- 1Department of Marine Biosciences, Tokyo University of Marine Science and Technology, Tokyo, Japan
- 2Institute of Infectology, Friedrich-Loeffler-Institute, Federal Research Institute for Animal Health, Greifswald-Insel Riems, Germany
In mammals, M cells can take up antigens through mucosal surfaces of the gut and the respiratory tract. Since M cells are deficient of lysosomes and phagosomes, the antigens are directly delivered to the mucosa-associated lymphoid tissue (MALT) without degradation. In teleost fish, the entire body surface (gills, skin, and intestinal system) is covered by mucus; however, specific antigen-sampling cells have not yet been identified in their mucosal tissues. Here, we show that two phenotypes of antigen-sampling cells take up antigens through epithelial surfaces of the rainbow trout gill. One phenotype of antigen-sampling cells has features of monocyte/macrophage/dendritic cell-type cells; they have large vacuoles in the cytoplasm and express PTPRC (CD45), CD83, IL-1β, and IL-12p40b. The second phenotype exhibits similar characteristics to mammalian M cells; the corresponding cells bind the lectin UEA-1 but not WGA and show expression of M cell marker gene Anxa5. In contrast to mammalian M cells, teleost M-type cells were found to exhibit small vacuoles in their cytoplasm and to express almost all genes related to the “phagosome”, “lysosome,” and “antigen processing and presentation” pathways. Furthermore, MHC class II was constitutively expressed on a fraction of M-type cells, and this expression was significantly increased after antigen uptake, suggesting that the MHC class II is inducible by antigen stimulation. Here, we suggest that teleost M-type cells play a role in the phylogenetically primitive teleost immune system, similar to bona-fide M cells. In addition, the presence of MHC class II expression suggests an additional role in antigen presentation in the gills, which are an organ with high T cell abundance, especially in interbranchial lymphoid tissue. The present results suggest an unconventional antigen presentation mechanism in the primitive mucosal immune system of teleosts, which generally lack highly organized lymphoid tissues. Moreover, the results of this work may be valuable for the development of mucosal vaccines that specifically target M-type cells; mucosal vaccines significantly reduce working costs and the stress that is usually induced by vaccination via injection of individual fish.
Introduction
The mucosal surfaces of the digestive and respiratory systems are continuously exposed to the external environment and therefore represent potential ports of pathogen entry. The immune system has evolved to prevent pathogen entry in mucosal tissues, and if an entry is unavoidable, to mount a local immune response. In mammals, recognition of pathogens, and initialization of the immune response occurs in mucosa-associated lymphoid tissue (MALT). MALT consists of germinal centers, lymphoid follicles, and T cell regions and harbors professional antigen-presenting cells (APCs), such as macrophages and dendritic cells (1). M (membranous epithelial, microfold, or microvillous) cells (2) are atypical epithelial cells that phagocytize antigens and macromolecules in the follicle-associated (dome) epithelium (FAE) of gut-associated lymphoid tissue (GALT) and nasopharynx-associated lymphoid tissue (NALT) in mammals. In mice, the lectin Ulex europaeus agglutinin-1 (UEA-1), which specifically binds to α (1, 2) fucose and it has been established as an excellent marker for human endothelial cells, is routinely used to identify M cells. In contrast, M cells do not test positive for the lectin wheat germ agglutinin (WGA), which binds to UEA-1+ goblet cells in FAE (3). Molecules on the surface of M cells such as glycoprotein 2 (4), integrin β1 (5), and α2-3-linked sialic acid (6) have been identified as receptors involved in the uptake of FimH+ bacteria, Yersinia enterocolitica and type 1 reovirus, respectively. Following their capture by the corresponding receptors, M cells mainly transcytose the respective antigens and deliver them to subjacent APCs (7), and the APCs then present antigens to T lymphocytes in MALT. Finally, antigen-specific immune responses, such as production of IgA by B cells, are induced in mucosal tissues.
Fish inhabit aquatic environments, in which microorganisms are more abundant than in terrestrial environments. The entire body surface of fish (gills, intestine, and skin) is covered by mucus, which is one of the initial immune barriers preventing the invasion of pathogens. Unlike mammals, teleost fish lack lymphoid structures such as germinal centers, B-cell follicles, lymph nodes, and structured MALT. Zapata and Amemiya (8) described the teleost GALT as diffuse subepithelial lymphoid aggregates. Another lymphoid structure that complies with the definition of a tissue is found in the gill epithelium and is referred to as interbranchial lymphoid tissue (ILT). Although the function of ILT is yet to be elucidated, it is considered to represent a phylogenetically early form of leukocyte accumulation in a respiratory organ (9–11). Another special feature of teleost fish is the production of a unique immunoglobulin, IgT, that is suggested to be specialized for mucosal immunity and to possess similar functions to mammalian IgA, although IgT, and IgA are phylogenetically distant immunoglobulins (12).
Mucosal delivery of vaccines, for example, via immersion or oral immunization, is the preferred vaccination method for preventing infectious diseases in aquaculture (13). These vaccination methods significantly decrease the working cost of vaccination in aquaculture since they are suitable approaches for mass vaccination. Vaccine antigens that are administered via the oral route are taken up by the intestinal epithelium of teleost fish (14). The first evidence for the existence of M cells in fish was found in rainbow trout, in which the M-like cells were shown to exhibit similar characteristics to mammalian M cells, exemplified by their morphology (with openly arranged microvilli) and their affinity for the lectin UEA-1 but not WGA (15). In zebrafish, M-like cells have not been yet described, but nanoparticles, and bacteria (Mycobacterium marinum) are taken up in the intestinal epithelium when administered via an oral route (16). Bath vaccination of teleost fish is effective against pathogenic bacteria such as Aeromonas salmonicida subsp. salmonicida (Ass) (17), Vibrio anguillarum (18), and Yersinia ruckeri (19). Large numbers of fish are dipped into a vaccine solution that is traditionally composed of formalin-killed bacteria. While soluble antigens in the vaccine solution are mainly taken up via the skin (20), particulate antigens, such as bacterins from Y. ruckeri, are taken up not only via the gill epithelium but also via the gastrointestinal tract, probably after ingestion of the vaccine solution (21, 22). Kato et al. (23) showed that V. anguillarum bacterin was taken up primarily via gill epithelial cells, inducing the up-regulation of inflammatory cytokine genes. However, little is known about the exact antigen-sampling mechanisms in the gill epithelium of teleost fish or about the cell populations involved and the resulting local and systemic immune responses.
In this study, we identified and characterized two types of antigen-sampling cells in the rainbow trout gill epithelium that are involved in bacterin uptake during bath vaccination: resident DC/macrophage-type cells in the gill epithelium and another group of antigen-sampling cells that exhibit phenotypic characteristics of M cells, expressing MHC class II molecules on their surface. The M-type antigen-sampling cells showing MHC class II expression were significantly increased in the gill epithelium after bath vaccination. Thus, we hypothesize that antigen-sampling cells are involved in direct antigen presentation to T cells in the gill mucosal tissue of teleosts, which lack highly organized lymphoid organs.
Materials and Methods
Fish Rearing and Bacterial Propagation
Rainbow trout (56–126 g body weight) were reared in 400 L tanks at 15°C in a recirculating water system at the Friedrich-Loeffler-Institut and the Tokyo University of Marine Science and Technology. Fish were anesthetized with benzocaine or 2-phenoxyethanol prior to dissection. All animal experiments were approved by the Institutional Animal Care and Use Committee in TUMSAT and FLI. Ass bacteria isolated from diseased rainbow trout were cultured in veal infusion broth (VIB) or tryptic soy broth (TSB). V. anguillarum (serotype J-O-1, J-O-2, and J-O-3) isolated from diseased ayu Plecoglossus altivelis were cultured in TSB. The cultured bacteria were inactivated in 0.3% formalin overnight and then washed.
