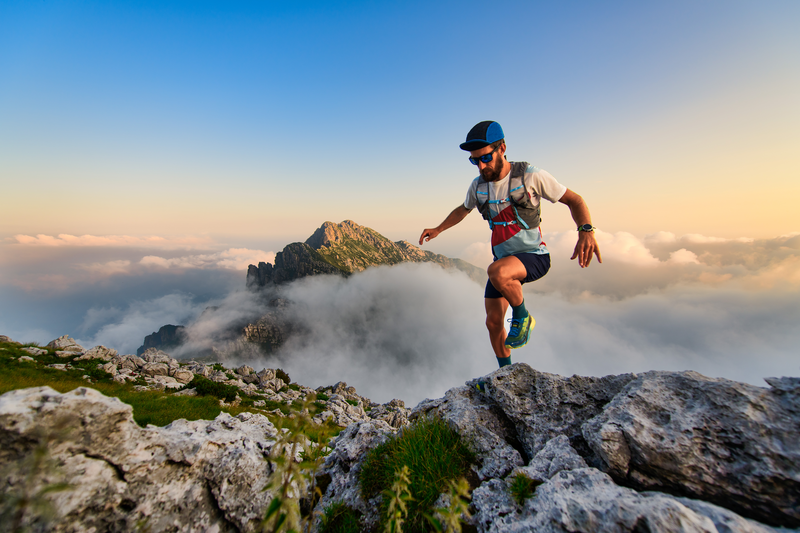
94% of researchers rate our articles as excellent or good
Learn more about the work of our research integrity team to safeguard the quality of each article we publish.
Find out more
ORIGINAL RESEARCH article
Front. Immunol. , 17 August 2018
Sec. Inflammation
Volume 9 - 2018 | https://doi.org/10.3389/fimmu.2018.01706
Degenerative disc disease is associated with increased expression of pro-inflammatory cytokines in the intervertebral disc (IVD). However, it is not completely clear how inflammation arises in the IVD and which cellular compartments are involved in this process. Recently, the endoplasmic reticulum (ER) has emerged as a possible modulator of inflammation in age-related disorders. In addition, ER stress has been associated with the microenvironment of degenerated IVDs. Therefore, the aim of this study was to analyze the effects of ER stress on inflammatory responses in degenerated human IVDs and associated molecular mechanisms. Gene expression of ER stress marker GRP78 and pro-inflammatory cytokines IL-6, IL-8, IL-1β, and TNF-α was analyzed in human surgical IVD samples (n = 51, Pfirrmann grade 2–5). The expression of GRP78 positively correlated with the degeneration grade in lumbar IVDs and IL-6, but not with IL-1β and TNF-α. Another set of human surgical IVD samples (n = 25) was used to prepare primary cell cultures. ER stress inducer thapsigargin (Tg, 100 and 500 nM) activated gene and protein expression of IL-6 and induced phosphorylation of p38 MAPK. Both inhibition of p38 MAPK by SB203580 (10 µM) and knockdown of ER stress effector CCAAT-enhancer-binding protein homologous protein (CHOP) reduced gene and protein expression of IL-6 in Tg-treated cells. Furthermore, the effects of an inflammatory microenvironment on ER stress were tested. TNF-α (5 and 10 ng/mL) did not activate ER stress, while IL-1β (5 and 10 ng/mL) activated gene and protein expression of GRP78, but did not influence [Ca2+]i flux and expression of CHOP, indicating that pro-inflammatory cytokines alone may not induce ER stress in vivo. This study showed that IL-6 release in the IVD can be initiated following ER stress and that ER stress mediates IL-6 release through p38 MAPK and CHOP. Therapeutic targeting of ER stress response may reduce the consequences of the harsh microenvironment in degenerated IVD.
Low back pain (LBP) is the leading cause of disability, activity limitation, and lost productivity throughout the world today, with approximately 80% of all people suffering from back pain at least once in their life (1). Degenerative disc disease (DDD), a progressive multifactorial disorder of intervertebral disc (IVD), often caused by inflammation and subsequent irritation of spinal nerves, is one of the main factors in the development of LBP (1). Despite the significant socio-economic consequences associated with DDD, no therapy can presently stop or reverse the degenerative process (2, 3). Current treatments are still only symptomatic, mainly comprising of general pain medication and spinal surgeries (4). Success of novel biological therapies (e.g., stem cell implantation) is often hindered by the pre-existing harsh inflammatory microenvironment in the degenerated IVD (5, 6). Nevertheless, mechanisms underlying the degenerative microenvironment in the IVD are not yet sufficiently understood.
DDD is characterized by a catabolic shift in IVD cells and a decline in extracellular matrix, altering load distribution and predisposing to LBP. LBP can raise both from IVD-induced damage of dorsal root ganglions (DRG) and from nociceptive stimulation of DRGs by molecules released from the degenerated IVD (7–9). Indeed, severity of DDD correlates with increased expression of inflammation mediators/markers in the IVD tissue (10), including interleukin-1 beta (IL-1β) (11), tumor necrosis factor-alpha (TNF-α) (12), interleukin-6 (IL-6), and interleukin-8 (IL-8) (13). However, it is still not completely clear how inflammation arises in the IVD and which cellular compartments or molecular pathways are involved in this process.
The endoplasmic reticulum (ER) is a cellular compartment responsible for protein synthesis, signaling, and intracellular calcium homeostasis. Recently, dysregulated ER function has emerged as a possible modulator of/contributor to inflammation in age-related pathologies, such as neurodegenerative diseases, type 2 diabetes, and rheumatoid arthritis (14–16). Dysregulation of ER function, the so-called ER stress, occurs when misfolded and/or unfolded proteins accumulate in the ER. ER stress upregulates chaperone glucose-regulated protein (GRP78, also HSPA5, and Bip), which then triggers unfolded protein response (UPR or ER stress response) to correct protein synthesis by increasing protein-folding capacity, decreasing protein translation rate, and degrading unfolded and misfolded proteins (17). However, prolonged UPR is commonly not able to resolve ER stress, activating pathways leading to cell death. In aging tissues, the UPR function deteriorates, shifting the balance toward degeneration and apoptosis (18).
Endoplasmic reticulum stress in the IVD can be induced by multiple mechanisms, including biomechanical alterations and non-physiological loading: unbalanced dynamic and static forces in the spine activated ER stress in a rat model (19). Likewise, ER stress was present in a rat model of puncture-induced IVD degeneration, where the UPR was accompanied by increased gene expression of IL-6 (20). The expression of GRP78 and its downstream targets in IVD cells was also found to be regulated by compression loading and low pH (21, 22). In addition, serum starvation and glucose deprivation induced ER stress in rat and goat IVD cells (20, 23). As such, ER stress may have protective, but also detrimental effects on IVD homeostasis. Recently, published rodent studies indicated that ER stress-induced apoptosis contributes to IVD degeneration (19, 20) and that ER stress could correlate with inflammation in human IVD tissue (20). Zhao et al. (19) showed that the instability of the lumbar spine significantly upregulated the expression of ER stress markers [GRP78 and CCAAT-enhancer-binding protein homologous protein (CHOP)] as well as markers of apoptosis (caspase-12 and cytochrome C) in the rat IVD. Moreover, the degree of IVD cell apoptosis correlated with the expression of GRP78 (19). Fujii et al. (20) revealed that the induction of ER stress in rat and human AF cells activated gene expression of TNF-α and IL-6, with protein kinase R-like ER kinase (PERK) and nuclear factor κB (NF-κB) likely being involved (20).
