- 1Industrial Biotechnology Centre and School of Biosciences, University of Kent, Canterbury, United Kingdom
- 2Department of Microbiology, Immunology and Genetics, Faculty of Health Sciences, Ben-Gurion University of the Negev, Beer-Sheva, Israel
- 3Department of Emerging and Re-Emerging Diseases and Special Pathogens, Uganda Virus Research Institute (UVRI), Entebbe, Uganda
- 4Research & Development Institute, National Infection Service, Public Health England, Porton Down, Salisbury, United Kingdom
The recent West African Ebola virus pandemic, which affected >28,000 individuals increased interest in anti-Ebolavirus vaccination programs. Here, we systematically analyzed the requirements for a prophylactic vaccination program based on the basic reproductive number (R0, i.e., the number of secondary cases that result from an individual infection). Published R0 values were determined by systematic literature research and ranged from 0.37 to 20. R0s ≥ 4 realistically reflected the critical early outbreak phases and superspreading events. Based on the R0, the herd immunity threshold (Ic) was calculated using the equation Ic = 1 − (1/R0). The critical vaccination coverage (Vc) needed to provide herd immunity was determined by including the vaccine effectiveness (E) using the equation Vc = Ic/E. At an R0 of 4, the Ic is 75% and at an E of 90%, more than 80% of a population need to be vaccinated to establish herd immunity. Such vaccination rates are currently unrealistic because of resistance against vaccinations, financial/logistical challenges, and a lack of vaccines that provide long-term protection against all human-pathogenic Ebolaviruses. Hence, outbreak management will for the foreseeable future depend on surveillance and case isolation. Clinical vaccine candidates are only available for Ebola viruses. Their use will need to be focused on health-care workers, potentially in combination with ring vaccination approaches.
Introduction
The genus Ebolavirus contains five species: Zaire ebolavirus (type virus: Ebola virus), Sudan ebolavirus (type virus: Sudan virus), Bundibugyo ebolavirus (type virus: Bundibugyo virus), Taï Forest ebolavirus (type virus: Taï Forest virus, previously also referred to by names such as Côte d’Ivoire ebolavirus or Ivory Coast ebolavirus), Reston ebolavirus (type virus: Reston virus) (1). Four Ebolaviruses (Ebola virus, Sudan virus, Bundibugyo virus, Taï Forrest virus) are endemic to Africa and can cause severe disease in humans (2). Reston viruses are endemic to Asia and considered to be non-pathogenic in humans (2). However, very few genetic changes may result in human-pathogenic Reston viruses (2–4). Since the discovery of the first two members of the Ebolavirus family in 1976 in Sudan (today South Sudan) and Zaïre (today Democratic Republic of Congo), Ebolaviruses had until 2013 only caused small outbreaks in humans affecting up to a few 100 individuals (5, 6). The recent Ebola virus outbreak in West Africa (2013–2016) resulted in 28,616 confirmed, probable, and suspected cases of Ebola virus disease and 11,310 deaths (6), which may still underestimate the actual numbers (7). It was the first Ebolavirus outbreak that affected multiple countries, was introduced to another country via air travel, and resulted in a significant number of human disease cases outside of Africa (5, 6). Prior to this outbreak, only isolated human cases were treated outside of Africa. A scientist who had become infected by Taï Forest virus after an autopsy of a Chimpanzee was treated in Switzerland (8), and two laboratory infections were reported in Russia (9, 10). In addition, Reston virus-infected non-human primates were exported from the Philippines to the US and Italy (11). Finally, Marburg virus (which belongs like the Ebolaviruses to the Filoviruses) was exported out of Africa (12, 13) and was associated with laboratory infections (14, 15). Due to its unique size, the West African Ebolavirus outbreak emphasized the health threats posed by Ebolaviruses and the importance of protection strategies (6, 7).
Vaccination programs are effective in controlling infectious diseases, as demonstrated by the WHO-driven smallpox eradication (16). However, eradication is likely to be more difficult for zoonotic viruses like the Ebolaviruses that circulate in animal reservoirs (17). Only herd immunity could prevent future outbreaks and protect individuals that cannot be vaccinated due to health issues (16). The herd immunity threshold (lc) describes the number of society members that need to be protected (18) to prevent outbreaks. It is based on the basic reproductive number R0 (number of secondary cases caused per primary case) of a pathogen (18–22).
Here, we performed a systematic analysis to determine the critical vaccine coverage (Vc) required to prevent Ebolavirus outbreaks by a prophylactic mass vaccination program based on the R0 associated with Ebolavirus infection in humans. The results were further critically considered in the context of (1) the status of current Ebolavirus vaccine candidates and (2) the feasibility of a large-scale prophylactic Ebolavirus vaccination program taking into account (a) the preparedness to participate in vaccination programs in the affected societies, (b) logistic challenges, and (c) costs.
Materials and Methods
Identification of Studies That Report on the Basic Reproductive Number (R0) of Ebolaviruses
To identify scientific articles that have calculated the basic reproductive number (R0) for Ebolaviruses, we performed a literature search using PubMed (www.ncbi.nlm.nih.gov/pubmed) for the search term combinations “Ebola R0,” “Ebola basic reproductive number,” and “Ebola basic reproduction number” (retrieved on 29th September 2017).
