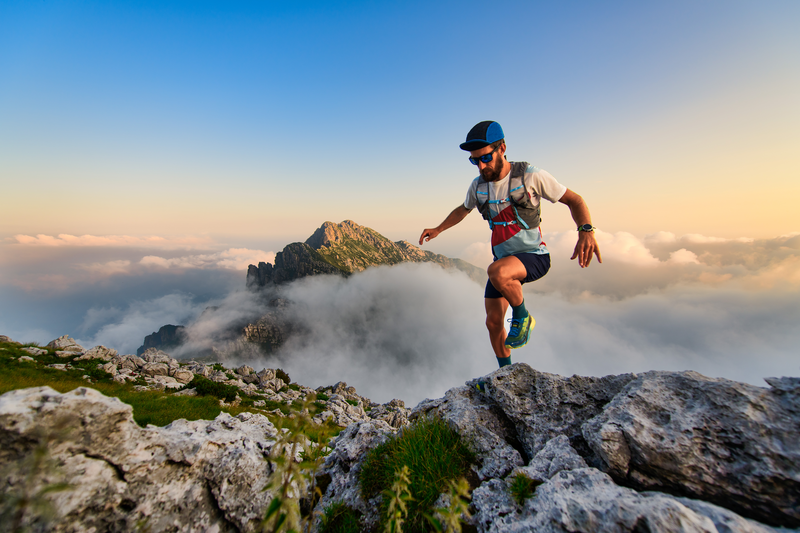
94% of researchers rate our articles as excellent or good
Learn more about the work of our research integrity team to safeguard the quality of each article we publish.
Find out more
REVIEW article
Front. Immunol. , 19 September 2018
Sec. Primary Immunodeficiencies
Volume 9 - 2018 | https://doi.org/10.3389/fimmu.2018.02105
This article is part of the Research Topic CARMA Proteins: Playing a Hand of Four CARDs View all 15 articles
CARD11 functions as a key signaling scaffold that controls antigen-induced lymphocyte activation during the adaptive immune response. Somatic mutations in CARD11 are frequently found in Non-Hodgkin lymphoma, and at least three classes of germline CARD11 mutations have been described as the basis for primary immunodeficiency. In this review, we summarize our current understanding of how CARD11 signals, how its activity is regulated, and how mutations bypass normal regulation to cause disease.
Our understanding of the immune system has benefited greatly from the study of the molecular circuitry in lymphocytes that governs antigen recognition and the cellular and systemic response to pathogens. This circuitry is elaborate, highly regulated, sensitive, and specific. As the study of immune cell signal transduction has highlighted networks of molecules that translate antigen sensing into lymphocyte action, it has also revealed how the dysregulation of signaling machinery can precipitate disease, including immunodeficiencies, autoimmunity syndromes, leukemia, and lymphoma.
CARD11 is a fascinating, multi-domain scaffold protein that plays a key role as a signaling hub during the adaptive immune response. Underscoring its importance, the CARD11 gene is extremely intolerant to loss-of-function (LOF) mutation or genetic variation in the human population (1, 2). Since its discovery in 2001 (3), many studies have revealed its obligate role in antigen-mediated lymphocyte activation and its susceptibility to mutations that can cause immunodeficiency or contribute to the development of lymphoma. CARD11 is best understood at present as a signal integrator that translates B cell receptor (BCR) and T cell receptor (TCR) triggering into the activation of NF-κB, JNK, and mTOR (Figure 1). Several excellent reviews have summarized the biological roles of CARD11 gleaned from mice and humans deficient in CARD11 or its signaling partner proteins (4–8). Here we will review our current understanding of (1) how the structure of CARD11 allows it to function as a signal-responsive scaffold; (2) how CARD11 orchestrates downstream signaling events; (3) the mechanisms that limit or terminate CARD11 signaling activity; (4) how gain-of-function (GOF) disease-associated CARD11 mutations bypass normal regulation; and (5) how LOF disease-associated CARD11 mutations disrupt CARD11 activity.
CARD11 can be thought of as a string of protein-protein interaction domains, each of which presents surfaces for the binding and regulation of interaction targets (Figure 2A). The protein interacts with more than 20 different proteins during signaling, and it has evolved to present its interaction surfaces dynamically in response to signaling inputs (Figure 2B). Prior to receptor engagement the protein exists in a closed, inactive state. Receptor engagement leads to the conversion of CARD11 to an open, active scaffold that binds signaling partners. Cofactor binding leads to the generation of downstream signaling intermediates that activate the IKK complex, a central target in the canonical NF-κB signaling pathway, as well as JNK2, and mTOR. Following activation, binding partners dissociate from CARD11 and the protein returns to the inactive state. This cycle, from closed to open and back to closed states, is thought to occur over the course of approximately 60 minutes following BCR or TCR engagement.
Figure 2. Activation of CARD11 activity during antigen receptor signaling. (A) Domain structure of CARD11. (B) Transition of CARD11 from closed, inactive to open, active states and back again. Dashed lines indicate mapped protein-protein interactions. Abbreviations: CARD, Caspase Recruitment Domain; L, Latch; CC, coiled-coil; ID, Inhibitory Domain; PDZ, PSD-95/Discs-Large/ZO-1 domain; SH3, Src Homology 3 domain; GUK, Guanylate Kinase domain; RE1, Repressive Element 1; RE2, Repressive Element 2; RE3, Repressive Element 3; RE4, Repressive Element 4; L3, Linker 3; L4, Linker 4.
Like many signaling proteins that promote cellular proliferation and activation and whose signaling could be dangerous if dysregulated, CARD11 contains an internal autoinhibitory domain that keeps CARD11 inactive in the absence of receptor triggering (9–11). This domain, termed the Inhibitory Domain (ID), is located in the primary sequence between the Coiled-coil and PDZ domain (Figure 2A). Remarkably, the autoinhibitory action of the ID is accomplished by four small Repressive Elements (REs), ranging in size from 11 to 53 amino acids, that function cooperatively with redundancy (12, 13). Other than a short 5-residue region of homology between RE2 and RE3, the four REs do not resemble each other. While the mutation of any single RE has little to no effect on basal CARD11 signaling, their combinatorial mutation cooperatively increases activity, and the simultaneous mutation of all four leads to a remarkable 640-fold enhancement of activity (12). The four REs function together to prevent cofactor binding to CARD11 prior to receptor engagement, but it is not completely clear how they do so. RE1, RE2, and RE3 interact with other CARD11 domains, including the CARD, LATCH, Coiled-coil, L3, and GUK, with somewhat overlapping intramolecular specificities, presumably to maintain CARD11 in a conformation in which protein-interaction surfaces of the CARD and Coiled-coil are inaccessible (13). However, RE4, which may be the most potent inhibitory element, does not appear to participate in intramolecular interactions and likely functions in cooperation with the other REs through an unknown mechanism, possibly via the recruitment of a repressor in trans.
