective alternatively activated phenotype through IL-4- and IL-13-mediated IL-4Rα-signaling. The M2 phenotype is associated with the increased expression of Arginase-1, an enzyme converting L-arginine to L-orthinine, which is further converted to proline–a critical amino acid for the production of collagen, and therefore, the development of fibrosis (112). While this process is detrimental to host survival through fibrotic liver damage, it might not be as critical in the intestinal granulomatous response as the surrounding granulomas are overall more temporarily restricted. Therefore, other properties of IL-4Rα-mediated M2-polarized macrophages, such as IL-10 production, PD-L2 and Relmα expression, may be more crucial to prevent aberrant inflammation.
When macrophages are unable to respond to IL-4 and IL-13 cytokine signals in IL4RαLysMCre mice the hosts succumb to endotoxemia, which can be in part rescued by antibiotic treatment (87). More inflammatory cells were observed surrounding intestinal granulomas suggesting that M2 macrophages are specifically required to prevent excessive damage to the intestinal wall and promote the efficient transport of eggs into the intestinal lumen. In contrast, Arginase-1-expressing macrophages were shown to limit Th2 responses (113) and prevent the formation of exacerbated granulomas in the liver. Later, it was found that Lyz2lo macrophages were able to escape LysMCre-mediated IL-4Rα-deletion and acquire M2 properties in response to S. mansoni egg injection, which may account for some of the observed differences between studies (114). It was further shown that during S. mansoni infection macrophages require IL-4/IL-13 released from Th2 cells to acquire an M2 phenotype (25). Importantly, granuloma formation was significantly impaired in mice with T cell-derived IL-4/IL-13-deficiency and egg excretion in the feces was compromised, although it did not reach statistical significance. These results suggest that while M2 macrophages contribute to the increased fibrosis surrounding the trapped eggs in the liver, they also promote efficient shielding of the eggs in their transition through the intestinal tissue in order to prevent excessive damage. Macrophage phenotype and granuloma formation is ultimately determined by Th cell polarization.
T cells are the most important cells for successful egg excretion in the intestine. Antibody-mediated depletion of T cells was shown decades ago to lead to impaired granuloma formation, egg retention and exacerbated disease resulting in increased mortality (78). Later targeting of L3T4 (CD4) T cells phenocopied anti-CD3 mediated depletion highlighting the role of the T helper subset (115). In S. mansoni infected mice subjected to anti-CD4 mAb-depletion the size of the granuloma is significantly reduced in liver and intestines [Figure 2C, (116)]. Furthermore, the cellular granuloma composition in CD4-depleted mice consists of fewer eosinophils and more neutrophils, which are virtually absent in the eosinophil-rich granulomas of immunocompetent mice. Similarly, the absence of T cell-derived IL-4/IL-13, e.g., the functional absence of Th2 cells, impairs granuloma formation and egg excretion, and was found to increase mortality (25).
The critical role of Th2 cells for the protection of host tissue from cytotoxic effects and correct granuloma formation is further substantiated by studies showing that injection of the Th1 cytokine IFNγ interferes with granuloma formation and IL-12- deficiency–in fact Th1-ablation–increases granuloma size and Th2-mediated inflammation (117, 118). Although Th1 cells seem to be required for the early release of IFNγ and IL-2 facilitating granuloma formation as part of a delayed type hypersensitivity response, prolongation of the Th1 response led to increased pathology and mortality (44, 117, 119, 120). Furthermore, it was shown that hepatosplenic schistosomiasis in human patients is associated with increased levels of TNFα and IFNγ, while type 2 cytokines are reduced (121).
IL-17-producing Th17 cells have also been implicated in schistosome granuloma formation as IL-17 levels in susceptible and resistant mouse strains correlated with granuloma formation (122). This has been shown to be a direct effect on the IL-23 and IL-1 release of DCs in response to SEA (123, 124). In the context of the roles of egg glycans in immune priming, theses pathological effects are mediated through the C-type lectin receptor CD209a (125).
In the absence of Th1 cells (Tbet−/−) Th17 cells drive exaggerated inflammation with an increase of neutrophil infiltration into the granuloma, while the combined absence of Th1 and Th17 cells led to smaller granulomas with increased type 2 biased infiltration of M2 macrophages and eosinophils (126).
Regulatory T cells expand during the chronic stage of S. mansoni murine infection and are present in S. mansoni infected patients (127–129). It has speculated that immunosuppression by Treg cell by the release of IL-10 and TGFβ may limit tissue pathology toward trapped eggs during infection (130–132).
The role of B cells and antibodies in S. mansoni infection of mice has been extensively investigated. While B cells were found to be involved in the development of Th2 polarization, their role in granuloma formation is still not entirely clear (133–135). Antibodies specific for SEA arise after egg deposition and expand throughout infection (136) and multiple studies have shown regulatory roles of antibodies in granuloma formation (137–139). Interestingly, immune complexes of chronically infected patients were capable to inhibit granuloma formation in vitro (140). Indeed, infection of Fc-receptor deficient mice (γ or ε) led to the formation of larger and collagen-rich granulomas (138, 139), suggesting regulatory potential of Fc-bearing effector cells within the granuloma or sequestration of egg antigens. Whether this process is involved in the maintenance of intestinal granulomas remains to be investigated.
Identification of regulatory B (Breg) cells in response to schistosome eggs (141) and their production of IL-10 in response to the schistosome glycan LNFPIII (142, 143) led to subsequent studies using live S. mansoni infections (144–146). Indeed, α1 drives the expansion of Breg cells (147). Because Bregs arise late during chronic infection it seems unlikely that they contribute to the natural intestinal granuloma formation facilitating egg excretion and rather may contribute to limit chronic intestinal inflammation.
The functions of the tuft cell as IL-4Rα-expressing IL-13-responsive cell type in the intestine has been described during gastrointestinal nematode infections (148–150). Whether these cells contribute to the intestinal granuloma formation by their IL-25 release or facilitate the egg excretion process during S. mansoni infection has not been investigated.
Taken together, egg excretion through the intestinal wall can only be achieved in the presence of a Th2-biased granulomatous inflammation. All cells described here take part in the shaping of the intestinal granuloma and may therefore influence Th2 polarization, toxicity protection and promote or inhibit egg excretion. While the roles of CD4+ T cells and macrophages is well-understood, more research is required focusing on other immune cells function in intestinal granulomas.
Yet, the question remains: What drives the egg+granuloma to transit from the endothelium toward the epithelium?
Stage III. Transition Through the Lamina Propria Is Achieved by Toxic Effects and Displacement
The mechanism of how the viable egg transits through the intestinal lamina remains elusive, however, some findings suggest a role for the developing granuloma, extracellular matrix degradation, and schistosome egg antigens.
Recently, the collagen, fibronectin and plasmin contents of intestinal granulomas have been investigated (151). After extravasation, fibrinolysis can be observed around the biologically successful eggs, i.e., those that will achieve egg excretion, in contrast to trapped eggs that are not excreted (Figure 3). Plasma fibronectin is part of the blood clotting and wound healing response and it was recently found that S. mansoni is able to express extracellular tegumental calpains that cleave fibronectin (152). Fibronectin was also found in early granulomas, while chronic granulomas are collagen-rich (30, 153). Eggs also express SmCalp1 and may actively contribute to the fibronectin degradation (152). Further, SmEnolase, is highly expressed by eggs, and is able to promote plasminogen activation (69). Plasmin degrades fibrin and fibronectin–among other targets–and thereby contributes to the degradation of extracellular matrix proteins. Indeed, a striking difference exists between hepatic and intestinal granulomas with regard to perioval fibrin and fibronectin deposition (151). While they were found in 23 and 77% in hepatic granulomas, respectively, only 3 and 11% were positive in the intestine. Viable eggs actively degrade fibrin and fibronectin, therefore the increase of these ECM components in hepatic eggs might stem from the increased proportion of dead eggs. However, as the data set is limited, in-depth investigation of the plasmin, fibrin, fibronection, and collagen content of intestinal granulomas is warranted. Fibrinolysis and plasmin around eggs could be a result of macrophage invasion or proteolytic activity of the eggs. At the moment, it remains unclear what the exact order of events in fibrin and collagen deposition, plasminogen activation and fibrinolysis is and thus a more detailed investigation is required.
