- Faculty of Medicine, Center for Chronic Immunodeficiency, Medical Center – University of Freiburg, Freiburg, Germany
The BAFF-receptor (BAFFR) is encoded by the TNFRSF13C gene and is one of the main pro-survival receptors in B cells. Its function is impressively documented in humans by a homozygous deletion within exon 2, which leads to an almost complete block of B cell development at the stage of immature/transitional B cells. The resulting immunodeficiency is characterized by B-lymphopenia, agammaglobulinemia, and impaired humoral immune responses. However, different from mutations affecting pathway components coupled to B cell antigen receptor (BCR) signaling, BAFFR-deficient B cells can still develop into IgA-secreting plasma cells. Therefore, BAFFR deficiency in humans is characterized by very few circulating B cells, very low IgM and IgG serum concentrations but normal or high IgA levels.
BAFFR Structure and Expression
Structurally, BAFFR is an atypical representative of the TNF-receptor super-family. Members of this family are typically characterized by several extracellular cysteine-rich domains (CRDs), which serve for ligand binding as well as for ligand-independent assembly of receptor monomers into dimers, trimers or multimers (1–6). Unlike most other TNF-R family members, BAFFR contains only a partial CRD which serves for ligand binding as well as for self-assembly (7).
B lymphocyte development in human bone marrow proceeds through successive stages that are defined by the immunoglobulin gene rearrangement process. After the assembly and cell surface expression of functional IgM molecules, immature IgM+ B cells are tested for their reactivity with self-antigens, which eventually can be corrected by receptor editing. Then, transitional B cells with low avidity to self-antigens can leave the bone marrow, enter the circulation, and migrate to the spleen where they complete these early steps of B cell development [reviewed in (8)]. BAFFR [BAFFR = B cell activating f actor of the TNF-f amily receptor (9); a.k.a. BR3, Bcmd (10–12)] expression starts when the immature B cells develop to transitional B cells (13, 14), which then receive BAFFR-dependent pro-survival signals to rescue them from premature cell death (15–17). At protein level, the BAFFR is expressed on the surface of all human peripheral B cell subsets except for plasma cells and for centroblasts located in the dark zone of germinal centers. Its expression is upregulated after the expression of functional B cell antigen receptors (BCR) in response to tonic BCR signaling, which enhances BAFFR expression by immature and transitional B cells (15, 18). Although the induction of BAFFR expression seems to depend on the expression of functional B cell receptors, it can be maintained in mice after the ablation of SYK, a key element of BCR signaling (19), as well as in cells which were depleted from Ig-α (CD79A), an essential BCR component (20), supporting in both cases the survival of B cells with impaired BCR-signaling. Figure 1 summarizes the development of immature/transitional B cells and the expression of receptors for BAFF in the different subsets.
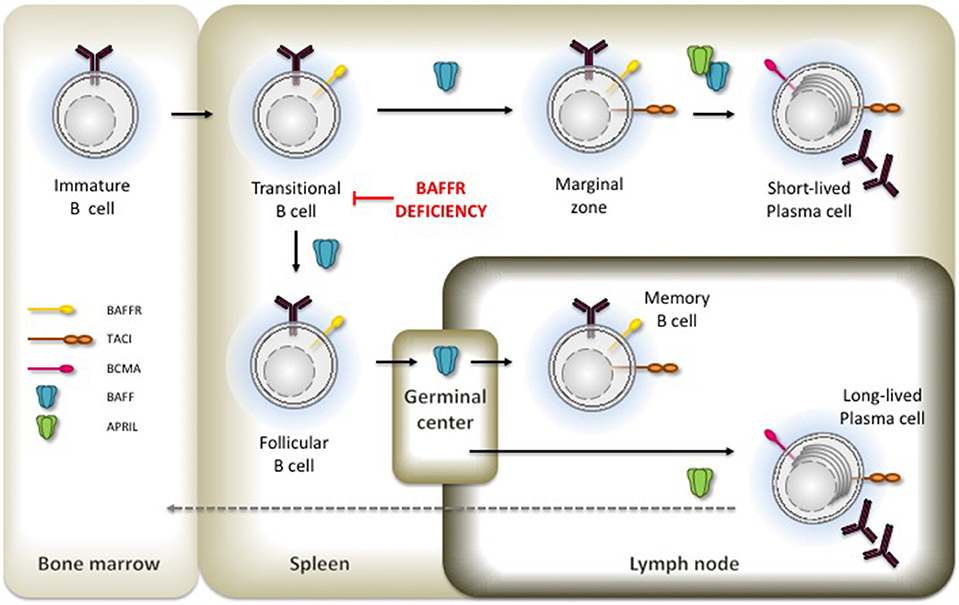
Figure 1. Expression of BAFFR. TACI and BCMA in B cell development. Critical developmental steps depending on BAFF and APRIL-induced signals are shown by the presence of the respective ligand.
Ligand Binding
BAFFR binds the TNF-like molecule BAFF [BAFF = B cell activating f actor of the TNF-f amily (21); a.k.a. BLYS (22), TALL-1 (23), zTNF4(24)] as single ligand (9, 12, 25). In contrast, the two other closely related, BAFF-binding receptors TACI (T cell activator and calcium modulating ligand interactor) and BCMA (B cell maturation antigen) (24) can additionally bind to APRIL [a proliferation-inducing ligand, (26)], another member of the TNF-α family, which supports the survival of plasma cells (27). TACI is expressed by activated B cells, marginal zone B cells, switched memory B cells and by plasma cells (24, 28–30). Compared to BAFFR, TACI has different functions by serving on the one hand as decoy receptor (31) while triggering, on the other hand, immunoglobulin class-switch recombination (32). Different from BAFFR and TACI, BCMA is upregulated in activated B cells and expressed constitutively by long-lived plasma cells supporting their survival (33). Both ligands, BAFF (21, 22) and APRIL (26) are type II transmembrane proteins forming homo- as well as heterotrimers (22, 34–36). BAFF is expressed as membrane-bound ligand (see also contribution of Kowalczyk-Quintas et al. to this Research Topic), which is then processed by the membrane-bound protease furin resulting in a soluble form. Soluble BAFF exists as trimer and can associate into a virus-like capsid called 60-mer, composed of 20 trimeric units (35, 37, 38). The assembly of 60-mers depends on trimer-trimer interactions mediated by a small loop called the “flap” (34, 35). Without the flap region BAFF can still assemble into trimers, which can bind to BAFFR but this form of BAFF does neither initiate downstream signaling cascades nor does it support B cell survival. Therefore, crosslinking of multiple BAFF-BAFFR complexes via the flap-region is essential for BAFFR-dependent B cell responses (39).
BAFF and APRIL are expressed by monocytes, macrophages, dendritic cells, bone marrow stroma cells (21, 23, 40), and by T cells (21, 41). The expression of both ligands increases under pro-inflammatory conditions (42) and correlates inversely with the expression of BAFFR and TACI (43).
BAFFR Signaling and Target Genes
BAFF binding to BAFFR activates several downstream pathways that regulate basic survival functions including protein synthesis and energy metabolism required to extend the half-life of immature, transitional, and mature B cells.
Like the TNF-receptor family members CD40, LTβR, and RANK, BAFFR triggers the non-canonical NF-κB2-dependent pathway (44, 45). NF-κB2 belongs together with NF-κB1, RelA, RelB, and c-Rel to the group of NF-κB/Rel transcription factors [reviewed in (46)]. Activation of NF-κB1 is a very rapid process (47). Upon MAP3K7 (TAK1)-dependent phosphorylation, IKKα, IKKβ, IKKγ assemble into the inhibitor of kappa-B kinase (IKK) complex and phosphorylate IkBα, the inhibitor of NF-κB1. This allows its proteasomal degradation and nuclear translocation of NF-κB p50/relA and p50-c-rel heterodimers, which are constantly generated by the association between the processed form of NF-κB1/p105 and relA or c-Rel. In the nucleus, the NF-κB heterodimers mainly act as transcriptional activators regulating a plethora of target genes including the gene for NF-κB2 (48).