Antigen receptor engagement by antigen in the appropriate context leads to a cascade of signaling events upstream of CARD11 that have been extensively reviewed (14). At present, it appears that the inputs that CARD11 receives from upstream signaling consist of phosphorylation events within the ID (9, 10, 15–17). Serines 564, 567, 577, and 657 all appear to be phosphorylated as a result of antigen receptor signaling. Somehow the modification of these residues leads to sufficient neutralization of RE activity to allow CARD11 signaling cofactors to bind and signaling events to ensue. Serines 564 and 657 appear to be targeted by PKCβ in B cells and PKCθ in T cells, while serine 567 appears to be phosphorylated by IKKβ. The kinase that targets serine 577 has not yet been reported. Additional phosphorylation events may also be important for maximal CARD11 activity, depending on cellular context (18–20). The phosphorylation-mediated activation of CARD11 scaffold activity may occur in a step-wise manner, with an initial step that elicits some IKKβ kinase activity and a subsequent step in which IKKβ phosphorylation of serine 577 promotes full scaffold activity (15, 21). Precisely how the phosphorylation events convert CARD11 into an active scaffold remains mysterious. It is possible that phosphorylation induces a conformational change in CARD11 that disallows RE-mediated inhibitory intramolecular interactions. Alternatively, the phosphorylated residues may be recognized by an unknown factor in trans that actively prevents cooperative RE action.
Upon activation, the protein interaction surfaces of CARD11 become accessible for binding to a variety of proteins (Figure 2B). Bcl10 was the first protein shown to bind CARD11, with MALT1 recruited indirectly through Bcl10. These observations led to the notion of a “CBM complex,” which is a misleading misnomer because it ignores the binding of other critical factors to CARD11. Bcl10 requires both the CARD and Coiled-coil domain of CARD11 for its recruitment at physiological levels of expression (11). The CARD and Coiled-coil are also required for the recruitment of TRAF6, IKKγ, and Caspase-8 (11). The CARD alone is required for TAK1 binding (11), while the Coiled-coil alone is required for binding to HOIP, the catalytic subunit of the Linear Ubiquitin Chain Assembly Complex (LUBAC) (22). CK1α is recruited through the Coiled-coil and ID (23). The preponderance of protein interactions occurs through the N-terminal half of CARD11 that includes the CARD, LATCH, and Coiled-coil, although the C-terminal PDZ-SH3-MAGUK region can bind the ADAP adapter (24) and AIP (25). In T cells, the site of CARD11 complex assembly appears to be the immunological synapse (26, 27).
In addition to the intramolecular interactions mediated by the REs in the ID, two other CARD11 regions have been shown to mediate CARD11-CARD11 interactions. The Coiled-coil domain, predicted to form four discontinuous regions with coiled-coil character, mediates assembly of CARD11 into an oligomer of undetermined stoichiometry. The SH3-GUK domain tandem has also been shown to participate in modular inter- and intramolecular binding interactions that appear to be required for higher order clustering of CARD11 visible via microscopy (28).
The transient recruitment of cofactors to CARD11 ultimately leads to the activation of the IKK complex in the canonical NF-κB activation pathway. As it assembles cofactors into complexes, CARD11 orchestrates ubiquitinylation and phosphorylation events that somehow work together to promote IKK kinase action on inhibitory IκB proteins that tether NF-κB in the cytoplasm. The phosphorylation of IκBs promotes their ubiquitinylation and degradation by the proteasome, which allows NF-κB to stably translocate to the nucleus to bind target genes.
The signal-induced recruitment of Bcl10 and HOIP to CARD11 allows HOIP (enzyme) to conjugate Bcl10 (substrate) with linear ubiquitin chains, to produce LinUbn-Bcl10 (22). LinUbn-Bcl10 then binds the IKK complex through the UBAN domain of IKKγ (22) in an interaction thought to be required for IKK complex kinase activation (29). LinUbn-Bcl10 is a signaling intermediate that determines the extent of NF-κB activation downstream of CARD11 triggering. For a range of hyperactive CARD11 variants (see below), the levels of LinUbn-Bcl10 produced by each variant correlates with the degree of NF-κB activation it achieves (22). Signaling through LinUbn-Bcl10 accounts for 50-60% of the signaling output of CARD11 to NF-κB. In addition to CARD11, TCR-induced LinUbn-Bcl10 generation also requires MALT1, but not the SHARPIN subunit of LUBAC (22).
Bcl10 is also conjugated with K63-linked ubiquitin chains during antigen receptor signaling (30, 31) to form Ubn(K63)-Bcl10. This modification, mediated by cIAPs, has been shown to be a prerequisite for linear ubiquitinylation of Bcl10 in the context of chronic BCR signaling (32).
CARD11 facilitates the polyubiquitinylation of IKKγ with K63-linked chains in response to antigen receptor triggering (33), which is accomplished by a MALT1-associated E3 ligase activity (34). Linear ubiquitinylation of IKKγ has also been implicated in the antigen receptor pathway (35, 36). Since CARD11 recruits both HOIP and IKKγ upon activation, CARD11 likely facilitates LUBAC action on IKKγ.
MALT1 is also conjugated with K63-linked ubiquitin chains during signaling, which facilitates its interaction with IKKγ (37); however, MALT1 polyubiquitinylation has not been formally shown to require CARD11. TRAF6 has been implicated as the E3 ligase for this process, and its recruitment by CARD11 may promote its action on MALT1.
CARD11 is also required for MALT1 protease activity on several targets, including Bcl10 and MALT1 itself (7, 38–40). MALT1 cleaves the inhibitory deubiquitinase A20 (41, 42) to limit its removal of polyubiquitinylated MALT1, thereby extending the time-course of NF-κB activation. MALT1 also cleaves the NF-κB subunit RelB, limiting its potential to repress transcriptional activation by RelA and c-Rel (43). In addition, MALT1 cleaves the HOIL-1L subunit of LUBAC and in so doing limits the degree of NF-κB activation downstream of CARD11 (36, 44, 45). MALT1 has also been shown to cleave the deubiquitinase CYLD to maximize NF-κB and JNK activation (46–48). CARD11 may promote MALT1 protease activity by activating enzymatic potential, by recruiting enzyme to substrate, or both, but further studies are required to define mechanisms.
The activation of mTOR downstream of TCR engagement also requires CARD11, in a role independent of IKK complex activation (49, 50). CARD11 signaling to mTORC1 depends upon the proteolytic activity of MALT1 (49) and the rapid uptake of glutamine through the ASCT2 glutamine transporter (50). CARD11 signaling promotes ASCT2 expression and ASCT2 associates with CARD11, Bcl10, and MALT1 during signaling, suggesting an active regulation of transporter activity (50).
CARD11 is also required for the activation of JNK signaling following antigen receptor triggering (51, 52), in a manner that also requires Bcl10 and MALT1. For JNK activation, CARD11 appears to promote Bcl10 oligomerization, followed by the binding of Bcl10 to TAK1, MKK7, and JNK2, which is thought to engage this MAP kinase cascade for JNK2 activation leading to increased levels of c-Jun and c-Jun phosphorylation (53, 54).
