- Department of Immunology, Juntendo University School of Medicine, Tokyo, Japan
Mucosal-associated invariant T (MAIT) cells are innate T cells restricted by MHC-related molecule 1 (MR1). MAIT cells express semi-invariant T-cell receptors TRAV1-2-TRAJ33/12/20 in humans and TRAV1-TRAJ33 in mice. MAIT cells recognize vitamin B2 biosynthesis derivatives presented by MR1. Similar to other innate lymphocytes, MAIT cells are also activated by cytokines in the absence of exogenous antigens. MAIT cells have the capacity to produce cytokines, such as IFNγ, TNFα, and IL-17, and cytotoxic proteins, including perforin and granzyme B. MAIT cells were originally named after their preferential location in the mucosal tissue of the gut, but they are also abundant in other peripheral organs, including the liver and lungs. In humans, the frequency of MAIT cells is high in peripheral blood, and these cells constitute approximately 5% of circulating CD3+ cells. Their abundance in tissues and rapid activation following stimulation have led to great interest in their function in various types of immune diseases. In this review, first, we will briefly introduce key information of MAIT cell biology required for better understating their roles in immune responses, and then describe how MAIT cells are associated with autoimmune and other immune diseases in humans. Moreover, we will discuss their functions based on information from animal models of autoimmune and immunological diseases.
Introduction
Two subsets of T cells express semi-invariant T-cell receptors (TCRs). The first subset includes the thoroughly studied invariant natural killer T (iNKT) cells that uniquely recognize lipid antigens presented by CD1d, a homolog of the MHC molecule. TCRα rearrangement in iNKT cells includes Vα24–Jα18 (TRAV10-TRAJ18) in humans and Vα14–Jα18 (TRAV 11–TRAJ 18) in mice. The second subset, mucosal-associated invariant T (MAIT) cells, are restricted by the MHC-related protein 1 (MR1) and express Vα7.2–Jα33 (TRAV1-2–TRAJ33) in humans and Vα19–Jα33 (TRAV1–TRAJ33) in mice (1). Vα7.2–Jα33 rearrangement was discovered by Porcelli et al. along with Vα24–Jα28 during analysis of the TCR repertoire of human CD4−CD8− (double-negative; DN) T cells (2). Later, Tilloy et al. discovered homologous Vα19–Jα33 in mice (1). MAIT cells were originally named after their preferential location in the gut lamina propria. Their absence in germ-free mice also indicated their association with mucosal immunity (3). In 2009, Martin et al. generated a monoclonal antibody against human Vα7.2 TCR and demonstrated that Vα7.2TCR+ cells with high expression of CD161 were MAIT cells (4). Human MAIT cells are abundant in peripheral blood and constitute up to 10% of blood CD3+ cells. Because the frequency of iNKT cells in human peripheral blood is 0.01–1%, MAIT cells are 10- to 1,000-fold more frequent than iNKT cells.
Recent studies using MR1 tetramers revealed that not all of the TCR usage of human MAIT cells is restricted to TRAV1-2–TRAJ33 (5). Approximately, 30% of MR1-restricted TRAV1-2+ cells use TRAV1-2 joined with TRAJ20 or TRAJ12 gene segments (5). Moreover, subsets of MR1-resticted T cells do not express TRAV1-2, and their features are discussed elsewhere (6). TRAV1-2–TRAJ33 are mostly paired with TRBV6-6 and TRBV20 (1, 5, 7). In mice, only TRAV1–TRAJ33 (Vα19–Jα33) has been reported as a murine MAIT TCR paired with TRBV13-3 (Vβ8.1), TRBV 13-2 (Vβ8.2), and TRBV19 (Vβ6) (1, 5, 8). The usage of different MAIT TCRs might be related to the tissue distribution of MAIT cells. Vα7.2–Jα33 is the dominant MAIT TCR Vα transcript in human peripheral blood, but the percentages of Vα7.2–Jα12 transcripts are higher than those of Vα7.2–Jα33 transcripts in kidney and intestine biopsies from some individuals (7).
Mait Cell Phenotype
Mucosal-associated invariant T cells in adult blood exhibit the effector memory phenotype (CD95hiCD62LloCD45RO+CD45RAlo CD27+ CD122+) (4, 9). In the thymus and cord blood, MAIT cells display a naïve phenotype and are present at very low numbers (4). However, these MAIT cells already express CD161 in the thymus and CD161 and IL-18Rα in cord blood (10) and produce TNFα in response to Mycobacterium tuberculosis-infected cells (11). MAIT cells also express PLZF, a master regulator of innate-like T cells (8, 12). PLZF expression appears important in the development of MAIT cells because PLZF deficient mice have a significantly lower frequency of MAIT cells. Approximately 95% of human MAIT cells are DN or CD8+. Most MHC-restricted CD8+T cells express the CD8αβ heterodimer, but CD8+ MAIT cells express CD8αα homodimers, and some of them coexpress the CD8αβ heterodimer (4, 5, 13). Approximately 60% of MAIT cells were CD4−CD8− in most tissues of C57BL6/J mice except for lymph nodes where 40% of MAIT cells were CD4+. CD8+ MAIT cells were more frequent in Balb/c mice than in C57Bl/6J mice, but CD4+MAIT cells were also enriched and constituted half of MAIT cells in lymph nodes (8).
Human peripheral blood MAIT cells are CCR5+CCR6+CCR7−CCR9+/− CXCR3−CXCR4+/− CXCR6+ (10, 14, 15). Mouse MAIT cells are CCR6+/− CCR9+/− CXCR6hi but negative for CCR4, CCR7, CXCR1, CXCR3, and CXCR4 (8). Lack of CCR7 and CD62L expression indicates their poor ability to migrate into lymph nodes via high endothelial venules, and expression of CCR9 and CXCR6 suggests their ability to migrate into the intestine and the liver. In fact, human MAIT cells are abundant in peripheral blood and enriched in tissues such as the liver (20–50% of CD3+ cells), intestine (1–10% of CD3+ cells), and lung (2–4% of CD3+ cells) (5, 10, 16–21). Human MAIT cells are also detected in other tissues, including female genital mucosa, kidney, prostate, and ovary (7, 22). FTY720, an agonist of sphingosine-1-phosphate receptors, inhibits the egress of naïve and central memory T and B cells from lymph nodes. FTY720 has been used for treatment of patients with multiple sclerosis (MS). FTY720 treatment decreased the total lymphocyte count but increased MAIT cell frequency; it also reduced DN cells and increased CD8hi and CD4+cells among MAIT cells (23). This finding indicates that MAIT cells are indeed rare in lymph nodes, and tissue distribution may differ among subsets of MAIT cells. Activated MAIT cells may obtain more migrating capacity because IL-18-stimulated MAIT cells express very late antigen-4 (VLA-4), an integrin important for migration into the site of inflammation (24). No antibody against murine Vα19TCR is available, and the frequency of MAIT cells in mice was unknown until the recent development of MR1 tetramers (8). Compared with iNKT cells, MAIT cells are relatively rare in laboratory strains of mice except for CAST/EiJ mice (1, 3, 25). The average frequency of MAIT cells among C57BL/6 mouse lymphocytes is 3.3, 0.7, 0.6, 0.2, 0.08, and 0.05% in the lung, lamina propria, liver, lymph nodes, spleen, and thymus, respectively (8).
