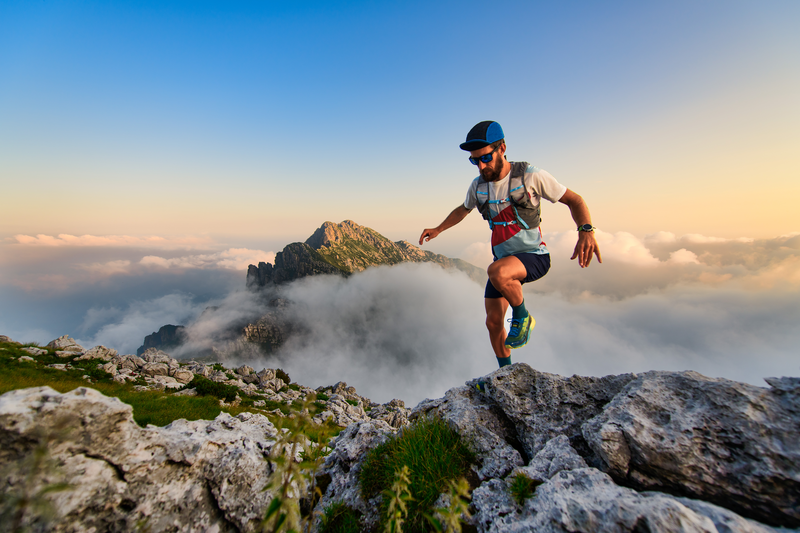
94% of researchers rate our articles as excellent or good
Learn more about the work of our research integrity team to safeguard the quality of each article we publish.
Find out more
ORIGINAL RESEARCH article
Front. Immunol. , 10 April 2018
Sec. Alloimmunity and Transplantation
Volume 9 - 2018 | https://doi.org/10.3389/fimmu.2018.00760
Virus-specific T-cell responses are crucial to control cytomegalovirus (CMV) infections/reactivation in immunocompromised individuals. Adoptive cellular therapy with CMV-specific T-cells has become a viable treatment option. High-affinity anti-viral cellular immune responses are associated with improved long-term immune protection against CMV infection. To date, the characterization of high-affinity T-cell responses against CMV has not been achieved in blood from patients after allogeneic hematopoietic stem cell transplantation (HSCT). Therefore, the purpose of this study was to describe and analyze the phenotype and clinical impact of different CMV-specific CD8+ cytotoxic T-lymphocytes (CMV-CTL) classes based on their T-cell receptor (TCR) affinity. T-cells isolated from 23 patients during the first year following HSCT were tested for the expression of memory markers, programmed cell death 1 (PD-1), as well as TCR affinity, using three different HLA-A*02:01 CMVNLVPMVATV-Pp65 tetramers (wild-type, a245v and q226a mutants). High-affinity CMV-CTL defined by q226a tetramer binding, exhibited a higher frequency in CD8+ T-cells in the first month post-HSCT and exhibited an effector memory phenotype associated with strong PD-1 expression as compared to the medium- and low-affinity CMV-CTLs. High-affinity CMV-CTL was found at higher proportion in patients with chronic graft-versus-host disease (p < 0.001). This study provides a first insight into the detailed TCR affinities of CMV-CTL. This may be useful in order to improve current immunotherapy protocols using isolation of viral-specific T-cell populations based on their TCR affinity.
Cytomegalovirus (CMV) is usually present in a latent state in a majority of the human population (1, 2). Active CMV disease and reactivation are common in immunocompromised individuals, e.g., in patients with human immunodeficiency virus (HIV) infection, solid organ transplant recipients, as well as in individuals who undergo hematopoietic stem cell transplantation (HSCT) depending on the CMV-serological status of the donor and recipient (2ā4). CMV mortality post-HSCT has greatly decreased over time although, CMV disease still remains a severe and life-threatening complication with an incidence around 5% (5). CMV reactivation and disease not only have a direct impact on the outcome of the transplanted individuals, but is also known to cause severe indirect effects, such as increased risk of bacterial and fungal infections (6) and increased incidence of graft-versus-host disease (GVHD) (7, 8). In contrast, positive effects of CMV infection/reactivation have also been reported, due to a promotion of graft-versus-leukemia effects by early CMV antigenemia ensuing immune responses, such as the cross-reactivity of γΓ T-cells (9ā12). Nonetheless, this relation remains controversial, since several studies did not observe a decreased risk of relapse concomitant with CMV reactivation (13ā15).
The standard treatment for CMV management post-HSCT is supported by prophylaxis, pre-emptive regimen, and therapy using acyclovir, valganciclovir, and ganciclovir (16). As an alternative to antiviral agents, adoptive T-cell therapy (ACT) is being used as a promising treatment of infections to overcome the immunodeficiency state of patients post-HSCT. Generation and selection of CMV-specific CD8+ cytotoxic T-lymphocytes (CMV-CTL) by ex vivo T-cells expansion, magnetic beads, HLA multimers, and IFN-γ capture have proven their efficiency by overcoming the lack of T-cell immunity and providing long-term protective immune response (17ā23). Optimization of T-cell products for ACT has been made possible by the better understanding and characterization of the mechanism and biology of immune-protection and long-lasting cellular immune responses against transformed cells and pathogens, such as CMV (24, 25). The cell number, frequency of antigen-specific T-cells, antigen-specific immune functions, as well as the maturation and differentiation status of transferred T-cells, have proven to be vital for protective immune effector functions (26ā28).
Despite high efficacy in diagnostic techniques, antiviral treatments and ACT, there is still room for improving the CMV management in patients post-HSCT. To date, the T-cell receptor (TCR) affinity of CMV-CTL using tetramers has not been analyzed in patients post-HSCT. In this report, we aimed at characterizing the HLA-A*02:01-restricted CMV-CTL repertoire in peripheral blood from HSCT recipients at various time points after transplantation based on immune reactivity to the immunodominant tegument protein CMV-pp65 (29) using three MHC class I-CMVNLVPMVATV peptide tetramers targeting TCRs of different affinities. We further correlate CMV-CTL frequencies with clinical events, such as CMV reactivation and GVHD post-HSCT, which may be helpful in predicting ACT outcome as well as refining cell products.
Twenty-three patients were recruited for T-cell analysis after HLA-matched HSCT, the treatment was performed at CAST, Karolinska University Hospital, Sweden (Table 1). This study was part of a larger study that prospectively recruited 262 patients post-HSCT with blood samples collected before HSCT and at 1, 2, 3, 6, 12, and 24 months post-HSCT at CAST from 2007 to 2016. IRB approval (Stockholm Ethical Committee South 2010/760-31/1) was in place and consent was obtained from each patient. Adult patients for this study were selected based on HLA-A*02:01 positive, no anti-thymocyte globulin (ATG) treatment and availability of more than four out of seven samples. Quality control based on cell count and viability excluded 11 samples. The study, therefore, included 81 samples with 12ā17 samples per time points. Most of the patients received peripheral blood stem cells from siblings after a reduced intensity conditioning (RIC) regimen and chemotherapy (Table 1). Neutrophil engraftment defined by an absolute count >0.5 Ć 109/L for three consecutive days was reached at a median of 18 days (min. 13, max. 25). Grading of GVHD was evaluated using established criteria (30). Patients with GVHD received ā„1 mg/kg/day prednisone equivalents of corticosteroids during the study as recently described (31). CMV DNAemia was routinely monitored and quantified post-HSCT by real-time PCR on whole blood (32). Patients (n = 3) who had a viral CMV DNAemia of more than 2,000 copies/ml were treated as previously described (33) with intravenous ganciclovir (n = 2) or oral valganciclovir (n = 1) between day 111 and 124 post-HSCT.
The anti-CMV pp65 HLA-A2*01:02NLVPMVATV tetramers were constructed in-house. The heavy and light chains of the HLA-A*02:01 allele were cloned into bacterial expression vectors (pET24d+ and pHN1). A245v and q226a mutations were intro-duced into the sequence of the A*02:01 heavy chain using a site-directed mutagenesis kit (Stratagene, La Jolla, CA, USA) (34, 35). The MHC molecules were produced as previously described (36, 37). Briefly, heavy and light chains were produced in Escherichia coli Bl21 DE3 pLys (Invitrogen, Carlsbad, CA, USA) as inclusion bodies. They were then solubilized in an 8 M urea buffer, pH 6.5. The heavy and light chains were purified, solubilized, and folded to correct trimeric structure in 100 mM Tris-400 mM arginine-5 mM EDTA buffer, pH 8.0 together with a peptide derived from the CMV-pp65 protein (NLVPMVATV) (Peptides&Elephants GmbH, Postdam, Germany). The correctly folded MHC monomers were biotinylated and affinity-purified. Unfolded proteins that do not form MHC monomers were precipitated and were filtered away or excluded via the affinity purification step. Monomeric MHC class I-peptide complexes were then tetramerized and fluorescently labeled with streptavidināphycoerythrin (PE, Life technologies, Carlsbad, CA, USA), streptavidināphycoerythrin/Cy7 (PE/Cy7, Biolegend, San Diego, CA, USA) or streptavidināallophycocyanin (APC, Life technologies, Carlsbad, CA, USA).