Determination of Herd Immunity Thresholds and Their Implications for Ebolavirus Diseases Prevention Strategies
Based on the basic reproductive number R0, i.e., the number of secondary cases that result from an individual infection, the herd immunity threshold (Ic) was calculated using Eq. 1.
where Ic indicates the proportion of a society that needs to be protected from infection to achieve herd immunity. Next, the critical vaccination coverage (Vc) that is needed to provide herd immunity was determined by including the vaccine effectiveness (E) using Eq. 2 (18–22).
Results
Basic Reproductive Number (R0) Values for Ebolaviruses
The PubMed search for “Ebola R0” provided 18 hits, the search for “Ebola basic reproductive number” provided 42 hits, and the search for “Ebola basic reproduction number” provided 35 hits (Figure 1; Data Sheet S1 in Supplementary Material). After removal of the overlaps and inclusion of an additional article [identified from the reference list of Ref. (21)], this resulted in 51 articles, 35 of which provided relevant information on Ebolavirus R0 values (Figure 1; Data Sheet S1 in Supplementary Material).
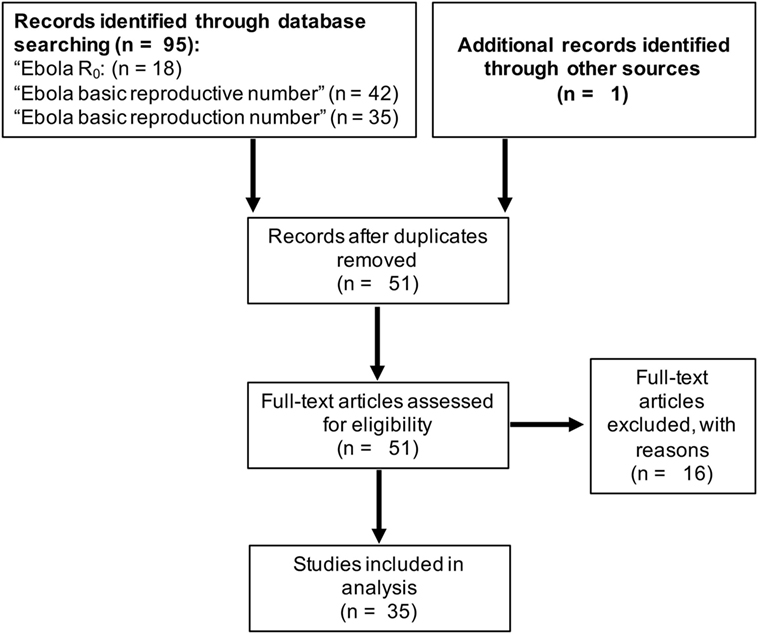
Figure 1. Summary of the literature search using PubMed (www.ncbi.nlm.nih.gov/pubmed) to identify articles that report on the basic reproductive number (R0) of Ebolaviruses.
R0 data were only available for Ebola virus and Sudan virus outbreaks (Data Sheet S1 in Supplementary Material). 29/35 studies analyzed data from the recent West African Ebola virus outbreak (Data Sheet S1 in Supplementary Material). The others reported on Ebola virus outbreaks in the Democratic Republic of Congo. Four studies also included data from the Sudan virus outbreak 2000/2001 in Gulu, Uganda. We also considered a review that summarized all available data until February 2015 (5) (Data Sheet S1 in Supplementary Material).
R0 indicates the number of new infections caused by an infected individual, and when greater than 1, an outbreak will spread. Different approaches to calculate R0s lead to varying results (22). Accordantly, R0 values calculated for the Sudan virus outbreak 2000/2001 in Gulu using identical data ranged from 1.34 to 3.54 (Data Sheets S1 and S2 in Supplementary Material). Small outbreak sizes may also limit the accuracy of the calculated R0 values. Additionally, virus transmission is influenced by socioeconomic and behavioral factors including the health-care response, society perceptions, religious practices, population density, and/or infrastructure (22, 23). Concordantly, R0s that were determined by the same methodology in different districts of Guinea, Liberia, and Sierra Leone during the West African Ebola virus epidemic ranged from 0.36 to 3.37 (24). Three studies directly compared the Ebola virus outbreak in Kikwit (1995, DR Congo) and the Sudan virus outbreak in Gulu (2000/2001, Uganda) (25–27), but did not reveal fundamental differences between the R0s of the viruses (Data Sheets S1 and S2 in Supplementary Material). Across all relevant studies, R0s ranged from 0.36 to 12 for Ebola virus and from 1.34 to 3.54 for Sudan virus (Data Sheet S1 in Supplementary Material). 9 of the 35 studies that provided R0 values showed that Ebola viruses can spread with an R0 > 3, and five studies suggested that Ebolaviruses can spread with R0 values > 4. High reproductive numbers (≥4) are typically observed at the beginning of Ebolavirus outbreaks, prior to the implementation of control measures (28–31). Also, the spread of Ebolaviruses may be substantially driven by “superspreaders” who infect a high number (up to 15–20) of individuals (23, 32–35). Studies from the West African Ebola virus outbreak suggested that relatively small numbers of superspreaders may have been responsible for the majority of cases (35, 36). Since the available data suggest that Ebolavirus transmission can occur with R0 values of 3, 4, or even higher, a prophylactic vaccination program should establish herd immunity against Ebolaviruses that spread at such levels.
