- 1Emory Vaccine Center, Emory University School of Medicine, Atlanta, GA, United States
- 2Department of Microbiology and Immunology, Emory University School of Medicine, Atlanta, GA, United States
- 3Division of Infectious Disease, Department of Medicine, Emory University School of Medicine, Atlanta, GA, United States
Antigen-specific CD4+ T cell responses to Mycobacterium tuberculosis (Mtb) infection are important for host defense against tuberculosis (TB). However, Mtb-specific IFN-γ-producing T cells do not distinguish active tuberculosis (ATB) patients from individuals with asymptomatic latent Mtb infection (LTBI). We reasoned that the immune phenotype of Mtb-specific IFN-γ+CD4+ T cells could provide an indirect gauge of Mtb antigen load within individuals. We sought to identify immune markers in Mtb-specific IFN-γ+CD4+ T cells and hypothesized that expression of caspase-3 Mtb-specific CD4+ T cells would be associated with ATB. Using polychromatic flow cytometry, we evaluated the expression of caspase-3 in Mtb-specific CD4+ T cells from LTBI and ATB as well as from ATB patients undergoing anti-TB treatment. We found significantly higher frequencies of Mtb-specific caspase-3+IFN-γ+CD4+ T cells in ATB compared to LTBI. Caspase-3+IFN-γ+CD4+ T cells were also more activated compared to their caspase-3-negative counterparts. Furthermore, the frequencies of caspase-3+IFN-γ+CD4+ T cells decreased in response to anti-TB treatment. Our studies suggest that the frequencies of caspase-3-expressing antigen-specific CD4+ T cells may reflect mycobacterial burden in vivo and may be useful for distinguishing Mtb infection status along with other host biomarkers.
Introduction
Tuberculosis (TB) is one of the world’s major causes of illness and mortality (1) with about 10 million new cases and 2 million deaths occurring each year. Approximately 10% of individuals infected with Mycobacterium tuberculosis (Mtb) develop active TB (ATB), while 90% have no overt signs of clinical disease and are considered to have latent Mtb infection (LTBI) (2) indicating that the host immune response is capable of controlling infection. Several studies have shown that the majority of individuals infected with Mtb mount robust antigen-specific CD4+ T cell responses involving T helper 1 (Th1) cytokines, such as IFN-γ and TNF-α, which are critical for activating macrophages and containing bacteria in the lung. However, Th1 cytokines are not sufficient for protection against ATB disease and Mtb-specific IFN-γ-producing CD4+ T cells are present in individuals with ATB disease as well as in asymptomatic individuals with LTBI. Moreover, Mtb-specific IFN-γ-producing T cells fail to discriminate between active and LTBI (3, 4) and are not useful for assessing response to active TB treatment, which is typically monitored by sputum culture conversion (5, 6).
In an effort to identify biomarkers in human peripheral blood mononuclear cells (PBMCs) that distinguish active and LTBI states, we previously characterized the immune phenotype of Mtb-specific IFN-γ-producing CD4+ T cells in ATB and LTBI. We showed that compared to individuals with LTBI, PBMCs from ATB patients harbored significantly higher frequencies of Mtb-specific IFN-γ+CD4+ T cells expressing immune activation markers CD38 and HLA-DR and the intracellular proliferation marker Ki-67 (7). These markers accurately identified ATB patients and correlated with response to anti-TB treatment (7). Our studies showed that activated Mtb-specific IFN-γ+ producing CD4+ T cells can serve as an indirect gauge of Mtb antigen load within individuals. In this study, we extend the concept of antigen-specific T cell phenotypes as readouts of pathogen burden and investigate the expression of active caspase-3 in individuals with ATB and LTBI. Caspase-3, a member of the caspase family of cysteine proteases is expressed in CD4 effector T cells downstream of anti-CD3-mediated T cell receptor (TCR) activation (8) and has been shown to orchestrate apoptotic pathways during microbial infection following T cell activation and regulate T cell activation, cell cycle entry, proliferation, and differentiation (8–12). Since ATB patients have higher frequencies of activated Mtb-specific CD4+ T cells compared to LTBI, we hypothesized that ATB would also harbor higher frequencies of Mtb-specific CD4+ T cells expressing active caspase-3.
Using polychromatic flow cytometry, we evaluated the expression of active caspase-3 in Mtb-specific CD4+ T cells from ATB patients and individuals with LTBI. We found significantly higher frequencies of active caspase-3+IFN-γ+CD4+ T cells in ATB compared to LTBI. Further, caspase-3-expressing IFN-γ+CD4+ T cells were more activated compared to their caspase-3-negative counterparts and the frequencies of caspase-3+IFN-γ+CD4+ T cells decreased following successful anti-TB treatment, indicating that caspase-3 expression in Mtb-specific IFN-γ+CD4+ T cells is associated with mycobacterial burden.
Materials and Methods
Study Participants
This study was conducted according to the principles expressed in the Declaration of Helsinki. Ethical approval was obtained from the Emory University Institutional Review Board. All participants were provided written informed consent for the collection of samples and subsequent analyses. HIV-negative subjects between 23 and 83 years of age with LTBI (n = 23) or with pulmonary ATB disease (n = 22) were recruited in Atlanta, GA, USA. The 22 patients with confirmed pulmonary ATB were enrolled at Grady Memorial Hospital (Atlanta, GA, USA), prior to initiation of anti-TB treatment. Diagnosis of pulmonary ATB was based on the presence of clinical symptoms, sputum positivity by acid-fast bacilli (AFB) smear, positive amplified Mycobacterium Tuberculosis Direct assay, and positive culture (Table 1). All the ATB patients underwent the anti-TB treatment. However, only eight patients receiving the standard regimen of anti-TB treatment were followed longitudinally for 6 months. Anti-TB treatment was provided according to Centers for Disease Control (CDC) guidelines (13) for drug-susceptible TB and consisted of 2 months of isoniazid, rifampicin, pyrazinamide, and ethambutol, followed by 4 months of isoniazid and rifampicin. Resolution of TB was assessed by clinical, radiological, and microbiological criteria as described in Table 1. Healthy subjects from Atlanta, GA were identified as having LTBI by a positive ESAT6-CFP10-specific IFNγ-ELISPOT assay as described previously (14). These individuals were all HIV negative, non-smokers with no recent history of severe respiratory disease and had a normal chest X-ray. The presence of IFN-γ+CD4+ T cells in PBMCs from both ATB and LTBI groups was assessed by flow cytometry and intracellular cytokine staining (ICS) following stimulation with Mtb-CW antigens and ESAT6 and CFP10 peptide pools.