Mait Cell Activation Mechanisms
Early studies demonstrated that MAIT cells are deficient in germ-free mice and activated by antigen-presenting cells in the presence of bacteria in an MR1-dependent manner (3, 26, 27). These findings suggested that MAIT cells might recognize microbial antigens presented by the MR1 molecule. Microbes that activated MAIT cells included various types of bacterial species and yeast. In 2012, Kjer-Nielsen et al. described several MR1-restricted antigens. They identified 6-formylpterin (6-FP), a photodegradation product of folic acid (vitamin B9), as an MR1 ligand. 6-FP upregulated surface expression of MR1 but failed to activate MAIT cells. The researchers found that reduced 6-hydroxymethyl-8-d-ribityllumazine (rRL-6-CH2OH) derived from the bacterial riboflavin (vitamin B2) biosynthetic pathway is a MAIT cell-activating MR1 ligand (28). Later, Corbett et al. revealed that some potent MR1 ligands, including 5- (2-oxopropylideneamino)-6-d-ribitylaminouracil (5-OP-RU), are produced by an interaction between early intermediates in the bacterial riboflavin synthesis pathway and either glyoxal or methylglyoxal, and these antigens are unstable unless they are captured and stabilized by the MR1 molecule (29). More recently, several MR1 ligands have been reported among drugs and drug metabolites, such as diclofenac and methotrexate (30). A photodegraded product of aminopterin or methotrexate captured by the MR1 molecule inhibited MAIT cell activation by 5-OP-RU, whereas diclofenac and its metabolites stimulated MAIT cells.
Similar to iNKT cells, MAIT cells are activated by cytokines in an MR1-independent manner (Figure 1). MR1 expression is indispensable for the development of MAIT cells but not for the effector functions of these cells. Our group demonstrated that MAIT cells exacerbated joint inflammation in arthritis models, and MAIT cells exerted their effector function even when they were adoptively transferred into MR1-deficient mice (31). A MAIT cell-enriched population from V19iTCR transgenic (Vα19iTg) mice produced IL-17 after exposure to IL-23 and proliferated upon IL-1β stimulation (31). Inhibition of bacterial growth of Mycobacterium by MAIT cells was more dependent on IL-12-mediated activation of these cells rather than on MR1 antigen recognition by MAIT cells (32). Human MAIT cells express high levels of IL-18Rα and are activated to produce IFNγ by IL-12 plus IL-18 (33–37). MAIT cells are also activated by type I IFN (33, 34). The kinetics of MAIT cell activation upon different types of stimuli might differ as activation of MAIT cells at early time points after incubation with E. coli was MR1-dependent, and IL-12 + IL-18-mediated activation took more time (35). MAIT cells are activated by TCR signals (anti-CD3/CD28) when they are stimulated in the presence of other peripheral blood mononuclear cells, but sorted MAIT cells (CD4−CD8+CD56+CD16−CD161hiVα7.2+ cells) did not respond to TCR signals. However, sorted MAIT cells produced IFNγ and granzyme B when they were activated with TCR signals in the presence of pro-inflammatory signals provided by monocytes activated by TLR agonists. Sorted MAIT cells also produced cytokines, such as IFNγ and TNFα, in response to IL-12/15/18 stimulation (37). Because MAIT cells are enriched in mucosal tissue where MR1 antigens produced by commensal bacteria are present, they may be programed to respond only when they are exposed to such antigens together with inflammatory signals to avoid unwanted tissue inflammation.
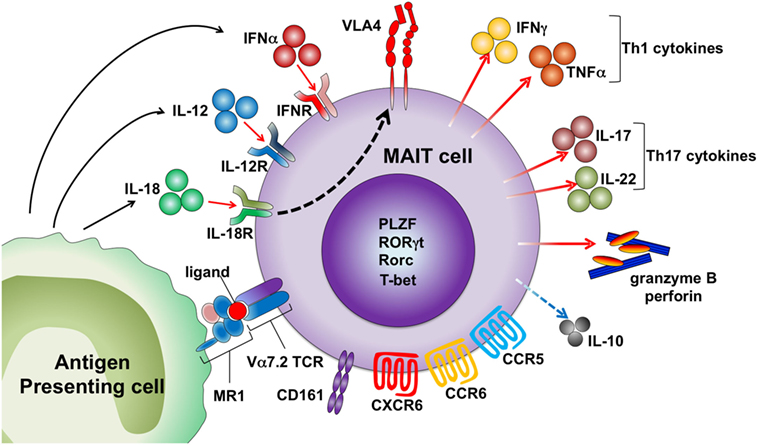
Figure 1. Activation mechanisms of human mucosal-associated invariant T (MAIT) cells. MAIT cells are activated by the MR1 ligand derived from microbes and drugs. Cytokines, including IL-12, IL-18, and IFNα, also activate MAIT cells by an antigen-independent mechanism. MAIT cells express several chemokine and cytokine receptors and homing receptors. Activated MAIT cells upregulate very late antigen-4 (VLA-4) and secrete granzyme B and perforin, Th1/Th17 cytokines, and low levels of IL-10.
Mait Cell Function
Upon stimulation with phorbol 12-myristate13-acetate (PMA) and ionomycin or anti-CD3 and anti-CD28, human MAIT cells produce IFNγ, TNFα, IL-17, IL-2, and granzyme B (10, 14, 26). Mouse spleen MAIT cells produce high levels of IL-17 and MIP-1α and low levels of IL-10, IFNγ, and TNFα following stimulation with PMA and ionomycin or anti-CD3 and anti-CD28 (8). This bias toward IL-17 production was also observed in MAIT cells in various types of organs, such as the thymus and lung (8). Unsurprisingly, mouse MAIT cells express high levels of retinoic acid-related orphan receptor (RORγτ) and low levels of T-bet (8). Human MAIT cells also express Rorc and T-bet; as expected, the expression of T-bet is higher than that of Rorc (10, 38). MAIT cells in different tissues may vary in their cytokine-producing capacity. Mouse thymus MAIT cells also produce other cytokines, such as GM-CSF, IL-4, and IL-13 (8). Mouse MAIT cells from iVα19–Vβ6 transgenic mouse spleen produced IL-2 after stimulation by E. coli-infected dendritic cells (26). In humans, adipose tissue MAIT cells but not peripheral blood MAIT cells produce more IL-10 than IL-17. Interestingly, MAIT cells in adipose tissue from obese individuals produced more IL-17 and less IL-10 (39).
Factors Affecting Mait Cell Frequency and Function
Mucosal-associated invariant T cell numbers are very low in peripheral blood at birth, and their frequency increases with age up to 40–50 years of age (9, 10, 40). Novak et al. studied MAIT cells from individuals at different ages and demonstrated that the frequency of MAIT cells is highest in women of fertile age and significantly declines in elderly individuals (40). MAIT cells are decreased in patients with type 2 diabetes (T2D) and/or obesity (39, 41, 42). Upon stimulation with PMA and ionomycin, MAIT cells from T2D patients produced higher levels of IL-17, and MAIT cells from obese T2D patients produced even higher levels of other cytokines, including IL-2, granzyme B, and IFNγ. In obese patients, MAIT cell frequency was higher in omental adipose tissue than in peripheral blood; moreover, MAIT cell frequency was increased, and cytokine-producing ability was decreased after bariatric surgery. Smoking appears to reduce the frequency of peripheral blood MAIT cells (43). Circulating MAIT cells are affected by not only systemic but also inhaled administration of corticosteroids (43). Some drugs and drug metabolites have been reported as MR1 ligands (30). MR1 ligands derived from microorganisms should be abundantly present in the gut. Although whether the frequency and function of MAIT cells are affected by drugs or indigenous microbes remains unknown, taking the possible influence of these factors into account when studying human MAIT cells might be important.