Emerging evidence hence suggests that ER stress is present in human degenerated IVDs and contributes to DDD, possibly through induction of inflammation, but the molecular connections between these processes remain unclear. Therefore, the aim of this study was to analyze the relationship and possible crosstalk between ER stress and inflammation in degenerated human IVDs. Understanding the crosstalk between inflammation and ER stress could help in designing therapies that mitigate the harsh environment in degenerated IVD, and thus provide new options for biological IVD repair.
51 human IVD specimens were obtained with informed consent from 45 patients [mean age = 52 years (age 16–79 years)] undergoing elective spinal surgery for treatment of DDD (23 patients) or disc herniation (DH) (22 patients) in the cervical (n = 24) or lumbar (n = 27) region. Due to the tissue size, cervical samples were collected as entire discs. Lumbar discs were intraoperatively excised as annulus fibrosus (AF, n = 12) and nucleus pulposus (NP, n = 15) samples, followed by macroscopic tissue evaluation. Assessment of the disease state was performed using Pfirrmann grading (IVD degeneration) and Modic grading (endplate changes). Detailed patient information is given in Ref. (13). The study was approved through the local ethics committee (Ethics Committee of the Canton Lucerne/Switzerland, #1007).
RNA extraction and the following steps were performed according to Ref. (13). For the cDNA synthesis, 2 µg of RNA were used in a total volume of 60 µL, using the reverse transcription kit (4374966, Applied Biosystems). For samples with lower yields, the reverse transcription was conducted at reduced concentrations. cDNA (10 ng/well) was mixed with TaqMan Fast Universal PCR Master Mix and TaqMan primers (GRP78, IL-6, IL-8, IL-1β, TNF-α, and GAPDH; Table 1) to quantify gene expression. The obtained Ct values were analyzed by a comparative method (gene of interest relative to GAPDH) and displayed as 2−dCt values.
Mixed human lumbar IVD tissue was removed from 25 patients during IVD surgeries [mean age = 51 years (age 29–76 years)]. Tissue was enzymatically digested using a mixture of 0.2% collagenase NB4 (17454, Serva, Heidelberg, Germany) and 0.3% dispase II (04942078001, Roche, Basel, Switzerland) for 4–8 h at 37°C. Isolated primary cells were seeded in Dulbecco’s Modified Eagle’s Medium (DMEM/F12, D8437, Sigma, St. Louis, MO, USA) supplemented with 10% fetal calf serum (FCS, F7524, Sigma) and 1% Antibiotics–Antimycotics (A/A) (15240062, Gibco, Carlsbad, CA USA) and sub-cultured up to passage 3 using 1.5× trypsin (15090-046, Gibco) at 37°C with 5% CO2. This study was approved by the Kantonale Ethikkommission Zürich EK-16/2005 and patient informed consents were granted.
Interverterbral disc cells were seeded in 6-well plate (2 × 105 cells/well; gene expression analysis), 12-well plate (1 × 105 cells/well; immunoblotting, MTT), or 96-well plate (0.4 × 105 cells/well; calcium flux assay) and cultured for 18 h. The next day, the cells were serum-starved for 2 h and exposed to the compounds shown in the Table 2 for 1, 3, and/or 24 h in FCS- and A/A-free media. Following the treatments, the cells were analyzed by RT-qPCR (gene expression), MTT assay (metabolic activity) and immunoblotting (protein expression). Cell culture media was used for enzyme-linked immunosorbent assay (ELISA) (protein release) and LDH (cytotoxicity test).
Intervertebral disc cells were seeded in 12-well plate (1 × 105 cells/well) in DMEM/F-12 supplemented with 10% FCS and 1% A/A and mixed with siRNA complexes according to the manufacturer’s protocol (Fast-Forward protocol, Flexi Tube system, Qiagen). Briefly, 5 nM siRNAs against CHOP (Table 2) and Hiperfect (HF, 301705, Qiagen) were incubated in Opti-MEM (31985, Gibco) for 7 min to form complexes. The complexes were then added to the cell suspension drop-wise and incubated for 18 h. Untreated cells and cells treated with Hiperfect were used as controls. On the next day, the media was changed to FCS-A/A-free media and incubated for 2 h before Tg was added. After 24 h, the cells and culture media were collected for immunoblot analysis of CHOP and ELISA of released IL-6, IL-8, and prostaglandin E2 (PGE2). As siRNA CHOP_2 did not produce sufficient knockdown, only the data obtained with siRNA_CHOP1 were shown.
RNA was extracted with the Trizol/chloroform (15596-018, Invitrogen, Carlsbad, CA, USA) according to the manufacturer’s instructions. 1 µg of cDNA was reverse transcribed from RNA using a reverse transcription kit (4374966, Applied Biosystems, Foster City, CA, USA) and mixed with the primers (Table 1) and Fast universal master mix (4352042, Applied Biosystems). Gene expression was examined by qPCR. Data were analyzed by the comparative 2−ΔΔCt method, with TATA box binding protein as housekeeping gene. Results were presented as gene expression relative to control (fold change) or gene expression relative to Tg treatment (%).
Cells were lysed and mixed with Laemmli buffer (S3401, Sigma). The lysates were boiled (96°C, 5 min) and loaded onto 4–20% SDS-polyacrylamide gels (456-8093, Biorad). Proteins were separated by electrophoresis in a Mini-PROTEAN Tetra cell chamber (Biorad) and transferred to polyvinylidene difluoride membranes (1704156, Biorad). Following transfer, the membranes were blocked in 5% non-fat milk in Tris-buffered saline-Tween (TBS-T) for 1 h at room temperature and primary antibodies (Table 3) were applied overnight at 4°C under gentle shaking. The next day, membranes were washed in 1% non-fat milk in TBS-T (3 × 10 min), incubated with secondary antibodies conjugated to horseradish peroxidase (HRP) for 1 h at room temperature, and then washed again in 1% non-fat milk in TBS-T (3 × 10 min). Tubulin was used as loading control. Visualization was performed by chemiluminescence kit West Dura (34076, Thermo Scientific, Waltham, MA, USA) on ChemiDoc imager (Biorad). The obtained bands were quantified in ImageJ x64 by normalizing to loading control and calculating band density relative to untreated control. Resulting graphs show an average of three independent donors.
Inflamed IVD cells typically release pro-inflammatory cytokines (24, 25). IL-6 and IL-8 secretion was measured by ELISA according to the producer’s protocols (BD OptEIA™ Human IL-6 ELISA set, 555220; Human IL-8 ELISA set, 555244; and Reagent set B, 550534, BD Biosciences, San Jose, CA, USA). Briefly, 96-well plate was coated by kit capture antibody at 4°C overnight, washed, and blocked. Samples and standards were pipetted into the wells in duplicates and the plates were incubated for 2 h at room temperature. The plates were washed and kit detection antibodies and solutions were applied. After the signal was developed, absorbance was measured at 450 nm with 570 nm correction. Total IL-6 and IL-8 release in culture media was plotted (pg/mL). PGE2 is a product of COX-2-mediated enzymatic transformation of arachidonic acid. PGE2 release in culture media was measured by PGE2 ELISA (KGE004B, RD systems) according to the manufacturer’s recommendations. Results are presented as protein release (pg/mL) or the release relative to Tg treatment (%).
To verify whether performed treatments influenced cell viability, Cytotoxicity Assay Kit (88954, Pierce) was used to measure lactate dehydrogenase (LDH) release into the culture media according to the manufacturer’s recommendations. Results were displayed as cytotoxicity relative to control (fold change).
