- 1School of Biosciences, College of Life and Environmental Sciences, University of Birmingham, Birmingham, United Kingdom
- 2Centre of Membrane Proteins and Receptors (COMPARE), University of Birmingham, Birmingham, United Kingdom
A disintegrin and metalloproteinase 10 (ADAM10) is a ubiquitous transmembrane protein that functions as a “molecular scissor” to cleave the extracellular regions from its transmembrane target proteins. ADAM10 is well characterized as the ligand-dependent activator of Notch proteins, which control cell fate decisions. Indeed, conditional knockouts of ADAM10 in mice reveal impaired B-, T-, and myeloid cell development and/or function. ADAM10 cleaves many other leukocyte-expressed substrates. On B-cells, ADAM10 cleavage of the low-affinity IgE receptor CD23 promotes allergy and asthma, cleavage of ICOS ligand impairs antibody responses, and cleavage of the BAFF–APRIL receptor transmembrane activator and CAML interactor, and BAFF receptor, reduce B-cell survival. On microglia, increased ADAM10 cleavage of a rare variant of the scavenger receptor triggering receptor expressed on myeloid cells 2 may increase susceptibility to Alzheimer’s disease. We and others recently showed that ADAM10 interacts with one of six different regulatory tetraspanin membrane proteins, which we termed the TspanC8 subgroup, comprising Tspan5, Tspan10, Tspan14, Tspan15, Tspan17, and Tspan33. The TspanC8s are required for ADAM10 exit from the endoplasmic reticulum, and emerging evidence suggests that they dictate ADAM10 subcellular localization and substrate specificity. Therefore, we propose that ADAM10 should not be regarded as a single scissor, but as six different scissors with distinct substrate specificities, depending on the associated TspanC8. In this review, we collate recent transcriptomic data to present the TspanC8 repertoires of leukocytes, and we discuss the potential role of the six TspanC8/ADAM10 scissors in leukocyte development and function.
Introduction
The proteolytic cleavage, or “shedding,” of the extracellular region (ectodomain) of transmembrane proteins is an important mechanism for the regulation of leukocyte development and function. Shedding can initiate intracellular signal transduction via the cell-associated cleavage fragment (e.g., Notch signaling to drive cell fate decisions), downregulate signaling or adhesion that requires cell surface receptor expression, or activate paracrine signaling through the release of growth factors, cytokines, and chemokines (1). The ADAMs (a disintegrin and metalloproteinases) are one of the main proteinase families that function as sheddases and can be regarded as “molecular scissors.” A total of 22 ADAM genes have been identified in humans, of which 12 (ADAM8, 9, 10, 12, 15, 17, 19, 20, 21, 28, 30, and 33) are active proteases with the consensus sequence (HExGHxxGxxHD) required for Zn2+-dependent protease activity (1).
The “Molecular Scissor” ADAM10
One of the best-characterized ADAMs is ADAM10, due to its essential role in ligand-dependent cleavage of Notch proteins to initiate Notch signaling (2). Indeed, ADAM10-knockout mice die at embryonic day 9.5, phenocopying double knockout mice for two of the four Notch proteins, Notch 1 and 4 (3, 4). ADAM10 has an N-terminal signal sequence, an inhibitory prodomain, a metalloproteinase domain, followed by disintegrin, cysteine-rich, transmembrane, and cytoplasmic domains (Figure 1). The prodomain is removed by proprotein convertases during biosynthesis to generate a mature sheddase (5). The first crystal structure of the mature ADAM10 ectodomain suggests that the metalloprotease exists in a closed conformation in which the cysteine-rich domain partially occludes the catalytic site, but with the catalytic site in position to cleave substrates close to the plasma membrane (6). ADAM10 has at least 40 substrates, including amyloid precursor protein (7), cellular prion protein (8), cadherins (9–11), and the platelet-activating collagen/fibrin receptor GPVI (12, 13). ADAM10 has a number of substrates that are expressed by leukocytes, or which impact on leukocyte function, including the low-affinity IgE receptor CD23 (14, 15), the endothelial cell–cell adhesion molecule vascular-endothelial (VE)-cadherin (11), the B-cell costimulatory molecule ICOS ligand (16), B-cell homeostasis proteins transmembrane activator and CAML interactor (TACI) (17) and BAFF receptor (BAFFR) (18), and triggering receptor expressed on myeloid cells 2 (TREM2) (19, 20). ADAM10 has been implicated in myriad immune diseases including T-cell acute lymphoblastic leukemia (T-ALL), asthma, atherosclerosis, and Alzheimer’s disease (2).
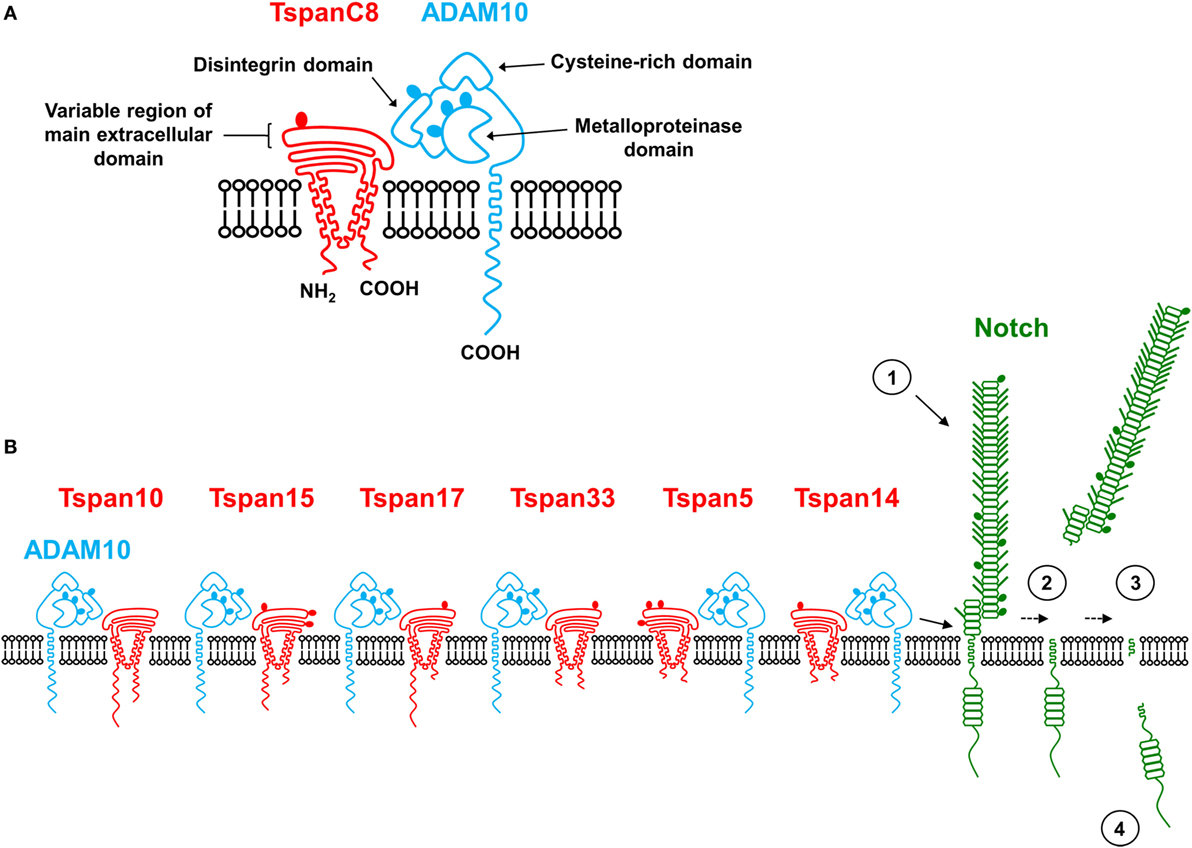