Herd Immunity Threshold (Ic)
At an R0 of 3, the Ic (Eq. 1) is 67%, which means that 67% of a population need to be immune to provide herd immunity (Figure 2A; Data Sheet S3 in Supplementary Material). The Ic further rises to 75% at an R0 of 4, to 80% at an R0 of 5, to 90% at an R0 of 10, and to 95% R0 of 20 (Figure 2A; Data Sheet S3 in Supplementary Material). This shows that high proportions of a population need to be immune to establish effective herd immunity.
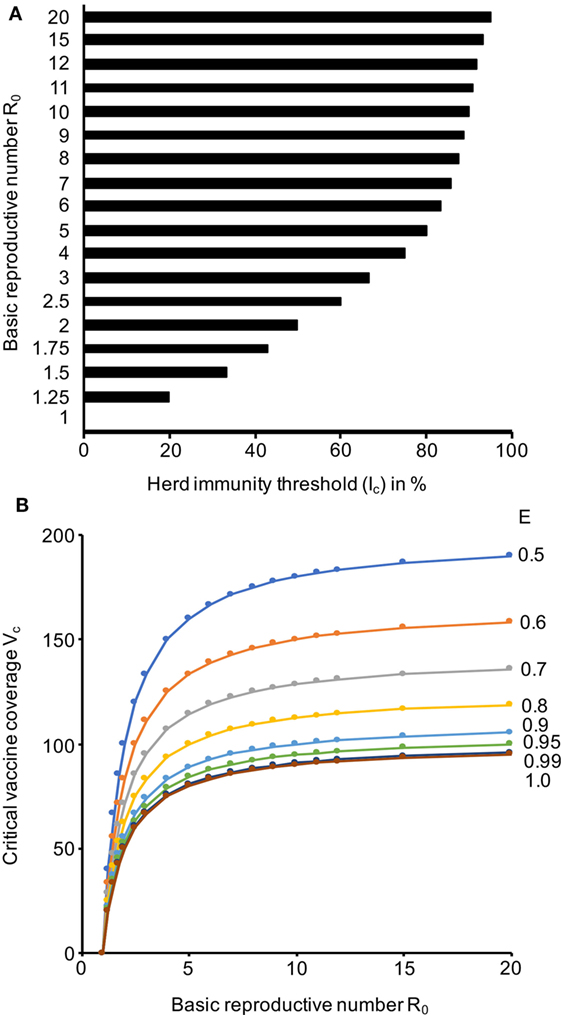
Figure 2. Herd immunity thresholds (Ic) and critical vaccine coverage (Vc) values in dependence of the basic reproductive number (R0) and the vaccine efficacy (E). (A) Ic values based on a range of R0 values that cover the range reported for Ebola viruses. (B) Vc values based on R0 values that cover the range reported for Ebola viruses and E values that are in the range of those reported for approved vaccines. The respective numerical data are presented in Data Sheet S3 in Supplementary Material.
Critical Vaccine Coverage (Vc)
As there is currently no approved vaccine for the prevention of Ebolavirus disease, we calculated a range of Vc (Eq. 2) scenarios that reflect the efficacy range covered by approved vaccines. Attenuated replication-competent measles virus vaccines have been reported to protect up to 95% of individuals from disease after one dose, which increased to up to 99% after a second dose (37). The efficacy of varicella zoster virus vaccines, another attenuated replication-competent vaccine, was recently calculated to be 81.9% after one dose and 94.4% after two doses (38). Inactivated seasonal influenza virus split vaccines have been reported to have a substantially lower efficiency of 50–60% (39–41). Hence, we considered a Vc range between 50 and 100% (Figure 2B; Data Sheet S3 in Supplementary Material). Vaccines, which provide high protection (ideally after a single vaccination), and high vaccination rates are required for prophylactic vaccination programs that establish a level of herd immunity that prevents Ebolavirus outbreaks. If we assume an R0 of 3 and a vaccination efficacy E of 90%, more than 70% of a population need to be vaccinated to establish herd immunity. At an R0 of 4 and a vaccination efficacy E of 90%, more than 80% of a population need to be vaccinated. If the R0 rises to 5, a vaccine coverage of 80% would be required, even if a vaccine with 100% efficacy was available (Figure 2B; Data Sheet S3 in Supplementary Material).
Discussion
We performed an analysis of the Ebolavirus vaccine requirements to achieve the Vc needed for prophylactic mass vaccination programs. A number of studies suggested that Ebolavirus transmission can occur with R0 values of 3, 4, or even higher, in particular during early outbreak stages (prior to the implementation of control measures) and/or as consequence of superspreading events (23, 24, 28–36). Therefore, a prophylactic vaccination program should establish herd immunity against Ebolaviruses that spread at such levels. At an R0 of 3, >70% of individuals and at an R0 of 4, >80% of individuals need to be vaccinated with a vaccination efficacy of 90% to achieve herd immunity. Hence, highly effective vaccines and a high vaccination coverage are essential for successful prophylactic mass vaccination programs against Ebolaviruses.