To verify whether performed treatments influenced cell metabolic activity, MTT (3-[4,5-dimethylthiazol-2-yl]-2,5-diphenyl tetrazolium bromide, M5655, Sigma) was used. Following seeding and treatments, fresh MTT solution (0.5 mg/mL) was added and kept for 3 h at 37°C. MTT was discarded, cells were lysed in DMSO (D8418, Sigma), and the absorbance was measured at 565 nm. Metabolic activity was calculated relative to the untreated control (100%).
Unbalanced intracellular calcium levels can initiate ER stress. Intracellular calcium [Ca2+]i was analyzed by Fura-2-AM solution (Fura-2 QBT™ Calcium Kit, component A, Molecular Devises, CA, USA) according to the manufacturers’ recommendations. Briefly, IVD cells were seeded in 96-well plate and incubated for 18 h. The next day, the media was changed to the phenol red-free media (11039, Gibco) supplemented with Fura-2-AM solution and cells were incubated for 1 h. Fura-2 fluorescence was recorded using a plate reader (Infinite M200pro TECAN, Switzerland) at excitation wavelengths of 340 and 380 nm and an emission wavelength of 510 nm. After 5 cycles, the cells were exposed to putative calcium flux activators IL-1β (10 ng/mL), TNF-α (10 ng/mL), and Tg (100 and 500 nM), with 1 µM ionomycin (I3909, Sigma) as positive control. Fura-2 fluorescence continued to be recorded for up to 15 min. The assay was performed in triplicates. The data were presented as the ratio of 340/380 signal.
Data consistency was checked and data were screened for outliers by using quantile plots and normality using Kolmogorov–Smirnov test. Due to the given distributions, generalized linear models were not applicable and, hence, Wilcoxon-matched pairs test, Mann–Whitney U test and Spearman correlation was used to analyze continuously distributed data. One-factorial ANOVA with Tukey’s post hoc test was used to test means among different groups. All reported tests were two sided, and p-values <0.05 were considered to be statistically significant. All statistical analyses in this report were performed using STATISTICA 13 (Hill, T. & Lewicki, P. Statistics: methods and applications. StatSoft, Tulsa, OK, USA) GraphPad Prism 7 and PASW 22 (IBM SPSS Statistics for Windows, Version 21.0., Armonk, NY, USA) and StatXact 10 (Cytel Software 2013, Cambridge MA, USA).
Gene expression of ER stress marker GRP78 in human IVD samples in relation to the IVD degeneration grade [grades 2–5 (26)], duration of pain, spine level (cervical or lumbar), type of pathology (DDD or DH), and disc region (NP or AF) are illustrated (Figure 1). GRP78 was expressed in 20 out of 24 cervical samples, 11 out of 12 lumbar AF samples, and 14 out of 15 lumbar NP samples. GRP78 expression in cervical and lumbar IVDs together showed a tendency to increase with the degeneration grade (p = 0.0593; Figure 1A) and association with the duration of the pain symptoms (p = 0.015, sub-acute vs chronic; Figure 1B), with high variability in the acute group. The expression of GRP78 was significantly lower in lumbar IVDs, when compared to cervical IVDs (p = 0.0044; Figure 1C). Due to the possible morphological and biomechanical differences between cervical and lumbar IVDs, the expression of GRP78 was displayed separately in cervical and lumbar spine in relation to the degeneration grade and type of pathology. Cervical IVDs showed no association between the expression of GRP78 and degeneration grade (Figure 1D) and the type of pathology (Figure 1E). In lumbar IVDs, the expression of GRP78 significantly increased with increasing degeneration grade (2 vs 3 p = 0.0019, 2 vs 4 p = 0.0399, and 2 vs 5 p = 0.0041; Figure 1F). However, caution must be taken when interpreting these data, as grades 2 and 5 (cervical) contain only two samples and grade 4 (lumbar) contains only three samples. GRP78 was expressed more in AF than NP (p = 0.0128) (Figure 1G). The expression of GRP78 was not significantly different between DDD and DH (Figure 1H). The expression of GRP78 in NP and AF samples in relation to degeneration grade and type of pathology is shown in the Figure S1 in Supplementary Material.
Figure 1. The expression of GRP78 in human degenerated intervertebral discs (IVDs). IVDs were collected from donors during spinal surgeries. Gene expression of GRP78 in cervical and lumbar IVDs (n = 51 donors) (A) tended to increase with degeneration grade (p = 0.0593) and significantly increased with (B) duration of pain (p = 0.015) and (C) in cervical IVDs, when compared with lumbar IVDs (p = 0.0044). (D) Gene expression of GRP78 in cervical IVDs (n = 20 donors) was not significantly different between grades and (E) type of pathology. (F) Gene expression of GRP78 in lumbar IVDs (n = 25 donors) increased with degeneration grade (2 vs 3 p = 0.0019, 2 vs 4 p = 0.0399, and 2 vs 5 p = 0.0041). (G) A difference in GRP78 expression was found between the disc regions [n = 12 (AF), 15 (NP), p = 0.0128], but not (H) between different pathologies. Calculated as 2−ΔCt values (relative to GAPDH). Abbreviations: DDD, degenerative disc disease; DH, disc herniation; AF, annulus fibrosus; NP, nucleus pulposus.
The expression of inflammation markers, such as IL-1β, TNF-α, IL-6, and IL-8 in these samples was measured as well (13). Interestingly, the expression of GRP78 positively correlated with IL-6 (r = 0.63, p < 0.0001) and IL-8 (r = 0.47, p = 0.0001), while no correlation between the expression of GRP78 and IL-1β or TNF-α was found (p = 0.4641, p = 0.1709, respectively) (Table 4). To confirm the presence of ER stress in IVD tissue, activating transcription factor (ATF4) was measured as another ER stress marker (n = 13). GRP78 positively correlated with ATF4 (r = 0.62, p = 0.0105). The expression of ATF4 positively correlated with the inflammation markers IL-6 (r = 0.72, p = 0.0021) and IL-8 (r = 0.63, p = 0.0110) (Table 4).
Table 4. The expression of GRP78 is positively correlated with IL-6 and IL-8, but not with IL-1β and TNF-α. The expression of ATF4 is positively correlated with GRP78, IL-6, and IL-8 (calculated by Spearman correlation test).
Due to the possible association between ER stress and degeneration grade in the lumbar region, cells isolated from lumbar IVDs were used in the following cell culture study. It has been shown that IVD cells can respond to stress by increasing the concentration of [Ca2+]i (27). Therefore, thapsigargin (Tg), an inhibitor of the sarco/ER Ca2+ ATPase, was used to chemically induce ER stress in IVD cells, as it raises [Ca2+]i by blocking the ability of the cell to pump calcium into the ER (28). IVD cells were treated with 100 and 500 nM Tg for 1, 3, or 24 h. Tg treatment elevated [Ca2+]i (Figure 2A) and increased the expression of ER stress markers, such as PERK, IRE1α, GRP78, and CHOP on the protein level (Figures 2B,C) and gene level (Figures 2D,E). The effects of 100 nM Tg on cell death were also tested. Tg did not significantly reduce cellular metabolic activity nor induce cell death (Figures 2F,G). ER stress-induced JNK activation, known to influence cell death through the regulation of BCL2 family proteins (29), was not observed (Figure 2H). Therefore, Tg was used further to mimic sub-lethal ER stress in IVD cells. P-values are shown in the Table S1 in Supplementary Material.