Figure 1. Six ADAM10 scissors: Tspan14 and Tspan5 may be important for Notch activation in immune cells. (A) A schematic representation of a TspanC8 and ADAM10. (B) A model figure to show the six different TspanC8/ADAM10 complexes, which have different subcellular localizations, distinct substrate specificities, and may have different ADAM10 conformations. Notch cleavage is initiated following engagement with a Notch ligand on another cell (1), which induces a conformational change that we hypothesize allows cleavage by a Tspan14/ADAM10 or Tspan5/ADAM10 scissor (2), followed by intramembrane cleavage by γ-secretase (3), to release the intracellular domain to act as a transcription factor and drive cell fate decisions (4). Tspan10 has also been implicated in Notch activation, but its relatively low expression by immune cells suggests no substantial role in these cells. N-linked and O-linked glycosylation sites are indicated by filled ovals and short lines, respectively.
Tetraspanins as “Membrane Organizers”
The tetraspanins are a superfamily of transmembrane proteins expressed in multicellular eukaryotes. They are characterized by four transmembrane domains and small and large extracellular loops (Figure 1), the latter containing structurally important cysteine residues. Mammals express 33 tetraspanins and each cell type has a distinct repertoire of tetraspanins (21); leukocytes express at least 20 tetraspanins (22). Tetraspanins are “membrane organizers” that form dynamic nanoclusters (21). Visualization of tetraspanins CD37, CD53, CD81, and CD82 on human B-cells and dendritic cells, by super-resolution microscopy, suggests that approximately 10 tetraspanins of a single type cluster together into individual nanodomains (23). Tetraspanins also associate directly with specific non-tetraspanin proteins, termed “partner proteins,” to regulate their intracellular trafficking, clustering, lateral mobility, and compartmentalization (21). Relatively well-studied tetraspanin–partner interactions are tetraspanin CD151 with the laminin-binding integrins (24), tetraspanin CD81 with the B-cell co-receptor CD19 (25), and Tspan12 with the Wnt/Norrin receptor Frizzled-4 (26). In each case, tetraspanin mutations yield phenotypes that are consistent with impaired partner protein function. For example, in CD81-deficient humans and mice, CD19 fails to traffic to the B-cell surface and antibody generation is impaired (25). The recently published crystal structure of full-length CD81, which is the first such structure for a tetraspanin, shows CD81 to be cone shaped with an intramembrane cholesterol-binding cavity within the transmembranes (27). Molecular dynamics simulations suggest that CD81 may exist in two different conformations, a closed conformation when cholesterol is present, and an open conformation when cholesterol is absent, in which the large extracellular loop swings upwards (27). Therefore, tetraspanins could function as “molecular switches” that control the activity of their partner proteins through cholesterol-regulated conformational change.
TspanC8 Tetraspanins Regulate ADAM10: The “Six Scissor” Hypothesis
In 2012, we and others showed that ADAM10 interacts with six tetraspanins that are closely related by protein sequence: Tspan5, Tspan10, Tspan14, Tspan15, Tspan17, and Tspan33 (28–30). We termed these the TspanC8 subgroup due to the eight cysteine residues in their large extracellular loops (28, 29); other tetraspanins have four, six, or seven cysteines. The TspanC8s are essential for promoting ADAM10 exit from the endoplasmic reticulum, its subsequent maturation in the Golgi through removal of the prodomain, and trafficking to the cell surface or other membrane compartments (28–30). The functional association between TspanC8s and ADAM10 has been demonstrated in TspanC8-knockout mice (29, 31) and the fruit fly Drosophila (28), and is reinforced by recent data demonstrating reciprocal regulation of Tspan5 exit from the endoplasmic reticulum by ADAM10 (32). Moreover, emerging evidence indicates that each TspanC8 can target ADAM10 to different subcellular localizations and to different substrates, and ADAM10 may adopt distinct conformations dictated by the associated TspanC8 (28, 32–35). For example, we and two other groups reported Tspan15 as the only TspanC8 to promote ADAM10 cleavage of neuronal (N)-cadherin (30, 33, 34). These in vitro data are supported by data from the recently characterized Tspan15-deficient mouse, which has strikingly reduced N-cadherin cleavage in the brain, despite only a subtle decrease in mature ADAM10 expression (31). ADAM10 shedding of Notch is promoted by Tspan5, Tspan10, and Tspan14 (Figure 1), but not by Tspan15 and 33 (28, 32, 33, 36). In addition, we have shown Tspan5 and Tspan17 to regulate VE-cadherin expression on endothelial cells (35). Taking these data together, we now propose that ADAM10 should be regarded as six different TspanC8/ADAM10 scissor complexes, rather than a single scissor (37, 38). This idea has implications for therapeutic targeting of ADAM10, which may be impractical due to toxic side effects. However, targeting one of the TspanC8/ADAM10 complexes, via the tetraspanin, may minimize toxicity while enabling substrate- and disease-specific targeting.
We and others have recently reviewed our current understanding of how TspanC8s regulate ADAM10 (37–39). In this review, we will analyze and present published RNA-Seq transcriptomic data for TspanC8 expression in different leukocyte subsets. We will discuss these expression profiles in the context of our current knowledge of ADAM10’s role in the development and function of T-cells, B-cells, and myeloid cells.