Immunohistochemistry
Rainbow trout were dipped into a vaccine solution initially corresponding to 2.6–4.4 × 108 CFU/ml of Ass bacterin, followed by incubation for 30 min. The gills of the bath-vaccinated fish were fixed overnight in Davidson's solution, embedded in paraffin and sectioned at a 3 μm thickness. The sections were subsequently incubated with polyclonal rabbit antiserum raised against Ass (1:1000 dilution) for 1 h at 4°C. After being washed three times with 1 × tris buffered saline (TBS), the sections were treated with a VECTASTAIN ABC Rabbit IgG kit (VECTOR Laboratories) following the manufacturer's instructions. Next, the sections were washed again, treated with 50 mM Tris-HCl (pH 7.6) containing 0.02% 3,3′-diaminobenzidine tetrahydrochloride (Dojindo) and 0.03% H2O2 for 3 min, and counterstained with hematoxylin. For immunofluorescence assays, sections stained with the anti-Ass serum were incubated with a UEA-1 TRITC conjugate (final concentration, 10 μg/ml; Sigma-Aldrich) or WGA rhodamine conjugate (10 μg/ml, VECTOR Laboratories) for 30 min at 4°C. A goat anti-rabbit IgG (H+L) Alexa Fluor 488 conjugate (4 μg/ml, Thermo Fisher Scientific) was used as a secondary antibody to detect the Ass bacterin. Paraffin sections of gills from unvaccinated fish were also stained with UEA-1 FITC conjugate (10 μg/ml, Sigma-Aldrich) and WGA rhodamine conjugate, as above. Cryosections of gills sampled 3 h after ex vivo bath vaccination were fixed with acetone for 10 min and incubated with an anti-rainbow trout MHC class II mAb (24) (a gift from Dr. C. Tafalla) for 30 min at 4°C. The slides were then washed three times and incubated with goat anti-mouse IgG (H+L) Alexa Fluor 555 (2 μg/ml, Thermo Fisher Scientific) for 30 min at 4°C. The sections were finally stained with Hoechst 33342 (Thermo Fisher Scientific) and mounted with ProLong Gold Antifade Mountant (Thermo Fisher Scientific), and digital images were captured and analyzed with a Nikon ECLIPSE Ti S fluorescence microscope and NIS Elements software (Nikon).
Flow Cytometry
Formalin-inactivated Ass and V. anguillarum J-O-1, J-O-2, and J-O-3 were stained with SYTO 61 (Thermo Fisher Scientific). The gills were treated with bacterins ex vivo as described by Torroba et al. (25). Briefly, the gills were removed from anesthetized naïve fish and washed twice using RPMI 1640 medium for 5 min with a stirring at 400 rpm. Four pieces of the gills were dipped into SYTO 61-stained bacterin (1.0–2.0 × 108 CFU/ml) or 1.0 × 107 particles/ml of Fluoresbrite YG Carboxylate microspheres (0.97 μm, Polysciences) suspended in RPMI 1640 medium (Nissui) for 30 min with a stirring at 400 rpm. The other four pieces of the gills were incubated in RPMI 1640 as above and used as a negative control. It was confirmed that the data obtained using the ex vivo method were equivalent to those from in vivo bath vaccination.
After ex vivo bath vaccination with SYTO 61-stained bacterin, gills were washed twice with RPMI 1640 medium. For separation of the gill epithelial cells, the gills were incubated in PBS containing 10 mM EDTA with a stirring at 400 rpm for 20 min at 4°C. The cells were then washed three times with RPMI 1640, counted, and resuspended in RPMI 1640 at 0.84–1.64 × 108 cells/ml (>85% viability). One-hundred microliter of the cell suspension was incubated with FITC-labeled UEA-1 diluted in the cell culture medium (10 μg/ml). Further, UEA-1-treated cells were also stained with a mAb against rainbow trout MHC class II (1: 1,000 dilutions in the cell culture medium) for 1 h at 4°C, and a goat anti-mouse IgG PE/Cy 5.5 conjugate (0.25 μg/ml, Abcam) diluted in RPMI 1640 was used as secondary antibodies. The epithelial cells from the gills dipped into the microspheres were stained with UEA-1 (10 μg/ml in RPMI 1640, without fluorescent conjugation). A rabbit anti-UEA-1 polyclonal antibody (5 μg/ml in RPMI 1640, Bioss Antibodies) and goat anti-rabbit IgG (H+L) Alexa 647 (2 μg/ml in RPMI 1640, Abcam) were used as secondary and tertiary antibodies to detect UEA-1, respectively. Afterbeing washed three times with RPMI 1640, the cells were re-suspended in the cell culture medium and stained with DAPI (4',6-diamidino-2-phenylindole, 1 μg/ml in RPMI 1640; Life Technologies). The epithelial cells collected from the gills exposed to RPMI 1640 were incubated with the secondary antibodies and used as negative controls. Flow cytometry analyses of 10,000 cells in each group were performed using a CytoFlex (Beckman Coulter). Data from the cytometers were analyzed with Kaluza Flow Cytometry Analysis Software (Beckman Coulter).
Cell Sorting by Flow Cytometry
Formalin-inactivated Ass were stained with SYTO 61 (Thermo Fisher Scientific) and used for bath vaccination. Rainbow trout were dipped into a vaccine solution initially corresponding to 1.0–2.0 × 108 CFU/ml of SYTO 61-stained bacterins, followed by incubation for 30 min. After in vivo bath vaccination with SYTO 61-stained bacterin, gills were washed twice with the ZB28 cell culture medium (50/50 mixture of Iscove's and Ham's F12 medium). The gill epithelial cells were prepared and stained with FITC-labeled UEA-1 (10 μg/ml in ZB28), as above. After being washed three times with ZB28, the cells were re-suspended in the cell culture medium and stained with propidium iodide (PI, 2 μg/ml; Life Technologies) or DAPI (4',6-diamidino-2-phenylindole, 1 μg/ml; Life Technologies). The gill epithelial cells collected from rainbow trout dipped into rearing water without SYTO61-stained bacterin were prepared and used as negative control. Cell sorting was performed with a MoFlo high-speed cell sorter (Dako Cytomation) or a FACSAria Fusion cell sorter (BD Biosciences). First, PI+ or DAPI+ cells were gated to exclude sorting dead cells. Then, 30,000 cells of UEA-1− Ass− population as a negative control, UEA-1− Ass+ population and UEA-1+ Ass+ population were sorted. Data from the cytometers were analyzed with Kaluza Flow Cytometry Analysis Software (Beckman Coulter).
Gene Expression Analysis
Total RNA was extracted from sorted cells using a NucleoSpin RNA XS kit (Machery-Nagel), following the manufacturer's instructions. Equal amounts of total RNA from each sorted cell population of three fish individuals were pooled prior to library construction. RNA libraries were constructed using the Illumina TruSeq Stranded mRNA Sample Preparation Kit (Illumina), following the manufacturer's instructions. Sequencing was performed using the Illumina MiSeq platform, employing the MiSeq Reagent Kit v2 (Illumina), with 151 paired-end reads. The whole transcriptome sequence raw data were deposited in the DDBJ Sequence Read Archive (Accession Number: DRA006692). De novo assembly of the MiSeq reads and gene expression analysis using the fragments per kilobase of exon per million mapped reads (FPKM) model was performed with Trinity v2.1.1 software (https://github.com/trinityrnaseq/trinityrnaseq). The assembled sequences were annotated using the BLAST program at the UniProtKB database (http://www.uniprot.org/help/uniprotkb) and using Blast2GO software (https://www.blast2go.com/). The UniProtKB accession numbers of the expressed genes of each library were submitted to Venny 2.1 software (http://bioinfogp.cnb.csic.es/tools/venny/) to construct a Venn diagram. The KAAS (http://www.genome.jp/tools/kaas/) was employed for orthologue assignment and pathway mapping.