Different from NF-κB1, the activation of the non-canonical NF-κB2 pathway by BAFF is a slow and complex process (44) which relies on the activation of the NF-κB inducing kinase NIK (MAP3K14) (49, 50). In the absence of BAFF (or of other NF-κB2-inducing factors), NIK binds TRAF3, which promotes the proteasomal degradation of the kinase after its ubiquitinylation by a complex with ubiquitin-E3 ligase activity composed of the cellular inhibitors of apoptosis cIAP1 and cIAP2, TRAF2 and TRAF3 (51). NIK degradation prevents the accumulation of the kinase and the phosphorylation of NF-κB2 by IKKα (IKK1), which itself is activated through phosphorylation by NIK (52). When BAFF binds to BAFFR, it leads to the aggregation of BAFF receptors which recruit TRAF3 to their intracellular part. This allows the dissociation of the NIK-TRAF2/3-cIAP1/2 complex (53–55), exposes the TRAF3 lysine residue K46 to ubiquitin ligases and allows proteasomal degradation of TRAF3 (56). By reducing the number of available TRAF3 molecules, newly synthesized NIK can accumulate (53) and phosphorylate IKK1 (52). The active form of IKK1 then phosphorylates NF-κB2 p100 at the C-terminal serine residues 866 and 870 (50), which now becomes a target of the E3 ubiquitin ligase βTrCP, which adds ubiquitin to lysine residue K856. Ubiquitinylated NF-κB2 then binds to the regulatory subunit of the proteasome (56) promoting the cleavage of the p100 precursor into the active p52 form which forms an heterodimer with relB and translocates into the nucleus to regulate the transcription of NF-κB2 target genes. The complexity of this multi-step reaction, which is dependent on the relative concentration of TRAF and its association with BAFFR (57) explains why BAFF-induced activation of NF-κB2 target genes is a slow process.
Downstream of NF-κB2 several target genes have been identified. These include ICOSL, a co-stimulatory ligand for ICOS, which is expressed by activated T cells, provides co-stimulatory signals, and promotes the development of follicular T-helper cells (58). Analysis of B cells from NIK-deficient patients underlines the role of NIK and NF-κB2 in activating ICOSL expression as NIK-deficient cells do not upregulate ICOSL in response to CD40L (59).
As pointed out above, BAFF binding to BAFFR recruits TRAF3 to the receptor reducing the concentrations of available TRAF3. This allows—similar to the accumulation of NIK—the accumulation of the transcriptional regulator “cAMP response element binding protein” CREB, which in resting cells is initially complexed to TRAF3 inducing its ubiquitinylation and degradation. Accumulation of CREB increases the expression of its target gene Mcl-1 (60), a Bcl-family member with powerful anti-apoptotic activity, which acts by stabilizing the mitochondrial outer membrane (61). The deubiquitinating enzyme OTUD7B represents another NF-κB2 target gene (62). Activation of BAFFR increases OTUD7B expression and the newly synthesized deubiquitinase then binds to TRAF3 resulting in the formation of a high molecular weight complex including OTUDB7, TRAF3, TRAF2, and cIAP1/2. This leads to the deubiqutinylation and stabilization of TRAF3, which now can bind and inactivate NIK again resulting in the downregulation of BAFFR signaling. Thus, OTUB7 acts as negative regulator of the NF-κB2 and limits BAFFR-dependent cellular activation (62).
In addition to NF-κB2 signaling, binding of BAFF to BAFFR activates the phosphoinositide-3-kinase-dependent signaling cascade. An elegant series of experiments carried out in mice demonstrated that activation PI3K pathway by BAFFR makes use of components belonging to the B cell antigen receptor pathway (63, 64). Similar to the B cell receptor (65), BAFFR-induced signals remodel the cytoskeleton by interacting with a network which includes the tetraspanin CD81, the co-receptor CD19 and the Wiscott-Aldrich syndrome interacting protein WIP (66). This suggests that BAFFR seems to be part of a large complex of transmembrane and membrane-associated proteins using common signaling components that are activated in a context-dependent manner. Downstream of PI3K, the AKT/mTOR axis initiates the metabolic reprogramming of B cells resulting in an increased cellular fitness and lifespan [reviewed in (67)]. In mice, BAFF induces the PI3K-depenent phosphorylation of AKT at both Ser437 and T308 (68, 69) allowing the phosphorylation of downstream substrates including GSK3β, the transcription factor FOXO, the small ribosomal subunit protein S6 and the translation inhibitor 4EBP1. Since phosphorylated S6 activates while non-phosphorylated 4EBP1 inhibits translation, BAFFR is an important regulator of protein synthesis. In addition to protein synthesis, BAFFR-dependent activation of the PI3K pathway stabilizes MCL-1 by phosphorylating GSK3β and PIM2 (61, 68, 69), leading to enhanced mitochondrial function and an increase in ATP production. Figure 2 summarizes essential features of BAFFR signaling.
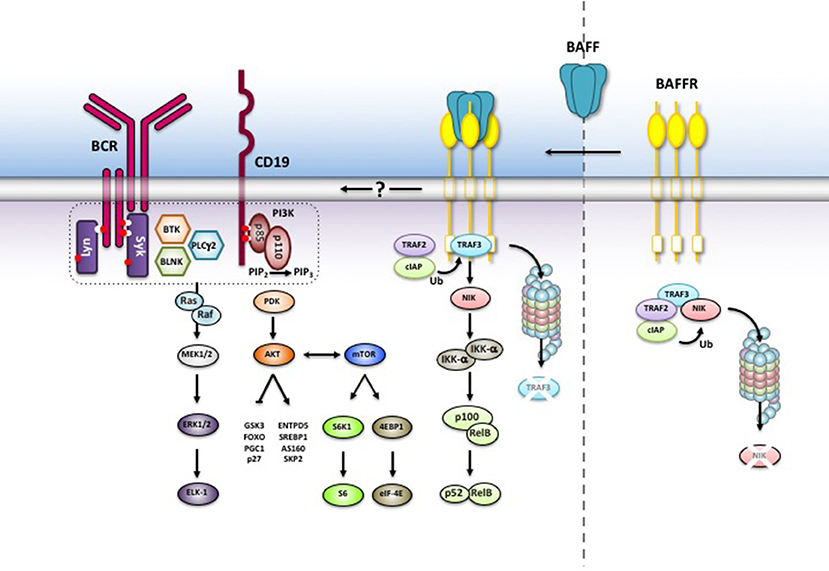
Figure 2. BAFFR-induced intracellular signaling. Without BAFF, NIK is complexed to TRAF3 and degraded in the proteasome. BAFF binding to BAFFR recruits TRAF3 to BAFFR and stabilizes NIK while TRAF3 is degraded by the proteasome. NIK activates the non-canonical NF-κB2 signaling pathway and allows nuclear translocation of NF-κB2 p52/relB heterodimers. BAFF binding also activates the PI3K pathway, which shares common components with BCR signaling. The exact mechanisms leading to PI3K activation are still not understood.
BAFFR Processing
Besides the activation of several downstream signaling pathways BAFF binding to BAFFR causes the shedding of the extracellular part of BAFFR (70). The proteolytic cleavage of BAFFR is catalyzed by the metalloprotease ADAM10 and requires the co-expression of TACI. Since TACI is expressed by marginal zone and by switched memory B cells, BAFFR shedding by ADAM10 in response to BAFF binding affects mainly these B cell subsets. After the extracellular part of BAFFR has been released, its transmembrane part and the cytoplasmic domain are internalized and translocated to lysosomes where they are most likely degraded. Thus, BAFFR processing differs from the shedding of TACI, which is cleaved constitutively by ADAM10 in the absence of ligand. Processing of TACI removes its extracellular domain, which can act as decoy receptor for both BAFF and APRIL (31). Similar to TACI, BCMA is also processed constitutively, not by ADAM proteases but by γ-secretase (71). As it has been described for Notch, γ-secretase also cleaves the part of TACI which remains in the plasma membrane after the extracellular part has been shed by ADAM10 (31), but it remains to be shown if the released intracellular form of TACI has a biological function analogous to the intracellular part of Notch, which participates directly in the transcriptional regulation of target genes (72).
Since BAFFR shedding is induced by ligand binding to BAFFR, the released extracellular domain of BAFFR is most likely still complexed with BAFF preventing its function as soluble decoy receptor like soluble TACI or BCMA. If BAFF dissociates from soluble BAFFR, the soluble form of BAFFR most likely also looses its ligand binding activity, which also argues against its decoy receptor function. As a result of the constitutive shedding of TACI and BCMA, the surface expression levels of both receptors are rather low (31, 71), and differs from BAFFR expression levels, which are high on all B cell subsets except for germinal center B cells (70).