Mait Cells in Autoimmune and Immunological Diseases
The role of MAIT cells in immunological disorders was largely unknown until our group described their protective role against experimental autoimmune myelitis (EAE), an animal model of MS, and their pro-inflammatory roles in arthritis models. Since anti-Vα7.2 TCR monoclonal antibody has become available, many groups, including ours, have conducted studies on MAIT cells in autoimmune and immunological diseases. MAIT cells appear to be involved in various types of diseases (Figure 2), but their contribution to each pathology is currently unknown. This has been mostly due to the lack of good tools to study murine MAIT cells. MAIT cells are usually rare in mice and there is no monoclonal antibody against murine MAIT cell TCR. New technical approaches such as MR1 tetramers and CAST/EiJ mice may overcome these issues. Here, we review MAIT cells in human immunological diseases and the corresponding animal models. In human studies, MAIT cells are identified by the Vα7.2 TCR and high CD161 expression unless otherwise specified.
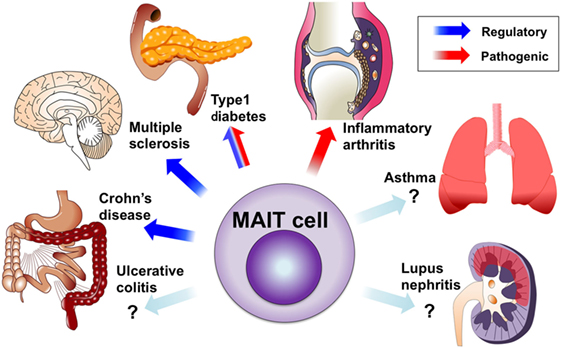
Figure 2. Mucosal-associated invariant T (MAIT) cells in autoimmune and immunological diseases. Circulating MAIT cells are reduced in various autoimmune and immunological diseases. MAIT cells are accumulated or present in inflamed tissues, including the central nervous system, intestine, lungs, and joints. The protective roles of MAIT cells were demonstrated in animal models of multiple sclerosis and Crohn’s disease, as well as onset of type 1 diabetes (T1D). The possible contribution of MAIT cells to tissue inflammation was demonstrated in arthritis models and pancreatitis in T1D.
Multiple Sclerosis
Multiple sclerosis is an inflammatory demyelinating disease affecting the central nervous system (CNS). Although the etiology of MS is not fully understood, MS is considered an autoimmune disease against the myelin component of the CNS. Illes et al. investigated invariant TCR expression in the CNS lesions of patients with MS by using the single-strand conformation polymorphism clonotype method (44). They found very low Vα24–Jα18 expression in MS CNS samples but observed Vα7.2–Jα33 expression in half of MS CNS samples and in most cerebral spinal fluid (CSF) samples. Later, the presence of Vα7.2TCR+CD161+ in MS lesions was confirmed by other groups (24, 45, 46). CD8+MAIT cells are present in MS brain lesions, and approximately 5% of CD8+T cells were Vα7.2TCR+CD161+ cells in acute and chronic active MS lesions, suggesting infiltration of MAIT cells into MS lesions (24). These studies were performed by using MS autopsies, and a more recent study demonstrated Vα7.2–Jα33 transcripts in the brain lesions of a MS patient with newly onset disease (47). There are several conflicting reports regarding the frequency of circulating MAIT cells in MS patients. Most reports demonstrate the reduction of all or subsets of MAIT cells in MS except for one report showing an increase in CD161hi CD8+T cells, and most of these cells are usually MAIT cells (48). Two reports demonstrated the reduction of V7.2TCR+ CD161high cells or CD161high memory CD8+T cells in MS (14, 24). Other groups showed that the frequency of MAIT cells was comparable between MS patients and healthy volunteers (23, 46), but MAIT cells were reduced in patients with progressive disease (46), and CD8hi cells among MAIT cells were decreased in MS (23). IFNβ treatment did not affect the frequency of MAIT cells in MS patients (23), but the frequency of MAIT cells in patients with relapse was increased along with clinical recovery after steroid treatment. Several findings suggest migration of MAIT cells into the CNS. In vitro, IL-18 increased surface expression of VLA-4 on CD8+ MAIT cells, and the frequency of CD8+MAIT cells was inversely correlated with the serum level of IL-18 in MS patients but not in healthy individuals (24). MS MAIT cells overexpress P-selectin glycoprotein ligand-1 (PSGL-1) and CD11a (part of the lymphocyte function-associated antigen 1), which are important for cell rolling and homing though the blood–brain barrier (46).
Therefore, what role do MAIT cells play in the pathogenesis of MS? IFNγ and TNFα production by MAIT cells was decreased in untreated MS patients, but FTY720 treatment recovered the cytokine-producing capacity of MAIT cells (23). Successful treatment of MS with autologous hematopoietic stem cell transplantation was accompanied by depletion of CD8+MAIT cells, whereas regulatory T cells and CD56high natural killer cells were increased in the peripheral blood of MS patients (45). These findings indicated a pro-inflammatory role for MAIT cells in MS, whereas MAIT cells played a protective role in EAE, an animal model of MS (49). The disease development and progression were suppressed in Vα19iTg mice, and MR1-deficient mice developed more severe EAE than did control mice. Adoptive transfer of T cells enriched with a MAIT cell population protected wild-type mice from EAE. Inhibition of EAE in Vα19iTg mice was associated with decreased Th1 and Th17 responses against myelin oligodendrocyte glycoprotein and increased secretion of IL-10. Cytokines produced by Vα19iT cells are different from those produced by human MAIT cells. IL-10 is heavily produced by Vα19iT cells, but human MAIT cells produce very little IL-10. Whether MAIT cells play a protective role in the development of human MS is unknown. As depletion of MAIT cells (CD5+CD19− TCRγδ− CD161highVα7.2TCR+ cells) increased IFNγ production by T cells in vitro, human MAIT cells may also have suppressive effect on other T cells (14).