Figure 2. Sub-lethal endoplasmic reticulum (ER) stress induction by thapsigargin in intervertebral disc (IVD) cells. IVD cells were isolated from degenerated lumbar IVDs and treated with 100 and 500 nM thapsigargin (Tg). Treatment with Tg (A) caused steady calcium flux (n = 3 donors; the arrow indicates the time point when Tg was added), (B,C) induced the expression of ER stress-associated proteins PERK, IRE, GRP78, and C/EBP homologous protein (CHOP) (n = 3 donors), and (D,E) activated gene expression of GRP78 and CHOP (n = 7 donors; relative to control = set at 1). (F–H) 100 nM Tg did not influence cell metabolic activity (n = 3 donors; relative to control = set at 100) and viability (n = 13 donors; relative to control = set at 1), nor activated JNK (n = 4 donors). IL-1β (10 ng/mL) was used as positive control for immunoblotting. Data are presented as mean ± SEM, *p < 0.05 (ANOVA with Tukey post hoc test).
The expression of inflammation-associated genes and proteins was tested in IVD cells treated with 100 and 500 nM Tg for 24 h. ER stress caused by Tg significantly induced gene expression of IL-6, IL-8, and COX-2 (Figures 3A,C,E). ER stress also significantly activated protein release of IL-6 (Figure 3B), indicating the involvement of ER stress in inflammatory responses of IVD cells. The release of IL-8 in the Tg group was not significantly elevated (Figure 3D), likely due to the modest increase in gene expression. Increased expression of COX-2 protein in Tg-treated cells was observed (Figure 3G). The protein release of PGE2, a downstream product COX-2, tended to increase in the Tg group, but not significantly (Figure 3F). It should be noted that PGE2 release was detectable only in one control sample and that the PGE2 release could be over/under estimated due to the kit detection limit (40 pg/mL). Therefore, the presented PGE2 data should be interpreted with caution. Tg did not influence the expression of matrix metalloproteinases 1 and 13, while IL-1β and TNF-α were expressed neither in controls, nor in Tg-treated samples (Ct values higher than 40, data not shown). Inter-donor differences in Tg-induced protein expression and release were detected. P-values of all tests are shown in the Table S1 in Supplementary Material.
Figure 3. The expression of IL-6, IL-8, and COX-2 in endoplasmic reticulum stress. Cells were isolated from degenerated lumbar intervertebral discs (IVDs) and treated with 100 and 500 nM thapsigargin (Tg). (A,C,E) Treatment with Tg significantly induced gene expression of IL-6, IL-8, and COX-2 (n = 7 donors, relative to control = set at 1) and (B,D,F) secretion of IL-6, but not IL-8 and PGE2 (n = 7 donors). (G) Tg treatment elevated the protein expression of COX-2 (n = 4 donors). IL-1β (10 ng/mL) was used as positive control for immunoblotting. Data are presented as mean ± SEM, *p < 0.05 (ANOVA with Tukey post hoc test).
The functional crosstalk between ER stress and inflammatory responses was examined by testing activation of typical mediators involved in IVD inflammation, namely p38 mitogen-activated protein kinase (p38 MAPK) and NF-κB. A common inducer of these pathways, IL-1β (10 ng/mL), was used as a positive control. 3 h treatment with 100 nM Tg stimulated the phosphorylation of p38 MAPK (Figure 4A). The NF-κB pathway, induction of which manifests by phosphorylation of NF-κB (p65) and degradation of its inhibitor IκB-α, was not activated by 100 nM Tg (Figure 4B).
Figure 4. The involvement of p38 MAPK in the expression and release of IL-6, IL-8, and PGE2. Cells were isolated from degenerated lumbar intervertebral discs and treated with 100 nM thapsigargin (Tg) alone or in combination with 10 µM small molecule inhibitor of p38 SB203580 (SB). (A) 3 h treatment with Tg induced phosphorylation of p38 MAPK (n = 4 donors). (B) NF-κB pathway (tested by phosphorylation of NF-κB and simultaneous degradation of IKB-α) was not activated by 3 h treatment with Tg (n = 4 donors). (C,E,G) Combination of Tg and SB reduced gene expression of IL-6, and COX2, but not IL-8 (n = 4 donors). (D,F,H) SB reduced protein release of IL-6, but not IL-8 and PGE2 (n = 4 donors). IL-1β (10 ng/mL) was used as a positive control for immunoblotting. Data are presented as mean ± SEM, *p < 0.05 (ANOVA with Tukey post hoc test).
To test the functional involvement of p38 in Tg-induced gene and protein expression of IL-6, IL-8, and COX-2, the small molecule inhibitor of p38 MAPK SB203580 (SB; 10 µM) was used. Inhibition of p38 significantly reduced gene expression of IL-6 and COX-2, while the expression of IL-8 remained unchanged (Figures 4C,E,G). In addition, the release of IL-6 into the culture media was significantly inhibited by SB, indicating functional involvement of p38 in ER stress-induced inflammatory responses of the IVD. SB did not influence the release of IL-8 and PGE2 (Figures 4D,F,H). Cell viability (tested by LDH assay) in all treatment groups remained comparable to control (Figure S2 in Supplementary Material).
A putative downstream target of p38 is CHOP (or GADD153), a transcription factor that mediates ER stress-induced autophagy, apoptosis, or inflammation (30). The involvement of CHOP in the observed effects of Tg was tested by CHOP siRNA knockdown. The CHOP knockdown (Figure 5D) reduced the release of IL-6, indicating the participation of CHOP in ER stress-induced inflammatory responses of IVD cells. CHOP knockdown did not influence the release of IL-8 and PGE2 (Figures 5A–C). The transfection reagent Hiperfect (HF) did not influence gene expression of CHOP and IL-6 release (Figure S3 in Supplementary Material). Cell viability in all treatment groups remained similar to control (tested by LDH) (Figure S2 in Supplementary Material). The relationship between p38 and CHOP was evaluated in Tg-treated cells by gene expression analysis of CHOP with and without SB as well as by activation of p38 in the presence and absence of siRNA against CHOP. p38 inhibition partially reduced gene expression of CHOP (Figure 5E), while CHOP knockdown did not seem to reduce p38 phosphorylation (Figure 5F). However, caution must be taken when interpreting quantified immunoblotting results, as non-significant results may arise from disease-related inter-donor variability.
Figure 5. The effects of C/EBP homologous protein (CHOP) knockdown on the protein release of IL-6, IL-8, and PGE2. Cells were isolated from degenerated lumbar intervertebral discs (IVDs) and treated with 100 nM thapsigargin (Tg) in combination with 5 nM negative control siRNA (siRNA NC, scrambled) or 5 nM siRNA against CHOP (siCHOP). (A–C) CHOP knockdown reduced the release of IL-6, but not IL-8 and PGE2 (n = 3 donors). (D) Tg-activated endoplasmic reticulum stress, as shown by expression of GRP78, PERK, IRE1α, and their downstream effector CHOP. siRNA produced partial knockdown of CHOP gene. (E) p38 inhibition partially reduced gene expression of CHOP in Tg-treated cells (n = 4 donors). (F) CHOP knockdown did not reduce p38 phosphorylation by Tg (n = 3 donors). Data are presented as mean ± SEM, *p < 0.05 (ANOVA with Tukey post hoc test).