Germinal center B cells undergo proliferation, somatic hypermutation and immunoglobulin class-switch recombination in the dark zone, from where they migrate to the light zone, where they are selected by their affinity to their cognate antigen into the switched memory B cell and long-lived plasma cell pool (73). While light zone B cells express close to normal levels of BAFFRs which are not bound to BAFF, BAFFRs expressed by dark zone B cells are heavily loaded with BAFF inducing BAFFR-dependent survival signals as well as BAFFR processing by ADAM17. Thus, while BAFF-induced BAFFR processing limits initial survival signals for dark zone cells (70), the survival of light zone B cells is regulated by the affinity of their surface immunoglobulins, which has to be above the thresholds set by interacting T-follicular helper cells and by the competing IgG and IgA antibodies secreted by plasma cells surrounding the B cell follicle (74).
BAFFR and B Cell Survival
From the analysis of the BAFFR encoding Bcmd mutation discovered in the A/WySnJ mouse strain (10, 11) and after the identification of BAFF as pro-survival cytokine for B cells (21, 75) it became clear that both proteins form a ligand-receptor pair which is essential for B cell survival (9, 12). Of interest, the different mouse models revealed that not all B cell subsets are equally dependent on BAFFR-induced survival signals. While Baffr-deficient A/WySnJ or Baff- and Baffr-KO mice had much less follicular and marginal zone B cells (B2 B cells) than the corresponding controls, the inactivation of the Baff or Baffr genes did not affect the population of peritoneal B1 B cells (11, 25, 76). In the mouse, B1 cells form a distinct, innate-like B cell subset, which develops before and shortly after birth and is maintained by self-renewal through limited proliferation but not, as follicular and marginal zone B cells, by de novo generation from hematopoietic precursor cells [reviewed in (77, 78)]. Apart from differences in CD5 expression, B1 B cells can be separated into two subsets by the expression of plasma cell alloantigen (PC1; a.k.a ectonucleotide pyrophosphatase phosphodiesterase 1; ENPP1). PC1low B1 cells develop from early B1 precursor cells during fetal life and differentiate in the gut into IgA secreting plasma cells (79). Interestingly, Baff- and Baffr-deficient mice as well as BAFFR-deficient humans have decreased titers of serum IgG and IgM but not of IgA (11, 25, 76, 80), which may be due to the differentiation of BAFF-independent PC1low B1 cells developing in the gut into IgA secreting plasma cells. Similar to genetic ablation of BAFF and BAFFR expression, injection of anti-BAFF (81) or anti-BAFFR antibodies (82) or of TACI-Ig (83, 84) resulted in the depletion of transitional-2, follicular and of marginal zone B cells but spared pro- and pre-B cells, immature, and transitional-1 B cells. In addition, these depletion experiments showed that switched memory B cells and plasma cells can survive after depleting BAFF or blocking BAFF-BAFFR interactions. Thus, the development and maintenance of the follicular and marginal zone B cell pool strongly depends on BAFF and BAFFR, whereas B1 B cells and switched memory B cells can survive without BAFF, although they express similar levels of BAFFR as the BAFF-dependent subsets. Future studies have to reveal, why BAFFR is expressed on these cells and if it has any function.
The Role of BAFFR in B Cell Selection and Autoimmunity
BAFFR expression starts in the bone marrow when B cells become IgM+ immature B cells, which undergo negative selection for autoreactive B cells. Since BAFFR-dependent signals prolong the survival of B cells, it was of interest to analyze if BAFF binding to BAFFR plays a role in the selection of B cells into the pool of mature B lymphocytes. Using a rearranged immunoglobulin V-gene knock-in mouse model (18), R. Pelanda and her group demonstrated that IgM+ IgD− autoreactive B cells with low IgM surface expression express low levels of BAFFR and do not respond to BAFF-induced survival signals. In contrast, non-autoreactive IgM+ IgD− B cells expressing more IgM on the cell surface also expressed, proportional to IgM, more BAFFR and developed into IgM+ IgD+ CD21+ CD23− transitional-1 B cells. These cells respond to BAFFR by developing into IgM+ IgD+ CD21+ CD23+ transitional-2 cells and later into mature B lymphocytes as it had been shown before in other mouse models (76, 81). A similar conclusion was reached by the group of Rolink (85) by carefully analyzing RAG-2 expression and B cell receptor editing by mouse and human IgM+ immature B cells from bone marrow. They found that BAFFR surface levels correlate directly with IgM but inversely with RAG-2 expression and receptor editing. Interestingly, anti-IgM treatment downregulates BAFFR on the surface of immature and transitional B cells while it enhances its expression on the surface of mature B cells (85). Since the inactivation of two Rho-GTPase encoding genes Rac1 and Rac2 does not only abolish BCR-induced intracellular calcium flux and the activation of the PI3K pathway but also BAFFR expression (86), BCR-dependent activation of Rac GTPases seems to induce the transcription of the Baffr gene in immature B cells.
B cells undergo a second phase of selection in germinal centers. Since excess of BAFF promotes the development of autoreactive B cells (75), BAFF-induces signals which interfere with mechanisms regulating the selection of B cells in the germinal center and with the equilibrium between BAFF-induced survival of dark zone B cells and affinity-based selection of centrocytes in the light zone. Genome-wide genetic association studies carried out with samples from multiple sclerosis (MS) and systemic lupus erythematosus (SLE) patients now provide evidence that genetically encoded changes of BAFF levels result in increased concentrations and correlate with the increased risk of developing autoimmunity (87).The genetic change results from a small deletion within the 3'UTR of BAFF mRNA. The deletion creates a new polyadenylation site allowing the premature termination of BAFF transcription. This shorter version of BAFF mRNA lacks an important regulatory sequence containing the binding site for miRNA-15a. This prevents micro-RNA directed control of excessive BAFF mRNA resulting in 1.5 to 2-fold increase in BAFF levels in a gene-dosage dependent manner. Like in the BAFF-transgenic mice, higher BAFF levels in humans increase the numbers of circulating B cells, promote the development of plasma cells, and result in higher serum IgG and IgM concentrations in homozygous carriers of this TNFSF13B variant (87).
Ablation of TACI expression or function not only cause immunodeficiency but also increases the risk of developing autoimmunity (88–90). The autoimmunity is now best explained by the decoy receptor function of TACI. In humans, the TACI variants C104R or C104Y, which reside in the second CRD abolish ligand-binding activity of TACI without preventing cell surface expression of the receptor. ADAM10-induced processing therefore sheds soluble forms of TACI, which cannot serve as decoy receptors to neutralize excessive BAFF levels. Therefore BAFF levels are increased in TACI-deficient patients (43) enhancing the risk of developing autoimmunity and lymphoproliferation, two characteristic features described in TACI deficiency in humans (89, 90) and mice (12, 88, 91).
However, point mutations or ablation of TACI expression also causes immunodeficiency. This can be best explained by the role of TACI in supporting T-independent immune responses (32, 92–95) and the survival of plasma cells (28, 30).
