- 1Animal Nutrition Institute, Sichuan Agricultural University, Chengdu, China
- 2Key Laboratory of Animal Disease-Resistance Nutrition, Chinese Ministry of Education, Chengdu, China
- 3College of Animal Science, Anhui Science and Technology University, Fengyang, China
- 4Department of Animal Science, Oklahoma State University, Stillwater, OK, United States
Rotavirus (RV) infection is one of the main pathogenic causes of severe gastroenteritis and diarrhea in infants and young animals. This study aimed to determine how dietary l-isoleucine supplementation improves the growth performance and immune response in weaned piglets with RV infection. In cell culture experiment, after IPEC-J2 and 3D4/31 cells were treated by 8 mM l-isoleucine for 24 h, the gene expressions of β-defensins and pattern recognition receptors (PRR) signaling pathway were significantly increased. Then, in the in vivo experiment, 28 crossbred weaned pigs were randomly divided into two groups fed with basal diet with or without l-isoleucine for 18 days. On the 15th day, the oral RV gavage was executed in the half of piglets. Average daily feed intake and gain of piglets were impaired by RV infection (P < 0.05). RV infection also induced severe diarrhea and the increasing serum urea nitrogen concentration (P < 0.05), and decreased CD4+ lymphocyte and CD4+/CD8+ ratio of peripheral blood (P < 0.05). However, dietary l-isoleucine supplementation attenuated diarrhea and decreasing growth performance (P < 0.05), decreased the NSP4 concentration in ileal mucosa, and enhanced the productions and/or expressions of immunoglobulins, RV antibody, cytokines, and β-defensins in serum, ileum, and/or mesenteric lymph nodes of weaned piglets (P < 0.05), which could be relative with activation of PRR signaling pathway and the related signaling pathway (P < 0.05) in the weaned pigs orally infused by RV. These results indicate that dietary l-isoleucine could improve the growth performance and immune function, which could be derived from l-isoleucine treatment improving the innate and adaptive immune responses via activation of PRR signaling pathway in RV-infected piglets. It is possible that l-isoleucine can be used in the therapy of RV infection in infants and young animals.
Introduction
Rotavirus (RV), a double-strained RNA (dsRNA) virus of the family Reoviridae, is the main cause of severe diarrhea hospitalization among infants, and causing ~527,000 deaths per year worldwide (especially developing country) (1–3). The previous study revealed that the replication of RV was restricted in the interferon (IFN)-treated cells (4), which indicates that IFN may play a vital role in antiviral immune response. RV replication is recognized by pattern recognition receptors (PRRs), such as toll-like receptor 3 (TLR3), retinoic acid inducible gene I (RIG-I), and/or melanoma differentiation-associated protein 5 (MDA5), and then activates the interferon regulatory factor 3 (IRF3) and nuclear factor-kappa B (NF-κB) that lead to the expression of type I IFN and pro-inflammation cytokines (5, 6). However, during the initial stage of RV infection, RV may suppress the expression of type I IFN and pro-inflammation cytokines through stimulating the proteasome-mediated degradation of IRF3 and NF-κB (4, 7, 8). These may impair the antiviral immune response and lead to a severe gastroenteritis.
Isoleucine is a kind of branched chain amino acid, which is essential for some physiological functions of humans and pigs (7, 9–11). Burns (9) has shown that isoleucine will specifically be incorporated into proteins of immune cells, such as lymphocytes, eosinophils, and neutrophils. And, in humans and animals, isoleucine plays a critical role for immune functions, including maintaining the development of immune organs and cells, and stimulating the secretion of immune molecules substances (12–16). l-Isoleucine administration can improve the health status of cells and animals under some challenge conditions (17, 18). In recent clinical study, dietary l-isoleucine supplementation can relieve the acute diarrhea induced by the malnourishment in children, which is related to the production of host defense peptides induced by isoleucine (19). Thus, isoleucine has the capacity of preventing the invasion of pathogens via the increase of immunity.
The aim of this study was to verify the hypothesis that supplementing l-isoleucine in diets might alleviate the effect of RV infection on growth and diarrhea in piglets, and analyze the possible change and mechanism of immune response during this process. We wanted to research the effect of isoleucine on gut. In the gut, there are including many types of cells, such as epithelial cells and immune cells. IPEC-J2 is a kind of porcine intestinal epithelial cell line. However, we chose to culture alveolar macrophage (3D4/31) that is a kind of porcine immune cell line in the cell culture experiment, which was used to evaluate the effect of l-isoleucine on immune cell. Previous studies have shown that there is the resemblance between humans and pigs (including gut function and immunity) (20), so pigs are usually considered as a research model for humans. Moreover, piglets are susceptible to heterotypic RV infection that leads to the serious diarrhea (21), which is similar to infants and young children. Thus, piglets are an ideal animal model on studying the effect of RV infection on immune response in vivo.
Materials and Methods
Cell Culture Experiment
Materials
Dulbecco’s Modified Eagle’s Medium/F12 (DMEM/F12) medium, Roswell Park Memorial Institute (RPMI) 1640 medium, and insulin–transferrin–selenium (ITS) solution were purchased from Lonza (Walkersville, MD, USA). Antibiotics (penicillin–streptomycin solution), trypsin–EDTA solution, and fetal bovine serum (FBS) were from Atlanta Biologicals (Flowery Branch, GA, USA). Sodium pyruvate solution was purchased from Hyclone Laboratories Inc. (Logan, UT, USA). RNAzol RT was from Molecular Research Center (Cincinnati, OH, USA). The iScript™ complementary DNA (cDNA) Synthesis Kit and iTaq™ Universal SYBR Green Supermix were purchased from Bio-Rad Laboratories, Inc. (Hercules, CA, USA). The genes included TLR3, retinoic-acid-inducible protein 1 (RIG-I), IRF3, mitochondrial antiviral-signaling protein (MAVS), MDA5, NF-κB, interferon beta (IFNβ), IFNγ, porcine beta-defensin 2 (pBD2), and pBD3. The primers (Table S1 in Supplementary Material) were from Integrated DNA Technologies, Inc. (Coralville, IA, USA).
Cell Culture
The IPEC-J2 cell line (porcine intestinal epithelial cells) and 3D4/31 cell line (porcine lung alveolar macrophage cells) were kindly provided by Dr. Guolong Zhang at Oklahoma State University (Stillwater, OK, USA). Both cells were cultured as described previously with some modifications (22). Briefly, IPEC-J2 cells were cultured in 6-well plates at 2.5 × 105 cells/well with DMEM/F12 medium added 10% FBS, 1% antibiotics, and 0.2% ITS. 3D4/31 cells were cultured in 6-well plates at 2.5 × 105 cells/well with RPMI 1640 medium added 10% FBS, 1% antibiotics, and 1 mM sodium pyruvate. According to some previous studies, different doses (0.4–10 mM) of isoleucine can affect the physiological functions in different cells (10, 23–25). Combining with our pre-experiments, we found that 8 mM of isoleucine can regulate the mRNA expressions of some genes (data no published). Thus, 8 mM was used as the treating dose in this experiment. Following 16–20 h culture, cells were treated by 8 mM l-isoleucine for 24 h (n = 6).
RNA Isolation and Quantitative Real-Time PCR
The RNA isolation and quantitative real-time PCR were performed by described previously with some modifications (22). Following l-isoleucine treatment, the RNA of cells was extracted by using RNAzol according to the manufacturer’s instruction. The RNA concentrations of all samples were analyzed by Nanodrop (Thermo Scientific, Wilmington, DE, USA). The first strand of cDNA was synthesized with iScript™ cDNA Synthesis Kit according to the manufacturer’s instruction. And then, in MyiQ Real-Time PCR System (Bio-Rad), quantitative real-time PCR was performed with iTaq™ Universal SYBR Green Supermix as described previously. Cycling conditions were as follows: 95°C for 30 s, and 40 cycles involving a combination of 94°C for 5 s and 60°C for 30 s. The gene-specific primers used in this study are listed in Table S1 in Supplementary Material (22, 26). Relative gene expression to the reference gene (β-actin) was performed and calculated according to the ΔΔCt method as described previously (22).