Peripheral blood mononuclear cells (PBMCs) were isolated over Ficoll-Hypaque gradient (GE Healthcare, Uppsala, Sweden) and frozen at ā190°C in fetal bovine serum (FBS, Life technologies, Carlsbad, CA, USA) and 10% DMSO (38). PBMCs were thawed in RPMI supplemented with 10% FBS (Life Technologies, Carlsbad, CA, USA) and washed twice in PBS-0.1% FBS. One million cells were first incubated for 30 min in dark and at 20°C with a LIVE/DEAD fixable aqua dead cell stain marker (Invitrogen, Carlsbad, CA, USA) according to the manufacturerās instructions. After a single wash with PBS, cells were incubated for 30 min at 37°C with the three different MHC HLA-A2āNLVPMVATV (CMV-pp65) class I tetramers as wild-type (wt) CMV tetramer PE/Cy7, a245v mutant tetramer APC, and q226a mutant tetramer PE. After 30 min cells were washed in PBS-0.1% FBS and then incubated at 4°C for 15 min with the following surface marker antibodies: anti-CD3 brilliant violet 570 (clone UCHT1), anti-CD4 PE/Cy5 (clone RPA-T4), anti-CD8 APC Alexa Fluor 700 (clone SK1), anti-CCR7 brilliant violet (clone G043H7), anti-CD45RA PerCP/Cy5.5 (clone HI100), anti-PD-1 APC/Cy7 (clone EH12-2H7), and anti-IL21R PE-CF594 (clone 17A12). After washing with PBS-0.1% FBS, the cells were acquired on a FACS Aria flow cytometer (BD Biosciences, Stockholm, Sweden) and analyzed using FlowJo software (Treestar Inc., Ashland, OR, USA). Due to the HSCT procedure, the T-cell number was in some instances very low; the tetramer responses and subpopu-lations were, therefore, reported only if we could detect more than 30 absolute events. High-affinity CTLs were defined as tetramer q226a-positive events. Medium-affinity CTLs were defined as tetramer a245v-positive, q226a-negative events. Low-affinity CTLs were defined as tetramer wt-positive a245v- and q226a-negative events. The gating strategy used to define tetramer reactive T-cells is shown in Figures S1A,B in Supplementary Material.
High-affinity HLA-A2*01:02NLVPMVATV sorted CMV-CTL from an HLA-A*02:01 positive healthy individual were seeded in duplicates in 96-well plates at a density of 100,000 cells/well in RPMI medium. Prior to the addition of CMV pp65 (NLVPMVATV) peptide, cells were pre-treated 30 min at 4°C with addition of human CD8 blocking antibody clone DK25 (0.5 µg/ml, EMD Millipore, Billerica, MA, USA) known for its potent anti-CD8 blocking ability as described in Ref. (39, 40). Total fraction of CD8+ T-cells was used for comparison; isotype IgGĪŗ antibody was used as control. After overnight incubation at 37°C, supernatants were harvested and IFN-γ production was measured by ELISA (Mabtech, Stockholm, Sweden) according to the manufacturerās instructions.
To analyze the impact of the different CMV-CTL classes based on TCR affinity (low-, medium-, and high-affinity), frequencies were normalized as a proportion of the total number of CMV-CTL. Briefly, the proportion of CMV-CTL classes was calculated as the following: for each individual sample the addition of low-, medium-, and high-affinity CMV-CTL represented the total number of CMV-CTL (100%). The proportion of low-, medium,- and high-affinity CMV-CTL from that total number of CMV-CTL was reported for each individual sample. Data were analyzed using GraphPad Prism 6 software (La Jolla, CA, USA). Differences were analyzed using Wilcoxon matched-pairs signed rank test or Friedman test to detect differences between two matched timepoints or repeated matched time points after HSCT and MannāWhitney U-test to detect differences between two groups of unpaired samples at single time points. Two-way ANOVA was used to analyze differences between two groups over time. If significant, the Tukeyās test for multiple comparisons was used to detect differences at specific time points. The significance threshold was set at 0.05.
We monitored the immune reconstitution profile of 23 HLA-A*02:01 positive patients over a period of 12 months post-HSCT. Blood samples were obtained at defined intervals after transplantation. The CMV-serological status of the donors (D) and recipients (R) was determined before HSCT. In this cohort, 56.5% of the patients (13/23) belonged to the D+R+ group, 21.7% (5/23) belonged to the DāR+ group, 13.1% (3/23) belonged to the D+Rā group, and 8.7% (2/23) were characterized as DāRā. 11/23 patients, exclusively R+, had CMV reactivation post-HSCT (Table 1). The median time to onset of acute GVHD (aGVHD: 8/23, grade IIāIV) and chronic GVHD (cGVHD: 13/23) was 30 (range 17ā107) and 145 (range 87ā965) days, respectively. 6 out of 23 patients died after HSCT (4 of them during the study time): three due to disease relapse and the other three patients due to HSCT-related complications. Two out of six patients experienced aGVHD, while one patient was diagnosed with a secondary malignancy. One patient received a boost (donor lymphocyte infusion of 10 Ć 106 CD34+ cells/kg at day 106 post-HSCT) (see Table S1 in Supplementary Material for more information). Finally, the chimerism analysis of the CD3+ cells showed a median range of donor chimerism above 99.9% at the two last time points post-HSCT (Figure S1C in Supplementary Material).
The gating strategy used to characterize the CMV-CTL populations in this study is shown in Figure 1A. While we did not observe significant differences in the CD8+ T-cell population reconstitution over the five time points post-HSCT using the Friedman test, a mean increase from 32.5 to 49.6% of CD8+ T-cells and a significant increase between months 1 and 3 as well as 6 (p = 0.006 and p = 0.042, Figure S1D in Supplementary Material) was observed. CD8+ T-cell immunophenotyping based on CD45RA and CCR7 expression revealed a predominant effector memory (TEM: CD45RAā CCR7ā) phenotype with a stable frequency of approximately 50% (Figure S1E in Supplementary Material). The terminally differentiated effector memory (TEMRA, Figure S1F in Supplementary Material) CD8+ T-cell population showed a dynamic change of over time post-HSCT. This subset presented an increase in frequency from 31.6 to 42.2% over time, with a statistically significant difference between months 2 and 6 post-HSCT (p < 0.001). Over the course of the follow-up, the low proportion of central memory (TCM: CD45RAā CCR7+) CD8+ T cells decreased from month 1 to month 12 post-HSCT (median from 2.77 to 1.31%, p = 0.039). We analyzed the expression and the mean fluorescence intensity (MFI) of PD-1 in CD8+ T-cell populations. Mean frequency of PD-1 expression among CD8+ T-cells ranged from 11.4 to 17.2% and the MFI for PD-1 did not differ significantly over time post-HSCT (Figures S1G,H in Supplementary Material).
Figure 1. HLA-A2 cytomegalovirus (CMV) tetramer reactive CD8+ T-cells and CMV-specific T-cells post-hematopoietic stem cell transplantation (HSCT). (A) Analysis of the CMV-specific CD8+ cytotoxic T-lymphocytes (CMV-CTL) in 23 patients followed individually over time post-HSCT. (B) IFN-γ production evaluated after overnight incubation of CMV-CTL isolated by q226a mutant tetramer (square) and total fraction of CMV-CTL (circle) with different concentration of CMV peptide and CD8 blocking antibody (in red, DK25) or isotype control (green). (C) HLA-A2 CMV tetramer reactive CD8+ T-cells frequency post-HSCT. Dark circles represent wild-type tetramer reactive T-cells, blue squares represent a245v mutant tetramer reactive T-cells and red triangles represent q226a mutant tetramer reactive T-cells. (D) HLA-A2 CMV tetramer reactive CD8+ T-cells allow the analysis of the different CMV-specific CD8+ T-cells depending on the affinity of the T-cell receptor (TCR). (E) Frequency of CMV-CTL with different TCR affinity in peripheral blood of patientās post-HSCT. Mean values are represented. (F) Analyses of the difference between the CMV-CTL subpopulations frequency in CD8+ T-cells. Mean and SD are represented. (G) Proportion analysis of the different subpopulations (low, medium, high-affinity) in the total of CMV-CTL. Mean and SD are represented. Black circles: low-affinity CMV-CTL, blue squares: medium-affinity CMV-CTL, red triangles: high-affinity CMV-CTL. Differences were analyzed using Wilcoxon matched-pairs signed rank test or Friedman test to detect differences between two groups of paired samples (A,E) and two-way ANOVA was used to detect differences between two groups over time (F,G). *p < 0.05, **p < 0.01, ***p < 0.001.
To summarize, in the HLA-A*02:01+ patient cohort CD8+ T-cells exhibited predominantly a TEM phenotype, and an increased TEMRA proportion among CD8+ T cells over 12 months following HSCT.
CMV-specific CD8+ cytotoxic T-lymphocytes reconstitution after HSCT was investigated in 23 patients at 1, 2, 3, 6, and 12 months post-HSCT by tetramer analysis. A total of 13 blood samples were found negative for CMV-CTL (Figure 1A), while some patients showed a frequency up to 21.3% CMV-CTL among total CD8+ T-cells over time. We observed a decreasing CMV-CTL mean frequency from 3.9 to 2.5% between months 6 and 12 post-HSCT (p = 0.006), yet no significant difference was seen over time post-HSCT using the Friedman test.