BAFFR Deficiency in Humans
In humans, only two cases of BAFFR-deficiency resulting from complete inactivation of the BAFFR encoding gene TNFRSF13C have been described so far. In both cases, the autosomal-recessive, homozygous 24bp in-frame deletion (80) removes the codons of highly conserved eight amino acids (LVLALVLV) from the transmembrane region of BAFFR, which extends from residues (76–98). The truncated BAFFR protein is highly unstable although in silico modeling predicts that the mutant BAFFR protein would be able to form a new transmembrane region between the resulting residues (70–92), which partially overlaps the TM region of the wild type protein. The lack of BAFFR expression causes an arrest of B cell differentiation at the transition from CD10+ immature/transitional 1 B cells to transitional 2 / naïve and marginal zone B cells. The homozygous mutation has full penetrance whereas the heterozygous deletion is phenotypically indistinguishable from healthy donors. Many of the immunological characteristics of human BAFFR deficiency have been described in Baffr-/- mice. The common features include very low numbers of circulating B cells, low IgM and IgG antibody titers but increased levels of serum IgA. Nevertheless, there are some clear differences between BAFFR-deficient humans and mice. First of all, inactivation of the BAFFR encoding Tnfsf13c gene in mice still allows normal development of B1 B cells, whereas in BAFFR-deficient humans have neither B1 B cells nor any other B cell subset, which would resemble B1 B cells. However, if B1 B cells would develop in humans as in mice from precursor cells during embryonic life and persist by homeostatic proliferation (78, 96, 97), they might have been disappeared in the BAFFR deficient patients like they also can disappear in old mice. On the other hand, the severe block in the development of follicular and marginal zone B cells might have created more space for the expansion of B1 B cells which then also would have had the chance to compensate the lack of these B2 B cell populations and to develop into IgA secreting plasma cells in the gut as it has been observed in mice (79). Interestingly, both BAFFR deficient patients have an effective output of immature B cells from bone marrow resulting in high numbers of transitional B cells, which are comparable to much younger adults. Different from Baffr-/- mice, both BAFFR-deficient humans suffered from severe lymphopenia. This difference might also be age-related or it might depend on differences regulating the size of the B cell pool, which are not well understood but known to vary between different individuals (98–100). Since IgM+ CD27+ marginal zone B cells control infections with encapsulated bacteria (101), the absence of marginal zone B cells resulted in defective T-independent vaccination responses against Pneumovax, which consists of a mixture of 23 different S.pneumoniae cell wall polysaccharides. Notably, the low but detectable IgG response against the T-dependent antigen tetanus toxoid, the high IgA serum concentrations and the presence of IgA+ plasma cells in the lamina propria of the small intestine showed that BAFFR-deficiency does not completely exclude the development of B cells into plasma cells. Similar results were observed in in Baffr−/− mice in which BAFFR-deficient B cells were found to complete the germinal center reaction (102) and to develop into switched memory B cells and plasma cells which survive without BAFFR (103). Upon depletion of BAFF by BAFF-neutralizing treatment with anti-BAFF monoclonal antibodies (Belimumab, Benlysta) or with soluble TACI-Ig (Atacicept), BAFFR-independent long-term survival of memory B cells has also been detected in SLE patients. These clinical studies show the strong (>75%) decline of naive B cell numbers that is followed by an increase in switched memory B cells (104, 105). Similar to the numbers of switched memory B cells, IgG antibody concentrations, which were build-up before starting the BAFF-neutralizing therapy, remained constant whereas the increase of antibody titers against neoantigens from influenza virus was significantly lower in belimumab-treated patients than in controls (106). In a similar study, the population of switched memory B cells did also not decrease within a half year treatment of rheumatoid arthritis patients with TACI-Ig fusion protein atacicept (107).
In addition to the BAFFR deletion, different missense mutations have been described for BAFFR. The mutants were found in patients suffering from common variable immunodeficiency (CVID), the most frequent form of primary immunodeficiency which is characterized by low or absent IgM, IgG, and IgA serum titers, low numbers or absent circulating switched memory B cells and the absence of circulating plasma cells (108, 109). The BAFFR missense mutations change amino acid residues in the extra- or intracellular part of BAFFR (110–112) but they do interfere with B cell development or survival in a way which would be comparable to the BAFFR deletion mutant. Therefore, their contribution to the development of CVID and antibody deficiency remains to be shown. In this context, the P21R BAFFR variant, which is encoded by a frequent single nucleotide polymorphism (rs77874543), represents one exception. The proline 21 is located in a small loop directly preceding the BAFF binding domain. Functional and biochemical studies showed that this small loop region is essential for ligand-independent association of BAFFR polypeptide chains into multimers. It therefore represents the pre-ligand assembly domain of BAFFR (7). Although the P21R-related defect in BAFFR clustering reduces the number of BAFF molecules able to bind BAFFR on the surface of B cells by at least 50%, it does not interfere with the development of transitional B cells to naive mature B cells. Since BAFFR multimerization strongly enhances BAFF binding, B cells carrying the P21R mutation develop less efficiently into IgM secreting plasmablasts. Moreover, the homozygous P21R variant is completely resistant against BAFF-induced processing of BAFFR by ADAM10 (70): The mutation seems to compensate its reduced ability to bind BAFF. This feature may mask in part the impaired differentiation of P21R+ B cells into IgM secreting plasmablasts and prevent the development of overt immunodeficiency. Table 1 summarizes the main differences in the studies of BAFF and BAFFR in humans and mouse models.
Perspectives and Conclusions
Studying the roles of BAFF and BAFF-receptors in experimental systems allowed the development of drugs which are now used to treat autoimmunity. So far, the BAFF-neutralizing monoclonal antibody belimumab is the only FDA- and EMA-approved biological drug for the treatment of patients with active refractory SLE. Application of the anti-BAFF antibody leads to a persistent, 50% improvement in about half of the SLE patients as reflected by lower anti-dsDNA titers, less corticosteroids, less cutaneous manifestations and less flares, while its adverse effects are similar as but less severe than the defects observed in BAFFR-deficiency and include respiratory, digestive and urinary tract infections [reviewed in (113)]. However, because of the lack of matched control groups, the efficacy of belimumab is still not completely clear. A second anti-BAFF antibody (tabalumab or LY2127399) has been tested in phase III studies and had similar effects like belimumab with an increase in complement activity and a decrease of anti-dsDNA IgG titers and B cell numbers leading to a mild clinical improvement in about 35% of patients treated in response to high dose treatment (114). The combination of tabalumab and bortezomib in a phase II study with multiple myeloma patients did not improve progression-free survival of the patients indicating that BAFF plays little or no role in disease progression (115). Similar to SLE, hyperactivated B cells are also discussed to play an important pathological role in the Sjögren's syndrome (116). Although in a trial study the treatment with the B cell-depleting anti-CD20 antibody did not lead to any improvement (117), new clinical trials with a novel BAFFR-specific monoclonal (ianalumab/VAY736/NOV-5) just have started. Since increased BAFF levels correlate with the risk of developing multiple sclerosis (87), new clinical trials with MS patients with tabalumab are now being performed (118). However, it should be kept in mind that treatment of MS patients with atacicept resulted in severe adverse effects, suggesting that in MS the blockade of BAFF and APRIL removes B cells with regulatory and immunosuppressive function and spares the pathological cells (119, 120).
In summary, switched memory B cells can perfectly survive without receiving BAFFR- or TACI-dependent pro-survival signals and develop into IgA secreting plasma cells. Different from B1 B cells and from switched memory B cells, the survival of transitional, follicular and marginal zone B cells as well as the differentiation of transitional B cells into follicular and marginal zone B cells depends essentially on BAFFR-induced survival signals, which increase the life span of these cells by stabilizing mitochondria and by enhancing protein synthesis. Since IgA-secreting plasma cells can develop even in the absence of BAFFR, BAFFR-deficiency does not become manifest as dramatically as NIK or NF-κB2 deficiency, which both strongly impair B and T cell responses. Since BAFFR, TACI and BCMA play different but critical roles in regulating B cell development and survival, analysis of coupled signaling pathways, of processing reactions affecting the half-life of surface BAFFR, TACI and BCMA and of their protein interaction partners will provide deep insights into the mechanisms regulating B cell selection, autoimmunity and aging.
Author Contributions
All authors listed have made a substantial, direct and intellectual contribution to the work, and approved it for publication.
Funding
This work was funded by the DFG through the TRR130 (P06) and through Ei235/9-1.
Conflict of Interest Statement
The authors declare that the research was conducted in the absence of any commercial or financial relationships that could be construed as a potential conflict of interest.
References
2. Siegel RM, Frederiksen JK, Zacharias DA, Chan FK, Johnson M, Lynch D, Tsien RY, et al. Fas preassociation required for apoptosis signaling and dominant inhibition by pathogenic mutations. Science (2000) 288:2354–7. doi: 10.1126/science.288.5475.2354
3. Chan FK, Chun HJ, Zheng L, Siegel RM, Bui KL, Lenardo MJ. A domain in TNF receptors that mediates ligand-independent receptor assembly and signaling. Science (2000) 288:2351–4. doi: 10.1126/science.288.5475.2351
4. Clancy L, Mruk K, Archer K, Woelfel M, Mongkolsapaya J, Screaton G, et al. Preligand assembly domain-mediated ligand-independent association between TRAIL receptor 4 (TR4) and TR2 regulates TRAIL-induced apoptosis. Proc Natl Acad Sci USA. (2005) 102:18099–104. doi: 10.1073/pnas.0507329102
5. Smulski CR, Beyrath J, Decossas M, Chekkat N, Wolff P, Estieu-Gionnet K, et al. Cysteine-rich domain 1 of CD40 mediates receptor self-assembly. J Biol Chem, (2013) 288:10914–22.