While collagen deposition leads to immunopathology in the liver, collagen degradation may be more important in intestinal granulomas. Eight weeks after infection the collagen content in hepatic granulomas is already significantly increased but only minimal deposition is observed in the small intestine and even less in the colon (154). While collagen content increased over time in all three organs, in intestinal granulomas collagen marks chronic granulomas–and therefore trapped eggs (153). Furthermore, collagen structure appeared concentric in the liver while a discontinuous deposition was observed in the intestine (154). While eggs themselves were not able to degrade collagen in vitro (155), egg-activated plasmin may contribute to collagen degradation. Furthermore, M2-like macrophages can degrade collagen in a mannose receptor-dependent manner (156). Additionlly, many matrix metalloproteinases are expressed during S. mansoni infection with elevated expression of MMP-2, MMP-3, and MMP-8 transcripts in the colon of chronically infected mice (157), all of which possess collagenase activity.
While this array of ECM-degrading mechanisms may promote the movement through the lamina propria–but where does directionality come from? One possibility is that the influx of immune cells follows the egg through the initial crossing of the endothelium. Therefore, the granuloma first develops basally at the egg. The first cells may shield from the cytotoxic effects of the SEA, while SmEnolase and calpains exert their effects apically and degrade the ECM in front of the egg. Over time, macrophages will surround the egg and become activated by IL-4 and SEA to promote collagen degradation. It was shown that excretion is a relatively quick process compared to the development of liver fibrosis (158), therefore collagen deposition may occur only at the rim or basally of the granuloma to maintain the surrounding tissue architecture, while the apical ECM is degraded and more cells and fluid infiltrates from the basal side displacing the egg forward toward the epithelium. Simultaneously, cells around the egg succumb to the cytotoxic effects of IPSE/α1 and ω1. If the cells infiltrate from the basal side of the granuloma the cells at the apical side are more exposed to the cytotoxic molecules and therefore the first to die from necrosis. Further studies using intravital microscopy approaches are required to get more insight into the mechanisms by which the granulomas move the egg through the intestinal wall.
Another possibility is that because eggs are more or less deposited at the same site in the vasculature and often more than one egg is found in a granuloma, the intestinal tissue may develop an ill-defined “tunnel” structure with more collagen, fibrin and fibronectin deposition at the “tunnel wall,” while the inner tissue has a less rigid composition from repeated ECM degradation. Peristaltic movement of the bowel may also play a supportive role in moving the egg+granuloma along the beaten path. However, to date there is no experimental data available to support this theory, mainly because the larger granulomas surrounding unsuccessful eggs trapped in the intestinal wall may gain more attention and are more similar to what we expect from the research on hepatic granulomas than smaller successful egg granulomas.
Stage IV. Release Into the Intestinal Lumen
The process of actual release of the egg after transit through the intestinal wall into the gastrointestinal lumen has not–to our knowledge–been addressed in detail experimentally. The parasite eggs that are found in the feces of infected humans, as well as experimental mice, are devoid of an encapsulating granuloma suggesting that the egg leaves the granuloma as it enters the fecal matter. This poses the questions: How does it leave the granuloma? What happens to the remaining granulomatous tissue in the intestinal epithelia? A possible mechanism of granuloma resolution may be interaction of microbiota and the immune cells at the outer rim of the granuloma that come in direct contact with the mucous layer and get successively destroyed concomitant to the cytotoxic and fibrinolytic effects mediated by the egg and its secretions. Whether the composition of the mucus layer affects the survival of schistosome eggs is currently unclear. After the egg is released the remaining cells in the granuloma will likely be resolved as part of the intestinal wound healing response, where the already present macrophages may contribute to (Figure 3). Clinically, the colonic mucosa becomes atrophic and acquires a granular yellowish appearance (159), which are probably macrophages from the granulomas shed with tissue remodeling.
One complication observed in patients is the occurrence of colon polyps that are shed and cause intestinal bleeding (160). Probably this may happen when unsuccessful trapped eggs become calcified, losing their fibrinolytic potential and leading to chronic granulomas as seen in the liver.
Microbiome Influences on Egg Excretion
In recent years it became increasingly understood that the microbiome composition will impact on the outcome of most immune responses (161) and perturbations of this complex system may lead to detrimental consequences for the hosts health (162–164). A significant alteration of the microbial communities that impacts on the hosts immune response has been shown for gastrointestinal helminth infections with Heligmosomoides polygyrus (165), Trichuris muris (166), and Trichinella spiralis (167).
With respect to S. mansoni infection one study found that the absence of gut microbiota through the administration of antibiotics alters the immune response against S. mansoni (168). Further, intestinal inflammation was significantly reduced in antibiotics treated mice and fecal egg secretion was impaired. As the microbial translocation in immunocompromised mice will only occur after the first eggs have traversed the intestine–or at least entered it to compromise the integrity of the gut barrier–the importance of the microbiome composition for egg secretion itself becomes evident. In infected humans, while one study failed to find statistically significant differences (albeit a relatively small cohort size) between the microbiome composition of non-infected, S. mansoni-infected and praziquantel-treated children (169), it has to be noted that another study found significant differences in S. heamatobium-infected children (170).
For future studies it would be interesting to infect germ free or gnotobiotic mice to further investigate the impact of the microbiome during schistosome infection on the immune response or to comprehensively analyze the microbiome composition of infected mice and perform fecal transplantation experiments.
Conclusion and Future Directions
Research over the past decades has uncovered many fascinating facets of Schistosoma mansoni biology and the host immune response against both worms and eggs. The immunology of egg granuloma formation has been extensively investigated due to its central role in S. mansoni infection-associated immunopathologies. We previously posited on helminth immunobiology that despite such discoveries, there are many unknown unknowns yet to be not only identified but also to be experimentally investigated (171). Indeed, the processes involved in the granulomas evolutionary role in facilitating egg transit through the intestinal wall is still relatively unclear. With more sophisticated approaches, lessons learned from studying the hepatic granulomas, and ever more transgenic mouse models together with translational studies, the mechanism of egg transit may finally be unveiled.
Novel techniques, such as the injection directly into the epithelium will greatly improve our understanding of the intimate processes happening in the formation of a functional egg granuloma within the intestine (29). However, the initial interaction of the egg with the immune system–when it is deposited in the blood stream–will not be accessible by this system. High-resolution ultrasonography has been recently shown to be applicable for noninvasive time-course observations of hepatic lesions in S. japonicum infection in mice (172). Furthermore, the development of other noninvasive techniques, such as small-animal PET-MRI or intravital 2-photon microscopy may be helpful tools to study early events of granuloma formation, as undertaken in the liver (173). Furthermore, the manipulation of S. mansoni eggs by lentiviral transduction, as it has been shown for ω-1 knockdown (174), will make noninvasive observation techniques more powerful. Identification of immune-modulation egg products has also been facilitated by the fact that the cost and availability of core facilities for transcriptome sequencing became feasible (175) and “omics” data is made available at WormBase ParaSite (176). Using the lentiviral knockdown model or schistosome egg-antigen coated beads (177, 178) either in vivo or in in vitro models, such as intestine-on-a-chip (179), intestinal organoid cultures (180), or other novel models (181, 182), are potential approaches to provide new insights.
Future studies on this unique S. mansoni immune dependent “eggs-cretion” process will reveal novel insights on the host-pathogen-interface that will impact on our understanding of fundamental functions of the immune system in both health and disease.
Author Contributions
Both authors have made substantial, direct and intellectual contributions to the work, and approved it for publication.
Conflict of Interest Statement
The authors declare that the research was conducted in the absence of any commercial or financial relationships that could be construed as a potential conflict of interest.
Acknowledgments
CS is a EMBO Long-term fellowship holder (ALTF 587-2016). PF helminth research has received support from Science Foundation Ireland and the Wellcome Trust. We are grateful to Ciara Byrne and Joseph Roberts for their critical reading of the manuscript.
References
1. Vos T, Flaxman AD, Naghavi M, Lozano R, Michaud C, Ezzati M, et al. Years lived with disability (YLDs) for 1160 sequelae of 289 diseases and injuries 1990–2010: a systematic analysis for the Global Burden of Disease Study 2010. Lancet (2012) 380:2163–96. doi: 10.1016/S0140-6736(12)61729-2
2. Who_Fact_Sheet WHO. Schistosomiasis Fact Sheet (2018). Available online at: http://www.who.int/news-room/fact-sheets/detail/schistosomiasis (Accessed June 27, 2018).
3. Berry A, Mone H, Iriart X, Mouahid G, Aboo O, Boissier J, et al. Schistosomiasis haematobium, Corsica, France. Emerg Infect Dis. (2014) 20:1595–7. doi: 10.3201/eid2009.140928
4. Berry A, Fillaux J, Martin-Blondel G, Boissier J, Iriart X, Marchou B, et al. Evidence for a permanent presence of schistosomiasis in Corsica, France, 2015. Euro Surveill. (2016) 21. doi: 10.2807/1560-7917.ES.2016.21.1.30100
5. Colley DG, Andros TS, Campbell CH Jr. Schistosomiasis is more prevalent than previously thought: what does it mean for public health goals, policies, strategies, guidelines and intervention programs? Infect Dis Poverty (2017) 6:63. doi: 10.1186/s40249-017-0275-5
6. Gahi GAOHI,. Global burden. London School of Hygiene Tropical Medicine (2018). Available online at: http://www.thiswormyworld.org/worms/global-burden (Accessed June 27, 2018).