PBMC Isolation, Antigens, and Peptides for Cell Stimulations
Blood samples were collected from all subjects at baseline and longitudinal time points. PBMCs were isolated from blood as described previously (14) using cell preparation tubes (CPT, BD Biosciences) and cryopreserved in 90% fetal FBS (Hyclone, South Logan, UT) and 10% dimethyl sulfoxide (Sigma-Aldrich, St. Louis, MO, USA). PBMCs were stimulated with Mtb cell wall (CW) antigens (NIH-TBVRM contract, BEI) and ESAT6-CFP10 peptides pools, which were composed of 15-mers with 11 amino-acid overlap (Genemed Synthesis Inc., San Antonio, TX, USA).
Flow Cytometry and Staining
For ICS, cryopreserved PBMCs were rested overnight at 37°C, 5% CO2 in RPMI-1640 medium (Lonza, Walkersville, MD, USA) containing 10% FBS, 2 mM glutamine, 100 IU/ml penicillin, and 100 µg/ml streptomycin. The viability of the lymphocytes was 75–95%. 1–2 × 106 PBMCs were each stimulated with Mtb CW antigens (10 µg/ml; BEI Resources) and ESAT-6 and CFP-10 peptide pools (10 µg/ml) for 2 h followed by the addition of Brefeldin A (10 µg/ml) (BD Biosciences, San Diego, CA, USA) and further incubated for 16 h. PBMCs were stained for dead cells with the LIVE/DEAD Fixable Yellow Dead Cell Stain (Life Technologies, OR) at the beginning, and then surface-stained with appropriate antibodies: CD4 PerCp-Cy5.5 (clone L200), CD8 V500 (clone SK1), HLA-DR PE-Cy7 (clone L243), all from BD Biosciences, CD38 ECD (clone LS198.4.3) from Beckman-Coulter (Fullerton, CA, USA), CD45RA BV711 (clone HI100) from Biolegend (San Diego, CA, USA); permeabilized with Cytofix/Cytoperm Kit (BD Biosciences), stained intracellularly with appropriate antibodies: active caspase-3 FITC (clone C92-605), IFN-γ Alexa Fluor 700 (clone B27), IL-2-APC (clone MQ1-17H12), Ki-67 PE (clone B56), and CD3 APC-H7 (clone UCHT1), all from BD Biosciences, TNF-α BV650 (Clone MAb11, Biolegend); and fixed with 1% paraformaldehyde before acquisition on an LSR-II flow cytometry (BD Biosciences). Flow cytometry data were analyzed with FlowJo software (Tree Star Inc., San Carlos, CA, USA). Positive Mtb-specific CD4+ T cell responses were defined by a frequency of CD4+IFN-γ+ T cells of ≥0.05%. The minimum number of CD4+IFN-γ+ T cells used in this study to assess caspase-3 expression was 175 events.
Statistical Analysis
Data were analyzed using Graphpad Prism 6.0b software. The Mann–Whitney U test was used to compare two groups. The means was used for descriptive statistics for each parameter. Differences between paired samples were analyzed using the Wilcoxon matched-paired rank test. A P-value of less than 0.05 was considered to be statistically significant.
Results
Expression of Caspase-3 in Mtb-Specific CD4+ T Cells in Individuals With ATB and LTBI
Gating on live lymphocytes (Figure S1 in Supplementary Material), we evaluated the expression of active caspase-3 on IFN-γ+CD4+ T cells in 22 ATB patients and 23 healthy subjects with LTBI (Table 1), after stimulating PBMCs with Mtb-CW antigens and ESAT6-CFP10 peptides pools. A representative flow cytometry plot from one ATB patient and one LTBI subject (Figure 1A) and the summarized data (Figure 1B) show that ATB patients harbor significantly higher frequencies of Mtb-specific IFN-γ+CD4+ T cells expressing caspase-3 compared to LTBI (9.1 vs 0.1, p < 0.0001 with Mtb CW antigens; 4.1 vs 0.1, p < 0.0001 with ESAT6-CFP10 peptides pools). These differences were restricted to antigen-specific IFN-γ+CD4+ T cells as expression of caspase-3 in bulk, non-stimulated populations of CD4+ T cells was similar in both groups (Figure S2 in Supplementary Material).
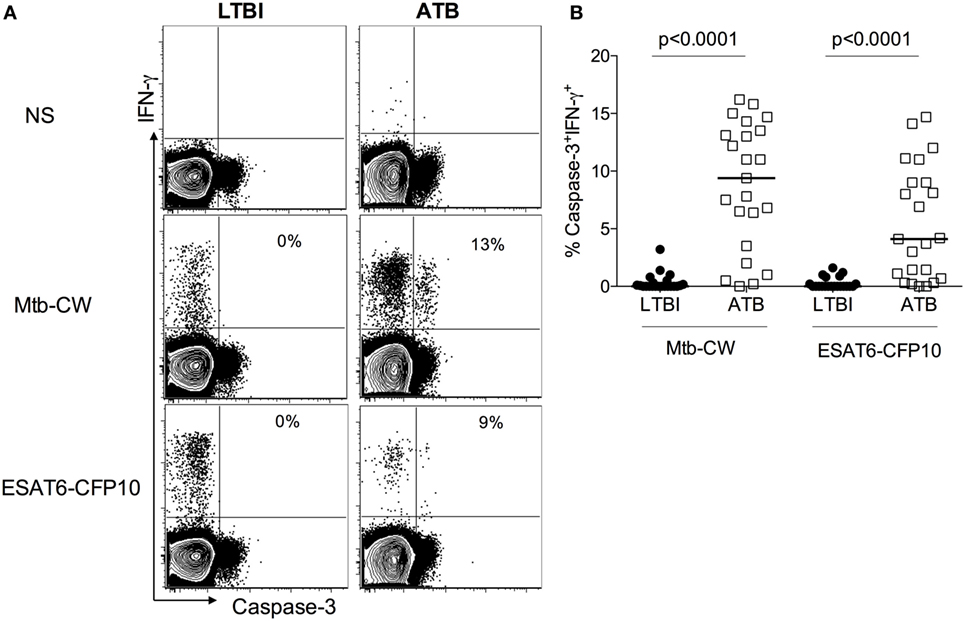
Figure 1. Caspase-3 expression on Mycobacterium tuberculosis (Mtb)-specific IFN-γ+CD4+ T cells differentiates between active tuberculosis (ATB) and latent Mtb infection (LTBI). Peripheral blood mononuclear cells from individuals with LTBI and ATB were stimulated with Mtb cell wall antigens and ESAT6-CFP10 peptide pools or non-stimulated (NS) (A) Representative flow plots for one active tuberculosis (ATB) and one LTBI individual and (B) cumulative data for ATB (n = 22) and LTBI (n = 23) groups. The frequencies of caspase-3+IFN-γ+ T cells were compared between LTBI and ATB groups. Mann–Whitney U test was used to compare the two groups. A P-value of less than 0.05 was considered to be statistically significant. Bars represent means.