CARD11 fragments that include the CARD, LATCH and portions of the Coiled-coil domain have been shown in vitro to nucleate the formation of helical filaments of Bcl10 (55, 56). These Bcl10 filaments assemble through interactions between Bcl10 CARD domains and polymerize in a unidirectional manner (56). MALT1 and TRAF6 cooperatively associate with the Bcl10 filament (56) and stimulate MALT1 proteolytic activity (55). Bcl10 mutations that affect filament formation perturb NF-κB activation by overexpressed Bcl10 in cells (55), implicating these structures in CARD11 signaling. However, further work is needed to bolster the importance of Bcl10 filaments in antigen receptor signaling through CARD11 in vivo at physiological levels of expression, resolve how Bcl10 and MALT1 ubiquitinylation is accommodated or promoted by Bcl10 filament formation, and explain the mechanistic relationship between Bcl10 filament formation and other steps in CARD11 signaling that require the L3, SH3, L4, and GUK domains, which are required for physiological CARD11 signaling but are not required to nucleate Bcl10 filaments.
Multiple mechanisms have evolved to tune and terminate CARD11 signaling to ensure appropriate pathway output and avoid pathological immune cell activation and proliferation. First, cofactor association to CARD11 is regulated. The E3 ligase RNF181 limits the steady-state level of Bcl10 through K48-linked ubiquitinylation and degradation, thereby limiting the amount of Bcl10 that can bind CARD11 (57). Once signaling initiates, the kinesin GAKIN competes with Bcl10 for binding to CARD11 and limits the dwell time of CARD11 at the immune synapse (58). The phosphatase PP2A removes an activating phosphate from CARD11, which limits cofactor binding to CARD11 (59). Once CK1α is recruited to CARD11 in a step required for signaling, it phosphorylates CARD11 to inhibit the extent of CARD11 signaling (23).
Second, cofactors that associate with CARD11 in a signal-inducible manner rapidly dissociate from CARD11 during the initial ~60 minutes after receptor triggering. The mechanisms of complex disassembly remain poorly defined, but disassembly limits the generation of CARD11-promoted signaling intermediates. In some contexts, cofactors disassemble from CARD11 in an obligate step in productive signaling, such as in the assembly of complexes containing p62, Bcl10, MALT1, and the IKK complex that can prolong IKK activation (60, 61).
Third, key polyubiquitinylated signaling intermediates, including LinUbn-Bcl10, Ubn(K63)-Bcl10, and Ubn(K63)-MALT1 rapidly disappear as the result of the action of A20 (42), CYLD (46, 47), and likely other deubiquitinases. The removal of these potent intermediates attenuates the extent and duration of IKK complex activation. Fourth, CARD11 and Bcl10 are themselves degraded to limit signaling, and perhaps make cells refractory to immediate re-initiation of the CARD11-dependent pathway (15, 62–66).
The dysregulated, constitutive signaling to NF-κB observed in several types of leukemia and lymphoma endows the transformed cells with a proliferative and survival advantage through the induction of pro-proliferative and anti-apoptotic NF-κB gene targets (40 67–69). Leukemias and lymphomas exploit a variety of strategies of genomic alteration to achieve constitutive NF-κB activity. The regulation of CARD11 activity by an internal autoinhibitory domain makes CARD11 highly susceptible to mutations that can cause GOF signaling independent of upstream antigen receptor engagement. GOF CARD11 mutations occur in approximately 10% of cases of the Activated B Cell-Like (ABC) subtype of Diffuse Large B Cell Lymphoma (DLBCL) (70), but they have also been observed in other DLBCL subtypes (71–76), as well as in Acute T-cell Leukemia/Lymphoma (77), Sézary syndrome (78, 79) Mantle Cell Lymphoma (80), and Angioimmunoblastic T-cell lymphoma (81). In many cases, however, the signaling potency of CARD11 alleles found in patient biopsies has not been thoroughly characterized, or confirmed to be required for aberrant proliferation, as has been done for many CARD11 mutations found in ABC DLBCL. Figure 3 depicts lymphoma-associated mutations that have been directly shown to potently increase CARD11 signaling.
Figure 3. Lymphoma-associated CARD11 mutations. Mutations are depicted that have been validated to have at least a 3-fold increase in CARD11 signaling in a quantitative signaling assay. ABC, Activated B Cell-like; DLBCL, Diffuse Large B Cell Lymphoma; GCB, Germinal Center B cell; MCL, Mantle Cell Lymphoma; PCNSL, Primary Central Nervous System Lymphoma; ATLL, Acute T cell Leukemia/Lymphoma; TFH, Follicular T helper; PTCL, Peripheral T cell Lymphoma.
GOF CARD11 mutations cause constitutive hyperactive CARD11 signaling by bypassing the action of the four REs in the ID that normally keep CARD11 basally inactive (13, 22, 31, 82) (Figure 4). Mutations in the CARD, LATCH, and Coiled-coil of CARD11 disrupt the function of multiple REs to allow partial conversion of CARD11 to an open, active state that can recruit Bcl10 (31, 82) and HOIP (22), but not other factors recruited during normal antigen receptor signaling, including TAK1, TRAF6, IKKγ, and Caspase-8 (31, 82), at least for the mutants that have been characterized. The spontaneous recruitment of Bcl10 and HOIP to GOF CARD11 variants leads to the spontaneous generation of LinUbn-Bcl10, the levels of which appear to determine the quantitative output of NF-κB activation (22). Potent GOF mutations in the CARD, LATCH, and Coiled-coil can enhance basal CARD11 signaling by 80- to 160-fold (31, 82), and they appear to do so in part by interfering with inhibitory intramolecular interactions mediated by multiple REs (13). Potent GOF point mutations do not occur in the ID itself, due to the redundant action of the four REs; three or more REs would have to be disabled to achieve a comparable level of dysregulated signaling (12). For ABC DLBCL cells, the quantitative degree to which a GOF CARD11 allele activates NF-κB largely correlates with its ability to support aberrant cell proliferation (31, 70). However, the dysregulation of B cell proliferation in vivo by a CARD11 GOF allele requires both NF-κB and JNK activation (83). CARD11 GOF mutations in lymphoma occur in the presence of many other genomic alterations. While overexpression of an extremely potent CARD11 GOF allele is sufficient to cause lethal B cell proliferation (83), in human lymphomas it is likely that multiple genomic lesions cooperate with a GOF CARD11 mutation to maintain the proliferation and survival of the transformed cells.
Figure 4. Oncogenic CARD11 signaling. Lymphoma-associated gain-of-function mutations in the CARD, LATCH, and Coiled-coil are represented by asterisks.
One of the most exciting recent developments in the study of CARD11 has been the recognition of germline CARD11 mutations in primary immunodeficiency. Three different forms of primary immunodeficiency (PID) have been described so far that result from germline mutations in the CARD11 gene, (1) CARD11 deficiency, (2) BENTA disease, and (3) Immunodeficiency with atopy.
CARD11 deficiency caused by homozygous LOF mutations in CARD11 was first reported in two studies in 2013 (84, 85). These patients, who presented with severe Pneumocystis jirovecii infections as infants, displayed normal T and B cell counts but hypogammaglobulinemia, deficits in mature or differentiated B and T cells (CD4 and CD8), reduced Treg numbers, and defective B and T cell activation in vitro. The homozygous mutations found in these patients include a deletion of exon 21 that results in a lack of detectable CARD11 expression (85) and a premature stop codon at glutamine 945 in the GUK domain (84) (Figure 5). Notably, family members that are heterozygous for these alleles do not present with immunodeficiency.