Total RNA was extracted from each sorted cell population using Nucleospin RNA XS kit (Machery-Nagel), following manufacturer's instructions. First-strand cDNAs were synthesized using 100 ng of total RNA using M-MLV reverse transcriptase (Thermo Fisher Scientific). The primers employed for qRT-PCR are shown in Table S3. The expression levels of several genes of interest in each sorted cell population from three individuals were analyzed by quantitative RT-PCR (qRT-PCR) using THUNDERBIRD SYBR qPCR Mix (TOYOBO), following the manufacturer's instructions. Since the PCR efficiency of all primer sets for qPCR were higher than 95%, the relative values for expression levels were calculated using the 2−ΔΔCt method, taking into account the expression of elongation factor 1α (Accession No. AF498320) as an internal control. Significant differences among samples were assessed using one-way analysis of variance (ANOVA) and Tukey's post-hoc test. P < 0.05 were considered statistically significant.
Results
Identification of Two Phenotypes of Antigen-Sampling Cells in the Gill Epithelium of Rainbow Trout
Since in antigen sampling in rainbow trout has been studied only using live Ass (26), and the site of Ass bacterin uptake is still unknown, we first aimed to determine the localization of the respective antigen-sampling cells. Immunohistochemistry analysis showed that inactivated Ass bacteria were frequently taken up by gill epithelial cells after bath vaccination (Figure 1A). Lectin staining revealed numerous UEA-1+ WGA− cells in the epithelial cells of the primary and secondary lamellae. This UEA1+ WGA− phenotype, which is typical of mouse M cells, was found both in the epithelia of the outer lamellae and close to the base of the gill filament's septae, where ILT is located (Figure 1B). UEA-1+ WGA+ staining, which is typical of mammalian goblet cells, was scarcely observed in the gill epithelial cells of rainbow trout. Double staining with an anti-Ass polyclonal antibody and either of the lectins after bath vaccination revealed that both UEA-1+ and UEA-1− cells were able to take up Ass bacterin (Figure 1C), while WGA+ cells were not (Figure 1D). To further characterize the antigen-sampling cells, gill epithelial cells were isolated and analyzed via flow cytometry. Similar to the immunohistochemistry analysis, flow cytometry revealed two cell populations that take up Ass bacterin in the gill epithelium: UEA-1+ cells and UEA-1− cells (Figure 2A left panel). These two populations showed distinct side scatter characteristics, with higher side scatter being observed in UEA-1+ Ass+ cells than in UEA-1− Ass+ cells, suggesting that UEA-1+ Ass+ cells are more highly granulated than UEA-1− Ass+ cells (Figure 2A right panel). Since Kato et al. (23) showed previously that V. anguillarum bacterin is taken up by the gill epithelium in bath-vaccinated Japanese flounder, we further analyzed gill epithelial cells of rainbow trout that were bath vaccinated with V. anguillarum (J-O-1, J-O-2, and J-O-3 serotype) bacterin. Similar to Ass bacterin, V. anguillarum bacterin was taken up by UEA-1+ cells (Figures 2B–D left panels). Additionally, UEA-1− V. anguillarum+ cells were observed in the gill epithelium of fish that were bath vaccinated with V. anguillarum bacterin (Figures 2B–D right panels). However, fluorescence microbeads were taken up neither by UEA-1+ nor UEA-1− cells (Figure 2E). In addition, the gill epithelial cells that took up Ass bacterin (Figure S1A) did not react with mAbs against CD8α, IgM, and thrombocytes (Figures S1B–D). Based on these results, we identified two cell populations that take up bacterins in the gill epithelium of bath-vaccinated rainbow trout.
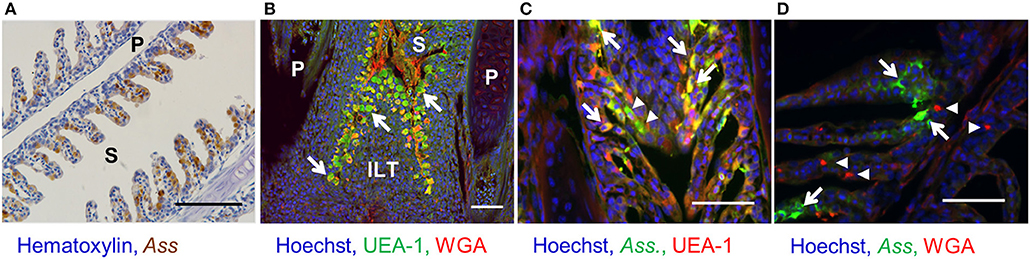
Figure 1. Bacterin uptake by UEA-1+ and UEA-1− gill epithelial cells after bath vaccination. (A) Immunohistochemistry for the detection of Ass bacterin in paraffin section of gills from bath-vaccinated fish. Scale bars, 100 μm. (B) Lectin staining in a paraffin gill section. White arrows indicate UEA-1+ WGA− cells. Scale bars, 50 μm. (C) Fluorescent staining with an anti-Ass polyclonal antibody (in green) and the lectin UEA-1 (in red) in gill paraffin section from bath-vaccinated fish. White arrows and white arrowheads indicate UEA-1+ and UEA-1− antigen-sampling cells, respectively. Scale bars, 50 μm. (D) Fluorescent staining with an anti-Ass polyclonal antibody (in green) and the lectin WGA (in red) in gill paraffin section from bath-vaccinated fish. White arrows and arrowheads indicate antigen-sampling cells and WGA-stained cells, respectively. Scale bars, 50 μm. P, primary lamellae; S, secondary lamellae; ILT, interbrachial lymphoid tissue. Experiments were done by in vivo bath vaccination and data are from one experiment (A) and representative of three experiments (B–D).
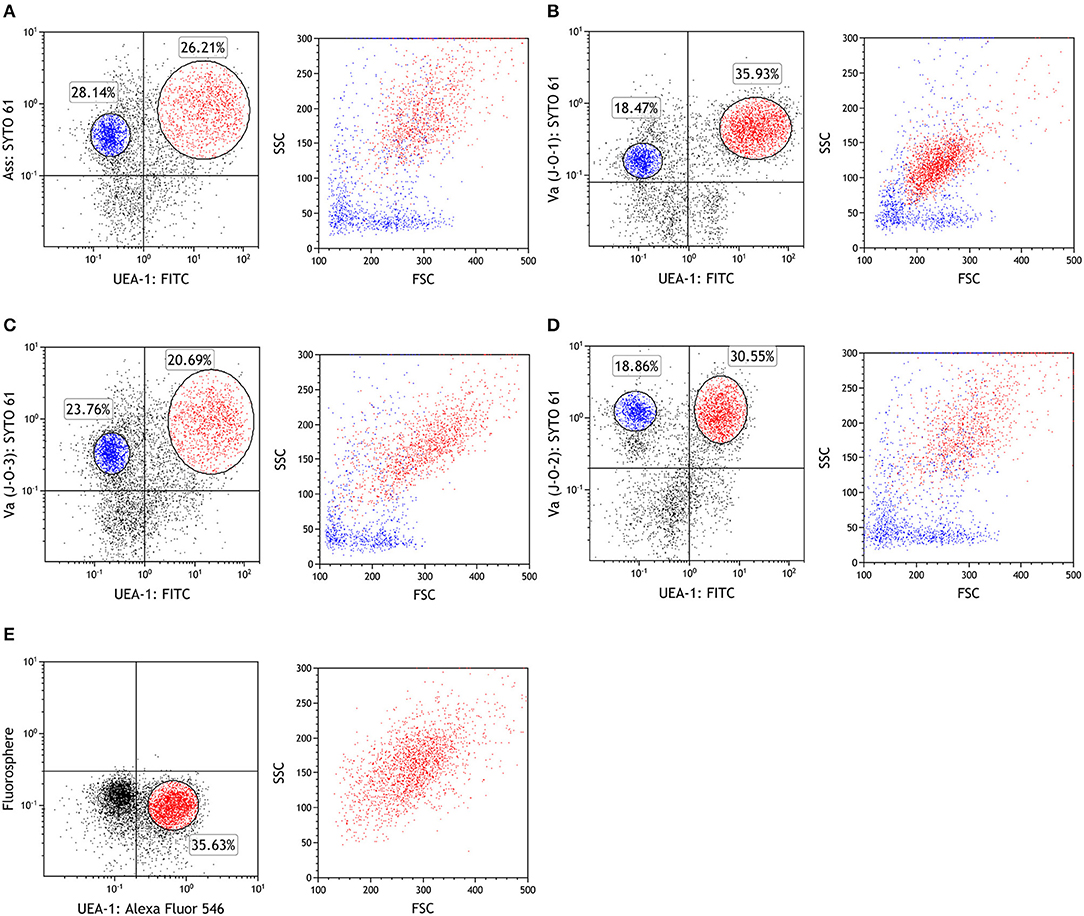
Figure 2. UEA-1+ and UEA-1− gill epithelial cells take up bacterins but not microbeads during bath administration. (A–D) Flow cytometry of fish gill epithelial cells bath vaccinated with Ass bacterin in (A), with V. anguillarum J-O-1 serotype bacterin in (B), with V. anguillarum J-O-2 serotype bacterin in (C), and with V. anguillarum J-O-3 serotype bacterin in (D). (E) Flow cytometry of gill epithelial cells from fish immersed with fluorescent microbeads (diameter: 1 μm). Left panels show dot plots of depicting antigen or bead staining on the Y-axis against UEA-1 staining on the X-axis. Red dots represent UEA-1+ bacterin+ cells, while blue dots represent UEA-1− bacterin+ cells in the right panels. Experiments were done by ex vivo bath vaccination and data are representative of two experiments.