Caspase-3+IFN-γ+CD4+ T Cells in ATB Patients Display an Activated Effector Phenotype
Since LTBI individuals do not express caspase-3+IFN-γ+CD4+ T cells (Figure 1), to assess the activation state of caspase-3+IFN-γ+CD4+ T cells in ATB, we examined the expression of immune activation markers CD38, HLA-DR, and the intracellular proliferative marker Ki-67 in caspase-3+IFN-γ+ and caspase-3−IFN-γ+CD4+ T cells. We found that caspase-3+IFN-γ+CD4+ T cells express higher levels of CD38 (75 vs 55%, p = 0.019), HLA-DR (98 vs 80%), and Ki-67 (40 vs 20%) compared to caspase-3−IFN-γ+CD4+ T cells (Figure 2) upon stimulation with Mtb-CW antigens. These data show that the expression of caspase-3 in Mtb-specific CD4+ T cells is associated with an activated cycling state. To assess the differentiation state associated with caspase-3+IFN-γ+CD4+ T cells, we analyzed the expression of CD27, CD45RA, and CD127. Caspase-3+IFN-γ+CD4+ T cells were characterized by mostly CD27−CD45RA−CD127− cells indicating an effector phenotype (Figure 3).
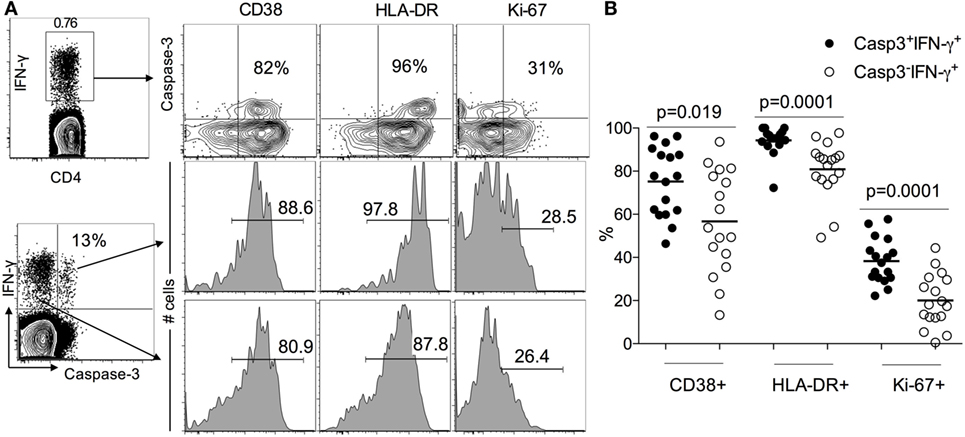
Figure 2. Caspase-3+IFN-γ+CD4+ T cells are more activated than caspase-3−IFN-γ+CD4+ T in ATB patients. (A) Representative flow plots for one patient and (B) cumulative data for 17 patients. The frequencies of immune activation markers CD38, HLA-DR, and the intracellular proliferative marker Ki-67 were compared between caspase-3+IFN-γ+ and caspase-3− IFN-γ+CD4+ T cells. Mann–Whitney U test was used to compare the two groups. A P-value of less than 0.05 was considered to be statistically significant. Bars represent means.
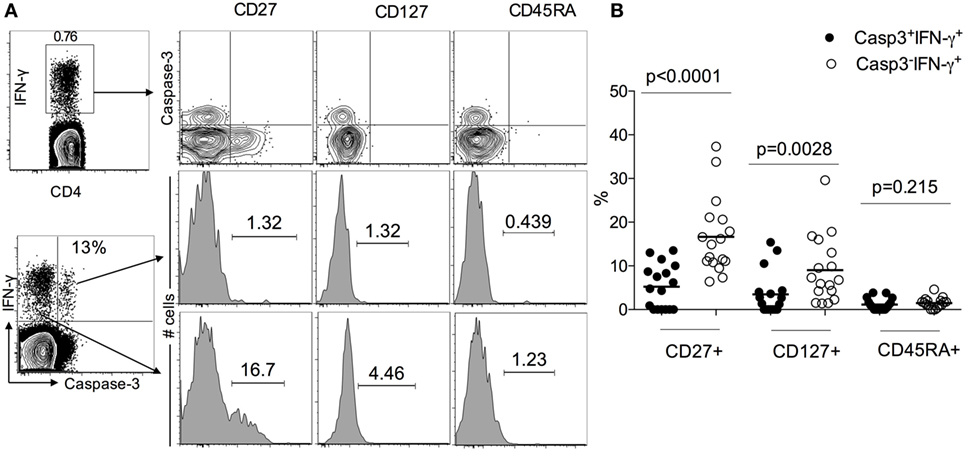
Figure 3. Caspase-3+IFN-γ+CD4+ T cells display more effector phenotype than caspase-3− IFN-γ+CD4+ T in active tuberculosis patients. (A) Representative flow plots for one patient and (B) cumulative data for 17 patients. The frequencies of the memory differentiation markers CD27, CD127, and CD45RA were compared between caspase-3+IFN-γ+ and caspase-3−IFN-γ+CD4+ T cells. Mann–Whitney U test was used to compare the two groups. A P-value of less than 0.05 was considered to be statistically significant. Bars represent means.
Reduced IL-2 Production in Mtb-Specific Caspase-3+IFN-γ+ Compared to Caspase-3−IFN-γ+CD4+ T Cells in ATB Patients
Several studies have demonstrated the presence of functional Mtb-specific CD4+ T cell responses in treatment-naïve pulmonary TB patients by assessing the production of IL-2, TNF-α, and IFN-γ in PBMCs stimulated with Mtb antigens. To evaluate the functionality of caspase 3-expressing Mtb-specific CD4 T cells, we investigated the expression of IL-2 and TNF-α in caspase-3+IFN-γ+ and caspase-3−IFN-γ+ CD4 T cell subsets. Both caspase-3+ and caspase-3− subsets exhibited polyfunctional responses, as seen by their capacity to produce IFN-γ, TNF-α, and IL-2 in response to Mtb antigen stimulation (Figure 4). However, caspase-3+IFN-γ+CD4+ T cells exhibited lower levels of IL-2 compared to caspase-3−IFN-γ+CD4+ T cells (40 vs 55%, p = 0.003; Figures 5A,B). Thus, caspase-3 expression in Mtb-specific CD4+ T cells in ATB is associated with reduced IL-2 levels.
