- 1Centre for Virus Research, Westmead Institute for Medical Research, Sydney, NSW, Australia
- 2Faculty of Medicine and Health, University of Sydney, Sydney, NSW, Australia
The advent of antiretroviral therapy (ART) has seen a dramatic decrease in the morbidity and mortality of individuals infected with human immunodeficiency virus (HIV). However, ART is not curative and HIV persists in treated individuals within a pool of infected CD4+ memory T cells. The targeting and elimination of these cells, termed the latent HIV reservoir, may be essential in establishing a cure for HIV. Current HIV reservoir research is focused on identifying cells that harbor latent, replication-competent, HIV provirus using specific cell surface markers. Recently, studies have turned to immune checkpoint (IC) molecules, such as programmed cell death protein 1 (PD-1). IC molecules are regulators of the immune system and have previously been linked to HIV infection. Furthermore, cells isolated from treated individuals co-expressing PD-1 alongside other IC molecules are enriched for HIV DNA. Administration of a IC blocking antibodies resulted in an increase of cell-associated HIV RNA within an individual, indicating the potential for this therapeutic to be utilized as a latency reversing agent. IC inhibitors could target CD4+ T cells expressing IC molecules and possibly enhance HIV transcription, allowing for the elimination of these cells by either ART or the immune system. However, treatment with IC inhibitors has been associated with toxicities such as immune-related adverse events and therefore future studies should proceed with caution.
Introduction
Current HIV reservoir research is focused on targeting and eliminating latently infected cells to establish a functional cure for HIV. To achieve this, researchers are utilizing cell surface markers to identify which cell types harbor greater quantities of HIV proviral DNA. Due to the error-prone nature of reverse transcription, HIV DNA often contains mutations that render the provirus incapable of producing new infectious virions (1–3). Only proviruses that contain all replication-essential genes are considered genetically intact and are replication competent (2, 4). It is these genetically-intact proviruses, harbored by memory CD4+ T cells, that are responsible for the viral rebound seen following the interruption of antiretroviral therapy (ART) and thus must be targeted (5). Studies have shown that levels of these latently infected cells, termed the functional HIV reservoir, are established early during acute infection and remain stable (6, 7), with little decay occurring after more than 7 years of therapy (4, 8–10). Moreover, due to the long half-life of memory cells that harbor latent HIV, as well as ongoing cellular proliferation (10, 11), it is estimated that more than 60 years of continuous therapy would be necessary to eliminate the virus from an individual (12).
The traditional memory CD4+ T cell subsets include central memory, transitional memory, and effector memory (TCM, TTM, and TEM, respectively). Initial investigations into memory cell subsets revealed TCM cells to be enriched for replication-competent HIV provirus (13, 14). However, studies were limited by either design or the methods employed in their investigation. The study performed by Chomont et al. (13) identified TCM as being enriched for inducible HIV DNA and showed that the contribution of TEM cells was significantly less. However, TEM are more difficult to isolate as they comprise a smaller proportion of total memory CD4+ T cells compared with their counterparts. In fact, in this study, TCM cells made up more than 45% of total CD4+ T counts whilst only 10% was attributed to TEM cells. Therefore, researchers were unable to identify significant amounts of HIV DNA within the TEM cell population. The latter study, performed by Soriano-Sarabia et al. failed to include the TEM cell subset in its investigation preventing a full assessment of all memory T cell subsets. With the advent of next-generation sequencing (NGS), we are able to sequence HIV DNA and gain insight into the location of genetically-intact and presumably replication-competent provirus, and consequently assess the contributions of cell populations to the functioning HIV reservoir (3, 15, 16).
Data obtained using NGS revealed TEM cells to contain increased proportions of genetically-intact HIV provirus (3) when compared with other memory cell subsets, indicating that TEM cells contribute significantly to the functioning latent reservoir. Preliminary data investigating the role of activation status of a cell and the latent HIV reservoir has indicated that the presence of the classical activation marker HLA-DR corresponds to an increased proportion of genetically intact integrated HIV provirus (17). Despite these findings, whether genetically-intact provirus isolated from cells expressing HLA-DR is capable of replicating remains to be demonstrated. Nevertheless, this concept supports the data implicating TEM cells as defining the replication-competent reservoir, as the TEM phenotype is one of increased cellular activation. It has become clear that cells can be found in a partially activated state and that the concept of cellular activation is better represented by a sliding scale than an on/off switch (18).
Investigations into HLA-DR as a marker of the latent reservoir have only begun in recent years. Prior to data published linking the marker with cells harboring integrated, genetically-intact HIV DNA (17, 19), HLA-DR was thought to indicate cells that were transcriptionally active and as such producing new infectious viral progeny—thus not in a latent state. The fact that cells expressing the activation marker HLA-DR are still capable of harboring latent HIV provirus has encouraged the exploration of other known markers of cellular activation. One such marker is the programmed cell-death protein 1 (PD-1), a known marker of immune regulation that is also categorized as an activation marker. Despite the inhibitory signaling it instigates within the cells which express it, PD-1 has been shown to cluster with HLA-DR (20) and is classified as a late stage cellular activation marker. The remainder of this review will discuss the evidence supporting the expression of PD-1, and other immune markers, as being intrinsically linked to latently infected cells and how this marker could be exploited in future HIV therapeutic approaches.