Animal Experiment
Animals, Diets, and Experimental Design
The experimental protocol was approved by Animal Care Advisory Committee of Sichuan Agricultural University. All the test operations were executed with documents to Regulations on Animal Welfare and Animal Testing and carried out at the Experimental Farm of Sichuan Agricultural University. The animal Ethics Committee approval number is CD-SYXK-2017-015. Twenty-eight DLY (Duroc × Landrace × Yorkshire) weaned barrows (6.95 ± 0.41 kg) were fed in the metabolic cages (1.5 m × 0.7 m × 1.0 m) with a self-feeder and a nipple watering device, which were located in a temperature-controlled room.
The basal diet was formulated to meet National Research Council-recommended nutrient requirements (27) for pigs (7–11 kg) (28). And all the diets were prepared at the Experimental Farm of Sichuan Agricultural University. The composition and nutrient content of basal diet were presented in Table 1. Either 1% (w/w) l-isoleucine or 0.68% (w/w) l-alanine (isonitrogenous control) was added to the basal diet through replacing the corn starch.
Rotavirus used in this study was a tissue culture-adapted Ohio State University strain (ATCC#VR-893). RV preparation and virus titer determination [tissue culture infective dose 50 (TCID50)] were determined as described previously (28, 29).
Following 3 days for orientation, piglets were divided into two groups on basis of body weight and litter origin and were fed with basal diet with 0.68% l-alanine (CON group, n = 14) or with 1% l-isoleucine (Ile group, n = 14) for 18 days. On 15th day, all piglets were infused 5 mL of sterile NaHCO3 solution (100 mmol/L). Then, half of piglets in two groups were orally administrated with 4 mL (106 TCID50/mL) of RV while the others were infused with 4 mL of the sterile medium. After RV treatment, the diarrhea state was recorded in each day. Fecal consistency was scored as follows: 0, normal; 1, pasty; 2, semiliquid; and 3, liquid. Piglets were considered as diarrhea when fecal consistency score is ≥2 (30). Average daily gain (ADG), average daily feed intake (ADFI), feed/gain ratio (F/G ratio), and gain (G) were calculated via weighing body weight and feed intake of all piglets at 08:00 of days 1, 15, and 19.
Tissue Sample Collection
The blood of pigs were gathered through the jugular vein, and 5 mL of whole blood was added in EDTA-coated tube for lymphocyte subtypes assay, then the residual blood was used to separate serum sample by centrifugation at 3,500 g for 15 min. The isolated serum samples were stored at −20°C until analysis. Following sample collection, all piglets were euthanized via intracardially injecting Na pentobarbital (50 mg/kg of body weight) and exsanguinated. The abdomen was immediately opened. The mucosa of mid ileum and mesenteric lymph nodes were removed, flushed with ice-cold saline solution, frozen in liquid nitrogen, and then stored at −80°C until analysis.
Serum Urea Nitrogen (UN) and Amino Acid Assay
Serum UN concentration was analyzed with an assay kit from Nanjing Jiancheng Biochemistry Institute (Nanjing, China) according to the manufacturer’s instructions. Serum-free amino acid concentrations were determined by ion-exchange chromatography with physiological fluid analysis conditions (L-8900 AA Analyzer, Hitachi, Japan) as described previously (29).
Lymphocyte Subtype Assay
Premixed cocktail of monoclonal antibodies: CD3-PerCP, CD4-FITC, and CD8-PE (BD Biosciences, USA) were added into 100 µL of EDTA blood in a 12 mm × 75 mm tube. The tube was gently mixed and incubated at room temperature for 20 min in the dark, then added 2 mL of RBC lysing solution (BD Biosciences, USA) and incubated for another 10 min. Centrifugation at 500 g for 5 min and subsequently washed with 2 mL of PBS, the cell pellets were resuspended in 0.5 mL of 1% paraformaldehyde in PBS. The stained cells were then analyzed by a flow cytometer (FACSverse, BD Biosciences, USA), using CellQuest software program (BD Biosciences, USA). The percentage of CD3+, CD4+, and CD8+ lymphocytes and CD4+/CD8+ were determined from the CD3+/CD4+ and CD3+/CD8+ tubes, using FL1 and FL2 (31).
Serum and Ileal Cytokines Assay
Eighty mg of ileum was added into ice-cold PBS solution and executed the pulverization by using ultrasonic cell disruption system (Scientz-IID, Scientz, Ningbo, China) at 4°C, and then centrifuged at 3,500 g for 15 min at 4°C. The ileal supernatant and serum was used to detect the IFNβ, IFNγ, interleukin 1β (IL-1β), IL-10, and tumor necrosis factor α (TNF-α) concentrations with commercially available porcine enzyme-linked immunosorbent assay (ELISA) kits (Xinle Bio Co. Ltd., Shanghai, China) according to the manufacturer’s instructions.
Serum and Ileal NSP4, RV Antibody (RV-ab), and Immunoglobulin Assay
The concentration of RV-ab and NSP4 (non-structural protein) in serum and ileum were detected with a commercially available porcine ELISA kit (TSZ, Framingham, MA, USA) according to the manufacturer’s instructions. The concentrations of immunoglobulins (IgA, IgM, and IgG) in serum and secretory IgA (sIgA) in ileum were determined with available porcine ELISA kits (Xinle Bio Co. Ltd., Shanghai, China) according to the manufacturer’s instructions.
Total RNA Isolation and Real-Time Quantitative PCR
The materials and methods of total RNA isolation and quantitative real-time PCR are the same as the cell culture experiment. The genes included TLR3, RIG-I, MDA5, MAVS, toll-like receptor-associated activator of interferon (TRIF), TNF receptor associated factor 3 (TRAF3), NF-κB essential modulator (NEMO), transforming growth factor-β-activating kinase 1 (TAK1), IRF3, NF-κB, IL-1β, IL-10, TNF-α, IFNβ, IFNγ, PG1–5 pBD1, pBD2, and pBD3. The gene-specific primers used in this study are listed in Table S1 in Supplementary Material (22, 26). Relative gene expression to the reference gene (β-actin) was performed and calculated according to the ΔΔCt method as described previously (22).
Statistics Analysis
Cell Culture Experiment
In cell experiments, statistical analyses were performed with GraphPad PRISM 5 (GraphPad Software, La Jolla, CA, USA). Data were analyzed using unpaired Student’s two-tailed t test. Statistical significance was considered at P < 0.05.
Animal Experiment
In animal experiment, the growth performance data of all piglets before RV infusion were analyzed with the unpaired t test. The diarrhea rate data of all piglets were analyzed with chi-square test. The other data were analyzed as a 2 × 2 factorial with the general linear model procedures of the Statistical Analysis Package. The model factors included the effects of l-isoleucine administration, RV infection and their interaction. All data were analyzed with SPSS (Version 21.0; IBM, USA) and indicated as means with their SEs. P < 0.05 was considered statistical significance while P < 0.10 was considered statistical tendency.
Results
Cell Culture Experiment
l-Isoleucine Regulates the Expressions of Innate Immunity-Relative Genes in IPEC-J2 and 3D4/31 Cells
Recent studies have shown that isoleucine treatment can improve the immune functions of cells, animals and humans under normal and disease conditions (10, 14, 18, 19). To initially understand the effect of isoleucine on innate immunity, we measured the expressions of innate immunity-relative genes after isoleucine treatment in IPEC-J2 and 3D4/31 cells. And in this study, 8 mM l-isoleucine treatment significantly increased the expressions of TLR3, RIG-I, IRF3, MAVS, MDA5, NF-κB, IFNβ, IFNγ, pBD2, and pBD3 in IPEC-J2 cells (P < 0.05, Figure 1A) and significantly enhanced the expressions of TLR3, RIG-I, IRF3, MAVS, MDA5, NF-κB, IFNβ, and pBD2 in 3D4/31 cells (P < 0.05, Figure 1B).