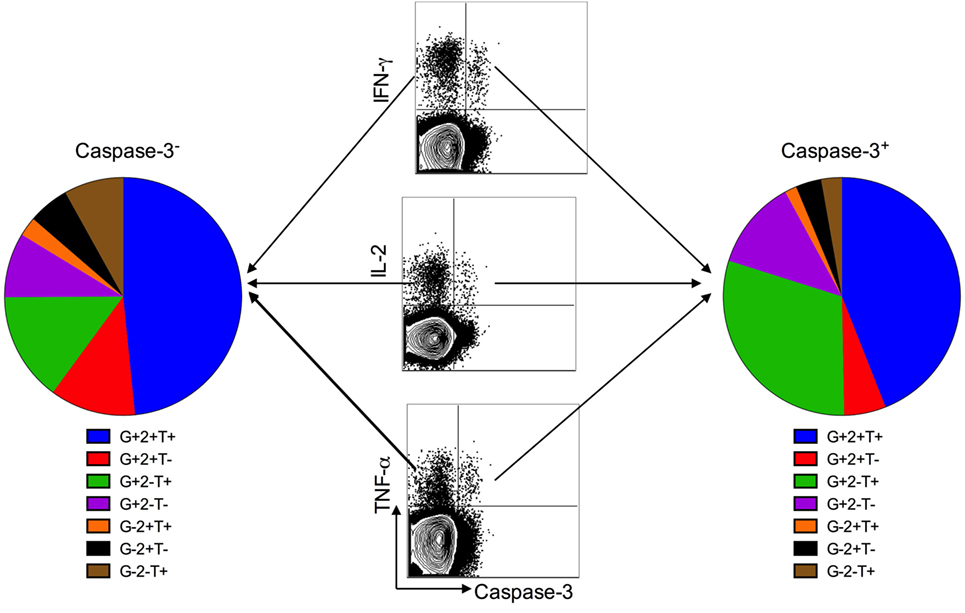
Figure 4. Caspase-3+ and caspase-3− antigen-specific CD4+ T cell subsets are polyfunctional. Polyfunctional cytokine responses from caspase-3+ and caspase-3− antigen-specific CD4+ T cell subsets in active tuberculosis patients. Data are represented as the percentage of responding CD4+ T cells that are triple producers, double producers, or single producers of IFN-γ (G), TNF-α (T), and IL-2 (2) and summarized by the pie charts. Each slice of the pie represents the fractions of the total response that consists of CD4+ T cells positive for a given function.
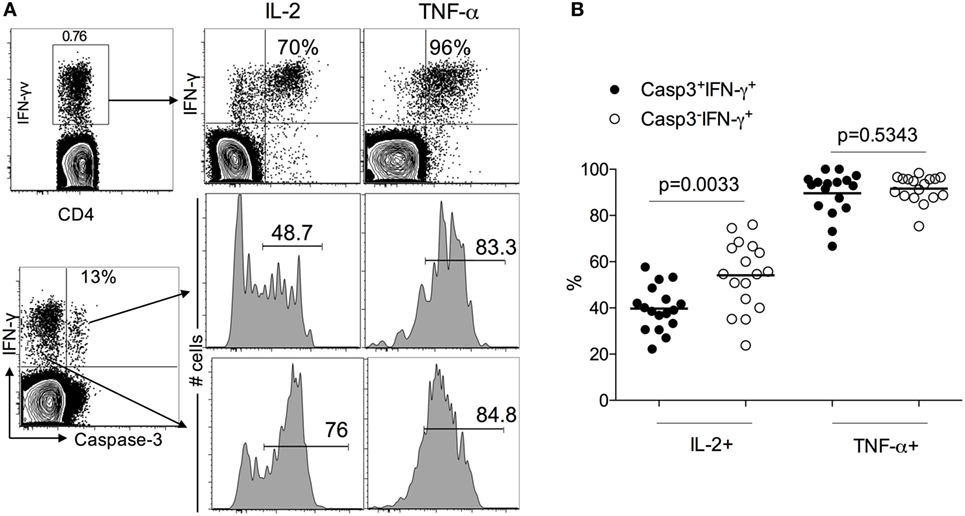
Figure 5. IL-2 and TNF-a expression caspase-3+ and caspase-3− antigen-specific CD4+ T cell subsets in active tuberculosis patients. (A) Representative flow plots for one patient and (B) cumulative data for 17 patients.
Frequencies of Mtb-Specific Caspase-3+IFN-γ+ CD4 T Cells Decrease Following Successful Anti-TB Treatment
The standard treatment regimen for TB consists of a 2-month intensive phase with isoniazid, rifampicin, pyrazinamide, and ethambutol (HRZE) followed by a month-continuation phase with isoniazid and rifampicin (HR) (15). To investigate whether frequencies of caspase-3+IFN-γ+CD4+ T cells in treatment-naive ATB patients are altered following anti-TB treatment, we compared treatment-naïve ATB patients with those who successfully completed 6 months of anti-TB treatment (n = 8) by assessing frequencies of caspase-3+IFN-γ+CD4+ T cells in PBMCs stimulated with Mtb-CW and ESAT6-CFP10. The frequencies of caspase-3+IFN-γ+CD4+ T cells were significantly lower in the treated ATB group (ATB treated-6 months) compared with untreated ATB group (Figure 6). We next assessed caspase-3+IFN-γ+ CD4+ T cell frequencies at baseline (time 0) and at multiple time points after treatment initiation for a subset of individuals for whom we had longitudinal samples (n = 8). Baseline diagnosis by sputum AFB smear and culture is indicated for each patient, and sputum was monitored for AFB smear and culture conversion during treatment (Figure 7). Conversion to a negative sputum culture at 2 months after the initiation is currently the most objective indicator of response to treatment (15). Caspase-3+IFN-γ+CD4+ T cells were relatively prevalent until 30–60 days after anti-TB treatment, after which their levels were greatly reduced (Figures 7A–C). Overall, these data show that, compared to treatment naive ATB patients, the frequencies of caspase-3+IFN-γ+CD4+ T cells were significantly reduced after successful completion of the 6-month standard regimen of anti-TB treatment (Figures 6 and 7). These data suggest that reduction in mycobacterial burdens during successful anti-TB treatment correlates with reduced frequencies of caspase-3 in Mtb-specific IFN-γ+CD4+ T cells.
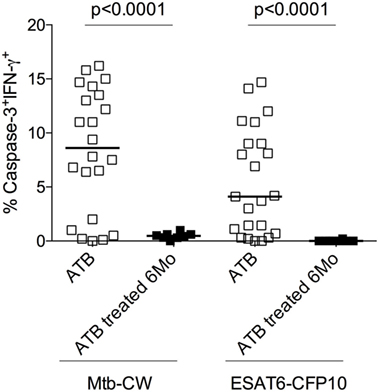
Figure 6. Frequencies of caspase-3+ Mycobacterium tuberculosis (Mtb)-specific CD4+ T cells at baseline and 6 months after anti-TB treatment. Analysis of the frequencies of caspase-3+IFN-γ+CD4+ T cells in individuals with treatment-naive active tuberculosis (ATB) (n = 23) as well as those who received 6 months of anti-TB treatment (ATB treated 6 months; n = 8). Mann–Whitney U test was used to compare the two groups. A P-value of less than 0.05 was considered to be statistically significant. Bars represent means.