7. Crellen T, Allan F, David S, Durrant C, Huckvale T, Holroyd N, et al. Whole genome resequencing of the human parasite Schistosoma mansoni reveals population history and effects of selection. Sci Rep. (2016) 6:20954. doi: 10.1038/srep20954
8. Fulford AJ, Butterworth AE, Ouma JH, Sturrock RF. A statistical approach to schistosome population dynamics and estimation of the life-span of Schistosoma mansoni in man. Parasitology (1995) 110(Pt 3):307–16. doi: 10.1017/S0031182000080896
9. Colley DG, Bustinduy AL, Secor WE, King CH. Human schistosomiasis. Lancet (2014) 383:2253–64. doi: 10.1016/S0140-6736(13)61949-2
10. Cheever AW, Macedonia JG, Mosimann JE, Cheever EA. Kinetics of egg production and egg excretion by Schistosoma mansoni and S. japonicum in mice infected with a single pair of worms. Am J Trop Med Hyg. (1994) 50:281–95. doi: 10.4269/ajtmh.1994.50.281
11. Onguru D, Liang Y, Griffith Q, Nikolajczyk B, Mwinzi P, Ganley-Leal L. Human schistosomiasis is associated with endotoxemia and toll-like receptor 2- and 4-bearing B cells. Am J Trop Med Hyg. (2011) 84:321–4. doi: 10.4269/ajtmh.2011.10-0397
12. Colley DG, Secor WE. Immunology of human schistosomiasis. Parasite Immunol. (2014) 36:347–57. doi: 10.1111/pim.12087
13. Janse JJ, Langenberg MCC, Kos-Van Oosterhoud J, Ozir-Fazalalikhan A, Brienen EAT, Winkel BMF, et al. Establishing the production of male Schistosoma mansoni cercariae for a controlled human infection model. J Infect Dis. (2018) 218:1142–6. doi: 10.1093/infdis/jiy275
14. Sadun EH, Von Lichtenberg F, Cheever AW, Erickson DG. Schistosomiasis mansoni in the chimpanzee. The natural history of chronic infections after single and multiple exposures. Am J Trop Med Hyg. (1970) 19:258–77. doi: 10.4269/ajtmh.1970.19.258
15. Abe K, Kagei N, Teramura Y, Ejima H. Hepatocellular carcinoma associated with chronic Schistosoma mansoni infection in a chimpanzee. J Med Primatol. (1993) 22:237–9.
16. Yole DS, Pemberton R, Reid GD, Wilson RA. Protective immunity to Schistosoma mansoni induced in the olive baboon Papio anubis by the irradiated cercaria vaccine. Parasitology (1996) 112(Pt 1):37–46. doi: 10.1017/S0031182000065057
17. Nyindo M, Farah IO. The baboon as a non-human primate model of human schistosome infection. Parasitol Today (1999) 15:478–82. doi: 10.1016/S0169-4758(99)01569-0
18. Farah IO, Mola PW, Kariuki TM, Nyindo M, Blanton RE, King CL. Repeated exposure induces periportal fibrosis in Schistosoma mansoni-infected baboons: role of TGF-beta and IL-4. J Immunol. (2000) 164:5337–43. doi: 10.4049/jimmunol.164.10.5337
19. Houldcroft CJ, Ramond JB, Rifkin RF, Underdown SJ. Migrating microbes: what pathogens can tell us about population movements and human evolution. Ann Hum Biol. (2017) 44:397–407. doi: 10.1080/03014460.2017.1325515
20. Fallon PG. Immunopathology of schistosomiasis: a cautionary tale of mice and men. Immunol Today (2000) 21:29–35. doi: 10.1016/S0167-5699(99)01551-0
21. Vella AT, Hulsebosch MD, Pearce EJ. Schistosoma mansoni eggs induce antigen-responsive CD44-hi T helper 2 cells and IL-4-secreting CD44-lo cells. Potential for T helper 2 subset differentiation is evident at the precursor level. J Immunol. (1992) 149:1714–22.
22. Brunet LR, Finkelman FD, Cheever AW, Kopf MA, Pearce EJ. IL-4 protects against TNF-alpha-mediated cachexia and death during acute schistosomiasis. J Immunol. (1997) 159:777–85.
23. Fallon PG, Richardson EJ, Mckenzie GJ, Mckenzie AN. Schistosome infection of transgenic mice defines distinct and contrasting pathogenic roles for IL-4 and IL-13: IL-13 is a profibrotic agent. J Immunol. (2000) 164:2585–91. doi: 10.4049/jimmunol.164.5.2585
24. Pearce EJ, Macdonald AS. The immunobiology of schistosomiasis. Nat Rev Immunol. (2002) 2:499–511. doi: 10.1038/nri843
25. Schwartz C, Oeser K, Prazeres Da Costa C, Layland LE, Voehringer D. T cell-derived IL-4/IL-13 protects mice against fatal Schistosoma mansoni infection independently of basophils. J Immunol. (2014) 193:3590–9. doi: 10.4049/jimmunol.1401155
26. Hams E, Aviello G, Fallon PG. The schistosoma granuloma: friend or foe? Front Immunol. (2013) 4:89. doi: 10.3389/fimmu.2013.00089
27. Jourdane J, Theron A. Larval development: eggs to cercariae. In: Rollinson D, Simpson AJG, editors. The Biology of Schistosomes: From Genes to Latrines, New York, NY: Academic Press (1987).
28. Weinstock JV, Boros DL. Organ-dependent differences in composition and function observed in hepatic and intestinal granulomas isolated from mice with Schistosomiasis mansoni. J Immunol. (1983) 130:418–22.
29. Mayer JU, Demiri M, Agace WW, Macdonald AS, Svensson-Frej M, Milling SW. Different populations of CD11b(+) dendritic cells drive Th2 responses in the small intestine and colon. Nat Commun. (2017) 8:15820. doi: 10.1038/ncomms15820
30. Amaral KB, Silva TP, Dias FF, Malta KK, Rosa FM, Costa-Neto SF, et al. Histological assessment of granulomas in natural and experimental Schistosoma mansoni infections using whole slide imaging. PLoS ONE (2017) 12:e0184696. doi: 10.1371/journal.pone.0184696
31. Andrade ZA, Santana TS. Angiogenesis and schistosomiasis. Mem Inst Oswaldo Cruz (2010) 105:436–9. doi: 10.1590/S0074-02762010000400013
32. Dunne DW, Lucas S, Bickle Q, Pearson S, Madgwick L, Bain J, et al. Identification and partial purification of an antigen (omega 1) from Schistosoma mansoni eggs which is putatively hepatotoxic in T-cell deprived mice. Trans R Soc Trop Med Hyg. (1981) 75:54–71. doi: 10.1016/0035-9203(81)90013-4
33. Ashton PD, Harrop R, Shah B, Wilson RA. The schistosome egg: development and secretions. Parasitology (2001) 122:329–38. doi: 10.1017/S0031182001007351
34. Dewalick S, Tielens AG, Van Hellemond JJ. Schistosoma mansoni: the egg, biosynthesis of the shell and interaction with the host. Exp Parasitol. (2012) 132:7–13. doi: 10.1016/j.exppara.2011.07.018
35. Dunne DW, Bain J, Lillywhite J, Doenhoff MJ. The stage-, strain- and species-specificity of a Schistosoma mansoni egg antigen fraction (CEF6) with serodiagnostic potential. Trans R Soc Trop Med Hyg. (1984) 78:460–70. doi: 10.1016/0035-9203(84)90061-0
36. Dunne DW, Jones FM, Doenhoff MJ. The purification, characterization, serological activity and hepatotoxic properties of two cationic glycoproteins (alpha 1 and omega 1) from Schistosoma mansoni eggs. Parasitology (1991) 103(Pt 2):225–36. doi: 10.1017/S0031182000059503
37. Wuhrer M, Balog CI, Catalina MI, Jones FM, Schramm G, Haas H, et al. IPSE/alpha-1, a major secretory glycoprotein antigen from schistosome eggs, expresses the Lewis X motif on core-difucosylated N-glycans. FEBS J. (2006) 273:2276–92. doi: 10.1111/j.1742-4658.2006.05242.x
38. Schramm G, Mohrs K, Wodrich M, Doenhoff MJ, Pearce EJ, Haas H, et al. Cutting edge: IPSE/alpha-1, a glycoprotein from Schistosoma mansoni eggs, induces IgE-dependent, antigen-independent IL-4 production by murine basophils in vivo. J Immunol. (2007) 178:6023–7. doi: 10.4049/jimmunol.178.10.6023
39. Kaur I, Schramm G, Everts B, Scholzen T, Kindle KB, Beetz C, et al. Interleukin-4-inducing principle from Schistosoma mansoni eggs contains a functional C-terminal nuclear localization signal necessary for nuclear translocation in mammalian cells but not for its uptake. Infect Immun. (2011) 79:1779–88. doi: 10.1128/IAI.01048-10
40. Pennington LF, Alouffi A, Mbanefo EC, Ray D, Heery DM, Jardetzky TS, et al. H-IPSE is a pathogen-secreted host nucleus infiltrating protein (infiltrin) expressed exclusively by the Schistosoma haematobium egg stage. Infect Immun. (2017) 85:e00301–17. doi: 10.1128/IAI.00301-17
