- 1Laboratório de Biologia das Interações Celulares, Departamento de Morfologia, Instituto de Ciências Biológicas, Universidade Federal de Minas Gerais, Belo Horizonte, Brazil
- 2Instituto René Rachou, Fundação Oswaldo Cruz - FIOCRUZ, Belo Horizonte, Brazil
- 3Instituto Nacional de Ciência e Tecnologia em Doenças Tropicais, INCT-DT, Salvador, Brazil
- 4NUPEB, Universidade Federal de Ouro Preto, Ouro Preto, Brazil
In the chronic phase of Chagas disease, 60% of the patients develop the asymptomatic form known as indeterminate (IND). The remaining 30% of the patients develop a life-threatening form in which digestive and/or cardiac (CARD) alterations take place. The mechanisms underlying the development of severe forms of Chagas disease remain poorly understood. It is well known that interactions between immune cells such as monocytes and lymphocytes drive immune responses. Further, the co-stimulatory molecules CD80 and CD86 expressed by monocytes and subsets induce lymphocyte activation, thereby triggering cellular immune response. Here, we revealed, for the first time, the functional-phenotypic profile of monocytes subsets in Chagas disease. Using flow cytometry, we evaluated the effect of in vitro stimulation with Trypanosoma cruzi antigens on the expression of the co-stimulatory molecules CD80 and CD86 in different monocyte subsets of patients with IND and CARD clinical forms of Chagas disease. We also assessed the expression of toll-like receptor (TLR)-2, TLR-4, TLR-9, HLA-DR, IL-10, and IL-12 in the monocyte subsets and of CTLA-4 and CD28, ligands of CD80 and CD86, in T lymphocytes. CD86 expression in all monocyte subsets was higher in IND patients when compared with non-infected (NI) individuals. After stimulation with T. cruzi, these patients also showed a higher frequency of CD4+CTLA-4+ T lymphocytes than NI individuals. We found an association between CD80 and CD28, and between CD86 and CTLA-4 expression, with a high frequency of regulatory T (Treg) cells in IND patients. We proposed that CD86 may be involved in immunoregulation by its association with CTLA-4 in asymptomatic patients. CD86 and CTLA-4 interaction may influence Treg activation, and this could represent a new strategy to control inflammation and tissue damage.
Introduction
Infection with the etiologic agent of Chagas disease, the protozoan Trypanosoma cruzi, leads to the accumulation of parasites in the blood stream and several tissues, thus characterizing the acute phase of the disease (1–4). Afterward, the host’s immune system attempts to control the parasite replication but, as it cannot completely do so, the result is two chronic clinical outcomes (5–7). The main outcome, affecting around 60% of the patients is a life-long asymptomatic clinical form known as indeterminate (IND) (8–11). The remaining 30% of the patients, many of whom were once asymptomatic, develop severe, life-threatening forms of the disease, in which digestive and/or cardiac (CARD) alterations take place (9, 12, 13). The CARD clinical form is particularly important as it represents the clinical form associated with greater morbidity and mortality.
The mechanisms involved in the development of severe forms of Chagas disease are still poorly understood. Research conducted by our team and other laboratories revealed that important factors in the disease progression include host genetics, parasite characteristics, and host immune response (3, 12, 14, 15).
Monocytes/macrophages are innate immune cells that recognize T. cruzi antigens and activate lymphocytes and the immune response system (7, 16). Monocyte subsets display distinct functions and, despite their importance in regulating the immune response (17), their role in Chagas disease has not yet been determined. Moreover, the studies published so far have focused on the specific roles of monocytes and lymphocytes on the immune response induced by T. cruzi and did not explore the relationship between them in the development of the clinical forms of Chagas disease.
The co-stimulatory molecules CD80 and CD86 expressed on monocytes are essential for activating lymphocytes and thus adaptive immunity (18, 19). These cells constitute a heterogeneous population presenting distinct subsets according to the expression of the surface molecules CD14 and CD16 (20–22). Classical monocytes (CD14++CD16−) show high-phagocytosis capacity, have a pro-inflammatory profile and, under normal conditions, correspond to approximately 85–90% of the monocytes (22–25). Non-classical monocytes (CD14+CD16++) show an anti-inflammatory profile and a patrol behavior in vivo (22, 25). Molecular studies showed that intermediate monocytes (CD14++CD16+) are between the classical and non-classical monocyte subsets and express the highest levels of the major histocompatibility complex class II (26). However, there are no studies regarding the functional-phenotypic profile of monocyte subsets in Chagas disease.
The two main receptors present on the surface of T lymphocytes that bind CD80 and CD86 are CD28 and cytotoxic T-lymphocyte associated protein 4 (CTLA-4). Binding to CD28 activates lymphocytes and consequently enhances the immune response, whereas binding to CTLA-4 inhibits activation of lymphocytes, thereby downregulating immunity (19, 27–29). T lymphocytes play a crucial role in the establishment and development of human Chagas disease, displaying both immunoregulatory and effector functions. These functions determine the disease dynamics and are involved in the polarity found in the clinical forms of the disease (30–34).
Previous studies evaluated the expression of CD80 and CD86 co-stimulatory molecules by monocytes of Chagas patients (35). However, no study to date explored the effect of the expression of these molecules by classical (CD14++CD16−), intermediate (CD14++CD16+), and non-classical (CD14+CD16++) monocyte subsets on the activation of T helper (Th) type 1 (Th1), type 2 (Th2), type 17 (Th17), and regulatory T (Treg) cells. We addressed this knowledge gap by profiling the expression of CD80 and CD86 by monocyte subsets of IND and CARD individuals in the activation of Th cells following the challenge by T. cruzi antigens. Ultimately, we aimed to characterize the functional-phenotypic profile of monocyte subsets and understand the CD80/CD86 co-stimulatory expression by monocyte subsets in the protective and/or regulatory immunity against T. cruzi infection to further understand Chagas disease progression.
Materials and Methods
Study Population
Nineteen patients who agreed to participate in this study were selected at René Rachou Institute ambulatory, Oswaldo Cruz Foundation (FIOCRUZ), Belo Horizonte, Minas Gerais, Brazil. Patients were considered infected when they tested positive for Chagas disease by at least two different serological tests (indirect immunofluorescence, ELISA, or indirect hemagglutination).
The patients infected with T. cruzi were grouped as IND and CARD. The IND (n = 10) group included individuals tested positive for Chagas disease albeit with no significant alterations in electrocardiography, chest X-ray, echocardiogram, esophagogram, and barium enema. The CARD (n = 9) group presented dilated cardiomyopathy, characterized by the echocardiographic finding of a dilated left ventricle with impaired ventricular systolic function, which were classified as belonging to the group CARD V, as previously reported (36). Left ventricular end-diastolic diameter/body surface area ≥31 mm (64.8 ± 5.9 mm) and left ventricular ejection fraction <55% (34 ± 10%) were used as echocardiographic parameters of Chagas dilated cardiomyopathy. Normal healthy individuals that tested negative for the infection were included as a control group [non-infected (NI), n = 6]. All patients included in this study were between 30 and 75 years of age.
Ethics Statement
This study was approved by the Ethics Committee of the René Rachou Institute, FIOCRUZ (15/2011). All enrolled patients signed an informed consent form prior to the inclusion in the study.
T. cruzi Soluble Antigen Preparations (TRIPO)
Trypomastigote forms were obtained from culture of LLC cells maintained in RPMI-1640 medium (Gibco, Thermo Fisher Scientific, USA) supplemented with 10% fetal bovine serum, as previously described (33). Parasites were subjected to rupture and homogenization in cold phosphate-buffered saline (PBS, Sigma, USA), using a glass homogenizer and Teflon pestle, on ice to prevent overheating. Subsequently, the suspensions were centrifuged at 23.000 g for 60 min at 4°C. The supernatant was collected, dialyzed for 24 h at 4°C against PBS, and sterilized by filtration on 0.2 μm-pore-size membranes. The protein concentration was measured by Nanodrop (Thermo Scientific, USA) and the material was separated into aliquots and stored at −70°C until use.
Whole Blood Cultures
Whole blood collected in sodic heparin was cultured for 18 h at 37°C and 5% CO2 with medium alone (RPMI-1640 supplemented with 1.6% l-glutamine, 3% antibiotic–antimycotic, 5% of AB Rh-positive heat-inactivated normal human serum) or medium plus 20 µL/mL of soluble T. cruzi antigens (TRIPO). Previously, 20 µL of Brefeldin A (Sigma, USA) at 1 mg/mL concentration was added to the tubes and they were incubated for another 4 h at 37°C and 5% CO2. Subsequently, the culture tubes were incubated for 15 min after addition of 200 µL of EDTA (Sigma, USA) to reach the concentration of 2 mM. Then, 3 mL of PBS-W (PBS pH 7.4, containing 0.5% BSA, and 0.1% sodium azide) were added to the tubes and the samples were centrifuged at 400 g for 10 min at 18°C. The supernatant was aspirated leaving a final volume of 2.5 mL. Subsequently, 200 µL of this blood was added in polystyrene tubes (Falcon, USA) with corresponding surface antibodies and was incubated for 30 min. After the incubation, the samples were lysed and fixed in 2 mL of FACS Lysing Solution (BD, USA) for 10 min at room temperature. The samples were washed in PBS-W and subsequently, 2.5 mL of PBS-P (PBS, pH 7.4 containing 0.5% BSA, 0.1% sodium azide, and 0.5% saponin) were added followed by an incubation of 30 min. To detect intracellular markers, 2 µL of anti-cytokine antibody was added to each tube and incubated for 1 h at room temperature. Then, the cells were washed with 1 mL PBS-W and centrifuged at 400 g for 10 min at 18°C. At the end, 200 µL of fixative solution (Sigma, USA) were added in the tubes.