6. Smulski CR, Decossas M, Chekkat N, Beyrath J, Willen L, Guichard G, et al. Hetero-oligomerization between the TNF receptor superfamily members CD40, Fas and TRAILR2 modulate CD40 signalling. Cell Death Dis. (2017) 8:e2601. doi: 10.1038/cddis.2017.22
7. Pieper K, Rizzi M, Speletas M, Smulski CR, Sic H, Kraus H, et al. A common single nucleotide polymorphism impairs B-cell activating factor receptor's multimerization, contributing to common variable immunodeficiency. J Allergy Clin Immunol. (2014) 133:1222–5. doi: 10.1016/j.jaci.2013.11.021
8. Pieper K, Grimbacher B, Eibel H. B-cell biology and development. J Allergy Clin Immunol, (2013) 131:959–71. doi: 10.1016/j.jaci.2013.01.046
9. Thompson JS, Bixler SA, Qian F, Vora K, Scott ML, Cachero TG, et al. BAFF-R, a newly identified TNF receptor that specifically interacts with BAF. Science (2001) 293:2108–11. doi: 10.1126/science.1061965
10. Lentz VM, Cancro MP, Nashold FE, Hayes CE. Bcmd governs recruitment of new B cells into the stable peripheral B cell pool in the A/WySnJ mouse. J Immunol. (1996) 157:598–606.
11. Lentz VM, Hayes CE, Cancro MP. Bcmd decreases the life span of B-2 but not B-1 cells in A/WySnJ mice. J Immunol. (1998) 160:3743–7.
12. Yan M, Brady JR, Chan B, Lee WP, Hsu B, Harless S, et al. Identification of a novel receptor for B lymphocyte stimulator that is mutated in a mouse strain with severe B cell deficiency. Curr Biol. (2001) 11:1547–52. doi: 10.1016/S0960-9822(01)00481-X
13. Hsu BL, Harless SM, Lindsley RC, Hilbert DM, Cancro MP. Cutting edge: BLyS enables survival of transitional and mature B cells through distinct mediators. J Immunol. (2002) 168:5993–6. doi: 10.4049/jimmunol.168.12.5993
14. Mihalcik SA, Huddleston PM, III, Wu X, Jelinek DF. The structure of the TNFRSF13C promoter enables differential expression of BAFF-R during B cell ontogeny and terminal differentiation. J Immunol. (2010) 185:1045–54. doi: 10.4049/jimmunol.1001120
15. Smith SH, Cancro MP. Cutting edge: B cell receptor signals regulate BLyS receptor levels in mature B cells and their immediate progenitors. J Immunol. (2003) 170:5820–3. doi: 10.4049/jimmunol.170.12.5820
16. Ng LG, Sutherland AP, Newton R, Qian F, Cachero TG, Scott ML, et al. B cell-activating factor belonging to the TNF family (BAFF)-R is the principal BAFF receptor facilitating BAFF costimulation of circulating T and B cells. J Immunol. (2004) 173:807–17. doi: 10.4049/jimmunol.173.2.807
17. Rodig SJ, Shahsafaei A, Li B, Mackay CR, Dorfman DM. BAFF-R, the major B cell-activating factor receptor, is expressed on most mature B cells and B-cell lymphoproliferative disorders. Hum Pathol, (2005) 36:1113–9. doi: 10.1016/j.humpath.2005.08.005
18. Rowland SL, Leahy KF, Halverson R, Torres RM, Pelanda R. BAFF receptor signaling aids the differentiation of immature B cells into transitional B cells following tonic BCR signaling. J Immunol. (2010) 185:4570–81. doi: 10.4049/jimmunol.1001708
19. Hobeika E, Levit-Zerdoun E, Anastasopoulou V, Pohlmeyer R, Altmeier S, Alsadeq A, et al. CD19 and BAFF-R can signal to promote B-cell survival in the absence of Syk. EMBO J, (2015) 34:925–39. doi: 10.15252/embj.201489732
20. Levit-Zerdoun E, Becker M, Pohlmeyer R, Wilhelm I, Maity PC, Rajewsky K, et al. Survival of igalpha-deficient mature B cells requires BAFF-R function. J Immunol. (2016) 196:2348–60. doi: 10.4049/jimmunol.1501707
21. Schneider P, Mackay CR, Steiner V, Hofmann K, Bodmer JL, Holler N, et al. BAFF, a Novel Ligand of the Tumor Necrosis Factor Family, Stimulates B Cell Growth. J. Exp. Med. (1999) 189:1747–56.
22. Moore PA, Belvedere O, Orr A, Pieri K, LaFleur DW, Feng P, et al. BLyS: Member of the Tumor Necrosis Factor Family and B Lymphocyte Stimulator. Science (1999) 285:260–3.
23. Shu HB, Hu WH, Johnson H. TALL-1 is a novel member of the TNF family that is down-regulated by mitogens. J Leukocyte Biol. (1999) 65:680–3.
24. Gross JA, Johnston J, Mudri S, Enselman R, Dillon SR, Madden K, et al. TACI and BCMA are receptors for a TNF homologue implicated in B-cell autoimmune disease. Nature, (2000) 404:995–9. doi: 10.1038/35010115
25. Schiemann B, Gommerman JL, Vora K, Cachero TG, Shulga-Morskaya S, Dobles M, et al. An Essential Role for BAFF in the Normal Development of B Cells Trough a BCMA-Independent Pathway. Science (2001) 293:2111–4. doi: 10.1126/science.1061964
26. Hahne M, Kataoka T, Schröter M, Hofmann K, Irmler M, Bodmer JL, et al. APRIL, a New Ligand of the Tumor Necrosis Factor Family Stimulates Tumor Cell Growth. J Exp Med. (1998) 188:1185–90.
27. Belnoue E, Pihlgren M, McGaha TL, Tougne C, Rochat AF, Bossen C, et al. APRIL is critical for plasmablast survival in the bone marrow and poorly expressed by early-life bone marrow stromal cells. Blood (2008) 111:2755–64. doi: 10.1182/blood-2007-09-110858
28. Mantchev GT, Cortesao CS, Rebrovich M, Cascalho M, Bram RJ. TACI is required for efficient plasma cell differentiation in response to T-independent type 2 Antigens. J Immunol. (2007) 179:2282–8. doi: 10.4049/jimmunol.179.4.2282
29. Ozcan E, Garibyan L, Lee JJ, Bram RJ, Lam KP, Geha RS. Transmembrane activator, calcium modulator, and cyclophilin ligand interactor drives plasma cell differentiation in LPS–activated B cells. J Allergy Clin Immunol. (2009) 123:1277–86 e5. doi: 10.1016/j.jaci.2009.03.019
30. Tsuji S, Cortesao C, Bram RJ, Platt JL, Cascalho M. TACI deficiency impairs sustained Blimp-1 expression in B cells decreasing long-lived plasma cells in the bone marrow. Blood (2011) 118:5832–9. doi: 10.1182/blood-2011-05-353961
31. Hoffmann FS, Kuhn PH, Laurent SA, Hauck SM, Berer K, Wendlinger SA, et al. The immunoregulator soluble TACI is released by ADAM10 and reflects B cell activation in autoimmunity. J Immunol. (2015) 194:542–52. doi: 10.4049/jimmunol.1402070
32. He B, Santamaria R, Xu W, Cols M, Chen K, Puga I, et al. The transmembrane activator TACI triggers immunoglobulin class switching by activating B cells through the adaptor MyD88. Nat Immunol. (2010) 11:836–45. doi: 10.1038/ni.1914
33. O'Connor BP, Raman VS, Erickson LD, Cook WJ, Weaver LK, Ahonen C, et al. BCMA is essential for the survival of long-lived bone marrow plasma cells. J Exp Med. (2004) 199:91–8. doi: 10.1084/jem.20031330
34. Karpusas M, Cachero TG, Qian F, Sjodin AB, Mullen C, Strauch K, et al. Crystal structure of extracellular human BAFF, a TNF family member that stimulates B lymphocytes. J. Mol. Biol. (2002) 315:1145–54. doi: 10.1006/jmbi.2001.5296