41. Fahel JS, Macedo GC, Pinheiro CS, Caliari MV, Oliveira SC. IPSE/alpha-1 of Schistosoma mansoni egg induces enlargement of granuloma but does not alter Th2 balance after infection. Parasite Immunol. (2010) 32:345–53. doi: 10.1111/j.1365-3024.2009.01192.x
42. Fallon PG, Alcami A. Pathogen-derived immunomodulatory molecules: future immunotherapeutics? Trends Immunol. (2006) 27:470–6. doi: 10.1016/j.it.2006.08.002
43. Smith P, Fallon RE, Mangan NE, Walsh CM, Saraiva M, Sayers JR, et al. Schistosoma mansoni secretes a chemokine binding protein with antiinflammatory activity. J Exp Med. (2005) 202:1319–25. doi: 10.1084/jem.20050955
44. Fallon PG, Richardson EJ, Smith P, Dunne DW. Elevated type 1, diminished type 2 cytokines and impaired antibody response are associated with hepatotoxicity and mortalities during Schistosoma mansoni infection of CD4-depleted mice. Eur J Immunol. (2000) 30:470–80. doi: 10.1002/1521-4141(200002)30:2<470::AID-IMMU470>3.0.CO;2-T
45. Mbanefo EC, Le L, Pennington LF, Odegaard JI, Jardetzky TS, Alouffi A, et al. Therapeutic exploitation of IPSE, a urogenital parasite-derived host modulatory protein, for chemotherapy-induced hemorrhagic cystitis. FASEB J. (2018) 32:4408–19. doi: 10.1096/fj.201701415R
46. Fitzsimmons CM, Schramm G, Jones FM, Chalmers IW, Hoffmann KF, Grevelding CG, et al. Molecular characterization of omega-1: a hepatotoxic ribonuclease from Schistosoma mansoni eggs. Mol Biochem Parasitol. (2005) 144:123–7. doi: 10.1016/j.molbiopara.2005.08.003
47. Everts B, Perona-Wright G, Smits HH, Hokke CH, Van Der Ham AJ, Fitzsimmons CM, et al. Omega-1, a glycoprotein secreted by Schistosoma mansoni eggs, drives Th2 responses. J Exp Med. (2009) 206:1673–80. doi: 10.1084/jem.20082460
48. Steinfelder S, Andersen JF, Cannons JL, Feng CG, Joshi M, Dwyer D, et al. The major component in schistosome eggs responsible for conditioning dendritic cells for Th2 polarization is a T2 ribonuclease (omega-1). J Exp Med. (2009) 206:1681–90. doi: 10.1084/jem.20082462
49. Everts B, Hussaarts L, Driessen NN, Meevissen MH, Schramm G, Van Der Ham AJ, et al. Schistosome-derived omega-1 drives Th2 polarization by suppressing protein synthesis following internalization by the mannose receptor. J Exp Med. (2012) 209:1753–1767, S1751. doi: 10.1084/jem.20111381
50. Hussaarts L, Smits HH, Schramm G, Van Der Ham AJ, Van Der Zon GC, Haas H, et al. Rapamycin and omega-1: mTOR-dependent and -independent Th2 skewing by human dendritic cells. Immunol Cell Biol. (2013) 91:486–9. doi: 10.1038/icb.2013.31
51. Thomas PG, Harn DA Jr. Immune biasing by helminth glycans. Cell Microbiol. (2004) 6:13–22. doi: 10.1046/j.1462-5822.2003.00337.x
52. Saunders SP, Barlow JL, Walsh CM, Bellsoi A, Smith P, Mckenzie AN, et al. C-type lectin SIGN-R1 has a role in experimental colitis and responsiveness to lipopolysaccharide. J Immunol. (2010) 184:2627–37. doi: 10.4049/jimmunol.0901970
53. Meevissen MH, Yazdanbakhsh M, Hokke CH. Schistosoma mansoni egg glycoproteins and C-type lectins of host immune cells: molecular partners that shape immune responses. Exp Parasitol. (2012) 132:14–21. doi: 10.1016/j.exppara.2011.05.005
54. Kalantari P, Morales Y, Miller EA, Jaramillo LD, Ponichtera HE, Wuethrich MA, et al. CD209a Synergizes with dectin-2 and mincle to drive severe Th17 cell-mediated schistosome egg-induced immunopathology. Cell Rep. (2018) 22:1288–300. doi: 10.1016/j.celrep.2018.01.001
55. Cooke A, Tonks P, Jones FM, O'shea H, Hutchings P, Fulford AJ, et al. Infection with Schistosoma mansoni prevents insulin dependent diabetes mellitus in non-obese diabetic mice. Parasite Immunol. (1999) 21:169–76. doi: 10.1046/j.1365-3024.1999.00213.x
56. Ferguson BJ, Newland SA, Gibbs SE, Tourlomousis P, Fernandes Dos Santos P, Patel MN, et al. The Schistosoma mansoni T2 ribonuclease omega-1 modulates inflammasome-dependent IL-1beta secretion in macrophages. Int J Parasitol. (2015) 45:809–13. doi: 10.1016/j.ijpara.2015.08.005
57. Zaccone P, Burton O, Miller N, Jones FM, Dunne DW, Cooke A. Schistosoma mansoni egg antigens induce Treg that participate in diabetes prevention in NOD mice. Eur J Immunol. (2009) 39:1098–107. doi: 10.1002/eji.200838871
58. Zaccone P, Burton OT, Gibbs SE, Miller N, Jones FM, Schramm G, et al. The S. mansoni glycoprotein omega-1 induces Foxp3 expression in NOD mouse CD4(+) T cells. Eur J Immunol. (2011) 41:2709–18. doi: 10.1002/eji.201141429
59. Hams E, Bermingham R, Wurlod FA, Hogan AE, O'shea D, Preston RJ, et al. The helminth T2 RNase omega1 promotes metabolic homeostasis in an IL-33- and group 2 innate lymphoid cell-dependent mechanism. FASEB J. (2016) 30:824–35. doi: 10.1096/fj.15-277822
60. Yang J, Qiu C, Xia Y, Yao L, Fu Z, Yuan C, et al. Molecular cloning and functional characterization of Schistosoma japonicum enolase which is highly expressed at the schistosomulum stage. Parasitol Res. (2010) 107:667–77. doi: 10.1007/s00436-010-1913-z
61. Samoil V, Dagenais M, Ganapathy V, Aldridge J, Glebov A, Jardim A, et al. Vesicle-based secretion in schistosomes: analysis of protein and microRNA (miRNA) content of exosome-like vesicles derived from Schistosoma mansoni. Sci Rep. (2018) 8:3286. doi: 10.1038/s41598-018-21587-4
62. Esgleas M, Li Y, Hancock MA, Harel J, Dubreuil JD, Gottschalk M. Isolation and characterization of alpha-enolase, a novel fibronectin-binding protein from Streptococcus suis. Microbiology (2008) 154:2668–79. doi: 10.1099/mic.0.2008/017145-0
63. Bhowmick IP, Kumar N, Sharma S, Coppens I, Jarori GK. Plasmodium falciparum enolase: stage-specific expression and sub-cellular localization. Malar J. (2009) 8:179. doi: 10.1186/1475-2875-8-179
64. Bock A, Tucker N, Kelher MR, Khan SY, Gonzalez E, Wohlauer M, et al. Alpha-Enolase causes proinflammatory activation of pulmonary microvascular endothelial cells and primes neutrophils through plasmin activation of protease-activated receptor 2. Shock (2015) 44:137–42. doi: 10.1097/SHK.0000000000000394
65. File S. Interaction of schistosome eggs with vascular endothelium. J Parasitol. (1995) 81:234–8. doi: 10.2307/3283925
66. Wu YP, Lenting PJ, Tielens AG, De Groot PG, Van Hellemond JJ. Differential platelet adhesion to distinct life-cycle stages of the parasitic helminth Schistosoma mansoni. J Thromb Haemost. (2007) 5:2146–8. doi: 10.1111/j.1538-7836.2007.02725.x
67. Ngaiza JR, Doenhoff MJ. Blood platelets and schistosome egg excretion. Proc Soc Exp Biol Med. (1990) 193:73–9. doi: 10.3181/00379727-193-42993
68. Dewalick S, Hensbergen PJ, Bexkens ML, Grosserichter-Wagener C, Hokke CH, Deelder AM, et al. Binding of von Willebrand factor and plasma proteins to the eggshell of Schistosoma mansoni. Int J Parasitol. (2014) 44:263–8. doi: 10.1016/j.ijpara.2013.12.006
69. Figueiredo BC, Da'dara AA, Oliveira SC, Skelly PJ. Schistosomes enhance plasminogen activation: the role of tegumental enolase. PLoS Pathog. (2015) 11:e1005335. doi: 10.1371/journal.ppat.1005335
70. Carmo AA, Costa BR, Vago JP, De Oliveira LC, Tavares LP, Nogueira CR, et al. Plasmin induces in vivo monocyte recruitment through protease-activated receptor-1-, MEK/ERK-, and CCR2-mediated signaling. J Immunol. (2014) 193:3654–63. doi: 10.4049/jimmunol.1400334
71. Ritter DM, Mckerrow JH. Intercellular adhesion molecule 1 is the major adhesion molecule expressed during schistosome granuloma formation. Infect Immun. (1996) 64:4706–13.