BENTA disease (B cell Expansion with NF-κB and T cell Anergy), caused by heterozygous GOF CARD11 mutations, has been described in 16 patients so far beginning in 2012 (86–90). BENTA patients experience recurrent ear, sinopulmonary, and viral infections (molluscum contagiosum, BK virus, Epstein-Barr virus), and exhibit a profound expansion in the number of B cells, a skewing of B cells toward transitional states, an unresponsiveness of T cells to antigen, and a poor antibody response to pneumococcal and meningococcal capsular polysaccharides. BENTA-associated mutations are located in the CARD (C49Y), LATCH (G116S, G123S, G123D, E127H), and Coiled-coil (E134G, H234L+Δ235-8) domains (Figure 5). Some (C49Y, G123S, G123D) are identical to those found in DLBCL. The alleles induce constitutive NF-κB activation in lymphocytes, presumably through the same signaling intermediates discussed above, but it remains unclear precisely how their constitutive signaling results in disparate effects in different immune cell subtypes.
Immunodeficiency with atopy, caused by heterozygous, LOF CARD11 mutations that appear to act as strong dominant negative alleles, was reported in 2017 by two studies (91, 92). In the five families described so far, affected individuals experience severe atopic dermatitis, recurrent pneumonia, and other upper respiratory tract infections, asthma, and food allergies with varying severities. While patient B cells exhibit mild defects in antigen-induced activation, patient T cells display reduced activation and proliferation in vitro, consistent with a poor T cell response to prior antigen exposure. Patients also display elevated serum IgE levels but low-to-normal levels of other Ig classes. CARD11 mutations that cause Immunodeficiency with atopy have been found in the CARD (R30W, E57D), Coiled-coil (L194P, dupM183-K196), and GUK (R975W) (Figure 5). E57D and L194P have been shown to interfere with recruitment of Bcl10 and MALT1 to CARD11 following TCR signaling (91), while R30W appears to have a milder effect on recruitment of these cofactors to CARD11 (92). This class of dominant negative mutants has also been shown to disrupt CARD11 signaling to mTOR and to the activation of MALT1 protease activity (91).
A thorough understanding of how CARD11 is kept inactive prior to signaling, and how CARD11 converts to an open, active scaffold will require determination of the three-dimensional structure of CARD11 in “closed” and “open” states. Structural studies so far have solved the structure of the CARD domain of CARD11 and have modeled how the CARD11 CARD can nucleate the formation of Bcl10 filaments (55, 56, 93, 94). However, the structure of 90% of the protein is unknown. Structural information will be invaluable for understanding how the multiple domains in CARD11 signal to its targets and how GOF and LOF mutations induce or disrupt CARD11 activity.
It remains unclear precisely how CARD11 signaling results in the activation of IKK kinase activity. The generation of ubiquitinylated Bcl10, MALT1, and IKKγ species is thought to induce a network of interacting proteins, in which ubiquitin chains are recognized by specific domains within signaling cofactors. This web of intermolecular binding can induce the proximity and clustering of IKK complexes, but how kinase activity is induced under physiological conditions, and whether other requisite components have yet to be discovered are unclear. It should also be explored whether CARD11 simply recruits enzymes (E3 ligases, kinases, protease) to their substrates or whether CARD11 binding plays a more active role in allosteric regulation of catalytic activity or substrate competency.
Although it is clear that the four REs within the ID function cooperatively to keep CARD11 inactive prior to signaling, it remains unclear why the protein has evolved this unique array of redundant repressive elements. RE redundancy does prevent unwanted GOF mutations from occurring in the ID, but it does not prevent their occurrence in the CARD, LATCH, and Coiled-coil. It is possible that the REs determine the kinetics of CARD11 “activation” during signaling, or the kinetics by which CARD11 returns to the basal inactive state following signaling, but further studies are needed to test these hypotheses.
The discovery of patients with germline CARD11 LOF and GOF mutations provides exciting opportunities for obtaining insight into the molecular mechanisms of CARD11 signaling and the cellular interplay of immune cell subtypes affected by CARD11 dysfunction. It remains unclear why some CARD11 LOF mutations are dominant negative and manifest disease when heterozygous, while other CARD11 LOF mutations manifest disease only when homozygous. In addition, it is not firmly established whether all disease-associated CARD11 alleles affect signaling to mTOR and JNK, or which dysregulated pathways downstream of CARD11 are responsible for which disease manifestations. Also unknown is whether modifier genes in the patients studied have influenced their presentation, since only a small number of patients have been identified so far. It will be interesting to see whether additional CARD11 alleles will be discovered in the human population, leading to variable phenotypes of immunodeficiency and atopy.
Several studies have implicated a role for CARD11 in pathways distinct from antigen receptors, including those emanating from activating NK cell receptors (95–97), OX40 (98), and the IL-2 receptor (99). The mechanistic role of CARD11 in these pathways deserves further study. It is possible that additional signaling pathways will be identified that rely on CARD11 activity, and if dysregulated, may contribute to human disease.
All authors listed have made substantial, direct, and intellectual contribution to the work and approved it for publication.
This work was supported by RO1CA177600 from the National Institutes of Health.
The authors declare that the research was conducted in the absence of any commercial or financial relationships that could be construed as a potential conflict of interest.