Systemic Lupus Erythematosus (SLE)
Systemic lupus erythematosus is a systemic autoimmune disease that affects various types of organs, including the skin, kidneys, and CNS. The most characteristic features of SLE are the production of autoantibodies targeting nucleic acids and immune activation by the generation of nucleic acid-containing immune complexes. Thus, the impaired tolerance of T and B cells has been considered one of the major causes of the disease. However, abnormalities in function and number have been reported in innate lymphocytes including natural killer cells and iNKT cells in patients with SLE (50–56). The frequency of MAIT cells was also reduced in the peripheral blood of SLE patients, and the reduction of these cells was more profound than that of γδT cells and iNKT cells (33). We confirmed that the reduction of MAIT cells in SLE was not a result of downregulation of surface markers by single-cell PCR for the expression of Vα7.2–Jα33 TCR. Moreover, the reduction of MAIT cells was not due to the use of corticosteroids in SLE. Lupus MAIT cells were less responsive to stimuli and prone to death, and there were more apoptotic cells among circulating MAIT cells in SLE. Cho et al. also reported the decrease of circulating MAIT cell in SLE patients (57). They showed impaired IFNγ production by lupus MAIT cells that was accompanied by elevated PD-1 expression and an intrinsic defect in the Ca2+/calcineurin/NFAT1 signaling pathway of these cells. In our study, MAIT cells from SLE patients with active disease expressed high levels of CD69, and the activated status of MAIT cells positively correlated with disease activity. Thus, MAIT cells in SLE patients appear to be activated and lost due to activation-induced cell death in vivo; moreover, the remaining MAIT cells are less responsive to stimuli. We elucidated two possible mechanisms of MAIT cell activation. First, monocytes from SLE patients exerted higher MR1 antigen-presenting capacity to MAIT cells. Second, elevated IFNα appeared to be associated with activation of MAIT cells in SLE. Overexpression of type I IFNs and IFN-inducible genes has been reported in SLE patients, and type I IFN is thought to play a central role in the pathogenesis of lupus (58). CD69 expression on MAIT cells positively correlated with serum levels of IL-18 and IFNα in SLE, and exposure to IFNα-induced MAIT cell activation in vitro, suggesting that these cytokines may also contribute to the activation of MAIT cells in SLE. MAIT cells migrate into inflamed tissues including kidneys (59). MAIT cells constitutively express chemokine receptors, and exposure to IL-18 upregulates the surface expression of VLA-4 (24), which mediates T-cell migration through an interaction with vascular cell adhesion molecule (VCAM-1). Urinary levels of IL-18 and VCAM-1 were increased and associated with nephritis activity in SLE (60, 61). DN T cells infiltrated the kidneys in lupus, and the majority of these cells were neither γδT cells nor iNKT cells (62). Thus, MAIT cells may migrate into inflamed tissues in SLE.
Inflammatory Arthritis
Rheumatoid arthritis (RA) is the most common inflammatory arthritis that typically affects the small joints of the hands and feet, and the synovium is the primary site of inflammation. RA is characterized by production of rheumatoid factor (RF) and anti-citrullinated protein antibody. Spondyloarthritis (SpA) is a group of disorders comprising ankylosing spondylitis (AS), psoriatic arthritis, reactive arthritis, arthropathy of inflammatory bowel disease (IBD), and undifferentiated SpA. The features of SpA include the absence of RF and association with HLA-B27; the main targets are the enthesis and axial skeleton. Neutralizing antibodies against the TNFα and IL-6 signaling pathways are widely used in RA treatment; in addition to TNF inhibitors, blocking the IL-23/IL-17 axis is beneficial in AS. Circulating MAIT cells are reduced in patients with RA and SpA including AS (63–65). MAIT cells displayed enhanced IL-17-producing capacity and activated status of these cells correlated with disease activity in AS (63, 64). Cell death of circulating MAIT cells was not enhanced, but they were accumulated in the synovial fluid (SF) in AS. SF MAIT cells displayed high levels of CD69 and enhanced producing capacity of IL-17 and granzyme B in AS. Additionally, SF MAIT cells are enriched in RA (57). Interestingly, IL-17 production by SF MAIT cells was higher in AS than in RA, but TNFα- and IFNγ-producing SF MAIT cells in AS were comparable to those in RA (64). IL-7R polymorphisms are associated with AS, and IL-7 primes MAIT cells (20, 66). Gracey et al. demonstrated that IL-7R expression is increased on AS MAIT cells, and exposure to IL-7 exacerbated the IL-17-producing capacity of AS MAIT cells. Considering their capacity to produce inflammatory cytokines at the site of tissue inflammation, MAIT cells appear to contribute to tissue inflammation in arthritis. In animal models of inflammatory arthritis, MAIT cells enhanced arthritic inflammation (31). DBA1J mice immunized with type II collagen (CII) develop collagen-induced arthritis (CIA), and MR1 deficiency attenuated the disease severity of CIA. Because MR1 deficiency had little effect on T and B cell responses against CII, MAIT cells appeared to contribute to the effector phase of arthritis. In fact, MAIT cell deficiency reduced the disease severity of collagen antibody-induced arthritis (CAIA), and adoptive transfer of a T-cell population enriched with iVα19 TCR+ cells from iVα19 TCR T g mice enhanced CAIA in MR1-deficient mice. This iVα19 TCR+ cell population was activated by IL-1β or IL-23 in the absence of exogenous antigens. Therefore, MAIT cell activation in the CAIA model may be mediated by cytokines and does not require TCR stimulation.
Inflammatory Bowel Diseases
Inflammatory bowel diseases are chronic relapsing disorders of the gastrointestinal tract, comprising Crohn’s disease (CD) and ulcerative colitis (UC). CD affects the distal ileum and colon, and UC involves only the colon. Inflammation in UC is superficial and includes the mucosa and submucosa, whereas CD involves transmural inflammation. The etiology of IBD is not fully understood, but the clinical efficacy of neutralizing antibodies specific for TNFα indicates the role of cytokine-producing immune cells in IBD (67). Both innate and adaptive immune systems appear to contribute to the pathogenesis of IBD. CD is thought to be mediated by Th1 and Th17 cell responses against gut commensal microbiota. UC is believed to be mediated by Th2 responses; however, anti-IL-13 therapy was not beneficial, and cytokines involved in the pathogenesis of UC appear to be more complicated (68, 69). MAIT cells are reduced in the peripheral blood of patients with CD and UC (18, 70–72). However, IL-17 production by MAIT cells was increased in UC patients (71). CD69 expression on MAIT cells was associated with disease activity. Increased IL-17 production by MAIT cells and correlation of CD69 expression on these cells with disease activity indicated the association of MAIT cells with the pathogenesis of UC. T cells expressing CD161, IL-23R and RORγt are enriched in intestinal mucosa from patients with IBD; thus, MAIT cells may be associated with tissue inflammation in IBD (67, 73, 74). In fact, MAIT cells accumulated in the inflamed mucosa of patients with CD and UC (18, 71, 72). Plasma IL-18 levels were positively correlated with CD69 expression on MAIT cells in UC. Thus, the reduction of circulating MAIT cells may be a result of the recruitment of these cells to the inflamed tissue. A report by Hiejima et al. showed reduced MAIT cell frequency in intestinal mucosa from IBD patients (70). They demonstrated enhanced cell death of MAIT cells in peripheral blood in IBD patients and inflamed mucosa of those with CD. Further studies are required to understand the differences among reports and their function in IBD pathology. One report demonstrated that adoptive transfer of Jα33+ cells into mice reduced the severity of intestine inflammation in 2,4,6-trinitrobenzene sulfonic acid (TNBS) colitis, suggesting their protective role in an animal model of CD (75).