Clinical vaccine candidates providing protection against all three to four human-pathogenic Ebolaviruses (Ebola virus, Sudan virus, Bundibugyo virus, potentially Taï Forest virus) do not currently exist (Data Sheet S4 in Supplementary Material), although preclinical data suggest that the development of such vaccines may be feasible (6). Current vaccine candidates may also not provide the long-term protective immunity (≥10 years) necessary for sustainable protection against spillover events from animal reservoirs. Two studies reported immune responses 12 months after vaccination with different Ebola virus vaccine candidates (42, 43). One of them described seroconversion in >90% of individuals after a single injection of rVSV-ZEBOV, a vesicular stomatitis virus-based Ebola virus vaccine. No or only a minor drop in antibody titers and neutralization capacity was reported 360 days after vaccination (42). A study investigating rVSV-ZEBOV and ChAd3-EBO-Z, a chimpanzee adenovirus type-3 vector-based Ebola virus vaccine, found lower seroconversion rates (rVSV-ZEBOV: 83.7%; ChAd3-EBO-Z: 70.8%) and reported the highest antibody response after 1 month and a decline afterward (43). Thus, it is not clear, whether the vaccine-induced immunity covers the time frame of 2 years (or perhaps even longer) that Ebolavirus survivors may remain contagious for (6, 42–52). It is also not clear whether (and if yes, to which extent) immunity to Ebolaviruses is mediated by cell-mediated and/or humoral immune responses (53). A challenge study using non-human primates suggested that protection by adenovirus-based vaccines is cell mediated (54). This means that antigen binding and/or neutralization titers may not always correlate with protection from disease. Consequently, the efficacy levels of vaccines cannot be determined with certainty based on antibody responses at various time points post vaccination. Thus, it remains unknown whether current vaccine candidates offer the long-term protection necessary for mass vaccination programs that effectively prevent zoonotic Ebolavirus outbreaks. Ebola virus recurrences and reinfections indicate that, although natural Ebolavirus infections are generally assumed to provide long-term protection, natural infections may not always result in sustained protective immunity in every survivor, which may further complicate the development of vaccines that provide long-term protection (55, 56). In this context, the establishment of long-term immunity may be influenced by the disease treatment. In a case of relapse 9 months after discharge, it was speculated whether the treatment of the initial disease with convalescent plasma and monoclonal antibodies might have contributed to the recurrence (55).
Limited acceptance of vaccinations may also limit Ebolavirus vaccination programs. In a rVSV-ZEBOV ring vaccination trial, only 5,837/11,841 patient contacts could be vaccinated. 34% of the contacts refused the vaccination (57). In a survey in Sierra Leone during the West African Ebola epidemic, 106/400 respondents (26.6%) were prepared to pay for a vaccination, while 290 respondents (72.5%) would have accepted a free vaccination (58). Since 74% of the population need to be vaccinated by a vaccine with a 90% efficacy to prevent an outbreak that spreads with an R0 of 3 and 83% of the population to prevent an outbreak that spreads with an R0 of 4 (Data Sheet S3 in Supplementary Material), such levels of vaccine coverage seem currently unachievable, even under the threat of an ongoing epidemic, although attitudes may change in the future if more (clinical) data becomes available. Therefore, more differentiated vaccination strategies with a focus on health-care workers and patient contacts appear more feasible.
The median maximum fee that survey participants in Sierra Leone during the West African Ebola epidemic were prepared to pay for a vaccine was about 5,000 leones ($0.65 as of 11th January 2018) (58). The international organization GAVI (www.gavi.org) is providing $5 million for the development of rVSV-ZEBOV, which is expected to pay for 300,000 vaccine doses (about $16.70/dose) (59). Within a rVSV-ZEBOV ring vaccination trial, 11,841 contacts requiring vaccination from 117 clusters were identified over a 10-month period, i.e., about 101 individuals per confirmed Ebola virus disease patient (57). Hence, 300,000 doses will enable vaccination of the contacts of approximately 2,970 Ebola virus disease patients. If an effective vaccine (which provided protection against all human-pathogenic Ebolaviruses) was available, a vaccination program would comprise about 462 million individuals in the countries that have been affected by Ebolavirus outbreaks (Data Sheet S5 in Supplementary Material). Notably, the countries, which have been affected by Ebolavirus outbreaks so far, have large rural populations ranging from 13% (Gabon) to 84% (Uganda) (Data Sheet S5 in Supplementary Material). Vaccination programs in rural areas are associated with logistical issues including transport difficulties, lack of equipment and trained medical specialists, and cultural and language barriers (60, 61).