To further investigate the CMV-CTL reconstitution, three different HLA-A2 tetramers presenting the same CMVNLVPMVATV epitope (pp65 protein) were constructed to gauge MHC class I/CMV-specific T-cell responses (34, 35). The tetramers were (i) the wild-type tetramer; (ii) the mutant a245v tetramer, which due to the amino acid change reduces the MHC class I-CD8 co-receptor interaction (41); and (iii) the mutant q226a tetramer, which totally abrogates the interaction between MHC class I and the CD8 co-receptor (Figures S1A,B in Supplementary Material) (42ā44). The q226a mutant tetramer has not been reported previously; we, therefore, validated its ability to function independently of CD8 co-receptor binding. The anti-human CD8 blocking antibody (clone DK25) is known to target CD8α and blocks the activation of CD8+ T-cells at low-affinity TCR/MHC-I interaction (39). Q226a tetramer-reactive T-cells were isolated, incubated with an anti-CD8 blocking antibody, and stimulated with CMVNLVPMVATV peptide in dose-dependent manner. We did observe any inhibition of immune function detected by IFN-γ production. In contrast, total fraction of CM-CTL presented an inhibited production of IFN-γ when exposed to CD8 blocking antibody (Figure 1B). Thus, we concluded that the mutant q226a tetramer exclusively binds to high-affinity T-cells which do not require CD8 co-receptor interaction and stimulation to be activated. Wt, a245v, and q226a tetramer-reactive CD8+ T cells were present over time post-HSCT at similar proportions (Figure 1C). The wt tetramer binds to all the CMV-CTL population regardless of their TCR affinity, while the mutant a245v tetramer binds to CMV-CTL with medium and high-affinity TCR, and the mutant q226a tetramer exclusively binds to high-affinity TCR CMV-CTL (Figures 1C,D).
Cytomegalovirus tetramer-reactive CD8+ T cells do not inform on CMV-CTL affinity (Figures S1A,B in Supplementary Material). We, therefore, determined the āmediumā affinity CMV-CTL as a245v positive and q226a negative, and the ālowā affinity CMV-CTL as wt-positive, a245v-, and q226a-negative. The proportion of the CMV-CTL based on the TCR affinity was different from patients to patients and over time post-HSCT as some samples. In some patients, CMV-CTL with specific TCR affinity were more frequent, while other patients showed more equal proportion between the CM-CTL classes (as illustrated in Figure S2 in Supplementary Material). We observed significant differences in the frequency of low- and medium-affinity CMV-CTL between months 2 and 12 post-HSCT (p = 0.031 and 0.023, respectively, Figure 1E). High-affinity CMV-CTL was found to be at significantly higher frequency compared to the medium-affinity CMV-CTL (p = 0.007). Overall, the frequency of high- and low-affinity CMV-CTL did not differ significantly, although a trend was observed, where high-affinity CMV-CTL had a higher frequency than low-affinity CMV-CTL among CD8+ T cells (p = 0.052) (Figure 1F).
To further investigate the CMV-CTL classes based on the TCR affinity, we reported the proportion of the respective affinity categories (low/medium/high) of the total CMV-CTL population. While high-affinity CMV-CTL were present at higher frequencies over time post-HSCT, the medium-affinity CMV-CTL were found to be at a significant lower proportion compared to the low- and high-affinity CMV-CTL populations (p < 0.001 and 0.004). No differences in the proportions of high- and low-affinity CMV-CTL were observed (Figure 1G).
To summarize, mutant q226a tetramer enables the exclusive detection of CD8+ T cells with a high-affinity TCR without the requirement of the CD8ā coreceptor interaction. CMV-CTL with low- and high-affinity TCRs can be found at different frequencies and proportion in patients at various time points post-HSCT.
Analyzing the CD45RA and CCR7 expression of the three different CMV-CTL affinity populations, we determined the presence of different memory phenotypes within the CMV-CTL overtime post-HSCT. Overall, the three different classes of CMV-CTL presented a low proportion of naĆÆve (CD45RA+ CCR7+) and TCM T-cells. Low- and medium-affinity CMV-CTL demonstrated a predominant TEMRA phenotype while high-affinity CMV-CTL showed a predominant TEM phenotype (p < 0.001) overtime post-HSCT (Figure 2A). The TEMRA population in the low- and medium-affinity CMV-CTL presented an increase mean from 45.9 to 63.6% and from 39.1 to 55.3%, respectively. This increase was significant between months 1 and 6 in the medium-affinity CMV-CTL (p = 0.019). Reversely, the TEM population of the high-affinity CMV-CTL decreased over time (mean from 57.9 to 43.7%) and significant between 2 and 6 months post-HSCT (p = 0.037) (Figure S3A in Supplementary Material).
Figure 2. Memory phenotype and PD-1 expression of the cytomegalovirus(CMV)-specific CD8+ cytotoxic T-lymphocytes (CMV-CTL) with different T-cell receptor affinities over time post-hematopoietic stem cell transplantation (HSCT). (A) Memory phenotype of the different CMV-CTL subpopulations. (B) PD-1 expression in the different CMV-CTL subpopulations (C) Proportion of the high/medium/low-affinity CMV-CTL in the CD8+PD-1+ T-cell population. (D) Comparison of the high-affinity CMV-CTL frequency between the total CD8+ T-cell population and the CD8+PD-1+ T-cell population. Mean and SD are represented. Two-way ANOVA was used to detect differences between two groups over time. *p < 0.05, **p < 0.01, ***p < 0.001. (E) Pie charts with the proportion of high/medium/low-affinity CMV-CTL the total CD8+ T-cell population and the CD8+PD-1+ T-cell population at month 1 and 2 post-HSCT.
We analyzed the CMV-CTL affinity classes within the different memory subsets and observed that high-affinity CMV-CTL were found in a significantly higher proportion as compared to the medium and low CMV-CTL classes in the TEM CD8+ T-cell subset (p < 0.001 and 0.005). Conversely, the TEMRA population was preferentially enriched in CMV-CTL with low-affinity TCR (p = 0.001) (Figure S3B in Supplementary Material).
To summarize, phenotypic differences were observed within the CMV-CTL population depending on the TCR affinity, with high-affinity CMV-CTL displaying predominantly a TEM phenotype while low and medium-affinity CMV-CTL exhibited a TEMRA profile.
To further characterize the different classes of CMV-CTL during the reconstitution, we examined PD-1 expression. CD8+PD-1+ T-cells displayed a memory phenotype similar to the total CD8+ population with a predominant TEM phenotype, and an increasing TEMRA phenotype (mean from 28.5 to 37.4%, p < 0.039) between months 2 and 6 (Figure S3C in Supplementary Material).
Despite being a marker of exhaustion, PD-1 expression also identifies antigen-experienced T-cells (45). We, therefore, investigated the correlation between PD-1 expression and the different affinities of the CMV-CTL. PD-1 expression was significantly higher among cells with high-affinity TCR compared to the medium-affinity CMV-CTL (p = 0.013) post-HSCT, while no difference in PD-1 expression between low- and high-affinity CMV-CTL was observed (Figure 2B). Within the CD8+PD-1+ T-cell population, the proportion of the different CMV-CTL classes was found to be different as compared to the proportion described above in the total CD8+ T-cell population (Figure 1G): PD1+ high- and low-affinity CMV-CTL were found in higher proportion as compared to medium-affinity CMV-CTL (p < 0.001 and 0.003, respectively, Figure 2C; Figure S3D in Supplementary Material). Additionally, at month 1 post-HSCT only, PD1+ CMV-CTL with high-affinity TCR were found at significant higher proportion as compared to PD1+ low-affinity CMV-CTL (p = 0.012). Altogether, the frequency of CMV-CTL seemed to be higher in CD8+ T-cells expressing the PD-1 marker: high-affinity CMV-CTL appeared to be at higher frequency in CD8+PD-1+ T-cells as compared to the total CD8+ T-cell population (p < 0.001), and at 1 month post-HSCT (p = 0.036, Figure 2D). To a lesser extent, low-affinity CMV-CTL was as well found at higher frequency in the CD8+PD1+ subset as compared to the total CD8+ population (p = 0.049, Figure S3E in Supplementary Material). Consequently, the CMV-CTL with high-affinity TCR were found at higher proportion in the CD8+PD-1+ population as compared to the medium and low-affinity CMV-CTL and in the total CD8+ population in the early months post-HSCT (Figure 2E).
To summarize, CMV-CTL were found at higher frequency in the CD8+PD-1+ T-cells as compared to the total CD8+ T-cells. In the CD8+PD-1+ T-cell population, CMV-CTL with high-affinity TCR were found mostly early post-HSCT at higher proportion as compared to CMV-CTL with low- and medium-affinity TCR.