Samples containing the cell suspension were used to acquire data on a flow cytometer (FACS LSR Fortessa, BD, USA). A total of 60,000 events inside the lymphocyte population were analyzed using size (FSC) and granularity (SSC) parameters. Antibodies were conjugated to FITC, PERCP (or PE-Cy5 or PERCP-Cy5.5), antigen-presenting cell (APC), APCCy7, PE-Cy7, and BV421. The analyzed molecules were: CD4 (RPA-T4), CD28 (CD28.2), CTLA-4 (BNI3), CD14 (MφP9), CD16 (3G8), CD80 (2D10), CD86 (2331), Tbet (4B10), GATA-3 (L50-823), RORγT (Q21-559), CD25 (M-A251), FOXP3 (PCH101), IFN-γ (25723.11), IL-4 (8D4-8), IL-10 (JES3-19F1), IL-17 (eBio64DEC17), toll-like receptor (TLR)-2 (TL2.1), TLR-4 (HTA125), TLR-9 (eB72-1665), HLA-DR (G46-6), and IL-12 (C11.5).
Statistical Analysis
To verify statistical differences between control and TRIPO cultures, a paired analysis was performed employing the Wilcoxon test, followed by Rank through the statistical program GraphPad Prism version 5.0 software (San Diego, CA, USA). Differences between the clinical forms in the control and TRIPO cultures were determined with the Kruskal–Wallis test.
The association between the monocytes and lymphocytes subsets was determined from the linear regression, considering the coefficient of determination (R2) for the quality of fit and the F test to measure the variance between pairs (p < 0.05). Correlation analysis was done using the Spearman’s (ρ) coefficient contained in the JMP software version 5.0. The defined confidence interval was 95% and significant statistical differences were considered when p < 0.05.
Results
Chagas Patients Show Different Frequencies of Monocyte Subsets in Control and T. cruzi-Stimulated Cultures
The frequency of total monocytes and monocyte subsets obtained from Chagas disease patients and healthy individuals is presented in Figures 1A,B. Our results showed a significant reduction in the frequency of total monocytes in the CARD group after in vitro stimulation with T. cruzi antigens (TRIPO culture) when compared with the control culture. We observed a higher frequency of intermediate monocytes in the CARD group when compared with the NI group in control culture. Interestingly, there was a reduction in the frequency of this monocyte subset in the CARD group challenged with T. cruzi in comparison with CARD group cultured in control medium. Other significant differences were not observed.
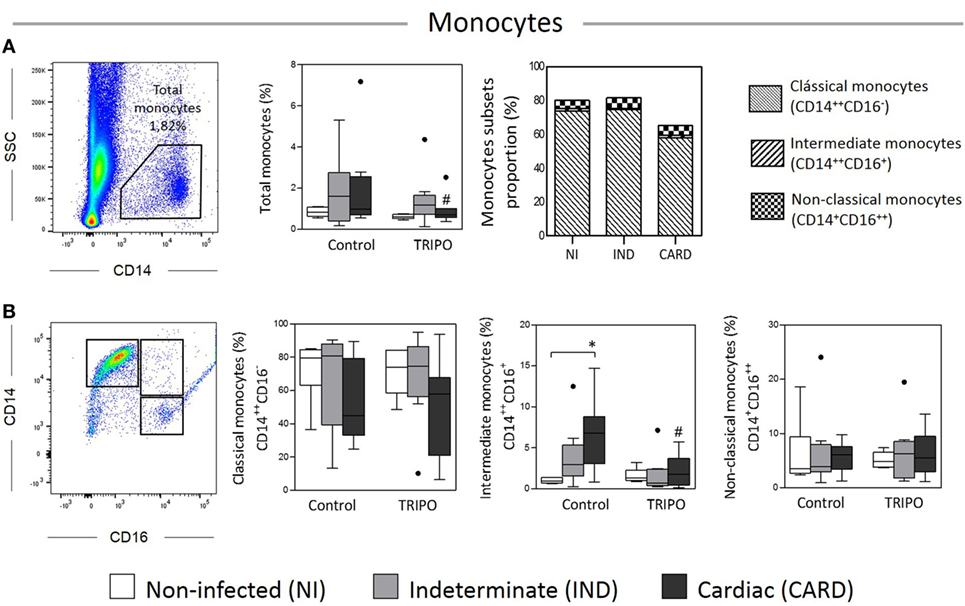
Figure 1. Analysis of total monocytes and subsets. Flow cytometry gate strategy of non-infected (NI) individuals and frequency of total monocytes (A), classical (CD14++CD16−), intermediate (CD14++CD16+), and non-classical (CD14+CD16++) subsets in culture without stimulation (control) and after in vitro stimulation with T. cruzi antigens (TRIPO) (B). The groups evaluated were NI individuals (n = 6), indeterminate (IND, n = 10), and cardiac (CARD, n = 9) clinical forms of Chagas disease. Significant differences (p < 0.05) between groups are evidenced by lines and asterisks (*) according Kruskal–Wallis test, followed by Dunn’s post hoc test. The # sign indicates significant difference between control and TRIPO cultures using Wilcoxon signed-rank test. Boxes show the median and interquartile ranges, whiskers indicate the highest and lowest observation, and dots represent the outliers.
When we analyzed the proportions of the monocyte subsets of Chagas disease patients and healthy individuals in TRIPO cultures, we found that the NI, IND, and CARD groups presented a higher proportion of classical than intermediate and non-classical monocytes. We also observed a small increase in the proportion of non-classical monocytes in the IND group when compared with the individuals of the NI and CARD groups. A higher proportion of intermediate monocytes were found in the CARD group in comparison with NI and IND groups.
Lower Expression of TLR in the Monocytes of Chagas Disease Patients
Toll-like receptors are crucial to recognize structures conserved in microorganisms such as PAMPs (37). In Chagas disease, TLR-2 is associated with the initiation of the immune response by recognizing GPI anchor-linked mucin molecules (38), TLR-4 with the recognition of glycoinositolphospholipid in the parasite cell surface (39), and TLR-9 receptors with the identification of unmethylated CpG motifs in the T. cruzi genome (38, 40). Thus, TLRs are of great importance for parasite recognition and to drive the production of cytokines in response to the microorganism (41).
Stimulation with T. cruzi led to an increased expression of TLR-2 on intermediate monocytes of the NI group and a reduced expression of this receptor on the same monocyte subset of the CARD group, when compared with control cultures. Challenge with T. cruzi also led to lower expression of TLR-4 on non-classical monocytes of the IND patients when compared with the NI group. Additionally, after in vitro stimulation with T. cruzi antigens, total monocytes of IND individuals showed high-expression TLR-9. In contrast, we observed a lower expression of this receptor on intermediate and non-classical monocytes of the IND group when compared with NI individuals, in both control and TRIPO cultures (Figure 2A). Other significant differences were not observed.