Regulation of T-Cell Development and Transmigration by ADAM10 and TspanC8s
Two publications have shown that ADAM10 is important for normal T-cell development, most likely through regulation of Notch signaling (40, 41). In the first, the embryonic lethality of ADAM10-knockout mice was circumvented by the generation of transgenic mice that express dominant negative ADAM10 under the control of the T-cell-specific Lck promoter (40). The dominant negative ADAM10 construct yields a similar phenotype to T-cell-specific deletion of Notch1, the Notch family member with the major role in thymocyte development. Thymocyte numbers are reduced by 60–90% due to a partial block in the CD4/CD8 double negative to double positive transition, with accompanying reduction in expression of T-cell receptor (TCR) β (40). Defective Notch signaling is the probable mechanism, since expression of Notch-responsive genes is partially reduced, and partial rescue is achieved by transgenic overexpression of the Notch ligand Delta-1, or a dominant active form of Notch1, in thymocytes. The dominant negative construct lacks the metalloproteinase domain and is expressed at several-fold higher levels than endogenous ADAM10 (40); one mechanism of action may be the sequestration of endogenous TspanC8s, since we have shown such a construct to interact with TspanC8s (34). In the second publication, conditional T-cell-specific ADAM10-knockout mice were generated by crossing ADAM10 floxed mice with mice expressing Cre recombinase driven by the Lck promoter (41). This model phenocopies T-cell-specific Notch1 deletion in showing a twofold to threefold reduction in thymocyte numbers, due to a partial block in development from the double negative to double positive stage. There is reduced expression of Notch target genes but, unlike the dominant negative ADAM10 model, no defect in TCRβ expression is observed (41).
The most highly expressed TspanC8 in human and mouse T-cells is Tspan14, followed by Tspan5 and Tspan17; Tspan15 is also expressed by human T-cells but not mouse (Figures 2A,B). However, it is important to note that such publically available transcriptomic data have not been independently validated, nor have the expression profiles been confirmed using validated antibodies. Nevertheless, since both Tspan14 and Tspan5 promote Notch signaling (28, 32, 33), we hypothesize that Tspan14/ADAM10 will have a major role in thymocyte development via activation of Notch1, while Tspan5/ADAM10 may have a minor role. The future analyses of the respective knockout mice will help to test this hypothesis; the Tspan14-knockout mouse has yet to be made, while the Tspan5-knockout mouse is viable but functionally uncharacterized (32). It is possible that Tspan14 and/or Tspan5 play a role in the aggressive blood cancer T-ALL. Approximately 50% of T-ALL is driven by activating mutations in Notch1, some of which require ADAM10 for full activation in a ligand-independent manner. Knockdown of ADAM10 reduces Notch signaling and T-ALL proliferation (42). Targeting Tspan14 or Tspan5 may achieve a similar result, yet without the toxicity of global ADAM10 inhibition. Interestingly, antibody targeting of Tspan5 can impair Notch signaling (32).
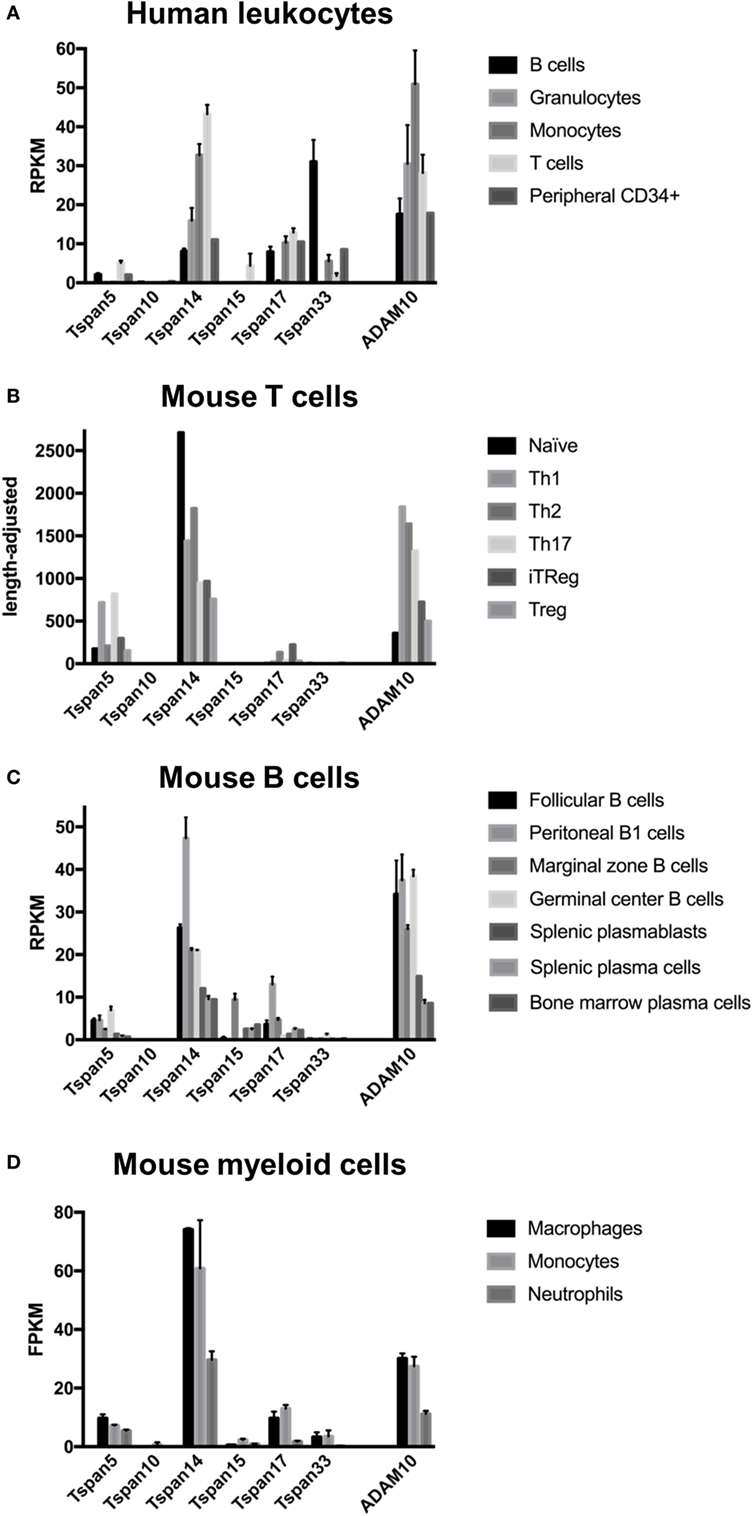
Figure 2. Leukocytes express ADAM10, but different cell subsets have distinct TspanC8 repertoires. Publically available RNA-Seq data for (A) human leukocytes [Gene Expression Omnibus (GEO) accession GSE51984], (B) mouse T-cell subsets (43), (C) mouse B-cells (GEO accession GSE60927), and (D) mouse macrophages, monocytes, and neutrophils (GEO accession GSE59831). Data are presented as reads per kilobase of transcript per million mapped reads (RPKM) (A,C), as length-adjusted values that provide a measure equivalent to RPKM (43) (B), or as fragments per kilobase of transcript per million mapped reads (FPKM) (D). Error bars represent the SD. Number of samples are as follows: five for panel (A), except for CD34+ peripheral cells (hematopoietic stem cells from the blood) which has one; two for panel (C), except for splenic plasmablasts and bone marrow plasma cells which have one, and splenic plasma cells which have three; and two for panel (D), with the exception of neutrophils which have two.