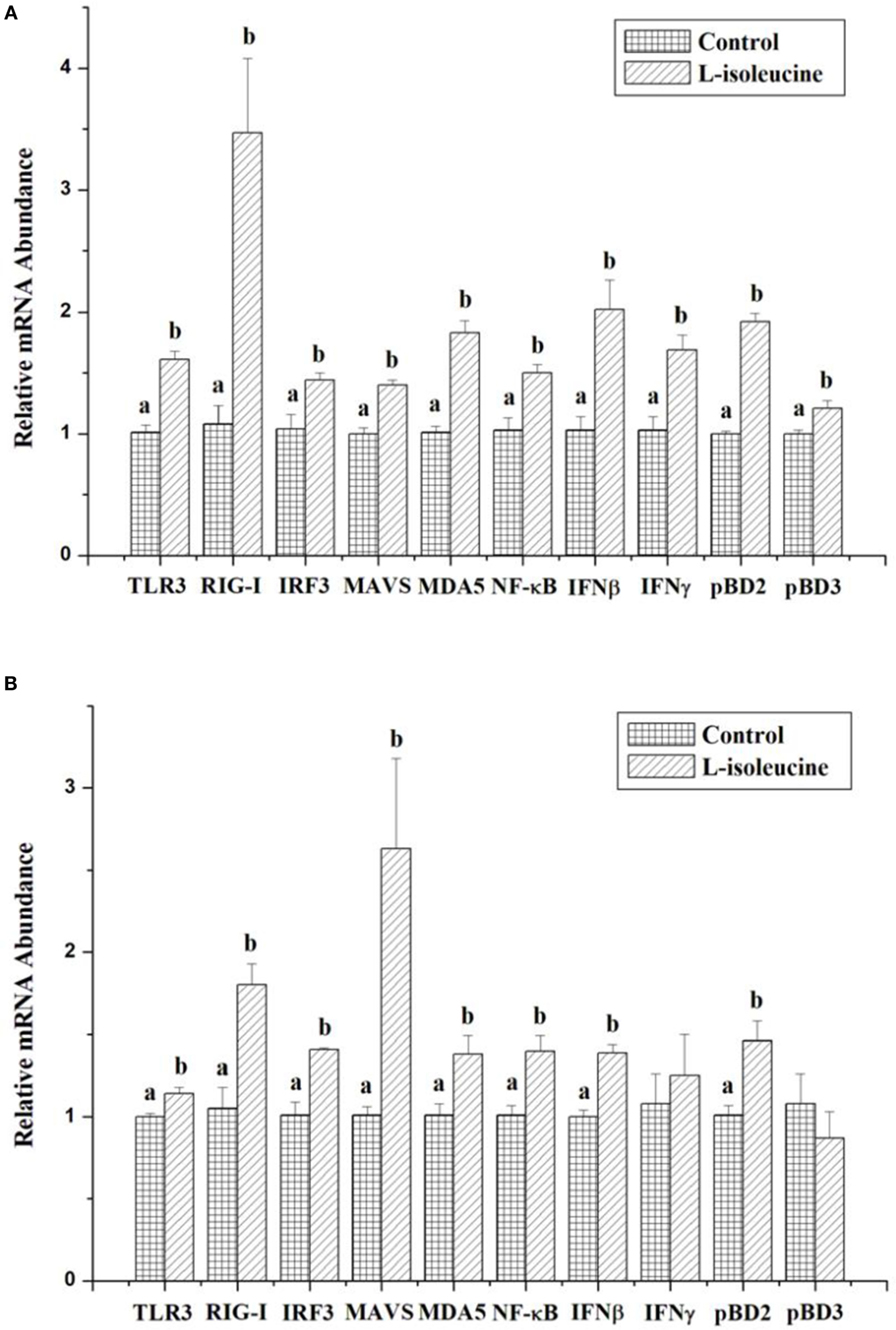
Figure 1. The effect of l-isoleucine treatment on the expressions of innate immunity-relative genes in IPEC-J2 (A) and 3D4/31 (B) cells. The relative mRNA abundance for the innate immunity-relative genes was normalized to that for β-actin. Values are means ± SEM; n = 6. Values with different letters are significantly different (P < 0.05).
Animal Experiment
Growth Performance
During entire trial period, there were not any unexpected deaths. Due to the RV infection, some piglets showed weight losing, and then G were calculated and analyzed instead of ADG and F/G ratio during 15–18 days. Dietary l-isoleucine supplementation increased ADG and ADFI and reduced F/G ratio of piglets in the first 14 days of trial (P < 0.05, Table 2). RV infusion reduced ADFI and G of weaned piglets (P < 0.05). But dietary l-isoleucine supplementation attenuated the effect of RV infection on ADFI and G in weaned piglets (P < 0.05).
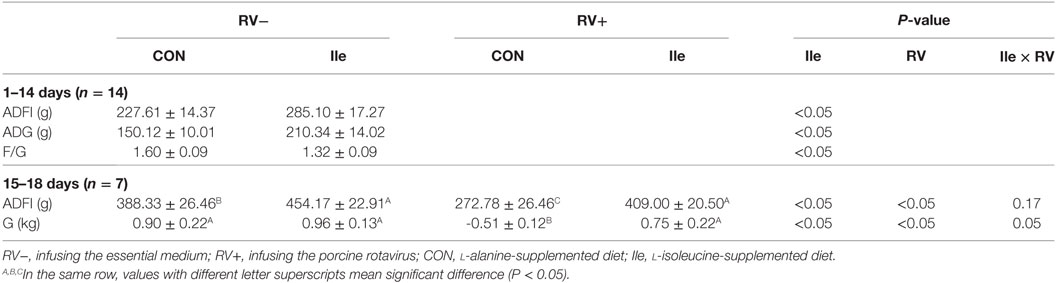
Table 2. The effect of dietary l-isoleucine supplementation and/or rotavirus (RV) challenge on the growth performance in the weaned pig.
Serum UN and Free AA Concentrations
Rotavirus infusion increased serum UN (P < 0.05), proline (P < 0.05), cysteine (P < 0.05), arginine (P < 0.05), serine (P = 0.05), glycine (P = 0.09), and alanine (P = 0.08) concentrations in weaned piglets (Table 3). Dietary l-isoleucine supplementation decreased serum UN (P < 0.05) and glycine (P = 0.06) concentrations, increased serum isoleucine (P < 0.05) and arginine (P = 0.06) concentrations, and attenuated the effect of RV infection on serum UN and glycine concentrations in weaned piglets (P < 0.05, Table 3).
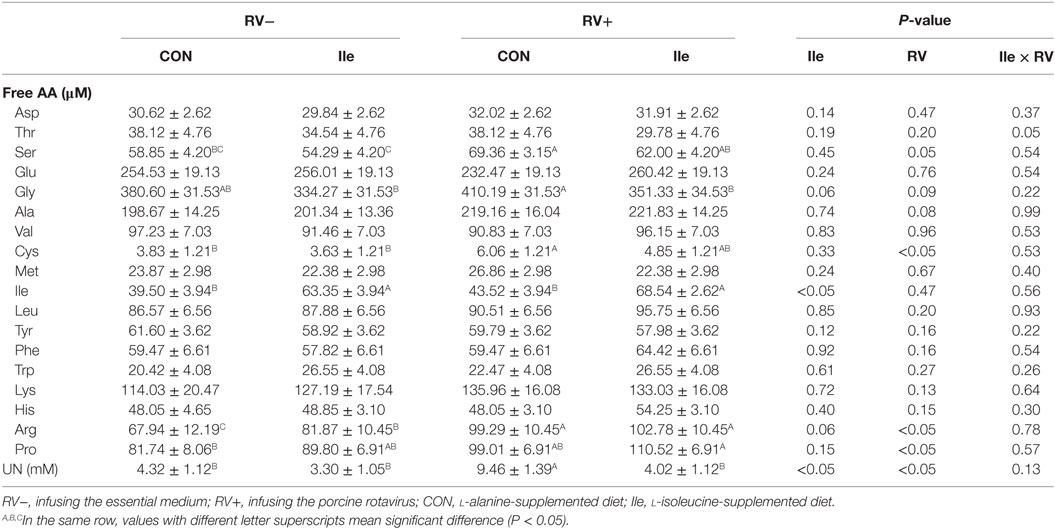
Table 3. The effect of dietary l-isoleucine supplementation and/or rotavirus (RV) challenge on the concentrations of amino acids and nitrogen in the serum of weaned pigs (n = 7).