35. Liu Y, Xu L, Opalka N, Kappler J, Shu HB, Zhang B. Crystal structure of sTALL-1 reveals a virus-like assembly of TNF family ligands. Cell Press (2002) 108:383–394. doi: 10.1016/S0092-8674(02)00631-1
36. Schuepbach-Mallepell S, Das D, Willen L, Vigolo M, Tardivel A, Lebon L, et al. Stoichiometry of heteromeric BAFF and APRIL cytokines dictates their receptor binding and signaling properties. J Biol Chem. (2015) 290:16330–42. doi: 10.1074/jbc.M115.661405
37. Liu Y, Hong X, Kappler J, Jiang L, Zhang R, Xu L, et al. Ligand–receptor binding revealed by the TNF family member TALL-1. Nature (2003) 423:49–56. doi: 10.1038/nature01543
38. Cachero TG, Schwartz IM, Qian F, Day ES, Bossen C, Ingold K, et al. Formation of virus-like clusters is an intrinsic property of the tumor necrosis factor family member BAFF (B Cell Activating Factor). Biochemistry (2005) 45:2006–2013. doi: 10.1021/bi051685o
39. Vigolo M, Chambers MG, Willen L, Chevalley D, Maskos K, Lammens A, et al. A loop region of BAFF controls B cell survival and regulates recognition by different inhibitors. Nat Commun. (2018) 9: 1199. doi: 10.1038/s41467-018-03323-8
40. Nardelli B, Belvedere O, Roschke V, Moore PA, Olsen HS, Migone TS, et al. Synthesis and release of B-lymphocyte stimulator from myeloid cells. Blood J. (2001) 97:198–204. doi: 10.1182/blood.V97.1.198
41. Thangarajh M, Masterman T, Helgeland L, Rot U, Jonsson MV, Eide GE, et al. The thymus is a source of B-cell-survival factors-APRIL and BAFF-in myasthenia gravis. J Neuroimmunol. (2006) 178:161–6. doi: 10.1016/j.jneuroim.2006.05.023
42. Litinskiy MB, Nardelli B, Hilbert DM, He B, Schaffer A, Casali P, Cerutti A. DCs induce CD40-independent immunoglobulin class switching through BLyS and APRIL. Nat Immunol. (2002) 3:822–9. doi: 10.1038/ni829
43. Kreuzaler M, Rauch M, Salzer U, Birmelin J, Rizzi M, Grimbacher B, et al. Soluble BAFF levels inversely correlate with peripheral B cell numbers and the expression of BAFF receptors. J Immunol, (2012) 188:497–503. doi: 10.4049/jimmunol.1102321
44. Claudio E, Brown K, Park S, Wang H, Siebenlist U. BAFF-induced NEMO-independent processing of NF-kappa B2 in maturing B cells. Nat Immunol. (2002) 3:958–65. doi: 10.1038/ni842
45. Kayagaki N, Yan M, Seshasayee D, Wang H, Lee W, French DM, et al. BAFF/BLyS receptor 3 binds the B cell survival factor BAFF ligand through a discrete surface loop and promotes processing of NF-kappaB2. Immunity (2002) 17:515–24. doi: 10.1016/S1074-7613(02)00425-9
46. Zhang Q, Lenardo MJ, Baltimore D. 30 Years of NF-kappaB: a blossoming of relevance to human pathobiology. Cell (2017) 168:37–57. doi: 10.1016/j.cell.2016.12.012
47. Vallabhapurapu S, Karin M. Regulation and function of NF-kappaB transcription factors in the immune system. Annu Rev Immunol. (2009) 27:693–733. doi: 10.1146/annurev.immunol.021908
48. Gilmore TD. Introduction to NF-kappaB: players, pathways, perspectives. Oncogene (2006) 25:6680–4. doi: 10.1038/sj.onc.1209954
49. Heusch M, Lin L, Gelezinunas R, Greene WC. The generation of nfkb2 p52: mechanism and efficiency. Oncogene (1999) 18:6201–8.
50. Xiao G, Harhaj EW, Sun SC. NF-kappaB-inducing kinase regulates the processing of NF-kappaB2 p100. Mol Cell. (2001) 7:401–9. doi: 10.1016/S1097-2765(01)00187-3
51. Liao G, Zhang M, Harhaj EW, Sun SC. Regulation of the NF-kappaB-inducing kinase by tumor necrosis factor receptor-associated factor 3-induced degradation. J Biol Chem, (2004) 279:26243–50. doi: 10.1074/jbc.M403286200
52. Ling L, Cao Z, Goeddel DV. NF-kappaB-inducing kinase activates IKK-alpha by phosphorylation of Ser-176. Proc Natl Acad Sci USA. (1998) 95:3792–7.
53. Gardam S, Sierro F, Basten A, Mackay F, Brink R. TRAF2 and TRAF3 signal adapters act cooperatively to control the maturation and survival signals delivered to B cells by the BAFF receptor. Immunity (2008) 28:391–401. doi: 10.1016/j.immuni.2008.01.009
54. Ni CZ, Oganesyan G, Welsh K, Zhu X, Reed JC, Satterthwait AC, et al. Key molecular contacts promote recognition of the BAFF receptor by TNF receptor-associated factor 3: implications for intracellular signaling regulation. J Immunol. (2004) 173:7394–400. doi: 10.4049/jimmunol.173.12.7394
55. Xu LG, Shu HB. TNFR-associated factor-3 is associated with BAFF-R and negatively regulates BAFF-R-mediated NF-kappa B activation and IL-10 production. J Immunol. (2002) 169:6883–9. doi: 10.4049/jimmunol.169.12.6883
56. Amir RE, Haecker H, Karin M, Ciechanover A. Mechanism of processing of the NF-kappa B2 p100 precursor: identification of the specific polyubiquitin chain-anchoring lysine residue and analysis of the role of NEDD8-modification on the SCF(beta-TrCP) ubiquitin ligase. Oncogene, (2004) 23:2540–7. doi: 10.1038/sj.onc.1207366
57. Lin WW, Hildebrand JM, Bishop GA. A complex relationship between TRAF3 and non-canonical NF-kappaB2 Activation in B Lymphocytes. Front Immunol. (2013) 4:477. doi: 10.3389/fimmu.2013.00477
58. Hu H, Wu X, Jin W, Chang M, Cheng X, Sun SC. Noncanonical NF-kappaB regulates inducible costimulator (ICOS) ligand expression and T follicular helper cell development. Proc Natl Acad Sci USA. (2011) 108:12827–32. doi: 10.1073/pnas.1105774108
59. Willmann KL, Klaver S, Dogu F, Santos-Valente E, Garncarz W, Bilic I, et al. Biallelic loss-of-function mutation in NIK causes a primary immunodeficiency with multifaceted aberrant lymphoid immunity. Nat Commun. (2014) 5:5360. doi: 10.1038/ncomms6360
60. Mambetsariev N, Lin WW, Stunz LL, Hanson BM, Hildebrand JM, Bishop GA. Nuclear TRAF3 is a negative regulator of CREB in B cells. Proc Natl Acad Sci USA. (2016) 113:1032–7. doi: 10.1073/pnas.1514586113
61. Maurer U, Charvet C, Wagman AS, Dejardin E, Green DR. Glycogen synthase kinase-3 regulates mitochondrial outer membrane permeabilization and apoptosis by destabilization of MCL-1. Mol Cell. (2006) 21:749–60. doi: 10.1016/j.molcel.2006.02.009
62. Hu H, Brittain GC, Chang JH, Puebla-Osorio N, Jin J, Zal A, et al. OTUD7B controls non-canonical NF-kappaB activation through deubiquitination of TRAF3. Nature (2013) 494:371–4. doi: 10.1038/nature11831
63. Schweighoffer E, Vanes L, Nys J, Cantrell D, McCleary S, Smithers N, et al. The BAFF receptor transduces survival signals by co-opting the B cell receptor signaling pathway. Immunity (2013) 38:475–88. doi: 10.1016/j.immuni.2012.11.015
