- 1Division of Immunology, Institute of Infectious Disease and Molecular Medicine, University of Cape Town, Cape Town, South Africa
- 2South African Tuberculosis Vaccine Initiative (SATVI), Department of Pathology, Institute of Infectious Disease, Molecular Medicine and University of Cape Town, Cape Town, South Africa
- 3Institutes of Immunology and Immunotherapy and Microbiology and Infection, University of Birmingham, Birmingham, United Kingdom
- 4The Jenner Institute, University of Oxford, Oxford, United Kingdom
- 5Laboratory of Molecular and Experimental Immunology and Neurogenetics, UMR 7355, Le Studium Institute for Advanced Studies, CNRS-University of Orléans, Orléans, France
Background: It is unclear whether antibodies can prevent Mycobacterium tuberculosis (Mtb) infection. In this study, we examined the relationship between total plasma IgG levels, IgG elicited by childhood vaccines and soil-transmitted helminths, and Mtb infection prevalence, defined by positive QuantiFERON (QFT) test.
Methods: We studied 100 Mtb uninfected infants, aged 4–6 months. Ten infants (10%) converted to positive QFT test (QFT+) within 2 years of follow-up for Mtb infection. Antibody responses in plasma samples acquired at baseline and tuberculosis investigation were analyzed by enzyme-linked immunosorbent assay and ImmunoCAP® assay.
Results: QFT− infants displayed a significant increase in total IgG titers when re-tested, compared to IgG titers at baseline, which was not observed in QFT+ infants. Bacille Calmette-Guérin (BCG) vaccine-specific IgG2 and live-attenuated measles vaccine-specific IgG were raised in QFT− infants, and infants who acquired an Mtb infection did not appear to launch a BCG-specific IgG2 response. IgG titers against the endemic helminth Ascaris lumbricoides increased from baseline to QFT re-testing in all infants.
Conclusion: These data show raised IgG associates with a QFT-status. Importantly, this effect was also associated with a trend showing raised IgG titers to BCG and measles vaccine. Our data suggest a possible protective association between raised antibody titers and acquisition of Mtb infection, potentially mediated by exposure to antigens both related and unrelated to Mtb.
Introduction
Mycobacterium tuberculosis (Mtb) infection and tuberculosis (TB) disease represents one of the greatest global infectious disease burdens (1). The only licensed TB vaccine, Bacille Calmette-Guérin (BCG), may partially protect against Mtb infection and provides protection against disseminated forms of active TB disease (2–5), but protection against pulmonary TB disease varies widely with age and mycobacterial exposure (6). Development of a vaccine that is more effective than BCG is crucial for the ultimate control of both Mtb infection and TB disease (7, 8).
Protection conferred by most clinically efficacious vaccines against their target pathogens requires a protective antibody response (9–11). Antibody responses against Mtb do correlate with, and may provide protection against, TB disease (12–17). However, there is no clinical evidence to support the hypothesis that an effective TB vaccine might be based on induction of an antibody response that controls Mtb infection.
Our current understanding of how antibodies could protect against Mtb infection or TB disease is limited, when compared with our understanding of, for example, T cell-driven immunity to Mtb. However, classical functions of antibody such as neutralization, opsonization, and antibody-dependent cellular cytotoxicity (ADCC) have all been demonstrated to contribute to protective immunity against Mtb. Antibody opsonization can enhance Mtb uptake by (for example) macrophages (12–14), while both antibody neutralization (12, 13) and ADCC-mediated control of Mtb have been shown to inhibit bacterial growth (18). The intrinsic features of an antibody also contribute to their ability to control Mtb. For example, variation in immunoglobulin glycosylation can influence antibody-mediated control of bacterial replication (18). Different roles for antibody classes in the control of Mtb infection have also been demonstrated. In active TB disease, IgG has been shown to impair control and IgA enhance control of disease (19). Therefore, the full range of antibody functions can influence host immunity to Mtb, but whether these effects enhance or impede control of infection is likely to vary, depending on whether the host is responding to a latent infection or pathogenic disease.
Any antibody-mediated protection against Mtb may also be influenced by host vaccination status and/or history of co-infections unrelated to Mtb. Childhood vaccinations, such as live attenuated measles, have been suggested to alter Mtb-associated immune responses (20, 21). Additionally, in Sub-Saharan Africa, the potential effect of common endemic infections, such as helminths, on immune responses to concurrent or subsequent Mtb infection is an important consideration due to the substantial overlap in prevalence of these diseases (22). Clear evidence exists that helminths can alter host immunity to Mtb (23, 24). However, our overall understanding of the influence of bystander antigen/pathogen challenges and immunity to Mtb remains unclear, warranting further investigation.
Our study participants live in a region endemic for both TB and soil-transmitted helminths (STH) (25). They receive routine Expanded Programme on Immunization (EPI) vaccinations, which provides a platform to investigate the potential interplay between vaccines, helminth exposure, and Mtb immunity. In this study, we addressed whether plasma antibody levels associate with risk of acquiring Mtb infection in infants. Additionally, we sought to identify if associations existed between exposure to both related and unrelated antigens/pathogens and altered risk of Mtb infection.
Materials and Methods
Study Participants
This is a sub-study of a double-blind, placebo-controlled efficacy trial of the MVA85A vaccine candidate, performed in the Western Cape Province of South Africa, and which has been described previously (7). Infants aged 4–6 months, who had received BCG vaccine within 7 days of birth, were enrolled. Inclusion criteria at baseline were a negative HIV enzyme-linked immunosorbent assay (ELISA) result; negative QuantiFERON-TB Gold In-tube (QFT) test (used to detect Mtb infection by measuring IFNγ responses to specific mycobacterial proteins); and no known exposure to an adult with active TB disease. Infants were expected to have received all routine vaccinations, per the EPI schedule. The trial showed that MVA85A boost vaccination did not provide additional protection against Mtb infection or TB disease, compared to BCG vaccination alone in newborns (7). Therefore, this sub-study combines infants from the placebo and MVA85A arms in the same analysis. 112 infants were sequentially recruited into the sub-study at the time of TB investigation (Figure 1A). However, complete clinical data and sample sets were not collected for 12 participants, thus leaving clinical data and samples from 100 participants (89.29%) available for analysis in this study (Figure 1A).