Role of Immune Checkpoint Molecules
In response to chronic viral infections, the immune system attenuates the effector function of T cells in a process known as T cell exhaustion. It is hypothesized that this process evolved to protect against the tissue-damaging consequences of a prolonged immune response, characteristic of ongoing chronic viral infections (21). T cell exhaustion was initially identified as specific to CD8+ T cells, however studies have demonstrated CD4+ T cells are also prone to exhaustion (22). It is worth noting that although T cell exhaustion can lead to the death of T cells, effector-function of exhausted cells can also be rescued (23). Exhausted T cells are characterized by expression of various inhibitory receptors, termed immune checkpoint (IC) molecules, such as PD-1 and cytotoxic T-lymphocyte-associated antigen 4 (CTLA-4). The ligation of these IC molecules induces intracellular signaling that inhibits cytokine production and ultimately results in cellular apoptosis (21). Current immunotherapies manipulate IC molecules in the treatment of several cancers, including melanoma, renal cancer and non-small cell lung cancer (NSCLC). Antibodies directed at IC molecules block their inhibitory effects, preventing the exhaustion of CD8+ T cells and enhancing anti-tumor immunity (24). The exhausted immune state that occurs in response to tumor formation is analogous to that which is seen during chronic infection with a virus—for example, HIV (21). This has sparked a wave of interest in IC molecules and their role in HIV infection and latency.
The expression of PD-1 and CTLA-4 on CD4+ T cells in the context of both treated and untreated HIV infection has been explored in several studies. In untreated infection, HIV-specific CD8+ T cells upregulate PD-1 expression as their effector function declines. PD-1 is also upregulated on HIV-specific CD4+ T cells, with in vitro PD-1 blockade restoring the proliferative nature of these cells that is lost following exhaustion (25). Moreover, PD-1 expression, in conjunction with other exhaustion markers TIM-3 and LAG3, was found to predict both time to viral rebound (>400 viral copies/ml) in patients that underwent treatment interruption (20) and disease pathogenesis in patients not receiving active treatment (26). With regards to CTLA-4, expression of this molecule by HIV-specific CD4+ T cells is elevated in all classes of infected individuals, with in vitro blockade resulting in significant levels of proliferation of these cells (27).
Recently, associations between IC molecules and the HIV latent reservoir have been identified. Specifically, memory CD4+ T cells (TCM and TTM) expressing higher levels of PD-1 were found to harbor larger quantities of HIV DNA when compared to their PD-1 low counterparts (13). However, this study failed to investigate the replication competency of the integrated proviruses isolated, meaning the contribution of these cells to the latent reservoir is yet to be fully elucidated. A more recent study demonstrated that CD4+ T cells, taken from treated individuals, co-expressing IC molecules PD-1, TIGIT, and LAG3 contain 10-fold the amount of integrated HIV DNA when compared with IC molecule negative CD4+ T cells. Moreover, CD4+ T cells expressing any one of these markers alone were found to harbor replication-competent integrated HIV DNA (28). This same study identified that cells expressing PD-1 were enriched for HIV genomes exclusively in TEM and TTM cells, supporting the findings of Hiener et al. (3). Additionally, CD4+ T cells expressing both PD-1 and CXCR5—markers of TFH cells—isolated from lymph nodes of long-term supressed individuals have also shown to harbor replication-competent HIV provirus (29). CTLA-4 has also been linked with the HIV latent reservoir, with CTLA-4+ CD4+ T cells (T regulatory cells) isolated from the lymph nodes of treated macaques shown to be enriched for SIV DNA (30).
Cells expressing IC molecules that harbor HIV provirus could not only comprise the latent reservoir but also contribute to its maintenance. The genetic characterisation of integrated HIV DNA revealed a large portion is genetically defective and identical (10, 11, 13), indicating ongoing cellular proliferation maintains the persistent HIV reservoir. It is known that PD-1 is a marker of both homeostatic and antigen-induced cellular proliferation (31, 32). Moreover, a preliminary study which sequenced HIV DNA isolated from PD-1 expressing cells found a large expansion of identical sequences, some of which were genetically intact (preliminary data from our laboratory, data not shown). These findings signify that natural cellular mechanisms could potentially contribute to the maintenance and persistence of the replication-competent HIV within PD-1 cells and represent a barrier in achieving a full cure for HIV.
It is possible that expression of PD-1 is correlated with the formation of the latent HIV reservoir. HIV infected cells expressing PD-1 will have attenuated gene expression and will not produce viral proteins that contribute to viral cytopathic death of the cell. Instead these PD-1+ cells will survive, with the provirus integrated into their cellular genome (33). Further research is required to fully elucidate the mechanistic role PD-1 expression may play regarding the establishment and/or the perpetuation of HIV latency within memory CD4+ T cells. There has been some suggestion that PD-1 expression is indicative of the presence of transcription factors that may inhibit HIV-1 transcription. One such factor is Blimp-1, which has been shown to be associated with exhausted CD4+ T cells in the context of chronic infection (34). This same study reported that exhausted CD4+ T cells correlated with increased expression of PD-1. Another study found Blimp-1 expression to be elevated within memory CD4+ T cells that contribute to the latent HIV reservoir (35). It is therefore conceivable that Blimp-1 and PD-1 expression may facilitate conserving latency in memory T cells.