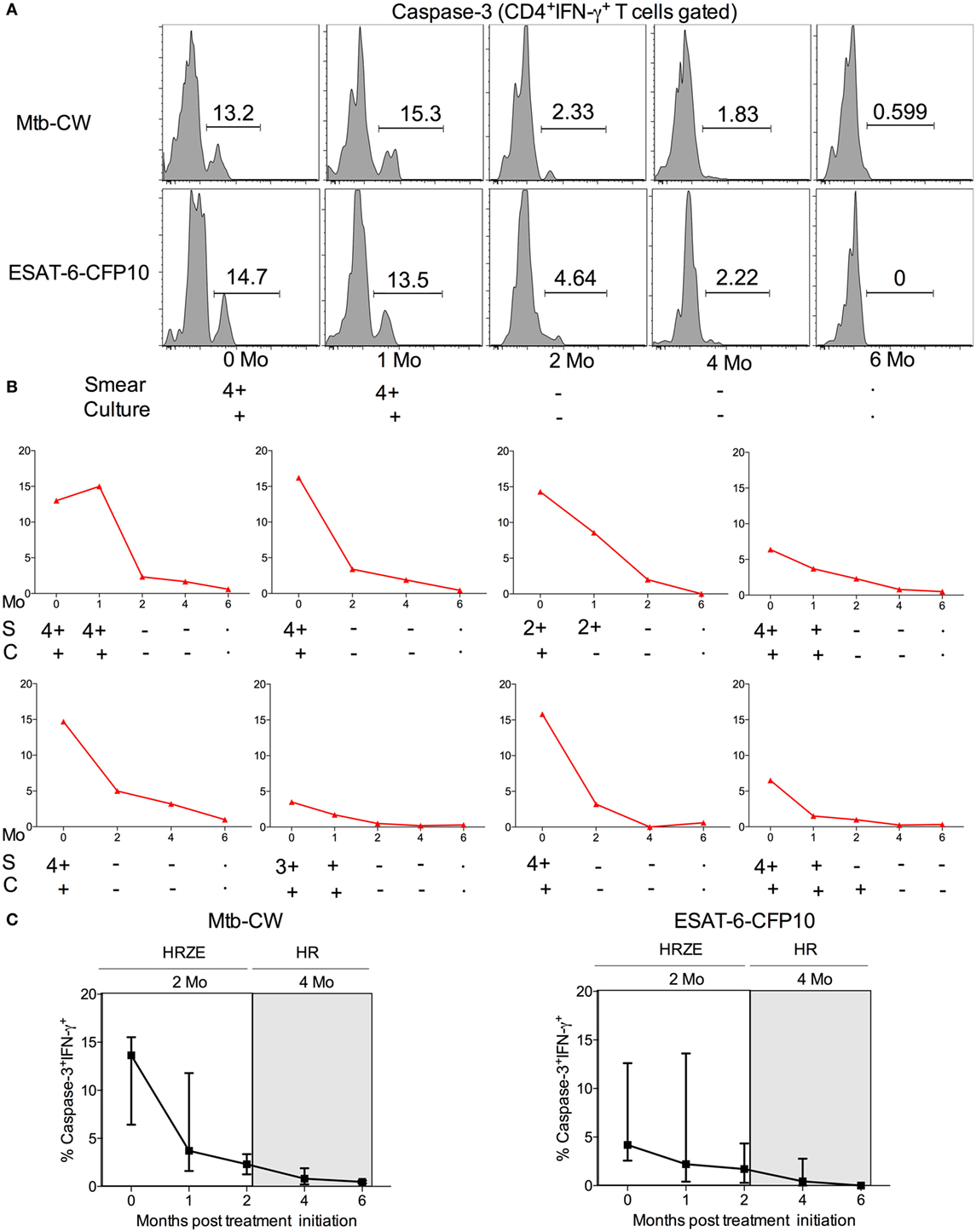
Figure 7. Comparison of the frequencies of caspase-3+IFN-γ+CD4+ T cells in 7 TB patients undergoing the 6-month regimen of anti-TB treatment. (A) Representative flow plots for one patient, (B) frequencies of caspase-3+IFN-γ+CD4+ T cells in eight different ATB patients over the course of anti-TB treatment after stimulation with Mtb-CW, and (C) cumulative data for eight patients are shown for the frequencies of caspase-3+IFN-γ+ T cells. The 2-month intensive phase (HRZE) and 4-month continuation phase (HR) are indicated. Abbreviations: S = sputum smear, C = culture.
Discussion
Several studies have demonstrated that the immune phenotypes of antigen-specific T cells, including the expression of activation markers, their maturation, and differentiation states and cytokine profiles, generally reflects the antigen burden within individuals infected with viral and bacterial pathogens (7, 16–18). Distinct T cell phenotypes have been associated with active pulmonary TB, where antigen burdens are typically high, compared to persistent or chronic infections (LTBI) with low antigenic burdens or after treatment-induced clearance of infection (7, 14, 19–28). We previously showed that frequencies of Mtb-specific IFN-γ+CD4+ T cells expressing each of the immune activation markers CD38 and HLA-DR and the intracellular proliferation marker Ki-67, accurately classified ATB and LTBI status and correlated with decreasing mycobacterial loads during treatment (7). In this study, we extended the concept of antigen-specific T cell phenotypes as indirect readouts of pathogen burden and investigated the expression of active caspase-3 in activated Mtb-specific CD4+ T cells. Caspase-3 is known to be a major executor of apoptosis in antigen-stimulated T cells (29). However, several reports have suggested that caspase-3 might have an additional role in the immune system by promoting lymphocyte activation and proliferation (8–11, 30). For example, in studies on acute LCMV infection in mice, caspase-3 mRNA levels were shown to be selectively increased in peripheral T cells after antigen-specific stimulation (9) or following TCR stimulation (31). It has also been shown that caspase-3 is expressed in non-apoptotic T lymphocytes (32–34). In this study and gating on live lymphocytes, we showed higher frequencies of caspase-3-expressing Mtb-specific IFN-γ+CD4+ T cells in individuals with ATB compared to LTBI subjects, suggesting that the caspase-3 pathway is operant during active TB phase but not during the latent phase. We also showed that the caspase-3-expressing Mtb-specific IFN-γ+CD4+ T cells express high levels of T cell activation markers CD38 and HLA-DR and the proliferation marker Ki-67 (Figure 2), suggesting that caspase-3 expression is associated with activation and proliferation of CD4+ T cells during active TB disease. Indeed, caspase-3 has been shown to be involved in regulating early steps of lymphocyte activation, cell cycle entry, and proliferation (8, 35). The importance of caspases in T cell activation is also highlighted by defective T cell activation observed in humans lacking functional caspase-8, and caspase blockers have been shown to inhibit human T cells proliferation in response to various antigen stimulations (36, 37). It has recently been shown that the activation profile of Mtb-specific CD4+ T cells as assessed by the expression of CD38, HLA-DR, and Ki-67 reflects TB disease in both HIV infected and uninfected individuals (24). It is interesting to speculate that expression of caspase-3 in Mtb-specific CD4+ T cells from HIV-infected ATB patients will be similarly higher compared to HIV infected individuals with LTBI despite the reduction of peripheral CD4+ T cells during HIV infection (25, 38, 39).