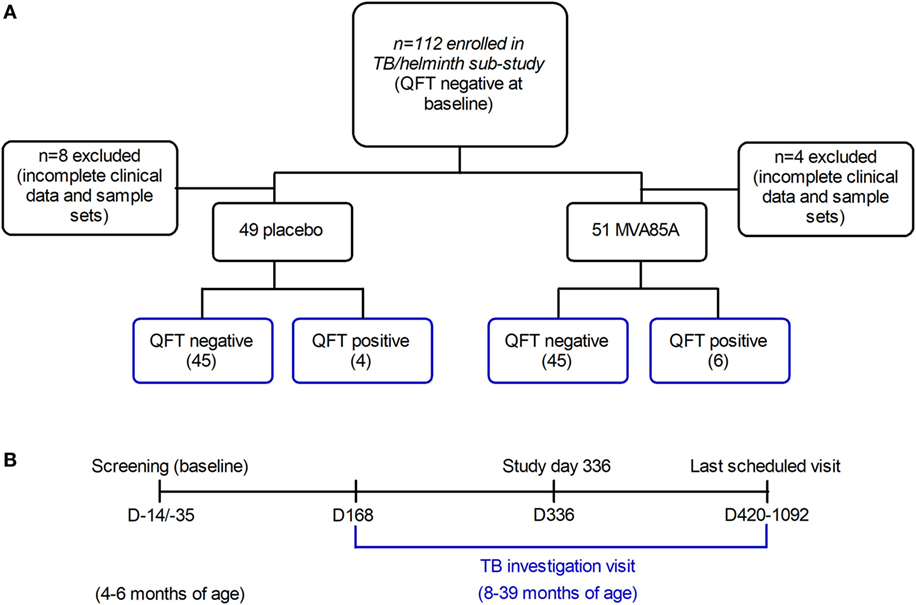
Figure 1. Infants recruited to the tuberculosis (TB)/helminth study. Infants enrolled in the MVA85A trial were randomly assigned to the placebo or MVA85A vaccination arms (A). 112 infants were recruited to the TB/helminth study; incomplete clinical data and sample sets were available for n = 12 infants, leaving n = 100 available for further analysis. Time-points of blood collection for QFT (QuantiFERON) (B) were at baseline, study day 336, at last scheduled visit and/or upon TB investigation. Per participant, serum was analyzed at baseline and upon TB investigation. The age range of infants at baseline was 4–6 months, and the age range at TB investigation was 8–39 months.
The sub-study and the parent trial (C-020-485) were approved by the University of Cape Town Faculty of Health Sciences Human Research Ethics Committee (032/2010 and 291/2008 respectively); and written informed consent was obtained from the infants’ parents or legal guardians prior to participation.
Mtb Infection
Infants were followed up at 3-monthly intervals for symptoms consistent with TB disease, which if detected, triggered admission for standardized investigation, including QFT re-testing. Infants with symptoms of weight loss or failure to thrive would routinely receive empirical anthelmintic therapy, in addition to regular mass deworming campaigns. Information about prior anthelmintic therapy was not collected. Asymptomatic infants were admitted if an individual with TB disease became a new household contact (7). Plasma samples were analyzed at baseline and at admission for TB disease investigation (Figure 1B). One sample was analyzed per participant, per time-point. Mtb infection was defined by a single positive QFT test (i.e., interferon-gamma release assay conversion) at the time of TB investigation. Infants who were QFT positive, but did not show evidence of probable or definite TB disease were classified as Mtb-infected. None of the infants, irrespective of QFT status, were diagnosed with active TB disease. Infants were stratified by QFT negative (Mtb-uninfected), and QFT positive (Mtb-infected) status in the subsequent analyses; none of the infants had active TB disease.
Antigen Preparation for ELISA
Vaccine Antigens
Bacille Calmette-Guérin (Danish strain 1331, Statens Serum Institut, Denmark), live-attenuated measles (Rouvax, Sanofi Pasteur), and tetanus toxoid (Tetavax, Sanofi Pasteur) vaccines were reconstituted in 1× phosphate-buffered saline (PBS) to produce antigen stocks (500 µg/ml); aliquots of stocks were stored at −80°C until required.
Helminth Antigen
Whole Ascaris lumbricoides worms were treated with a 10× penicillin/streptomycin solution in 1× Amphotericin B (Thermo Fisher Scientific), following which they were washed in filter-sterilized (FS) 1× PBS. Worm sections were then homogenized in FS 1× PBS and centrifuged to remove cellular debris. The protein concentration of the soluble fraction was measured and adjusted to a stock concentration. Aliquots of the stock solution were frozen at −80°C until required.
Non-Specific and Antigen-Specific ELISA
The coating IgG antibody used for the total IgG ELISAs was used at 1:5,000 (anti-human IgG Fc-specific; Sigma-Aldrich). All vaccine and helminth coating antigens were used at 5 µg/ml for antigen-specific IgG or 10 µg/ml for antigen-specific IgG subtypes. Initial plasma sample dilutions were 1:50 (total IgG) and 1:20 (antigen-specific), followed by serial 1:5 dilutions of the initial dilutions. Secondary IgG (Southern Biotech) was used at 1:1,000, and secondary IgG subtypes (Southern Biotech) were used at 1:500. All secondary antibodies were alkaline phosphatase-linked.
Antibody ELISAs were performed similarly to methods described previously (19, 26). Briefly, Nunc Immuno Maxisorp 96-well plates (Thermo Fisher Scientific) were coated with antibody or antigen and incubated at 37°C for 3 h, following which plates were blocked overnight at 4°C. Sample was added (50 μl/well) and plates were incubated at 4°C overnight. Secondary antibody was added (50 μl/well) after which the reactions were developed using PNP substrate (Sigma Aldrich). Reactions were stopped with 1 M NaOH and plates read on a Versamax 96-well plate reader (Molecular Devices) at 405 nm (492 nm reference filter). Arbitrary antibody responses were determined from sample titration curves (optical density vs. sample dilution) as previously described (27), enabling a relative assessment of antibody levels in serum. According to this analysis, antibody levels in certain samples and for certain antibody types fell below the detection limit and are observed at 0 on the y-axes. This does not preclude the possibility of specific antibody responses within those samples, but may require detection with a more sensitive assay.
ImmunoCAP® Test
The ImmunoCAP® in vitro assay was used to detect antigen-specific IgE responses in serum samples and was used according to the manufacturer’s instructions. An Ascaris suum-derived antigen that is cross-reactive with A. lumbricoides was used to detect antigen-specific IgE. Responses to this antigen are described as Ascaris specific.