Type 1 Diabetes (T1D)
Harms et al. investigated CD161bright CD8+ T cells in patients with juvenile T1D whose clinical onset was within 12 months and demonstrated there was no reduction of these cells in the peripheral blood of patients (76). The frequency of CD27−MAIT cells was increased in patients, and these cells displayed more enhanced IL-17-producing capacity. CD161bright CD8+T cells increased with age in control individuals but not in juvenile T1D patients, suggesting that circulating MAIT cells may be decreased in patients with long-standing T1D. More recently, a lower frequency of MAIT cells was detected in children with recent onset T1D than in control children (77). MAIT cells from T1D patients expressed increased levels of CD25 and PD-1 and displayed enhanced cytokine production, including TNFα and granzyme B. Because human MAIT cells exerted cytotoxic activity against a pancreatic β-cell line, MAIT cells may contribute to β-cell destruction in T1D.
In non-obese diabetic (NOD) mice, the frequency and number of MAIT cells was lower in the spleen and pancreatic lymph nodes of NOD mice than in those of C57BL/6 mice. MAIT cells were present in pancreatic islets and enriched in the ileum of NOD mice, and these MAIT cells exhibited a more activated phenotype. The recruitment of MAIT cells into the ileum decreased and that into the pancreas increased with aging. IFNγ and granzyme B production by islet MAIT cells was already observed in prediabetic mice and further increased in diabetic mice. These findings indicate that MAIT cells are recruited to the pancreas and contribute to tissue inflammation. However, MR1 deficiency increased the rates of diabetes in NOD mice and the streptozotocin-induced T1D model (77). MR1 deficiency increased intestinal permeability, and this issue was associated with increased infiltration of lymphoid cells into the lamina propria and more bacterial translocation from the gut to pancreatic lymph nodes. Thus, MAIT cells appear to be important for the maintenance of tissue integrity, but they contribute to tissue damage once inflammation occurs.
Asthma
Asthma encompasses chronic airway inflammation characterized by increased airway hypersensitivity to various types of antigen-specific and non-specific stimuli. Th2 cytokines, such as IL-4, IL-5, and IL-13, play important roles in activating other cells including eosinophils. MAIT cell frequency was reduced in patients with asthma in peripheral blood, sputum, and endobronchial biopsy specimens (78, 79). The reduction in MAIT cell frequency was associated with disease severity, inhaled corticosteroid dose, respiratory function, and disease duration. Moreover, CD69+MAIT cells were associated with respiratory function (80). MAIT cells are present in the lung at similar or higher frequencies than those in peripheral blood and are enriched in the lung under inflammatory conditions, including infection (6, 81). Thus, MAIT cells may be involved in the pathogenesis of asthma, but it is difficult to determine the role MAIT cells play in asthma because human MAIT cells mostly produce Th1 and Th17 type cytokines. Therefore, studies of MAIT cells at the site of inflammation or using animal models are required to understand their role in asthma.
Concluding Remarks
Mucosal-associated invariant T cells appear to be involved in various types of immune disorders, and circulating MAIT cells were reduced in most diseases. We speculate that these findings are due to their unique characteristics. MAIT cells are very sensitive to stimuli, can be activated by antigens and cytokines, and have the capacity to migrate to inflamed tissues. There are several conflicting findings regarding MAIT cell frequencies in some diseases among different research groups. This discrepancy may be due to the methodology used to identify MAIT cells. Other potential reasons include the influence of factors, such as age, gender, obesity, and smoking, on MAIT cells (43). MR1 ligands derived from commensal microbes or drugs could modify MAIT cell function or frequency. Thus, these factors might have influenced these different findings. Studies using new tools such as MR1 tetramers and MR1 ligands may answer these questions and determine the potential of MAIT cells as a therapeutic target in immune diseases.
Author Contributions
AC, GM, and SM wrote the manuscript. Both AC and SM contributed equally to this work. GM drew schematics.
Conflict of Interest Statement
The authors declare that the research was conducted in the absence of any commercial relationships that could be construed as a potential conflict of interest.
Funding
This study was supported by the Japan Society for the Promotion of Science [Grant-in-Aid for Scientific Research (C) 17K09983 (to AC) and Grant-in-Aid for Scientific Research (B) 17H04218 (to SM)].
References
1. Tilloy F, Treiner E, Park SH, Garcia C, Lemonnier F, de la Salle H, et al. An invariant T cell receptor alpha chain defines a novel TAP-independent major histocompatibility complex class Ib-restricted alpha/beta T cell subpopulation in mammals. J Exp Med (1999) 189(12):1907–21. doi:10.1084/jem.189.12.1907
2. Porcelli SYC, Brenner MB, Balk SP. Analysis of T cell antigen receptor (TCR) expression by human peripheral blood CD4-8- alpha/beta T cells demonstrates preferential use of several V beta genes and an invariant TCR alpha chain. J Exp Med (1993) 178(1):1–16. doi:10.1084/jem.178.1.1
3. Treiner E, Duban L, Bahram S, Radosavljevic M, Wanner V, Tilloy F, et al. Selection of evolutionarily conserved mucosal-associated invariant T cells by MR1. Nature (2003) 422(6928):164–9. doi:10.1038/nature01433
4. Martin E, Treiner E, Duban L, Guerri L, Laude H, Toly C, et al. Stepwise development of MAIT cells in mouse and human. PLoS Biol (2009) 7(3):e54. doi:10.1371/journal.pbio.1000054
5. Reantragoon R, Corbett AJ, Sakala IG, Gherardin NA, Furness JB, Chen Z, et al. Antigen-loaded MR1 tetramers define T cell receptor heterogeneity in mucosal-associated invariant T cells. J Exp Med (2013) 210(11):2305–20. doi:10.1084/jem.20130958