In addition to Notch proteins, a number of other proteins have been reported to be cleaved by ADAM10 on T-cells: CD40 ligand (44), Fas ligand (45, 46), LAG-3 (47), CD44 (48), and T-cell immunoglobulin and mucin domain 3 (Tim-3) (49). How important these cleavage events are to T-cell function has yet to be determined, and for CD40 ligand, LAG-3 and Tim-3 is complicated by their additional cleavage by ADAM17 (44, 47, 49), an ADAM10-related metalloproteinase.
We have recently reported that endothelial cell-expressed ADAM10 promotes the transmigration (also known as extravasation or diapedesis) of T-cells in an in vitro model of inflammation (35). The mechanism involves ADAM10 regulation of VE-cadherin expression levels, since ADAM10 knockdown results in 50% elevated VE-cadherin expression and delayed transmigration, which is rescued by partial VE-cadherin knockdown to wild-type levels. This promotion of transmigration by endothelial ADAM10 appears restricted to T-cells, since no effects on neutrophils or B-cell transmigration are observed (35), nor on monocytes in a separate study (50). Among the TspanC8s, we have previously reported that Tspan14 is the most highly expressed on endothelial cells (29, 51). However, knockdown experiments show that Tspan5 and Tspan17 are the tetraspanins that promote ADAM10 regulation of VE-cadherin (35). Consistent with this common function, Tspan5 and Tspan17 are the most highly conserved pair of tetraspanins, with 72% protein sequence identity in human (35). However, it remains to be determined whether this role for Tspan5/17 holds true in vivo. Tspan17-knockout mice have yet to be made; ultimately a Tspan5/17 double knockout may be required to overcome functional redundancy.
Regulation of B-Cell Development and Function by ADAM10 and Potential Role for TspanC8s
An important role for ADAM10 in B-cell development and function has been demonstrated using B-cell-specific ADAM10-knockout mice, made by crossing ADAM10 floxed mice with CD19-Cre mice (52). Early development of B-cells in the bone marrow of these mice is unaltered, with normal numbers of pro-, pre-, and immature B-cell populations. B1 cell numbers in the peritoneal cavity are also normal. However, ADAM10-knockout immature B-cells entering the spleen fail to develop into marginal zone B-cells; these cells act as a first line of defense by rapid antibody generation against blood-borne pathogens that become trapped in the spleen. By contrast, follicular zone B-cell numbers in the spleen are slightly elevated. The mechanism underlying defective marginal zone B-cell development in the absence of ADAM10 appears to be defective Notch2 activation (52), and consistent with this, B-cells express relatively high levels of Notch2 and low levels of Notch 1, 3, and 4, and Notch2 is critical for development of marginal zone but not follicular B-cells (53–55).
B-cell-specific ADAM10-knockout mice have a striking reduction in antibody responses following immunization, associated with impaired germinal center formation in secondary lymphoid tissues (56). The mechanism responsible appears to be the upregulated expression of TNFα, a pro-inflammatory cytokine which is important for the maintenance of lymphoid tissue architecture, and ADAM17, which sheds TNFα (57). How ADAM10-deficiency leads to ADAM17 and TNFα upregulation in B-cells remains unknown. To investigate the function of ADAM10-knockout plasma cells in the context of normal germinal centers, ADAM10 floxed mice were crossed with IgG1-Cre mice to delete ADAM10 post-isotype switching. Antibody responses are strikingly reduced, despite normal plasma cell numbers (58). This may be due to elevated expression of ICOS ligand, a recently identified ADAM10 substrate, on ADAM10-knockout B-cells (16). The engagement of ICOS ligand with its receptor ICOS on T-cells is required for T-cell-dependent antibody responses. In ADAM10-knockout B-cells, elevated ICOSL causes a substantial reduction in surface ICOS by promoting its internalization (16), thus providing a mechanism for impaired antibody responses.
With the possible exception of Notch2, the best-studied ADAM10 substrate on B-cells is CD23, the low-affinity IgE receptor, which regulates allergic and inflammatory responses (14, 15). Indeed, on ADAM10-knockout B-cells, CD23 expression is increased approximately threefold, while soluble CD23 levels in plasma are substantially reduced (52). In an IgE-dependent asthma model, B-cell-specific ADAM10-knockout mice have strikingly reduced signs of allergic inflammation in the lung (59). Allergic patients and allergy-prone Th2 mice have increased expression of ADAM10 on B-cells and increased soluble CD23 and IgE levels in plasma (60). Although the regulation of IgE expression by CD23 is complex and not fully understood, these data have lead the authors to propose ADAM10 as a therapeutic target for asthma (59).
ADAM10 is emerging as a regulator of the BAFF–APRIL system which controls B-cell homeostasis. The two ligands are BAFF and APRIL, while the three receptors are B-cell maturation antigen, TACI, and BAFFR, the latter of which binds BAFF but not APRIL. ADAM10 can shed TACI to release a soluble ectodomain that acts as a decoy receptor, binding to BAFF and APRIL and so inhibiting survival of B-cells (17). In addition, cell survival can be reduced by ADAM10 or ADAM17 shedding of BAFFR (18).