Statistical Analysis
Dot plot graphs are represented with the median and interquartile range where appropriate. Antibody responses in matched sample pairs were compared using the Wilcoxon matched-pairs signed rank test; the Mann–Whitney test was utilized for the analysis of unpaired two-group data. Grouped analyses were investigated by the Kruskal–Wallis test, with the Dunn’s multiple comparison posttest used to compare all pairs of columns. Correlations were investigated using the Spearman correlation test. Where appropriate, analyses were two-tailed. Significance was accepted at p ≤ 0.05. Analyses of immunological data were performed using the GraphPad Prism software (v. 5.03).
Results
Cohort Description
The median age of infants at TB investigation was 20 months (interquartile range 16–25.63 months). Of the 100 infants analyzed, 10 (10%) tested QFT positive upon re-testing (Table 1).
Infants Displayed an Age-Related Increase in Total IgG Titers
Analysis of total IgG titers revealed a significant increase in total IgG detected at the TB investigation visit, when compared to baseline (Figure 2A). At TB investigation, total IgG titers showed a clear bimodal distribution approximately around the median; further analysis revealed a significant reduction in detected total IgG in older participants (Figure 2B). No significant difference in the median age between QFT− and QFT+ participants was found (median age in months 20 vs. 23, respectively; p = 0.14) (Figure 2C).
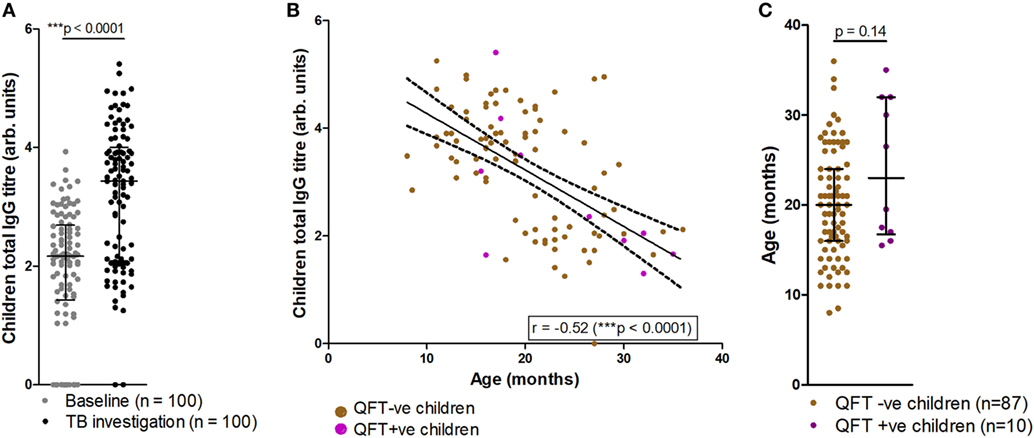
Figure 2. Comparisons of tuberculosis (TB) investigation total IgG responses to baseline total IgG, age and QFT outcome. Total serum IgG in participants at baseline and upon TB investigation visit (n = 100) as measured by enzyme-linked immunosorbent assay (A). Total IgG titers vs. age in months at TB investigation (n = 97), with samples from QFT positive participants indicated in purple (B); overlaid are the line-of-best-fit and 95% confidence bands (dashed lines). Association between age at TB investigation and QFT result (C). Antibody titers are presented as log-transformed arbitrary values. The Wilcoxon matched-pairs signed rank test was used to assess significance of the comparison in (A), and the Mann–Whitney test was used to assess significance in (C). The Spearman correlation was used to assess the strength of the correlation in (B).
Total IgG Titers Increase Significantly in QFT Negative Infants
At baseline, infants who did or did not progress to Mtb infection had similar total IgG titers (Figure 3A). Analysis of total IgG responses at TB investigation revealed that infants who remained QFT− showed significant increases in total IgG titers from baseline compared to infants who became Mtb-infected (QFT+) (Figure 3A). However, there was no significant difference in total IgG titers between the QFT− and QFT+ groups at TB investigation (Figure 3A).
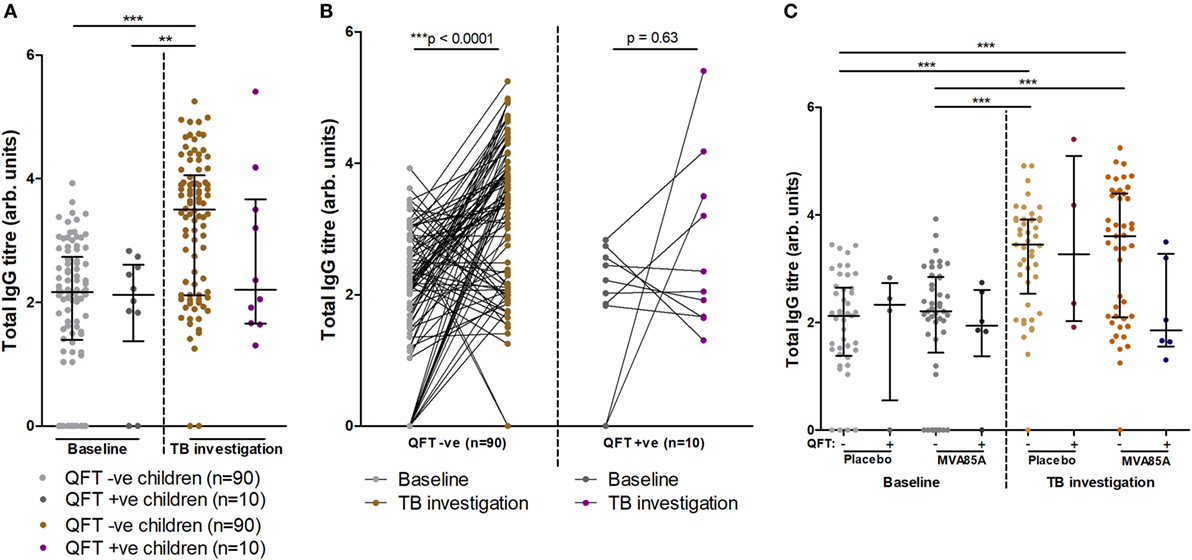
Figure 3. Total IgG titers stratified by QFT outcome and MVA vaccination status. Relationship between QFT outcome and total IgG titers from baseline and tuberculosis (TB) investigation participant samples (A). A before/after comparison of samples subdivided as in (A) is presented in (B). Relationship between QFT outcome, MVA85A vaccination status, and total IgG titers from baseline and TB investigation participant samples (C). Column sample numbers left-right (C): 45, 4, 45, 6, 45, 4, 45, 6. Antibody titers are presented as arbitrary values. Statistical analysis was performed with the Kruskal–Wallis test and Dunn’s post hoc test to assess significance of the comparisons in (A,C). The Wilcoxon matched-pairs signed rank test was used to assess significance of the associations in (B).