Collectively, these data indicate that PD-1 is a clear marker of memory CD4+ T cells that harbor latent HIV provirus. However, the question remains as to what proportion of the latent reservoir is represented by cells expressing this marker and whether it is a feasible target in eliminating HIV infection. Already, one study has identified a positive correlation between the percentage of PD-1+ CD4+ T cells and the amount of integrated HIV DNA (PD-1+ CD4+ expression at 64% correlates with HIV DNA levels of ~1100 copies/106 CD4+ T cells) (28). A preliminary study from our laboratory employing full-length sequencing of the integrated HIV genome, using samples obtained from supressed patients, identified PD-1+ CD4+ memory T cells harbor greater proportions of genetically intact provirus in contrast to PD-1− equivalents (preliminary data from our laboratory, data not shown).
Current Latency Reversing Agents
One approach to reduce the latent HIV reservoir is to combine current ART regimes with therapeutics that reactivate the latent form of the virus, a method known as “shock and kill.” The justification for this approach is that for the remaining hidden pool of virus to become visible to both the individual's immune cells and ART, it must be reactivated. This could be accomplished by treating individuals with latency reversing agents (LRA) that result in the transcription of latent, integrated HIV provirus. Various LRA exist, such as histone deacetylase inhibitors (HDACis) and protein kinase C class drugs, with studies having already proved their safety and the ability to increase levels of HIV transcription (36). These agents aim at directly modifying chromatin and reversing transcriptional latency and have been shown in vitro and in vivo to promote histone acetylation and increase HIV transcription, measured as cell-associated unspliced HIV RNA in resting memory CD4+ T cells (37) (Figure 1). Recent studies investigated the impact of the HDACis, vorinostat, panobinostat, and romidepsin upon the genetic composition of the HIV reservoir. HIV RNA sequences isolated from participant cells after therapy with these HDACis were found to be genetically diverse indicating that the drugs stimulated a broad activation of proviruses from latently infected cells (38, 39). However, multiple studies have shown that these drugs fail to significantly decrease the size of the latent reservoir (40). As we now know that some latently infected cells are already in a state of partial activation and express IC molecules (19, 28), it is conceivable that drugs could be used to target these cells via the expression of various markers, such as PD-1 and CTLA-4.
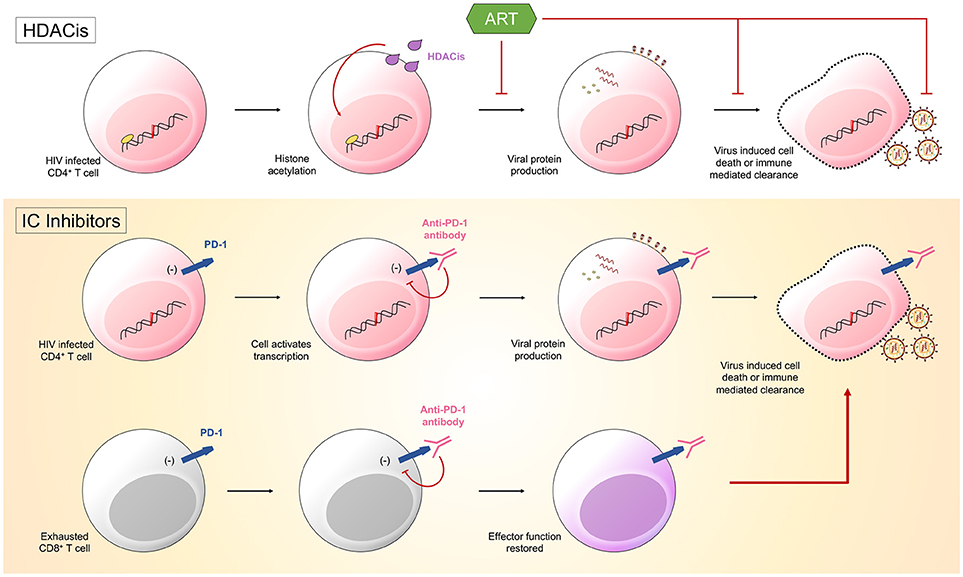
Figure 1. Latency reversing agents (LRA) are one half of the “shock and kill” approach. They function to induce HIV transcription. Histone deacetylase inhibitors (HDACis) promote histone acetylation and induce transcription of viral products. Immune checkpoint (IC) inhibitors could also function as LRA. Anti-PD-1 antibodies bind PD-1 on both HIV infected CD4+ T cells and HIV-specific CD8+ T cells. The binding of PD-1 by the antibody will disinhibit signals sent into the cells by the immune checkpoint molecule. Cells will become transcriptionally active, with CD4+ T cells producing viral products that ultimately result in the death of the cell, and CD8+ T cells having effector function restored, allowing them to target infected CD4+ T cells.