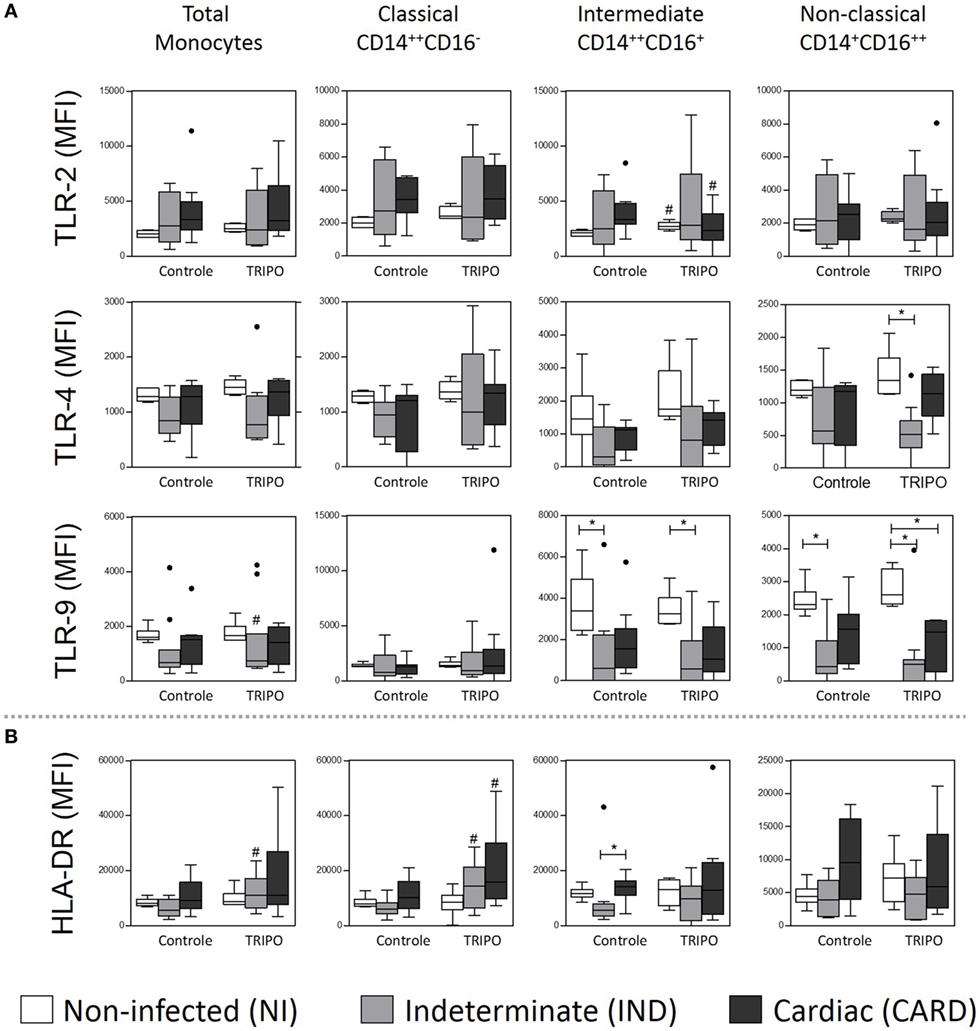
Figure 2. Expression of the recognition and activation molecules of total monocytes and subsets. Expression of toll-like receptors (TLR) (A), and HLA-DR (B) by total monocytes, classical (CD14++CD16), intermediate (CD14++CD16+), and non-classical (CD14+CD16++) monocytes subsets in cultures without stimulation (control) and after in vitro stimulation with T. cruzi antigens (TRIPO). The groups evaluated were non-infected (NI) individuals (n = 6), indeterminate (IND, n = 10), and cardiac (CARD, n = 9) clinical forms of Chagas disease. Significant differences (p < 0.05) between groups are evidenced by lines and asterisks (*) according Kruskal–Wallis test, followed by Dunn’s post hoc test. The # sign indicates significant difference between control and TRIPO cultures using Wilcoxon signed-rank test. Boxes show the median and interquartile ranges, whiskers indicate the highest and lowest observation, and dots represent the outliers. MFI, mean fluorescence intensity.
Monocyte Subsets of Patients with Chagas Disease Have Different HLA-DR Expression Levels
After in vitro stimulation with T. cruzi antigens, total monocytes of IND patients presented higher expression of HLA-DR when compared with the control culture. Classical monocytes of IND and CARD groups in TRIPO culture presented a higher expression of this molecule, in comparison with control cultures. The results also showed lower expression of HLA-DR on intermediate monocytes of IND patients when compared with the CARD group in control cultures (Figure 2B).
In Vitro Stimulation of Monocytes Subsets with T. cruzi Upregulates the Expression of CD80 and CD86 in Chagasic Patients
Next, we evaluated the effect of circulating monocytes of chronic Chagasic patients on the activation of T cells. To this end, we characterized the expression of the co-stimulatory molecules CD80 and CD86 on short-term in vitro cultures of whole blood samples in the presence of T. cruzi antigens (Figures 3A,B).
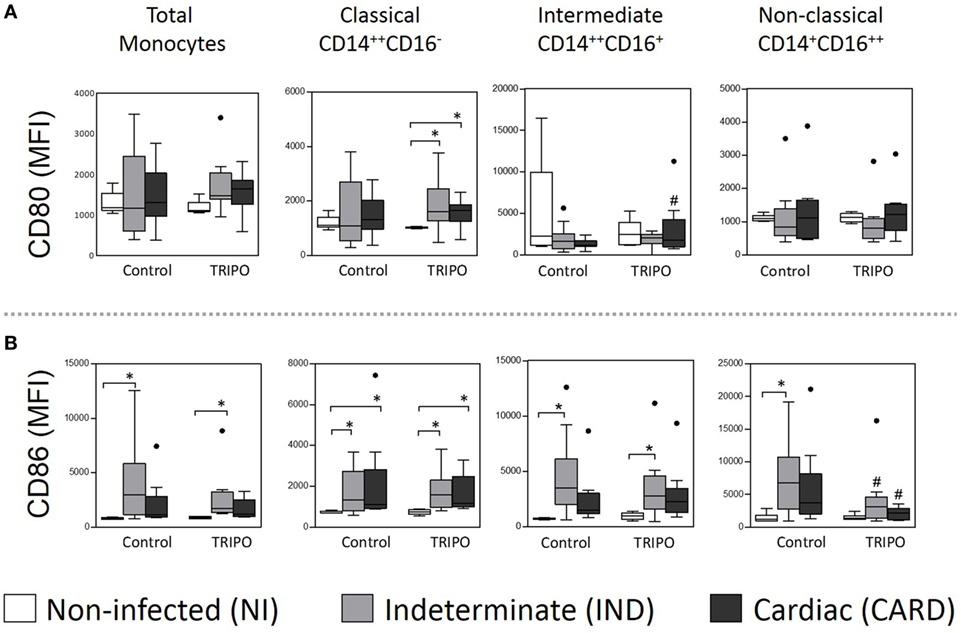
Figure 3. Expression of the co-stimulatory molecules of total monocytes and subsets. Expression of CD80 (A), and CD86 (B) co-stimulatory molecules by total monocytes, classical (CD14++CD16), intermediate (CD14++CD16+), and non-classical (CD14+CD16++) monocytes subsets in cultures without stimulation (control) and after in vitro stimulation with T. cruzi antigens (TRIPO). The groups evaluated were non-infected (NI) individuals (n = 6), indeterminate (IND, n = 10), and cardiac (CARD, n = 9) clinical forms of Chagas disease. Significant differences (p < 0.05) between groups are evidenced by lines and asterisks (*) according Kruskal–Wallis test, followed by Dunn’s post hoc test. The # sign indicates significant difference between control and TRIPO cultures using Wilcoxon signed-rank test. Boxes show the median and interquartile ranges, whiskers indicate the highest and lowest observation, and dots represent the outliers. MFI, mean fluorescence intensity.
In TRIPO stimulated cultures, the expression of CD80 on the classical monocytes of IND and CARD groups were higher than in the NI group. This co-stimulatory molecule was also highly expressed on intermediate monocytes of the CARD group challenged with T. cruzi antigens, when compared with the control culture. Significant differences were not observed in the expression of CD80 on non-classical monocytes (Figure 3A). Total monocytes and all the subsets of IND patients presented higher expression of CD86 when compared with NI group in control and TRIPO cultures, except in the case of non-classical monocytes after in vitro stimulation with T. cruzi antigens. CARD patients showed high-CD86 expression only on classical monocytes when compared with NI group in both control and TRIPO cultures. We also observed a reduced expression of this molecule on non-classical monocytes of IND and CARD groups after T. cruzi stimulation (Figure 3B). Other significant differences were not observed.
To compare the expression of CD86 and CD80 on monocytes—total and subsets—we calculated a relative expression index, considering the CD86/CD80 ratio and the proportion through the mean intensity fluorescence of these molecules in TRIPO cultures of NI, IND, and CARD individuals (Figures 4A,B). Our results showed higher CD86/CD80 expression ratio on total monocytes of IND compared with NI group. The highest CD80 expression was observed on the intermediate monocytes of the NI group. As the disease progresses, CD80 expression decreased on the intermediate and non-classical subsets and increased on the classical subset of the IND group. Further, in the CARD group, CD80 expression shifted from intermediate to non-classical monocytes while the expression observed on the classical monocyte subset remained stable. We found a similar proportion of CD86 expression on classical, intermediate, and non-classical monocytes of the NI group. As the disease progresses, CD86 expression increased on all monocyte subsets of IND patients in comparison with those of the NI group, and decreased on the subsets of the CARD group in comparison with the IND group, albeit CD86 expression remained higher than that observed in the NI group (Figure 4).
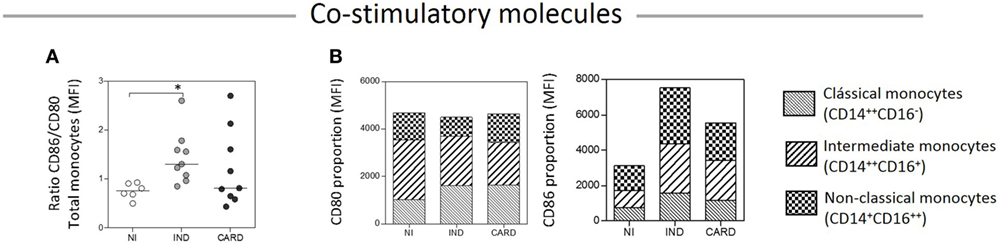
Figure 4. Ratio/proportion of CD80 and CD86 expression by monocytes. Ratio of CD86/CD80 expression by total monocytes (A), and proportion of these co-stimulatory molecules by the monocyte subsets (B). The groups evaluated were non-infected (NI) individuals (n = 6), indeterminate (IND, n = 10), and cardiac (CARD, n = 9) clinical forms of Chagas disease. Significant differences (p < 0.05) between groups are evidenced by lines and asterisks (*) according Kruskal–Wallis test, followed by Dunn’s post hoc test. MFI, mean fluorescence intensity.