Furthermore, longitudinal analysis of infant IgG responses at baseline and at TB investigation showed significantly increased total IgG titers compared to baseline in infants who remained QFT− on the level of individual participants (Figure 3B). Infants who were QFT+ at TB investigation did not demonstrate significant increases in total IgG (Figure 3B).
The potential influence on total IgG responses of vaccination with MVA85A or placebo (Candin®) was also addressed. In agreement with data presented in Figure 3A, we found no significant differences in total IgG between placebo and MVA85A vaccinated study participants at baseline or TB investigation. Moreover, no differences were found in IgG titers between QFT− or QFT+ placebo and MVA85A vaccinated infants (Figure 3C).
QFT− Status and Vaccine-Specific IgG titers After BCG and Measles Vaccination
We next tested whether QFT status associated with antibody responses to BCG or to heterologous antigens. Antigen-specific antibody responses to BCG, live-attenuated measles, and tetanus toxoid vaccines were also measured at TB investigation and compared to infant Mtb infection outcome. Analysis of IgG responses to BCG, measles, and tetanus toxoid revealed a trend for increased measles-specific IgG titers (p = 0.08) in infants who did not acquire Mtb infection, but no association between BCG or tetanus toxoid IgG and Mtb infection outcome (Figure 4A). Analysis of BCG IgG subtype-specific responses revealed a trend for increased BCG-specific IgG2 titers (p = 0.08) in infants who did not acquire Mtb infection, but no association between BCG-specific IgG1 or IgG3 and Mtb infection outcome (Figure 4B). This finding was related to significant inverse correlations between measles-specific IgG and quantitative QFT values, and between BCG-specific IgG2 and quantitative QFT values (Figures S1A,B in Supplementary Material). No associations were found with BCG-specific IgG/IgG1/IgG3 or tetanus-specific IgG titers and quantitative QFT values (Figures S1C–F in Supplementary Material). However, it is important to note that all participants who had Mtb infection but not disease had low levels of detectable IFNγ. As expected (28), tetanus toxoid-specific IgG titers significantly correlated with age at follow-up (Figure S2F in Supplementary Material). No relationship with age was found with anti-BCG or live-attenuated measles IgG titers at follow-up (Figure S2 in Supplementary Material).
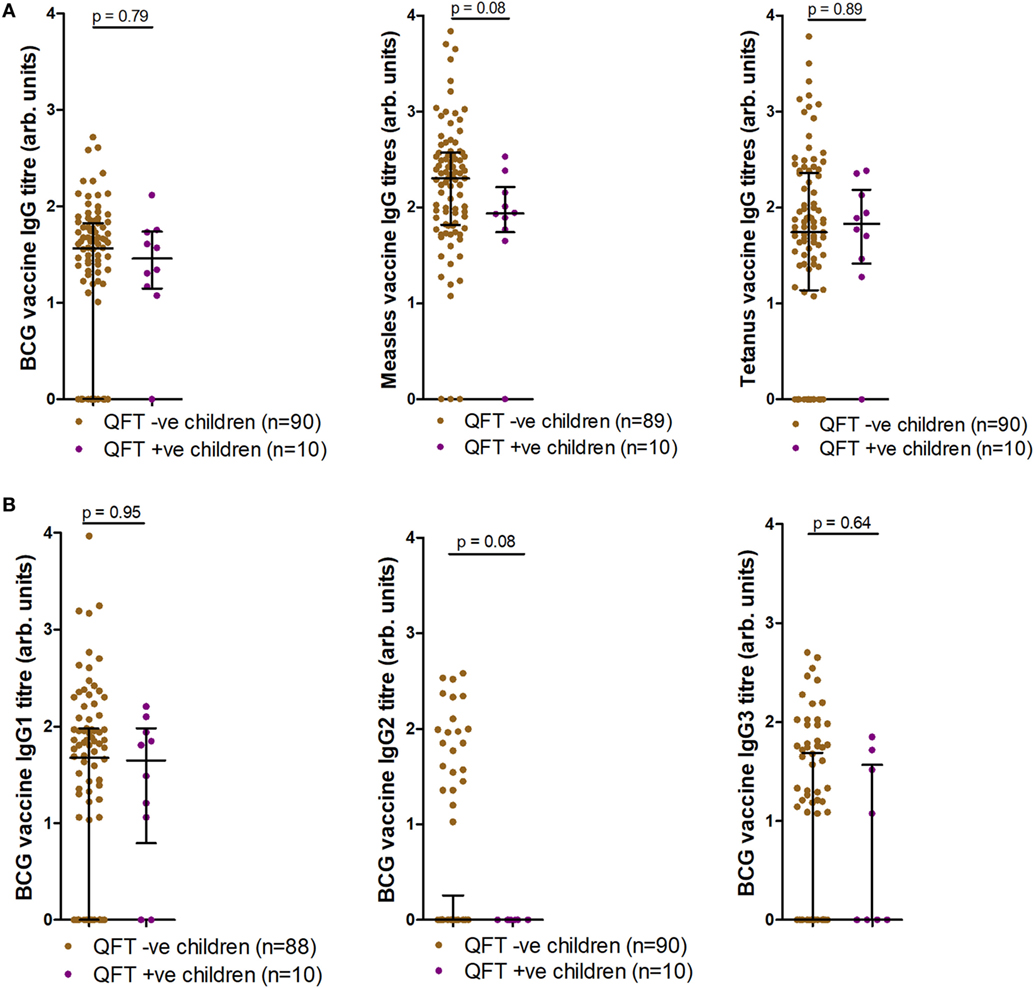
Figure 4. Antibody responses at tuberculosis investigation to childhood vaccines. Anti-Bacille Calmette-Guérin (BCG), measles, and tetanus IgG titers (A), as well as anti-BCG IgG1, IgG2, and IgG3 titers (B) as measured by enzyme-linked immunosorbent assay and stratified by QFT result. One sample was excluded in the anti-measles IgG analysis (A) (participants had not received measles vaccination). Two fewer samples reported for BCG IgG1 (B) due to a lack of sample availability. Antibody titers are presented as arbitrary values. Comparisons were assessed for significance by the Mann–Whitney test.