Characterization of the Two Phenotypes of Antigen-Sampling Cells
To characterize the two antigen-sampling cell populations in the gill epithelium, the UEA-1+ Ass+ and UEA-1− Ass+ cell populations and the negative cell population were sorted via flow cytometry (Figure S2). May-Grünwald-Giemsa (MGG) staining of the sorted cells confirmed that UEA-1+ Ass+ cells exhibited round nuclei, with reddish-purple granules and small vacuoles in the cytoplasm (Figure 3A). Most of the UEA-1− Ass+ cells showed typical monocyte/macrophage morphologies, including oval nuclei with a homogenous chromatin pattern and pale-blue cytoplasm with vacuoles (Figure 3B). Negative cell population included many cell phenotypes such as erythrocytes, monocyte-like cells and others (Figure 3C). Total RNA samples from the sorted cells were subjected to transcriptome analysis. Deep sequencing yielded 174,245 contigs from sixty-five million total reads (Table S1) and revealed clear differences in gene expression patterns among the three cell populations (Figure 3D). Venn diagrams of the three RNA libraries showed that there were 5,002, 30,290, and 39,260 unique genes expressed in the negative, UEA-1+ Ass+ and UEA-1− Ass+ cell populations, respectively, (Figure 3E). Pathway analysis using the Kyoto Encyclopedia of Genes and Genome Automatic Annotation Server (KAAS) revealed that the RNA libraries from both UEA-1+ Ass+ and UEA-1− Ass+ cells contained an almost full set of genes related to the “lysosome,” “phagosome,” and “antigen processing and presentation” pathways (Table S2, and Figures S3, S4). Genes of interest that were highly expressed in UEA-1+ Ass+ and UEA-1− Ass+ cells are shown in Table 1. Several genes encoding epithelial cell markers, CDH1, Cldn3, KRT13, KRTS8, KRTE1, KRTE3, and IL-17R, were highly expressed in UEA-1+ Ass+ cells. Several genes that are highly expressed in mammalian M cells, such as Anxa5, Ctsh, CCL20, Dnclc, and Gpx I, were highly expressed in UEA-1+ Ass+ cells as well. However, UEA-1+ Ass+ cells also expressed MHC-IIA, MHC-IIB, and MHC-II Ii at high levels. Macrophage, monocyte and dendritic cell makers such as CD83, IL-1β, IL-10R, and IL-12 p40 were highly expressed in the UEA-1− Ass+ cell population, while these genes were not expressed or negligibly expressed in UEA-1+ Ass+ cells. Gene expression levels determined via MiSeq sequencing in the negative, UEA-1+ Ass+ and UEA-1− Ass+ cell populations were confirmed using qRT-PCR. The expression level of the M cell marker gene Anxa5 was significantly higher in UEA-1+ Ass+ cells than in the negative and UEA-1− Ass+ cell populations (p < 0.05, Figure 4A). The epithelial cell markers Cldn3, CDH1, KRT13, KRTE1, and IL-17R also showed significantly higher expression in UEA-1+ Ass+ cells than in the other two cell populations (p < 0.01, Figures 4B–F). In contrast, the gene expression levels of CD83, IL-1β, and IL-12 p40 were significantly higher in UEA-1− Ass+ cells (p < 0.05, Figures 4G–I). In addition, the gene expression level of PTPRC (CD45), a lineage maker for hematopoietic origin, was significantly higher in UEA-1− Ass+ cells (p < 0.05, Figure 4J). qRT-PCR analyses further confirmed the significantly higher expression level of MHC-IIB and MHC-II Ii in UEA-1+ Ass+ cells and UEA-1− Ass+ cells than in the negative cell population (p < 0.05, Figures 4K–L). Despite the high mRNA levels of MHC class II-related genes observed in UEA-1+ Ass+ cells, we conclude that these cells do not belong to the macrophage/monocyte/dendritic cell lineage but to a cell type that is reminiscent of M cells. The second class of antigen-sampling cells, the UEA-1− Ass+ cell population, is suggested to be gill epithelium-resident macrophages, monocytes or dendritic cells.
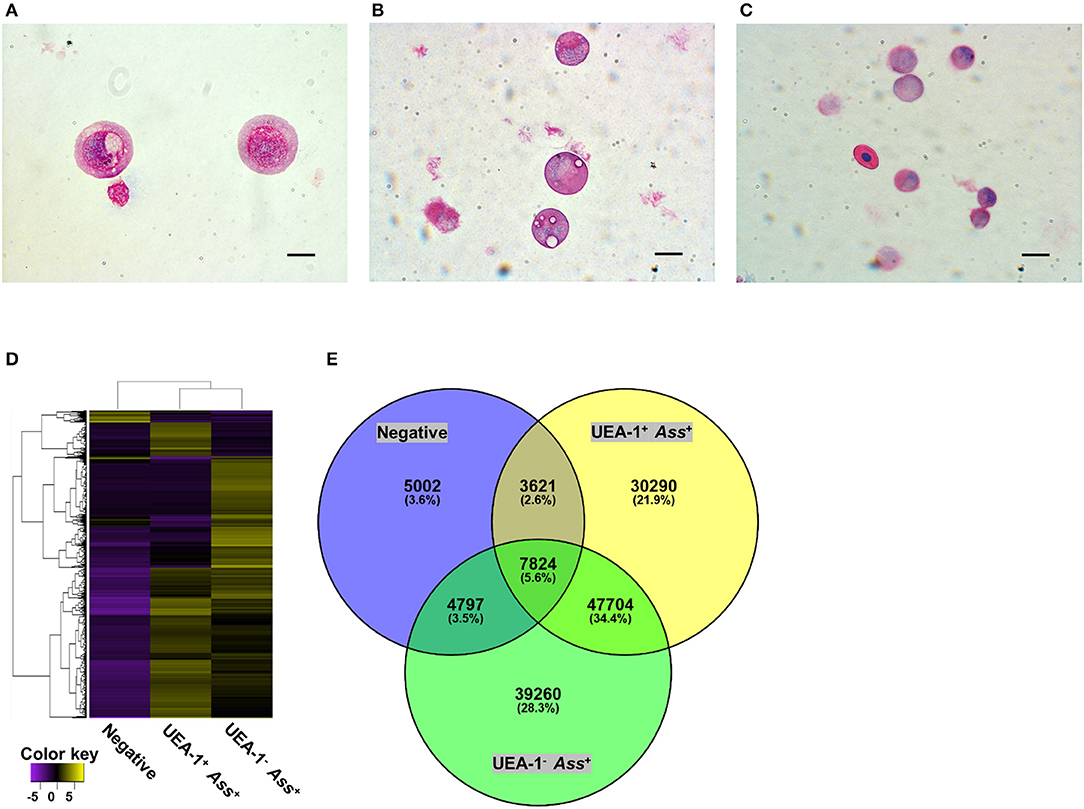
Figure 3. The two antigen-sampling cell populations in the gill epithelium show distinct morphologies and gene expression profiles. (A–C) May-Grünwald-Giemsa staining of sorted UEA-1+ Ass+ cells in (A), UEA-1− Ass+ cells in B, and negative cell population in (C). scale bars, 10 μm. (D) Heat map of differentially expressed genes in each sample, constructed with the Trinity program. (E) Venn diagram summary of differentially expressed genes in the UEA-1+ Ass+, UEA-1− Ass+ and negative cell populations. Experiments were done by in vivo bath vaccination and data are representative of two experiments (A–C) or come from one experiment involving three individuals (D,E).