Various events involved in IVD degeneration can possibly activate ER stress either directly or through disturbed [Ca2+]i homeostasis. Pro-inflammatory cytokines IL-1β and TNF-α are common inducers of inflammatory responses in the IVD. As an example, IL-1β was shown to activate p38-mediated expression of IL-6, COX-2, and PGE2 in Ref. (31) and in Figure S4 in Supplementary Material. Therefore, the next step was to test the effects of IL-1β and TNF-α on [Ca2+]i and ER stress. It was found that 5 and 10 ng/mL IL-1β, but not 5 and 10 ng/mL TNF-α, slightly induced gene and protein expression of GRP78 (Figures 6A,B). Both cytokines neither activated [Ca2+]i flux (Figure 6C), nor the expression of CHOP (Figure 6D), suggesting that IL-1β and TNF-α alone are not able to induce ER stress.
Figure 6. The effects of pro-inflammatory cytokines Il-1β and TNF-α on the expression of GRP78, CCAAT-enhancer-binding protein homologous protein (CHOP), and calcium flux. Cells were isolated from degenerated intervertebral discs and treated with 5 and 10 ng/mL of IL-1β and TNF-α. (A,B) IL-1β, but not TNF-α, activated gene and protein expression of GRP78 [n = 4 donors (gene), n = 3 donors (protein)]. (C) Activation of [Ca2+]i flux by IL-1β and TNF-α was not found (n = 3 donors; the arrow indicates the time point when the cytokines were added). (D) Neither IL-1β nor TNF-α activated gene expression of CHOP (n = 4 donors). Data are presented as mean ± SEM, *p < 0.05 (ANOVA with Tukey post hoc test).
Cytokines including IL-6, IL-8, or TNF-α are significantly elevated in degenerated human IVDs (32) and contribute to the inflammatory responses (25) and neuronal sensitization during LBP (33). However, the sources of inflammation in the IVD are still not fully identified. Over the past years, the participation of the ER in IVD homeostasis has been increasingly studied (20), but the role of ER stress in the pathogenesis of DDD still remains unclear. This study aimed to investigate the involvement of ER stress in inflammatory responses of the IVD and to elucidate the molecular crosstalk between these processes.
The study showed an association between ER stress and degeneration grade in lumbar, but not cervical IVDs, which could arise from differences in morphology and biomechanics between these regions. In lumbar IVDs, GRP78 was significantly more expressed in the AF, which could be explained by its association with stretch-induced behavior (34).
To our knowledge, this is the first study that tested and found a correlation between gene expression of IL-6 and ER stress markers in human surgical IVD tissue. The complementary cell culture tests confirmed that ER stress regulates the expression and release of IL-6 by IVD cells, supporting the observations from the patient’s IVDs. Previously, it has been shown that ER stress is accompanied by increased gene expression of IL-6 in a rat model of puncture-induced IVD degeneration (20). ER stress also activated the expression of COX-2, an enzyme involved in the synthesis of PGE2 and clinically relevant target of nonsteroidal anti-inflammatory drugs. Although our data on PGE2 release did not correspond to the COX-2 gene expression data, it should be noted that the measured values were close to the lower detection limit of the used ELSIA (40 pg/mL), and thus this data should be interpreted with caution (for a comparison, the lower detection limits of IL-6 and IL-8 ELISAs were 4 pg/mL).
Common inducers of inflammatory responses in the IVD, IL-1β and TNF-α, were previously shown to activate ER stress in IVD cells (35): 10 ng/mL TNF-α upregulated ER stress markers and initiated UPR in rat IVD cells (36), while 75 ng/mL IL-1β activated gene and protein expression of ER stress markers (including GRP78 and CHOP) in human primary IVD cells (37). However, in our study, IL-1β and TNF-α did not induce a prolonged ER stress response. Although 10 ng/mL IL-1β enhanced the expression of GRP78, it did not activate calcium flux and gene expression of CHOP. The data suggest that IL-1β and TNF-α alone are likely not able to induce ER stress in degenerated human IVDs in vivo, where their concentrations are typically lower than in our study (32). This could explain the lack of correlation between IL-1β, TNF-α, and GRP78 in surgical IVD samples. Importantly, IL-6 release in human IVD cells could be initiated without participation of IL-1β and TNF-α, as a direct consequence of ER stress.
Not only cytokines, but also other factors contribute to the harsh microenvironment in degenerated IVDs. Factors like biomechanical alterations, low pH, and glucose deprivation were shown to induce ER stress in IVD tissue and cells (20–23). Our data suggest that ER stress can activate IL-6 release through p38 and CHOP, as shown in the scheme (Figure 7). Prolonged p38 activation is generally associated with cellular stress and induces inflammatory responses (31), cell cycle arrest (38), or apoptosis (39). For example, p38 was shown to promote ER stress-induced apoptosis in fibroblasts (40) and ER stress-induced inflammation in macrophages (41). Importantly, p38 can phosphorylate CHOP and this phosphorylation is required for CHOP activation (42, 43). In addition, p38 can activate the transcription of CHOP through ATF6 (44). In our study, p38 inhibition partially reduced gene expression of CHOP, while CHOP knockdown did not reduce p38 phosphorylation. Therefore, we suggest that the observed ER stress-induced IL-6 release may be partially dependent on transcriptional regulation of CHOP by p38.
Figure 7. Suggested mechanism of endoplasmic reticulum (ER) stress-induced inflammatory responses in intervertebral disc (IVD) cells. Stress stimuli (such as biomechanical alterations or low pH) can cause dysregulation of calcium homeostasis and sub-lethal ER stress. This in turn activates p38, which induces the expression and phosphorylation of C/EBP homologous protein (CHOP) and subsequent IL-6 release. In its initial phase, ER stress response may help IVD cells to cope with non-physiological stimuli, while severe ER stress may promote inflammation and apoptosis. A previous IVD-related study (20) showed that ER stress activates IL-6 release through NF-κB, which is negatively regulated by CHOP (shown in gray).
In the previous study on rat AF cells, ER stress activated gene expression of inflammatory cytokines through the NF-κB pathway (20). We found no JNK and NF-κB activation at the 1 and 3 h time point, which were selected to test prolonged activation of inflammatory mediators commonly associated with ER stress response (45, 46). Possible transient activation of JNK and/or NF-κB at shorter time points (e.g., 15 min) cannot be excluded. It has been shown that NF-κB-induced IL-6 release in the IVD can be negatively regulated by CHOP (20), suggesting yet unexplored crosstalks between ER stress and inflammation may exist (Figure 7). Severe ER stress is known to activate cell death. In previous ER stress-related studies, active JNK-induced caspase-12-mediated apoptosis (47, 48), while ER stress-induced activation of another MAP kinase ERK (not tested in our study) had pro-survival effects (49).
The activation of CHOP has traditionally been considered pro-apoptotic (50); however, in our study the mild induction of CHOP was not associated with cell death. Recent literature suggests that CHOP plays a role in the pathogenesis of inflammation (other cell types) (30, 51), could thus constitute a link between ER stress and inflammatory responses in the IVD. As an example, CHOP was shown to be involved in the release of IL-6 in macrophages during atherosclerosis (41) and generation of reactive oxygen species (ROS) and ROS-induced damage in diabetes (52, 53). The mechanistic details on CHOP involvement in IL-6 release should be investigated further, preferably in IVD cells with naturally induced ER stress (e.g., by low pH or starvation).
Tissues and primary cells were isolated from randomized donors with different demographics (e.g., age and associated pathologies). Commonly, natural variability in such sample group leads to inter-donor differences in protein activation and release, reducing potential statistical significance of observed effects. Although not all statistically significant, our data suggest that ER stress could indeed participate in inflammatory responses of the IVD through p38 and CHOP. Statistically significant association between ER stress and IVD degeneration was confirmed previously in animal models, which do not suffer from non-uniformity arising from natural variability (19).