Asymptomatic Patients with Chagas Disease Showed a Balance between IL-12 and IL-10 Expression
In Chagas disease, while the cytokine IL-12 leads to the activation of a Th1-lymphocyte-mediated response, the cytokine IL-10 contributes to the inhibition of IL-12 production by monocytes, lymphocytes, and dendritic cells, leading to regulation of immune response (32).
We found that the total monocytes of patients with Chagas disease present a higher expression of IL-12 when compared with the NI group, in both control and TRIPO cultures. After in vitro stimulation with T. cruzi antigens, total monocytes of the CARD group showed a lower expression of this cytokine (Figure 5A).
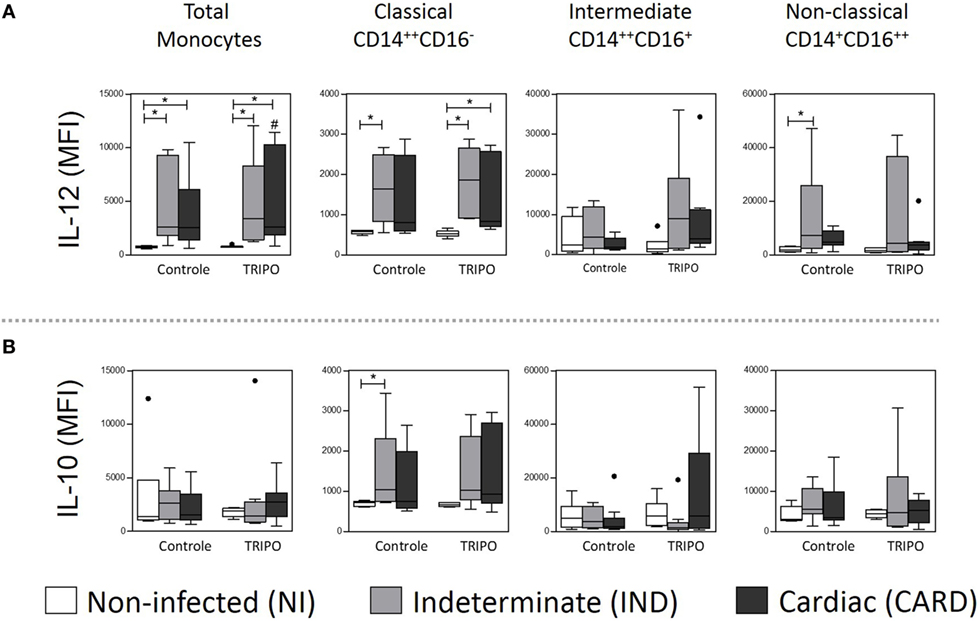
Figure 5. Expression of cytokines of total monocytes and subsets. Expression of IL-10 (A), and IL-12 (B) by total monocytes, classical (CD14++CD16), intermediate (CD14++CD16+), and non-classical (CD14+CD16++) monocytes subsets in cultures without stimulation (control) and after in vitro stimulation with T. cruzi antigens (TRIPO). The groups evaluated were non-infected (NI) individuals (n = 6), indeterminate (IND, n = 10), and cardiac (CARD, n = 9) clinical forms of Chagas disease. Significant differences (p < 0.05) between groups are evidenced by lines and asterisks (*) according Kruskal–Wallis test, followed by Dunn’s post hoc test. The # sign indicates significant difference between control and TRIPO cultures using Wilcoxon signed-rank test. Boxes show the median and interquartile ranges, whiskers indicate the highest and lowest observation, and dots represent the outliers. MFI, mean fluorescence intensity.
When we analyzed the monocyte subsets, we observed a higher expression of IL-12 on classical monocytes of IND patients when compared with the NI group in control culture and, after in vitro stimulation with T. cruzi antigens, in IND and CARD patients in comparison with the NI group. We also detected higher expression of IL-12 on non-classical monocytes of IND patients when compared with the NI group in control culture (Figure 5A). In addition, classical monocytes showed higher expression of IL-10 only in IND patients, when compared with NI group in control culture (Figure 5B). Other significant differences were not observed.
CTLA-4 Molecule Is Upregulated in CD4+ T Lymphocytes in the IND Clinical Form of Chagas Disease
CD25, CD28, and CTLA-4 are important activation molecules present on the surface of T lymphocytes that are essential to the adaptive immune response (19, 27, 42). We evaluated the expression of these molecules in CD4+ T cells of NI, IND, and CARD individuals. We found a higher frequency of CD4+ CD25+ T lymphocytes in TRIPO cultures of IND and CARD patients in comparison with NI group and in comparison with the frequency observed in the control cultures. We also detected a slight but significant increase of CD4+CD28+ T lymphocytes frequency in the TRIPO culture compared with control cultures of IND patients. When we evaluated the frequency of CD4+ CTLA-4+ T lymphocytes, we observed that, after stimulation with T. cruzi antigens, IND and, CARD patients showed an increased frequency of these cells in comparison with control culture. Interestingly only IND patients showed a higher frequency of CD4+ CTLA-4+ T lymphocytes when compared with NI group in TRIPO cultures (Figure 6A). Other significant differences were not observed.
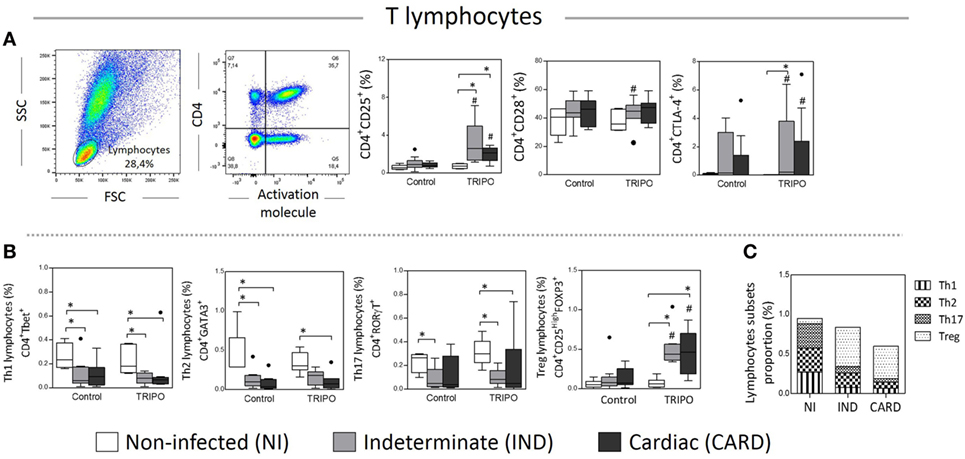
Figure 6. Analysis of lymphocytes and subsets. Flow cytometry gate strategy of non-infected (NI) individuals is represented. Frequency of CD4+ lymphocytes expressing CD25, CD28, and CTLA-4 (A) in culture without stimulation (control) and after in vitro stimulation with T. cruzi antigens (TRIPO). Frequency of Th1, Th2, Th17, and T regulatory (Treg) lymphocytes subsets (B) and, proportion of lymphocyte subsets (C). The groups evaluated were NI individuals (n = 6), indeterminate (IND, n = 10), and cardiac (CARD, n = 9) clinical forms of Chagas disease. Significant differences (p < 0.05) between groups are evidenced by lines and asterisks (*) according Kruskal–Wallis test, followed by Dunn’s post hoc test. The # sign indicates the significant difference between control and TRIPO cultures using Wilcoxon signed-rank test. Boxes show the median and interquartile ranges, whiskers indicate the highest and lowest observation, and dots represent the outliers.
Chagasic Patients Decrease Th1, Th2, and Th17 and Increase of Treg Cells after Stimulation with T. cruzi Antigens
The immune response mediated by CD4+ T lymphocytes is highly important for the development of Chagas disease involving the Th1, Th2, Th17, or Treg CD4+ lymphocytes that differ in their functions, secreted cytokine pattern, and specific expression of transcription factors (16, 43). Therefore, we evaluated the frequency of Th1, Th2, Th17, and Treg lymphocytes in the peripheral blood following in vitro stimulation with T. cruzi antigens (Figure 6B). The data show a general decrease in the frequency of Th1, Th2, and Th17 lymphocytes of Chagasic patients in comparison with NI individuals, in the control, and TRIPO cultures. In contrast, the frequency of Treg lymphocytes was significantly higher in IND and CARD groups compared with NI individuals after in vitro stimulation with T. cruzi antigens and in comparison, to the frequency observed in the control cultures (Figure 6B). Other significant differences were not observed.