6. Moreira ML, Tsuji M, Corbett AJ, Araujo MSS, Teixeira-Carvalho A, Martins-Filho OA, et al. MAIT-cells: a tailor-made mate in the ancient battle against infectious diseases? Immunol Lett (2017) 187:53–60. doi:10.1016/j.imlet.2017.05.007
7. Lepore M, Kalinichenko A, Colone A, Paleja B, Singhal A, Tschumi A, et al. Parallel T-cell cloning and deep sequencing of human MAIT cells reveal stable oligoclonal TCRbeta repertoire. Nat Commun (2014) 5:3866. doi:10.1038/ncomms4866
8. Rahimpour A, Koay HF, Enders A, Clanchy R, Eckle SB, Meehan B, et al. Identification of phenotypically and functionally heterogeneous mouse mucosal-associated invariant T cells using MR1 tetramers. J Exp Med (2015) 212(7):1095–108. doi:10.1084/jem.20142110
9. Koay HF, Gherardin NA, Enders A, Loh L, Mackay LK, Almeida CF, et al. A three-stage intrathymic development pathway for the mucosal-associated invariant T cell lineage. Nat Immunol (2016) 17(11):1300–11. doi:10.1038/ni.3565
10. Dusseaux M, Martin E, Serriari N, Peguillet I, Premel V, Louis D, et al. Human MAIT cells are xenobiotic-resistant, tissue-targeted, CD161hi IL-17-secreting T cells. Blood (2011) 117(4):1250–9. doi:10.1182/blood-2010-08-303339
11. Gold MC, Eid T, Smyk-Pearson S, Eberling Y, Swarbrick GM, Langley SM, et al. Human thymic MR1-restricted MAIT cells are innate pathogen-reactive effectors that adapt following thymic egress. Mucosal Immunol (2013) 6(1):35–44. doi:10.1038/mi.2012.45
12. Gerart S, Siberil S, Martin E, Lenoir C, Aguilar C, Picard C, et al. Human iNKT and MAIT cells exhibit a PLZF-dependent proapoptotic propensity that is counterbalanced by XIAP. Blood (2013) 121(4):614–23. doi:10.1182/blood-2012-09-456095
13. Walker LJ, Kang YH, Smith MO, Tharmalingham H, Ramamurthy N, Fleming VM, et al. Human MAIT and CD8alphaalpha cells develop from a pool of type-17 precommitted CD8+ T cells. Blood (2012) 119(2):422–33. doi:10.1182/blood-2011-05-353789
14. Miyazaki Y, Miyake S, Chiba A, Lantz O, Yamamura T. Mucosal-associated invariant T cells regulate Th1 response in multiple sclerosis. Int Immunol (2011) 23(9):529–35. doi:10.1093/intimm/dxr047
15. Mpina M, Maurice NJ, Yajima M, Slichter CK, Miller HW, Dutta M, et al. Controlled human malaria infection leads to long-lasting changes in innate and innate-like lymphocyte populations. J Immunol (2017) 199(1):107–18. doi:10.4049/jimmunol.1601989
16. Cosgrove C, Ussher JE, Rauch A, Gartner K, Kurioka A, Huhn MH, et al. Early and nonreversible decrease of CD161++/MAIT cells in HIV infection. Blood (2013) 121(6):951–61. doi:10.1182/blood-2012-06-436436
17. Leeansyah E, Ganesh A, Quigley MF, Sonnerborg A, Andersson J, Hunt PW, et al. Activation, exhaustion, and persistent decline of the antimicrobial MR1-restricted MAIT-cell population in chronic HIV-1 infection. Blood (2013) 121(7):1124–35. doi:10.1182/blood-2012-07-445429
18. Serriari NE, Eoche M, Lamotte L, Lion J, Fumery M, Marcelo P, et al. Innate mucosal-associated invariant T (MAIT) cells are activated in inflammatory bowel diseases. Clin Exp Immunol (2014) 176(2):266–74. doi:10.1111/cei.12277
19. Fergusson JR, Huhn MH, Swadling L, Walker LJ, Kurioka A, Llibre A, et al. CD161(int)CD8+ T cells: a novel population of highly functional, memory CD8+ T cells enriched within the gut. Mucosal Immunol (2016) 9(2):401–13. doi:10.1038/mi.2015.69
20. Tang XZ, Jo J, Tan AT, Sandalova E, Chia A, Tan KC, et al. IL-7 licenses activation of human liver intrasinusoidal mucosal-associated invariant T cells. J Immunol (2013) 190(7):3142–52. doi:10.4049/jimmunol.1203218
21. Jeffery HC, van Wilgenburg B, Kurioka A, Parekh K, Stirling K, Roberts S, et al. Biliary epithelium and liver B cells exposed to bacteria activate intrahepatic MAIT cells through MR1. J Hepatol (2016) 64(5):1118–27. doi:10.1016/j.jhep.2015.12.017
22. Gibbs A, Leeansyah E, Introini A, Paquin-Proulx D, Hasselrot K, Andersson E, et al. MAIT cells reside in the female genital mucosa and are biased towards IL-17 and IL-22 production in response to bacterial stimulation. Mucosal Immunol (2017) 10(1):35–45. doi:10.1038/mi.2016.30
23. Sugimoto C, Hirotani M, Yoshikiyo K, Koshimizu U, Wakao R, Horinouchi T, et al. The dynamics of mucosal-associated invariant T cells in multiple sclerosis. Springerplus (2016) 5(1):1259. doi:10.1186/s40064-016-2923-9
24. Willing A, Leach OA, Ufer F, Attfield KE, Steinbach K, Kursawe N, et al. CD8(+) MAIT cells infiltrate into the CNS and alterations in their blood frequencies correlate with IL-18 serum levels in multiple sclerosis. Eur J Immunol (2014) 44(10):3119–28. doi:10.1002/eji.201344160
25. Cui Y, Franciszkiewicz K, Mburu YK, Mondot S, Le Bourhis L, Premel V, et al. Mucosal-associated invariant T cell-rich congenic mouse strain allows functional evaluation. J Clin Invest (2015) 125(11):4171–85. doi:10.1172/JCI82424
26. Le Bourhis L, Martin E, Péguillet I, Guihot A, Froux N, Coré M, et al. Antimicrobial activity of mucosal-associated invariant T cells. Nat Immunol (2010) 11(8):701–8. doi:10.1038/ni.1890
27. Gold MC, Cerri S, Smyk-Pearson S, Cansler ME, Vogt TM, Delepine J, et al. Human mucosal associated invariant T cells detect bacterially infected cells. PLoS Biol (2010) 8(6):e1000407. doi:10.1371/journal.pbio.1000407
28. Kjer-Nielsen L, Patel O, Corbett AJ, Le Nours J, Meehan B, Liu L, et al. MR1 presents microbial vitamin B metabolites to MAIT cells. Nature (2012) 491(7426):717–23. doi:10.1038/nature11605
29. Corbett AJ, Eckle SB, Birkinshaw RW, Liu L, Patel O, Mahony J, et al. T-cell activation by transitory neo-antigens derived from distinct microbial pathways. Nature (2014) 509(7500):361–5. doi:10.1038/nature13160
30. Keller AN, Eckle SB, Xu W, Liu L, Hughes VA, Mak JY, et al. Drugs and drug-like molecules can modulate the function of mucosal-associated invariant T cells. Nat Immunol (2017) 18(4):402–11. doi:10.1038/ni.3679