It is known that the harsh microenvironment in degenerated IVD can hinder biological regenerative therapies (54). Therapeutic modulation of ER stress could possibly contribute to mitigate these effects. ER stress-inflammation crosstalk could be therapeutically targeted on several levels. [Ca2+]i dysregulation and/or protein misfolding could be targeted by molecular chaperones, compounds that prevent aggregation and promote ER function, a decrease of which is typical for degenerative disorders (55). To date, the beneficial effects of some compounds modulating ER stress responses (e.g., Tauroursodeoxycholic acid) have been shown in preclinical studies for neurodegenerative, cardiovascular, and metabolic disorders (55–57). ER stress effectors, such as the transcription factor CHOP, could be modulated, e.g., by gene editing using clustered regularly interspaced short palindromic repeats (CRISPR)/CRISPR-associated protein 9 techniques (knock-out, knock-down) (58). It should not be forgotten that ER stress in its initial stages has protective effects toward restoring cellular homeostasis. Healthy cells are able to resolve ER stress by increased expression of molecular chaperones and UPR genes, and thus inhibition of UPR molecules (e.g., GRP78, PERK, and IRE) may not be desired at this stage. On the other hand, chronic ER stress has detrimental consequences that inhibition of ER stress effectors (e.g., CHOP) could help to reduce.
This study is one of the first to describe an active role of ER stress in inflammatory responses of human IVD. More studies will follow to elucidate the importance of related molecular mechanisms as therapeutic targets to mitigate the harsh microenvironment of degenerated IVD.
51 human IVD specimens were obtained with informed consent from 45 patients [mean age = 52 years (age 16–79 years)] undergoing elective spinal surgery for treatment of degenerative disc disease (23 patients) or disc herniation (22 patients) in the cervical (n = 24) or lumbar (n = 21) region. The study was approved through the local ethics committee (Ethics Committee of the Canton Lucerne/Switzerland, #1007). Human IVD tissue for the cell tests was removed from 25 patients during IVD surgeries [mean age = 51 years (age 29–76 years)]. The study was approved by the Kantonale Ethikkommission Zürich EK-16/2005. Patient informed consents were granted.
OK planed the study, drafted the manuscript, and performed the cell culture experiments. AS performed the tissue-related experiments. TK performed the calcium imaging experiments. WH performed statistical analysis on tissue samples. OH and JK collected human IVD samples and provided clinical expertise. KW-K conceived funding, provided expertise, and helped to draft the manuscript.
The authors declare that the research was conducted in the absence of any commercial or financial relationships that could be construed as a potential conflict of interest.
The authors would like to thank Helen Greutert for lab management support.
The Supplementary Material for this article can be found online at https://www.frontiersin.org/articles/10.3389/fimmu.2018.01706/full#supplementary-material.
Figure S1. The expression of GRP78 in NP and AF of human lumbar intervertebral discs (IVDs). IVDs were collected from donors during spinal surgeries. Gene expression of GRP78 in lumbar NP (n = 15 donors) according to the (A) degeneration grade and (B) type of pathology. Gene expression of GRP78 in lumbar AF (n = 12 donors) according to the (C) degeneration grade and (D) type of pathology. Calculated as 2−ΔCt values (relative to GAPDH). Abbreviations: DDD, degenerative disc disease; DH, disc herniation; AF, annulus fibrosus; NP, nucleus pulposus. The data are not significant.
Figure S2. Cytotoxicity of the used treatments. Cells were isolated from degenerated intervertebral discs and treated for 24 h with 100 and 500 nM thapsigargin (Tg) alone or in combination without (A) p38 inhibitor SB2013580 (SB, 10 µM) or (B) siRNA against C/EBP homologous protein (CHOP) (siCHOP, 5 nM) and scrambled siRNA negative control (siNC, 5 nM). None of the treatments caused significant cells death, when compared with controls. Data are presented as mean ± SEM relative to control, *p < 0.05, n = 4 (ANOVA with Tukey post hoc test).
Figure S3. The effects of transfection reagent Hiperfect (HF) on gene expression of CCAAT-enhancer-binding protein homologous protein (CHOP) and protein release of IL-6. (E) The transfection reagent (HF) alone did not induce gene expression of CHOP (n = 3 donors). (F) HF alone did not induce the release of IL-6 (n = 3 donors). Data are presented as mean ± SEM relative to control, *p < 0.05, n = 4 (ANOVA with Tukey post hoc test).
Figure S4. The involvement of p38 pathway in IL-1β-induced expression of IL-6, IL-8, and COX-2, and protein release of PGE2. Intervertebral disc (IVD) cells were isolated from degenerated IVDs and treated with 10 ng/mL IL-1β alone or in combination with 10 µM small molecule inhibitor of p38 SB203580 (SB). (A–C) Combination of IL-1β and SB-reduced gene expression of IL-6, and COX2, but not IL-8. (D) Combination of IL-1β and SB-reduced protein release of PGE2 (n = 3). Data are presented as mean ± SEM, *p < 0.05 (ANOVA with Tukey post hoc test).
1. Pai S, Sundaram LJ. Low back pain: an economic assessment in the United States. Orthop Clin North Am (2004) 35:1–5. doi:10.1016/S0030-5898(03)00101-9
2. Morlion B. Pharmacotherapy of low back pain: targeting nociceptive and neuropathic pain components. Curr Med Res Opin (2011) 27:11–33. doi:10.1185/03007995.2010.534446
3. van den Eerenbeemt KD, Ostelo RW, van Royen BJ, Peul WC, van Tulder MW. Total disc replacement surgery for symptomatic degenerative lumbar disc disease: a systematic review of the literature. Eur Spine J (2010) 19:1262–80. doi:10.1007/s00586-010-1445-3
4. Taher F, Essig D, Lebl DR, Hughes AP, Sama AA, Cammisa FP, et al. Lumbar degenerative disc disease: current and future concepts of diagnosis and management. Adv Orthop (2012) 2012:970752. doi:10.1155/2012/970752
5. Suzuki S, Fujita N, Hosogane N, Watanabe K, Ishii K, Toyama Y, et al. Excessive reactive oxygen species are therapeutic targets for intervertebral disc degeneration. Arthritis Res Ther (2015) 17:316. doi:10.1186/s13075-015-0834-8
6. Le Maitre CL, Hoyland JA, Freemont AJ. Catabolic cytokine expression in degenerate and herniated human intervertebral discs: IL-1 beta and TNF alpha expression profile. Arthritis Res Ther (2007) 9:R77. doi:10.1186/ar2275