In conclusion, the achievement of a Vc of 75% that is necessary to prevent an outbreak that spreads with an R0 of 4 with a vaccine that has an efficacy of 100% is currently unrealistic because of limited vaccine acceptance in the affected populations and because of financial and logistical challenges. In addition, concurrent diseases such as HIV and cancer, along with potential side effects of vaccination, may remove significant numbers of potential vaccines (6, 62). Alternative vaccination strategies will be required for such patients. Replication-deficient vaccines such as DNA vaccines, virus-like particles, nanoparticle-based vaccines, and viral vectors (e.g., Modified Vaccinia Ankara, which was already demonstrated to be safe in immunocompromised individuals) may be safer alternatives (6, 63). Moreover, vaccines that provide long-term immunity against all three (or including Taï Forest virus, four) human-pathogenic Ebolaviruses, which would be needed to protect populations effectively from large Ebolavirus outbreaks in endemic areas, do not exist. Therefore, outbreak control of Ebolaviruses will for the foreseeable future depend on surveillance and the isolation of cases. Clinical vaccine candidates are only available for Ebola viruses and will need to be focused on health-care workers, who are often involved in disease transmission (30), potentially in combination with the vaccination of patient contacts. Hence, our findings support the conclusions of the WHO Strategic Advisory Group of Experts on immunization (SAGE) at the WHO SAGE meeting on 25th to 27th April 2017 (64). SAGE acknowledged the need for further research on Ebolavirus vaccines, including the generation of conclusive data on the duration of protection provided by Ebolavirus vaccine candidates. In case of future Ebolavirus outbreaks, SAGE recommended the use of rVSV-ZEBOV ring vaccination strategies (64).
Author Contributions
SM performed the calculations. MM performed the literature search. All authors analyzed the data. SM, MM, and MW wrote the manuscript. All authors gave their final approval of the version to be published.
Conflict of Interest Statement
The authors declare that the research was conducted in the absence of any commercial or financial relationships that could be construed as a potential conflict of interest.
Supplementary Material
The Supplementary Material for this article can be found online at https://www.frontiersin.org/articles/10.3389/fimmu.2018.01025/full#supplementary-material.
References
1. Kuhn JH, Becker S, Ebihara H, Geisbert TW, Johnson KM, Kawaoka Y, et al. Proposal for a revised taxonomy of the family Filoviridae: classification, names of taxa and viruses, and virus abbreviations. Arch Virol (2010) 155:2083–103. doi:10.1007/s00705-010-0814-x
2. Michaelis M, Rossman JS, Wass MN. Computational analysis of Ebolavirus data: prospects, promises and challenges. Biochem Soc Trans (2016) 44:973–8. doi:10.1042/BST20160074
3. Pappalardo M, Juliá M, Howard MJ, Rossman JS, Michaelis M, Wass MN. Conserved differences in protein sequence determine the human pathogenicity of Ebolaviruses. Sci Rep (2016) 6:23743. doi:10.1038/srep23743
4. Pappalardo M, Reddin IG, Cantoni D, Rossman JS, Michaelis M, Wass MN. Changes associated with Ebola virus adaptation to novel species. Bioinformatics (2017) 33:1911–5. doi:10.1093/bioinformatics/btx065
5. Van Kerkhove MD, Bento AI, Mills HL, Ferguson NM, Donnelly CA. A review of epidemiological parameters from Ebola outbreaks to inform early public health decision-making. Sci Data (2015) 2:150019. doi:10.1038/sdata.2015.19
6. Keshwara R, Johnson RF, Schnell MJ. Toward an effective Ebola virus vaccine. Annu Rev Med (2017) 68:371–86. doi:10.1146/annurev-med-051215-030919
7. Rojek A, Horby P, Dunning J. Insights from clinical research completed during the West Africa Ebola virus disease epidemic. Lancet Infect Dis (2017) 17:e280–92. doi:10.1016/S1473-3099(17)30234-7
8. Le Guenno B, Formenty P, Wyers M, Gounon P, Walker F, Boesch C. Isolation and partial characterisation of a new strain of Ebola virus. Lancet (1995) 345:1271–4. doi:10.1016/S0140-6736(95)90925-7
9. Akinfeyeva LA, Aksyonova OI, Vasilyevich IV, Ginko ZI, Zarkov KA, Zubavichene NM, et al. A case of Ebola hemorrhagic fever. Infektsionnye Bolezni (Moscow) (2005) 3:85–8.
10. Borisevich IV, Markin VA, Firsova IV, Evseev AA, Khamitov RA, Maksimov VA. Hemorrhagic (Marburg, Ebola, Lassa, and Bolivian) fevers: epidemiology, clinical pictures, and treatment. Vopr Virusol (2006) 51:8–16.
11. Cantoni D, Hamlet A, Michaelis M, Wass MN, Rossman JS. Risks posed by reston, the forgotten Ebolavirus. mSphere (2016) 1:e322–316. doi:10.1128/mSphere.00322-16
12. Timen A, Koopmans MP, Vossen AC, van Doornum GJ, Günther S, van den Berkmortel F, et al. Response to imported case of Marburg hemorrhagic fever, the Netherland. Emerg Infect Dis (2009) 15:1171–5. doi:10.3201/eid1508.090015
13. Rougeron V, Feldmann H, Grard G, Becker S, Leroy EM. Ebola and Marburg haemorrhagic fever. J Clin Virol (2015) 64:111–9. doi:10.1016/j.jcv.2015.01.014
14. Nikiforov VV, Turovskiĭ IuI, Kalinin PP, Akinfeeva LA, Katkova LR, Barmin VS, et al. A case of a laboratory infection with Marburg fever. Zh Mikrobiol Epidemiol Immunobiol (1994) 3:104–6.