Immune Checkpoint Inhibitors and HIV Infection
There is now a push to optimize IC inhibitors currently used in the treatment of malignancies for the therapeutic treatment of HIV. Hypothetically, treatment would work in two potential ways: through enhancing HIV-specific CD8+ T cell effector function and through reversing HIV latency. It has already been established with SIV rhesus macaque animal models that the administration IC blockades have significant effects, with PD-1 blockade resulting in rapid expansion of SIV-specific CD8+ T cells with increased functionality (41) and CTLA-4 blockade resulting in increases in plasma viremia and T cell activation (42). IC blockades could function to reverse HIV latency through limiting inhibitory signals sent from IC molecules into cells harboring latent HIV. Preventing these inhibitory signals would result in increased gene expression and the subsequent production of viral proteins, causing these cells to become “visible” to both the immune system and ART, as well as susceptible to virus mediated cytotoxicity (33) (Figure 1).
Already there have been both case-reports and clinical trials that have investigated the use of IC blockades to treat malignancies in HIV-infected patients (Table 1). Two recent studies describe an HIV-infected individual who received treatment for melanoma in the form of multiple rounds of a CTLA-4 blocker (48) followed by the single administration of a PD-1 blocker (49). Following the administration of anti-CTLA-4 (ipilimumab), a 20-fold increase in cell-associated HIV RNA was measured in CD4+ T cells. After receiving the single dose of anti-PD-1 antibody (nivolumab), a 25-fold increase in cell-associated HIV RNA was observed (48, 49). These data indicate an increase in HIV transcription and a potential reversal of latency. Moreover, another case report of malignancy in an HIV-infected patient treated using PD-1 blockers demonstrated increased HIV transcription with a concurrent decrease in both reservoir size and PD-1+ CD4+ T cells after dosing (46). Conversely, a recent study analyzed data obtained from HIV-infected patients receiving treatment for NSCLC with a PD-1 blocker and came to different conclusions. Lavolé et al. (44) showed that both the proviral load and CD4+ T cell counts remained stable in patients receiving multiple doses of nivolumab, suggesting the drug does not function as an LRA as efficiently as previously thought (44). Furthermore, a second study found no consistent changes in either virological or immunological status in HIV-infected participants treated for malignancy with PD-1 blockers (45). However, it is worth noting that these studies all enrolled small cohorts (between 1 and 10 participants) and investigated doses that were optimized for the treatment of cancer. Only one study to date has explored IC inhibitors for the treatment of HIV in participants without any malignancies, using an anti-PD-L1 antibody. This study, a phase-I dose-escalation, observed increased Gag-specific CD8+ T cells in 2 of the 6 dosed participants. As this study