31. Chiba A, Mizuno M, Tomi C, Tajima R, Alloza I, di Penta A, et al. A 4-trifluoromethyl analogue of celecoxib inhibits arthritis by suppressing innate immune cell activation. Arthritis Res Ther (2012) 14(1):R9. doi:10.1186/ar3683
32. Chua WJ, Truscott SM, Eickhoff CS, Blazevic A, Hoft DF, Hansen TH. Polyclonal mucosa-associated invariant T cells have unique innate functions in bacterial infection. Infect Immun (2012) 80(9):3256–67. doi:10.1128/Iai.00279-12
33. Chiba A, Tamura N, Yoshikiyo K, Murayama G, Kitagaichi M, Yamaji K, et al. Activation status of mucosal-associated invariant T cells reflects disease activity and pathology of systemic lupus erythematosus. Arthritis Res Ther (2017) 19(1):58. doi:10.1186/s13075-017-1257-5
34. van Wilgenburg B, Scherwitzl I, Hutchinson EC, Leng T, Kurioka A, Kulicke C, et al. MAIT cells are activated during human viral infections. Nat Commun (2016) 7:11653. doi:10.1038/ncomms11653
35. Ussher JE, Bilton M, Attwod E, Shadwell J, Richardson R, de Lara C, et al. CD161++ CD8+ T cells, including the MAIT cell subset, are specifically activated by IL-12+IL-18 in a TCR-independent manner. Eur J Immunol (2014) 44(1):195–203. doi:10.1002/eji.201343509
36. Jo J, Tan AT, Ussher JE, Sandalova E, Tang XZ, Tan-Garcia A, et al. Toll-like receptor 8 agonist and bacteria trigger potent activation of innate immune cells in human liver. PLoS Pathog (2014) 10(6):e1004210. doi:10.1371/journal.ppat.1004210
37. Slichter CK, McDavid A, Miller HW, Finak G, Seymour BJ, McNevin JP, et al. Distinct activation thresholds of human conventional and innate-like memory T cells. JCI Insight (2016) 1(8):e86292. doi:10.1172/jci.insight.86292
38. Gherardin NA, Keller AN, Woolley RE, Le Nours J, Ritchie DS, Neeson PJ, et al. Diversity of T cells restricted by the mhc class I-related molecule MR1 facilitates differential antigen recognition. Immunity (2016) 44(1):32–45. doi:10.1016/j.immuni.2015.12.005
39. Carolan E, Tobin LM, Mangan BA, Corrigan M, Gaoatswe G, Byrne G, et al. Altered distribution and increased IL-17 production by mucosal-associated invariant T cells in adult and childhood obesity. J Immunol (2015) 194(12):5775–80. doi:10.4049/jimmunol.1402945
40. Novak J, Dobrovolny J, Novakova L, Kozak T. The decrease in number and change in phenotype of mucosal-associated invariant T cells in the elderly and differences in men and women of reproductive age. Scand J Immunol (2014) 80(4):271–5. doi:10.1111/sji.12193
41. Magalhaes I, Pingris K, Poitou C, Bessoles S, Venteclef N, Kiaf B, et al. Mucosal-associated invariant T cell alterations in obese and type 2 diabetic patients. J Clin Invest (2015) 125(4):1752–62. doi:10.1172/JCI78941
42. Magalhaes I, Kiaf B, Lehuen A. iNKT and MAIT cell alterations in diabetes. Front Immunol (2015) 6:341. doi:10.3389/fimmu.2015.00341
43. Ammitzboll C, Bornsen L, Romme Christensen J, Ratzer R, Romme Nielsen B, Sondergaard HB, et al. Smoking reduces circulating CD26hiCD161hi MAIT cells in healthy individuals and patients with multiple sclerosis. J Leukoc Biol (2017) 101(5):1211–20. doi:10.1189/jlb.3A0616-267R
44. Illes Z. Accumulation of V 7.2-J 33 invariant T cells in human autoimmune inflammatory lesions in the nervous system. Int Immunol (2004) 16(2):223–30. doi:10.1093/intimm/dxh018
45. Abrahamsson SV, Angelini DF, Dubinsky AN, Morel E, Oh U, Jones JL, et al. Non-myeloablative autologous haematopoietic stem cell transplantation expands regulatory cells and depletes IL-17 producing mucosal-associated invariant T cells in multiple sclerosis. Brain (2013) 136(Pt 9):2888–903. doi:10.1093/brain/awt182
46. Salou M, Nicol B, Garcia A, Baron D, Michel L, Elong-Ngono A, et al. Neuropathologic, phenotypic and functional analyses of mucosal associated invariant T cells in multiple sclerosis. Clin Immunol (2016) 16(6–167):1–11. doi:10.1016/j.clim.2016.03.014
47. Held K, Bhonsle-Deeng L, Siewert K, Sato W, Beltran E, Schmidt S, et al. alphabeta T-cell receptors from multiple sclerosis brain lesions show MAIT cell-related features. Neurol Neuroimmunol Neuroinflamm (2015) 2(4):e107. doi:10.1212/NXI.0000000000000107
48. Annibali V, Ristori G, Angelini DF, Serafini B, Mechelli R, Cannoni S, et al. CD161(high)CD8+T cells bear pathogenetic potential in multiple sclerosis. Brain (2011) 134(Pt 2):542–54. doi:10.1093/brain/awq354
49. Croxford JL, Miyake S, Huang YY, Shimamura M, Yamamura T. Invariant V(alpha)19i T cells regulate autoimmune inflammation. Nat Immunol (2006) 7(9):987–94. doi:10.1038/ni1370
50. Wang L, Kang N, Zhou J, Guo Y, Zhang X, Cui L, et al. Downregulation of CD94/NKG2A inhibitory receptor on decreased gammadelta T cells in patients with systemic lupus erythematosus. Scand J Immunol (2012) 76(1):62–9. doi:10.1111/j.1365-3083.2012.02705.x
51. Cho YN, Kee SJ, Lee SJ, Seo SR, Kim TJ, Lee SS, et al. Numerical and functional deficiencies of natural killer T cells in systemic lupus erythematosus: their deficiency related to disease activity. Rheumatology (Oxford) (2011) 50(6):1054–63. doi:10.1093/rheumatology/keq457
52. Wither J, Cai YC, Lim S, McKenzie T, Roslin N, Claudio JO, et al. Reduced proportions of natural killer T cells are present in the relatives of lupus patients and are associated with autoimmunity. Arthritis Res Ther (2008) 10(5):R108. doi:10.1186/ar2505
53. Green MR, Kennell AS, Larche MJ, Seifert MH, Isenberg DA, Salaman MR. Natural killer cell activity in families of patients with systemic lupus erythematosus: demonstration of a killing defect in patients. Clin Exp Immunol (2005) 141(1):165–73. doi:10.1111/j.1365-2249.2005.02822.x
54. Baxter AG, Smyth MJ. The role of NK cells in autoimmune disease. Autoimmunity (2002) 35(1):1–14. doi:10.1080/08916930290005864
55. Oishi Y, Sumida T, Sakamoto A, Kita Y, Kurasawa K, Nawata Y, et al. Selective reduction and recovery of invariant Valpha24JalphaQ T cell receptor T cells in correlation with disease activity in patients with systemic lupus erythematosus. J Rheumatol (2001) 28(2):275–83.