72. Lukacs NW, Chensue SW, Strieter RM, Warmington K, Kunkel SL. Inflammatory granuloma formation is mediated by TNF-alpha-inducible intercellular adhesion molecule-1. J Immunol. (1994) 152:5883–9.
73. Secor WE, Dos Reis MG, Ramos EA, Matos EP, Reis EA, Do Carmo TM, et al. Soluble intercellular adhesion molecules in human schistosomiasis: correlations with disease severity and decreased responsiveness to egg antigens. Infect Immun. (1994) 62:2695–701.
74. Jacobs W, Bogers J, Deelder A, Van Marck E. Expression of intercellular adhesion molecule-1 and lymphocytefunction-associated antigen-1 in experimental Schistosoma mansoni infection and in synchronous periparticular hepatic granulomas in mice: immunohistochemistry, confocal laser scanning microscopy, and immunoelectron microscopy. Parasitol Res. (1997) 83:405–12. doi: 10.1007/s004360050273
75. Jacobs W, Bogers JJ, Timmermans JP, Deelder AM, Van Marck EA. Adhesion molecules in intestinal Schistosoma mansoni infection. Parasitol Res. (1998) 84:276–80. doi: 10.1007/s004360050395
76. Turner JD, Narang P, Coles MC, Mountford AP. Blood flukes exploit Peyer's patch lymphoid tissue to facilitate transmission from the mammalian host. PLoS Pathog. (2012) 8:e1003063. doi: 10.1371/journal.ppat.1003063
77. Doenhoff M, Musallam R, Bain J, Mcgregor A. Studies on the host-parasite relationship in Schistosoma mansoni-infected mice: the immunological dependence of parasite egg excretion. Immunology (1978) 35:771–8.
78. Dunne DW, Doenhoff MJ. Schistosoma mansoni egg antigens and hepatocyte damage in infected T cell-deprived mice. Contrib Microbiol Immunol. (1983) 7:22–9.
79. Cheever AW, Eltoum IA, Andrade ZA, Cox TM. Biology and pathology of Schistosoma mansoni and Schistosoma japonicum infections in several strains of nude mice. Am J Trop Med Hyg. (1993) 48:496–503. doi: 10.4269/ajtmh.1993.48.496
80. Amiri P, Locksley RM, Parslow TG, Sadick M, Rector E, Ritter D, et al. Tumour necrosis factor alpha restores granulomas and induces parasite egg-laying in schistosome-infected SCID mice. Nature (1992) 356:604–7. doi: 10.1038/356604a0
81. Cheever AW, Poindexter RW, Wynn TA. Egg laying is delayed but worm fecundity is normal in SCID mice infected with Schistosoma japonicum and S. mansoni with or without recombinant tumor necrosis factor alpha treatment. Infect Immun. (1999) 67:2201–8.
82. Karanja DM, Colley DG, Nahlen BL, Ouma JH, Secor WE. Studies on schistosomiasis in western Kenya: I. Evidence for immune-facilitated excretion of schistosome eggs from patients with Schistosoma mansoni and human immunodeficiency virus coinfections Am J Trop Med Hyg. (1997) 56:515–21. doi: 10.4269/ajtmh.1997.56.515
83. Colombe S, Lee MH, Masikini PJ, Van Lieshout L, De Dood CJ, Hoekstra PT, et al. Decreased sensitivity of Schistosoma sp. egg microscopy in women and HIV-infected individuals. Am J Trop Med Hyg. (2018) 98:1159–64. doi: 10.4269/ajtmh.17-0790
84. Muok EM, Simiyu EW, Ochola EA, Ng'ang'a ZW, Secor WE, Karanja DM, et al. Association between CD4+ T-lymphocyte counts and fecal excretion of Schistosoma mansoni eggs in patients coinfected with S. mansoni and human immunodeficiency virus before and after initiation of antiretroviral therapy. Am J Trop Med Hyg. (2013) 89:42–5. doi: 10.4269/ajtmh.13-0045
85. Kallestrup P, Zinyama R, Gomo E, Butterworth AE, Van Dam GJ, Erikstrup C, et al. Schistosomiasis and HIV-1 infection in rural Zimbabwe: implications of coinfection for excretion of eggs. J Infect Dis. (2005) 191:1311–20. doi: 10.1086/428907
86. Kleppa E, Klinge KF, Galaphaththi-Arachchige HN, Holmen SD, Lillebo K, Onsrud M, et al. Schistosoma haematobium infection and CD4+ T-cell levels: a cross-sectional study of young South African women. PLoS ONE (2015) 10:e0119326. doi: 10.1371/journal.pone.0119326
87. Herbert DR, Holscher C, Mohrs M, Arendse B, Schwegmann A, Radwanska M, et al. Alternative macrophage activation is essential for survival during schistosomiasis and downmodulates T helper 1 responses and immunopathology. Immunity (2004) 20:623–35. doi: 10.1016/S1074-7613(04)00107-4
88. Chiaramonte MG, Mentink-Kane M, Jacobson BA, Cheever AW, Whitters MJ, Goad ME, et al. Regulation and function of the interleukin 13 receptor alpha 2 during a T helper cell type 2-dominant immune response. J Exp Med. (2003) 197:687–701. doi: 10.1084/jem.20020903
89. Moore DL, Grove DI, Warren KS. The Schistosoma mansoni egg granuloma: quantitation of cell populations. J Pathol. (1977) 121:41–50. doi: 10.1002/path.1711210107
90. Lenzi HL, Lenzi JA, Sobral AC. Eosinophils favor the passage of eggs to the intestinal lumen in schistosomiasis. Braz J Med Biol Res. (1987) 20:433–5.
91. Sher A, Coffman RL, Hieny S, Cheever AW. Ablation of eosinophil and IgE responses with anti-IL-5 or anti-IL-4 antibodies fails to affect immunity against Schistosoma mansoni in the mouse. J Immunol. (1990) 145:3911–6.
92. Sher A, Coffman RL, Hieny S, Scott P, Cheever AW. Interleukin 5 is required for the blood and tissue eosinophilia but not granuloma formation induced by infection with Schistosoma mansoni. Proc Natl Acad Sci USA. (1990) 87:61–5.
93. Swartz JM, Dyer KD, Cheever AW, Ramalingam T, Pesnicak L, Domachowske JB, et al. Schistosoma mansoni infection in eosinophil lineage-ablated mice. Blood (2006) 108:2420–7. doi: 10.1182/blood-2006-04-015933
94. Hsu SY, Hsu HF, Mitros FA, Helms CM, Solomon RI. Eosinophils as effector cells in the destruction of Schistosoma mansoni eggs in granulomas. Ann Trop Med Parasitol. (1980) 74:179–83. doi: 10.1080/00034983.1980.11687328
95. Palacios-Macapagal D, Connor J, Mustelin T, Ramalingam TR, Wynn TA, Davidson TS. Cutting edge: eosinophils undergo caspase-1-mediated pyroptosis in response to necrotic liver cells. J Immunol. (2017) 199:847–53. doi: 10.4049/jimmunol.1601162
96. Knuhr K, Doenhoff M, Fehrenbach H, Haas H, Schramm G. IPSE/alpha-1, a secreted glycoprotein from Schistosoma mansoni eggs, may inhibit inflammation. In: 27th Spring Symposium of the German Society of Allergy and Clinical Immunology: Allergo Journal International (2015), 24–43.