was performed to assess the safety of the therapeutic, a low dose was used (0.3-mg/kg) and participants were monitored for adverse events (50). However, the investigation was halted due to observed retinal toxicities seen in concurrent macques studies. It is worth noting that IC inhibitors are associated with relative toxicities, with adverse events (AE) ranging from mild (diarrhea, fatigue, rash) to more severe (pancreatitis, nephritis, neurological conditions) (51). Future investigations should be cautious in their approach in regard to these AE.
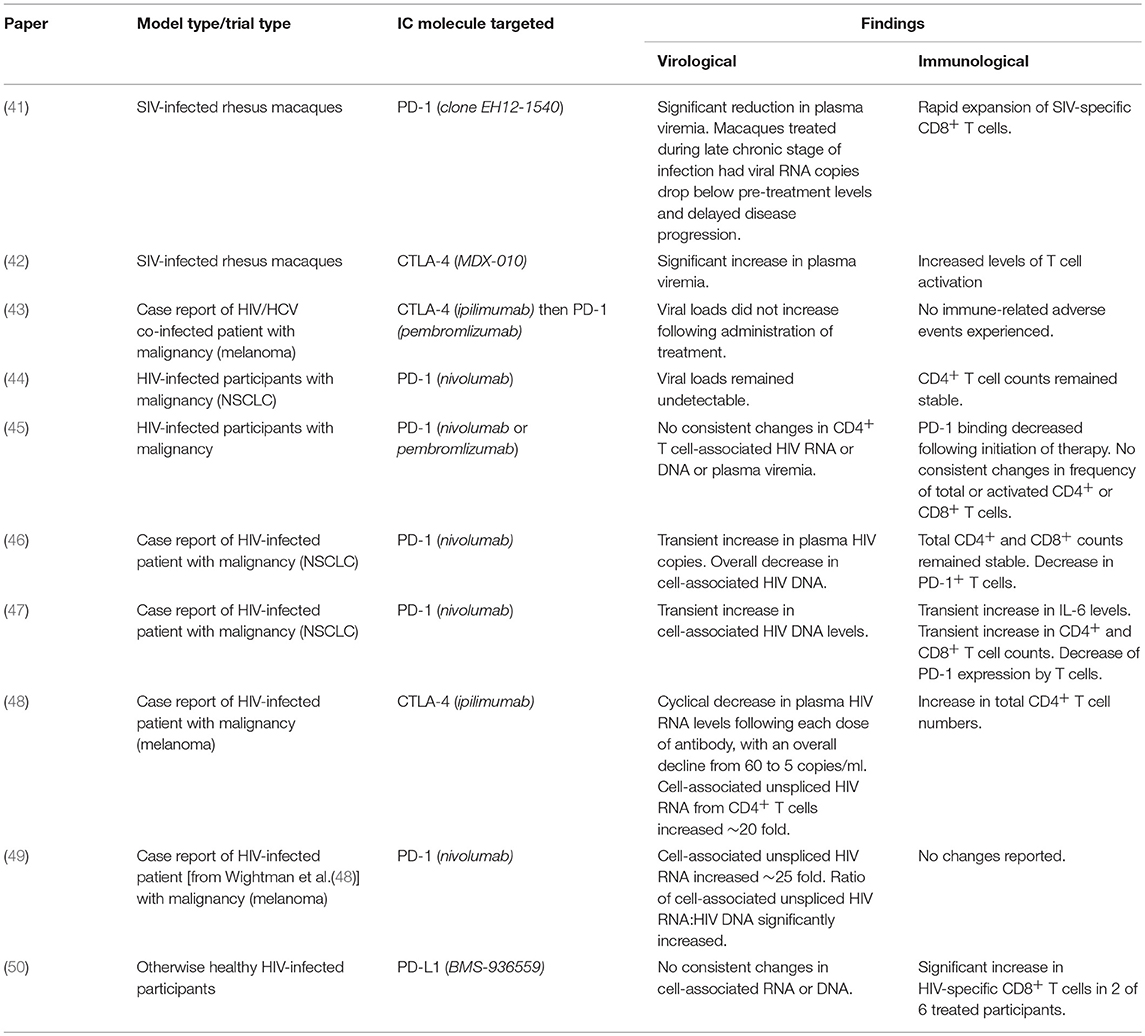
Table 1. Summary of published trials and case-reports investigating immune checkpoint inhibitors in HIV.
As CD4+ T cells that express both single and multiple IC molecules are enriched for HIV DNA (28), it is unlikely that an effective HIV-directed immunotherapy would involve only a single IC inhibitor. Rather, a combination of multiple blockers would be required for a synergistic approach to target all cells harboring latent HIV. The efficacy of combination immunotherapy has already been established in the treatment of NSCLC and other malignancies (52). However, the use of multiple IC inhibitors is associated with an increase in AE when compared with therapy using only one IC inhibitor (51). Furthermore, further research into exploiting other IC molecules, such as TIGIT and LAG-3, as targets for a potential HIV LRA is warranted, as cells expressing these markers contain integrated HIV DNA (28).
Conclusion
Any curative strategy for HIV will need to eliminate the functioning latent reservoir. Previous approaches have used LRA, such as HDACis, to reawaken and eliminate cells latently infected with HIV. However these drugs do not decrease the size of the latent reservoir (40). As HIV latency research continues, new markers of latently infected cells are being identified (3, 17). IC molecules, such as PD-1, are promising therapeutic targets for the elimination of the HIV latent reservoir, as CD4+ T cells expressing IC molecules harbor latent, replication-competent HIV (13, 20, 28, 30). IC inhibitors are already used in a clinical setting and have transformed the treatment of cancer. Further investigation into these drugs, their effectiveness in treating HIV and the adverse immune events they could potentially cause is warranted. Furthermore, the impact of administering multiple classes of IC inhibitors should be investigated as it is unlikely that a single class will be sufficient to reactivate the HIV reservoir.
Author Contributions
Both ZB and SP made a significant and intellectual contribution to the work and approved it for publication.
Conflict of Interest Statement
The authors declare that the research was conducted in the absence of any commercial or financial relationships that could be construed as a potential conflict of interest.
Acknowledgments
This work was supported the Delaney AIDS Research Enterprise (DARE) to Find a Cure (1U19AI096109 and 1UM1AI126611-01) and the Australian National Health and Medical Research Council (AAP1061681).