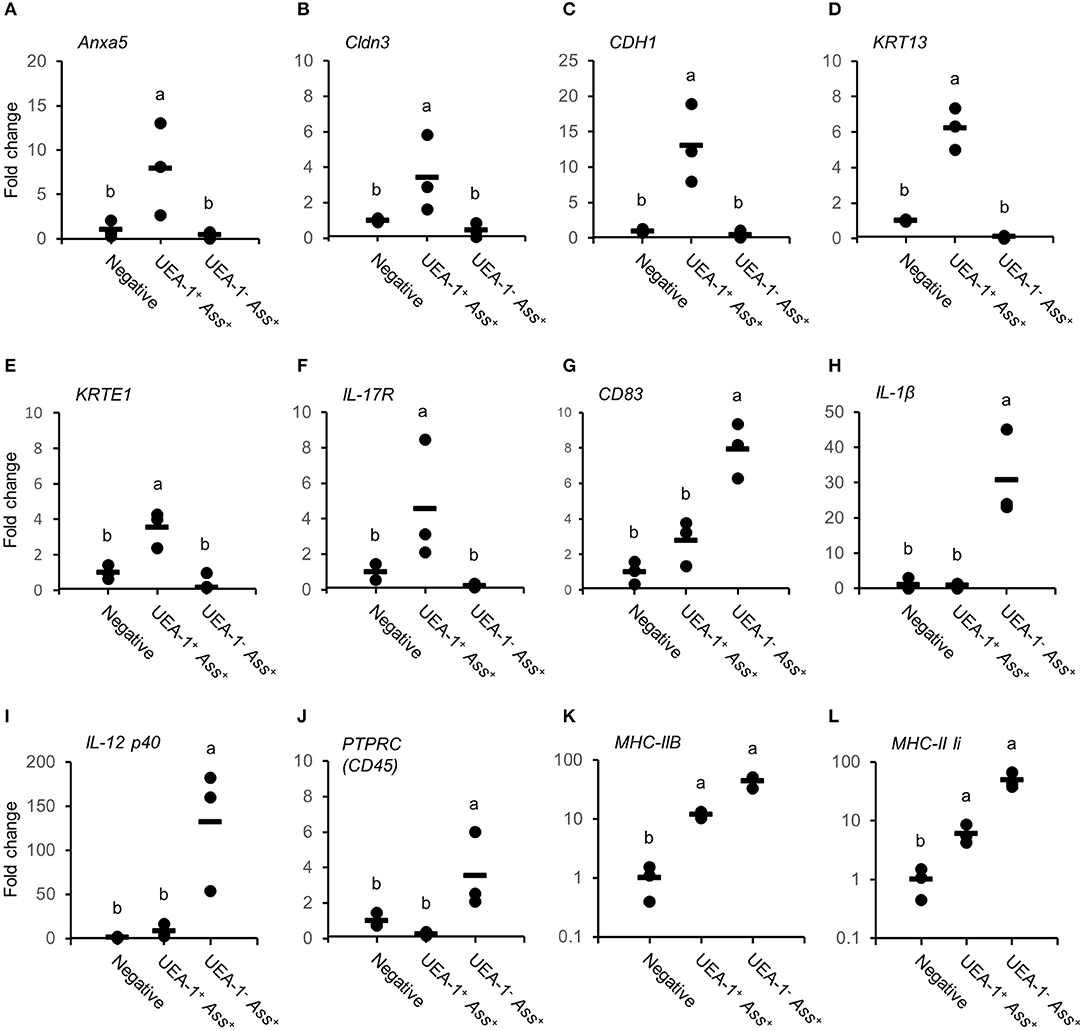
Figure 4. Expression levels of genes expressed in UEA-1+ Ass+ and in UEA-1− Ass+ cells. (A–L) Gene expression levels of Anxa5 in (A), Cldn3 in (B), CDH1 in (C), KRT13 in (D), KRTE1 in (E), IL-17R in (F), CD83 in (G), IL-1β3 in (H), IL-12p40b in (I), PTPRC (CD45) in (J), MHC-IIB in (K), and MHC-II Ii in (L), in the three sorted cell populations after bath vaccination. Each dot indicates the gene expression level relative to the mean expression level in the negative cell population, which was set as 1. Horizontal lines indicate the mean expression levels (n = 3). Different letters represent significant differences between cell populations (p < 0.05, one-way ANOVA with Tukey's post-hoc test). Experiments were done by in vivo bath vaccination and Data are from one experiment with three individual fish.
MHC Class II Expression in the Two Phenotypes of Antigen-Sampling Cells
Since almost all UEA-1+ gill epithelial cells were M-type antigen-sampling cells, the fraction of MHC class II+ cells among UEA-1+ population was compared between mock-vaccination and ex vivo-vaccination. In the mock-vaccination, 17.7% of UEA-1+ cells showed MHC class II expression on their surface (Figure 5A), while the percentage of MHC class II+ UEA-1+ cells in the ex vivo-vaccination was 26.2% (Figure 5B). The difference was statistically significant between mock-vaccination and ex vivo-vaccination (p < 0.05, Figure 5C). The presence of UEA-1+ MHC class II+ cells in the gill epithelium after ex vivo-vaccination was confirmed by fluorescence microscopy (Figure 5D).