15. Beer B, Kurth R, Bukreyev A. Characteristics of Filoviridae: Marburg and Ebola viruses. Naturwissenschaften (1999) 86:8–17. doi:10.1007/s001140050562
16. Bonanni P, Sacco C, Donato R, Capei R. Lifelong vaccination as a key disease-prevention strategy. Clin Microbiol Infect (2014) 20(Suppl 5):32–6. doi:10.1111/1469-0691.12537
17. Judson SD, Fischer R, Judson A, Munster VJ. Ecological contexts of index cases and spillover events of different Ebolaviruses. PLoS Pathog (2016) 12:e1005780. doi:10.1371/journal.ppat.1005780
18. Anderson RM. The concept of herd immunity and the design of community based immunization programs. Vaccine (1992) 10:928–35. doi:10.1016/0264-410X(92)90327-G
19. Fine P, Eames K, Heymann DL. “Herd immunity”: a rough guide. Clin Infect Dis (2011) 52:911–6. doi:10.1093/cid/cir007
20. Plans-Rubió P. The vaccination coverage required to establish herd immunity against influenza viruses. Prev Med (2012) 55:72–7. doi:10.1016/j.ypmed.2012.02.015
21. Gittings K, Matson KL. Establishing herd immunity against Ebola through vaccination. Vaccine (2016) 34:2644–7. doi:10.1016/j.vaccine.2016.04.047
22. Guerra FM, Bolotin S, Lim G, Heffernan J, Deeks SL, Li Y, et al. The basic reproduction number (R0) of measles: a systematic review. Lancet Infect Dis (2017) 17:e420–8. doi:10.1016/S1473-3099(17)30307-9
23. Skrip LA, Fallah MP, Gaffney SG, Yaari R, Yamin D, Huppert A, et al. Characterizing risk of Ebola transmission based on frequency and type of case-contact exposures. Philos Trans R Soc Lond B Biol Sci (2017) 372(1721): 20160301. doi:10.1098/rstb.2016.0301
24. Krauer F, Gsteiger S, Low N, Hansen CH, Althaus CL. Heterogeneity in district-level transmission of Ebola virus disease during the 2013–2015 epidemic in West Africa. PLoS Negl Trop Dis (2016) 10:e0004867. doi:10.1371/journal.pntd.0004867
25. Chowell G, Hengartner NW, Castillo-Chavez C, Fenimore PW, Hyman JM. The basic reproductive number of Ebola and the effects of public health measures: the cases of Congo and Uganda. J Theor Biol (2004) 229:119–26. doi:10.1016/j.jtbi.2004.03.006
26. Legrand J, Grais RF, Boelle PY, Valleron AJ, Flahault A. Understanding the dynamics of Ebola epidemics. Epidemiol Infect (2007) 135:610–21. doi:10.1017/S0950268806007217
27. Chen T, Ka-Kit Leung R, Liu R, Chen F, Zhang X, Zhao J, et al. Risk of imported Ebola virus disease in China. Travel Med Infect Dis (2014) 12:650–8. doi:10.1016/j.tmaid.2014.10.015
28. Althaus CL. Rapid drop in the reproduction number during the Ebola outbreak in the Democratic Republic of Congo. PeerJ (2015) 3:e1418. doi:10.7717/peerj.1418
29. Althaus CL, Low N, Musa EO, Shuaib F, Gsteiger S. Ebola virus disease outbreak in Nigeria: transmission dynamics and rapid control. Epidemics (2015) 11:80–4. doi:10.1016/j.epidem.2015.03.001
30. Rosello A, Mossoko M, Flasche S, Van Hoek AJ, Mbala P, Camacho A, et al. Ebola virus disease in the Democratic Republic of the Congo, 1976–2014. Elife (2015) 4:e09015. doi:10.7554/eLife.09015
31. Kucharski AJ, Eggo RM, Watson CH, Camacho A, Funk S, Edmunds WJ. Effectiveness of ring vaccination as control strategy for Ebola virus disease. Emerg Infect Dis (2016) 22:105–8. doi:10.3201/eid2201.151410
32. Volz E, Pond S. Phylodynamic analysis of Ebola virus in the 2014 Sierra Leone epidemic. PLoS Curr (2014) 6. doi:10.1371/currents.outbreaks.6f7025f1271821d4c815385b08f5f80e
33. Osterholm MT, Moore KA, Kelley NS, Brosseau LM, Wong G, Murphy FA, et al. Transmission of Ebola viruses: what we know and what we do not know. MBio (2015) 6:e00137. doi:10.1128/mBio.00137-15
34. Althaus CL. Ebola superspreading. Lancet Infect Dis (2015) 15:507–8. doi:10.1016/S1473-3099(15)70135-0
35. Lau MS, Dalziel BD, Funk S, McClelland A, Tiffany A, Riley S, et al. Spatial and temporal dynamics of superspreading events in the 2014–2015 West Africa Ebola epidemic. Proc Natl Acad Sci U S A (2017) 114:2337–42. doi:10.1073/pnas.1614595114