References
1. Ho Y-C, Shan L, Hosmane NN, Wang J, Laskey SB, Rosenbloom DIS, et al. Replication-competent noninduced proviruses in the latent reservoir increase barrier to HIV-1 cure. Cell (2013) 155:540–51. doi: 10.1016/j.cell.2013.09.020
2. Bruner KM, Murray AJ, Pollack RA, Soliman MG, Laskey SB, Capoferri AA, et al. Defective proviruses rapidly accumulate during acute HIV-1 infection. Nat Med. (2016) 22:1043–9. doi: 10.1038/nm.4156
3. Hiener B, Horsburgh BA, Eden J-S, Barton K, Schlub TE, Lee E, Stockenstrom von S, et al. Identification of genetically intact HIV-1 proviruses in specific CD4 + T cells from effectively treated participants. Cell Rep. (2017) 21:813–22. doi: 10.1016/j.celrep.2017.09.081
4. Siliciano JD, Kajdas J, Finzi D, Quinn TC, Chadwick K, Margolick JB, et al. Long-term follow-up studies confirm the stability of the latent reservoir for HIV-1 in resting CD4+ T cells. Nat Med. (2003) 9:727–8. doi: 10.1038/nm880
5. Pinkevych M, Cromer D, Tolstrup M, Grimm AJ, Cooper DA, Lewin SR, et al. HIV reactivation from latency after treatment interruption occurs on average every 5-8 days–implications for HIV remission. PLoS Pathog. (2015) 11:e1005000. doi: 10.1371/journal.ppat.1005000
6. Chun TW, Engel D, Berrey MM, Shea T, Corey L, Fauci AS. Early establishment of a pool of latently infected, resting CD4(+) T cells during primary HIV-1 infection. Proc Natl Acad Sci USA. (1998) 95:8869–73.
7. Colby DJ, Trautmann L, Pinyakorn S, Leyre L, Pagliuzza A, Kroon E, et al. Rapid HIV RNA rebound after antiretroviral treatment interruption in persons durably suppressed in Fiebig I acute HIV infection. Nat Med. (2018) 24:923–6. doi: 10.1038/s41591-018-0026-6
8. Palmer S, Maldarelli F, Wiegand A, Bernstein B, Hanna GJ, Brun SC, et al. Low-level viremia persists for at least 7 years in patients on suppressive antiretroviral therapy. Proc Natl Acad Sci USA. (2008) 105:3879–84. doi: 10.1073/pnas.0800050105
9. Palmer S, Josefsson L, Coffin JM. HIV reservoirs and the possibility of a cure for HIV infection. J Intern Med. (2011) 270:550–60. doi: 10.1111/j.1365-2796.2011.02457.x
10. Josefsson L, Palmer S, Faria NR, Lemey P, Casazza J, Ambrozak D, et al. Single cell analysis of lymph node tissue from HIV-1 infected patients reveals that the majority of CD4+ T-cells contain one HIV-1 DNA molecule. PLoS Pathog. (2013) 9:e1003432. doi: 10.1371/journal.ppat.1003432
11. Stockenstrom von S, Odevall L, Lee E, Sinclair E, Bacchetti P, Killian M, et al. Longitudinal genetic characterization reveals that cell proliferation maintains a persistent HIV Type 1 DNA pool during effective HIV therapy. J Infect Dis. (2015) 212:596–607. doi: 10.1093/infdis/jiv092
12. Finzi D, Blankson J, Siliciano JD, Margolick JB, Chadwick K, Pierson T, et al. Latent infection of CD4+ T cells provides a mechanism for lifelong persistence of HIV-1, even in patients on effective combination therapy. Nat Med. (1999) 5:512–7. doi: 10.1038/8394
13. Chomont N, El-Far M, Ancuta P, Trautmann L, Procopio FA, Yassine-Diab B, et al. HIV reservoir size and persistence are driven by T cell survival and homeostatic proliferation. Nat Med. (2009) 15:893–900. doi: 10.1038/nm.1972
14. Soriano-Sarabia N, Bateson RE, Dahl NP, Crooks AM, Kuruc JD, Margolis DM, et al. Quantitation of replication-competent HIV-1 in populations of resting CD4+ T cells. J Virol. (2014) 88:14070–7. doi: 10.1128/JVI.01900-14
15. Lee SA, Bacchetti P, Chomont N, Fromentin R, Lewin SR, O'Doherty U, et al. Anti-HIV Antibody Responses and the HIV Reservoir Size during Antiretroviral Therapy. PLoS ONE (2016) 11:e0160192. doi: 10.1371/journal.pone.0160192
16. Imamichi H, Dewar RL, Adelsberger JW, Rehm CA, O'Doherty U, Paxinos EE, et al. Defective HIV-1 proviruses produce novel protein-coding RNA species in HIV-infected patients on combination antiretroviral therapy. Proc Natl Acad Sci USA. (2016) 113:8783–8. doi: 10.1073/pnas.1609057113
17. Horsburgh BA, Hiener B, Eden J-S, Lee E, Schlub TE, Stockenstrom von S, et al. Intact HIV-1 provirus is enriched in effector memory and HLA-DR+ memory CD4+ T cells. In: IAS Conference on HIV Science, Paris (2017).