To understand the clinical impact of the CMV-CTL classes based on their TCR affinity, we evaluated the correlation between clinical data and the findings mentioned above, i.e., CMV serostatus, GVHD and CMV reactivation, CMV-CTL affinity classes, and PD-1 expression. The CMV-serological status of the donor and the CMV reactivation appeared to correlate to the total frequency of CMV-CTL, since patients with a donor presenting a CMV-positive serostatus (D+, n = 16) presented a higher frequency of CMV-CTL as compared to the patients with a CMV-negative donor (Dā, n = 7). Interestingly, when looking at the proportion of the different classes of CMV-CTL, we observed that the D+ patients presented a significant higher proportion of CMV-CTL with high-affinity (p = 0.012), while in the Dā patients, CMV-CTL expressed predominantly low-affinity TCRs (p = 0.011) (Figure 3A). No difference was observed in the proportion of medium-affinity CMV-CTL between the Dā and D+ patient group. Out of the five patients with a negative serological status, only four patients presented measurable CMV-CTL frequencies, therefore, no correlation analysis between the recipient serological status and the proportion of the different CMV-CTL could be performed.
Figure 3. Correlation with the donor serostatus and cytomegalovirus (CMV) reactivation. (A) Comparison of CMV-CTL frequency, low-, medium-, and high-affinity proportion between patients with donor presenting different CMV-serological status (D+: blue square and Dā: black circle) (B) Analysis of CMV-CTL in between patients diagnosed with CMV reactivation (red circle) and patients who did not present sign of reactivation (blue square). Mean and SD are represented. Two-way ANOVA was used to detect differences between two groups over time. *p < 0.05.
11 out of 23 patients were diagnosed for CMV reactivation during the 12 months post-transplantation. Patients diagnosed for CMV reactivation were found to have a higher frequency of CMV-CTL, yet, due to the small number of patients where there were no significant differences between the groups (p = 0.067, Figure 3B). As reported previously (2), we showed that CMV reactivation shapes the memory phenotype of the CD8+ T-cells, as in patients with CMV reactivation, CMV-CTL presented a significant higher TEM phenotype (p = 0.001) over time post-HSCT as compared to patients without CMV reactivation. On the other hand, patients with CMV reactivation showed a lower frequency of TEMRA CD8+ T-cells (p = 0.005, Figure S4A in Supplementary Material). PD-1 expression has also been described to be rapidly expressed after antigen exposure and is associated with an impairment of the immune system associated with chronic viral infections (46, 47), but no such observation was made in our study as we could not see differences in PD-1 expression between the patients with and without CMV reactivation (Figure S4B in Supplementary Material).
No significant correlation between clinically relevant grade (IIāIV) of acute GVHD (8/23) and our immunological characterization could be determined. However, patients with chronic GVHD (13/23, Table 1) had a higher frequency of CMV-CTL over time post-HSCT as compared to patients who did not present any sign of cGVHD (p < 0.001). High-affinity CMV-CTL were found in higher proportion in patients with cGVHD and conversely, low-affinity CMV-CTL proportion was higher in patients who did not present sign of cGVHD (p < 0.001, Figure 4A). Within the cGVHD patientsā group, CMV-CTL with high-affinity TCR was the dominant proportion of CMV-CTL, yet a significant difference was only observed with the medium-affinity CMV-CTL proportion (p < 0.001, Figure 4B). In patients diagnosed with CMV reactivation, 8/11 (72.7%) were diagnosed with cGVHD, while among the 12 patients that did not present any CMV reactivation, five of them (41.6%) presented cGVHD symptoms, but no correlation could be made between CMV reactivation and chronic GVHD in this small study cohort. Also, no correlation between the death of patients and the different CMV-CTL based on TCR affinity could be found possibly due to the limited number of patients included for this study.
Figure 4. Correlation between cytomegalovirus(CMV)-specific CD8+ cytotoxic T-lymphocytes(CTL) affinity and cgraft-versus-host disease(GVHD). (A) CMV-CTL frequency, low and high-affinity proportion correlated with cGVHD. Green circle: no cGVHD, orange square: cGVHD (IāIII) (B) Proportion of high/medium/low-affinity CMV-CTL in patients presenting clinical symptoms of cGVHD (IāIII). Black circles: low-affinity CMV-CTL, blue squares: medium-affinity CMV-CTL, red triangles: high-affinity CMV-CTL. Mean and SD are represented. Two-way ANOVA was used to detect differences between two groups over time.
To summarize, in patients with CMV reactivation, CMV-CTL showed a TEM phenotype over time post-HSCT. CMV-CTL with high-affinity TCR was significantly found at higher proportion in CMV-D+ patients and in patients diagnosed with cGVHD.
Cytomegalovirus-specific immune responses are crucial post-HSCT; transplant recipients with poor reconstitutions of CMV-specific T-cell response are more prone to develop CMV disease (3). While antiviral treatments remain the standard therapy for CMV infection post-HSCT, the clinical use of adoptive CMV-specific T-cell therapy has become a successful alternative over the past 25 years (19, 22, 23, 48). In this paper, we show the existence of different proportion of CMV-CTL based on their TCR affinity. This description was only possible by using three different HLA-A*02:01 CMVNLVPMVATV tetramers. To our knowledge, we reported here for the first time the ability of the mutant q226a tetramer to enable the detection of high-affinity CMV-CTL independent of the CD8-coreceptor interaction.
The current viewpoint on CMV-mediated immune responses is that high- and low-affinity T-cells have specific as well as overlapping functions, which partly shapes the diversity of the immune response during infection and disease (49, 50). In healthy individuals, high- and low-affinity antigen-specific CTL would preferably function together to maintain a broad TCR diversity, while eliciting an efficient and targeted immune response (44). High-affinity CTL is more likely to arise from clonal expansion of CD8+ T-cells and is, therefore, more suitable for ACT (51). In the context of HSCT, a245v mutant CMV tetramer T-cells was used in D+R+ patients to study the different avidities of CTL presenting similar phenotype and functional activity, but with different TCR repertoire from donor origin exclusively (52), however, the characterization of TCR affinity was not part of this study.
Affinity of CMV-specific T-cells has been indirectly described using blockade of the MHC class I interaction with an antibody directed against CD8 (51); different CMV-CTL clones reacted variably to infected cells. Their cytotoxic activity appeared to be influenced by the abrogation of CD8 binding (49). In our study, we observed that the same population of virus-specific T-cells could have different memory phenotypes, since the high-affinity CD8+ T-cells were mostly effector memory T-cells as compared to the medium- and low-affinity CD8+ T-cells. Correlation between high-affinity TCR and effector phenotype has been described earlier and high-affinity T-cells may detect early viral antigens during low-level infection (53ā56). Similar observations were made by Price DA et al. in which they showed that distinct CMV-CTL clones exhibited different memory phenotypes by using a D227K/T228A mutant tetramer to target high affinity CD8+ T-cells (57). Furthermore, high-affinity clones have been found to dominate the adaptive immune response at the peak of the effector response (58) and enriched in large frequency in the memory pool, with T-cells expressing high-affinity TCR during secondary response (59). Together, this observation suggests that long-lived protective T-cell responses may be fueled by high-affinity CD8+ T-cells with an effector memory phenotype (60, 61).
Programmed death-1 expression has been previously associated with post-HSCT relapse in patients with acute myeloid leukemia, but does not exert a deleterious effect on the functionality of T-cells, based on their ability to produce cytokines in response to polyclonal stimulation (62). In addition, the increase expression of PD-1 in T-cells has also been linked to persistent CMV infection and GVHD (46, 47). However, no significant differences in the PD-1 expression pattern were observed in our study over time post-HSCT, certainly due to the few number of patients diagnosed with CMV disease (n = 3). Conversely, we demonstrate here a correlation between PD-1 expression and CMV-specific CD8+ T-cells response, i.e., the CMV-CTL population with high-affinity TCR was found at higher frequency among the PD-1 positive T-cells compared to the frequency observed in total CD8+ T-cells. Considering that the PD-1+CD8+ T-cells from HSCT recipients mainly express an effector memory phenotype, the expression of PD-1 is not necessarily associated with T-cell dysfunction. CMV-specific response has its particularity; unlike antigen-specific T-cells in other chronic viral infections, i.e., LCMV, HIV, HCV, or HBV, where PD-1 expression is rather high (63ā66), CMV-specific T-cells seem to express PD-1 at a much lower levels (approximately 5ā12%). CD8+PD-1+ T-cells specific for CMV epitopes may preserve the full spectrum of their functional capacity by producing cytokines albeit without cytotoxic or proliferative potential (67). CD8+PD-1+ T-cells are very likely to have undergone antigen-driven clonal expansion in the HSCT recipients during immune reconstitution, and are possibly associated with recognition of private epitopes, such as in advanced cancers (68, 69). PD-1 expression may in fact prevent the exhausted/antigen-experienced T-cells from being programmed for terminal differentiation and excessive proliferation (70). It was shown that virus-specific CD8+ tumor-infiltrating lymphocytes expressing PD-1 were not impaired, but described as newly antigen experienced (71). In the tumor environment, high-affinity CD8+ T-cell clone presented a significant anti-tumor response with a lower expression of PD-1, while the low-affinity clone increasingly expressed several co-inhibitory molecules (72).