In human B-cells, Tspan33 expression is highest, followed by Tspan14, Tspan17, and then Tspan5 (Figure 2A). In mouse, Tspan14 expression is highest, followed by Tspan5, Tspan15, and Tspan17, while Tspan33 expression is minimal (Figure 2C). Therefore, Tspan14 is likely to be the main regulator of Notch2 in B-cells, with a less important role for Tspan5. A role for TspanC8s in regulating CD23 shedding has not been reported, but Tspan5, Tspan14, Tspan17, and/or Tspan33 are candidates; this is important work for the future, because such a tetraspanin is a potential therapeutic target for asthma. Tspan33 expression in human B-cells has been confirmed at the protein level (61), but the ADAM10 substrates that it regulates have not been investigated.
Regulation of Myeloid Cell Function by ADAM10 and Potential Role for TspanC8s
Dendritic cell-specific ADAM10-knockout mice have been generated by crossing ADAM10 floxed mice with CD11c-Cre mice (62). These mice have strikingly impaired Th2 responses, but Th1 and Th17 responses are unaffected. As a consequence, the mice are protected from IgE-mediated anaphylaxis and allergic lung inflammation. This appears to be due to defective Notch signaling, since rescue is observed by transgenic expression of the Notch1 intracellular domain, and dendritic cell-specific Notch1-knockout mice have a similar phenotype (62). Our RNA-Seq analyses have found Tspan14 to be most highly expressed in human dendritic cells, with lower expression of Tspan17 and Tspan33 (data not shown). Therefore, Tspan14 is likely to be important in dendritic cells for Notch signaling.
Specific knockout of ADAM10 in myeloid cells has been investigated by crossing ADAM10 floxed mice with LysM-cre mice (63). This does not achieve complete knockout in myeloid cells, but surface levels of ADAM10 on bone marrow-derived macrophages are reduced by 85%. No major abnormalities in the mice are observed, and leukocyte populations are present in normal numbers. However, ADAM10-knockout results in a reduced inflammatory phenotype in macrophages and a reduced capacity to migrate and to degrade extracellular matrix (63). Macrophages play a central role in the initiation and progression of the inflammatory disease atherosclerosis, and can take up lipids to become pro-inflammatory foam cells within atherosclerotic plaques. To investigate the role of macrophage ADAM10 in this disease, bone marrow from myeloid-specific ADAM10-knockout mice was transplanted into atherosclerosis-prone low-density lipoprotein receptor knockout mice. Consistent with a less inflammatory macrophage phenotype, atherosclerotic plaques appear more stable, with higher collagen content, although plaque size is similar to wild type (63). Nevertheless, this has important implications for human disease; stable plaques are less susceptible to the rupture that causes thrombosis, vessel occlusion, and heart attack or stroke. Interestingly, Notch signaling promotes an inflammatory macrophage phenotype, and blockade of Notch signaling in an atherosclerosis model reduces atherosclerosis development while increasing plaque stability (64). Macrophages express relatively high levels of the Notch-promoting Tspan14 (Figures 2A,D), highlighting the macrophage Tspan14/ADAM10 complex as a potential therapeutic target for maintaining plaque stability in atherosclerosis.
An additional study generated mice with leukocyte- and myeloid-specific ADAM10 deficiency by crossing floxed ADAM10 mice with Vav-Cre and LysM-Cre mice, respectively. In an inflammatory lung model, neutrophil and monocyte recruitment are reduced by approximately 50% in the absence of ADAM10 (65). The underlying mechanism is not clear. Tspan14 appears to be the only TspanC8 expressed by human granulocytes and is also highly expressed by mouse neutrophils, which have relatively weak Tspan5 expression (Figures 2A,D). Therefore, Tspan14/ADAM10-induced Notch activation may potentially promote neutrophil inflammatory responses.
Finally, the scavenger receptor TREM2 has been recently identified as an ADAM10 substrate with a potential role in Alzheimer’s disease (19, 20). TREM2 is expressed on macrophages, microglia, osteoclasts, and dendritic cells. A rare H157Y variant of TREM2 is associated with increased risk of Alzheimer’s disease; amino acid 157 is at the cleavage site for ADAM10, and H157Y is shed more readily. The loss of TREM2 renders macrophages less phagocytic, and the authors propose that this renders the individual more prone to Alzheimer’s disease (19, 20). It will be interesting to determine if any macrophage-expressed TspanC8, namely Tspan5, Tspan14, Tspan17, or Tspan33, can promote TREM2 cleavage, and could thus be considered a therapeutic target for TREM2-associated Alzheimer’s disease.
Concluding Remarks
The six scissor hypothesis suggests that ADAM10 should be studied in the context of its regulatory tetraspanins. In leukocytes, the relatively high expression of Tspan14, together with its capacity to promote Notch activation, suggest that the Tspan14/ADAM10 complex may be critical for leukocyte development and function. The future analyses of cells and mice deficient in Tspan14, and other TspanC8s, will determine which scissor cleaves which substrates. This may direct therapeutic targeting of individual TspanC8/ADAM10 complexes, using antibodies or small molecules, to modulate a specific substrate while avoiding the toxicity of global ADAM10 targeting. Such an approach could provide new treatments for ADAM10-associated diseases, including T-ALL, asthma, atherosclerosis, and Alzheimer’s disease.
Author Contributions
AM, CK, JS, NH, and MT: conception, design, and writing of the manuscript. AK: data analysis and editing of the manuscript.
Conflict of Interest Statement
The authors declare that the research was conducted in the absence of any commercial or financial relationships that could be construed as a potential conflict of interest.
Acknowledgments
We are grateful to Steve Blacklow and Andy Lovering for discussions on tetraspanin and ADAM10 structure.
Funding
AM and JS were funded by Midlands Integrative Biosciences Training Partnership (MIBTP) Ph.D. Studentships from the Biotechnology and Biological Sciences Research Council. CK was funded by the Birmingham-Nottingham Centre of Membrane Proteins and Receptors (COMPARE) and by a Ph.D. Studentship from the British Heart Foundation (FS/18/9/33388). NH was funded by a Project Grant from the Biotechnology and Biological Sciences Research Council (BB/P00783X/1).