36. International Ebola Response Team, Agua-Agum J, Ariyarajah A, Aylward B, Bawo L, Bilivogui P, et al. Exposure patterns driving Ebola transmission in West Africa: a retrospective observational study. PLoS Med (2016) 13:e1002170. doi:10.1371/journal.pmed.1002170
37. Holzmann H, Hengel H, Tenbusch M, Doerr HW. Eradication of measles: remaining challenges. Med Microbiol Immunol (2016) 205:201–8. doi:10.1007/s00430-016-0451-4
38. Rieck T, Feig M, An der Heiden M, Siedler A, Wichmann O. Assessing varicella vaccine effectiveness and its influencing factors using health insurance claims data, Germany, 2006 to 2015. Euro Surveill (2017) 22:30521. doi:10.2807/1560-7917.ES.2017.22.17.30521
39. Beyer WE, Nauta JJ, Palache AM, Giezeman KM, Osterhaus AD. Immunogenicity and safety of inactivated influenza vaccines in primed populations: a systematic literature review and meta-analysis. Vaccine (2011) 29:5785–92. doi:10.1016/j.vaccine.2011.05.040
40. Osterholm MT, Kelley NS, Sommer A, Belongia EA. Efficacy and effectiveness of influenza vaccines: a systematic review and meta-analysis. Lancet Infect Dis (2012) 12:36–44. doi:10.1016/S1473-3099(11)70295-X
41. Tricco AC, Chit A, Soobiah C, Hallett D, Meier G, Chen MH, et al. Comparing influenza vaccine efficacy against mismatched and matched strains: a systematic review and meta-analysis. BMC Med (2013) 11:153. doi:10.1186/1741-7015-11-153
42. Heppner DG Jr., Kemp TL, Martin BK, Ramsey WJ, Nichols R, Dasen EJ, et al. Safety and immunogenicity of the rVSVΔG-ZEBOV-GP Ebola virus vaccine candidate in healthy adults: a phase 1b randomised, multicentre, double-blind, placebo-controlled, dose-response study. Lancet Infect Dis (2017) 17:854–66. doi:10.1016/S1473-3099(17)30313-4
43. Kennedy SB, Bolay F, Kieh M, Grandits G, Badio M, Ballou R, et al. Phase 2 placebo-controlled trial of two vaccines to prevent Ebola in liberia. N Engl J Med (2017) 377:1438–47. doi:10.1056/NEJMoa1614067
44. Diallo B, Sissoko D, Loman NJ, Bah HA, Bah H, Worrell MC, et al. Resurgence of Ebola virus disease in guinea linked to a survivor with virus persistence in seminal fluid for more than 500 days. Clin Infect Dis (2016) 63:1353–6. doi:10.1093/cid/ciw601
45. Sissoko D, Duraffour S, Kerber R, Kolie JS, Beavogui AH, Camara AM, et al. Persistence and clearance of Ebola virus RNA from seminal fluid of Ebola virus disease survivors: a longitudinal analysis and modelling study. Lancet Glob Health (2017) 5:e80–8. doi:10.1016/S2214-109X(16)30243-1
46. Li JX, Hou LH, Meng FY, Wu SP, Hu YM, Liang Q, et al. Immunity duration of a recombinant adenovirus type-5 vector-based Ebola vaccine and a homologous prime-boost immunisation in healthy adults in China: final report of a randomised, double-blind, placebo-controlled, phase 1 trial. Lancet Glob Health (2017) 5:e324–34. doi:10.1016/S2214-109X(16)30367-9
47. Winslow RL, Milligan ID, Voysey M, Luhn K, Shukarev G, Douoguih M, et al. Immune responses to novel adenovirus type 26 and modified Vaccinia virus Ankara-vectored Ebola vaccines at 1 year. JAMA (2017) 317:1075–7. doi:10.1001/jama.2016.20644
48. Zhu FC, Wurie AH, Hou LH, Liang Q, Li YH, Russell JB, et al. Safety and immunogenicity of a recombinant adenovirus type-5 vector-based Ebola vaccine in healthy adults in Sierra Leone: a single-centre, randomised, double-blind, placebo-controlled, phase 2 trial. Lancet (2017) 389:621–8. doi:10.1016/S0140-6736(16)32617-4
49. Soka MJ, Choi MJ, Baller A, White S, Rogers E, Purpura LJ, et al. Prevention of sexual transmission of Ebola in Liberia through a national semen testing and counselling programme for survivors: an analysis of Ebola virus RNA results and behavioural data. Lancet Glob Health (2016) 4:e736–43. doi:10.1016/S2214-109X(16)30175-9
50. Uyeki TM, Erickson BR, Brown S, McElroy AK, Cannon D, Gibbons A, et al. Ebola virus persistence in semen of male survivors. Clin Infect Dis (2016) 62:1552–5. doi:10.1093/cid/ciw202
51. Barnes KG, Kindrachuk J, Lin AE, Wohl S, Qu J, Tostenson SD, et al. Evidence of Ebola virus replication and high concentration in semen of a patient during recovery. Clin Infect Dis (2017) 65:1400–3. doi:10.1093/cid/cix518