Donor CMV serostatus and conditioning of patients can impact the outcome of HSCT as well as the reconstitution of the CMV-specific immune response post-HSCT (73ā77). CMV-CTL from the donors have been described to be multifunctional as defined by production of IFN-γ and TNF-α, co-expression of the degranulation marker CD107a and macrophage inflammatory protein-1β (78). Similar observations were made in this study as patients with D+ CMV serostatus presented a higher frequency of CMV-CTL most likely from donor origin, since the chimerism analysis of the CD3+ cells indicated that the CMV-CTL were of donor origin, at least at the later time points post-HSCT.
Association between viral reactivation, its specific immune response, and GVHD have been studied extensively and several studies showed that GVHD treatment increases the risk of CMV replication, whereas the data showing the reverse relationship remain controversial (79ā81). One strong argument against this reverse relationship would be the use of CMV-CTL ACT for restoring anti-viral T-cell immunity that has been clinically applied over the past 25 years without showing any increased risk to induce cGVHD (23, 82, 83). Isolation with MHC tetramer of CMV-CTL for ACT purposes have, to our knowledge, previously been used on non-mutated tetramers, therefore, the affinity of the ACT product has never been specifically characterized before infusion (18, 84ā87). In this study, we give a more general insight on possible improvement of the ACT protocols as the selection of CD8+ T-cells with specific TCR affinity may be more effective in clearing infected or tumor cells and conferring a better long-term protective memory. The affinity of the interaction between the TCR and the peptide bound to the MHC molecule is known to play a major role in the efficacy of ACT and it is suggested that high-affinity T-cells with TCR independent of CD8 binding to the MHC may be beneficial for clinical applications, including ACT (88). Thus, assessment and selection of antigen-specific T-cells with optimal affinity/reactivity from a heterogeneous T-cell population may help in the development to improve the T-cell product and thereby enhance clinical efficacy. This selection based on TCR affinity has to be careful as we observed that patients with cGVHD exhibit a higher frequency of CMV-CTL and a significantly higher proportion of CMV-CTL with high-affinity TCR, while low-affinity CMV-CTL were at higher proportion in patients who did not present any symptoms of cGVHD. Interestingly, previous studies have suggested that T-cells with the highest affinity for its antigen were the most alloreactive (89, 90). A recent clinical trial using engineered T-cells expressing an affinity-enhanced TCR for tumor-associated antigens demonstrated unpredictable off-target due to recognition of an unrelated peptide resulting in the death of two treated patients (91).
This study is limited by its small cohort size and the low number of T-cell events due to the HSCT conditioning. This study was also mostly composed by sibling donors and RIC transplants cohort as we selected patients not treated with ATG in order to increase the chance to observe CMV-CTL at sufficient number to further phenotype and characterize them. Therefore, further investigation using the CMV tetramers identifying TCRs with different affinities in a larger and more heterogeneous cohort and in healthy individuals would allow to explore the function of the different CMV-CTL subpopulations. This would give a more descriptive picture and clear insight of the TCR affinity implication following HSCT procedure. Cell expansion of the different CMV-CTL subpopulations would give a more comprehensive characterization of their proliferation potentials that could also be tailored for adaptive cellular immunotherapies targeting viral epitopes. It would also be interesting to see whether blockade of the PD-1 pathway can differently affect the expansion and function of the different subpopulations of CD8+ T-cells in patients undergoing HSCT.
In conclusion, we have investigated the reconstitution of CMV-CTL with different TCR affinities in HSCT patients by using three different tetramers. The q226a mutant tetramer identifies exclusively high-affinity CMV-CTL that does not require the CD8 co-receptor for MHC-TCR interaction. High-affinity CMV-CTL could be found at higher proportion in experienced CD8+PD-1+ population and presented an effector phenotype in contrast to the CMV-CTL with low- and medium-affinity TCR that exhibited a dominant TEMRA phenotype in patients after HSCT. Patients with chronic GHVD exhibited a higher proportion of high-affinity CMV-CTL, while patients who did not present any sign of cGVHD showed a dominant proportion of low-affinity CMV-CTL. Further studies on the TCR affinity of CMV-CTL after HSCT are needed to elucidate their functions and possible clinical implication post-HSCT and for ACT.
Ethical approval was obtained from the regional review board of ethics in research of Karolinska Institutet (Stockholm Ethical Committee South 2010/760-31/1). Written informed consent was received from participants prior to inclusion in the study.
TP conceived, designed, performed, and analyzed the experiments, interpreted the results, performed statistical analysis, and wrote the paper. RA-R conceived and designed the tetramers, interpreted and analyzed the experiments and results, and wrote the paper. MRemberger interpreted the results and performed statistical analysis. MRao and X-HL interpreted the results and wrote the paper. AN and AL performed the experiments. IE, OR and MM conceived, designed the experiments, and wrote the paper. All authors participated in final approval of the manuscript.
The authors declare that the research was conducted in the absence of any commercial or financial relationships that could be construed as a potential conflict of interest.
The reviewer EA and handling Editor declared their shared affiliation.
The authors are grateful to the nurses at CAST and to Pr. Per Ljungman from the Department of Hematology, Karolinska University Hospital for his valuable advices; we are indebted to our patients who agreed to participate in the study.
This work was supported by Barncancerfonden grant number is PR2016-0076, Sweden to IE, MM, and OR.
The Supplementary Material for this article can be found online at https://www.frontiersin.org/articles/10.3389/fimmu.2018.00760/full#supplementary-material.
Figure S1. Phenotype of the CD8+ T-cell population post-HSCT. (A) Representative flow cytometry plots from one patient showing the gating strategies to identify the different cell subsets. (B) Gating strategy to separate the different subset of CMV-CTL based on the tetramer staining. (C) Donor chimerism on CD3+ cells in peripheral blood post-HSCT expressed as percentage of donor DNA medians are represented. (D) CD8+ T-cells reconstitution post-HSCT. (E) Memory phenotype composition of the CD8+ T-cells based on CD45RA and CCR7 markers. (F) Memory phenotype of the CD8+ T-cells in individual patients followed post-HSCT. (G) PD1 frequency in CD8+ T-cell population post-HSCT. (H) PD1 mean fluorescence intensity (MFI) in the CD8+ T-cell subsets. Means are represented for (E,G,H). Wilcoxon matched-pairs signed rank test or Friedman test to detect differences across between two matched timepoints or repeated matched time points after HSCT. *p < 0.05, **p < 0.01, ***p < 0.001.
Figure S2. Representative flow cytometry plots from three different patients illustrating the different configurations of CMV-CTL proportion based on the TCR affinity. Configuration 1 represents a minor proportion of high affinity CMV-CTL, configuration 2 represents an equal proportion between CMV-CTL with different TCR affinity, and configuration 3 represent a dominant proportion of high affinity CMV-CTL.
Figure S3. Memory subsets and PD1 expression of the different CD8+ T-cell affinity subgroups CMV tetramer reactive CD8+ T-cells. (A) Low- and medium-affinity CMV-CTL presented a more TEMRA profile over the time post-HSCT. High affinity CMV-CTL presented a dominant but decreasing TEM phenotype over time post-HSCT. *p < 0.05. (B) Proportion of CMV-CTL with different TCR affinities in the TEM and TEMRA memory subsets post-HSCT. Means and SD are represented. (C) Memory phenotype composition of the CD8+ PD-1+ T-cells based on CD45RA and CCR7 markers. Means are represented. (D) Low-, medium-, and high-affinity CMV-CTL frequency in the CD8+ PD-1+ T-cells. (E) Comparison of low-affinity CMV-CTL frequency in CD8+ T-cells and CD8+ PD-1+ T-cells. Means and SD are represented. Wilcoxon matched-pairs signed rank test or Friedman test to detect differences across between two matched timepoints or repeated matched time points after HSC in (A) and two-way ANOVA was used to detect differences between two groups over time in (B,E).
Figure S4. Clinical correlation (A) CD8+ T-cell memory phenotype correlates to CMV reactivation. Patients with CMV reactivation presented a higher frequency of TEM T-cells and a lower frequency of TEMRA T-cells. (B) Expression of PD-1 did not correlate with CMV reactivation over time post-HSCT. Means and SD are represented and two-way ANOVA was used to detect differences between two groups over time.
1. Hanley PJ, Bollard CM. Controlling cytomegalovirus: helping the immune system take the lead. Viruses (2014) 6:2242ā58. doi:10.3390/v6062242
2. Klenerman P, Oxenius A. T cell responses to cytomegalovirus. Nat Rev Immunol (2016) 16:367ā77. doi:10.1038/nri.2016.38
3. Ariza-Heredia EJ, Nesher L, Chemaly RF. Cytomegalovirus diseases after hematopoietic stem cell transplantation: a mini-review. Cancer Lett (2014) 342:1ā8. doi:10.1016/j.canlet.2013.09.004
4. Patel EU, Gianella S, Newell K, Tobian AA, Kirkpatrick AR, Nalugoda F, et al. Elevated cytomegalovirus IgG antibody levels are associated with HIV-1 disease progression and immune activation. AIDS (2017) 31:807ā13. doi:10.1097/QAD.0000000000001412
5. Gooley TA, Chien JW, Pergam SA, Hingorani S, Sorror ML, Boeckh M, et al. Reduced mortality after allogeneic hematopoietic-cell transplantation. N Engl J Med (2010) 363:2091ā101. doi:10.1056/NEJMoa1004383
6. Nichols WG, Corey L, Gooley T, Davis C, Boeckh M. High risk of death due to bacterial and fungal infection among cytomegalovirus (CMV)-seronegative recipients of stem cell transplants from seropositive donors: evidence for indirect effects of primary CMV infection. J Infect Dis (2002) 185:273ā82. doi:10.1086/338624
7. Cantoni N, Hirsch HH, Khanna N, Gerull S, Buser A, Bucher C, et al. Evidence for a bidirectional relationship between cytomegalovirus replication and acute graft-versus-host disease. Biol Blood Marrow Transplant (2010) 16:1309ā14. doi:10.1016/j.bbmt.2010.03.020
8. Broers AE, van Der Holt R, van Esser JW, Gratama JW, Henzen-Logmans S, Kuenen-Boumeester V, et al. Increased transplant-related morbidity and mortality in CMV-seropositive patients despite highly effective prevention of CMV disease after allogeneic T-cell-depleted stem cell transplantation. Blood (2000) 95:2240ā5.