Diarrhea Status, RV-ab, and NSP4 Concentration of Serum and Ileum
Following RV challenge, diarrhea rate was increased (Figure 2; Table 4), serum and ileal RV-ab and NSP4 concentrations were enhanced (P < 0.05, Table 5) in weaned piglets. Furthermore, in the weaned piglets challenged by RV, l-isoleucine administration attenuated the increasing NSP4 level (P < 0.05, Table 5), diarrhea rate, shortened the duration of diarrhea (Figure 2; Table 4), but further enhanced serum and ileal RV-Ab levels (P < 0.05, Table 5).
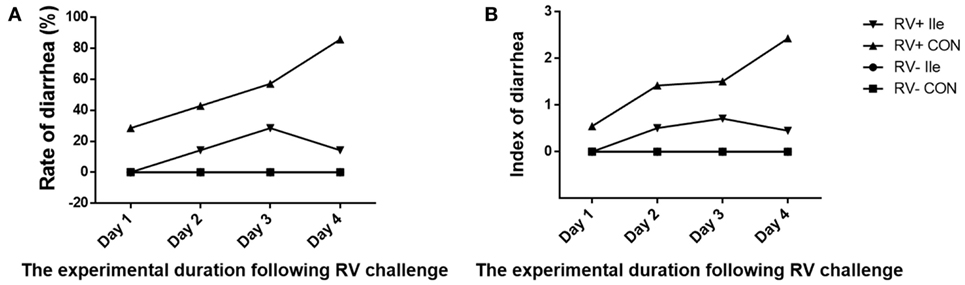
Figure 2. Dynamic variation of diarrhea rate (A) and index (B) of weaned piglets by adding l-Ile after RV challenged over time.
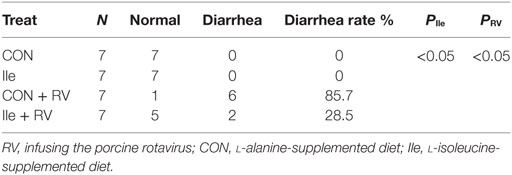
Table 4. The effect of dietary Ile supplementation and/or RV challenge on the diarrhea rate in weaned pigs.
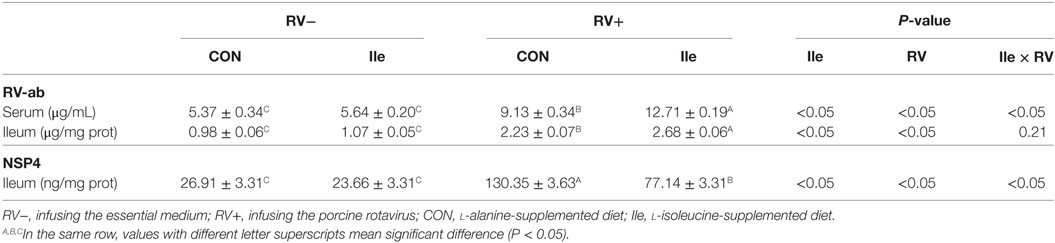
Table 5. The effect of dietary l-isoleucine supplementation and/or rotavirus (RV) challenge on the contents of RV antibody (RV-ab) and NSP4 in the ileum and/or serum of weaned pigs (n = 7).
Lymphocyte Subpopulation
In Table 6, the effect of RV challenge and l-isoleucine administration on lymphocyte subpopulation in the peripheral blood of weaned piglets was shown. RV infusion decreased CD4+ rate and CD4+/CD8+ ratio in the peripheral blood (P < 0.05). However, dietary l-isoleucine supplementation attenuated the effect of RV infection on CD4+ rate in the peripheral blood (P < 0.05).
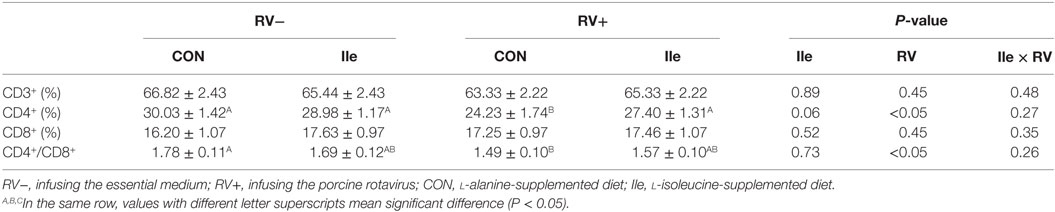
Table 6. The effect of dietary l-isoleucine supplementation and/or rotavirus (RV) challenge on the subgroup of lymphocytes in the serum in weaned pigs (n = 7).
Serum and Ileal Concentrations of Immunoglobulins and Cytokines
Rotavirus infusion increased the serum IgA, IgG, IFNβ, IFNγ, IL-1β, and TNF-α levels in weaned piglets (P < 0.05, Table 7). However, dietary l-isoleucine supplementation also enhanced the serum IgA, IgG, IFNβ, IFNγ, IL-1β, TNF-α, and IL-10 levels and further promoted serum concentrations of these immunoglobulins and cytokines in the weaned piglets infected by RV (P < 0.05, Table 7).
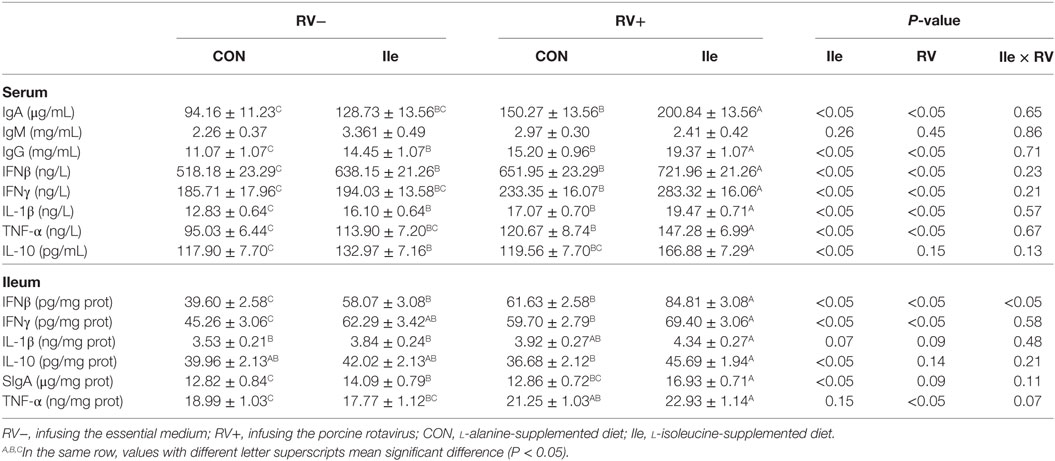
Table 7. The effect of dietary l-isoleucine supplementation and/or rotavirus (RV) challenge on immunoglobulin and cytokine levels in the serum and ileum of weaned pigs (n = 7).
Rotavirus infusion increased IFN-β (P < 0.05), IFN-γ (P < 0.05), TNF-α (P < 0.05), IL-1β (P = 0.08), and sIgA (P = 0.09) levels in the ileum of weaned piglets (Table 7). However, dietary l-isoleucine supplementation also enhanced the ileal IFNβ (P < 0.05), IFNγ (P < 0.05), IL-10 (P < 0.05), sIgA (P < 0.05), and IL-1β (P = 0.07) levels and further promoted IFNβ, IFNγ, IL-10 and sIgA concentrations in the ileum of weaned piglets infected by RV (P < 0.05, Table 7).
Gene Expressions of Cytokines in Ileum and Mesenteric Lymph Nodes
In the weaned piglets, RV infusion increased the mRNA expressions of IFNβ, IFNγ, IL-1β, and TNF-α in the ileum (P < 0.05, Table 8) and enhanced the mRNA expressions of IFNβ, IFNγ, IL-1β, IL-10, and TNF-α in the mesenteric lymph nodes (P < 0.05, Table 8). Supplementing l-isoleucine in diets also stimulated the mRNA expressions of IFN-β, IFN-γ, IL-10, IL-1β, and TNF-α in the ileum and mesenteric lymph nodes, although to different extents (P < 0.05 or P < 0.10, Table 8), and further enhanced the mRNA expressions of IFN-β, IFN-γ, IL-10, IL-1β, and/or TNF-α in the ileum and mesenteric lymph nodes of weaned piglets infected by RV (P < 0.05, Table 8).