7. Le Maitre CL, Freemont AJ, Hoyland JA. Accelerated cellular senescence in degenerate intervertebral discs: a possible role in the pathogenesis of intervertebral disc degeneration. Arthritis Res Ther (2007) 9:R45. doi:10.1186/ar2198
8. Galbusera F, van Rijsbergen M, Ito K, Huyghe JM, Brayda-Bruno M, Wilke HJ. Ageing and degenerative changes of the intervertebral disc and their impact on spinal flexibility. Eur Spine J (2014) 23(Suppl 3):S324–32. doi:10.1007/s00586-014-3203-4
9. Neogi T. The epidemiology and impact of pain in osteoarthritis, osteoarthritis and cartilage/ OARS. Osteoarthritis Res Soc (2013) 21:1145–53. doi:10.1016/j.joca.2013.03.018
10. Molinos M, Almeida CR, Caldeira J, Cunha C, Goncalves RM, Barbosa MA. Inflammation in intervertebral disc degeneration and regeneration. J R Soc Interface (2015) 12:20141191. doi:10.1098/rsif.2014.1191
11. Le Maitre CL, Freemont AJ, Hoyland JA. The role of interleukin-1 in the pathogenesis of human intervertebral disc degeneration. Arthritis Res Ther (2005) 7:R732–45. doi:10.1186/ar1732
12. Weiler C, Nerlich AG, Bachmeier BE, Boos N. Expression and distribution of tumor necrosis factor alpha in human lumbar intervertebral discs: a study in surgical specimen and autopsy controls. Spine (2005) 30:44–53. doi:10.1097/01.brs.0000149186.63457.20
13. Sadowska A, Touli E, Hitzl W, Greutert H, Ferguson SJ, Wuertz-Kozak K, et al. Inflammaging in cervical and lumbar degenerated intervertebral discs: analysis of proinflammatory cytokine and TRP channel expression. Eur Spine J (2018) 27(3):564–77. doi:10.1007/s00586-017-5360-8
14. Kawasaki N, Asada R, Saito A, Kanemoto S, Imaizumi K. Obesity-induced endoplasmic reticulum stress causes chronic inflammation in adipose tissue. Sci Rep (2012) 2:799. doi:10.1038/srep00799
15. Dandekar A, Mendez R, Zhang K. Cross talk between ER stress, oxidative stress, and inflammation in health and disease. Methods Mol Biol (2015) 1292:205–14. doi:10.1007/978-1-4939-2522-3_15
16. Sprenkle NT, Sims SG, Sanchez CL, Meares GP. Endoplasmic reticulum stress and inflammation in the central nervous system. Mol Neurodegener (2017) 12:42. doi:10.1186/s13024-017-0183-y
17. Lee AS. The ER chaperone and signaling regulator GRP78/BiP as a monitor of endoplasmic reticulum stress. Methods (2005) 35:373–81. doi:10.1016/j.ymeth.2004.10.010
18. Tameire F, Verginadis II, Koumenis C. Cell intrinsic and extrinsic activators of the unfolded protein response in cancer: mechanisms and targets for therapy. Semin Cancer Biol (2015) 33:3–15. doi:10.1016/j.semcancer.2015.04.002
19. Zhao CQ, Zhang YH, Jiang SD, Jiang LS, Dai LY. Both endoplasmic reticulum and mitochondria are involved in disc cell apoptosis and intervertebral disc degeneration in rats. Age (2010) 32:161–77. doi:10.1007/s11357-009-9121-4
20. Fujii T, Fujita N, Suzuki S, Tsuji T, Takaki T, Umezawa K, et al. The unfolded protein response mediated by PERK is casually related to the pathogenesis of intervertebral disc degeneration. J Orthop Res (2017) 36(5):1334–45. doi:10.1002/jor.23787
21. Chooi WH, Chan BP. Compression loading-induced stress responses in intervertebral disc cells encapsulated in 3D collagen constructs. Sci Rep (2016) 6:26449. doi:10.1038/srep26449
22. Xie ZY, Chen L, Wang F, Liu L, Zhang C, Wang K, et al. Endoplasmic reticulum stress is involved in nucleus pulposus degeneration and attenuates low pH-induced apoptosis of rat nucleus pulposus cells. DNA Cell Biol (2017) 36:627–37. doi:10.1089/dna.2017.3736
23. Vonk LA, Doulabi BZ, Huang CL, Helder MN, Everts V, Bank RA. Endoplasmic reticulum stress inhibits collagen synthesis independent of collagen-modifying enzymes in different chondrocyte populations and dermal fibroblasts. Biochem Cell Biol (2010) 88:539–52. doi:10.1139/o09-174
24. Molinos M, Almeida CR, Caldeira J, Cunha C, Goncalves RM, Barbosa MA. Inflammation in intervertebral disc degeneration and regeneration. J R Soc Interface (2015) 12:20150429. doi:10.1098/rsif.2015.0429
25. Wuertz K, Haglund L. Inflammatory mediators in intervertebral disk degeneration and discogenic pain. Global Spine J (2013) 3(3):175–84. doi:10.1055/s-0033-1347299
26. Pfirrmann CWA, Metzdorf A, Zanetti M, Hodler J, Boos N. Magnetic resonance classification of lumbar intervertebral disc degeneration. Spine (2001) 26:1873–8. doi:10.1097/00007632-200109010-00011
27. Pritchard S, Erickson GR, Guilak F. Hyperosmotically induced volume change and calcium signaling in intervertebral disk cells: the role of the actin cytoskeleton. Biophys J (2002) 83:2502–10. doi:10.1016/S0006-3495(02)75261-2
28. Paschen W, Doutheil J, Gissel C, Treiman M. Depletion of neuronal endoplasmic reticulum calcium stores by thapsigargin: effect on protein synthesis. J Neurochem (1996) 67:1735–43. doi:10.1046/j.1471-4159.1996.67041735.x
29. Szegezdi E, Logue SE, Gorman AM, Samali A. Mediators of endoplasmic reticulum stress-induced apoptosis. EMBO Rep (2006) 7:880–5. doi:10.1038/sj.embor.7400779
30. Nishitoh H. CHOP is a multifunctional transcription factor in the ER stress response. J Biochem (2012) 151:217–9. doi:10.1093/jb/mvr143
31. Studer RK, Gilbertson LG, Georgescu H, Sowa G, Vo N, Kang JD. p38 MAPK inhibition modulates rabbit nucleus pulposus cell response to IL-1. J Orthop Res (2008) 26:991–8. doi:10.1002/jor.20604
32. Sutovsky J, Benco M, Sutovska M, Kocmalova M, Pappova L, Miklusica J, et al. Cytokine and chemokine profile changes in patients with lower segment lumbar degenerative spondylolisthesis. Int J Surg (2017) 43:163–70. doi:10.1016/j.ijsu.2017.06.024
33. Ozaktay AC, Kallakuri S, Takebayashi T, Cavanaugh JM, Asik I, DeLeo JA, et al. Effects of interleukin-1 beta, interleukin-6, and tumor necrosis factor on sensitivity of dorsal root ganglion and peripheral receptive fields in rats. Eur Spine J (2006) 15:1529–37. doi:10.1007/s00586-005-0058-8
34. Zhang YH, Zhao CQ, Jiang LS, Dai LY. Cyclic stretch-induced apoptosis in rat annulus fibrosus cells is mediated in part by endoplasmic reticulum stress through nitric oxide production. Eur Spine J (2011) 20:1233–43. doi:10.1007/s00586-011-1718-5
35. Kharroubi I, Ladriere L, Cardozo AK, Dogusan Z, Cnop M, Eizirik DL. Free fatty acids and cytokines induce pancreatic beta-cell apoptosis by different mechanisms: role of nuclear factor-kappaB and endoplasmic reticulum stress. Endocrinology (2004) 145:5087–96. doi:10.1210/en.2004-0478
36. Chen L, Liu L, Xie ZY, Wang F, Sinkemani A, Zhang C, et al. Endoplasmic reticulum stress facilitates the survival and proliferation of nucleus pulposus cells in TNF-alpha stimulus by activating unfolded protein response. DNA Cell Biol (2018) 37(4):347–58. doi:10.1089/dna.2017.4029