9. Takenaka K, Nishida T, Asano-Mori Y, Oshima K, Ohashi K, Mori T, et al. Cytomegalovirus reactivation after allogeneic hematopoietic stem cell transplantation is associated with a reduced risk of relapse in patients with acute myeloid leukemia who survived to day 100 after transplantation: the Japan Society for Hematopoietic Cell Transplantation Transplantation-related Complication Working Group. Biol Blood Marrow Transplant (2015) 21:2008ā16. doi:10.1016/j.bbmt.2015.07.019
10. Lonnqvist B, Ringden O, Ljungman P, Wahren B, Gahrton G. Reduced risk of recurrent leukaemia in bone marrow transplant recipients after cytomegalovirus infection. Br J Haematol (1986) 63:671ā9. doi:10.1111/j.1365-2141.1986.tb07551.x
11. Elmaagacli AH, Steckel NK, Koldehoff M, Hegerfeldt Y, Trenschel R, Ditschkowski M, et al. Early human cytomegalovirus replication after transplantation is associated with a decreased relapse risk: evidence for a putative virus-versus-leukemia effect in acute myeloid leukemia patients. Blood (2011) 118:1402ā12. doi:10.1182/blood-2010-08-304121
12. Scheper W, van Dorp S, Kersting S, Pietersma F, Lindemans C, Hol S, et al. GammadeltaT cells elicited by CMV reactivation after allo-SCT cross-recognize CMV and leukemia. Leukemia (2013) 27:1328ā38. doi:10.1038/leu.2012.374
13. Teira P, Battiwalla M, Ramanathan M, Barrett AJ, Ahn KW, Chen M, et al. Early cytomegalovirus reactivation remains associated with increased transplant-related mortality in the current era: a CIBMTR analysis. Blood (2016) 127:2427ā38. doi:10.1182/blood-2015-11-679639
14. Thomson KJ, Mackinnon S, Peggs KS. CMV-specific cellular therapy for acute myeloid leukemia? Blood (2012) 119:1088ā90; author reply 1090ā1. doi:10.1182/blood-2011-10-383943
15. Schmidt-Hieber M, Labopin M, Beelen D, Volin L, Ehninger G, Finke J, et al. CMV serostatus still has an important prognostic impact in de novo acute leukemia patients after allogeneic stem cell transplantation: a report from the Acute Leukemia Working Party of EBMT. Blood (2013) 122:3359ā64. doi:10.1182/blood-2013-05-499830
16. Ljungman P, Hakki M, Boeckh M. Cytomegalovirus in hematopoietic stem cell transplant recipients. Hematol Oncol Clin North Am (2011) 25:151ā69. doi:10.1016/j.hoc.2010.11.011
17. Uhlin M, Gertow J, Uzunel M, Okas M, Berglund S, Watz E, et al. Rapid salvage treatment with virus-specific T cells for therapy-resistant disease. Clin Infect Dis (2012) 55:1064ā73. doi:10.1093/cid/cis625
18. Cobbold M, Khan N, Pourgheysari B, Tauro S, McDonald D, Osman H, et al. Adoptive transfer of cytomegalovirus-specific CTL to stem cell transplant patients after selection by HLA-peptide tetramers. J Exp Med (2005) 202:379ā86. doi:10.1084/jem.20040613
19. Einsele H, Roosnek E, Rufer N, Sinzger C, Riegler S, Loffler J, et al. Infusion of cytomegalovirus (CMV)-specific T cells for the treatment of CMV infection not responding to antiviral chemotherapy. Blood (2002) 99:3916ā22. doi:10.1182/blood.V99.11.3916
20. Feuchtinger T, Opherk K, Bethge WA, Topp MS, Schuster FR, Weissinger EM, et al. Adoptive transfer of pp65-specific T cells for the treatment of chemo-refractory cytomegalovirus disease or reactivation after haploidentical and matched unrelated stem cell transplantation. Blood (2010) 116:4360ā7. doi:10.1182/blood-2010-01-262089
21. Peggs KS, Thomson K, Samuel E, Dyer G, Armoogum J, Chakraverty R, et al. Directly selected cytomegalovirus-reactive donor T cells confer rapid and safe systemic reconstitution of virus-specific immunity following stem cell transplantation. Clin Infect Dis (2011) 52:49ā57. doi:10.1093/cid/ciq042
22. Peggs KS, Verfuerth S, Pizzey A, Khan N, Guiver M, Moss PA, et al. Adoptive cellular therapy for early cytomegalovirus infection after allogeneic stem-cell transplantation with virus-specific T-cell lines. Lancet (2003) 362:1375ā7. doi:10.1016/S0140-6736(03)14634-X
23. Riddell SR, Watanabe KS, Goodrich JM, Li CR, Agha ME, Greenberg PD. Restoration of viral immunity in immunodeficient humans by the adoptive transfer of T cell clones. Science (1992) 257:238ā41. doi:10.1126/science.1352912
24. Mahnke YD, Schwendemann J, Beckhove P, Schirrmacher V. Maintenance of long-term tumour-specific T-cell memory by residual dormant tumour cells. Immunology (2005) 115:325ā36. doi:10.1111/j.1365-2567.2005.02163.x
25. Smith CJ, Quinn M, Snyder CM. CMV-specific CD8 T cell differentiation and localization: implications for adoptive therapies. Front Immunol (2016) 7:352. doi:10.3389/fimmu.2016.00352
26. Parker BS, Rautela J, Hertzog PJ. Antitumour actions of interferons: implications for cancer therapy. Nat Rev Cancer (2016) 16:131ā44. doi:10.1038/nrc.2016.14
27. Gamadia LE, Remmerswaal EB, Weel JF, Bemelman F, van Lier RA, Ten Berge IJ. Primary immune responses to human CMV: a critical role for IFN-gamma-producing CD4+ T cells in protection against CMV disease. Blood (2003) 101:2686ā92. doi:10.1182/blood-2002-08-2502
28. Klebanoff CA, Gattinoni L, Restifo NP. CD8+ T-cell memory in tumor immunology and immunotherapy. Immunol Rev (2006) 211:214ā24. doi:10.1111/j.0105-2896.2006.00391.x
29. Jahn G, Scholl BC, Traupe B, Fleckenstein B. The two major structural phosphoproteins (pp65 and pp150) of human cytomegalovirus and their antigenic properties. J Gen Virol (1987) 68(Pt 5):1327ā37. doi:10.1099/0022-1317-68-5-1327
30. Glucksberg H, Storb R, Fefer A, Buckner CD, Neiman PE, Clift RA, et al. Clinical manifestations of graft-versus-host disease in human recipients of marrow from HL-A-matched sibling donors. Transplantation (1974) 18:295ā304. doi:10.1097/00007890-197410000-00001
31. Solders M, Erkers T, Gorchs L, Poiret T, Remberger M, Magalhaes I, et al. Mucosal-associated invariant T cells display a poor reconstitution and altered phenotype after allogeneic hematopoietic stem cell transplantation. Front Immunol (2017) 8:1861. doi:10.3389/fimmu.2017.01861
32. Yun Z, Lewensohn-Fuchs I, Ljungman P, Ringholm L, Jonsson J, Albert J. A real-time TaqMan PCR for routine quantitation of cytomegalovirus DNA in crude leukocyte lysates from stem cell transplant patients. J Virol Methods (2003) 110:73ā9. doi:10.1016/S0166-0934(03)00103-4
33. Bjorklund AT, Clancy T, Goodridge JP, Beziat V, Schaffer M, Hovig E, et al. Naive donor NK cell repertoires associated with less leukemia relapse after allogeneic hematopoietic stem cell transplantation. J Immunol (2016) 196:1400ā11. doi:10.4049/jimmunol.1501434
34. Salter RD, Norment AM, Chen BP, Clayberger C, Krensky AM, Littman DR, et al. Polymorphism in the alpha 3 domain of HLA-A molecules affects binding to CD8. Nature (1989) 338:345ā7. doi:10.1038/338345a0
35. Salter RD, Benjamin RJ, Wesley PK, Buxton SE, Garrett TP, Clayberger C, et al. A binding site for the T-cell co-receptor CD8 on the alpha 3 domain of HLA-A2. Nature (1990) 345:41ā6. doi:10.1038/345041a0
36. Altman JD, Moss PA, Goulder PJ, Barouch DH, McHeyzer-Williams MG, Bell JI, et al. Phenotypic analysis of antigen-specific T lymphocytes. Science (1996) 274:94ā6. doi:10.1126/science.274.5284.94
37. Garboczi DN, Hung DT, Wiley DC. HLA-A2-peptide complexes: refolding and crystallization of molecules expressed in Escherichia coli and complexed with single antigenic peptides. Proc Natl Acad Sci U S A (1992) 89:3429ā33. doi:10.1073/pnas.89.8.3429
38. Magalhaes I, Vudattu NK, Ahmed RK, Kuhlmann-Berenzon S, Ngo Y, Sizemore DR, et al. High content cellular immune profiling reveals differences between rhesus monkeys and men. Immunology (2010) 131:128ā40. doi:10.1111/j.1365-2567.2010.03284.x