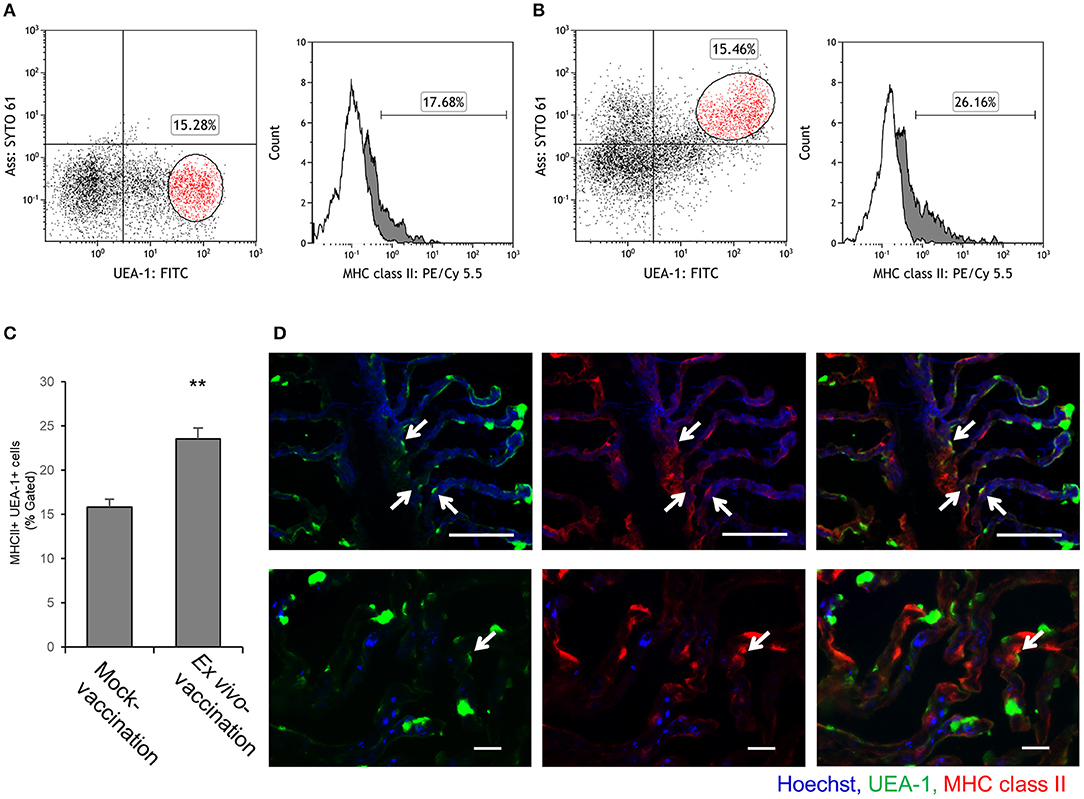
Figure 5. MHC class II expression on UEA-1+ cells after ex vivo bath vaccination with Ass bacterin. (A,B) Flow cytometry for UEA-1, Ass and MHC class II staining of gill epithelial cells from the gills ex vivo bath-vaccinated with Ass bacterin in A and mock-vaccinated in B. Left panels show dot plots for UEA-1 and Ass staining of gill epithelial cells. Right panels show the percentage of MHC class II+ cells, gated on the UEA-1+ cell population. White areas show cells treated only with secondary antibodies (conjugate control), and gray areas show cells positive for MHC class II. (C) Mean values for the percentage of MHC class II+ cells gated for UEA-1+ gill epithelial cells of mock-vaccination and ex vivo bath-vaccination (n = 3). ** p < 0.01, Student's t-test (two-sided). (D) MHC class II+ UEA-1+ gill epithelium cells in the gills ex vivo bath-vaccinated with Ass bacterin in lower magnification (upper panels) and higher magnification (lower panels). Arrows indicate MHC class II+ UEA-1+ cells. P, primary lamellae; S, secondary lamellae; scale bar, 50 μm (upper panels) and 20 μm (lower panels). Experiments were done by ex vivo bath vaccination. Data are representative of three experiments (A,B), or one experiment with three individuals (C), or representative of three experiments (D).
Discussion
The mechanisms of antigen sampling in the mucosal epithelium of teleost fish are mostly unknown. In mammals, M cells in the gut mucosal epithelium take up antigens and deliver them to MALT, where antigen presentation by APCs occurs, and a local immune response is induced. Although teleost fish possess an acquired immune system, they lack highly organized lymphoid structures consisting of lymphoid follicles and germinal centers (8). In this study, we identified two phenotypes of gill epithelial antigen-sampling (GAS) cells in rainbow trout: M-type GAS cells with similar characteristics to mammalian M cells; and macrophage/DC-type GAS cells. Furthermore, we demonstrated that the M-type GAS cells show the potential for direct antigen presentation in the gill epithelium. These results suggest an unconventional system of antigen sampling and presentation in the teleost gill epithelium. Our findings may shed light on the mechanisms of local mucosal immune response induction in gill-breathing vertebrates.
It was shown in this work that M-type GAS cells are able to take up bacterins but not fluorescence microbeads during bath administration, suggesting a receptor-based mechanism. The engulfment of bacteria by M cells is dependent on the recognition of bacteria by several receptors. For example, mammalian M cells are able to take up wild-type Escherichia coli but not FimH-deficient E. coli since glycoprotein 2, a receptor expressed on M cells, selectively binds to FimH on the outer membrane of the bacteria (4). The gill epithelium is the major route for the entry of bacterins of species such as Y. ruckeri (21) and V. anguillarum (23) during bath vaccination. Immunohistochemistry analyses revealed that the gill epithelial cells actively sample vaccine antigens during bath vaccination (21, 23, 25, 27), while fluorescent microbeads administered by bath immersion are rarely taken up (28). These data suggest that M-type GAS cells take up antigens with a certain pattern through receptor-based mechanisms.
The M-type GAS cells showed similar characteristics to mammalian M cells, exemplified by their affinity for lectins (UEA-1+ WGA−) and by high expression of Anxa5. Other typical gene markers of mammalian M cells, such as CCL20, Ctsh, Dnclc, and Gpx I (29, 30), were also expressed in M-type GAS cells, although at similar levels to those in negative and macrophage/DC-type GAS cells, based on qRT-PCR analysis (data not shown). The α (1, 2) fucose carbohydrate moiety recognized by UEA-1 has been suggested to play a role in antigen trapping by M cells (31). A role for annexin V, which is important in endocytic transport and membrane scaffolding, has been suggested in M cell-mediated transcytosis (30). The gene expression patterns of M-type GAS cells suggest that they are closely related to mammalian M cells.
The main function of M cells is transcytosis of antigens to dendritic cells and macrophages in mammalian MALT (7). Although there are several reports suggesting that MHC class II is expressed on M cells (32, 33), the general view is that these cells are unable to process and present antigens to T cells since they are markedly deficient in lysosomes (34). In contrast, M-type GAS cells were found to exhibit small vacuoles in their cytoplasm and to express almost all genes related to the “phagosome,” “lysosome,” and “antigen processing and presentation” pathways. Further, MHC class II was constitutively expressed on a fraction of M-type GAS cells, which was significantly increased after bath vaccination, suggesting that the MHC class II expression is inducible by antigen stimulation. Similar to our results, MHC class II expression in the gill epithelium has also been reported in Atlantic salmon infected with Neoparamoeba sp. amoebae, although the corresponding cells have not been fully characterized (35). Thus, M-type GAS cells are more likely to present antigens in the gill epithelium than bona-fide mammalian M cells in the intestinal epithelium.
In mammals, cells other than M cells in mucosal tissues are able to take up antigens. These cells include type II alveolar epithelial cells, keratinocytes and goblet cells. Multiple reports suggest that most of these epithelial cells show constitutive MHC class II expression and present antigens to T cells (36). Debbabi et al. (37) reported that type II alveolar epithelial cells present exogenous antigens from Mycobacterium tuberculosis through MHC class II and that they can prime antigen-specific CD4+ T cells. Keratinocytes are also able to efficiently process and present soluble antigens only to CD4+ memory T cells, resulting in Th1 and Th2 cytokine secretion (38). However, in the present study, no genes characteristic of type II alveolar epithelial cells and keratinocytes, such as surfactant protein A, cytokeratin-8, keratin-6, and aquaporin-3 (39), were found in the mRNA library from M-type GAS cells. Due to the unique gene expression pattern observed in this phenotype of GAS cells and the fact that this cell population constitutively expresses MHC class II in teleosts, M-type GAS cells should be a different cell type from mammalian intestinal M cells.
It has been shown in the past that after bath vaccination with bacterins, the corresponding antigens are transported to the head kidney, trunk kidney and spleen via the blood circulation (21). It has been proposed that vaccine components are processed primarily at the site of melano-macrophage centers in lymphoid organs (40). The spleen and head kidney are the major lymphoid organs of teleost fish (41), and their melano-macrophage centers are considered to be the phylogenetic precursors of germinal centers in higher vertebrates (42). Mammalian macrophages and dendritic cells play an important role in antigen transportation. Rességuier et al. (43) showed that dendritic cells take up surfactant-free poly (lactic acid) nanoparticles in the gill epithelium. In the present work, numerous gill epithelium-resident macrophage/DC-like GAS cells that contained bacterin were found following bath vaccination. This second type of GAS cells might be important for the transportation of vaccine antigens into the lymphoid organs and for the subsequent induction of systemic immune responses, such as the production of specific antibodies (44).