97. Meyer NH, Mayerhofer H, Tripsianes K, Blindow S, Barths D, Mewes A, et al. A crystallin fold in the interleukin-4-inducing principle of Schistosoma mansoni eggs (IPSE/alpha-1) mediates IgE binding for antigen-independent basophil activation. J Biol Chem. (2015) 290:22111–26. doi: 10.1074/jbc.M115.675066
98. Siracusa MC, Saenz SA, Hill DA, Kim BS, Headley MB, Doering TA, et al. TSLP promotes interleukin-3-independent basophil haematopoiesis and type 2 inflammation. Nature (2011) 477:229–33. doi: 10.1038/nature10329
99. Schwartz C, Turqueti-Neves A, Hartmann S, Yu P, Nimmerjahn F, Voehringer D. Basophil-mediated protection against gastrointestinal helminths requires IgE-induced cytokine secretion. Proc Natl Acad Sci USA (2014) 111:E5169–77. doi: 10.1073/pnas.1412663111
100. Anyan WK, Seki T, Kumagai T, Obata-Ninomiya K, Furushima-Shimogawara R, Kwansa-Bentum B, et al. Basophil depletion downregulates Schistosoma mansoni egg-induced granuloma formation. Parasitol Int. (2013) 62:508–13. doi: 10.1016/j.parint.2013.07.003
101. Chuah C, Jones MK, Burke ML, Mcmanus DP, Owen HC, Gobert GN. Defining a pro-inflammatory neutrophil phenotype in response to schistosome eggs. Cell Microbiol. (2014) 16:1666–77. doi: 10.1111/cmi.12316
102. Morais SB, Figueiredo BC, Assis NRG, Alvarenga DM, De Magalhaes MTQ, Ferreira RS, et al. Schistosoma mansoni SmKI-1 serine protease inhibitor binds to elastase and impairs neutrophil function and inflammation. PLoS Pathog. (2018) 14:e1006870. doi: 10.1371/journal.ppat.1006870
103. Klose CS, Artis D. Innate lymphoid cells as regulators of immunity, inflammation and tissue homeostasis. Nat Immunol (2016) 17:765–74. doi: 10.1038/ni.3489
104. Ebbo M, Crinier A, Vely F, Vivier E. Innate lymphoid cells: major players in inflammatory diseases. Nat Rev Immunol. (2017) 17:665–78. doi: 10.1038/nri.2017.86
105. Hams E, Armstrong ME, Barlow JL, Saunders SP, Schwartz C, Cooke G, et al. IL-25 and type 2 innate lymphoid cells induce pulmonary fibrosis. Proc Natl Acad Sci USA. (2014) 111:367–72. doi: 10.1073/pnas.1315854111
106. Vannella KM, Ramalingam TR, Borthwick LA, Barron L, Hart KM, Thompson RW, et al. Combinatorial targeting of TSLP, IL-25, and IL-33 in type 2 cytokine-driven inflammation and fibrosis. Sci Transl Med. (2016) 8:337ra365. doi: 10.1126/scitranslmed.aaf1938
107. Hepworth MR, Fung TC, Masur SH, Kelsen JR, Mcconnell FM, Dubrot J, et al. Immune tolerance. Group 3 innate lymphoid cells mediate intestinal selection of commensal bacteria-specific CD4(+) T cells. Science (2015) 348:1031–5. doi: 10.1126/science.aaa4812
108. Nausch N, Appleby LJ, Sparks AM, Midzi N, Mduluza T, Mutapi F. Group 2 innate lymphoid cell proportions are diminished in young helminth infected children and restored by curative anti-helminthic treatment. PLoS Negl Trop Dis. (2015) 9:e0003627. doi: 10.1371/journal.pntd.0003627
109. Macdonald AS, Straw AD, Dalton NM, Pearce EJ. Cutting edge: Th2 response induction by dendritic cells: a role for CD40. J Immunol. (2002) 168:537–40. doi: 10.4049/jimmunol.168.2.537
110. Phythian-Adams AT, Cook PC, Lundie RJ, Jones LH, Smith KA, Barr TA, et al. CD11c depletion severely disrupts Th2 induction and development in vivo. J Exp Med. (2010) 207:2089–96. doi: 10.1084/jem.20100734
111. Lundie RJ, Webb LM, Marley AK, Phythian-Adams AT, Cook PC, Jackson-Jones LH, et al. A central role for hepatic conventional dendritic cells in supporting Th2 responses during helminth infection. Immunol Cell Biol. (2016) 94:400–10. doi: 10.1038/icb.2015.114
112. Hesse M, Modolell M, La Flamme AC, Schito M, Fuentes JM, Cheever AW, et al. Differential regulation of nitric oxide synthase-2 and arginase-1 by type 1/type 2 cytokines in vivo: granulomatous pathology is shaped by the pattern of L-arginine metabolism. J Immunol. (2001) 167:6533–44. doi: 10.4049/jimmunol.167.11.6533
113. Pesce JT, Ramalingam TR, Mentink-Kane MM, Wilson MS, El Kasmi KC, Smith AM, et al. Arginase-1-expressing macrophages suppress Th2 cytokine-driven inflammation and fibrosis. PLoS Pathog. (2009) 5:e1000371. doi: 10.1371/journal.ppat.1000371
114. Vannella KM, Barron L, Borthwick LA, Kindrachuk KN, Narasimhan PB, Hart KM, et al. Incomplete deletion of IL-4Ralpha by LysM(Cre) reveals distinct subsets of M2 macrophages controlling inflammation and fibrosis in chronic schistosomiasis. PLoS Pathog. (2014) 10:e1004372. doi: 10.1371/journal.ppat.1004372
115. Mathew RC, Boros DL. Anti-L3T4 antibody treatment suppresses hepatic granuloma formation and abrogates antigen-induced interleukin-2 production in Schistosoma mansoni infection. Infect Immun. (1986) 54:820–6.
116. Fallon PG, Smith P, Dunne DW. Type 1 and type 2 cytokine-producing mouse CD4+ and CD8+ T cells in acute Schistosoma mansoni infection. Eur J Immunol. (1998) 28:1408–16. doi: 10.1002/(SICI)1521-4141(199804)28:04<1408::AID-IMMU1408>3.0.CO;2-H
117. Boros DL, Whitfield JR. Endogenous IL-10 regulates IFN-gamma and IL-5 cytokine production and the granulomatous response in Schistosomiasis mansoni-infected mice. Immunology (1998) 94:481–7. doi: 10.1046/j.1365-2567.1998.00544.x
118. Kaplan MH, Whitfield JR, Boros DL, Grusby MJ. Th2 cells are required for the Schistosoma mansoni egg-induced granulomatous response. J Immunol. (1998) 160:1850–6.
119. Fallon PG, Dunne DW. Tolerization of mice to Schistosoma mansoni egg antigens causes elevated type 1 and diminished type 2 cytokine responses and increased mortality in acute infection. J Immunol. (1999) 162:4122–32.
120. Wynn TA, Hoffmann KF. Defining a schistosomiasis vaccination strategy–is it really Th1 versus Th2? Parasitol Today (2000) 16:497–501. doi: 10.1016/S0169-4758(00)01788-9
121. Mwatha JK, Kimani G, Kamau T, Mbugua GG, Ouma JH, Mumo J, et al. High levels of TNF, soluble TNF receptors, soluble ICAM-1, and IFN-gamma, but low levels of IL-5, are associated with hepatosplenic disease in human schistosomiasis mansoni. J Immunol. (1998) 160:1992–9.