39. Clement M, Pearson JA, Gras S, van den Berg HA, Lissina A, Llewellyn-Lacey S, et al. Targeted suppression of autoreactive CD8(+) T-cell activation using blocking anti-CD8 antibodies. Sci Rep (2016) 6:35332. doi:10.1038/srep35332
40. Wooldridge L, Hutchinson SL, Choi EM, Lissina A, Jones E, Mirza F, et al. Anti-CD8 antibodies can inhibit or enhance peptide-MHC class I (pMHCI) multimer binding: this is paralleled by their effects on CTL activation and occurs in the absence of an interaction between pMHCI and CD8 on the cell surface. J Immunol (2003) 171:6650ā60. doi:10.4049/jimmunol.171.12.6650
41. Dutoit V, Guillaume P, Ayyoub M, Hesdorffer CS, Luescher IF, Valmori D. Decreased binding of peptides-MHC class I (pMHC) multimeric complexes to CD8 affects their binding avidity for the TCR but does not significantly impact on pMHC/TCR dissociation rate. J Immunol (2003) 170:5110ā7. doi:10.4049/jimmunol.170.10.5110
42. Wooldridge L, van den Berg HA, Glick M, Gostick E, Laugel B, Hutchinson SL, et al. Interaction between the CD8 coreceptor and major histocompatibility complex class I stabilizes T cell receptor-antigen complexes at the cell surface. J Biol Chem (2005) 280:27491ā501. doi:10.1074/jbc.M500555200
43. Choi EM, Chen JL, Wooldridge L, Salio M, Lissina A, Lissin N, et al. High avidity antigen-specific CTL identified by CD8-independent tetramer staining. J Immunol (2003) 171:5116ā23. doi:10.4049/jimmunol.171.10.5116
44. Griffiths SJ, Riddell NE, Masters J, Libri V, Henson SM, Wertheimer A, et al. Age-associated increase of low-avidity cytomegalovirus-specific CD8+ T cells that re-express CD45RA. J Immunol (2013) 190:5363ā72. doi:10.4049/jimmunol.1203267
45. Francisco LM, Sage PT, Sharpe AH. The PD-1 pathway in tolerance and autoimmunity. Immunol Rev (2010) 236:219ā42. doi:10.1111/j.1600-065X.2010.00923.x
46. Gallez-Hawkins GM, Thao L, Palmer J, Dagis A, Li X, Franck AE, et al. Increased programmed death-1 molecule expression in cytomegalovirus disease and acute graft-versus-host disease after allogeneic hematopoietic cell transplantation. Biol Blood Marrow Transplant (2009) 15:872ā80. doi:10.1016/j.bbmt.2009.03.022
47. Kato T, Nishida T, Ito Y, Murase M, Murata M, Naoe T. Correlations of programmed death 1 expression and serum IL-6 level with exhaustion of cytomegalovirus-specific T cells after allogeneic hematopoietic stem cell transplantation. Cell Immunol (2014) 288:53ā9. doi:10.1016/j.cellimm.2014.02.007
48. Papadopoulou A, Gerdemann U, Katari UL, Tzannou I, Liu H, Martinez C, et al. Activity of broad-spectrum T cells as treatment for AdV, EBV, CMV, BKV, and HHV6 infections after HSCT. Sci Transl Med (2014) 6:242ra83. doi:10.1126/scitranslmed.3008825
49. Khan N, Cobbold M, Cummerson J, Moss PA. Persistent viral infection in humans can drive high frequency low-affinity T-cell expansions. Immunology (2010) 131:537ā48. doi:10.1111/j.1365-2567.2010.03326.x
50. Martinez RJ, Evavold BD. Lower affinity T cells are critical components and active participants of the immune response. Front Immunol (2015) 6:468. doi:10.3389/fimmu.2015.00468
51. Trautmann L, Rimbert M, Echasserieau K, Saulquin X, Neveu B, Dechanet J, et al. Selection of T cell clones expressing high-affinity public TCRs within human cytomegalovirus-specific CD8 T cell responses. J Immunol (2005) 175:6123ā32. doi:10.4049/jimmunol.175.9.6123
52. Ogonek J, Verma K, Schultze-Florey C, Varanasi P, Luther S, Schweier P, et al. Characterization of high-avidity cytomegalovirus-specific T cells with differential tetramer binding coappearing after allogeneic stem cell transplantation. J Immunol (2017) 199:792ā805. doi:10.4049/jimmunol.1601992
53. Huang J, Zarnitsyna VI, Liu B, Edwards LJ, Jiang N, Evavold BD, et al. The kinetics of two-dimensional TCR and pMHC interactions determine T-cell responsiveness. Nature (2010) 464:932ā6. doi:10.1038/nature08944
54. Frost EL, Kersh AE, Evavold BD, Lukacher AE. Cutting edge: resident memory CD8 T cells express high-affinity TCRs. J Immunol (2015) 195:3520ā4. doi:10.4049/jimmunol.1501521
55. Busch DH, Pamer EG. T cell affinity maturation by selective expansion during infection. J Exp Med (1999) 189:701ā10. doi:10.1084/jem.189.4.701
56. King CG, Koehli S, Hausmann B, Schmaler M, Zehn D, Palmer E. T cell affinity regulates asymmetric division, effector cell differentiation, and tissue pathology. Immunity (2012) 37:709ā20. doi:10.1016/j.immuni.2012.06.021
57. Price DA, Brenchley JM, Ruff LE, Betts MR, Hill BJ, Roederer M, et al. Avidity for antigen shapes clonal dominance in CD8+ T cell populations specific for persistent DNA viruses. J Exp Med (2005) 202:1349ā61. doi:10.1084/jem.20051357
58. Ozga AJ, Moalli F, Abe J, Swoger J, Sharpe J, Zehn D, et al. pMHC affinity controls duration of CD8+ T cell-DC interactions and imprints timing of effector differentiation versus expansion. J Exp Med (2016) 213:2811ā29. doi:10.1084/jem.20160206
59. Savage PA, Boniface JJ, Davis MM. A kinetic basis for T cell receptor repertoire selection during an immune response. Immunity (1999) 10:485ā92. doi:10.1016/S1074-7613(00)80048-5
60. Corse E, Gottschalk RA, Allison JP. Strength of TCR-peptide/MHC interactions and in vivo T cell responses. J Immunol (2011) 186:5039ā45. doi:10.4049/jimmunol.1003650
61. Gourley TS, Wherry EJ, Masopust D, Ahmed R. Generation and maintenance of immunological memory. Semin Immunol (2004) 16:323ā33. doi:10.1016/j.smim.2004.08.013
62. Schnorfeil FM, Lichtenegger FS, Emmerig K, Schlueter M, Neitz JS, Draenert R, et al. T cells are functionally not impaired in AML: increased PD-1 expression is only seen at time of relapse and correlates with a shift towards the memory T cell compartment. J Hematol Oncol (2015) 8:93. doi:10.1186/s13045-015-0189-2
63. Wherry EJ, Ha SJ, Kaech SM, Haining WN, Sarkar S, Kalia V, et al. Molecular signature of CD8+ T cell exhaustion during chronic viral infection. Immunity (2007) 27:670ā84. doi:10.1016/j.immuni.2007.11.006
64. Day CL, Kaufmann DE, Kiepiela P, Brown JA, Moodley ES, Reddy S, et al. PD-1 expression on HIV-specific T cells is associated with T-cell exhaustion and disease progression. Nature (2006) 443:350ā4. doi:10.1038/nature05115
65. Golden-Mason L, Palmer B, Klarquist J, Mengshol JA, Castelblanco N, Rosen HR. Upregulation of PD-1 expression on circulating and intrahepatic hepatitis C virus-specific CD8+ T cells associated with reversible immune dysfunction. J Virol (2007) 81:9249ā58. doi:10.1128/JVI.00409-07
66. Evans A, Riva A, Cooksley H, Phillips S, Puranik S, Nathwani A, et al. Programmed death 1 expression during antiviral treatment of chronic hepatitis B: impact of hepatitis B e-antigen seroconversion. Hepatology (2008) 48:759ā69. doi:10.1002/hep.22419
67. Trautmann L, Janbazian L, Chomont N, Said EA, Gimmig S, Bessette B, et al. Upregulation of PD-1 expression on HIV-specific CD8+ T cells leads to reversible immune dysfunction. Nat Med (2006) 12:1198ā202. doi:10.1038/nm1106-1329b
68. Gros A, Robbins PF, Yao X, Li YF, Turcotte S, Tran E, et al. PD-1 identifies the patient-specific CD8(+) tumor-reactive repertoire infiltrating human tumors. J Clin Invest (2014) 124:2246ā59. doi:10.1172/JCI73639
69. McGranahan N, Furness AJ, Rosenthal R, Ramskov S, Lyngaa R, Saini SK, et al. Clonal neoantigens elicit T cell immunoreactivity and sensitivity to immune checkpoint blockade. Science (2016) 351:1463ā9. doi:10.1126/science.aaf1490