Antigen-specific mucosal immune responses have been frequently reported in mammals (45). Recently, Xu et al. (46) further showed that rainbow trout survived an infection with the parasite Ichthyophthirius multifiliis by inducing local proliferation of IgT+ B cells and IgT production in the gills. In addition, Kai et al. demonstrated that high IgT levels are induced by bath and immersion vaccination, whereas injection vaccination induces high IgM levels (47). These reports suggest that distinct local mucosal immune responses have evolved during the course of vertebrate evolution. Here, we suggest that teleost M-type GAS cells represent a primitive type of epithelial antigen-sampling cells. Bona-fide M cells are critical in terms of inducing local mucosal immune responses in mammalian MALTs, mainly via the transcytosis of antigens (7). Teleost M-type GAS cells may play a similar role in the phylogenetically primitive teleost immune system. In addition, the presence of MHC class II expression suggests an additional role in antigen presentation in the gills, which are an organ with high T cell abundance, especially in ILT (9, 10). Moreover, the results of this work may be valuable for the development of mucosal vaccines that specifically target GAS cells. Due to the rapidly growing aquaculture industry and declining capture fishery resources, effective mucosal vaccines for farmed fish are urgently needed. Bath vaccines significantly reduce working costs and the stress that is usually induced by vaccination via injection of individual fish.
Availability of Data and Material
The whole transcriptome sequence raw data were deposited in the DDBJ Sequence Read Archive (Accession Number: DRA006692).
Author Contributions
GK and UF designed the study. GK wrote the manuscript. GK, UF, HM, YN, YI, and TY performed the experiments. HK analyzed the RNA-seq data. MS, UF, and TY edited the manuscript.
Funding
This work was supported in part by KAKENHI (26850128 and 16H06201) and by the FP7 EU project TargetFish (311993).
Conflict of Interest Statement
The authors declare that the research was conducted in the absence of any commercial or financial relationships that could be construed as a potential conflict of interest.
Acknowledgments
We thank Dr. C. Tafalla for giving us the monoclonal antibody against rainbow trout MHC class II. Ms. G. Czerwinski for assistance with immunohistochemistry. and Ms. S. Schares for assistance with bacterial propagation and cell preparation.
Supplementary Material
The Supplementary Material for this article can be found online at: https://www.frontiersin.org/articles/10.3389/fimmu.2018.02116/full#supplementary-material
References
1. Hein WR. Organization of mucosal lymphoid tissue. Curr Top Microbiol Immunol. (1999) 236:1–15. doi: 10.1007/978-3-642-59951-4_1
2. Bockman DE, Cooper MD. Pinocytosis by epithelium associated with lymphoid follicles in the bursa of Fabricius, appendix, and Peyer's patches. An electron microscopic study. Am J Anat. (1973) 136:455–77. doi: 10.1002/aja.1001360406
3. Clark MA, Jepson MA, Simmons NL, Booth TA, Hirst BH. Differential expression of lectin-binding sites defines mouse intestinal M-cells. J Histochem Cytochem. (1993) 41:1679–87. doi: 10.1177/41.11.7691933
4. Hase K, Kawano K, Nochi T, Pontes GS, Fukuda S, Ebisawa M, et al. Uptake through glycoprotein 2 of FimH (+) bacteria by M cells initiates mucosal immune response. Nature (2009) 462:226–30. doi: 10.1038/nature08529
5. Clark MA, Hirst BH, Jepson MA. M-cell surface beta1 integrin expression and invasin-mediated targeting of Yersinia pseudotuberculosis to mouse Peyer's patch M cells. Infect Immun. (1998) 66:1237–43.
6. Helander A, Silvey KJ, Mantis NJ, Hutchings AB, Chandran K, Lucas WT, et al. The viral σ1 protein and glycoconjugates containing α2-3-linked sialic acid are involved in type 1 reovirus adherence to M cell apical surfaces. J Virol. (2003) 77:7964–77. doi: 10.1128/JVI.77.14.7964-7977.2003
7. Mabbott NA, Donaldson DS, Ohno H, Williams IR, Mahajan A. Microfold (M) cells: important immunosurveillance posts in the intestinal epithelium. Mucosal Immunol. (2013) 6:666–77. doi: 10.1038/mi.2013.30
8. Zapata A, Amemiya CT. Phylogeny of lower vertebrates and their immunological structures. Curr Top Microbiol Immunol. (2000) 248:67–107. doi: 10.1007/978-3-642-59674-2_5
9. Haugarvoll E, Bjerkås I, Nowak BF, Hordvik I, Koppang EO. Identification and characterization of a novel intraepithelial lymphoid tissue in the gills of Atlantic salmon. J Anat. (2008) 213:202–9. doi: 10.1111/j.1469-7580.2008.00943.x
10. Koppang EO, Fischer U, Moore L, Tranulis MA, Dijkstra JM, Köllner B, et al. Salmonid T cells assemble in the thymus, spleen and in novel interbranchial lymphoid tissue. J Anat. (2010) 217:728–39. doi: 10.1111/j.1469-7580.2010.01305.x
11. Aas IB, Austbø L, König M, Syed M, Falk K, Hordvik I, et al. Transcriptional characterization of the T cell population within the salmonid interbranchial lymphoid tissue. J Immunol. (2014) 193:3463–9. doi: 10.4049/jimmunol.1400797
12. Zhang YA, Salinas I, Li J, Parra D, Bjork S, Xu Z, et al. IgT, a primitive immunoglobulin class specialized in mucosal immunity. Nat Immunol. (2010) 11:827–35. doi: 10.1038/ni.1913
13. Gudding R, Van Muiswinkel WB. A history of fish vaccination: science-based disease prevention in aquaculture. Fish Shellfish Immunol. (2013) 35:1683–8. doi: 10.1016/j.fsi.2013.09.031
14. Adelmann M, Köllner B, Bergmann SM, Fischer U, Lange B, Weitschies W, et al. Development of an oral vaccine for immunisation of rainbow trout (Oncorhynchus mykiss) against viral haemorrhagic septicaemia. Vaccine (2008) 26:837–44. doi: 10.1016/j.vaccine.2007.11.065
15. Fuglem B, Jirillo E, Bjerkås I, Kiyono H, Nochi T, Yuki Y, et al. Antigen-sampling cells in the salmonid intestinal epithelium. Dev Comp Immunol. (2010) 34:768–74. doi: 10.1016/j.dci.2010.02.007
16. Løvmo SD, Speth MT, Repnik U, Koppang EO, Griffiths GW, Hildahl JP. Translocation of nanoparticles and Mycobacterium marinum across the intestinal epithelium in zebrafish and the role of the mucosal immune system. Dev Comp Immunol. (2017) 67:508–18. doi: 10.1016/j.dci.2016.06.016
17. Villumsen KR, Raida MK. Long-lasting protection induced by bath vaccination against Aeromonas salmonicida subsp. salmonicida in rainbow trout. Fish Shellfish Immunol. (2013) 35:1649–53. doi: 10.1016/j.fsi.2013.09.013
18. Horne MT, Tatner M, McDerment S, Agius C, Ward P. Vaccination of rainbow trout, Salmo gairdneri Richardson, at low temperatures and the long-term persistence of protection. J Fish Dis. (1982) 5:343–5. doi: 10.1111/j.1365-2761.1982.tb00490.x
19. Tebbit GL, Erickson JD, Van de Water RB. Development and use of Yersinia ruckeri bacterins to control enteric redmouth disease. Dev Biol Stand. (1981) 49:395–401.
20. Ototake M, Iwama GK, Nakanishi T. The uptake of bovine serum albumin by the skin of bath-immunised rainbow trout Oncorhynchus mykiss. Fish Shellfish Immunol. (1996) 6:321–33. doi: 10.1006/fsim.1996.0033
21. Ohtani M, Villumsen KR, Koppang EO, Raida MK. Global 3D imaging of Yersinia ruckeri bacterin uptake in rainbow trout fry. PLoS ONE (2015) 10:e0117263. doi: 10.1371/journal.pone.0117263
22. Korbut R, Mehrdana F, Kania PW, Larsen MH, Frees D, Dalsgaard I, et al. Antigen uptake during different life stages of zebrafish (Danio rerio) using a gfp-tagged Yersinia ruckeri. PLoS ONE (2016) 11:e0158968. doi: 10.1371/journal.pone.0158968
23. Kato G, Takano T, Sakai T, Matsuyama T, Nakayasu C. Vibrio anguillarum bacterin uptake via the gills of Japanese flounder and subsequent immune responses. Fish Shellfish Immunol. (2013) 35:1591–7. doi: 10.1016/j.fsi.2013.09.007
24. Granja AG, Leal E, Pignatelli J, Castro R, Abós B, Kato G, et al. Identification of teleost skin CD8α+ dendritic-like cells, representing a potential common ancestor for mammalian cross-presenting dendritic cells. J Immunol. (2015) 195:1825–37. doi: 10.4049/jimmunol.1500322
25. Torroba M, Anderson DP, Dixon OW, Casares F, Varas A, Alonso L, et al. In vitro antigen trapping by gill cells of the rainbow trout: an immunohistochemical study. Histol Histopathol. (1993) 8:363–7.