When we analyzed the proportions of lymphocyte subsets in Chagas disease patients and healthy individuals in TRIPO cultures, we found that NI group presented similar proportions of Th1, Th2, and Th17 lymphocytes and a small proportion of Treg cells. As the disease progresses, the proportion of Th1, Th2, and Th17 cells diminishes in IND and CARD individuals. In contrast, the proportion of Treg cells increases dramatically in Chagasic patients compared with NI group, mainly in IND group (Figure 6C).
The expression of IFN-γ, IL-4, IL-17, and IL-10 by CD4+ T lymphocytes is a key factor for the differentiation and characterization of Th1, Th2, Th17, and Treg subsets, respectively (16). We found a lower frequency of Th2 IL-4+ lymphocytes in control cultures of IND and CARD patients in comparison with NI group. We also detected a lower frequency of Th17 IL-17+ lymphocytes in control and TRIPO cultures of CARD and IND individuals when compared with NI group, respectively. No significant differences were observed regarding Th1 IFN-γ+ lymphocytes and Treg IL-10+ lymphocytes (Figure 7).
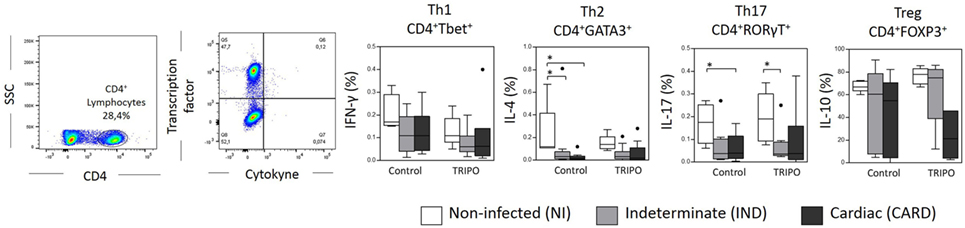
Figure 7. Frequency of cytokines+ lymphocytes subsets. Flow cytometry gate strategy of non-infected (NI) individuals is represented. Expression of IFN-γ, IL-4, IL-17, and IL-10 by CD4+ T lymphocytes that characterize Th1, Th2, Th17, and regulatory T subsets, respectively. The groups evaluated were NI individuals (n = 6), indeterminate (IND, n = 10), and cardiac (CARD, n = 9) clinical forms of Chagas disease. Significant differences (p < 0.05) between groups are evidenced by lines and asterisks (*) according Kruskal–Wallis test, followed by Dunn’s post hoc test. Boxes show the median and interquartile ranges, whiskers indicate the highest and lowest observation, and dots represent the outliers.
CD86 Co-Stimulatory Molecule May Induce an Immunomodulatory Treg Response in IND Patients
As detailed above, patients with the IND clinical form of Chagas disease show a higher expression of CD86 than CD80 co-stimulatory molecules by all monocyte subsets. The interaction between CD80 or CD86 with CD28 activates lymphocytes and enhances the immune response, whereas their binding to CTLA-4 inhibits the activation of lymphocytes activation thus downmodulating the immune response (35). We investigated this interaction by performing regression and correlation analyzes.
First, we evaluated the interaction between CD80/CD86 of total monocytes and CD28/CTLA-4 in TRIPO cultures of NI, IND, and CARD individuals and found a significant association between CD80+ and CD4+ CD28+ T cells of the NI group (R2 = 0.73/p = 0.03). We also detected, in these individuals, a significant positive correlation between CD80+ and CTLA-4+ T cells (ρ = 0.93/p = 0.008). A significant association between CD86+ and CTLA-4+ T cells was found in CARD patients (R2 = 0.81/p = 0.001) (Table 1).
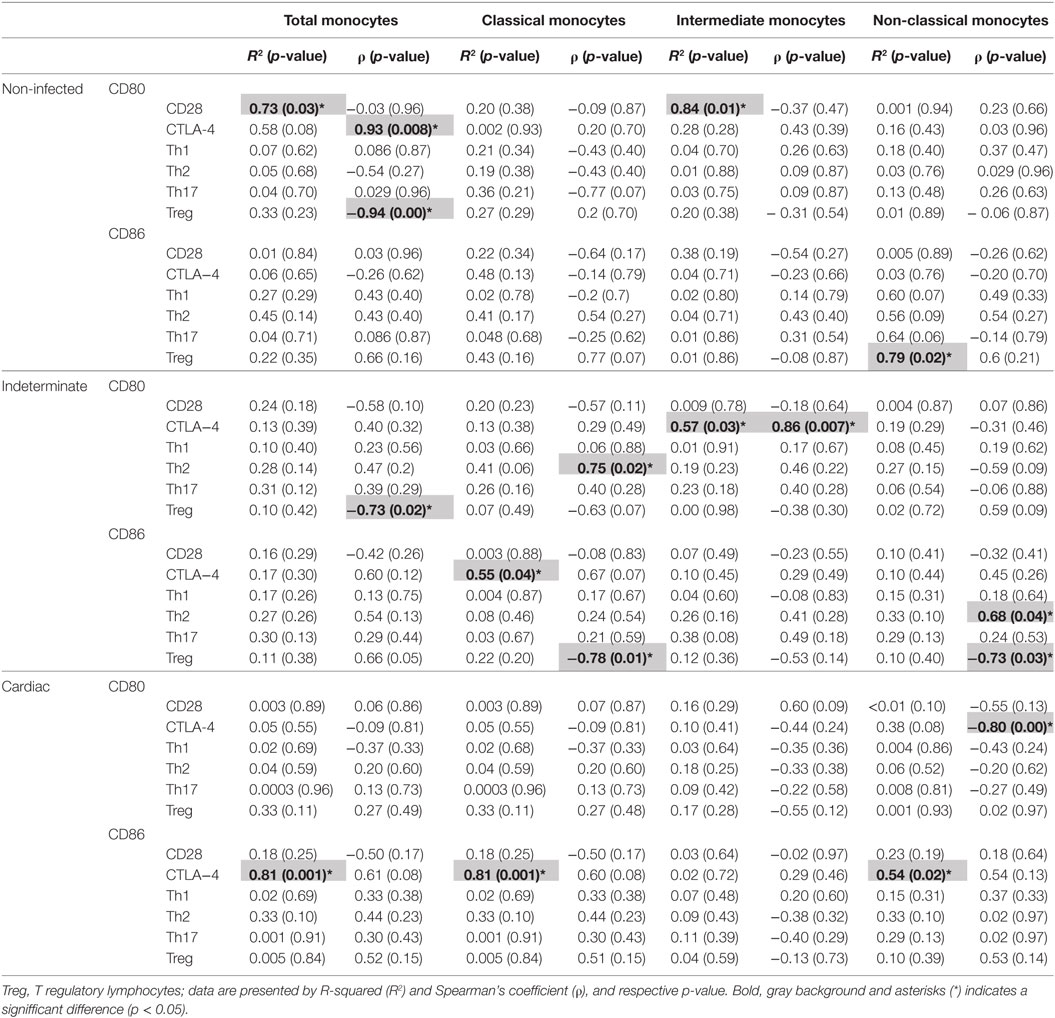
Table 1. Linear regression and correlation analysis of CD80 and CD86 co-stimulatory molecules by monocytes and their subsets with CD28 and CTLA-4 molecules and Th1 Th2, Th17, and regulatory T (Treg) cells.
Analysis of the interaction between CD80 and CD86 of monocyte subsets in TRIPO cultures of NI, IND, and CARD patients revealed a significant association between CD80+ intermediate monocytes and CD28+ T cells in NI individuals (R2 = 0.84/p = 0.01). We observed a significant association between CD86+ classical monocytes and CTLA-4+ T cells in IND (R2 = 0.55/p = 0.04) and CARD (R2 = 0.81/p = 0.001) patients. We also found a significant association (R2 = 0.57/p = 0.03) and positive correlation (ρ = 0.86/p = 0.007) between CD80+ intermediate monocytes and CTLA-4+ T cells of IND patients. In addition, there was a negative correlation between CD80+ non-classical monocytes and CTLA-4+ T cells in CARD patients (ρ = −0.80/p = 0.00). A significant association between CD86+ non-classical monocytes and CTLA-4+ T cells was observed in CARD patients (R2 = 0.54/p = 0.02) (Table 1).
Next, we used both linear regression and correlation analyzes to explore the relationship between the expression of CD80 and CD86 by total monocytes and their subsets in driving a Th1, Th2, Th17, and Treg response in TRIPO cultures of NI, IND, and CARD individuals. A negative correlation between CD80+ with Treg cells was found in total monocytes of NI (ρ = −0.94/p = 0.00) and IND (ρ = −0.73/p = 0.02) patients (Table 1).