122. Rutitzky LI, Lopes Da Rosa JR, Stadecker MJ. Severe CD4 T cell-mediated immunopathology in murine schistosomiasis is dependent on IL-12p40 and correlates with high levels of IL-17. J Immunol. (2005) 175:3920–6. doi: 10.4049/jimmunol.175.6.3920
123. Rutitzky LI, Bazzone L, Shainheit MG, Joyce-Shaikh B, Cua DJ, Stadecker MJ. IL-23 is required for the development of severe egg-induced immunopathology in schistosomiasis and for lesional expression of IL-17. J Immunol. (2008) 180:2486–95. doi: 10.4049/jimmunol.180.4.2486
124. Shainheit MG, Smith PM, Bazzone LE, Wang AC, Rutitzky LI, Stadecker MJ. Dendritic cell IL-23 and IL-1 production in response to schistosome eggs induces Th17 cells in a mouse strain prone to severe immunopathology. J Immunol. (2008) 181:8559–67. doi: 10.4049/jimmunol.181.12.8559
125. Ponichtera HE, Stadecker MJ. Dendritic cell expression of the C-type lectin receptor CD209a: a novel innate parasite-sensing mechanism inducing Th17 cells that drive severe immunopathology in murine schistosome infection. Exp Parasitol. (2015) 158:42–7. doi: 10.1016/j.exppara.2015.04.006
126. Rutitzky LI, Stadecker MJ. Exacerbated egg-induced immunopathology in murine Schistosoma mansoni infection is primarily mediated by IL-17 and restrained by IFN-gamma. Eur J Immunol. (2011) 41:2677–87. doi: 10.1002/eji.201041327
127. Walsh CM, Smith P, Fallon PG. Role for CTLA-4 but not CD25+ T cells during Schistosoma mansoni infection of mice. Parasite Immunol. (2007) 29:293–308. doi: 10.1111/j.1365-3024.2007.00947.x
128. Layland LE, Mages J, Loddenkemper C, Hoerauf A, Wagner H, Lang R, et al. Pronounced phenotype in activated regulatory T cells during a chronic helminth infection. J Immunol. (2010) 184:713–24. doi: 10.4049/jimmunol.0901435
129. Ondigo BN, Ndombi EM, Nicholson SC, Oguso JK, Carter JM, Kittur N, et al. Functional studies of T regulatory lymphocytes in human schistosomiasis in Western Kenya. Am J Trop Med Hyg. (2018) 98:1770–81. doi: 10.4269/ajtmh.17-0966
130. Hori S, Nomura T, Sakaguchi S. Control of regulatory T cell development by the transcription factor Foxp3. Science (2003) 299:1057–61. doi: 10.1126/science.1079490
131. Baumgart M, Tompkins F, Leng J, Hesse M. Naturally occurring CD4+Foxp3+ regulatory T cells are an essential, IL-10-independent part of the immunoregulatory network in Schistosoma mansoni egg-induced inflammation. J Immunol. (2006) 176:5374–87. doi: 10.4049/jimmunol.176.9.5374
132. Turner JD, Jenkins GR, Hogg KG, Aynsley SA, Paveley RA, Cook PC, et al. CD4+CD25+ regulatory cells contribute to the regulation of colonic Th2 granulomatous pathology caused by schistosome infection. PLoS Negl Trop Dis. (2011) 5:e1269. doi: 10.1371/journal.pntd.0001269
133. Hernandez HJ, Wang Y, Stadecker MJ. In infection with Schistosoma mansoni, B cells are required for T helper type 2 cell responses but not for granuloma formation. J Immunol. (1997) 158:4832–7.
134. Ferru I, Roye O, Delacre M, Auriault C, Wolowczuk I. Infection of B-cell-deficient mice by the parasite Schistosoma mansoni: demonstration of the participation of B cells in granuloma modulation. Scand J Immunol. (1998) 48:233–40. doi: 10.1046/j.1365-3083.1998.00376.x
135. Gaubert S, Viana Da Costa A, Maurage CA, Lima EC, Fontaine J, Lafitte S, et al. X-linked immunodeficiency affects the outcome of Schistosoma mansoni infection in the murine model. Parasite Immunol. (1999) 21:89–101.
136. Boros DL, Pelley RP, Warren KS. Spontaneous modulation of granulomatous hypersensitivity in schistosomiasis mansoni. J Immunol. (1975) 114:1437–41.
137. Goes AM, Ramalho-Pinto FJ. Protective immunity to Schistosoma mansoni in mice is dependent on antibody and complement but not on radiosensitive leukocytes. Immunol Lett. (1991) 28:57–63. doi: 10.1016/0165-2478(91)90127-V
138. Jankovic D, Kullberg MC, Dombrowicz D, Barbieri S, Caspar P, Wynn TA, et al. Fc epsilonRI-deficient mice infected with Schistosoma mansoni mount normal Th2-type responses while displaying enhanced liver pathology. J Immunol. (1997) 159:1868–75.
139. Jankovic D, Cheever AW, Kullberg MC, Wynn TA, Yap G, Caspar P, et al. CD4+ T cell-mediated granulomatous pathology in schistosomiasis is downregulated by a B cell-dependent mechanism requiring Fc receptor signaling. J Exp Med. (1998) 187:619–29. doi: 10.1084/jem.187.4.619
140. Goes AM, Gazzinelli G, Rocha R, Katz N, Doughty BL. Granulomatous hypersensitivity to Schistosoma mansoni egg antigens in human schistosomiasis. III in vitro granuloma modulation induced by immune complexes. Am J Trop Med Hyg. (1991) 44:434–43. doi: 10.4269/ajtmh.1991.44.434
141. Cheever AW, Byram JE, Hieny S, Von Lichtenberg F, Lunde MN, Sher A. Immunopathology of Schistosoma japonicum and S. mansoni infection in B cell depleted mice Parasite Immunol. (1985) 7:399–413. doi: 10.1111/j.1365-3024.1985.tb00086.x
142. Velupillai P, Harn DA. Oligosaccharide-specific induction of interleukin 10 production by B220+ cells from schistosome-infected mice: a mechanism for regulation of CD4+ T-cell subsets. Proc Natl Acad Sci USA. (1994) 91:18–22. doi: 10.1073/pnas.91.1.18
143. El Ridi R, Velupillai P, Harn DA. Regulation of schistosome egg granuloma formation: host-soluble L-selectin enters tissue-trapped eggs and binds to carbohydrate antigens on surface membranes of miracidia. Infect Immun. (1996) 64:4700–5.
144. Mangan NE, Fallon RE, Smith P, Van Rooijen N, Mckenzie AN, Fallon PG. Helminth infection protects mice from anaphylaxis via IL-10-producing B cells. J Immunol. (2004) 173:6346–56. doi: 10.4049/jimmunol.173.10.6346
145. Smits HH, Hammad H, Van Nimwegen M, Soullie T, Willart MA, Lievers E, et al. Protective effect of Schistosoma mansoni infection on allergic airway inflammation depends on the intensity and chronicity of infection. J Allergy Clin Immunol. (2007) 120:932–40. doi: 10.1016/j.jaci.2007.06.009
146. Amu S, Saunders SP, Kronenberg M, Mangan NE, Atzberger A, Fallon PG. Regulatory B cells prevent and reverse allergic airway inflammation via FoxP3-positive T regulatory cells in a murine model. J Allergy Clin Immunol. (2010) 125:1114–24 e1118. doi: 10.1016/j.jaci.2010.01.018
147. Haeberlein S, Obieglo K, Ozir-Fazalalikhan A, Chaye MAM, Veninga H, Van Der Vlugt L, et al. Schistosome egg antigens, including the glycoprotein IPSE/alpha-1, trigger the development of regulatory B cells. PLoS Pathog. (2017) 13:e1006539. doi: 10.1371/journal.ppat.1006539
148. Gerbe F, Sidot E, Smyth DJ, Ohmoto M, Matsumoto I, Dardalhon V, et al. Intestinal epithelial tuft cells initiate type 2 mucosal immunity to helminth parasites. Nature (2016) 529:226–30. doi: 10.1038/nature16527
149. Howitt MR, Lavoie S, Michaud M, Blum AM, Tran SV, Weinstock JV, et al. Tuft cells, taste-chemosensory cells, orchestrate parasite type 2 immunity in the gut. Science (2016) 351:1329–33. doi: 10.1126/science.aaf1648
150. Von Moltke J, Ji M, Liang HE, Locksley RM. Tuft-cell-derived IL-25 regulates an intestinal ILC2-epithelial response circuit. Nature (2016) 529:221–5. doi: 10.1038/nature16161
152. Wang Q, Da'dara AA, Skelly PJ. The human blood parasite Schistosoma mansoni expresses extracellular tegumental calpains that cleave the blood clotting protein fibronectin. Sci Rep. (2017) 7:12912. doi: 10.1038/s41598-017-13141-5
153. Al Adnani MS. Concomitant immunohistochemical localization of fibronectin and collagen in schistosome granulomata. J Pathol. (1985) 147:77–85. doi: 10.1002/path.1711470202
154. Grimaud JA, Boros DL, Takiya C, Mathew RC, Emonard H. Collagen isotypes, laminin, and fibronectin in granulomas of the liver and intestines of Schistosoma mansoni-infected mice. Am J Trop Med Hyg. (1987) 37:335–44. doi: 10.4269/ajtmh.1987.37.335