Our observation of reduced IL-2 production in Mtb-specific caspase-3+ CD4+ T cells compared to caspase-3− CD4+ T cells (Figure 5) suggests that caspase-3 expression may also be associated with reduced proliferative capacity in TB (19). Further, the functional differences between caspase-3+ and caspase-3− CD4+ T cells suggested by this study may be explained by the maturation phenotype of the cells. Caspase-3-expressing Mb-specific CD4+ T cells exhibited a more differentiated effector T cell phenotype (CD27−CD45RA−CD127−) (Figure 3) in ATB patients (40–43). Expression of the costimulatory molecule CD27 on Mtb-specific T cells has been shown to identify ATB status in both adults (21, 26) and children (44). Whether caspase-3 expression in Mtb-specific T cells, along with CD38, HLA-DR, and Ki-67, will be useful as biomarkers for diagnosing TB in children needs further investigation.
A number of immunological markers measured in the blood before and after anti-TB treatment, have shown promising results as prognostic markers of clinical severity and/or predictors of microbiological outcome in TB patients (45–47). However, the majority of these studies have been focused on the plasma. Further, Berry et al. identified an IFN-inducible neutrophil-driven transcriptional signature that was associated with ATB disease and correlated with treatment response (48). We examined the effect of anti-TB treatment on the frequencies of caspase-3 expressing Mtb-specific IFN-γ+CD4+ T cells in active TB patients and found that treatment-mediated resolution was associated with the decline of antigen-specific caspase-3+IFN-γ+CD4+ T cells which correlate with Mtb burden in vivo using sputum smear and culture measurement (Figures 6 and 7). This is similar to what was reported in HIV-infected individuals where anti-retroviral therapy led to reduced frequencies of HIV-1-specific caspase-3 expressing CD4+ T cells (49). Overall, our studies show that frequencies of caspase-3 expressing antigen-specific CD4+ T cells may reflect mycobacterial burden in vivo and could, therefore, be useful for assessing anti-TB treatment response along with other host biomarkers (50–53).
Ethics Statement
This study was conducted according to the principles expressed in the Declaration of Helsinki. Ethical approval was obtained from the Emory University Institutional Review Board. All participants were provided written informed consent for the collection of samples and subsequent analyses.
Author Contributions
TA and JR conceived the study design. TA, SC, and SR recruited cohorts that were used in this study. TA, CI, SR, and JR acquired and/or analyzed and interpreted the data. TA and JR wrote the manuscript with assistance from CI. All authors reviewed, revised, and approved the manuscript for submission.
Conflict of Interest Statement
The authors declare that the research was conducted in the absence of any commercial or financial relationships that could be construed as a potential conflict of interest.
Acknowledgments
We thank the volunteers who participated in this study and the staff at Grady Memorial Hospital and the Fulton County Health Department. We gratefully acknowledge Sohni Gill for technical assistance.
Funding
This work was supported by the following funding sources: an Emory-CFAR R03 Developmental grant (to TA) from Core grant P30AI050409, a pilot project grant awarded (to JR) from the NIH CCHI Grant AI057266, an Emory Global Health Institute Faculty Distinction Award (to JR), and the Yerkes National Primate Center base grant RR000165, a Center For AIDS Research (CFAR) Immunology Core grant P30AI050409 (to Emory University).
Supplementary Material
The Supplementary Material for this article can be found online at https://www.frontiersin.org/articles/10.3389/fimmu.2018.01481/full#supplementary-material.
Figure S1. Representative gating strategy derived from total peripheral blood mononuclear cells (PBMCs): Cell doublets were excluded from the total population of PBMCs using forward scatter area (FSC-A) and height (FSC-H); the lymphocyte population was gated according to FSC-A and size scatter area, the dead lymphocytes were excluded using live-dead staining; CD3+ T cells were gated, followed by CD4+ T cells gating to assess the IFN-γ+ T cells.
Figure S2. Caspase-3 expression on bulk CD4+ T cells from latent Mtb infection (LTBI) and treatment-naïve active tuberculosis (ATB). (A) Representative flow plots for one ATB and one LTBI individual and (B) cumulative data for ATB (n = 22) and LTBI (n = 23) groups. Data were derived from non-stimulated peripheral blood mononuclear cells. Mann–Whitney U test was used to compare the two groups. A P-value of less than 0.05 was considered to be statistically significant.
References
2. Andersen P. Vaccine strategies against latent tuberculosis infection. Trends Microbiol (2007) 15(1):7–13. doi:10.1016/j.tim.2006.11.008
3. Meier T, Eulenbruch HP, Wrighton-Smith P, Enders G, Regnath T. Sensitivity of a new commercial enzyme-linked immunospot assay (T SPOT-TB) for diagnosis of tuberculosis in clinical practice. Eur J Clin Microbiol Infect Dis (2005) 24(8):529–36. doi:10.1007/s10096-005-1377-8
4. Janssens JP, Roux-Lombard P, Perneger T, Metzger M, Vivien R, Rochat T. Quantitative scoring of an interferon-gamma assay for differentiating active from latent tuberculosis. Eur Respir J (2007) 30(4):722–8. doi:10.1183/09031936.00028507
5. Wallis RS, Peppard T, Hermann D. Month 2 culture status and treatment duration as predictors of recurrence in pulmonary tuberculosis: model validation and update. PLoS One (2015) 10(4):e0125403. doi:10.1371/journal.pone.0125403
6. Wallis RS. Sputum culture conversion as a tuberculosis biomarker: a glass half empty or half full? Lancet Respir Med (2015) 3(3):174–5. doi:10.1016/S2213-2600(15)00058-2
7. Adekambi T, Ibegbu CC, Cagle S, Kalokhe AS, Wang YF, Hu Y, et al. Biomarkers on patient T cells diagnose active tuberculosis and monitor treatment response. J Clin Invest (2015) 125(5):1827–38. doi:10.1172/JCI77990
8. Alam A, Cohen LY, Aouad S, Sekaly RP. Early activation of caspases during T lymphocyte stimulation results in selective substrate cleavage in nonapoptotic cells. J Exp Med (1999) 190(12):1879–90. doi:10.1084/jem.190.12.1879
9. Sabbagh L, Kaech SM, Bourbonniere M, Woo M, Cohen LY, Haddad EK, et al. The selective increase in caspase-3 expression in effector but not memory T cells allows susceptibility to apoptosis. J Immunol (2004) 173(9):5425–33. doi:10.4049/jimmunol.173.9.5425
10. Algeciras-Schimnich A, Barnhart BC, Peter ME. Apoptosis-independent functions of killer caspases. Curr Opin Cell Biol (2002) 14(6):721–6. doi:10.1016/S0955-0674(02)00384-8
11. Puga I, Rao A, Macian F. Targeted cleavage of signaling proteins by caspase 3 inhibits T cell receptor signaling in anergic T cells. Immunity (2008) 29(2):193–204. doi:10.1016/j.immuni.2008.06.010
12. Wall DM, McCormick BA. Bacterial secreted effectors and caspase-3 interactions. Cell Microbiol (2014) 16(12):1746–56. doi:10.1111/cmi.12368
13. Blumberg HM, Burman WJ, Chaisson RE, Daley CL, Etkind SC, Friedman LN, et al. American Thoracic Society/Centers for Disease Control and Prevention/Infectious Diseases Society of America: treatment of tuberculosis. Am J Respir Crit Care Med (2003) 167(4):603–62. doi:10.1164/rccm.167.4.603
14. Adekambi T, Ibegbu CC, Kalokhe AS, Yu T, Ray SM, Rengarajan J. Distinct effector memory CD4+ T cell signatures in latent Mycobacterium tuberculosis infection, BCG vaccination and clinically resolved tuberculosis. PLoS One (2012) 7(4):e36046. doi:10.1371/journal.pone.0036046
15. World Health Organization. Treatment of Tuberculosis: Guidelines. 4th ed. Geneva: World Health Organization Document (2010). p. 1–147.