1. Petrovski S, Wang Q, Heinzen EL, Allen AS, Goldstein DB. Genic intolerance to functional variation and the interpretation of personal genomes. PLoS Genet. (2013) 9:e1003709. doi: 10.1371/annotation/32c8d343-9e1d-46c6-bfd4-b0cd3fb7a97e
2. Lek M, Karczewski KJ, Minikel EV, Samocha KE, Banks E, Fennell T, et al. Analysis of protein-coding genetic variation in 60,706 humans. Nature (2016) 536:285–91. doi: 10.1038/nature19057
3. Bertin J, Wang L, Guo Y, Jacobson MD, Poyet JL, Srinivasula SM, et al. CARD11 and CARD14 are novel caspase recruitment domain (CARD)/membrane-associated guanylate kinase (MAGUK) family members that interact with BCL10 and activate NF-kappa B. J Biol Chem. (2001) 276:11877–82. doi: 10.1074/jbc.M010512200
4. Turvey SE, Durandy A, Fischer A, Fung SY, Geha RS, Gewies A, et al. The CARD11-BCL10-MALT1 (CBM) signalosome complex: stepping into the limelight of human primary immunodeficiency. J Allergy Clin Immunol. (2014) 134:276–84. doi: 10.1016/j.jaci.2014.06.015
5. Perez De Diego R, Sanchez-Ramon S, Lopez-Collazo E, Martinez-Barricarte R, Cubillos-Zapata C, Ferreira Cerdan A, et al. Genetic errors of the human caspase recruitment domain-B-cell lymphoma 10-mucosa-associated lymphoid tissue lymphoma-translocation gene 1 (CBM) complex: molecular, immunologic, and clinical heterogeneity. J Allergy Clin Immunol. (2015) 136:1139–49. doi: 10.1016/j.jaci.2015.06.031
6. Shi JH, Sun SC. TCR signaling to NF-κB and mTORC1: expanding roles of the CARMA1 complex. Mol Immunol. (2015) 68:546–57. doi: 10.1016/j.molimm.2015.07.024
7. Meininger I, Krappmann D. Lymphocyte signaling and activation by the CARMA1-BCL10-MALT1 signalosome. Biol Chem. (2016) 397:1315–33. doi: 10.1515/hsz-2016-0216
8. Zhang Q, Lenardo MJ, Baltimore D. 30 Years of NF-κB: a blossoming of relevance to human pathobiology. Cell (2017) 168:37–57. doi: 10.1016/j.cell.2016.12.012
9. Matsumoto R, Wang D, Blonska M, Li H, Kobayashi M, Pappu B, et al. Phosphorylation of CARMA1 plays a critical role in T cell receptor-mediated NF-κB Activation. Immunity (2005) 23:575–85. doi: 10.1016/j.immuni.2005.10.007
10. Sommer K, Guo B, Pomerantz JL, Bandaranayake AD, Moreno-Garcia ME, Ovechkina YL, et al. Phosphorylation of the CARMA1 linker controls NF-κB activation. Immunity (2005) 23:561–74. doi: 10.1016/j.immuni.2005.09.014
11. Mccully RR, Pomerantz JL. The protein kinase C-responsive inhibitory domain of CARD11 functions in NF-κB activation to regulate the association of multiple signaling cofactors that differentially depend on Bcl10 and MALT1 for association. Mol Cell Biol. (2008) 28:5668–86. doi: 10.1128/MCB.00418-08
12. Jattani RP, Tritapoe JM, Pomerantz JL. Cooperative control of Caspase Recruitment Domain-containing protein 11 (CARD11) signaling by an unusual array of redundant repressive elements. J Biol Chem. (2016) 291:8324–36. doi: 10.1074/jbc.M115.683714
13. Jattani RP, Tritapoe JM, Pomerantz JL. Intramolecular interactions and regulation of cofactor binding by the four repressive elements in the Caspase Recruitment Domain-containing protein 11 (CARD11) Inhibitory domain. J Biol Chem. (2016) 291:8338–48. doi: 10.1074/jbc.M116.717322
14. Paul S, Schaefer BC. A new look at T cell receptor signaling to nuclear factor-kappaB. Trends Immunol. (2013) 34:269–81. doi: 10.1016/j.it.2013.02.002
15. Wegener E, Oeckinghaus A, Papadopoulou N, Lavitas L, Schmidt-Supprian M, Ferch U, et al. Essential role for IkappaB kinase beta in remodeling Carma1-Bcl10-Malt1 complexes upon T cell activation. Mol Cell (2006) 23:13–23. doi: 10.1016/j.molcel.2006.05.027
16. Shinohara H, Maeda S, Watarai H, Kurosaki T. IkappaB kinase beta-induced phosphorylation of CARMA1 contributes to CARMA1 Bcl10 MALT1 complex formation in B cells. J Exp Med. (2007) 204:3285–93. doi: 10.1084/jem.20070379
17. Thome M, Charton JE, Pelzer C, Hailfinger S. Antigen receptor signaling to NF-{kappa}B via CARMA1, BCL10, and MALT1. Cold Spring Harb Perspect Biol. (2010) 2:a003004. doi: 10.1101/cshperspect.a003004
18. Ishiguro K, Green T, Rapley J, Wachtel H, Giallourakis C, Landry A, et al. Ca2+/calmodulin-dependent protein kinase II is a modulator of CARMA1-mediated NF-κB activation. Mol Cell Biol. (2006) 26:5497–508. doi: 10.1128/MCB.02469-05
19. Brenner D, Brechmann M, Rohling S, Tapernoux M, Mock T, Winter D, et al. Phosphorylation of CARMA1 by HPK1 is critical for NF-κB activation in T cells. Proc Natl Acad Sci USA. (2009) 106:14508–13. doi: 10.1073/pnas.0900457106
20. Cheng J, Hamilton KS, Kane LP. Phosphorylation of Carma1, but not Bcl10, by Akt regulates TCR/CD28-mediated NF-κB induction and cytokine production. Mol Immunol. (2014) 59:110–6. doi: 10.1016/j.molimm.2014.01.011
21. Shinohara H, Behar M, Inoue K, Hiroshima M, Yasuda T, Nagashima T, et al. Positive feedback within a kinase signaling complex functions as a switch mechanism for NF-κB activation. Science (2014) 344:760–4. doi: 10.1126/science.1250020
22. Yang YK, Yang C, Chan W, Wang Z, Deibel KE, Pomerantz JL. Molecular determinants of scaffold-induced linear ubiquitinylation of B cell lymphoma/Leukemia 10 (Bcl10) during T cell receptor and oncogenic Caspase Recruitment Domain-containing protein 11 (CARD11) signaling. J Biol Chem. (2016b) 291:25921–36. doi: 10.1074/jbc.M116.754028
23. Bidere N, Ngo VN, Lee J, Collins C, Zheng L, Wan F, et al. Casein kinase 1alpha governs antigen-receptor-induced NF-κB activation and human lymphoma cell survival. Nature (2009) 458:92–6. doi: 10.1038/nature07613
24. Medeiros RB, Burbach BJ, Mueller KL, Srivastava R, Moon JJ, Highfill S, et al. Regulation of NF-κB activation in T cells via association of the adapter proteins ADAP and CARMA1. Science (2007) 316:754–8. doi: 10.1126/science.1137895
25. Schimmack G, Eitelhuber AC, Vincendeau M, Demski K, Shinohara H, Kurosaki T, et al. AIP augments CARMA1-BCL10-MALT1 complex formation to facilitate NF-κB signaling upon T cell activation. Cell Commun Signal. (2014) 12:49. doi: 10.1186/s12964-014-0049-7