26. Svendsen YS, Dalmo RA, Bøgwald J. Tissue localization of Aeromonas salmonicida in Atlantic salmon, Salmo salar L., following experimental challenge. J Fish Dis. (1999) 22:125–31. doi: 10.1046/j.1365-2761.1999.00153.x
27. Zapata AG, Torroba M, Alvarez F, Anderson DP, Dixon OW, Wisniewski M. Electron microscopic examination of antigen uptake by salmonid gill cells after bath immunization with a bacterin. J Fish Biol. (1987) 31:209–17. doi: 10.1111/j.1095-8649.1987.tb05315.x
28. Kiryu I, Ototake M, Nakanishi T, Wakabayashi H. The uptake of fluorescent microspheres into the skin, fins and gills of rainbow trout during immersion. Fish Pathol. (2000) 35:41–8. doi: 10.3147/jsfp.35.41
29. Terahara K, Yoshida M, Igarashi O, Nochi T, Pontes GS, Hase K, et al. Comprehensive gene expression profiling of Peyer's patch M cells, villous M-like cells, and intestinal epithelial cells. J Immunol. (2008) 180:7840–6. doi: 10.4049/jimmunol.180.12.7840
30. Verbrugghe P, Waelput W, Dieriks B, Waeytens A, Vandesompele J, Cuvelier CA. Murine M cells express annexin V specifically. J Pathol. (2006) 209:240–9. doi: 10.1002/path.1970
31. Nochi T, Yuki Y, Matsumura A, Mejima M, Terahara K, Kim DY, et al. A novel M cell-specific carbohydrate-targeted mucosal vaccine effectively induces antigen-specific immune responses. J Exp Med. (2007) 204:2789–96. doi: 10.1084/jem.20070607
32. Nagura H, Ohtani H, Masuda T, Kimura M, Nakamura S. HLA-DR expression on M cells overlying Peyer's patches is a common feature of human small intestine. Acta Pathol Jpn. (1991) 41:818–23. doi: 10.1111/j.1440-1827.1991.tb01624.x
33. Allan CH, Mendrick DL, Trier JS. Rat intestinal M cells contain acidic endosomal-lysosomal compartments and express class II major histocompatibility complex determinants. Gastroenterology (1993) 104:698–708. doi: 10.1016/0016-5085(93)91004-2
34. Owen RL, Apple RT, Bhalla DK. Morphometric and cytochemical analysis of lysosomes in rat Peyer's patch follicle epithelium: their reduction in volume fraction and acid phosphatase content in M cells compared to adjacent enterocytes. Anat Rec. (1986) 216:521–7. doi: 10.1002/ar.1092160409
35. Koppang EO, Hordvik I, Bjerkås I, Torvund J, Aune L, Thevarajan J, et al. Production of rabbit antisera against recombinant MHC class II β chain and identification of immunoreactive cells in Atlantic salmon (Salmo salar). Fish Shellfish Immunol. (2003) 14:115–32. doi: 10.1006/fsim.2002.0424
36. Kambayashi T, Laufer TM. Atypical MHC class II-expressing antigen-presenting cells: can anything replace a dendritic cell? Nat Rev Immunol. (2014) 14:719–30. doi: 10.1038/nri3754
37. Debbabi H, Ghosh S, Kamath AB, Alt J, Demello DE, Dunsmore S, et al. Primary type II alveolar epithelial cells present microbial antigens to antigen-specific CD4+ T cells. Am J Physiol Lung Cell Mol Physiol. (2005) 289:L274–9. doi: 10.1152/ajplung.00004.2005
38. Black AP, Ardern-Jones MR, Kasprowicz V, Bowness P, Jones L, Bailey AS, et al. Human keratinocyte induction of rapid effector function in antigen-specific memory CD4+ and CD8+ T cells. Eur J Immunol. (2007) 37:1485–93. doi: 10.1002/eji.200636915
39. Mao P, Wu S, Li J, Fu W, He W, Liu X, et al. Human alveolar epithelial type II cells in primary culture. Physiol Rep. (2015) 3:e12288. doi: 10.14814/phy2.12288
40. Press CM, Evensen O, Reitan LJ, Landsverk T. Retention of furunculosis vaccine components in Atlantic salmon, Salmo salar L., following different routes of vaccine administration. J Fish Dis. (1996) 19:215–24. doi: 10.1111/j.1365-2761.1996.tb00128.x
41. Press CM, Evensen Ø. The morphology of the immune system in teleost fishes. Fish Shellfish Immunol. (1999) 9:309–18. doi: 10.1006/fsim.1998.0181
42. Vigliano FA, Bermúdez R, Quiroga MI, Nieto JM. Evidence for melano-macrophage centres of teleost as evolutionary precursors of germinal centres of higher vertebrates: an immunohistochemical study. Fish Shellfish Immunol. (2006) 21:467–71. doi: 10.1016/j.fsi.2005.12.012
43. Rességuier J, Delaune E, Coolen AL, Levraud JP, Boudinot P, Le Guellec D, et al. Specific and efficient uptake of surfactant-free poly (lactic acid) nanovaccine vehicles by mucosal dendritic cells in adult zebrafish after bath immersion. Front Immunol. (2017) 8:190. doi: 10.3389/fimmu.2017.00190
44. Palm RC, Landolt ML, Busch RA. Route of vaccine administration: effects on the specific humoral response in rainbow trout Oncorhynchus mykiss. Dis Aquat Org. (1998) 33:157–66. doi: 10.3354/dao033157
45. Lamichhane A, Azegami T, Kiyono H. The mucosal immune system for vaccine development. Vaccine (2014) 32:6711–23. doi: 10.1016/j.vaccine.2014.08.089
46. Xu Z, Takizawa F, Parra D, Gómez D, von Gersdorff Jørgensen L, LaPatra SE, et al. Mucosal immunoglobulins at respiratory surfaces mark an ancient association that predates the emergence of tetrapods. Nat Commun. (2017) 7:10728. doi: 10.1038/ncomms10728
Keywords: Oncorhynchus mykiss, comparative immunology, mucosal vaccination, atypical antigen presenting cell, lower vertebrate
Citation: Kato G, Miyazawa H, Nakayama Y, Ikari Y, Kondo H, Yamaguchi T, Sano M and Fischer U (2018) A Novel Antigen-Sampling Cell in the Teleost Gill Epithelium With the Potential for Direct Antigen Presentation in Mucosal Tissue. Front. Immunol. 9:2116. doi: 10.3389/fimmu.2018.02116
Received: 06 June 2018; Accepted: 28 August 2018;
Published: 20 September 2018.
Edited by:
Hetron Mweemba Munang'andu, Norwegian University of Life Sciences, NorwayReviewed by:
Chiu-Ming Wen, National University of Kaohsiung, TaiwanHeidrun Wergeland, University of Bergen, Norway
Copyright © 2018 Kato, Miyazawa, Nakayama, Ikari, Kondo, Yamaguchi, Sano and Fischer. This is an open-access article distributed under the terms of the Creative Commons Attribution License (CC BY). The use, distribution or reproduction in other forums is permitted, provided the original author(s) and the copyright owner(s) are credited and that the original publication in this journal is cited, in accordance with accepted academic practice. No use, distribution or reproduction is permitted which does not comply with these terms.
*Correspondence: Goshi Kato, gkato00@kaiyodai.ac.jp