64. Jellusova J, Miletic AV, Cato MH, Lin WW, Hu Y, Bishop GA, et al. Context-specific BAFF-R signaling by the NF-kappaB and PI3K pathways. Cell Rep (2013) 5:1022–35. doi: 10.1016/j.celrep.2013.10.022
65. Mattila PK, Feest C, Depoil D, Treanor B, Montaner B, Otipoby KL, et al. The actin and tetraspanin networks organize receptor nanoclusters to regulate B cell receptor-mediated signaling. Immunity, (2013) 38:461–74. doi: 10.1016/j.immuni.2012.11.019
66. Keppler SJ, Gasparrini F, Burbage M, Aggarwal S, Frederico B, Geha RS, et al. Wiskott-aldrich syndrome interacting protein deficiency uncovers the role of the co-receptor CD19 as a generic hub for PI3 kinase signaling in B cells. Immunity (2015) 43:660–73. doi: 10.1016/j.immuni.2015.09.004
67. Jellusova J, Rickert RC. The PI3K pathway in B cell metabolism. Crit Rev Biochem Mol Biol. (2016) 51:359–78. doi: 10.1080/10409238.2016.1215288
68. Patke A, Mecklenbrauker I, Erdjument-Bromage H, Tempst P, Tarakhovsky A. BAFF controls B cell metabolic fitness through a PKC beta- and Akt-dependent mechanism. J Exp Med. (2006) 203:2551–62. doi: 10.1084/jem.20060990
69. Woodland RT, Fox CJ, Schmidt MR, Hammerman PS, Opferman JT, Korsmeyer SJ, et al. Multiple signaling pathways promote B lymphocyte stimulator dependent B-cell growth and survival. Blood (2008) 111:750–60. doi: 10.1182/blood-2007-03-077222
70. Smulski CR, Kury P, Seidel LM, Staiger HS, Edinger AK, Willen L, et al. BAFF- and TACI-dependent processing of BAFFR by adam proteases regulates the survival of B cells. Cell Rep, (2017) 18:2189–202. doi: 10.1016/j.celrep.2017.02.005
71. Laurent SA, Hoffmann FS, Kuhn PH, Cheng Q, Chu Y, Schmidt-Supprian M, et al. Gamma-Secretase directly sheds the survival receptor BCMA from plasma cells. Nat Commun. (2015) 6:7333. doi: 10.1038/ncomms8333
72. Kopan R, Ilagan MX. The canonical Notch signaling pathway: unfolding the activation mechanism. Cell (2009) 137:216–33. doi: 10.1016/j.cell.2009.03.045
73. Mesin L, Ersching J, Victora GD. Germinal center B cell dynamics. Immunity (2016) 45:471–82. doi: 10.1016/j.immuni.2016.09.001
74. Kräutler NJ, Suan D, Butt D, Bourne K, Hermes JR, Chan TD, et al. Differentiation of germinal center B cells into plasma cells is initiated by high-affinity antigen and completed by Tfh cells. J Exp Med. (2017) 214:1259–67. doi: 10.1084/jem.20161533
75. Mackay F, Woodcock SA, Lawton P, Ambrose C, Baetscher M, Schneider P, et al. Mice transgenic for BAFF develop lymphocytic disorders along with autoimmune manifestations. J Exp Med. (1999) 190:1697–710.
76. Sasaki Y, Casola S, Kutok JL, Rajewsky K, Schmidt-Supprian M. TNF family member B cell-activating factor (BAFF) receptor-dependent and -independent roles for BAFF in B cell physiology. J Immunol. (2004) 173:2245–52.doi: 10.4049/jimmunol.173.4.2245
77. Baumgarth N. The double life of a B-1 cell: self-reactivity selects for protective effector functions. Nat Rev Immunol. (2010) 11:34. doi: 10.1038/nri2901
78. Hardy RR, Hayakawa K. Perspectives on fetal derived CD5+ B1 B cells. Eur J Immunol. (2015) 45:2978–84. doi: 10.1002/eji.201445146
79. Wang H, Shin DM, Abbasi S, Jain S, Kovalchuk AL, Beaty N, et al. Expression of plasma cell alloantigen 1 defines layered development of B-1a B-cell subsets with distinct innate-like functions. Proc Natl Acad Sci USA. (2012) 109:20077–82. doi: 10.1073/pnas.1212428109
80. Warnatz K, Salzer U, Rizzi M, Fischer B, Gutenberger S, Bohm J, et al. B-cell activating factor receptor deficiency is associated with an adult-onset antibody deficiency syndrome in humans. Proc Natl Acad Sci USA. (2009) 106:13945–50. doi: 10.1073/pnas.0903543106
81. Scholz JL, Crowley JE, Tomayko MM, Steinel N, O'Neill PJ, Quinn WJ, 3rd, et al. BLyS inhibition eliminates primary B cells but leaves natural and acquired humoral immunity intact. Proc Natl Acad Sci USA. (2008) 105:15517–22. doi: 10.1073/pnas.0807841105
82. Rauch M, Tussiwand R, Bosco N, Rolink AG. Crucial role for BAFF-BAFF-R signaling in the survival and maintenance of mature B cells. PLoS ONE (2009) 4:e5456. doi: 10.1371/journal.pone.0005456
83. Schneider P, Takatsuka H, Wilson A, Mackay F, Tardivel A, Lens S, et al. Maturation of marginal zone and follicular B cells requires B cell activating factor of the tumor necrosis factor family and is independent of B cell maturation antigen. J Exp Med. (2001) 194:1691–7. doi: 10.1084/jem.194.11.1691
84. Gross JA, Dillon SR, Mudri S, Johnston J, Littau A, Roque R, et al. TACI-Ig neutralizes molecules critical for B cell development and autoimmune disease: impaired B cell maturation in mice lacking BLyS. Immunity (2001) 15:289–302. doi: 10.1016/S1074-7613(01)00183-2
85. Tussiwand R, Rauch M, Fluck LA, Rolink AG. BAFF-R expression correlates with positive selection of immature B cells. Eur J Immunol. (2012) 42:206–16. doi: 10.1002/eji.201141957
86. Walmsley MJ, Ooi SK, Reynolds LF, Smith SH, Ruf S, Mathiot A, et al. Critical roles for Rac1 and Rac2 GTPases in B cell development and signaling. Science (2003) 302:459–62.doi: 10.1126/science.1089709
87. Steri M, Orru V, Idda ML, Pitzalis M, Pala M, Zara I, et al. Overexpression of the cytokine BAFF and autoimmunity risk. N Engl J Med. (2017) 376:1615–26. doi: 10.1056/NEJMoa1610528
88. Yan M, Wang H, Chan B, Roose-Girma M, Erickson S, Baker T, et al. Activation and accumulation of B cells in TACI-deficient mice. Nat Immunol. (2001) 2:638–43. doi: 10.1038/89790
89. Salzer U, Chapel HM, Webster AD, Pan-Hammarstrom Q, Schmitt-Graeff A, Schlesier M, et al. Mutations in TNFRSF13B encoding TACI are associated with common variable immunodeficiency in humans. Nat Genet. (2005) 37:820–8. doi: 10.1038/ng1600
90. Castigli E, Wilson SA, Garibyan L, Rachid R, Bonilla F, Schneider L, et al. TACI is mutant in common variable immunodeficiency and IgA deficiency. Nat Genet. (2005) 37:829–34. doi: 10.1038/ng1601
91. Seshasayee D, Valdez P, Yan M, Dixit VM, Tumas D, Grewal IS. Loss of TACI causes fatal lymphoproliferation and autoimmunity, establishing TACI as an inhibitory BLyS receptor. Immunity (2003) 18:279–88. doi: 10.1016/S1074-7613(03)00025-6
92. von Bülow G, van Deursen JM, Bram RJ. Regulation of the T-independent humoral response by TACI. Immunity (2001) 14:573–82. doi: 10.1016/S1074-7613(01)00130-3
93. Puga I, Cols M, Barra CM, He B, Cassis L, Gentile M, et al. B cell-helper neutrophils stimulate the diversification and production of immunoglobulin in the marginal zone of the spleen. Nat Immunol. (2011) 13:170–80. doi: 10.1038/ni.2194.