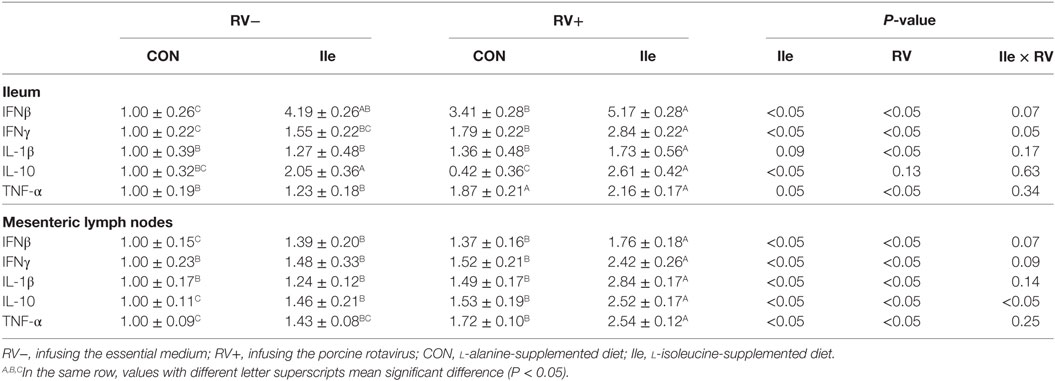
Table 8. The effect of dietary l-isoleucine supplementation and/or rotavirus (RV) challenge on gene expressions of cytokines in the ileum and mesenteric lymph nodes of weaned pigs (n = 7).
Expressions of Genes Related to Innate Immune in Ileum and Mesenteric Lymph Nodes
In the weaned piglets, RV infusion, to different degrees, increased the mRNA expressions of NF-κB, TLR3, RIG-I, MDA5, TRIF, TRAF3, NEMO, TAK1, IRF3, MAVS, pBD2, and pBD3 in the ileum and/or mesenteric lymph nodes (P < 0.05 or P < 0.10, Table 9). In addition, l-isoleucine administration also enhanced the mRNA expressions of these genes related to innate immune in the ileum and/or mesenteric lymph nodes, although to different extents (P < 0.05 or P < 0.10, Table 9), and further enhanced the mRNA expressions of these genes related to innate immune in the ileum and/or mesenteric lymph nodes of weaned piglets infected by RV (P < 0.05, Table 9).
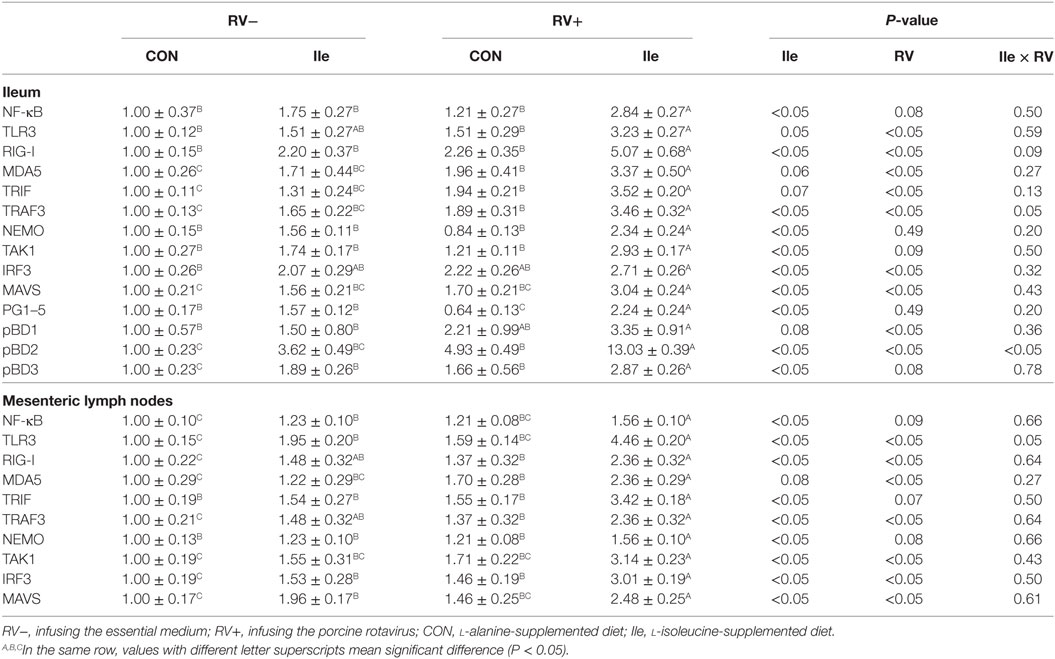
Table 9. The effect of dietary l-isoleucine supplementation and/or rotavirus (RV) challenge on expressions of genes related to innate immune in the ileum and mesenteric lymph nodes of weaned pigs (n = 7).
Discussion
As a function amino acid, isoleucine was well known as its effects on glucolipid metabolism and protein synthesis. But recently studies have shown that supplementing l-isoleucine can improve the health status and growth of human infants and young animals that were challenged by some pathogens (such as Escherichia coli) via improving the immune function (10, 14, 18, 19). l-Isoleucine treatment should improve the innate immunity possibly via some signaling pathways, such as TLRs, RIG-I, MAVS and MDA5, which can stimulate the generation and expression of anti-pathogen components. In this study, l-isoleucine treatment significantly stimulated the expressions of TLR3, RIG-I, MDA5, MAVS, IRF3, NF-κB, IFNβ, IFNγ, pBD2, and/or pBD3 in IPEC-J2 and 3D4/31 cells. These genes play the important role for preventing invasion of dsRNA viruses (including rotavirus). Therefore, l-isoleucine administration could alleviate the effect of rotavirus on humans and animals.
Rotavirus has been considered as a major pathogen causing severe gastroenteritis in infants and young children, as well as in other young animals (3, 32–35). Many previous study have reported that RV infection resulted in growth performance reduction, inflammation response, severe diarrhea, changes of cytokines and generation of RV-ab in piglets (15, 26, 29, 35–38), which were similar with this study. However, there was a few difference of RV infection affecting cytokines’ levels in serum and intestine between this study and our previous studies, which could be related to experimental duration and RV-infused dose. Besides, this study observed that RV infection enhanced the mRNA expression of genes related to innate immune in the ileum and/or mesenteric lymph nodes, increased some free amino acid concentrations in serum, and decreased the quantity of CD4+ and CD4+/CD8+ ratio in peripheral blood and the concentration of NSP4 in ileal mucosa. Therefore, it is proposed that RV infection model was successfully established.
Previous studied indicated that dietary l-isoleucine supplementation could promote growth of piglets, fattening pigs, laying hens and juvenile Jian carp (39–43). In this study, l-isoleucine administration could improve ADFI, ADG and F/G ratio of piglets before RV infusion and also enhanced ADFI and G under the condition of RV infection. In addition, dietary l-isoleucine supplementation could increase serum isoleucine and arginine levels, and decrease serum UN and glycine concentrations in the normal piglets, then assuaged the increasing serum UN and glycine concentrations in RV-infected piglets. Serum UN and free amino acid concentration can directly reflect the level of amino acid utilization and protein metabolism in the whole body (44, 45). These indicated that RV infection impaired protein metabolism, which could be alleviated by l-isoleucine administration. Therefore, dietary l-isoleucine supplementation enhanced the growth performance of the RV-infected piglets potentially through improvement of protein metabolism and utilization in whole body.
Immune function, including the humoral and cellular immune, is critical to obliterate the pathogens, which could keep the health and growth of animals and human. Various immunoglobulins (such as IgA, IgG and some specific antibodies) are the main constituents of humoral immune (46), in which sIgA is important to protect the gut mucosa from the pathogen invasion (47). In this study, we observe that supplementing l-isoleucine in diets increased serum IgA, IgG and RV-ab levels, and ileal sIgA and RV-ab concentrations in weaned piglets under normal and/or RV-infected conditions, which is similar to the results in different animals (15, 48, 49). It was possible that l-isoleucine elevate humoral immune of weaned piglets infected by RV via upregulating the immunoglobulin generation.