Soil-Transmitted Helminth-Specific IgG Titers Were Similar in QFT− and QFT+ Infants
Since helminth exposure can alter risk and outcome of Mtb disease (29–31), we examined if any relationship existed between infant exposure to A. lumbricoides and Mtb infection. Of 91 stool samples obtained, none tested positive for active helminth infection (Table S1 in Supplementary Material). However, anti-Ascaris IgE and IgG, indicative of prior exposure to the parasite, were found in infants (Figure 5A). At TB investigation, 2/50 (4%) infants tested for IgE responses presented with raised Ascaris-specific IgE, as detected by ImmunoCAP® (Figure 5A). Additionally, 85/100 (85%) infants at baseline and all infants at TB investigation had detectable anti-A. lumbricoides IgG, with these levels increasing significantly from baseline to TB investigation (Figure 5A). Analysis of IgG subtype responses revealed that 6/100 (6%) infants at baseline and 48/100 (48%) infants at TB investigation had detectable levels of anti-A. lumbricoides IgG4 (Figure 5A). Anti-A. lumbricoides IgG nor IgG4 titers were not significantly different between QFT− infants and QFT+ infants (Figure 5B).
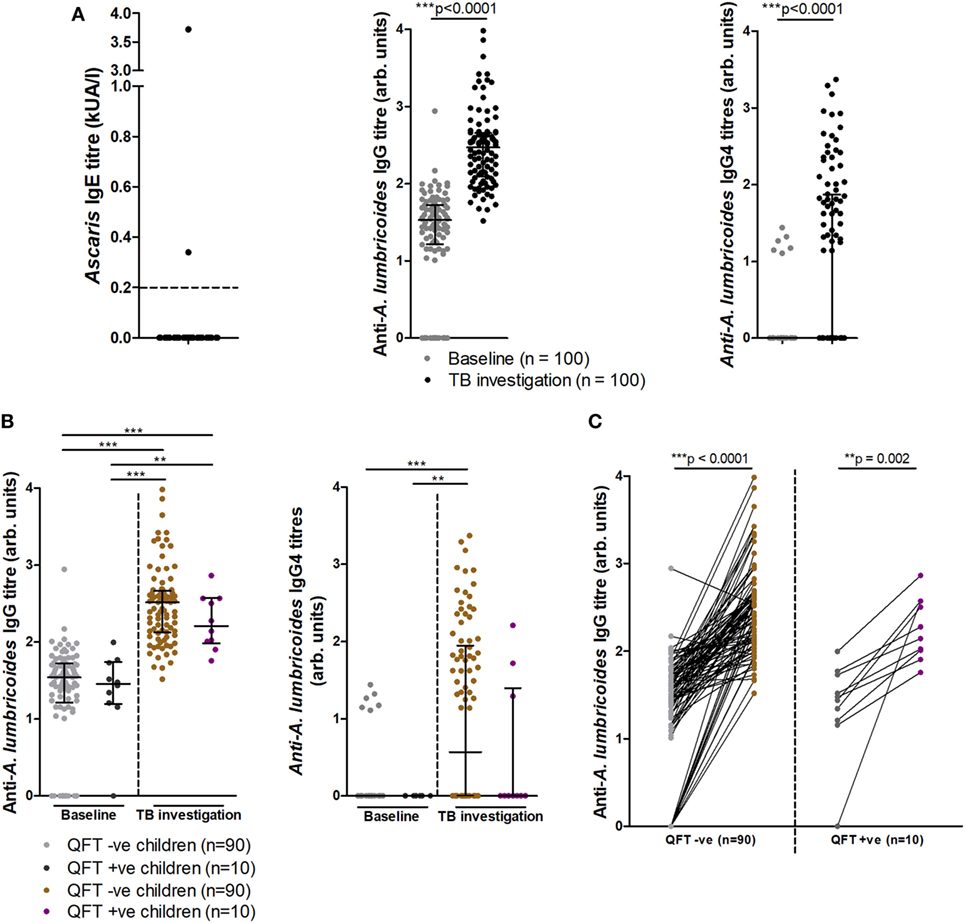
Figure 5. Soil-transmitted helminth-specific IgG titers and QFT outcome at baseline and upon tuberculosis investigation. Anti-Ascaris lumbricoides IgE (n = 50), IgG, and IgG4 (n = 100) titers (A). Anti-A. lumbricoides IgG and IgG4 titers stratified by QFT outcome (B). A before/after comparison of anti-A. lumbricoides IgG titers as subdivided in (B) is presented in (C). Antibody titers are presented as arbitrary values. The Mann–Whitney test was used to assess two-group comparisons for significance in (A), the Kruskal–Wallis test with Dunn’s post hoc test was used for the multi-group comparisons in (B), and the Wilcoxon matched-pairs signed rank test was used to assess for significance in (C).
Longitudinal analysis of individual infant anti-A. lumbricoides IgG responses at baseline and TB investigation showed significantly increased total IgG titers in both QFT− and QFT+ infants, indicating an increase in anti-A. lumbricoides IgG responses over time (Figure 5C). Others have suggested that helminth infection negatively influences the magnitude of the QFT IFNγ response, resulting in a higher likelihood of an indeterminate result (32, 33). Although no association was found between anti-A. lumbricoides IgG and quantitative IFNγ in the same samples (as detected by QFT), a significant inverse correlation was found between anti-A. lumbricoides IgG4 and quantitative IFNγ levels (Figure S3 in Supplementary Material).
Discussion
In this study, we found that infants who did not acquire Mtb infection had significantly increased total IgG titers from baseline to TB investigation, unlike infants who became Mtb infected. This effect was related to a trend showing raised IgG titers against BCG and measles vaccination. Together, these findings suggest that raised specific and heterologous IgG titers may play a role in host responses that protect against Mtb infection. Therefore, induction of protective IgG responses in QFT− infants may be a feature of immune activation by both pathogen-related and unrelated antigens.
Initial findings indicate that total IgG responses increase significantly from baseline to TB investigation; however, at TB investigation, there was an inverse correlation between total IgG titers and age. It is important to note that the total IgG titers presented are from two distinct age ranges, namely 4–6 months at baseline and 8–39 months at TB investigation, and that the range of antibody titer values is wide. Additionally, older infants tend to fall within the lower half of the bimodal sample distribution observed at TB investigation, indicating why an inverse correlation between age and antibody titer is possible despite a higher median total IgG titer at TB investigation than at baseline.
Our findings build on recent reports demonstrating that antibody responses differentially contribute to control of Mtb infection and disease. Studies have addressed Mtb antigen-specific immunoglobulin responses as contributing to a range of classical antibody-mediated responses (18, 19). In this study, we observed that only QFT− infants showed a significant increase in total IgG titers from baseline to TB investigation, suggesting an association between raised IgG and reduced risk of Mtb infection, highlighting the potential importance of antibodies in protective responses against Mtb.