26. Hara H, Bakal C, Wada T, Bouchard D, Rottapel R, Saito T, et al. The molecular adapter Carma1 controls entry of IkappaB kinase into the central immune synapse. J Exp Med. (2004) 200:1167–77. doi: 10.1084/jem.20032246
27. Wang D, Matsumoto R, You Y, Che T, Lin XY, Gaffen SL, et al. CD3/CD28 costimulation-induced NF-κB activation is mediated by recruitment of protein kinase C-theta, Bcl10, and IkappaB kinase beta to the immunological synapse through CARMA1. Mol Cell Biol. (2004) 24:164–71. doi: 10.1128/MCB.24.1.164-171.2003
28. Hara H, Yokosuka T, Hirakawa H, Ishihara C, Yasukawa S, Yamazaki M, et al. Clustering of CARMA1 through SH3-GUK domain interactions is required for its activation of NF-κB signalling. Nat Commun. (2015) 6:5555. doi: 10.1038/ncomms6555
29. Hadian K, Griesbach RA, Dornauer S, Wanger TM, Nagel D, Metlitzky M, et al. NF-κB essential modulator (NEMO) interaction with linear and lys-63 ubiquitin chains contributes to NF-κB activation. J Biol Chem. (2011) 286:26107–17. doi: 10.1074/jbc.M111.233163
30. Wu CJ, Ashwell JD. NEMO recognition of ubiquitinated Bcl10 is required for T cell receptor-mediated NF-κB activation. Proc Natl Acad Sci U.S.A. (2008) 105:3023–8. doi: 10.1073/pnas.0712313105
31. Chan W, Schaffer TB, Pomerantz JL. A quantitative signaling screen identifies CARD11 mutations in the CARD and LATCH domains that induce Bcl10 ubiquitination and human lymphoma cell survival. Mol Cell Biol. (2013) 33:429–43. doi: 10.1128/MCB.00850-12
32. Yang Y, Kelly P, Shaffer AL III, Schmitz R, Yoo HM, Liu X, et al. Targeting non-proteolytic protein ubiquitination for the treatment of diffuse large B cell lymphoma. Cancer Cell (2016) 29:494–507. doi: 10.1016/j.ccell.2016.03.006
33. Shambharkar PB, Blonska M, Pappu BP, Li H, You Y, Sakurai H, et al. Phosphorylation and ubiquitination of the IkappaB kinase complex by two distinct signaling pathways. Embo J. (2007) 26:1794–805. doi: 10.1038/sj.emboj.7601622
34. Zhou H, Wertz I, O'rourke K, Ultsch M, Seshagiri S, Eby M, et al. Bcl10 activates the NF-κB pathway through ubiquitination of NEMO. Nature (2004) 427:167–71. doi: 10.1038/nature02273
35. Tokunaga F, Sakata S, Saeki Y, Satomi Y, Kirisako T, Kamei K, et al. Involvement of linear polyubiquitylation of NEMO in NF-κB activation. Nat Cell Biol. (2009) 11:123–32. doi: 10.1038/ncb1821
36. Klein T, Fung SY, Renner F, Blank MA, Dufour A, Kang S, et al. The paracaspase MALT1 cleaves HOIL1 reducing linear ubiquitination by LUBAC to dampen lymphocyte NF-κB signalling. Nat Commun. (2015) 6:8777. doi: 10.1038/ncomms9777
37. Oeckinghaus A, Wegener E, Welteke V, Ferch U, Arslan SC, Ruland J, et al. Malt1 ubiquitination triggers NF-κB signaling upon T-cell activation. Embo J. (2007) 26:4634–45. doi: 10.1038/sj.emboj.7601897
38. Rebeaud F, Hailfinger S, Posevitz-Fejfar A, Tapernoux M, Moser R, Rueda D, et al. The proteolytic activity of the paracaspase MALT1 is key in T cell activation. Nat Immunol. (2008) 9:272–81. doi: 10.1038/ni1568
39. Baens M, Bonsignore L, Somers R, Vanderheydt C, Weeks SD, Gunnarsson J, et al. MALT1 auto-proteolysis is essential for NF-kappaB-dependent gene transcription in activated lymphocytes. PLoS ONE (2014) 9:e103774. doi: 10.1371/journal.pone.0103774
40. Juilland M, Thome M. Role of the CARMA1/BCL10/MALT1 complex in lymphoid malignancies. Curr Opin Hematol. (2016) 23:402–9. doi: 10.1097/MOH.0000000000000257
41. Coornaert B, Baens M, Heyninck K, Bekaert T, Haegman M, Staal J, et al. T cell antigen receptor stimulation induces MALT1 paracaspase-mediated cleavage of the NF-κB inhibitor A20. Nat Immunol. (2008) 9:263–71. doi: 10.1038/ni1561
42. Duwel M, Welteke V, Oeckinghaus A, Baens M, Kloo B, Ferch U, et al. A20 negatively regulates T cell receptor signaling to NF-κB by cleaving Malt1 ubiquitin chains. J Immunol. (2009) 182:7718–28. doi: 10.4049/jimmunol.0803313
43. Hailfinger S, Nogai H, Pelzer C, Jaworski M, Cabalzar K, Charton JE, et al. Malt1-dependent RelB cleavage promotes canonical NF-κB activation in lymphocytes and lymphoma cell lines. Proc Natl Acad Sci USA. (2011) 108:14596–601. doi: 10.1073/pnas.1105020108
44. Douanne T, Gavard J, Bidere N. The paracaspase MALT1 cleaves the LUBAC subunit HOIL1 during antigen receptor signaling. J Cell Sci. (2016) 129:1775–80. doi: 10.1242/jcs.185025
45. Elton L, Carpentier I, Staal J, Driege Y, Haegman M, Beyaert R. MALT1 cleaves the E3 ubiquitin ligase HOIL-1 in activated T cells, generating a dominant negative inhibitor of LUBAC-induced NF-κB signaling. FEBS J. (2016) 283:403–12. doi: 10.1111/febs.13597
46. Reiley WW, Jin W, Lee AJ, Wright A, Wu X, Tewalt EF, et al. Deubiquitinating enzyme CYLD negatively regulates the ubiquitin-dependent kinase Tak1 and prevents abnormal T cell responses. J Exp Med. (2007) 204:1475–85. doi: 10.1084/jem.20062694
47. Sun SC. CYLD: a tumor suppressor deubiquitinase regulating NF-κB activation and diverse biological processes. Cell Death Differ. (2010) 17:25–34. doi: 10.1038/cdd.2009.43
48. Staal J, Driege Y, Bekaert T, Demeyer A, Muyllaert D, Van Damme P, et al. T-cell receptor-induced JNK activation requires proteolytic inactivation of CYLD by MALT1. EMBO J. (2011) 30:1742–52. doi: 10.1038/emboj.2011.85
49. Hamilton KS, Phong B, Corey C, Cheng J, Gorentla B, Zhong X, et al. T cell receptor-dependent activation of mTOR signaling in T cells is mediated by Carma1 and MALT1, but not Bcl10. Sci Signal. (2014) 7:ra55. doi: 10.1126/scisignal.2005169
50. Nakaya M, Xiao Y, Zhou X, Chang JH, Chang M, Cheng X, et al. Inflammatory T cell responses rely on amino acid transporter ASCT2 facilitation of glutamine uptake and mTORC1 kinase activation. Immunity (2014) 40:692–705. doi: 10.1016/j.immuni.2014.04.007
51. Hara H, Wada T, Bakal C, Kozieradzki I, Suzuki S, Suzuki N, et al. The MAGUK family protein CARD11 is essential for lymphocyte activation. Immunity (2003) 18:763–75. doi: 10.1016/S1074-7613(03)00148-1
52. Jun JE, Wilson LE, Vinuesa CG, Lesage S, Blery M, Miosge LA, et al. Identifying the MAGUK protein Carma-1 as a central regulator of humoral immune responses and atopy by genome-wide mouse mutagenesis. Immunity (2003) 18:751–62. doi: 10.1016/S1074-7613(03)00141-9