In this study, we also found that l-isoleucine treatment significantly attenuated the decrease of CD4+ T cells in the peripheral blood of RV-infected piglets. As a part of cellular immune, CD4+ T cells contribute a myriad of activities for protecting animals and human against the pathogens (50). Especially, CD4+ T cells are essential as helper to enhance antibody production and response in B cell (51). Thus, these could partially explain the effect of dietary l-isoleucine supplementation on generation of immunoglobulins and RV-ab.
Cytokines are the key in keeping cell-to-cell communication, and regulate lymphocyte differentiation and maturity, and subsequent functional activity of the peripheral immune compartment (52, 53). The cytokines balance (especially Th1/Th2) and levels are critical to immunity maintenance and inflammation control. Moreover, type I IFN and pro-inflammation cytokines can effectively prevent the invasion and replication of virus, including RV (5, 6). The previous studies have shown that l-isoleucine administration affects the concentration of IL-1β, IFNβ and IFNγ in serum and ileum of weaned piglets (18) and regulates IL-1β, IL-4, IFNβ, and IFNγ generation in human peripheral blood mononuclear cells (18, 54). This study also revealed that dietary l-isoleucine supplementation elevated serum and ileal IL-1β, IFNβ, IFNγ, TNF-α, and IL-10 levels and further promoted serum and ileal concentrations of these cytokines in RV-infected piglets. Although the different lymphocyte subpopulation can affect antiviral capacity via regulating the production and secretion of cytokines (50), l-isoleucine treatment increasing cytokines’ levels, especially in the ileum, could not completely be explained by the improvement of CD4+ T cells. Meanwhile, in the normal and RV-infected piglets, the mRNA expressions of IL-1β, IFNβ, IFN-γ, TNF-α, and IL-10 were also upregulated by l-isoleucine administration in the ileum and mesenteric lymph nodes that are targets of RV challenge.
Innate immunity is the first and universal defense mechanism of host against various pathogens such as bacteria and viruses (55). Defensins, as a kind of antimicrobial peptides produced by leukocytes and epithelial cells, play an important role in antiviral strategy via acting directly on virion and host cells (56). In accordance with many studies, we also found that l-isoleucine treatment enhanced the expression of pBD2 and/or pBD3 in the in vitro and in vivo experiments (10, 11). It is possible that l-isoleucine preventing the invasion of RV be relative with the increase of pBD2 and pBD3 production.
Host–pathogen interactions are generally started via host recognition of pathogen-associated molecular patterns (PAMPs) that are essential for the survival of pathogens (i.e., dsRNA of RV) (55, 57, 58). And these PAMPs are sensed by the host’s PRRs, including toll-like receptors, RIG-I-like receptors, NOD-like receptors and DNA receptors, which are expressed on innate immune cells (59, 60). Effective sensing of PAMPs rapidly induces host immune response via activating several signaling pathways (such as IRFs, NF-κB, and MAVS) that lead to the transcription and release of various cytokines, type I IFN and defensins, which subsequently enhances the eradication of pathogens (61). Moreover, among these PRRs, TLR3, RIG-I, and MDA5 have the property of recognizing the dsRNA-virus (i.e., RV) (6, 62).
Several previous studies determined that, following infection, RV could interact with IRF3 and NF-κB, and result in the proteasome-mediated degradation of IRF3 and NF-κB, and then suppress host’s normal immune antiviral immune response and cytokines secretion, which is one of the reasons that RV lead to a severe gastroenteritis (63, 64). This study also showed that dietary l-isoleucine supplementation enhanced the mRNA expressions of NF-κB, TLR3, RIG-I, MDA5, TRIF, TRAF3, NEMO, TAK1, IRF3, and MAVS in the ileum and/or mesenteric lymph nodes of normal and RV-infected piglets, which is consistent with the results of in vitro experiment. l-Isoleucine has the property of stimulating PRRs and the relative pathways, which will promote the transcription and generation of antiviral proteins, including cytokines and defensins. Therefore, supplementing l-isoleucine in diets relieving the diarrhea induced by RV challenge could be due to the activation of innate immunity before infection. Previous study indicated that NSP4 is the main cause of piglet diarrhea, and it is also a good marker of RV level in tissue (65). This study showed that dietary l-isoleucine supplementation decreases the NSP4 level in ileum of infected piglet. Thus, it is possible that dietary l-isoleucine supplementation decreased RV level in intestine through improving the immune function of infected piglets.
Previous study indicated that oral l-isoleucine solution tended to relieve the cute diarrhea induced by mixed pathogens (including RV) in children (19), and it is similar with our findings. This study can also explain how l-isoleucine relieved the diarrhea and infection induced by RV. In addition, l-isoleucine administration can also relieve E. coli infection and its impairment (41), but the recognition of E. coli was not associated with TLR3, RIG-I, and MDA5. Thus, the effect of isoleucine on other PRRs expression needs to be further studied.
In summary, RV infection impaired growth performance and immune response in weaned piglets. However, l-isoleucine supplementation relieved the negative effect of RV infection on growth performance and diarrhea of piglets, which could be due that l-isoleucine treatment improved the immune response, especially generation and secretion of antiviral proteins, via activating PRRs and the relative pathways. However, the further mechanisms of isoleucine regulating immunity also need to be studied in the future. The gastrointestinal and immune systems of resemble those of human infants, so piglets are an ideal animal model on studying RV infection and immune response. Thus, according to this study, we can propose that l-isoleucine may be also used in the prevention and therapy of infant RV infection.
Ethics Statement
This study was carried out in accordance with the recommendations of Animal Welfare Regulations, Animal Care Advisory Committee of Sichuan Agricultural University. The protocol was approved by the Animal Care Advisory Committee of Sichuan Agricultural University.
Author Contributions
Conceived and designed the experiments: DC, BY, JH, XM, GT, and MR. Performed the experiments: CG, QY, XM, and JY. Analyzed the data: JW, PZ, and JL. Contributed reagents/materials/analysis tools: JW and MR. Wrote the paper: CG and XM.
Conflict of Interest Statement
The authors declare that the research was conducted in the absence of any commercial or financial relationships that could be construed as a potential conflict of interest.
Acknowledgments
Special thanks to Professor De Wu of Sichuan Agricultural University for revising this manuscript.
Funding
This work was supported by the grant from the China Agriculture Research System (CARS-35), the grant from Major Project of Education Department of Sichuan Province (17ZA0311), the grant from Science and Technology Support Project of Sichuan Province (2016NYZ0052 and 2016NZ0006), the grant from National Natural Science Foundation of China (31501968), and the fund from the research program of “Sheng Yang” students’ association (B2016010).
Supplementary Material
The Supplementary Material for this article can be found online at https://www.frontiersin.org/articles/10.3389/fimmu.2018.01654/full#supplementary-material.
References
1. Parashar UD, Gibson CJ, Bresee JS, Glass RI. Rotavirus and severe childhood diarrhea. Emerg Infect Dis (2006) 12(2):304–6. doi:10.3201/eid1202.050006
2. Mwenda JM, Ntoto KM, Abebe A, Enweronularyea C, Amina I, Mchomvu J, et al. Burden and epidemiology of rotavirus diarrhea in selected African countries: preliminary results from the African rotavirus surveillance network. J Infect Dis (2010) 202(Suppl 1):S5. doi:10.1086/653557
3. Tate JE, Burton AH, Boschipinto C, Steele AD, Duque J, Parashar UD. 2008 estimate of worldwide rotavirus-associated mortality in children younger than 5 years before the introduction of universal rotavirus vaccination programmes: a systematic review and meta-analysis. Lancet Infect Dis (2012) 12(2):136–41. doi:10.1016/S1473-3099(11)70253-5
4. Barro M, Patton JT. Rotavirus NSP1 inhibits expression of type I interferon by antagonizing the function of interferon regulatory factors IRF3, IRF5, and IRF7. J Virol (2007) 81(9):4473–81. doi:10.1128/JVI.02498-06
5. Akira S, Takeda K. Toll-like receptor signalling. Nat Rev Immunol (2004) 4(7):499–511. doi:10.1038/nri1391
6. Broquet AH, Hirata Y, Mcallister CS, Kagnoff MF. RIG-I/MDA5/MAVS are required to signal a protective IFN response in rotavirus-infected intestinal epithelium. J Immunol (2011) 186(3):1618–26. doi:10.4049/jimmunol.1002862
7. Barro M, Patton JT. Rotavirus nonstructural protein 1 subverts innate immune response by inducing degradation of IFN regulatory factor 3. Proc Natl Acad Sci U S A (2005) 102(11):4114–9. doi:10.1073/pnas.0408376102
8. Di FI, Pane JA, Holloway G, Coulson BS. NSP1 of human rotaviruses commonly inhibits NF-κB signalling by inducing β-TrCP degradation. J Gen Virol (2015) 96(7):1768–76. doi:10.1099/vir.0.000093
9. Burns CP. Isoleucine metabolism by leukemic and normal human leukocytes in relation to cell maturity and type. Blood (1975) 45(5):643–51.