References
1. Giebeler N, Zigrino P. A disintegrin and metalloprotease (ADAM): historical overview of their functions. Toxins (Basel) (2016) 8:122. doi:10.3390/toxins8040122
2. Wetzel S, Seipold L, Saftig P. The metalloproteinase ADAM10: a useful therapeutic target? Biochim Biophys Acta (2017) 1864(11 Pt B):2071–81. doi:10.1016/j.bbamcr.2017.06.005
3. Hartmann D, de Strooper B, Serneels L, Craessaerts K, Herreman A, Annaert W, et al. The disintegrin/metalloprotease ADAM 10 is essential for Notch signalling but not for alpha-secretase activity in fibroblasts. Hum Mol Genet (2002) 11:2615–24. doi:10.1093/hmg/11.21.2615
4. Krebs LT, Xue Y, Norton CR, Shutter JR, Maguire M, Sundberg JP, et al. Notch signaling is essential for vascular morphogenesis in mice. Genes Dev (2000) 14:1343–52. doi:10.1101/gad.14.11.1343
5. Wong E, Maretzky T, Peleg Y, Blobel CP, Sagi I. The functional maturation of a disintegrin and metalloproteinase (ADAM) 9, 10, and 17 requires processing at a newly identified proprotein convertase (PC) cleavage site. J Biol Chem (2015) 290:12135–46. doi:10.1074/jbc.M114.624072
6. Seegar TCM, Killingsworth LB, Saha N, Meyer PA, Patra D, Zimmerman B, et al. Structural basis for regulated proteolysis by the alpha-secretase ADAM10. Cell (2017) 171:1638–48.e7. doi:10.1016/j.cell.2017.11.014
7. Postina R, Schroeder A, Dewachter I, Bohl J, Schmitt U, Kojro E, et al. A disintegrin-metalloproteinase prevents amyloid plaque formation and hippocampal defects in an Alzheimer disease mouse model. J Clin Invest (2004) 113:1456–64. doi:10.1172/JCI20864
8. Altmeppen HC, Prox J, Krasemann S, Puig B, Kruszewski K, Dohler F, et al. The sheddase ADAM10 is a potent modulator of prion disease. Elife (2015) 4. doi:10.7554/eLife.04260
9. Maretzky T, Reiss K, Ludwig A, Buchholz J, Scholz F, Proksch E, et al. ADAM10 mediates E-cadherin shedding and regulates epithelial cell-cell adhesion, migration, and beta-catenin translocation. Proc Natl Acad Sci U S A (2005) 102:9182–7. doi:10.1073/pnas.0500918102
10. Reiss K, Maretzky T, Ludwig A, Tousseyn T, de Strooper B, Hartmann D, et al. ADAM10 cleavage of N-cadherin and regulation of cell-cell adhesion and beta-catenin nuclear signalling. EMBO J (2005) 24:742–52. doi:10.1038/sj.emboj.7600548
11. Schulz B, Pruessmeyer J, Maretzky T, Ludwig A, Blobel CP, Saftig P, et al. ADAM10 regulates endothelial permeability and T-cell transmigration by proteolysis of vascular endothelial cadherin. Circ Res (2008) 102:1192–201. doi:10.1161/CIRCRESAHA.107.169805
12. Bender M, Hofmann S, Stegner D, Chalaris A, Bosl M, Braun A, et al. Differentially regulated GPVI ectodomain shedding by multiple platelet-expressed proteinases. Blood (2010) 116:3347–55. doi:10.1182/blood-2010-06-289108
13. Gardiner EE, Karunakaran D, Shen Y, Arthur JF, Andrews RK, Berndt MC. Controlled shedding of platelet glycoprotein (GP)VI and GPIb-IX-V by ADAM family metalloproteinases. J Thromb Haemost (2007) 5:1530–7. doi:10.1111/j.1538-7836.2007.02590.x
14. Lemieux GA, Blumenkron F, Yeung N, Zhou P, Williams J, Grammer AC, et al. The low affinity IgE receptor (CD23) is cleaved by the metalloproteinase ADAM10. J Biol Chem (2007) 282:14836–44. doi:10.1074/jbc.M608414200
15. Weskamp G, Ford JW, Sturgill J, Martin S, Docherty AJ, Swendeman S, et al. ADAM10 is a principal ‘sheddase’ of the low-affinity immunoglobulin E receptor CD23. Nat Immunol (2006) 7:1293–8. doi:10.1038/ni1399
16. Lownik JC, Luker AJ, Damle SR, Cooley LF, El Sayed R, Hutloff A, et al. ADAM10-mediated ICOS ligand shedding on B cells is necessary for proper T cell ICOS regulation and T follicular helper responses. J Immunol (2017) 199:2305–15. doi:10.4049/jimmunol.1700833
17. Hoffmann FS, Kuhn PH, Laurent SA, Hauck SM, Berer K, Wendlinger SA, et al. The immunoregulator soluble TACI is released by ADAM10 and reflects B cell activation in autoimmunity. J Immunol (2015) 194:542–52. doi:10.4049/jimmunol.1402070
18. Smulski CR, Kury P, Seidel LM, Staiger HS, Edinger AK, Willen L, et al. BAFF- and TACI-dependent processing of BAFFR by ADAM proteases regulates the survival of B cells. Cell Rep (2017) 18:2189–202. doi:10.1016/j.celrep.2017.02.005
19. Schlepckow K, Kleinberger G, Fukumori A, Feederle R, Lichtenthaler SF, Steiner H, et al. An Alzheimer-associated TREM2 variant occurs at the ADAM cleavage site and affects shedding and phagocytic function. EMBO Mol Med (2017) 9:1356–65. doi:10.15252/emmm.201707672
20. Thornton P, Sevalle J, Deery MJ, Fraser G, Zhou Y, Stahl S, et al. TREM2 shedding by cleavage at the H157-S158 bond is accelerated for the Alzheimer’s disease-associated H157Y variant. EMBO Mol Med (2017) 9:1366–78. doi:10.15252/emmm.201707673
21. Charrin S, Jouannet S, Boucheix C, Rubinstein E. Tetraspanins at a glance. J Cell Sci (2014) 127:3641–8. doi:10.1242/jcs.154906
22. Tarrant JM, Robb L, van Spriel AB, Wright MD. Tetraspanins: molecular organisers of the leukocyte surface. Trends Immunol (2003) 24:610–7. doi:10.1016/j.it.2003.09.011
23. Zuidscherwoude M, Gottfert F, Dunlock VM, Figdor CG, van den Bogaart G, van Spriel AB. The tetraspanin web revisited by super-resolution microscopy. Sci Rep (2015) 5:12201. doi:10.1038/srep12201
24. Hemler ME. Tetraspanin proteins promote multiple cancer stages. Nat Rev Cancer (2014) 14:49–60. doi:10.1038/nrc3640