Analysis of the interaction between CD80 and CD86 in the monocyte subsets of TRIPO cultures of NI, IND, and CARD patients revealed a significant association between CD86+ non-classical monocytes and Treg cells of NI individuals (R2 = 0.79/p = 0.02). In addition, we observed a negative correlation between CD86+ classical monocytes (ρ = −0.78/p = 0.01) and CD86+ non-classical monocytes (ρ = −0.73/p = 0.02) with Treg cells of IND individuals. We also found a positive correlation between CD80 and CD86 with Th2 in classical (ρ = 0.75/p = 0.02) and non-classical monocytes (ρ = 0.68/p = 0.04) of IND patients, respectively (Table 1).
Discussion
Monocytes are important cell of the innate immune system by their capacity to process and present antigens, produce cytokines, express co-stimulatory molecules, and activate the subsequent adaptive immune response (35). However, little is known about monocytes subsets in Chagas disease and the influence of CD80 and CD86 co-stimulatory expression in the modulation of the immune response in Chagas patients. Herein, we characterized the functional-phenotypic profile of total monocytes and subsets and investigated the effect of CD80 and CD86 expression by monocytes in the activation of Th subsets. We observed a reduced frequency of total monocytes of CARD patients, but not in IND group. In addition, a reduction in the frequency of the intermediate monocytes was also observed in CARD group. It has been proposed that the intermediate monocytes are a transitional population bridging between the classical and non-classical subsets (26). We propose that, in the presence of the T. cruzi antigen, these cells may differentiate from classic and/or non-classic monocytes, being important to modulate the immune response. Previously, studies have reported that monocytes could be involved in the downregulation of the immune response by the production of the anti-inflammatory cytokine IL-10 in Chagas disease (17, 32). Thus, we suggest that T. cruzi may decrease the frequency of monocytes of CARD patients, mainly of intermediate monocytes, thereby contributing to a pro-inflammatory environment that may be involved in the tissue damage observed in these patients.
We demonstrated that the expression of molecules associated with antigen recognition was reduced in Chagas patients, mainly the TLR-4 and TLR-9 expression in asymptomatic group. TLR-9 activation plays a primary role in MyD88 induction that is dependent on the synthesis of IL-12 and IFN-γ during T. cruzi experimental infection and contributes to the formation of a pro-inflammatory environment (44). The downregulation of these receptors in monocytes could be an important event in directing a reduction in phagocytic capacity of these cells as well may be a mechanism developed by asymptomatic patients to control exacerbated inflammation and downregulate the immune response. Furthermore, TLR-2 and TLR-4 expression are associated with the production of pro-inflammatory cytokines in Chagas’ cardiomyopathy, while in asymptomatic patients these receptors as predominantly associated with IL-10 and TGF-β production (41). In agreement, we observed that Chagas patients showed higher expression of IL-12, and only IND patients demonstrated increased of the IL-10 production. These results suggest that monocytes of IND and CARD patients induce an inflammatory response by producing pro-inflammatory cytokines, such as IL-12, that trigger a Th1 lymphocyte-mediate response to control the infection. However, once again, only asymptomatic patients appear to regulate the inflammatory response by the production of the anti-inflammatory cytokine IL-10, thus averting the progression of inflammation.
We also demonstrated an increase of HLA-DR expression on the classical monocytes of Chagas disease patients. In the other hand, lower expression of this antigen presentation molecule was detected in intermediate monocytes only of IND individuals. The main characteristics of classical monocytes are their high-phagocytic capacity and pro-inflammatory profile (22, 25). Our group was demonstrated that impaired phagocytic capacity driven by downregulation of HLA-DR in monocytes supports the occurrence of particularities in the APC-activation-arm in IND (12). We suggest that HLA-DR expression by classical monocytes of Chagas patients may be associated with the increase of phagocytosis ability and antigenic presentation of these cells to control the infection, as well to activate the T cell-mediated immune response. In contrast, the lower expression of HLA-DR in intermediate monocytes from asymptomatic patients may be a way of reducing inflammation and avoiding tissue damage.
CD80 and CD86 co-stimulatory molecules in monocytes from patients with Chagas disease following induction by T. cruzi recombinant antigens are important for the activation of T cells (43). However, no study to date has demonstrated the expression of these molecules in the different monocytes subsets in Chagas disease. We observed that Chagas patients had increased CD80 expression by classical monocytes. On the other hand, only the IND group showed high-CD86 expression by total monocytes and all subsets. Moreover, CD86 expression in these cells of asymptomatic patients was higher than CD80 expression. We propose that CD80 expression may be involved in the activation of T cells in Chagas patients, while CD86 might be associated with the inhibition of them, downmodulating the responses of effector T cells in asymptomatic patients. Previous studies have shown that exposure of monocytes to T. cruzi leads to an increase in the frequency of CD80 by monocytes of IND and CARD patients (43), whereas CD86 expression is decreased in CARD but not in IND patients (35). We suggest that these co-stimulatory molecules have opposing functions and probably are a key role in the T cells activation in Chagas disease.
To confirm the effect of the co-stimulatory molecules CD80 and CD86 on the activation or inhibition of T lymphocytes, we evaluated the relationship between these molecules and their ligands. CD4+ CTLA-4+ T lymphocytes were increased in Chagas patients, mainly in IND group. We also observed an association between CD80 and CD28, and between CD86 and CTLA-4. It is possible that, after T. cruzi infection, CD80 binds to CD28 and promotes the activation of CD4+ T lymphocytes and triggering an inflammatory response. On the other hand, the association of CD86 and CTLA-4 in asymptomatic patients suggests the inhibition of T cells and downmodulation of the immune response. Corroborating with our results, Souza et al. showed a higher frequency of this inhibitory molecule in IND but not in CARD patients (35). CTLA-4 also implicated in the modulation of the immune response against T. cruzi by affecting the mechanisms that control IFN-γ and nitric oxide production during the acute phase of Chagas disease (45). We propose that the interaction of CD86 with CTLA-4 in asymptomatic patients may be a mechanism of immunoregulation to prevent tissue damage and the pathology progression.
Infected patients demonstrated a lower frequency of Th1, Th2, and Th17 subsets than NI group. In contrast, the proportion of Treg cells was the highest in asymptomatic patients. Furthermore, only CD86 was associated with Treg cells, while CD80 showed a negative correlation with these regulatory cells only in the groups without cardiomyopathy (NI and IND). We believe that CD86 may activate Treg cells, especially in IND patients, once they shown higher CD86 expression and Treg proportion. It has been proposed that CD80 expression in APCs induces Th1 differentiation, while CD86 drives Th2 profile (46–48). Conversely, the higher frequency of Treg cells was associated with better clinical prognosis in Chagas disease patients without CARD changes (49–51). We suggest that CD86 is a key molecule to direct immunoregulation in asymptomatic patients, control immune response, and prevent progressive fibrosis in the heart.
Survival of T. cruzi in the host depends on it escaping from immune recognition and/or it evading immune effector mechanisms. Several studies revealed that proteins secreted by the protozoan in the bloodstream, glycosylphosphatidylinositol-mucins molecules present in the parasite surface, and the blockade of complement pathways are strategies used by T. cruzi to escape immune recognition and delay the progression of immune response (52–54). The capacity of the parasite to survive has been attributed to the suppression of immune responsiveness. Infection of macrophages favors the secretion of anti-inflammatory cytokines such as IL-10 and TGF-β, which impair the development of protective immune responses and help the spread of the infection (55, 56). Here, we suggest that CD86 expression may control the immune response by binding to CTLA-4 and activating Treg lymphocyte in IND, but not CARD patients. These results suggest that T. cruzi infection induces an increase in CD86 expression on monocytes, thus contributing to the inhibition of T cells and restrain the immune response.
The present study is the first to reveal the functional-phenotypic profile of monocytes subsets in Chagas disease. We proposed that CD86 may be involved in immunoregulation by its association with CTLA-4 in asymptomatic patients. CD86 and CTLA-4 interaction may influence Treg activation, and this could represent a new strategy to control inflammation and tissue damage. Further studies are necessary to better understand this putative evasion mechanism. This study advances the current knowledge about the immunoregulatory mechanisms mediated by CD86, CTLA-4, and by Treg cells in Chagas disease.
Ethics Statement
This study was carried out in accordance with the recommendations of the Ethics Committee of the René Rachou Institute with written informed consent from all subjects. All subjects gave written informed consent in accordance with the Declaration of Helsinki. The protocol was approved by the Ethics Committee of the René Rachou Institute, FIOCRUZ (15/2011).
Author Contributions
BP performed the experiments, data analysis, and wrote the paper. NM delineated the experiments, discussed the results, and made the figures. AT-C gave statistical analysis support and discussed the results. SE-S carried out the clinical assessment. DR and TF-C helped to write the manuscript and prepare the samples. RC-O and WD contributed to discussions of the results and scientific resources. JG acquired and administered funding resources, and coordinated the study. All authors contributed to manuscript revision, read, and approved the submitted version.
Conflict of Interest Statement
The authors declare that the research was conducted in the absence of any commercial or financial relationships that could be construed as a potential conflict of interest.
Funding
JG received sources of support provided by Conselho Nacional do Desenvolvimento Científico e Tecnológico (CNPq - #474796/2012) and Fundação de Amparo à Pesquisa do Estado de Minas Gerais (FAPEMIG - #02419-15). NM and DR received financial support from FAPEMIG. BP, TF-C, WD, RC-O, AT-C, and JG received financial support from CNPq (PQ) fellowship program. The funders had no role in study design, data collection and analysis, decision to publish, or preparation of the manuscript.