10. Konno Y, Ashida T, Inaba Y, Ito T, Tanabe H, Maemoto A, et al. Isoleucine, an essential amino acid, induces the expression of human defensin 2 through the activation of the G-protein coupled receptor-ERK pathway in the intestinal epithelia. Food Nutr Sci (2012) 3(4):548–55. doi:10.4236/fns.2012.34077
11. Ren M, Zhang S, Liu X, Li S, Mao X, Zeng X, et al. Differ LPS, branched-chain amino acids modulate porcine intestinal endogenous β-defensin expression through Sirt1/ERK/90RSK pathway. J Agric Food Chem (2016) 64(17):3371–9. doi:10.1021/acs.jafc.6b00968
12. Chuang JC, Yu CL, Wang SR. Modulation of human lymphocyte proliferation by amino acids. Clin Exp Immunol (1990) 81(1):173–6. doi:10.1111/j.1365-2249.1990.tb05310.x
13. Hale LL, Pharr GT, Burgess SC, Corzo A, Kidd MT. Isoleucine needs of thirty- to forty-day-old female chickens: immunity. Poult Sci (2004) 83(12):1979–85. doi:10.1093/ps/83.12.1979
14. Rivas-Santiago CE, Rivas-Santiago B, León DA, Castañeda-Delgado J, Hernández PR. Induction of β-defensins by l-isoleucine as novel immunotherapy in experimental murine tuberculosis. Clin Exp Immunol (2011) 164(1):80–9. doi:10.1111/j.1365-2249.2010.04313.x
15. Zhao J, Feng L, Liu Y, Jiang W, Wu P, Jiang J, et al. Effect of dietary isoleucine on the immunity, antioxidant status, tight junctions and microflora in the intestine of juvenile Jian carp (Cyprinus carpio var. Jian). Fish Shellfish Immunol (2014) 41(2):663–73. doi:10.1016/j.fsi.2014.10.002
16. Gong BS, Ren M, Jin EH, Qian-Qian LI, Xiu-Rong LI, Sheng-He LI. Effect of isoleucine on the serum antioxidant levels and the structure of spleen in rats. J Anhui Sci Technol Univ (2015) 29(6):1–5. doi:10.3969/j.issn.1673-8772.2015.06.001
17. Alam NH, Ashraf H, Gyr NE, Meier RF. Mo1061 evaluation of the efficacy of L-isoleucine and L-arginine supplemented food in the treatment of acute diarrhea in malnourished children. Gastroenterology (2013) 144(5):S566–67. doi:10.1016/S0016-5085(13)62095-8
18. Ren M. Branched-Chain Amino Acids Modulate Intestinal Defensing Expression and Immune Barrier Function of Piglets. PhD Thesis. Beijing: China Agricultural University (2014).
19. Alam NH, Raqib R, Ashraf H, Qadri F, Ahmed S, Zasloff M, et al. L-isoleucine-supplemented oral rehydration solution in the treatment of acute diarrhoea in children: a randomized controlled trial. J Health Popul Nutr (2011) 29(3):183–90. doi:10.3329/jhpn.v29i3.7864
20. Tô TL, Ward LA, Yuan L, Saif LJ. Serum and intestinal isotype antibody responses and correlates of protective immunity to human rotavirus in a gnotobiotic pig model of disease. J Gen Virol (1998) 79(Pt 11):2661. doi:10.1099/0022-1317-79-11-2661
21. Saif L, Yuan L, Ward L, To T. Comparative studies of the pathogenesis, antibody immune responses, and homologous protection to porcine and human rotaviruses in gnotobiotic piglets. Adv Exp Med Biol (1997) 412(412):397–403. doi:10.1007/978-1-4899-1828-4_62
22. Zeng X, Sunkara LT, Jiang W, Bible M, Carter S, Ma X, et al. Induction of porcine host defense peptide gene expression by short-chain fatty acids and their analogs. PLoS One (2013) 8(8):e72922. doi:10.1371/journal.pone.0072922
23. Maltese WA, Reitz BA, Volpe JJ. Effects of isoleucine deprivation on synthesis of sterols and fatty acids in LM-cells. J Biol Chem (1981) 256(5):2185–93.
24. Mao X, Qi S, Yu B, He J, Yu J, Chen D. Zn(2+) and L-isoleucine induce the expressions of porcine β-defensins in IPEC-J2 cells. Mol Biol Rep (2013) 40(2):1547–52. doi:10.1007/s11033-012-2200-0
25. Castañedadelgado JE, Araujo Z, Gonzalezcuriel I, Serrano CJ, Rivas CS, Encisomoreno JA, et al. Vitamin D and L-isoleucine promote antimicrobial peptide hBD-2 production in peripheral blood mononuclear cells from elderly individuals. Int J Vitam Nutr Res (2017). doi:10.1024/0300-9831/a000423
26. Zhao Y, Yu B, Mao X, He J, Huang Z, Zheng P, et al. Dietary vitamin D supplementation attenuates immune responses of pigs challenged with rotavirus potentially through the retinoic acid-inducible gene I signalling pathway. Br J Nutr (2014) 112(3):1–9. doi:10.1017/S000711451400097X
27. National Research Council. Nutrient Requirements of Swine. 11th ed. Washington, DC: National Academy Press (2012).
28. Mao X, Xiao X, Chen D, Yu B, He J, Chen H, et al. Dietary apple pectic oligosaccharide improves gut barrier function of rotavirus-challenged weaned pigs by increasing antioxidant capacity of enterocytes. Oncotarget (2017) 8(54):92420–30. doi:10.18632/oncotarget.21367
29. Mao X, Liu M, Tang J, Chen H, Chen D, Yu B, et al. Dietary leucine supplementation improves the mucin production in the jejunal mucosa of the weaned pigs challenged by porcine rotavirus. PLoS One (2015) 10(9):e0137380. doi:10.1371/journal.pone.0137380
30. Yuan L, Kang SY, Ward LA, To TL, Saif LJ. Antibody-secreting cell responses and protective immunity assessed in gnotobiotic pigs inoculated orally or intramuscularly with inactivated human rotavirus. J Virol (1998) 72(1):330–8.
31. Raengsakulrach B, Pattanapunyasat K, Kasinrerk W. Novel three-color reagent for measurement of CD4 and CD8 positive lymphocytes by flow cytometry. CMU J (2003) 2(3):165–72.
32. Velázquez FR, Matson DO, Calva JJ, Guerrero ML, Morrow AL, Carter-Campbell S, et al. Rotavirus infection in infants as protection against subsequent infections. N Engl J Med (1996) 335(14):1022–8. doi:10.1056/NEJM199610033351404
33. Halaihel N, Liévin V, Alvarado F, Vasseur M. Rotavirus infection impairs intestinal brush-border membrane Na(+)-solute cotransport activities in young rabbits. Am J Physiol Gastrointest Liver Physiol (2000) 279(3):587–96. doi:10.1152/ajpgi.2000.279.3.G587
34. Dhama K, Chauhan RS, Mahendran M, Malik SVS. Rotavirus diarrhea in bovines and other domestic animals. Vet Res Commun (2009) 33(1):1–23. doi:10.1007/s11259-008-9070-x
35. Mao X, Gu C, Hu H, Tang J, Chen D, Yu B, et al. Dietary Lactobacillus rhamnosus GG supplementation improves the mucosal barrier function in the intestine of weaned piglets challenged by porcine rotavirus. PLoS One (2016) 11(1):e0146312. doi:10.1371/journal.pone.0146312
36. Williams MB, Rosé JR, Rott LS, Franco MA, Greenberg HB, Butcher EC. The memory B cell subset responsible for the secretory IgA response and protective humoral immunity to rotavirus expresses the intestinal homing receptor, α4β7. J Immunol (1998) 161(8):4227–35.