25. Levy S. Function of the tetraspanin molecule CD81 in B and T cells. Immunol Res (2014) 58:179–85. doi:10.1007/s12026-014-8490-7
26. Tomlinson MG. Eye-opening potential for tetraspanin Tspan12 as a therapeutic target for diseases of the retinal vasculature. Circulation (2017) 136:196–9. doi:10.1161/CIRCULATIONAHA.117.028521
27. Zimmerman B, Kelly B, McMillan BJ, Seegar TC, Dror RO, Kruse AC, et al. Crystal structure of a full-length human tetraspanin reveals a cholesterol-binding pocket. Cell (2016) 167:1041–51. doi:10.1016/j.cell.2016.09.056
28. Dornier E, Coumailleau F, Ottavi JF, Moretti J, Boucheix C, Mauduit P, et al. TspanC8 tetraspanins regulate ADAM10/Kuzbanian trafficking and promote Notch activation in flies and mammals. J Cell Biol (2012) 199:481–96. doi:10.1083/jcb.201201133
29. Haining EJ, Yang J, Bailey RL, Khan K, Collier R, Tsai S, et al. The TspanC8 subgroup of tetraspanins interacts with A disintegrin and metalloprotease 10 (ADAM10) and regulates its maturation and cell surface expression. J Biol Chem (2012) 287:39753–65. doi:10.1074/jbc.M112.416503
30. Prox J, Willenbrock M, Weber S, Lehmann T, Schmidt-Arras D, Schwanbeck R, et al. Tetraspanin15 regulates cellular trafficking and activity of the ectodomain sheddase ADAM10. Cell Mol Life Sci (2012) 69:2919–32. doi:10.1007/s00018-012-0960-2
31. Seipold L, Altmeppen H, Koudelka T, Tholey A, Kasparek P, Sedlacek R, et al. In vivo regulation of the A disintegrin and metalloproteinase 10 (ADAM10) by the tetraspanin 15. Cell Mol Life Sci (2018) 1–17. doi:10.1007/s00018-018-2791-2
32. Saint-Pol J, Billard M, Dornier E, Eschenbrenner E, Danglot L, Boucheix C, et al. New insights into the tetraspanin Tspan5 using novel monoclonal antibodies. J Biol Chem (2017) 292:9551–66. doi:10.1074/jbc.M116.765669
33. Jouannet S, Saint-Pol J, Fernandez L, Nguyen V, Charrin S, Boucheix C, et al. TspanC8 tetraspanins differentially regulate the cleavage of ADAM10 substrates, Notch activation and ADAM10 membrane compartmentalization. Cell Mol Life Sci (2016) 73:1895–915. doi:10.1007/s00018-015-2111-z
34. Noy PJ, Yang J, Reyat JS, Matthews AL, Charlton AE, Furmston J, et al. TspanC8 tetraspanins and A disintegrin and metalloprotease 10 (ADAM10) interact via their extracellular regions: evidence for distinct binding mechanisms for different TspanC8 proteins. J Biol Chem (2016) 291:3145–57. doi:10.1074/jbc.M115.703058
35. Reyat JS, Chimen M, Noy PJ, Szyroka J, Rainger GE, Tomlinson MG. ADAM10-Interacting tetraspanins Tspan5 and Tspan17 regulate VE-cadherin expression and promote T lymphocyte transmigration. J Immunol (2017) 199:666–76. doi:10.4049/jimmunol.1600713
36. Zhou J, Fujiwara T, Ye S, Li X, Zhao H. Downregulation of Notch modulators, tetraspanin 5 and 10, inhibits osteoclastogenesis in vitro. Calcif Tissue Int (2014) 95:209–17. doi:10.1007/s00223-014-9883-2
37. Matthews AL, Noy PJ, Reyat JS, Tomlinson MG. Regulation of A disintegrin and metalloproteinase (ADAM) family sheddases ADAM10 and ADAM17: the emerging role of tetraspanins and rhomboids. Platelets (2017) 28:333–41. doi:10.1080/09537104.2016.1184751
38. Matthews AL, Szyroka J, Collier R, Noy PJ, Tomlinson MG. Scissor sisters: regulation of ADAM10 by the TspanC8 tetraspanins. Biochem Soc Trans (2017) 45:719–30. doi:10.1042/BST20160290
39. Saint-Pol J, Eschenbrenner E, Dornier E, Boucheix C, Charrin S, Rubinstein E. Regulation of the trafficking and the function of the metalloprotease ADAM10 by tetraspanins. Biochem Soc Trans (2017) 45:937–44. doi:10.1042/BST20160296
40. Manilay JO, Anderson AC, Kang C, Robey EA. Impairment of thymocyte development by dominant-negative Kuzbanian (ADAM-10) is rescued by the Notch ligand, delta-1. J Immunol (2005) 174:6732–41. doi:10.4049/jimmunol.174.11.6732
41. Tian L, Wu X, Chi C, Han M, Xu T, Zhuang Y. ADAM10 is essential for proteolytic activation of Notch during thymocyte development. Int Immunol (2008) 20:1181–7. doi:10.1093/intimm/dxn076
42. Sulis ML, Saftig P, Ferrando AA. Redundancy and specificity of the metalloprotease system mediating oncogenic NOTCH1 activation in T-ALL. Leukemia (2011) 25:1564–9. doi:10.1038/leu.2011.130
43. Stubbington MJ, Mahata B, Svensson V, Deonarine A, Nissen JK, Betz AG, et al. An atlas of mouse CD4(+) T cell transcriptomes. Biol Direct (2015) 10:14. doi:10.1186/s13062-015-0045-x
44. Yacoub D, Benslimane N, Al-Zoobi L, Hassan G, Nadiri A, Mourad W. CD154 is released from T-cells by A disintegrin and metalloproteinase domain-containing protein 10 (ADAM10) and ADAM17 in a CD40 protein-dependent manner. J Biol Chem (2013) 288:36083–93. doi:10.1074/jbc.M113.506220
45. Kirkin V, Cahuzac N, Guardiola-Serrano F, Huault S, Luckerath K, Friedmann E, et al. The Fas ligand intracellular domain is released by ADAM10 and SPPL2a cleavage in T-cells. Cell Death Differ (2007) 14:1678–87. doi:10.1038/sj.cdd.4402175
46. Schulte M, Reiss K, Lettau M, Maretzky T, Ludwig A, Hartmann D, et al. ADAM10 regulates FasL cell surface expression and modulates FasL-induced cytotoxicity and activation-induced cell death. Cell Death Differ (2007) 14:1040–9. doi:10.1038/sj.cdd.4402101
47. Li N, Wang Y, Forbes K, Vignali KM, Heale BS, Saftig P, et al. Metalloproteases regulate T-cell proliferation and effector function via LAG-3. EMBO J (2007) 26:494–504. doi:10.1038/sj.emboj.7601520