16. Jones RB, Ndhlovu LC, Barbour JD, Sheth PM, Jha AR, Long BR, et al. Tim-3 expression defines a novel population of dysfunctional T cells with highly elevated frequencies in progressive HIV-1 infection. J Exp Med (2008) 205(12):2763–79. doi:10.1084/jem.20081398
17. Kaufmann DE, Kavanagh DG, Pereyra F, Zaunders JJ, Mackey EW, Miura T, et al. Upregulation of CTLA-4 by HIV-specific CD4+ T cells correlates with disease progression and defines a reversible immune dysfunction. Nat Immunol (2007) 8(11):1246–54. doi:10.1038/ni1515
18. Appay V, Dunbar PR, Callan M, Klenerman P, Gillespie GM, Papagno L, et al. Memory CD8+ T cells vary in differentiation phenotype in different persistent virus infections. Nat Med (2002) 8(4):379–85. doi:10.1038/nm0402-379
19. Day CL, Abrahams DA, Lerumo L, Janse van Rensburg E, Stone L, O’Rie T, et al. Functional capacity of Mycobacterium tuberculosis-specific T cell responses in humans is associated with mycobacterial load. J Immunol (2011) 187(5):2222–32. doi:10.4049/jimmunol.1101122
20. Day CL, Moshi ND, Abrahams DA, van Rooyen M, O’Rie T, de Kock M, et al. Patients with tuberculosis disease have Mycobacterium tuberculosis-specific CD8 T cells with a pro-apoptotic phenotype and impaired proliferative capacity, which is not restored following treatment. PLoS One (2014) 9(4):e94949. doi:10.1371/journal.pone.0094949
21. Streitz M, Tesfa L, Yildirim V, Yahyazadeh A, Ulrichs T, Lenkei R, et al. Loss of receptor on tuberculin-reactive T-cells marks active pulmonary tuberculosis. PLoS One (2007) 2(1):e735. doi:10.1371/journal.pone.0000735
22. Schuetz A, Haule A, Reither K, Ngwenyama N, Rachow A, Meyerhans A, et al. Monitoring CD27 expression to evaluate Mycobacterium tuberculosis activity in HIV-1 infected individuals in vivo. PLoS One (2011) 6(11):e27284. doi:10.1371/journal.pone.0027284
23. Riou C, Gray CM, Lugongolo M, Gwala T, Kiravu A, Deniso P, et al. A subset of circulating blood mycobacteria-specific CD4 T cells can predict the time to Mycobacterium tuberculosis sputum culture conversion. PLoS One (2014) 9(7):e102178. doi:10.1371/journal.pone.0102178
24. Wilkinson KA, Oni T, Gideon HP, Goliath R, Wilkinson RJ, Riou C. Activation profile of Mycobacterium tuberculosis-specific CD4(+) T cells reflects disease activity irrespective of HIV status. Am J Respir Crit Care Med (2016) 193(11):1307–10. doi:10.1164/rccm.201601-0116LE
25. Riou C, Berkowitz N, Goliath R, Burgers WA, Wilkinson RJ. Analysis of the phenotype of Mycobacterium tuberculosis-specific CD4+ T cells to discriminate latent from active tuberculosis in HIV-uninfected and HIV-infected individuals. Front Immunol (2017) 8:968. doi:10.3389/fimmu.2017.00968
26. Petruccioli E, Petrone L, Vanini V, Cuzzi G, Navarra A, Gualano G, et al. Assessment of CD27 expression as a tool for active and latent tuberculosis diagnosis. J Infect (2015) 71(5):526–33. doi:10.1016/j.jinf.2015.07.009
27. Harari A, Rozot V, Enders FB, Perreau M, Stalder JM, Nicod LP, et al. Dominant TNF-alpha+ Mycobacterium tuberculosis-specific CD4+ T cell responses discriminate between latent infection and active disease. Nat Med (2011) 17(3):372–6. doi:10.1038/nm.2299
28. Pollock KM, Whitworth HS, Montamat-Sicotte DJ, Grass L, Cooke GS, Kapembwa MS, et al. T-cell immunophenotyping distinguishes active from latent tuberculosis. J Infect Dis (2013) 208(6):952–68. doi:10.1093/infdis/jit265
29. Elmore S. Apoptosis: a review of programmed cell death. Toxicol Pathol (2007) 35(4):495–516. doi:10.1080/01926230701320337
30. McComb S, Mulligan R, Sad S. Caspase-3 is transiently activated without cell death during early antigen driven expansion of CD8(+) T cells in vivo. PLoS One (2010) 5(12):e15328. doi:10.1371/journal.pone.0015328
31. Sabbagh L, Bourbonniere M, Sekaly RP, Cohen LY. Selective up-regulation of caspase-3 gene expression following TCR engagement. Mol Immunol (2005) 42(11):1345–54. doi:10.1016/j.molimm.2004.12.011
32. Wilhelm S, Wagner H, Hacker G. Activation of caspase-3-like enzymes in non-apoptotic T cells. Eur J Immunol (1998) 28(3):891–900. doi:10.1002/(SICI)1521-4141(199803)28:03<891::AID-IMMU891>3.0.CO;2-X
33. Miossec C, Dutilleul V, Fassy F, Diu-Hercend A. Evidence for CPP32 activation in the absence of apoptosis during T lymphocyte stimulation. J Biol Chem (1997) 272(21):13459–62. doi:10.1074/jbc.272.21.13459
34. Kuranaga E, Miura M. Nonapoptotic functions of caspases: caspases as regulatory molecules for immunity and cell-fate determination. Trends Cell Biol (2007) 17(3):135–44. doi:10.1016/j.tcb.2007.01.001
35. Kennedy NJ, Kataoka T, Tschopp J, Budd RC. Caspase activation is required for T cell proliferation. J Exp Med (1999) 190(12):1891–6. doi:10.1084/jem.190.12.1891
36. Chun HJ, Zheng L, Ahmad M, Wang J, Speirs CK, Siegel RM, et al. Pleiotropic defects in lymphocyte activation caused by caspase-8 mutations lead to human immunodeficiency. Nature (2002) 419(6905):395–9. doi:10.1038/nature01063