37. Zijlstra RT, Mccracken BA, Odle J, Donovan SM, Gelberg HB, Petschow BW, et al. Malnutrition modifies pig small intestinal inflammatory responses to rotavirus. J Nutr (1999) 129(4):838–43. doi:10.1093/jn/129.4.838
38. Chen H, Hu H, Chen D, Tang J, Yu B, Luo J, et al. Dietary pectic oligosaccharide administration improves the growth performance and immunity in weaned pigs infected by rotavirus. J Agric Food Chem (2017) 65(14):2923–9. doi:10.1021/acs.jafc.7b00039
39. Lordelo MM, Gaspar AM, Le BL, Freire JP. Isoleucine and valine supplementation of a low-protein corn-wheat-soybean meal-based diet for piglets: growth performance and nitrogen balance. J Anim Sci (2008) 86(11):2936–41. doi:10.2527/jas.2007-0222
40. Zhao J, Liu Y, Jiang J, Wu P, Chen G, Jiang W, et al. Effects of dietary isoleucine on growth, the digestion and absorption capacity and gene expression in hepatopancreas and intestine of juvenile Jian carp (Cyprinus carpio var. Jian). Aquaculture (2012) 368–369(1):117–28. doi:10.1016/j.aquaculture.2012.09.019
41. Ren M, Zhang SH, Zeng XF, Liu H, Qiao SY. Branched-chain amino acids are beneficial to maintain growth performance and intestinal immune-related function in weaned piglets fed protein restricted diet. Asian-australas J Anim Sci (2015) 28(12):1742–50. doi:10.5713/ajas.14.0131
42. Dong XY, Azzam MM, Zou XT. Effects of dietary L-isoleucine on laying performance and immunomodulation of laying hens. Poult Sci (2016) 95(10):2297–305. doi:10.3382/ps/pew163
43. Luo Y, Zhang X, Qin C, Jiao N, Yin J. Effects of dietary isoleucine level on growth performance, carcass traits and meat quality of finishing pigs. Chin J Anim Nutr (2017) 29(6):1884–94. doi:10.3969/j.issn.1006-267x.2017.06.009
44. Eggum BO. Blood urea measurement as a technique for assessing protein quality. Br J Nutr (1970) 24(4):983–8. doi:10.1079/BJN19700101
45. Brown JA, Cline TR. Urea excretion in the pig: an indicator of protein quality and amino acid requirements. J Nutr (1974) 104(5):542–5. doi:10.1093/jn/104.5.542
46. Franklin EC. Structure and function of immunoglobulins. Proc Natl Acad Sci U S A (1975) 72(2):77–95.
47. Mantis NJ, Rol N, Corthésy B. Secretory IgA’s complex roles in immunity and mucosal homeostasis in the gut. Mucosal Immunol (2011) 4(6):603–11. doi:10.1038/mi.2011.41
48. Zhao J, Liu Y, Jiang J, Wu P, Jiang W, Li S, et al. Effects of dietary isoleucine on the immune response, antioxidant status and gene expression in the head kidney of juvenile Jian carp (Cyprinus carpio var. Jian). Fish Shellfish Immunol (2013) 35(2):572–80. doi:10.1016/j.fsi.2013.05.027
49. Huang Z, Zhou C, Lin H, Tan X, Peng J, Zhou W, et al. Effects of dietary isoleucine levels on activities of digestive enzymes and immune index of Trachinotus ovatus. South China Fish Sci (2017) 13(1):50–7. doi:10.3969/j.issn.2095-0780.2017.01.007
50. Sant AJ, Mcmichael A. Revealing the role of CD4+ T cells in viral immunity. J Exp Med (2012) 209(8):1391–5. doi:10.1084/jem.20121517
51. Swain SL, McKinstry KK, Strutt TM. Expanding roles for CD4+ T cells in immunity to viruses. Nat Rev Immunol (2012) 12(2):136. doi:10.1038/nri3152
52. Biron CA. Cytokines in the generation of immune responses to, and resolution of, virus infection. Curr Opin Immunol (1994) 6(4):530–8. doi:10.1016/0952-7915(94)90137-6
53. Meide PHVD, Schellekens H. Cytokines and the immune response. Biotherapy (1996) 8(3–4):243–9. doi:10.1007/BF01877210
54. Bassit RA, Sawada LA, Bacurau RF, Navarro F, Martins E Jr, Santos RV, et al. Branched-chain amino acid supplementation and the immune response of long-distance athletes. Nutrition (2002) 18(5):376–9. doi:10.1016/S0899-9007(02)00753-0
55. Janeway J, Medzhitov R. Innate immune recognition. Annu Rev Immunol (2002) 20(1):197–216. doi:10.1146/annurev.immunol.20.083001.084359
56. Klotman ME, Chang TL. Defensins in innate antiviral immunity. Nat Rev Immunol (2006) 6(6):447–56. doi:10.1038/nri1860
57. Medzhitov R, Janeway CA Jr. Innate immunity: impact on the adaptive immune response. Curr Opin Immunol (1997) 9(1):4–9. doi:10.1016/S0952-7915(97)80152-5
58. Medzhitov R, Janeway CA Jr. Innate immune recognition: mechanisms and pathways. Immunol Rev (2000) 173(173):89–97. doi:10.1034/j.1600-065X.2000.917309.x
59. Medzhitov R. Recognition of microorganisms and activation of the immune response. Nature (2007) 449(7164):819–26. doi:10.1038/nature06246
60. Kawai T, Akira S. The role of pattern-recognition receptors in innate immunity: update on toll-like receptors. Nat Immunol (2010) 11(5):373–84. doi:10.1038/ni.1863
61. Kumar H, Kawai T, Akira S. Pathogen recognition in the innate immune response. Biochem J (2009) 420(1):1–16. doi:10.1042/BJ20090272
62. Angel J, Franco MA, Greenberg HB. Rotavirus immune responses and correlates of protection. Curr Opin Virol (2012) 2(4):419–25. doi:10.1016/j.coviro.2012.05.003
63. Arnold MM, Patton JT. Rotavirus antagonism of the innate immune response. Viruses (2009) 1(3):1035–56. doi:10.3390/v1031035
64. Lan Q, Ren L, Zhuo Z, Lei X, Lan C, Xue Q, et al. Rotavirus nonstructural protein 1 antagonizes innate immune response by interacting with retinoic acid inducible gene I. Virol J (2011) 8(1):526. doi:10.1186/1743-422X-8-526
Keywords: isoleucine, rotavirus, immune response, pattern recognition receptors, weaned piglet
Citation: Mao X, Gu C, Ren M, Chen D, Yu B, He J, Yu J, Zheng P, Luo J, Luo Y, Wang J, Tian G and Yang Q (2018) l-Isoleucine Administration Alleviates Rotavirus Infection and Immune Response in the Weaned Piglet Model. Front. Immunol. 9:1654. doi: 10.3389/fimmu.2018.01654
Received: 20 March 2018; Accepted: 04 July 2018;
Published: 16 July 2018
Edited by:
Haruki Kitazawa, Tohoku University, JapanReviewed by:
Francisco José Pérez-Cano, University of Barcelona, SpainJue Hou, Virginia Mason Medical Center, United States
Copyright: © 2018 Mao, Gu, Ren, Chen, Yu, He, Yu, Zheng, Luo, Luo, Wang, Tian and Yang. This is an open-access article distributed under the terms of the Creative Commons Attribution License (CC BY). The use, distribution or reproduction in other forums is permitted, provided the original author(s) and the copyright owner(s) are credited and that the original publication in this journal is cited, in accordance with accepted academic practice. No use, distribution or reproduction is permitted which does not comply with these terms.
*Correspondence: Xiangbing Mao, acatmxb2003@163.com
†These authors have contributed equally to this work.