References
1. Laranja FS, Dias E, Nobrega G, Miranda A. Chagas disease—a clinical, epidemiologic, and pathologic study. Circulation (1956) 14:1035–60. doi:10.1161/01.CIR.14.6.1035
2. Prata A. Classificação da infecção chagásica no homem. Rev Soc Bras Med Trop (1990) 23:109–13. doi:10.1590/S0037-86821990000200008
3. Andrade LO, Machado CRS, Chiari E, Pena SDJ, Macedo AM. Trypanosoma cruzi: role of host genetic background in the differential tissue distribution of parasite clonal populations. Exp Parasitol (2002) 100:269–75. doi:10.1016/s0014-4894(02)00024-3
4. Ferreira ER, Bonfim-Melo A, Mortara RA, Bahia D. Trypanosoma cruzi extracellular amastigotes and host cell signaling: more pieces to the puzzle. Front Immunol (2012) 3:363. doi:10.3389/fimmu.2012.00363
5. Martín UO. Circulating immune complexes in different developmental stages of Chagas’ disease. Medicina (B Aires) (1987) 47:159–62.
6. Ribeiro AL, Nunes MP, Teixeira MM, Rocha MOC. Diagnosis and management of Chagas disease and cardiomyopathy. Nat Rev Cardiol (2012) 9:576–89. doi:10.1038/nrcardio.2012.109
7. Andrade DV, Gollob KJ, Dutra WO. Acute Chagas disease: new global challenges for an old neglected disease. PLoS Negl Trop Dis (2014) 8:7. doi:10.1371/journal.pntd.0003010
8. Mathews JC. Valor de la Prueba de Esfuerzo graduado (Ergometria) Para Determinar la Capacidad Laboral del Cardiópata Chagásico Crônico. [PhD Thesis]. Argentina: Universidad Nacional de Córdoba (1973).
9. Brener Z, Gazzinelli RT. Immunological control of Trypanosoma cruzi infection and pathogenesis of Chagas’ disease. Int Arch Allergy Immunol (1997) 114:103–10. doi:10.1159/000237653
10. Ribeiro ALP, Rocha MOC. Forma indeterminada da doença de Chagas: considerações acerca do diagnóstico e do prognóstico. Rev Soc Bras Med Trop (1998) 31:301–14. doi:10.1590/S0037-86821998000300008
11. Nogueira LG, Santos RHB, Fiorelli AI, Mairena EC, Benvenuti LA, Bocchi EA, et al. Myocardial gene expression of T-bet, GATA-3, Ror-t, FoxP3, and hallmark cytokines in chronic Chagas disease cardiomyopathy: an essentially unopposed TH1-Type response. Mediators Inflamm (2014) 2014:1–9. doi:10.1155/2014/914326
12. Gomes JAS, Campi-Azevedo AC, Teixeira-Carvalho A, Silveira-Lemos D, Vitelli-Avelar D, Sathler-Avelar R, et al. Impaired phagocytic capacity driven by downregulation of major phagocytosis-related cell surface molecules elicits an overall modulatory cytokine profile in neutrophils and monocytes from the indeterminate clinical form of Chagas disease. Immunobiology (2012) 217:1005–16. doi:10.1016/j.imbio.2012.01.014
13. Frade AF, Teixeira PC, Ianni BM, Pissetti CW, Saba B, Wang LHT, et al. Polymorphism in the alpha cardiac muscle Actin 1 gene is associated to susceptibility to chronic inflammatory cardiomyopathy. PLoS One (2013) 8:12. doi:10.1371/journal.pone.0083446
14. Magalhães LMD, Viana A, Chiari E, Galvao LMC, Gollob KJ, Dutra WO. Differential activation of human monocytes and lymphocytes by distinct strains of Trypanosoma cruzi. PLoS Negl Trop Dis (2015) 9:17. doi:10.1371/journal.pntd.0003816
15. Morrot A, Villar SR, González FB, Pérez AR. Evasion and immuno—endocrine regulation in parasite infection: two sides of the same coin in Chagas disease? Front Microbiol (2016) 7:704. doi:10.3389/fmicb.2016.00704
16. Teixeira ARL, Hecht MM, Guimaro MC, Sousa AO, Nitz N. Pathogenesis of Chagas’ disease: parasite persistence and autoimmunity. Clin Microbiol Rev (2011) 24:592–630. doi:10.1128/CMR.00063-10
17. Gomes JA, Molica AM, Keesen TS, Morato MJ, de Araujo FF, Fares RC, et al. Inflammatory mediators from monocytes down-regulate cellular proliferation and enhance cytokines production in patients with polar clinical forms of Chagas disease. Hum Immunol (2013) 75:20–8. doi:10.1016/j.humimm.2013.09.009
18. Linsley PS, Ledbetter JA. The role of the CD28 receptor during T-cell responses to antigen. Annu Rev Immunol (1993) 11:191–212. doi:10.1146/annurev.immunol.11.1.191
19. Linsley PS, Greene JL, Brady W, Bajorath J, Ledbetter JA, Peach R. Human B7-1 (CD80) and B7-2 (CD86) bind with similar avidities but distinct kinetics to CD28 and CTLA-4 receptors. Immunity (1994) 1:793–801. doi:10.1016/s1074-7613(94)80021-9
20. Ziegler-Heitbrock L, Ancuta P, Crowe S, Dalod M, Grau V, Hart DN, et al. Nomenclature of monocytes and dendritic cells in blood. Blood (2010) 116:74–80. doi:10.1182/blood-2010-02-258558
21. Mukherjee R, Barman PK, Thatoi PK, Tripathy R, Das BK, Ravindran B. Non-classical monocytes display inflammatory features: validation in sepsis and systemic lupus erythematous. Nat Sci Rep (2015) 5:14. doi:10.1038/srep13886
22. Thaler B, Hohensinner P, Krychtiuk K, Matzneller P, Koller L, Brekalo M, et al. Differential in vivo activation of monocyte subsets during lowgrade inflammation through experimental endotoxemia in humans. Nat Sci Rep (2016) 6:1–8. doi:10.1038/srep30162
23. Moniuszko M, Bodzenta-Lukaszyk A, Kowal K, Lenczewska D, Dabrowska M. Enhanced frequencies of CD14++CD16+, but not CD14+CD16+, peripheral blood monocytes in severe asthmatic patients. Clin Immunol (2008) 130:338–46. doi:10.1016/j.clim.2008.09.011
24. Costa MC, Rocha BD, Paixao CS, de Oliveira M, da Mota LMH, de Carvalho LP. Monocyte subpopulations study in patients with plaque psoriasis. Med Hypotheses (2017) 104:101–3. doi:10.1016/j.mehy.2017.05.031
25. Grad E, Zolotarevsky K, Danenberg HD, Nordling-David MM, Gutman D, Golomb G. The role of monocyte subpopulations in vascular injury following partial and transient depletion. Drug Deliv Transl Res (2017): 1–9. doi:10.1007/s13346-017-0404-5
26. Wong KL, Tai JJY, Wong WC, Han H, Sem X, Yeap WH, et al. Gene expression profiling reveals the defining features of the classical, intermediate, and nonclassical human monocyte subsets. Blood (2011) 118:15–30. doi:10.1182/blood-2010-12-326355
27. Hathcock KS, Laszlo G, Pucillo C, Linsley P, Hodes RJ. Comparative-analysis of b7-1 and b7-2 costimulatory ligands—expression and function. J Exp Med (1994) 180:631–40. doi:10.1084/jem.180.2.631
28. Zheng Y, Manzotti CN, Liu M, Burke F, Mead KI, Sansom DM. CD86 and CD80 differentially modulate the suppressive function of human regulatory T cells. J Immunol (2004) 172:2778–84. doi:10.4049/jimmunol.172.5.2778
29. Gu P, Gao JF, D’ Souza CA, Kowalczyk A, Chou KY, Zhang L. Trogocytosis of CD80 and CD86 by induced regulatory T cells. Cell Mol Immunol (2012) 9:136–46. doi:10.1038/cmi.2011.62
30. Higuchi MD, Debrito T, Reis MM, Barbosa A, Bellotti G, Pereirabarreto AC, et al. Correlation between Trypanosoma-cruzi parasitism and myocardial inflammatory infiltrate in human chronic chagasic myocarditis-light-microscopy and immunohistochemical findings. Cardiovasc Pathol (1993) 2:101–6. doi:10.1016/1054-8807(93)90021-s
31. Dutra WO, Gollob KJ, Pinto-Dias JC, Gazzinelli G, Correa-Oliveira R, Coffman RL, et al. Cytokine mRNA profile of peripheral blood mononuclear cells isolated from individuals with Trypanosoma cruzi chronic infection. Scand J Immunol (1997) 45:74–80. doi:10.1046/j.1365-3083
32. Gomes JA, Bahia-Oliveira LM, Rocha MOC, Martins-Filho OA, Gazzinelli G, Correa-Oliveira R. Evidence that development of severe cardiomyopathy in human Chagas’ disease is due to a Th1-specific immune response. Infect Immun (2003) 71:1185–93. doi:10.1128/IAI.71.3.1185–1193.2003