94. Magri G, Miyajima M, Bascones S, Mortha A, Puga I, Cassis L, et al. Innate lymphoid cells integrate stromal and immunological signals to enhance antibody production by splenic marginal zone B cells. Nat Immunol. (2014) 15:354–64. doi: 10.1038/ni.2830
95. Sintes J, Gentile M, Zhang S, Garcia-Carmona Y, Magri G, Cassis L, et al. mTOR intersects antibody-inducing signals from TACI in marginal zone B cells. Nat Commun. (2017) 8:1462. doi: 10.1038/s41467-017-01602-4
96. Martin F, Kearney JF, B1 cells: similarities and differences with other B cell subsets. Curr Opin Immunol. (2001) 13:195–201. doi: 10.1016/S0952-7915(00)00204-1
97. Pillai S, Cariappa A, Moran ST. Positive selection and lineage commitment during peripheral B-lymphocyte development. Immunol Rev. (2004) 197:206–18. doi: 10.1111/j.0105-2896.2003.097.x
98. Morbach H, Eichhorn EM, Liese JG, Girschick HJ. Reference values for B cell subpopulations from infancy to adulthood. Clin Exp Immunol. (2010) 162:271–9. doi: 10.1111/j.1365-2249.2010.04206.x
99. Perez-Andres M, Paiva B, Nieto WG, Caraux A, Schmitz A, Almeida J, et al. Human peripheral blood B-cell compartments: a crossroad in B-cell traffic. Cytometry B Clin Cytom. (2010) 78(Suppl. 1):S47–60. doi: 10.1002/cyto.b.20547
100. Aranburu A, Piano Mortari E, Baban A, Giorda E, Cascioli S, Marcellini V, et al. Human B-cell memory is shaped by age- and tissue-specific T-independent and GC-dependent events. Eur J Immunol. (2016) 47:327–344. doi: 10.1002/eji.201646642
101. Kruetzmann S, Rosado MM, Weber H, Germing U, Tournilhac O, Peter HH, et al. Human immunoglobulin M memory B cells controlling Streptococcus pneumoniae infections are generated in the spleen. J Exp Med. (2003) 197:939–45. doi: 10.1084/jem.20022020
102. Rahman ZS, Rao SP, Kalled SL, Manser T. Normal induction but attenuated progression of germinal center responses in BAFF and BAFF-R signaling-deficient mice. J Exp Med. (2003) 198:1157–69. doi: 10.1084/jem.20030495
103. Benson MJ, Dillon SR, Castigli E, Geha RS, Xu S, Lam KP, et al. Cutting edge: the dependence of plasma cells and independence of memory B cells on BAFF and APRI L J Immunol. (2008) 180:3655–9. doi: 10.4049/jimmunol.180.6.3655
104. Furie R, Petri M, Zamani O, Cervera R, Wallace DJ, Tegzova D, et al. A phase III, randomized, placebo-controlled study of belimumab, a monoclonal antibody that inhibits B lymphocyte stimulator, in patients with systemic lupus erythematosus. Arthritis Rheum. (2011) 63:3918–30. doi: 10.1002/art.30613
105. Chatham WW, Wallace DJ, Stohl W, Latinis KM, Manzi S, McCune WJ, et al. Effect of belimumab on vaccine antigen antibodies to influenza, pneumococcal, and tetanus vaccines in patients with systemic lupus erythematosus in the BLISS-76 trial. J Rheumatol. (2012) 39:1632–40. doi: 10.3899/jrheum.111587
106. Stohl W, Hiepe F, Latinis KM, Thomas M, Scheinberg MA, Clarke A, et al. Belimumab reduces autoantibodies, normalizes low complement levels, and reduces select B cell populations in patients with systemic lupus erythematosus. Arthritis Rheum. (2012) 64:2328–37. doi: 10.1002/art.34400
107. van Vollenhoven RF, Kinnman N, Vincent E, Wax S, Bathon J. Atacicept in patients with rheumatoid arthritis and an inadequate response to methotrexate: results of a phase II, randomized, placebo-controlled trial. Arthritis Rheum, (2011) 63:1782–92. doi: 10.1002/art.30372
108. Abbott JK, Gelfand EW. Common variable immunodeficiency: diagnosis, management, and treatment. Immunol Allergy Clin North Am. (2015) 35:637–58. doi: 10.1016/j.iac.2015.07.009
109. Ameratunga R, Lehnert K, Woon ST, Gillis D, Bryant VL, Slade CA, et al. Review: diagnosing common variable immunodeficiency disorder in the era of genome sequencing. Clin Rev Allergy Immunol. (2018) 54:261–8. doi: 10.1007/s12016-017-8645-0
110. Losi CG, Silini A, Fiorini C, Soresina A, Meini A, Ferrari S, et al. Mutational analysis of human BAFF receptor TNFRSF13C (BAFF-R) in patients with common variable immunodeficiency. J Clin Immunol. (2005) 25:496–502. doi: 10.1007/s10875-005-5637-2
111. Hildebrand JM, Luo Z, Manske MK, Price-Troska T, Ziesmer SC, Lin W, et al. A BAFF-R mutation associated with non-Hodgkin lymphoma alters TRAF recruitment and reveals new insights into BAFF-R signaling. J Exp Med. (2010) 207:2569–79. doi: 10.1084/jem.20100857
112. Kutukculer N, Gulez N, Karaca NE, Aksu G, Berdeli A. Three different classifications, B lymphocyte subpopulations, TNFRSF13B (TACI), TNFRSF13C (BAFF-R), TNFSF13 (APRIL) gene mutations, CTLA-4 and ICOS gene polymorphisms in Turkish patients with common variable immunodeficiency. J Clin Immunol. (2012) 32:1165–79. doi: 10.1007/s10875-012-9717-9
113. Trentin F, Gatto M, Zen M, Maddalena L, Nalotto L, Saccon F, et al. Effectiveness, tolerability, and safety of belimumab in patients with refractory SLE: a review of observational clinical-practice-based studies. Clin Rev Allergy Immunol. (2018) 54:331–43. doi: 10.1007/s12016-018-8675-2
114. Isenberg DA, Petri M, Kalunian K, Tanaka Y, Urowitz MB, Hoffman RW, et al. Efficacy and safety of subcutaneous tabalumab in patients with systemic lupus erythematosus: results from ILLUMINATE-1, a 52-week, phase III, multicentre, randomised, double-blind, placebo-controlled study. Ann Rheum Dis. (2016) 75:323–31. doi: 10.1136/annrheumdis-2015-20765
115. Raje NS, Moreau P, Terpos E, Benboubker L, Grzasko N, Holstein SA, et al. Phase 2 study of tabalumab, a human anti-B-cell activating factor antibody, with bortezomib and dexamethasone in patients with previously treated multiple myeloma. Br J Haematol, (2017) 176:783–795. doi: 10.1111/bjh.14483
116. Nocturne G, Mariette X. B cells in the pathogenesis of primary Sjogren syndrome. Nat Rev Rheumatol, (2018) 14:133–145. doi: 10.1038/nrrheum.2018.1
117. Devauchelle-Pensec V, Mariette X, Jousse-Joulin S, Berthelot JM, Perdriger A, Puechal X, et al. Treatment of primary Sjogren syndrome with rituximab: a randomized trial. Ann Intern Med, (2014) 160:233–42. doi: 10.7326/M13-1085
118. Rahmanzadeh R, Weber MS, Bruck W, Navardi S, Sahraian MA. B cells in multiple sclerosis therapy-A comprehensive review. Acta Neurol Scand, (2018) 137:544–556. doi: 10.1111/ane.12915
119. Kappos L, Hartung HP, Freedman MS, Boyko A, Radü EW, Mikol DD, et al. Atacicept in multiple sclerosis (ATAMS): a randomised, placebo-controlled, double-blind, phase 2 trial. Lancet Neurol. (2014) 13:353–63. doi: 10.1016/S1474-4422(14)70028-6
Keywords: BAFF - B-cell activating factor, TNFRSF13C, BAFF-R, BAFF-R deficiency, B cell, NF-k B, primary immumunodeficiencies
Citation: Smulski CR and Eibel H (2018) BAFF and BAFF-Receptor in B Cell Selection and Survival. Front. Immunol. 9:2285. doi: 10.3389/fimmu.2018.02285
Received: 12 June 2018; Accepted: 14 September 2018;
Published: 08 October 2018.
Edited by:
Harry W. Schroeder, University of Alabama at Birmingham, United StatesReviewed by:
Yi Hao, Tongji Medical College, Huazhong University of Science and Technology, ChinaMike Cancro, University of Pennsylvania, United States
Copyright © 2018 Smulski and Eibel. This is an open-access article distributed under the terms of the Creative Commons Attribution License (CC BY). The use, distribution or reproduction in other forums is permitted, provided the original author(s) and the copyright owner(s) are credited and that the original publication in this journal is cited, in accordance with accepted academic practice. No use, distribution or reproduction is permitted which does not comply with these terms.
*Correspondence: Hermann Eibel, aGVybWFubi5laWJlbEB1bmlrbGluaWstZnJlaWJ1cmcuZGU=