56. Kojo S, Adachi Y, Keino H, Taniguchi M, Sumida T. Dysfunction of T cell receptor AV24AJ18+, BV11+ double-negative regulatory natural killer T cells in autoimmune diseases. Arthritis Rheum (2001) 44(5):1127–38. doi:10.1002/1529-0131(200105)44:5<1127::AID-ANR194>3.0.CO;2-W
57. Cho YN, Kee SJ, Kim TJ, Jin HM, Kim MJ, Jung HJ, et al. Mucosal-associated invariant T cell deficiency in systemic lupus erythematosus. J Immunol (2014) 193(8):3891–901. doi:10.4049/jimmunol.1302701
58. Crow MK. Type I interferon in the pathogenesis of lupus. J Immunol (2014) 192(12):5459–68. doi:10.4049/jimmunol.1002795
59. Peterfalvi A, Gomori E, Magyarlaki T, Pal J, Banati M, Javorhazy A, et al. Invariant V 7.2-J 33 TCR is expressed in human kidney and brain tumors indicating infiltration by mucosal-associated invariant T (MAIT) cells. Int Immunol (2008) 20(12):1517–25. doi:10.1093/intimm/dxn111
60. Favilli F, Anzilotti C, Martinelli L, Quattroni P, De Martino S, Pratesi F, et al. IL-18 activity in systemic lupus erythematosus. Ann N Y Acad Sci (2009) 1173:301–9. doi:10.1111/j.1749-6632.2009.04742.x
61. Singh S, Wu T, Xie C, Vanarsa K, Han J, Mahajan T, et al. Urine VCAM-1 as a marker of renal pathology activity index in lupus nephritis. Arthritis Res Ther (2012) 14(4):R164. doi:10.1186/ar3912
62. Crispin JC, Oukka M, Bayliss G, Cohen RA, Van Beek CA, Stillman IE, et al. Expanded double negative T cells in patients with systemic lupus erythematosus produce IL-17 and infiltrate the kidneys. J Immunol (2008) 181(12):8761–6. doi:10.4049/jimmunol.181.12.8761
63. Hayashi E, Chiba A, Tada K, Haga K, Kitagaichi M, Nakajima S, et al. Involvement of mucosal-associated invariant T cells in ankylosing spondylitis. J Rheumatol (2016) 43(9):1695–703. doi:10.3899/jrheum.151133
64. Gracey E, Qaiyum Z, Almaghlouth I, Lawson D, Karki S, Avvaru N, et al. IL-7 primes IL-17 in mucosal-associated invariant T (MAIT) cells, which contribute to the Th17-axis in ankylosing spondylitis. Ann Rheum Dis (2016) 75(12):2124–32. doi:10.1136/annrheumdis-2015-208902
65. Sugimoto C, Konno T, Wakao R, Fujita H, Fujita H, Wakao H. Mucosal-associated invariant T cell is a potential marker to distinguish fibromyalgia syndrome from arthritis. PLoS One (2015) 10(4):e0121124. doi:10.1371/journal.pone.0121124
66. Cortes A, Hadler J, Pointon JP, Robinson PC, Karaderi T, Leo P, et al. Identification of multiple risk variants for ankylosing spondylitis through high-density genotyping of immune-related loci. Nat Genet (2013) 45(7):730–8. doi:10.1038/ng.2667
67. Neurath MF. Cytokines in inflammatory bowel disease. Nat Rev Immunol (2014) 14(5):329–42. doi:10.1038/nri3661
68. Reinisch W, Panes J, Khurana S, Toth G, Hua F, Comer GM, et al. Anrukinzumab, an anti-interleukin 13 monoclonal antibody, in active UC: efficacy and safety from a phase IIa randomised multicentre study. Gut (2015) 64(6):894–900. doi:10.1136/gutjnl-2014-308337
69. Danese S, Rudzinski J, Brandt W, Dupas JL, Peyrin-Biroulet L, Bouhnik Y, et al. Tralokinumab for moderate-to-severe UC: a randomised, double-blind, placebo-controlled, phase IIa study. Gut (2015) 64(2):243–9. doi:10.1136/gutjnl-2014-308004
70. Hiejima E, Kawai T, Nakase H, Tsuruyama T, Morimoto T, Yasumi T, et al. Reduced numbers and proapoptotic features of mucosal-associated invariant T cells as a characteristic finding in patients with inflammatory bowel disease. Inflamm Bowel Dis (2015) 21(7):1529–40. doi:10.1097/MIB.0000000000000397
71. Haga K, Chiba A, Shibuya T, Osada T, Ishikawa D, Kodani T, et al. MAIT cells are activated and accumulated in the inflamed mucosa of ulcerative colitis. J Gastroenterol Hepatol (2016) 31(5):965–72. doi:10.1111/jgh.13242
72. Tominaga K, Yamagiwa S, Setsu T, Kimura N, Honda H, Kamimura H, et al. Possible involvement of mucosal-associated invariant T cells in the progression of inflammatory bowel diseases. Biomed Res (2017) 38(2):111–21. doi:10.2220/biomedres.38.111
73. Kleinschek MA, Boniface K, Sadekova S, Grein J, Murphy EE, Turner SP, et al. Circulating and gut-resident human Th17 cells express CD161 and promote intestinal inflammation. J Exp Med (2009) 206(3):525–34. doi:10.1084/jem.20081712
74. Kobayashi T, Okamoto S, Hisamatsu T, Kamada N, Chinen H, Saito R, et al. IL23 differentially regulates the Th1/Th17 balance in ulcerative colitis and Crohn’s disease. Gut (2008) 57(12):1682–9. doi:10.1136/gut.2007.135053
75. Ruijing X, Mengjun W, Xiaoling Z, Shu P, Mei W, Yingcheng Z, et al. Jalpha33+ MAIT cells play a protective role in TNBS induced intestinal inflammation. Hepatogastroenterology (2012) 59(115):762–7. doi:10.5754/hge11432
76. Harms RZ, Lorenzo KM, Corley KP, Cabrera MS, Sarvetnick NE. Altered CD161 bright CD8+ mucosal associated invariant T (MAIT)-like cell dynamics and increased differentiation states among juvenile type 1 diabetics. PLoS One (2015) 10(1):e0117335. doi:10.1371/journal.pone.0117335
77. Rouxel O, Da Silva J, Beaudoin L, Nel I, Tard C, Cagninacci L, et al. Cytotoxic and regulatory roles of mucosal-associated invariant T cells in type 1 diabetes. Nat Immunol (2017) 18:1321–31. doi:10.1038/ni.3854
78. Hinks TS, Zhou X, Staples KJ, Dimitrov BD, Manta A, Petrossian T, et al. Innate and adaptive T cells in asthmatic patients: relationship to severity and disease mechanisms. J Allergy Clin Immunol (2015) 136(2):323–33. doi:10.1016/j.jaci.2015.01.014
79. Hinks TS. Mucosal-associated invariant T cells in autoimmunity, immune-mediated diseases and airways disease. Immunology (2016) 148(1):1–12. doi:10.1111/imm.12582
80. Ishimori A, Harada N, Chiba A, Harada S, Matsuno K, Makino F, et al. Circulating activated innate lymphoid cells and mucosal-associated invariant T cells are associated with airflow limitation in patients with asthma. Allergol Int (2017) 66(2):302–9. doi:10.1016/j.alit.2016.07.005
Keywords: mucosal-associated invariant T cells, multiple sclerosis, systemic lupus erythematosus, inflammatory arthritis, inflammatory bowel diseases, diabetes, asthma
Citation: Chiba A, Murayama G and Miyake S (2018) Mucosal-Associated Invariant T Cells in Autoimmune Diseases. Front. Immunol. 9:1333. doi: 10.3389/fimmu.2018.01333
Received: 30 March 2018; Accepted: 29 May 2018;
Published: 11 June 2018
Edited by:
Kazuya Iwabuchi, Kitasato University School of Medicine, JapanReviewed by:
Paula M. Oliver, University of Pennsylvania, United StatesAgnes Lehuen, Institut National de la Santé et de la Recherche Médicale (INSERM), France
Copyright: © 2018 Chiba, Murayama and Miyake. This is an open-access article distributed under the terms of the Creative Commons Attribution License (CC BY). The use, distribution or reproduction in other forums is permitted, provided the original author(s) and the copyright owner are credited and that the original publication in this journal is cited, in accordance with accepted academic practice. No use, distribution or reproduction is permitted which does not comply with these terms.
*Correspondence: Asako Chiba, YS1jaGliYSYjeDAwMDQwO2p1bnRlbmRvLmFjLmpw;
Sachiko Miyake, cy1taXlha2UmI3gwMDA0MDtqdW50ZW5kby5hYy5qcA==