37. Xu DL, Jin HM, Wen JX, Chen JX, Chen DH, Cai NY, et al. Hydrogen sulfide protects against endoplasmic reticulum stress and mitochondrial injury in nucleus pulposus cells and ameliorates intervertebral disc degeneration. Pharmacol Res (2017) 117:357–69. doi:10.1016/j.phrs.2017.01.005
38. Kim JY, Choi JA, Kim TH, Yoo YD, Kim JI, Lee YJ, et al. Involvement of p38 mitogen-activated protein kinase in the cell growth inhibition by sodium arsenite. J Cell Physiol (2002) 190:29–37. doi:10.1002/jcp.10049
39. Cai BB, Chang SH, Becker EBE, Bonni A, Xia ZG. p38 MAP kinase mediates apoptosis through phosphorylation of Bim(EL) at Ser-65. J Biol Chem (2006) 281:25215–22. doi:10.1074/jbc.M512627200
40. Kim DS, Kim JH, Lee GH, Kim HT, Lim JM, Chae SW, et al. p38 mitogen-activated protein kinase is involved in endoplasmic reticulum stress-induced cell death and autophagy in human gingival fibroblasts. Biol Pharm Bull (2010) 33:545–9. doi:10.1248/bpb.33.545
41. Li Y, Schwabe RF, DeVries-Seimon T, Yao PM, Gerbod-Giannone MC, Tall AR, et al. Free cholesterol-loaded macrophages are an abundant source of tumor necrosis factor-alpha and interleukin-6: model of NF-kappaB- and map kinase-dependent inflammation in advanced atherosclerosis. J Biol Chem (2005) 280:21763–72. doi:10.1074/jbc.M501759200
42. Wang XZ, Ron D. Stress-induced phosphorylation and activation of the transcription factor CHOP (GADD153) by p38 MAP kinase. Science (1996) 272:1347–9. doi:10.1126/science.272.5266.1347
43. Gotoh T, Endo M, Oike Y. Endoplasmic reticulum stress-related inflammation and cardiovascular diseases. Int J Inflamm (2011) 2011:8. doi:10.4061/2011/259462
44. Luo S, Lee AS. Requirement of the p38 mitogen-activated protein kinase signalling pathway for the induction of the 78 kDa glucose-regulated protein/immunoglobulin heavy-chain binding protein by azetidine stress: activating transcription factor 6 as a target for stress-induced phosphorylation. Biochem J (2002) 366:787–95. doi:10.1042/BJ20011802
45. Zhong Y, Li J, Chen Y, Wang JJ, Ratan R, Zhang SX. Activation of endoplasmic reticulum stress by hyperglycemia is essential for Muller cell-derived inflammatory cytokine production in diabetes. Diabetes (2012) 61:492–504. doi:10.2337/db11-0315
46. Hu P, Han Z, Couvillon AD, Kaufman RJ, Exton JH. Autocrine tumor necrosis factor alpha links endoplasmic reticulum stress to the membrane death receptor pathway through IRE1alpha-mediated NF-kappaB activation and down-regulation of TRAF2 expression. Mol Cell Biol (2006) 26:3071–84. doi:10.1128/MCB.26.8.3071-3084.2006
47. Li J, Holbrook NJ. Elevated gadd153/chop expression and enhanced c-Jun N-terminal protein kinase activation sensitizes aged cells to ER stress. Exp Gerontol (2004) 39:735–44. doi:10.1016/j.exger.2004.02.008
48. Tan YF, Dourdin N, Wu C, De Veyra T, Elce JS, Greer PA. Ubiquitous calpains promote caspase-12 and JNK activation during endoplasmic reticulum stress-induced apoptosis. J Biol Chem (2006) 281:16016–24. doi:10.1074/jbc.M601299200
49. Nguyen DT, Kebache S, Fazel A, Wong HN, Jenna S, Emadali A, et al. Nck-dependent activation of extracellular signal-regulated kinase-1 and regulation of cell survival during endoplasmic reticulum stress. Mol Biol Cell (2004) 15:4248–60. doi:10.1091/mbc.e03-11-0851
50. Darling NJ, Cook SJ. The role of MAPK signalling pathways in the response to endoplasmic reticulum stress. Biochim Biophys Acta (2014) 1843:2150–63. doi:10.1016/j.bbamcr.2014.01.009
51. Endo M, Mori M, Akira S, Gotoh T. C/EBP homologous protein (CHOP) is crucial for the induction of caspase-11 and the pathogenesis of lipopolysaccharide-induced inflammation. J Immunol (2006) 176:6245–53. doi:10.4049/jimmunol.176.10.6245
52. McCullough KD, Martindale JL, Klotz LO, Aw TY, Holbrook NJ. Gadd153 sensitizes cells to endoplasmic reticulum stress by down-regulating Bcl2 and perturbing the cellular redox state. Mol Cell Biol (2001) 21:1249–59. doi:10.1128/MCB.21.4.1249-1259.2001
53. Song BB, Scheuner D, Ron D, Pennathur S, Kaufman RJ. Chop deletion reduces oxidative stress, improves beta cell function, and promotes cell survival in multiple mouse models of diabetes. J Clin Invest (2008) 118:3378–89. doi:10.1172/JCI34587
54. Sakai D, Andersson GB. Stem cell therapy for intervertebral disc regeneration: obstacles and solutions. Nat Rev Rheumatol (2015) 11:243–56. doi:10.1038/nrrheum.2015.13
55. Cortez L, Sim V. The therapeutic potential of chemical chaperones in protein folding diseases. Prion (2014) 8(2):197–202. doi:10.4161/pri.28938
56. Rani S, Sreenivasaiah PK, Kim JO, Lee MY, Kang WS, Kim YS, et al. Tauroursodeoxycholic acid (TUDCA) attenuates pressure overload-induced cardiac remodeling by reducing endoplasmic reticulum stress. PLoS One (2017) 12:e0176071. doi:10.1371/journal.pone.0176071
57. Lee YY, Hong SH, Lee YJ, Chung SS, Jung HS, Park SG, et al. Tauroursodeoxycholate (TUDCA), chemical chaperone, enhances function of islets by reducing ER stress. Biochem Biophys Res Commun (2010) 397:735–9. doi:10.1016/j.bbrc.2010.06.022
Keywords: intervertebral disc, inflammation, endoplasmic reticulum stress, p38 MAPK, CHOP, GADD153, GRP78, IL-6
Citation: Krupkova O, Sadowska A, Kameda T, Hitzl W, Hausmann ON, Klasen J and Wuertz-Kozak K (2018) p38 MAPK Facilitates Crosstalk Between Endoplasmic Reticulum Stress and IL-6 Release in the Intervertebral Disc. Front. Immunol. 9:1706. doi: 10.3389/fimmu.2018.01706
Received: 27 April 2018; Accepted: 10 July 2018;
Published: 17 August 2018
Edited by:
Rudolf Lucas, Augusta University, United StatesReviewed by:
Ranjan KC, Cleveland Clinic Lerner College of Medicine, United StatesCopyright: © 2018 Krupkova, Sadowska, Kameda, Hitzl, Hausmann, Klasen and Wuertz-Kozak. This is an open-access article distributed under the terms of the Creative Commons Attribution License (CC BY). The use, distribution or reproduction in other forums is permitted, provided the original author(s) and the copyright owner(s) are credited and that the original publication in this journal is cited, in accordance with accepted academic practice. No use, distribution or reproduction is permitted which does not comply with these terms.
*Correspondence: Olga Krupkova, b2tydXBrb3ZhQGV0aHouY2g=
Disclaimer: All claims expressed in this article are solely those of the authors and do not necessarily represent those of their affiliated organizations, or those of the publisher, the editors and the reviewers. Any product that may be evaluated in this article or claim that may be made by its manufacturer is not guaranteed or endorsed by the publisher.
Research integrity at Frontiers
Learn more about the work of our research integrity team to safeguard the quality of each article we publish.