48. Anderegg U, Eichenberg T, Parthaune T, Haiduk C, Saalbach A, Milkova L, et al. ADAM10 is the constitutive functional sheddase of CD44 in human melanoma cells. J Invest Dermatol (2009) 129:1471–82. doi:10.1038/jid.2008.323
49. Moller-Hackbarth K, Dewitz C, Schweigert O, Trad A, Garbers C, Rose-John S, et al. and metalloprotease (ADAM) 10 and ADAM17 are major sheddases of T cell immunoglobulin and mucin domain 3 (Tim-3). J Biol Chem (2013) 288:34529–44. doi:10.1074/jbc.M113.488478
50. Tsubota Y, Frey JM, Tai PW, Welikson RE, Raines EW. Monocyte ADAM17 promotes diapedesis during transendothelial migration: identification of steps and substrates targeted by metalloproteinases. J Immunol (2013) 190:4236–44. doi:10.4049/jimmunol.1300046
51. Bailey RL, Herbert JM, Khan K, Heath VL, Bicknell R, Tomlinson MG. The emerging role of tetraspanin microdomains on endothelial cells. Biochem Soc Trans (2011) 39:1667–73. doi:10.1042/BST20110745
52. Gibb DR, El Shikh M, Kang DJ, Rowe WJ, El Sayed R, Cichy J, et al. ADAM10 is essential for Notch2-dependent marginal zone B cell development and CD23 cleavage in vivo. J Exp Med (2010) 207:623–35. doi:10.1084/jem.20091990
53. Moriyama Y, Sekine C, Koyanagi A, Koyama N, Ogata H, Chiba S, et al. Delta-like 1 is essential for the maintenance of marginal zone B cells in normal mice but not in autoimmune mice. Int Immunol (2008) 20:763–73. doi:10.1093/intimm/dxn034
54. Saito T, Chiba S, Ichikawa M, Kunisato A, Asai T, Shimizu K, et al. Notch2 is preferentially expressed in mature B cells and indispensable for marginal zone B lineage development. Immunity (2003) 18:675–85. doi:10.1016/S1074-7613(03)00111-0
55. Santos MA, Sarmento LM, Rebelo M, Doce AA, Maillard I, Dumortier A, et al. Notch1 engagement by delta-like-1 promotes differentiation of B lymphocytes to antibody-secreting cells. Proc Natl Acad Sci U S A (2007) 104:15454–9. doi:10.1073/pnas.0702891104
56. Chaimowitz NS, Martin RK, Cichy J, Gibb DR, Patil P, Kang DJ, et al. A disintegrin and metalloproteinase 10 regulates antibody production and maintenance of lymphoid architecture. J Immunol (2011) 187:5114–22. doi:10.4049/jimmunol.1102172
57. Folgosa L, Zellner HB, El Shikh ME, Conrad DH. Disturbed follicular architecture in B cell A disintegrin and metalloproteinase (ADAM)10 knockouts is mediated by compensatory increases in ADAM17 and TNF-alpha shedding. J Immunol (2013) 191:5951–8. doi:10.4049/jimmunol.1302042
58. Chaimowitz NS, Kang DJ, Dean LM, Conrad DH. ADAM10 regulates transcription factor expression required for plasma cell function. PLoS One (2012) 7:e42694. doi:10.1371/journal.pone.0042694
59. Mathews JA, Ford J, Norton S, Kang D, Dellinger A, Gibb DR, et al. A potential new target for asthma therapy: a disintegrin and metalloprotease 10 (ADAM10) involvement in murine experimental asthma. Allergy (2011) 66:1193–200. doi:10.1111/j.1398-9995.2011.02614.x
60. Cooley LF, Martin RK, Zellner HB, Irani AM, Uram-Tuculescu C, El Shikh ME, et al. Increased B Cell ADAM10 in allergic patients and Th2 prone mice. PLoS One (2015) 10:e0124331. doi:10.1371/journal.pone.0124331
61. Perez-Martinez CA, Maravillas-Montero JL, Meza-Herrera I, Vences-Catalan F, Zlotnik A, Santos-Argumedo L. Tspan33 is expressed in transitional and memory B cells, but is not responsible for high ADAM10 expression. Scand J Immunol (2017) 86:23–30. doi:10.1111/sji.12559
62. Damle SR, Martin RK, Cockburn CL, Lownik JC, Carlyon JA, Smith AD, et al. ADAM10 and Notch1 on murine dendritic cells control the development of type 2 immunity and IgE production. Allergy (2018) 73:125–36. doi:10.1111/all.13261
63. van der Vorst EP, Jeurissen M, Wolfs IM, Keijbeck A, Theodorou K, Wijnands E, et al. Myeloid A disintegrin and metalloproteinase domain 10 deficiency modulates atherosclerotic plaque composition by shifting the balance from inflammation toward fibrosis. Am J Pathol (2015) 185:1145–55. doi:10.1016/j.ajpath.2014.11.028
64. Fukuda D, Aikawa E, Swirski FK, Novobrantseva TI, Kotelianski V, Gorgun CZ, et al. Notch ligand delta-like 4 blockade attenuates atherosclerosis and metabolic disorders. Proc Natl Acad Sci U S A (2012) 109:E1868–77. doi:10.1073/pnas.1116889109
Keywords: a disintegrin and metalloproteinase 10, tetraspanins, TspanC8s, leukocytes
Citation: Matthews AL, Koo CZ, Szyroka J, Harrison N, Kanhere A and Tomlinson MG (2018) Regulation of Leukocytes by TspanC8 Tetraspanins and the “Molecular Scissor” ADAM10. Front. Immunol. 9:1451. doi: 10.3389/fimmu.2018.01451
Received: 20 April 2018; Accepted: 12 June 2018;
Published: 02 July 2018
Edited by:
Annemiek van Spriel, Radboud University Nijmegen Medical Centre, NetherlandsReviewed by:
Beatrice Charreau, University of Nantes, FrancePeter Daniel Burrows, University of Alabama at Birmingham, United States
Copyright: © 2018 Matthews, Koo, Szyroka, Harrison, Kanhere and Tomlinson. This is an open-access article distributed under the terms of the Creative Commons Attribution License (CC BY). The use, distribution or reproduction in other forums is permitted, provided the original author(s) and the copyright owner are credited and that the original publication in this journal is cited, in accordance with accepted academic practice. No use, distribution or reproduction is permitted which does not comply with these terms.
*Correspondence: Michael G. Tomlinson, bS5nLnRvbWxpbnNvbiYjeDAwMDQwO2JoYW0uYWMudWs=