53. Blonska M, Pappu BP, Matsumoto R, Li H, Su B, Wang D, et al. The CARMA1-Bcl10 signaling complex selectively regulates JNK2 kinase in the T cell receptor-signaling pathway. Immunity (2007) 26:55–66. doi: 10.1016/j.immuni.2006.11.008
54. Blonska M, Lin X. CARMA1-mediated NF-κB and JNK activation in lymphocytes. Immunol Rev. (2009) 228:199–211. doi: 10.1111/j.1600-065X.2008.00749.x
55. Qiao Q, Yang C, Zheng C, Fontan L, David L, Yu X, et al. Structural architecture of the CARMA1/Bcl10/MALT1 signalosome: nucleation-induced filamentous assembly. Mol Cell (2013) 51:766–79. doi: 10.1016/j.molcel.2013.08.032
56. David L, Li Y, Ma J, Garner E, Zhang X, Wu H. Assembly mechanism of the CARMA1-BCL10-MALT1-TRAF6 signalosome. Proc Natl Acad Sci USA. (2018) 115:1499–504. doi: 10.1073/pnas.1721967115
57. Pedersen SM, Chan W, Jattani RP, Mackie DS, Pomerantz JL. Negative Regulation of CARD11 Signaling and Lymphoma Cell Survival by the E3 Ubiquitin Ligase RNF181. Mol Cell Biol. (2016) 36:794–808. doi: 10.1128/MCB.00876-15
58. Lamason RL, Kupfer A, Pomerantz JL. The dynamic distribution of CARD11 at the immunological synapse is regulated by the inhibitor kinesin GAKIN. Mol Cell (2010) 40:798–809. doi: 10.1016/j.molcel.2010.11.007
59. Eitelhuber AC, Warth S, Schimmack G, Duwel M, Hadian K, Demski K, et al. Dephosphorylation of Carma1 by PP2A negatively regulates T-cell activation. EMBO J. (2011) 30:594–605. doi: 10.1038/emboj.2010.331
60. Carvalho G, Le Guelte A, Demian C, Vazquez A, Gavard J, Bidere N. Interplay between BCL10, MALT1 and IkappaBalpha during T-cell-receptor-mediated NFkappaB activation. J Cell Sci. (2010) 123:2375–80. doi: 10.1242/jcs.069476
61. Paul S, Traver MK, Kashyap AK, Washington MA, Latoche JR, Schaefer BC. T cell receptor signals to NF-κB are transmitted by a cytosolic p62-Bcl10-Malt1-IKK signalosome. Sci Signal. (2014) 7:ra45. doi: 10.1126/scisignal.2004882
62. Scharschmidt E, Wegener E, Heissmeyer V, Rao A, Krappmann D. Degradation of Bcl10 induced by T-cell activation negatively regulates NF-kappa B signaling. Mol Cell Biol. (2004) 24:3860–73. doi: 10.1128/MCB.24.9.3860-3873.2004
63. Lobry C, Lopez T, Israel A, Weil R. Negative feedback loop in T cell activation through IkappaB kinase-induced phosphorylation and degradation of Bcl10. Proc Natl Acad Sci USA. (2007) 104:908–13. doi: 10.1073/pnas.0606982104
64. Zeng H, Di L, Fu G, Chen Y, Gao X, Xu L, et al. Phosphorylation of Bcl10 negatively regulates T-cell receptor-mediated NF-κB activation. Mol Cell Biol. (2007) 27:5235–45. doi: 10.1128/MCB.01645-06
65. Moreno-Garcia ME, Sommer K, Shinohara H, Bandaranayake AD, Kurosaki T, Rawlings DJ. MAGUK-controlled ubiquitination of CARMA1 modulates lymphocyte NF-κB activity. Mol Cell Biol. (2010) 30:922–34. doi: 10.1128/MCB.01129-09
66. Paul S, Kashyap AK, Jia W, He YW, Schaefer BC. Selective autophagy of the adaptor protein Bcl10 modulates T cell receptor activation of NF-kappaB. Immunity (2012) 36:947–58. doi: 10.1016/j.immuni.2012.04.008
67. Jost PJ, Ruland J. Aberrant NF-κB signaling in lymphoma: mechanisms, consequences, and therapeutic implications. Blood (2007) 109:2700–7. doi: 10.1182/blood-2006-07-025809
68. Staudt LM. Oncogenic activation of NF-kappaB. Cold Spring Harb Perspect Biol. (2010) 2:a000109. doi: 10.1101/cshperspect.a000109
69. Rui L, Schmitz R, Ceribelli M, Staudt LM. Malignant pirates of the immune system. Nat Immunol (2011) 12:933–40. doi: 10.1038/ni.2094
70. Lenz G, Davis RE, Ngo VN, Lam L, George TC, Wright GW, et al. Oncogenic CARD11 mutations in human diffuse large B cell lymphoma. Science (2008) 319:1676–9. doi: 10.1126/science.1153629
71. Compagno M, Lim WK, Grunn A, Nandula SV, Brahmachary M, Shen Q, et al. Mutations of multiple genes cause deregulation of NF-κB in diffuse large B-cell lymphoma. Nature (2009) 459:717–21. doi: 10.1038/nature07968
72. Montesinos-Rongen M, Schmitz R, Brunn A, Gesk S, Richter J, Hong K, et al. Mutations of CARD11 but not TNFAIP3 may activate the NF-κB pathway in primary CNS lymphoma. Acta Neuropathol. (2010) 120:529–35. doi: 10.1007/s00401-010-0709-7
73. Pasqualucci L, Trifonov V, Fabbri G, Ma J, Rossi D, Chiarenza A, et al. Analysis of the coding genome of diffuse large B-cell lymphoma. Nat Genet. (2011) 43:830–7. doi: 10.1038/ng.892
74. Bu R, Bavi P, Abubaker J, Jehan Z, Al-Haqawi W, Ajarim D, et al. Role of NF-κB regulators-TNFAIP3 and CARD11 in middle eastern diffuse large B cell lymphoma. Leuk Lymphoma (2012) 53:1971–7. doi: 10.3109/10428194.2012.668286
75. Lohr JG, Stojanov P, Lawrence MS, Auclair D, Chapuy B, Sougnez C, et al. Discovery and prioritization of somatic mutations in diffuse large B-cell lymphoma (DLBCL) by whole-exome sequencing. Proc Natl Acad Sci USA. (2012) 109:3879–84. doi: 10.1073/pnas.1121343109
76. Schmitz R, Wright GW, Huang DW, Johnson CA, Phelan JD, Wang JQ, et al. Genetics and Pathogenesis of Diffuse Large B-Cell Lymphoma. N Engl J Med. (2018) 378:1396–407. doi: 10.1056/NEJMoa1801445
77. Kataoka K, Nagata Y, Kitanaka A, Shiraishi Y, Shimamura T, Yasunaga J, et al. Integrated molecular analysis of adult T cell leukemia/lymphoma. Nat Genet. (2015) 47:1304–15. doi: 10.1038/ng.3415
78. Da Silva Almeida AC, Abate F, Khiabanian H, Martinez-Escala E, Guitart J, Tensen CP, et al. The mutational landscape of cutaneous T cell lymphoma and Sezary syndrome. Nat Genet. (2015) 47:1465–70. doi: 10.1038/ng.3442
79. Wang L, Ni X, Covington KR, Yang BY, Shiu J, Zhang X, et al. Genomic profiling of Sezary syndrome identifies alterations of key T cell signaling and differentiation genes. Nat Genet. (2015) 47:1426–34. doi: 10.1038/ng.3444
80. Wu C, De Miranda NF, Chen L, Wasik AM, Mansouri L, Jurczak W, et al. Genetic heterogeneity in primary and relapsed mantle cell lymphomas: impact of recurrent CARD11 mutations. Oncotarget (2016) 7:38180–90. doi: 10.18632/oncotarget.9500