33. Souza PEA, Rocha MOC, Rocha-Vieira E, Menezes CAS, Chaves ACL, Gollob KJ, et al. Monocytes from patients with indeterminate and cardiac forms of Chagas’ disease display distinct phenotypic and functional characteristics associated with morbidity. Infect Immun (2004) 72:5283–91. doi:10.1128/IAI.72.9.5283-5291.2004
34. Vitelli-Avelar DM, Sathler-Avelar R, Teixeira-Carvalho A, Dias JCP, Gontijo ED, Faria AM, et al. Strategy to assess the overall cytokine profile of circulating leukocytes and its association with distinct clinical forms of human Chagas disease. Scand J Immunol (2008) 68:516–25. doi:10.1111/j.1365-3083.2008.02167.x
35. Souza PEA, Rocha MOC, Menezes CAS, Coelho JS, Chaves ACL, Gollob KJ, et al. Trypanosoma cruzi infection induces differential modulation of costimulatory molecules and cytokines by monocytes and T cells from patients with indeterminate and cardiac Chagas’ disease. Infect Immun (2007) 75:1886–94. doi:10.1128/IAI.01931-06
36. Rocha TO, Teixeira MM, Ribeiro AL. An update on the management of Chagas cardiomyopathy. Expert Rev Anti Infect Ther (2007) 5:727–43. doi:10.1586/14787210.5.4.727
37. Takeda K, Kaisho T, Shizuo A. Toll-like receptors. Annu Rev Immunol (2003) 21:335–76. doi:10.1146/annurev.immunol.21.120601.141126
38. Gravina HD, Antonelli L, Gazzinelli RT, Ropert C. Differential Use of TLR2 and TLR9 in the regulation of immune responses during the infection with Trypanosoma cruzi. PLoS One (2013) 8:5. doi:10.1371/journal.pone.0063100
39. Rodrigues DBR, Reis MA, Romano A, Pereira SAL, Teixeira VPA, Junior ST, et al. In situ expression of regulatory cytokines by heart inflammatory cells in Chagas’ disease patients with heart failure. Clin Dev Immunol (2012) 2012:1–7. doi:10.1155/2012/361730
40. Bartholomeu DC, Ropert C, Melo MB, Parroche P, Junqueira CF, Teixeira SM, et al. Recruitment and endo-lysosomal activation of TLR9 in dendritic cells infected with Trypanosoma cruzi. J Immunol (2008) 181:2. doi:10.4049/jimmunol.181.2.1333
41. Mendes da Silva LD, Gatto M, Miziara de Abreu Teodoro M, de Assis Golim M, Pelisson Nunes da Costa ÉA, Capel Tavares Carvalho F, et al. Participation of TLR2 and TLR4 in cytokines production by patients with symptomatic and asymptomatic chronic Chagas disease. Scand J Immunol (2016) 85:58–65. doi:10.1111/sji.12501
42. Bolkun L, Rusak M, Eljaszewicz A, Pilz L, Radzikowska U, Lapuc I, et al. Enhanced pretreatment CD25 expression on peripheral blood CD4+ T cell predicts shortened survival in acute myeloid leukemia patients receiving induction chemotherapy. Pharmacol Rep (2016) 68:12–9. doi:10.1016/j.pharep.2015.05.025
43. Soares AKD, Neves PAF, Cavalcanti M, Marinho SM, de Oliveira W, de Souza JR, et al. Expression of co-stimulatory molecules CD80 and CD86 is altered in CD14(+)HLA-DR+ monocytes from patients with Chagas disease following induction by Trypanosoma cruzi recombinant antigens. Rev Soc Bras Med Trop (2016) 49:632–6. doi:10.1590/0037-8682-0149-2016
44. Bafica A, Santiago HC, Goldszmid R, Ropert C, Gazzinelli RT, Sher A. Cutting edge: TLR9 and TLR2 signaling together account for MyD88-dependent control of parasitemia in Trypanosoma cruzi infection. J Immunol (2006) 177:3515–9. doi:10.4049/jimmunol.177.6.3515
45. Martins GA, Tadokoro CE, Silva RB, Silva JS, Rizzo LV. CTLA-4 blockage increases resistance to infection with the intracellular protozoan Trypanosoma cruzi. J Immunol (2004) 172:4893–901. doi:10.4049/jimmunol.172.8.4893
46. Balkhi MY, Latchumanan VK, Singh B, Sharma P, Natarajan K. Cross-regulation of CD86 by CD80 differentially regulates T helper responses from Mycobacterium tuberculosis secretory antigen-activated dendritic cell subsets. J Leukoc Biol (2004) 75(5):874–83. doi:10.1189/jlb.1003476
47. Asai-Tajiri Y, Matsumoto K, Fukuyama S, Kan-o K, Nakano T, Tonai K, et al. Small interfering RNA against CD86 during allergen challenge blocks experimental allergic asthma. Respir Res (2014) 15:132. doi:10.1186/s12931-014-0132-z
48. Said EA, Al-Reesi I, Al-Riyami M, Al-Naamani K, Al-Sinawi S, Al-Balushi MS, et al. Increased CD86 but Not CD80 and PD-L1 expression on liver CD68+ cells during chronic HBV infection. PLoS One (2016) 1–13. doi:10.1371/journal.pone.0158265
49. Vitelli-Avelar DM, Sathler-Avelar R, Dias JC, Pascoal VP, Teixeira-Carvalho A, Lage PS, et al. Chagasic patients with indeterminate clinical form of the disease have high frequencies of circulating CD3+CD16-CD56+ natural killer T cells and CD4+CD25High regulatory T lymphocytes. Scand J Immunol (2005) 62:297–308. doi:10.1111/j.1365-3083.2005.01668.x
50. de Araújo FF, Vitelli-Avelar DM, Teixeira-Carvalho A, Antas PR, Gomes JAS, Sathler-Avelar R, et al. Regulatory T cells phenotype in different clinical forms of Chagas’ disease. PLoS Negl Trop Dis (2011) 5:5. doi:10.1371/journal.pntd.0000992
51. de Araújo FF, Corrêa-Oliveira R, Rocha MOC, Chaves AT, Fiuza JA, Fares RCG, et al. Foxp3+CD25highCD4+ regulatory T cells from indeterminate patients with Chagas disease can suppress the effector cells and cytokines and reveal altered correlations with disease severity. Immunobiology (2012) 207:768–77. doi:10.1016/j.imbio.2012.04.008
52. Nardy AF, Freire-De-Lima CG, Morrot A. Immune evasion strategies of Trypanosoma cruzi. J Immunol Res (2015) 2015:178947. doi:10.1155/2015/178947
53. Cardoso MS, Reis-Cunha JL, Bartholomeu DC. Evasion of the imune response by Trypanossoma cruzi during acute infection. Front Immunol (2016) 6:659. doi:10.3389/fimmu.2015.00659
54. Nardy AF, Freire-de-Lima CG, Perez AR, Morrot A. Role of Trypanosoma cruzi Trans-sialidase on the escape from host immune surveillance. Front Microbiol (2016) 7:348. doi:10.3389/fmicb.2016.00348
55. Silva JS, Twardzik DR, Reed SG. Regulation of Trypanosoma cruzi infections in vitro and in vivo by transforming growth factor beta (TGF-beta). J Exp Med (1991) 174:539–45. doi:10.1084/jem.174.3.539
Keywords: chagas disease, monocytes, immunoregulation, innate immunity, immune response
Citation: Pinto BF, Medeiros NI, Teixeira-Carvalho A, Eloi-Santos SM, Fontes-Cal TCM, Rocha DA, Dutra WO, Correa-Oliveira R and Gomes JAS (2018) CD86 Expression by Monocytes Influences an Immunomodulatory Profile in Asymptomatic Patients with Chronic Chagas Disease. Front. Immunol. 9:454. doi: 10.3389/fimmu.2018.00454
Received: 12 January 2018; Accepted: 20 February 2018;
Published: 12 March 2018
Edited by:
Juarez Antonio Simões Quaresma, Instituto Evandro Chagas, BrazilReviewed by:
Suowen Xu, University of Rochester, United StatesM. Victoria Delpino, National Scientific and Technical Research Council (CONICET)
Copyright: © 2018 Pinto, Medeiros, Teixeira-Carvalho, Eloi-Santos, Fontes-Cal, Rocha, Dutra, Correa-Oliveira and Gomes. This is an open-access article distributed under the terms of the Creative Commons Attribution License (CC BY). The use, distribution or reproduction in other forums is permitted, provided the original author(s) and the copyright owner are credited and that the original publication in this journal is cited, in accordance with accepted academic practice. No use, distribution or reproduction is permitted which does not comply with these terms.
*Correspondence: Juliana A. S. Gomes, juliana@icb.ufmg.br