52. Deen GF, Broutet N, Xu W, Knust B, Sesay FR, McDonald SLR, et al. Ebola RNA persistence in semen of Ebola virus disease survivors – final report. N Engl J Med (2017) 377:1428–37. doi:10.1056/NEJMoa1511410
53. Lambe T, Bowyer G, Ewer KJ. A review of phase I trials of Ebola virus vaccines: what can we learn from the race to develop novel vaccines? Philos Trans R Soc Lond B Biol Sci (2017) 372(1721):20160295. doi:10.1098/rstb.2016.0295
54. Stanley DA, Honko AN, Asiedu C, Trefry JC, Lau-Kilby AW, Johnson JC, et al. Chimpanzee adenovirus vaccine generates acute and durable protective immunity against Ebola virus challenge. Nat Med (2014) 20:1126–9. doi:10.1038/nm.3702
55. Jacobs M, Rodger A, Bell DJ, Bhagani S, Cropley I, Filipe A, et al. Late Ebola virus relapse causing meningoencephalitis: a case report. Lancet (2016) 388:498–503. doi:10.1016/S0140-6736(16)30386-5
56. MacIntyre CR, Chughtai AA. Recurrence and reinfection – a new paradigm for the management of Ebola virus disease. Int J Infect Dis (2016) 43:58–61. doi:10.1016/j.ijid.2015.12.011
57. Henao-Restrepo AM, Camacho A, Longini IM, Watson CH, Edmunds WJ, Egger M, et al. Efficacy and effectiveness of an rVSV-vectored vaccine in preventing Ebola virus disease: final results from the Guinea ring vaccination, open-label, cluster-randomised trial (Ebola Ça Suffit!). Lancet (2017) 389:505–18. doi:10.1016/S0140-6736(16)32621-6
58. Huo X, Shi G, Li X, Lai X, Deng L, Xu F, et al. Knowledge and attitudes about Ebola vaccine among the general population in Sierra Leone. Vaccine (2016) 34:1767–72. doi:10.1016/j.vaccine.2016.02.046
59. Henao-Restrepo AM, Preziosi MP, Wood D, Moorthy V, Kieny MP; WHO Ebola Research, et al. On a path to accelerate access to Ebola vaccines: the WHO’s research and development efforts during the 2014–2016 Ebola epidemic in West Africa. Curr Opin Virol (2016) 17:138–44. doi:10.1016/j.coviro.2016.03.008
60. Alexander KA, Sanderson CE, Marathe M, Lewis BL, Rivers CM, Shaman J, et al. What factors might have led to the emergence of Ebola in West Africa? PLoS Negl Trop Dis (2015) 9:e0003652. doi:10.1371/journal.pntd.0003652
61. Moon S, Sridhar D, Pate MA, Jha AK, Clinton C, Delaunay S, et al. Will Ebola change the game? Ten essential reforms before the next pandemic. The report of the Harvard-LSHTM Independent Panel on the Global Response to Ebola. Lancet (2015) 386:2204–21. doi:10.1016/S0140-6736(15)00946-0
62. Kagina BM, Wiysonge CS, Lesosky M, Madhi SA, Hussey GD. Safety of licensed vaccines in HIV-infected persons: a systematic review protocol. Syst Rev (2014) 3:101. doi:10.1186/2046-4053-3-101
63. Volz A, Sutter G. Modified Vaccinia virus Ankara: history, value in basic research, and current perspectives for vaccine development. Adv Virus Res (2017) 97:187–243. doi:10.1016/bs.aivir.2016.07.001
Keywords: ebola virus, Ebolavirus, vaccines, herd immunity, basic reproduction number
Citation: Masterson SG, Lobel L, Carroll MW, Wass MN and Michaelis M (2018) Herd Immunity to Ebolaviruses Is Not a Realistic Target for Current Vaccination Strategies. Front. Immunol. 9:1025. doi: 10.3389/fimmu.2018.01025
Received: 12 January 2018; Accepted: 24 April 2018;
Published: 09 May 2018
Edited by:
Lee Mark Wetzler, Boston University, United StatesReviewed by:
Chad Edward Mire, University of Texas Medical Branch, United StatesAxel T. Lehrer, University of Hawaii at Manoa, United States
Copyright: © 2018 Masterson, Lobel, Carroll, Wass and Michaelis. This is an open-access article distributed under the terms of the Creative Commons Attribution License (CC BY). The use, distribution or reproduction in other forums is permitted, provided the original author(s) and the copyright owner are credited and that the original publication in this journal is cited, in accordance with accepted academic practice. No use, distribution or reproduction is permitted which does not comply with these terms.
*Correspondence: Mark N. Wass, bS5uLndhc3MmI3gwMDA0MDtrZW50LmFjLnVr;
Martin Michaelis, bS5taWNoYWVsaXMmI3gwMDA0MDtrZW50LmFjLnVr