18. Esser MT, Bess JW, Suryanarayana K, Chertova E, Marti D, Carrington M, et al. Partial activation and induction of apoptosis in CD4(+) and CD8(+) T lymphocytes by conformationally authentic noninfectious human immunodeficiency virus type 1. J Virol. (2001) 75:1152–64. doi: 10.1128/JVI.75.3.1152-1164.2001
19. Cockerham LR, Siliciano JD, Sinclair E, O'Doherty U, Palmer S, Yukl SA, et al. CD4+ and CD8+ T Cell activation are associated with HIV DNA in resting CD4+ T cells. PLoS ONE (2014) 9:e110731. doi: 10.1371/journal.pone.0110731
20. Hurst J, Hoffmann M, Pace M, Williams JP, Thornhill J, Hamlyn E, et al. Immunological biomarkers predict HIV-1 viral rebound after treatment interruption. Nat Commun. (2015) 6:8495. doi: 10.1038/ncomms9495
22. Ozkazanc D, Yoyen-Ermis D, Tavukcuoglu E, Buyukasik Y, Esendagli G. Functional exhaustion of CD4 +T cells induced by co-stimulatory signals from myeloid leukaemia cells. Immunology (2016) 149:460–71. doi: 10.1111/imm.12665
23. Yi JS, Cox MA, Zajac AJ. T-cell exhaustion: characteristics, causes and conversion. Immunology (2010) 129:474–81. doi: 10.1111/j.1365-2567.2010.03255.x
24. Dyck L, Mills KHG. Immune checkpoints and their inhibition in cancer and infectious diseases. Eur J Immunol. (2017) 47:765–79. doi: 10.1002/eji.201646875
25. D'Souza M, Fontenot AP, Mack DG, Lozupone C, Dillon S, Meditz A, et al. Programmed death 1 expression on HIV-specific CD4+ T cells is driven by viral replication and associated with T cell dysfunction. J Immunol. (2007) 179:1979–87. doi: 10.4049/jimmunol.179.3.1979
26. Rallón N, García M, García-Samaniego J, Cabello A, Álvarez B, Restrepo C, et al. Expression of PD-1 and Tim-3 markers of T-cell exhaustion is associated with CD4 dynamics during the course of untreated and treated HIV infection. PLoS ONE (2018) 13:e0193829. doi: 10.1371/journal.pone.0193829
27. Kaufmann DE, Walker BD. PD-1 and CTLA-4 inhibitory cosignaling pathways in HIV infection and the potential for therapeutic intervention. J Immunol. (2009) 182:5891–7. doi: 10.4049/jimmunol.0803771
28. Fromentin R, Bakeman W, Lawani MB, Khoury G, Hartogensis W, DaFonseca S, et al. CD4+ T Cells Expressing PD-1, TIGIT and LAG-3 Contribute to HIV Persistence during ART. PLoS Pathog. (2016) 12:e1005761. doi: 10.1371/journal.ppat.1005761
29. Banga R, Procopio FA, Noto A, Pollakis G, Cavassini M, Ohmiti K, et al. PD-1(+) and follicular helper T cells are responsible for persistent HIV-1 transcription in treated aviremic individuals. Nat Med. (2016) 22:754–61. doi: 10.1038/nm.4113
30. McGary CS, Deleage C, Harper J, Micci L, Ribeiro SP, Paganini S, et al. CTLA-4+PD-1- memory CD4+ T cells critically contribute to viral persistence in antiretroviral therapy-suppressed, SIV-infected rhesus macaques. Immunity (2017) 47:776–788.e5. doi: 10.1016/j.immuni.2017.09.018
31. Lin S-J, Peacock CD, Bahl K, Welsh RM. Programmed death-1 (PD-1) defines a transient and dysfunctional oligoclonal T cell population in acute homeostatic proliferation. J Exp Med. (2007) 204:2321–33. doi: 10.1084/jem.20062150
32. Hokey DA, Johnson FB, Smith J, Weber JL, Yan J, Hirao L, et al. Activation drives PD-1 expression during vaccine-specific proliferation and following lentiviral infection in macaques. Eur J Immunol. (2008) 38:1435–45. doi: 10.1002/eji.200737857
33. Wykes MN, Lewin SR. Immune checkpoint blockade in infectious diseases. Nat Rev Immunol. (2018) 18:91–104. doi: 10.1038/nri.2017.112
34. Crawford A, Angelosanto JM, Kao C, Doering TA, Odorizzi PM, Barnett BE, et al. Molecular and transcriptional basis of CD4? T cell dysfunction during chronic infection. Immunity (2014) 40:289–302. doi: 10.1016/j.immuni.2014.01.005
35. Kaczmarek Michaels K, Natarajan M, Euler Z, Alter G, Viglianti G, Henderson AJ. Blimp-1, an intrinsic factor that represses HIV-1 proviral transcription in memory CD4+ T cells. J Immunol. (2015) 194:3267–74. doi: 10.4049/jimmunol.1402581
36. Rasmussen TA, Tolstrup M, Brinkmann CR, Olesen R, Erikstrup C, Solomon A, et al. Panobinostat, a histone deacetylase inhibitor, for latent-virus reactivation in HIV-infected patients on suppressive antiretroviral therapy: a phase 1/2, single group, clinical trial. Lancet HIV (2014) 1:e13–21. doi: 10.1016/S2352-3018(14)70014-1
37. Rasmussen TA, Lewin SR. Shocking HIV out of hiding. Curr Opin HIV AIDS (2016) 11:394–401. doi: 10.1097/COH.0000000000000279