81. Vallois D, Dobay MP, Morin RD, Lemonnier F, Missiaglia E, Juilland M, et al. Activating mutations in genes related to TCR signaling in angioimmunoblastic and other follicular helper T-cell-derived lymphomas. Blood (2016) 128:1490–502. doi: 10.1182/blood-2016-02-698977
82. Lamason RL, Mccully RR, Lew SM, Pomerantz JL. Oncogenic CARD11 mutations induce hyperactive signaling by disrupting autoinhibition by the PKC-responsive inhibitory domain. Biochemistry (2010b) 49:8240–50. doi: 10.1021/bi101052d
83. Knies N, Alankus B, Weilemann A, Tzankov A, Brunner K, Ruff T, et al. Lymphomagenic CARD11/BCL10/MALT1 signaling drives malignant B-cell proliferation via cooperative NF-κB and JNK activation. Proc Natl Acad Sci USA. (2015) 112:E7230–8. doi: 10.1073/pnas.1507459112
84. Greil J, Rausch T, Giese T, Bandapalli OR, Daniel V, Bekeredjian-Ding I, et al. Whole-exome sequencing links caspase recruitment domain 11 (CARD11) inactivation to severe combined immunodeficiency. J Allergy Clin Immunol. (2013) 131:1376.e3–83.e3. doi: 10.1016/j.jaci.2013.02.012
85. Stepensky P, Keller B, Buchta M, Kienzler AK, Elpeleg O, Somech R, et al. Deficiency of caspase recruitment domain family, member 11 (CARD11), causes profound combined immunodeficiency in human subjects. J Allergy Clin Immunol. (2013) 131:477.e1–85.e1. doi: 10.1016/j.jaci.2012.11.050
86. Snow AL, Xiao W, Stinson JR, Lu W, Chaigne-Delalande B, Zheng L, et al. Congenital B cell lymphocytosis explained by novel germline CARD11 mutations. J Exp Med. (2012) 209:2247–61. doi: 10.1084/jem.20120831
87. Brohl AS, Stinson JR, Su HC, Badgett T, Jennings CD, Sukumar G, et al. Germline CARD11 Mutation in a Patient with Severe Congenital B Cell Lymphocytosis. J Clin Immunol. (2014) 35:32–46. doi: 10.1007/s10875-014-0106-4
88. Buchbinder D, Stinson JR, Nugent DJ, Heurtier L, Suarez F, Sukumar G, et al. Mild B-cell lymphocytosis in patients with a CARD11 C49Y mutation. J Allergy Clin Immunol. (2015) 136:819–21. doi: 10.1016/j.jaci.2015.03.008
89. Arjunaraja S, Nose BD, Sukumar G, Lott NM, Dalgard CL, Snow AL. Intrinsic plasma cell differentiation defects in B cell expansion with NF-κB and T cell anergy patient B cells. Front Immunol. (2017) 8:913. doi: 10.3389/fimmu.2017.00913
90. Arjunaraja S, Angelus P, Su HC, Snow AL. Impaired control of Epstein-Barr Virus infection in B-cell expansion with NF-κB and T-cell anergy disease. Front Immunol. (2018) 9:198. doi: 10.3389/fimmu.2018.00198
91. Ma CA, Stinson JR, Zhang Y, Abbott JK, Weinreich MA, Hauk PJ, et al. Germline hypomorphic CARD11 mutations in severe atopic disease. Nat Genet. (2017) 49:1192–201. doi: 10.1038/ng.3898
92. Dadi H, Jones TA, Merico D, Sharfe N, Ovadia A, Schejter Y, et al. Combined immunodeficiency and atopy caused by a dominant negative mutation in caspase activation and recruitment domain family member 11 (CARD11). J Allergy Clin Immunol. (2018) 141:1818.e2–30.e2. doi: 10.1016/j.jaci.2017.06.047
93. Li S, Yang X, Shao J, Shen Y. Structural insights into the assembly of CARMA1 and BCL10. PLoS ONE (2012) 7:e42775. doi: 10.1371/journal.pone.0042775
94. Jang TH, Park JH, Park HH. Novel disulfide bond-mediated dimerization of the CARD domain was revealed by the crystal structure of CARMA1 CARD. PLoS ONE (2013) 8:e79778. doi: 10.1371/journal.pone.0079778
95. Gross O, Grupp C, Steinberg C, Zimmermann S, Strasser D, Hannesschlager N, et al. Multiple ITAM-coupled NK-cell receptors engage the Bcl10/Malt1 complex via Carma1 for NF-κB and MAPK activation to selectively control cytokine production. Blood (2008) 112:2421–8. doi: 10.1182/blood-2007-11-123513
96. Hara H, Ishihara C, Takeuchi A, Xue L, Morris SW, Penninger JM, et al. Cell type-specific regulation of ITAM-mediated NF-κB activation by the adaptors, CARMA1 and CARD9. J Immunol. (2008) 181:918–30. doi: 10.4049/jimmunol.181.2.918
97. Rajasekaran K, Kumar P, Schuldt KM, Peterson EJ, Vanhaesebroeck B, Dixit V, et al. Signaling by Fyn-ADAP via the Carma1-Bcl-10-MAP3K7 signalosome exclusively regulates inflammatory cytokine production in NK cells. Nat Immunol. (2013) 14:1127–36. doi: 10.1038/ni.2708
98. So T, Soroosh P, Eun SY, Altman A, Croft M. Antigen-independent signalosome of CARMA1, PKCtheta, and TNF receptor-associated factor 2 (TRAF2) determines NF-κB signaling in T cells. Proc Natl Acad Sci USA. (2011) 108:2903–8. doi: 10.1073/pnas.1008765108
Keywords: CARD11, Bcl10, MALT1, primary immunodeficiency, lymphoma, T cell receptor, B cell receptor
Citation: Bedsaul JR, Carter NM, Deibel KE, Hutcherson SM, Jones TA, Wang Z, Yang C, Yang Y-K and Pomerantz JL (2018) Mechanisms of Regulated and Dysregulated CARD11 Signaling in Adaptive Immunity and Disease. Front. Immunol. 9:2105. doi: 10.3389/fimmu.2018.02105
Received: 05 June 2018; Accepted: 28 August 2018;
Published: 19 September 2018.
Edited by:
Frederic Bornancin, Novartis, SwitzerlandReviewed by:
Ruben Martinez-Barricarte, Rockefeller University, United StatesCopyright © 2018 Bedsaul, Carter, Deibel, Hutcherson, Jones, Wang, Yang, Yang and Pomerantz. This is an open-access article distributed under the terms of the Creative Commons Attribution License (CC BY). The use, distribution or reproduction in other forums is permitted, provided the original author(s) and the copyright owner(s) are credited and that the original publication in this journal is cited, in accordance with accepted academic practice. No use, distribution or reproduction is permitted which does not comply with these terms.
*Correspondence: Joel L. Pomerantz, am9lbC5wb21lcmFudHpAamhtaS5lZHU=
Disclaimer: All claims expressed in this article are solely those of the authors and do not necessarily represent those of their affiliated organizations, or those of the publisher, the editors and the reviewers. Any product that may be evaluated in this article or claim that may be made by its manufacturer is not guaranteed or endorsed by the publisher.
Research integrity at Frontiers
Learn more about the work of our research integrity team to safeguard the quality of each article we publish.