155. Pino-Heiss S, Brown M, Mckerrow JH. Schistosoma mansoni: degradation of host extracellular matrix by eggs and miracidia. Exp Parasitol. (1985) 59:217–21. doi: 10.1016/0014-4894(85)90075-X
156. Madsen DH, Leonard D, Masedunskas A, Moyer A, Jurgensen HJ, Peters DE, et al. M2-like macrophages are responsible for collagen degradation through a mannose receptor-mediated pathway. J Cell Biol. (2013) 202:951–66. doi: 10.1083/jcb.201301081
157. Singh KP, Gerard HC, Hudson AP, Boros DL. Differential expression of collagen, MMP, TIMP and fibrogenic-cytokine genes in the granulomatous colon of Schistosoma mansoni-infected mice. Ann Trop Med Parasitol. (2006) 100:611–20. doi: 10.1179/136485906X118530
158. Lenzi HL, Kimmel E, Schechtman H, Pelajo-Machado M, Vale BS, Panasco MS, et al. Collagen arrangement in hepatic granuloma in mice infected with Schistosoma mansoni: dependence on fiber radiation centers. Braz J Med Biol Res. (1999) 32:639–43. doi: 10.1590/S0100-879X1999000500018
159. Elbaz T, Esmat G. Hepatic and intestinal schistosomiasis: review. J Adv Res. (2013) 4:445–52. doi: 10.1016/j.jare.2012.12.001
160. Issa I, Osman M, Aftimos G. Schistosomiasis manifesting as a colon polyp: a case report. J Med Case Rep. (2014) 8:331. doi: 10.1186/1752-1947-8-331
161. Sharon G, Garg N, Debelius J, Knight R, Dorrestein PC, Mazmanian SK. Specialized metabolites from the microbiome in health and disease. Cell Metab. (2014) 20:719–30. doi: 10.1016/j.cmet.2014.10.016
162. Garrett WS, Gallini CA, Yatsunenko T, Michaud M, Dubois A, Delaney ML, et al. Enterobacteriaceae act in concert with the gut microbiota to induce spontaneous and maternally transmitted colitis. Cell Host Microbe (2010) 8:292–300. doi: 10.1016/j.chom.2010.08.004
163. Wu X, Ma C, Han L, Nawaz M, Gao F, Zhang X, et al. Molecular characterisation of the faecal microbiota in patients with type II diabetes. Curr Microbiol. (2010) 61:69–78. doi: 10.1007/s00284-010-9582-9
164. Berer K, Mues M, Koutrolos M, Rasbi ZA, Boziki M, Johner C, et al. Commensal microbiota and myelin autoantigen cooperate to trigger autoimmune demyelination. Nature (2011) 479:538–41. doi: 10.1038/nature10554
165. Reynolds LA, Smith KA, Filbey KJ, Harcus Y, Hewitson JP, Redpath SA, et al. Commensal-pathogen interactions in the intestinal tract: lactobacilli promote infection with, and are promoted by, helminth parasites. Gut Microbes (2014) 5:522–32. doi: 10.4161/gmic.32155
166. Hayes KS, Bancroft AJ, Goldrick M, Portsmouth C, Roberts IS, Grencis RK. Exploitation of the intestinal microflora by the parasitic nematode Trichuris muris. Science (2010) 328:1391–4. doi: 10.1126/science.1187703
167. Bautista-Garfias CR, Ixta-Rodriguez O, Martinez-Gomez F, Lopez MG, Aguilar-Figueroa BR. Effect of viable or dead Lactobacillus casei organisms administered orally to mice on resistance against Trichinella spiralis infection. Parasite (2001) 8:S226–228. doi: 10.1051/parasite/200108s2226
168. Holzscheiter M, Layland LE, Loffredo-Verde E, Mair K, Vogelmann R, Langer R, et al. Lack of host gut microbiota alters immune responses and intestinal granuloma formation during schistosomiasis. Clin Exp Immunol. (2014) 175:246–57. doi: 10.1111/cei.12230
169. Schneeberger PHH, Coulibaly JT, Panic G, Daubenberger C, Gueuning M, Frey JE, et al. Investigations on the interplays between Schistosoma mansoni, praziquantel and the gut microbiome. Parasit Vectors (2018) 11:168. doi: 10.1186/s13071-018-2739-2
170. Kay GL, Millard A, Sergeant MJ, Midzi N, Gwisai R, Mduluza T, et al. Differences in the faecal microbiome in schistosoma haematobium infected children vs. uninfected children. PLoS Negl Trop Dis. (2015) 9:e0003861. doi: 10.1371/journal.pntd.0003861
171. Khan AR, Fallon PG. Helminth therapies: translating the unknown unknowns to known knowns. Int J Parasitol. (2013) 43:293–9. doi: 10.1016/j.ijpara.2012.12.002
172. Maezawa K, Furushima-Shimogawara R, Yasukawa A, Ohta N, Iwanaga S. Real-time observation of pathophysiological processes during murine experimental Schistosoma japonicum infection using high-resolution ultrasound imaging. Trop Med Health (2018) 46:1. doi: 10.1186/s41182-017-0082-5
173. Girgis NM, Gundra UM, Ward LN, Cabrera M, Frevert U, Loke P. Ly6C(high) monocytes become alternatively activated macrophages in schistosome granulomas with help from CD4+ cells. PLoS Pathog. (2014) 10:e1004080. doi: 10.1371/journal.ppat.1004080
174. Hagen J, Young ND, Every AL, Pagel CN, Schnoeller C, Scheerlinck JP, et al. Omega-1 knockdown in Schistosoma mansoni eggs by lentivirus transduction reduces granuloma size in vivo. Nat Commun. (2014) 5:5375. doi: 10.1038/ncomms6375
175. Anderson L, Amaral MS, Beckedorff F, Silva LF, Dazzani B, Oliveira KC, et al. Schistosoma mansoni egg, adult male and female comparative gene expression analysis and identification of novel genes by RNA-seq. PLoS Negl Trop Dis. (2015) 9:e0004334. doi: 10.1371/journal.pntd.0004334
176. Howe KL, Bolt BJ, Shafie M, Kersey P, Berriman M. WormBase ParaSite–a comprehensive resource for helminth genomics. Mol Biochem Parasitol. (2017) 215:2–10. doi: 10.1016/j.molbiopara.2016.11.005
177. Doughty BL, Goes AM, Parra JC, Rocha RS, Katz N, Colley DG, et al. Granulomatous hypersensitivity to Schistosoma mansoni egg antigens in human schistosomiasis. I Granuloma formation and modulation around polyacrylamide antigen-conjugated beads. Mem Inst Oswaldo Cruz (1987) 82(Suppl. 4):47–54. doi: 10.1590/S0074-02761987000800009
178. Nady S, Shata MT, Mohey MA, El-Shorbagy A. Protective role of IL-22 against Schistosoma mansoni soluble egg antigen-induced granuloma in vitro. Parasite Immunol. (2017) 39. doi: 10.1111/pim.12392
179. Kasendra M, Tovaglieri A, Sontheimer-Phelps A, Jalili-Firoozinezhad S, Bein A, Chalkiadaki A, et al. Development of a primary human small intestine-on-a-chip using biopsy-derived organoids. Sci Rep. (2018) 8:2871. doi: 10.1038/s41598-018-21201-7
180. Kim GA, Spence JR, Takayama S. Bioengineering for intestinal organoid cultures. Curr Opin Biotechnol. (2017) 47:51–8. doi: 10.1016/j.copbio.2017.05.006
181. Schweinlin M, Wilhelm S, Schwedhelm I, Hansmann J, Rietscher R, Jurowich C, et al. Development of an advanced primary human in vitro model of the small intestine. Tissue Eng Part C Methods (2016) 22:873–83. doi: 10.1089/ten.tec.2016.0101
Keywords: schistosoma, inflammation, granuloma, egg, excretion, regulation, intestine, liver
Citation: Schwartz C and Fallon PG (2018) Schistosoma “Eggs-Iting” the Host: Granuloma Formation and Egg Excretion. Front. Immunol. 9:2492. doi: 10.3389/fimmu.2018.02492
Received: 30 July 2018; Accepted: 09 October 2018;
Published: 29 October 2018.
Edited by:
Thiago Almeida Pereira, Stanford University, United StatesReviewed by:
Fausto Edmundo Lima Pereira, Universidade Vila Velha, BrazilWilliam Evan Secor, Centers for Disease Control and Prevention (CDC), United States
Copyright © 2018 Schwartz and Fallon. This is an open-access article distributed under the terms of the Creative Commons Attribution License (CC BY). The use, distribution or reproduction in other forums is permitted, provided the original author(s) and the copyright owner(s) are credited and that the original publication in this journal is cited, in accordance with accepted academic practice. No use, distribution or reproduction is permitted which does not comply with these terms.
*Correspondence: Padraic G. Fallon, pfallon@tcd.ie
†Present Address: Christian Schwartz, IFIZ, Department of Immunology, University of Tübingen, Tübingen, Germany