38. Barton K, Hiener B, Winckelmann A, Rasmussen TA, Shao W, Byth K, et al. Broad activation of latent HIV-1 in vivo. Nat Commun. (2016) 7:12731. doi: 10.1038/ncomms12731
39. Winckelmann A, Barton K, Hiener B, Schlub TE, Shao W, Rasmussen TA, et al. Romidepsin-induced HIV-1 viremia during effective antiretroviral therapy contains identical viral sequences with few deleterious mutations. AIDS (2017) 31:771–9. doi: 10.1097/QAD.0000000000001400
40. Elliott JH, Wightman F, Solomon A, Ghneim K, Ahlers J, Cameron MJ, et al. Activation of HIV transcription with short-course vorinostat in HIV-infected patients on suppressive antiretroviral therapy. PLoS Pathog. (2014) 10:e1004473. doi: 10.1371/journal.ppat.1004473
41. Velu V, Titanji K, Zhu B, Husain S, Pladevega A, Lai L, et al. Enhancing SIV-specific immunity in vivo by PD-1 blockade. Nature (2009) 458:206–10. doi: 10.1038/nature07662
42. Cecchinato V, Tryniszewska E, Ma ZM, Vaccari M, Boasso A, Tsai WP, et al. Immune activation driven by CTLA-4 blockade augments viral replication at mucosal sites in simian immunodeficiency virus infection. J Immunol. (2008) 180:5439–47. doi: 10.4049/jimmunol.180.8.5439
43. Davar D, Wilson M, Pruckner C, Kirkwood JM. PD-1 Blockade in advanced melanoma in patients with hepatitis C and/or HIV. Case Rep Oncol Med. (2015) 2015:1–5. doi: 10.1155/2015/737389
44. Lavolé A, Guihot A, Veyri M, Lambotte O, Autran B, Cloarec N, et al. PD-1 blockade in HIV-infected patients with lung cancer: a new challenge or already a strategy? Ann Oncol. (2018) 29:1065–6. doi: 10.1093/annonc/mdx817
45. Scully EP, Rutishauser RL, Simoneau CR, Delagrèverie H, Euler Z, Thanh C, et al. Inconsistent HIV reservoir dynamics and immune responses following anti-PD-1 therapy in cancer patients with HIV infection. Ann Oncol. (2018) doi: 10.1093/annonc/mdy259. [Epub ahead of print].
46. Guihot A, Marcelin AG, Massiani MA, Samri A, Soulié C, Autran B, et al. Drastic decrease of the HIV reservoir in a patient treated with nivolumab for lung cancer. Ann Oncol. (2017) 29:517–8. doi: 10.1093/annonc/mdx696
47. Le Garff G, Samri A, Lambert-Niclot S, Even S, Lavolé A, Cadranel J, et al. Transient HIV-specific T cells increase and inflammation in an HIV-infected patient treated with nivolumab. AIDS (2017) 31:1048–51. doi: 10.1097/QAD.0000000000001429
48. Wightman F, Solomon A, Kumar SS, Urriola N, Gallagher K, Hiener B, et al. Effect of ipilimumab on the HIV reservoir in an HIV-infected individual with metastatic melanoma. AIDS (2015) 29:504–6. doi: 10.1097/QAD.0000000000000562
49. Evans VA, Van Der Sluis RM, Solomon A, Dantanarayana A, McNeil C, Garsia R, et al. PD-1 contributes to the establishment and maintenance of HIV-1 latency. AIDS (2018) 32:1491–7. doi: 10.1097/QAD.0000000000001849
50. Gay CL, Bosch RJ, Ritz J, Hataye JM, Aga E, Tressler RL, et al. Clinical Trial of the Anti-PD-L1 Antibody BMS-936559 in HIV-1 infected participants on suppressive antiretroviral therapy. J Infect Dis. (2017) 215:1725–33. doi: 10.1093/infdis/jix191
51. Day D, Hansen AR. Immune-related adverse events associated with immune checkpoint inhibitors. BioDrugs (2016) 30:571–84. doi: 10.1007/s40259-016-0204-3
Keywords: memory T cells, T cell exhaustion, immune checkpoint molecules, PD-1, CTLA-4, latent HIV reservoir
Citation: Boyer Z and Palmer S (2018) Targeting Immune Checkpoint Molecules to Eliminate Latent HIV. Front. Immunol. 9:2339. doi: 10.3389/fimmu.2018.02339
Received: 01 August 2018; Accepted: 20 September 2018;
Published: 15 October 2018.
Edited by:
John Frater, University of Oxford, United KingdomReviewed by:
Stephen Kent, The University of Melbourne, AustraliaMirko Paiardini, Emory University School of Medicine, United States
Copyright © 2018 Boyer and Palmer. This is an open-access article distributed under the terms of the Creative Commons Attribution License (CC BY). The use, distribution or reproduction in other forums is permitted, provided the original author(s) and the copyright owner(s) are credited and that the original publication in this journal is cited, in accordance with accepted academic practice. No use, distribution or reproduction is permitted which does not comply with these terms.
*Correspondence: Zoe Boyer, em9lLmJveWVyQHN5ZG5leS5lZHUuYXU=
Sarah Palmer, c2FyYWgucGFsbWVyQHN5ZG5leS5lZHUuYXU=