It has long been known that BCG vaccination stimulates Mycobacterium-specific antibody production (34–36). Induction of IgM and IgG has been observed, with IgG1, IgG2, and IgG3 as the most prevalent IgG subtypes detected (37, 38). Our identification of BCG-induced antibody responses in this study supports these previous studies. Our findings also suggest that infants who became Mtb infected did not launch a BCG-specific IgG2 response, which could indicate some impairment of the humoral response to BCG vaccination in these infants, which in turn may be implicated in increased susceptibility to Mtb infection. However, the current study lacks sufficient participant numbers and power to address this question adequately and a larger cohort study would be required to analyze the importance of this finding. Nevertheless, to the best of our knowledge, this observation is novel and warrants further investigation.
We also identified a trend toward increased measles vaccine-specific IgG titers in QFT− infants as compared to QFT+ infants. However, since this difference was not statistically significant, the possibility that measles vaccination might provide heterologous protection against acquisition of Mtb infection remains speculative. Measles vaccination has previously been shown to decrease responsiveness to the tuberculin skin test in individuals with a positive reaction to purified protein derivative and/or active TB disease (20, 21). Conversely others have suggested that certain childhood vaccines, such as measles (and BCG), may provide protection against all-cause mortality (39, 40).
We acknowledge that generalizability of the abovementioned results is limited by the sample size and that other infections at the time of TB investigation may have contributed to total IgG titers. However, it is unlikely that other infections would contribute differentially to IgG titers in QFT+ vs. QFT− children, or to BCG-specific or measles vaccine-specific antibody titers.
We found no association between anti-A. lumbricoides IgG or IgG4 responses and Mtb infection, suggesting that anti-helminth responses do not underlie the differences observed with the total IgG responses in this cohort. Our current understanding of how helminth infection/exposure may influence Mtb infection is incomplete. Rodent studies have presented evidence of positive (23), neutral (41–43) and negative effects (30, 44) on host control of Mtb infection by helminths. Clinical reports of the relationship between helminth and Mtb infections are also not consistent, which is likely to reflect the diverse contexts of both helminth or Mtb infection/disease. Similar studies to ours also did not identify positive or negative associations between helminth exposure and Mtb infection or BCG efficacy (45–47).
We found that few infants had raised anti-A. lumbricoides IgE, in agreement with reports by others that anti-Ascaris IgE is primarily detected in older children and IgG4 is primarily detected in younger children (48). We propose that the raised levels of anti-A. lumbricoides IgG we observed are reflective of prior exposure to helminths. The unexpectedly low levels of current infection (25) may be attributable to appropriate household sanitation, water provision (Table S1 in Supplementary Material), and mass de-worming campaigns in the study community. Regardless, infants in this study showed evidence of immunological memory to helminth infection.
This study did not address antibody responses to Mtb-specific antigens due to a lack of antigen availability, and limited samples could not be used to optimize functional assays for more in-depth analysis of antibody responses. It is also important to consider the impact that small sample size may have had on the findings presented here. Despite a 2-year cumulative incidence of Mtb infection of 10%, the number of infants in the study who demonstrated QFT conversion was small. A larger sample size would be required to investigate these initial findings more thoroughly. Notably, antigen-specific antibody responses and their association with Mtb infection and/or TB disease have been investigated in a similar cohort; here, antigen 85A-specfic IgG responses were associated with a difference in the occurrence of TB disease, but not Mtb infection (15). However, a direct comparison between studies would be inaccurate due to the difference in antigen specificity and design of the assays, as well as the discrepancy in sample size between studies.
The data presented here suggest that both pathogen related and heterologous immune activation of the immature immune system by childhood vaccination may be implicated in reduced risk of acquiring an Mtb infection. The central finding that infants who did not acquire Mtb infection exhibited significantly increased total IgG titers from baseline to the time of TB investigation warrants further investigation of the specific components of the total IgG response responsible for this observation.
Ethics Statement
This study was carried out in accordance with the recommendations of the University of Cape Town Faculty of Health Sciences Human Research Ethics Committee. The protocol was approved by the University of Cape Town Faculty of Health Sciences Human Research Ethics Committee. Written informed consent was obtained from the infants’ parents or legal guardians in accordance with the Declaration of Helsinki.
Author Contributions
AL, WH, and MH contributed to conceptualization and design of the study; EL performed experiments and data analysis; AL was the site investigator of the helminth study and analyzed clinical and sociodemographic data; MT and MH were principal investigators of the parent trial; HM was the study data manager; CO was the study coordinator; EL, TS, WH, and MH drafted the manuscript; EL, AL, DM, AC, MT, HS, WH, and MH contributed to manuscript revision and critical analysis.
Conflict of Interest Statement
The authors declare that the research was conducted in the absence of any commercial or financial relationships that could be construed as a potential conflict of interest.
Acknowledgments
The authors would like to acknowledge and thank Professor Michael Levin (Red Cross War Memorial Children’s Hospital) for his provision of the A. lumbricoides worms used in this study, as well as the ImmunoCAP® testing for helminth-specific IgE responses. The authors would also like to acknowledge and thank the study participants, helminth-TB and 020 study teams for their contribution and data collection.
Funding
This work was supported by the EDCTP Senior Fellowship grant (TA.2009.40200.015) and the National Research Foundation (88939).
Supplementary Material
The Supplementary Material for this article can be found online at https://www.frontiersin.org/articles/10.3389/fimmu.2018.01529/full#supplementary-material.
References
2. Fine PE. Variation in protection by BCG: implications of and for heterologous immunity. Lancet (1995) 346(8986):1339–45. doi:10.1016/S0140-6736(95)92348-9
3. Colditz GA, Berkey CS, Mosteller F, Brewer TF, Wilson ME, Burdick E, et al. The efficacy of bacillus Calmette-Guerin vaccination of newborns and infants in the prevention of tuberculosis: meta-analyses of the published literature. Pediatrics (1995) 96(1 Pt 1):29–35.