37. Falk M, Ussat S, Reiling N, Wesch D, Kabelitz D, Adam-Klages S. Caspase inhibition blocks human T cell proliferation by suppressing appropriate regulation of IL-2, CD25, and cell cycle-associated proteins. J Immunol (2004) 173(8):5077–85. doi:10.4049/jimmunol.173.8.5077
38. Geldmacher C, Schuetz A, Ngwenyama N, Casazza JP, Sanga E, Saathoff E, et al. Early depletion of Mycobacterium tuberculosis-specific T helper 1 cell responses after HIV-1 infection. J Infect Dis (2008) 198(11):1590–8. doi:10.1086/593017
39. Bunjun R, Riou C, Soares AP, Thawer N, Muller TL, Kiravu A, et al. Effect of HIV on the frequency and number of Mycobacterium tuberculosis-specific CD4+ T cells in blood and airways during latent M. tuberculosis infection. J Infect Dis (2017) 216(12):1550–60. doi:10.1093/infdis/jix529
40. Appay V, van Lier RA, Sallusto F, Roederer M. Phenotype and function of human T lymphocyte subsets: consensus and issues. Cytometry A (2008) 73(11):975–83. doi:10.1002/cyto.a.20643
41. Sallusto F, Geginat J, Lanzavecchia A. Central memory and effector memory T cell subsets: function, generation, and maintenance. Annu Rev Immunol (2004) 22:745–63. doi:10.1146/annurev.immunol.22.012703.104702
42. Sallusto F, Lanzavecchia A. Heterogeneity of CD4+ memory T cells: functional modules for tailored immunity. Eur J Immunol (2009) 39(8):2076–82. doi:10.1002/eji.200939722
43. Stubbe M, Vanderheyde N, Pircher H, Goldman M, Marchant A. Characterization of a subset of antigen-specific human central memory CD4+ T lymphocytes producing effector cytokines. Eur J Immunol (2008) 38(1):273–82. doi:10.1002/eji.200737611
44. Portevin D, Moukambi F, Clowes P, Bauer A, Chachage M, Ntinginya NE, et al. Assessment of the novel T-cell activation marker-tuberculosis assay for diagnosis of active tuberculosis in children: a prospective proof-of-concept study. Lancet Infect Dis (2014) 14(10):931–8. doi:10.1016/S1473-3099(14)70884-9
45. Riou C, Perez Peixoto B, Roberts L, Ronacher K, Walzl G, Manca C, et al. Effect of standard tuberculosis treatment on plasma cytokine levels in patients with active pulmonary tuberculosis. PLoS One (2012) 7(5):e36886. doi:10.1371/journal.pone.0036886
46. Jacobs R, Malherbe S, Loxton AG, Stanley K, van der Spuy G, Walzl G, et al. Identification of novel host biomarkers in plasma as candidates for the immunodiagnosis of tuberculosis disease and monitoring of tuberculosis treatment response. Oncotarget (2016) 7(36):57581–92. doi:10.18632/oncotarget.11420
47. Djoba Siawaya JF, Bapela NB, Ronacher K, Veenstra H, Kidd M, Gie R, et al. Immune parameters as markers of tuberculosis extent of disease and early prediction of anti-tuberculosis chemotherapy response. J Infect (2008) 56(5):340–7. doi:10.1016/j.jinf.2008.02.007
48. Berry MP, Graham CM, McNab FW, Xu Z, Bloch SA, Oni T, et al. An interferon-inducible neutrophil-driven blood transcriptional signature in human tuberculosis. Nature (2010) 466(7309):973–7. doi:10.1038/nature09247
49. Yue FY, Kovacs CM, Dimayuga RC, Gu XX, Parks P, Kaul R, et al. Preferential apoptosis of HIV-1-specific CD4+ T cells. J Immunol (2005) 174(4):2196–204. doi:10.4049/jimmunol.174.4.2196
50. Ferrian S, Manca C, Lubbe S, Conradie F, Ismail N, Kaplan G, et al. A combination of baseline plasma immune markers can predict therapeutic response in multidrug resistant tuberculosis. PLoS One (2017) 12(5):e0176660. doi:10.1371/journal.pone.0176660
51. Walzl G, Ronacher K, Hanekom W, Scriba TJ, Zumla A. Immunological biomarkers of tuberculosis. Nat Rev Immunol (2011) 11(5):343–54. doi:10.1038/nri2960
52. Wallis RS, Doherty TM, Onyebujoh P, Vahedi M, Laang H, Olesen O, et al. Biomarkers for tuberculosis disease activity, cure, and relapse. Lancet Infect Dis (2009) 9(3):162–72. doi:10.1016/S1473-3099(09)70042-8
Keywords: tuberculosis, caspase-3+IFN-γ+CD4+ T cells, active tuberculosis, latent Mtb infection, anti-tuberculosis treatment, monitoring
Citation: Adekambi T, Ibegbu CC, Cagle S, Ray SM and Rengarajan J (2018) High Frequencies of Caspase-3 Expressing Mycobacterium tuberculosis-Specific CD4+ T Cells Are Associated With Active Tuberculosis. Front. Immunol. 9:1481. doi: 10.3389/fimmu.2018.01481
Received: 29 March 2018; Accepted: 14 June 2018;
Published: 25 June 2018
Edited by:
Juarez Antonio Simões Quaresma, Instituto Evandro Chagas, BrazilReviewed by:
Dorothy Ellen Lewis, University of Texas, United StatesJohn Zaunders, University of New South Wales, Australia
Copyright: © 2018 Adekambi, Ibegbu, Cagle, Ray and Rengarajan. This is an open-access article distributed under the terms of the Creative Commons Attribution License (CC BY). The use, distribution or reproduction in other forums is permitted, provided the original author(s) and the copyright owner are credited and that the original publication in this journal is cited, in accordance with accepted academic practice. No use, distribution or reproduction is permitted which does not comply with these terms.
*Correspondence: Toidi Adekambi, tadekam@emory.edu;
Jyothi Rengarajan, jrengar@emory.edu