70. Odorizzi PM, Pauken KE, Paley MA, Sharpe A, Wherry EJ. Genetic absence of PD-1 promotes accumulation of terminally differentiated exhausted CD8+ T cells. J Exp Med (2015) 212:1125ā37. doi:10.1084/jem.20142237
71. Erkes DA, Smith CJ, Wilski NA, Caldeira-Dantas S, Mohgbeli T, Snyder CM. Virus-specific CD8(+) T cells infiltrate melanoma lesions and retain function independently of PD-1 expression. J Immunol (2017) 198:2979ā88. doi:10.4049/jimmunol.1601064
72. Bos R, Marquardt KL, Cheung J, Sherman LA. Functional differences between low- and high-affinity CD8(+) T cells in the tumor environment. Oncoimmunology (2012) 1:1239ā47. doi:10.4161/onci.21285
73. Ljungman P, Brand R, Hoek J, de la Camara R, Cordonnier C, Einsele H, et al. Donor cytomegalovirus status influences the outcome of allogeneic stem cell transplant: a study by the European group for blood and marrow transplantation. Clin Infect Dis (2014) 59:473ā81. doi:10.1093/cid/ciu364
74. Boland GJ, Vlieger AM, Ververs C, De Gast GC. Evidence for transfer of cellular and humoral immunity to cytomegalovirus from donor to recipient in allogeneic bone marrow transplantation. Clin Exp Immunol (1992) 88:506ā11. doi:10.1111/j.1365-2249.1992.tb06479.x
75. Hakki M, Riddell SR, Storek J, Carter RA, Stevens-Ayers T, Sudour P, et al. Immune reconstitution to cytomegalovirus after allogeneic hematopoietic stem cell transplantation: impact of host factors, drug therapy, and subclinical reactivation. Blood (2003) 102:3060ā7. doi:10.1182/blood-2002-11-3472
76. Li CR, Greenberg PD, Gilbert MJ, Goodrich JM, Riddell SR. Recovery of HLA-restricted cytomegalovirus (CMV)-specific T-cell responses after allogeneic bone marrow transplant: correlation with CMV disease and effect of ganciclovir prophylaxis. Blood (1994) 83:1971ā9.
77. Borchers S, Luther S, Lips U, Hahn N, Kontsendorn J, Stadler M, et al. Tetramer monitoring to assess risk factors for recurrent cytomegalovirus reactivation and reconstitution of antiviral immunity post allogeneic hematopoietic stem cell transplantation. Transpl Infect Dis (2011) 13:222ā36. doi:10.1111/j.1399-3062.2011.00626.x
78. Zhou W, Longmate J, Lacey SF, Palmer JM, Gallez-Hawkins G, Thao L, et al. Impact of donor CMV status on viral infection and reconstitution of multifunction CMV-specific T cells in CMV-positive transplant recipients. Blood (2009) 113:6465ā76. doi:10.1182/blood-2009-02-203307
79. Larsson K, Aschan J, Remberger M, Ringden O, Winiarski J, Ljungman P. Reduced risk for extensive chronic graft-versus-host disease in patients receiving transplants with human leukocyte antigen-identical sibling donors given polymerase chain reaction-based preemptive therapy against cytomegalovirus. Transplantation (2004) 77:526ā31. doi:10.1097/01.TP.0000109778.39235.F4
80. Soderberg C, Larsson S, Rozell BL, Sumitran-Karuppan S, Ljungman P, Moller E. Cytomegalovirus-induced CD13-specific autoimmunity ā a possible cause of chronic graft-vs-host disease. Transplantation (1996) 61:600ā9. doi:10.1097/00007890-199602270-00015
81. Lonnqvist B, Ringden O, Wahren B, Gahrton G, Lundgren G. Cytomegalovirus infection associated with and preceding chronic graft-versus-host disease. Transplantation (1984) 38:465ā8. doi:10.1097/00007890-198411000-00004
82. Walter EA, Greenberg PD, Gilbert MJ, Finch RJ, Watanabe KS, Thomas ED, et al. Reconstitution of cellular immunity against cytomegalovirus in recipients of allogeneic bone marrow by transfer of T-cell clones from the donor. N Engl J Med (1995) 333:1038ā44. doi:10.1056/NEJM199510193331603
83. Blyth E, Clancy L, Simms R, Ma CK, Burgess J, Deo S, et al. Donor-derived CMV-specific T cells reduce the requirement for CMV-directed pharmacotherapy after allogeneic stem cell transplantation. Blood (2013) 121:3745ā58. doi:10.1182/blood-2012-08-448977
84. Chen FE, Aubert G, Travers P, Dodi IA, Madrigal JA. HLA tetramers and anti-CMV immune responses: from epitope to immunotherapy. Cytotherapy (2002) 4:41ā8. doi:10.1080/146532402317251518
85. Peggs KS, Verfuerth S, Pizzey A, Chow SL, Thomson K, Mackinnon S. Cytomegalovirus-specific T cell immunotherapy promotes restoration of durable functional antiviral immunity following allogeneic stem cell transplantation. Clin Infect Dis (2009) 49:1851ā60. doi:10.1086/648422
86. Szmania S, Galloway A, Bruorton M, Musk P, Aubert G, Arthur A, et al. Isolation and expansion of cytomegalovirus-specific cytotoxic T lymphocytes to clinical scale from a single blood draw using dendritic cells and HLA-tetramers. Blood (2001) 98:505ā12. doi:10.1182/blood.V98.3.505
87. Hobeika A, Osada T, Serra D, Peplinski S, Hanson K, Tanaka Y, et al. Detailed analysis of cytomegalovirus (CMV)-specific T cells expanded for adoptive immunotherapy of CMV infection following allogeneic stem cell transplantation for malignant disease. Cytotherapy (2008) 10:289ā302. doi:10.1080/14653240801927040
88. Klebanoff CA, Rosenberg SA, Restifo NP. Prospects for gene-engineered T cell immunotherapy for solid cancers. Nat Med (2016) 22:26ā36. doi:10.1038/nm.4015
89. Holler PD, Chlewicki LK, Kranz DM. TCRs with high affinity for foreign pMHC show self-reactivity. Nat Immunol (2003) 4:55ā62. doi:10.1038/ni863
90. Donermeyer DL, Weber KS, Kranz DM, Allen PM. The study of high-affinity TCRs reveals duality in T cell recognition of antigen: specificity and degeneracy. J Immunol (2006) 177:6911ā9. doi:10.4049/jimmunol.177.10.6911
Keywords: cytomegalovirus, cytomegalovirus-specific CD8+ cytotoxic T-lymphocytes, T-cell receptor affinity, T-cell, hematopoietic stem cell transplantation, programmed cell death-1, tetramers
Citation: Poiret T, Axelsson-Robertson R, Remberger M, Luo X-H, Rao M, Nagchowdhury A, Von Landenberg A, Ernberg I, Ringden O and Maeurer M (2018) Cytomegalovirus-Specific CD8+ T-Cells With Different T-Cell Receptor Affinities Segregate T-Cell Phenotypes and Correlate With Chronic Graft-Versus-Host Disease in Patients Post-Hematopoietic Stem Cell Transplantation. Front. Immunol. 9:760. doi: 10.3389/fimmu.2018.00760
Received: 25 October 2017; Accepted: 27 March 2018;
Published: 10 April 2018
Edited by:
Katharina Fleischhauer, UniversitƤtsklinikum Essen, GermanyReviewed by:
Mirko Trilling, University of Duisburg, GermanyCopyright: Ā© 2018 Poiret, Axelsson-Robertson, Remberger, Luo, Rao, Nagchowdhury, Von Landenberg, Ernberg, Ringden and Maeurer. This is an open-access article distributed under the terms of the Creative Commons Attribution License (CC BY). The use, distribution or reproduction in other forums is permitted, provided the original author(s) and the copyright owner are credited and that the original publication in this journal is cited, in accordance with accepted academic practice. No use, distribution or reproduction is permitted which does not comply with these terms.
*Correspondence: Thomas Poiret, thomas.poiret@ki.se
Disclaimer: All claims expressed in this article are solely those of the authors and do not necessarily represent those of their affiliated organizations, or those of the publisher, the editors and the reviewers. Any product that may be evaluated in this article or claim that may be made by its manufacturer is not guaranteed or endorsed by the publisher.
Research integrity at Frontiers
Learn more about the work of our research integrity team to safeguard the quality of each article we publish.