4. Roy A, Eisenhut M, Harris RJ, Rodrigues LC, Sridhar S, Habermann S, et al. Effect of BCG vaccination against Mycobacterium tuberculosis infection in children: systematic review and meta-analysis. BMJ (2014) 349:g4643. doi:10.1136/bmj.g4643
5. Basu Roy R, Sotgiu G, Altet-Gomez N, Tsolia M, Ruga E, Velizarova S, et al. Identifying predictors of interferon-gamma release assay results in pediatric latent tuberculosis: a protective role of bacillus Calmette-Guerin? A pTB-NET collaborative study. Am J Respir Crit Care Med (2012) 186:378–84. doi:10.1164/rccm.201201-0026OC
6. Mangtani P, Abubakar I, Ariti C, Beynon R, Pimpin L, Fine PE, et al. Protection by BCG vaccine against tuberculosis: a systematic review of randomized controlled trials. Clin Infect Dis (2014) 58(4):470–80. doi:10.1093/cid/cit790
7. Tameris MD, Hatherill M, Landry BS, Scriba TJ, Snowden MA, Lockhart S, et al. Safety and efficacy of MVA85A, a new tuberculosis vaccine, in infants previously vaccinated with BCG: a randomised, placebo-controlled phase 2b trial. Lancet (2013) 381(9871):1021–8. doi:10.1016/S0140-6736(13)60177-4
8. Andersen P, Kaufmann SH. Novel vaccination strategies against tuberculosis. Cold Spring Harb Perspect Med (2014) 4(6):1–20. doi:10.1101/cshperspect.a018523
9. Achkar JM, Casadevall A. Antibody-mediated immunity against tuberculosis: implications for vaccine development. Cell Host Microbe (2013) 13(3):250–62. doi:10.1016/j.chom.2013.02.009
11. Robbins JB, Schneerson R, Szu SC. Hypothesis: how licensed vaccines confer protective immunity. Adv Exp Med Biol (1996) 397:169–82. doi:10.1007/978-1-4899-1382-1_22
12. de Valliere S, Abate G, Blazevic A, Heuertz RM, Hoft DF. Enhancement of innate and cell-mediated immunity by antimycobacterial antibodies. Infect Immun (2005) 73(10):6711–20. doi:10.1128/IAI.73.10.6711-6720.2005
13. Chen T, Blanc C, Eder AZ, Prados-Rosales R, Souza AC, Kim RS, et al. Association of human antibodies to arabinomannan with enhanced mycobacterial opsonophagocytosis and intracellular growth reduction. J Infect Dis (2016) 214(2):300–10. doi:10.1093/infdis/jiw141
14. Kumar SK, Singh P, Sinha S. Naturally produced opsonizing antibodies restrict the survival of Mycobacterium tuberculosis in human macrophages by augmenting phagosome maturation. Open Biol (2015) 5(12):150171. doi:10.1098/rsob.150171
15. Fletcher HA, Snowden MA, Landry B, Rida W, Satti I, Harris SA, et al. T-cell activation is an immune correlate of risk in BCG vaccinated infants. Nat Commun (2016) 7:11290. doi:10.1038/ncomms11290
16. Maglione PJ, Xu J, Casadevall A, Chan J. Fc gamma receptors regulate immune activation and susceptibility during Mycobacterium tuberculosis infection. J Immunol (2008) 180(5):3329–38. doi:10.4049/jimmunol.180.5.3329
17. Teitelbaum R, Glatman-Freedman A, Chen B, Robbins JB, Unanue E, Casadevall A, et al. A mAb recognizing a surface antigen of Mycobacterium tuberculosis enhances host survival. Proc Natl Acad Sci U S A (1998) 95(26):15688–93. doi:10.1073/pnas.95.26.15688
18. Lu LL, Chung AW, Rosebrock TR, Ghebremichael M, Yu WH, Grace PS, et al. A functional role for antibodies in tuberculosis. Cell (2016) 167(2):433–443.e14. doi:10.1016/j.cell.2016.08.072
19. Zimmermann N, Thormann V, Hu B, Kohler AB, Imai-Matsushima A, Locht C, et al. Human isotype-dependent inhibitory antibody responses against Mycobacterium tuberculosis. EMBO Mol Med (2016) 8(11):1325–39. doi:10.15252/emmm.201606330
20. Mellman WJ, Wetton R. Depression of the tuberculin reaction by attenuated measles virus vaccine. J Lab Clin Med (1963) 61:453–8.
21. Brody JA, Overfield T, Hammes LM. Depression of the tuberculin reaction by viral vaccines. N Engl J Med (1964) 271:1294–6. doi:10.1056/NEJM196412172712505
22. Hotez PJ, Mistry N, Rubinstein J, Sachs JD. Integrating neglected tropical diseases into AIDS, tuberculosis, and malaria control. N Engl J Med (2011) 364(22):2086–9. doi:10.1056/NEJMp1014637
23. du Plessis N, Kleynhans L, Thiart L, van Helden PD, Brombacher F, Horsnell WG, et al. Acute helminth infection enhances early macrophage mediated control of mycobacterial infection. Mucosal Immunol (2013) 6(5):931–41. doi:10.1038/mi.2012.131
24. Elias D, Akuffo H, Pawlowski A, Haile M, Schon T, Britton S. Schistosoma mansoni infection reduces the protective efficacy of BCG vaccination against virulent Mycobacterium tuberculosis. Vaccine (2005) 23(11):1326–34. doi:10.1016/j.vaccine.2004.09.038
25. Hotez PJ, Kamath A. Neglected tropical diseases in sub-saharan Africa: review of their prevalence, distribution, and disease burden. PLoS Negl Trop Dis (2009) 3(8):e412. doi:10.1371/journal.pntd.0000412
26. Cooper PJ, Espinel I, Wieseman M, Paredes W, Espinel M, Guderian RH, et al. Human onchocerciasis and tetanus vaccination: impact on the postvaccination antitetanus antibody response. Infect Immun (1999) 67(11):5951–7.
27. Bobat S, Darby M, Mrdjen D, Cook C, Logan E, Auret J, et al. Natural and vaccine-mediated immunity to Salmonella typhimurium is impaired by the helminth Nippostrongylus brasiliensis. PLoS Negl Trop Dis (2014) 8(12):e3341. doi:10.1371/journal.pntd.0003341
28. Borrow RBP, Roper MH. The Immunological Basis for Immunization Series. Module 3: Tetanus Update 2006. Switzerland: WHO (2007).
29. George PJ, Kumar NP, Sridhar R, Hanna LE, Nair D, Banurekha VV, et al. Coincident helminth infection modulates systemic inflammation and immune activation in active pulmonary tuberculosis. PLoS Negl Trop Dis (2014) 8(11):e3289. doi:10.1371/journal.pntd.0003289
30. Potian JA, Rafi W, Bhatt K, McBride A, Gause WC, Salgame P. Preexisting helminth infection induces inhibition of innate pulmonary anti-tuberculosis defense by engaging the IL-4 receptor pathway. J Exp Med (2011) 208(9):1863–74. doi:10.1084/jem.20091473
31. Perry S, Hussain R, Parsonnet J. The impact of mucosal infections on acquisition and progression of tuberculosis. Mucosal Immunol (2011) 4(3):246–51. doi:10.1038/mi.2011.11
32. Lucas M, Nicol P, McKinnon E, Whidborne R, Lucas A, Thambiran A, et al. A prospective large-scale study of methods for the detection of latent Mycobacterium tuberculosis infection in refugee children. Thorax (2010) 65(5):442–8. doi:10.1136/thx.2009.127555
33. Thomas TA, Mondal D, Noor Z, Liu L, Alam M, Haque R, et al. Malnutrition and helminth infection affect performance of an interferon gamma-release assay. Pediatrics (2010) 126(6):e1522–9. doi:10.1542/peds.2010-0885
34. Diena BB, Yugi H, Wallace R, Carriere J, Greenberg L. The bentonite flocculation test in the serology of tuberculosis. I. Purification of BCG antigens. Can J Microbiol (1968) 14(8):881–5. doi:10.1139/m68-148
35. Bardana EJ Jr, McClatchy JK, Farr RS, Minden P. Universal occurrence of antibodies to tubercle bacilli in sera from non-tuberculous and tuberculous individuals. Clin Exp Immunol (1973) 13(1):65–77.
36. Parlett RC, Youmans GP. An evaluation of the specificity and sensitivity of a gel double-diffusion test for tuberculosis. Am Rev Respir Dis (1959) 80:153–66.
37. Hoft DF, Kemp EB, Marinaro M, Cruz O, Kiyono H, McGhee JR, et al. A double-blind, placebo-controlled study of Mycobacterium-specific human immune responses induced by intradermal bacille Calmette-Guerin vaccination. J Lab Clin Med (1999) 134(3):244–52. doi:10.1016/S0022-2143(99)90204-4
38. Beyazova U, Rota S, Cevheroglu C, Karsligil T. Humoral immune response in infants after BCG vaccination. Tuber Lung Dis (1995) 76(3):248–53. doi:10.1016/S0962-8479(05)80013-9
39. Goodridge HS, Ahmed SS, Curtis N, Kollmann TR, Levy O, Netea MG, et al. Harnessing the beneficial heterologous effects of vaccination. Nat Rev Immunol (2016) 16(6):392–400. doi:10.1038/nri.2016.43
40. Higgins JP, Soares-Weiser K, Lopez-Lopez JA, Kakourou A, Chaplin K, Christensen H, et al. Association of BCG, DTP, and measles containing vaccines with childhood mortality: systematic review. BMJ (2016) 355:i5170. doi:10.1136/bmj.i5170
41. Rafi W, Bhatt K, Gause WC, Salgame P. Neither primary nor memory immunity to Mycobacterium tuberculosis infection is compromised in mice with chronic enteric helminth infection. Infect Immun (2015) 83(3):1217–23. doi:10.1128/IAI.03004-14
42. Hubner MP, Killoran KE, Rajnik M, Wilson S, Yim KC, Torrero MN, et al. Chronic helminth infection does not exacerbate Mycobacterium tuberculosis infection. PLoS Negl Trop Dis (2012) 6(12):e1970. doi:10.1371/journal.pntd.0001970
43. Frantz FG, Rosada RS, Turato WM, Peres CM, Coelho-Castelo AA, Ramos SG, et al. The immune response to toxocariasis does not modify susceptibility to Mycobacterium tuberculosis infection in BALB/c mice. Am J Trop Med Hyg (2007) 77(4):691–8. doi:10.4269/ajtmh.2007.77.691
44. Monin L, Griffiths KL, Lam WY, Gopal R, Kang DD, Ahmed M, et al. Helminth-induced arginase-1 exacerbates lung inflammation and disease severity in tuberculosis. J Clin Invest (2015) 125(12):4699–713. doi:10.1172/JCI77378
45. Biraro IA, Egesa M, Toulza F, Levin J, Cose S, Joloba M, et al. Impact of co-infections and BCG immunisation on immune responses among household contacts of tuberculosis patients in a Ugandan cohort. PLoS One (2014) 9(11):e111517. doi:10.1371/journal.pone.0111517
46. Lule SA, Mawa PA, Nkurunungi G, Nampijja M, Kizito D, Akello F, et al. Factors associated with tuberculosis infection, and with anti-mycobacterial immune responses, among five year olds BCG-immunised at birth in Entebbe, Uganda. Vaccine (2015) 33(6):796–804. doi:10.1016/j.vaccine.2014.12.015
47. Ndibazza J, Mpairwe H, Webb EL, Mawa PA, Nampijja M, Muhangi L, et al. Impact of anthelminthic treatment in pregnancy and childhood on immunisations, infections and eczema in childhood: a randomised controlled trial. PLoS One (2012) 7(12):e50325. doi:10.1371/journal.pone.0050325
Keywords: Mycobacterium tuberculosis infection, antibody, enzyme-linked immunosorbent assay, Bacille Calmette-Guérin, vaccine, helminth
Citation: Logan E, Luabeya AKK, Mulenga H, Mrdjen D, Ontong C, Cunningham AF, Tameris M, McShane H, Scriba TJ, Horsnell WGC and Hatherill M (2018) Elevated IgG Responses in Infants Are Associated With Reduced Prevalence of Mycobacterium tuberculosis Infection. Front. Immunol. 9:1529. doi: 10.3389/fimmu.2018.01529
Received: 13 March 2018; Accepted: 20 June 2018;
Published: 02 July 2018
Edited by:
Ana María Hernández, Center of Molecular Immunology, CubaReviewed by:
Giovanni Delogu, Università Cattolica del Sacro Cuore, ItalyYoelys Cruz-Leal, St. Michael’s Hospital, Canada
Copyright: © 2018 Logan, Luabeya, Mulenga, Mrdjen, Ontong, Cunningham, Tameris, McShane, Scriba, Horsnell and Hatherill. This is an open-access article distributed under the terms of the Creative Commons Attribution License (CC BY). The use, distribution or reproduction in other forums is permitted, provided the original author(s) and the copyright owner are credited and that the original publication in this journal is cited, in accordance with accepted academic practice. No use, distribution or reproduction is permitted which does not comply with these terms.
*Correspondence: William G. C. Horsnell, wghorsnell@gmail.com;
Mark Hatherill, mark.hatherill@uct.ac.za
†These authors have contributed equally to this work.