- 1Department of Pediatric Newborn Medicine, Brigham and Women’s Hospital, Boston, MA, United States
- 2Department of Medicine, Division of Infectious Diseases, Boston Children’s Hospital, Boston, MA, United States
- 3Harvard Medical School, Boston, MA, United States
- 4Precision Vaccines Program, Division of Infectious Diseases, Boston Children’s Hospital, Boston, MA, United States
- 5Department of Translational Medical Sciences, Center for Basic and Clinical Immunology Research (CISI), University of Naples Federico II, Naples, Italy
- 6WAO Center of Excellence, Naples, Italy
- 7Neonatal Intensive Care Unit, Department of Clinical Sciences and Community Health, Fondazione IRCCS Ca’ Granda Ospedale Maggiore Policlinico, Università degli Studi di Milano, Milan, Italy
- 8Division of Newborn Medicine, Boston Children’s Hospital, Boston, MA, United States
- 9Thomas Jefferson University, Philadelphia, PA, United States
- 10Medical Eli Lilly, Shanghai, China
- 11Department of Pediatrics, British Columbia Children’s Hospital, Vancouver, BC, Canada
Immunization is key to protecting term and preterm infants from a heightened risk of infection. However, preterm immunity is distinct from that of the term, limiting its ability to effectively respond to vaccines routinely given at birth, such as hepatitis B vaccine (HBV). As part of the Expanded Program on Immunization, HBV is often given together with the live-attenuated vaccine Bacille Calmette–Guérin (BCG), known to activate multiple pattern-recognition receptors. Of note, some clinical studies suggest BCG can enhance efficacy of other vaccines in term newborns. However, little is known about whether BCG can shape Th-polarizing cytokine responses to HBV nor the age-dependency of such effects, including whether they may extend to the preterm. To characterize the effects of BCG on HBV immunogenicity, we studied individual and combined administration of these vaccines to cord newborn and adult human whole blood and mononuclear cells in vitro and to neonatal and adult mice in vivo. Compared to either BCG or HBV alone, (BCG + HBV) synergistically enhanced in vitro whole blood production of IL-1β, while (BCG + HBV) also promoted production of several cytokines/chemokines in all age groups, age-specific enhancement included IL-12p70 in the preterm and GM-CSF in the preterm and term. In human mononuclear cells, (BCG + HBV) enhanced mRNA expression of several genes including CSF2, which contributed to clustering of genes by vaccine treatment via principle component analysis. To assess the impact of BCG on HBV immunization, mice of three different age groups were immunized subcutaneously with, BCG, HBV, (BCG + HBV) into the same site; or BCG and HBV injected into separate sites. Whether injected into a separate site or at the same site, co-administration of BCG with HBV significantly enhanced anti-HBV IgG titers in mice immunized on day of life-0 or -7, respectively, but not in adult mice. In summary, our data demonstrate that innate and adaptive vaccine responses of preterm and term newborns are immunologically distinct. Furthermore, BCG or “BCG-like” adjuvants should be further studied as a promising adjuvantation approach to enhance immunogenicity of vaccines to protect these vulnerable populations.
Introduction
Infectious diseases are a leading cause of childhood death with neonatal infection accounting for ~40% of mortality in those <5 years of age, ~7 million cases, and 700,000 deaths per year (1). Within the neonatal population, prematurity, defined as birth at <37 weeks of gestational age (GA), is the single most important cause of death in the first month of life and the second largest cause of death after pneumonia in children <5 years of age (1). Most preterm births (84%, 12.5 million) occur at >32 weeks of gestation (2). At this GA, many preterm newborns can survive with cost-effective supportive care. The mortality from neonatal infection in preterm infants has increased over the last 20 years (1). Moreover, preterm newborns remain at elevated risk of infection through 18 years of age (3). Accordingly, global progress in child survival and health to 2015 and beyond will depend on optimizing preventative care for preterm and term infants with vaccines one of the most effective biomedical approaches for disease prevention.
Vaccine-mediated prevention of infections is limited by reduced or distinct immune responses in early life (4). The current necessity for repeated vaccine booster doses to obtain full protection leaves a window of susceptibility in both the preterm and term infant during the first 6 months of life (5). Alongside efforts at maternal immunization, enhancement of responses to early-life vaccines via use of novel adjuvantation systems that demonstrate age-specific immune-enhancing activity is an attractive approach to address this problem. To date, development of pediatric vaccines has largely relied on ad hoc studies of adult vaccines, and has not taken the age-dependent development of the immune system into account (6). This holds true for vaccination of the preterm as well: the Advisory Committee on Immunization Practices (ACIP) of US Centers for Disease Control and Prevention as of 2011 has recommended that, with respect to most vaccines, preterm infants be immunized with the full-recommended dose according to the same schedule as full-term infants. However, even thought vaccine immunogenicity in preterm infants is often distinct compared with term responses (7–10), there remains a research knowledge gap. Specifically, hepatitis B vaccine (HBV) immunization is delayed in the preterm due to empiric evidence of reduced immunogenicity. In preterm newborns <1,500–1,800 g birth weight or <34–35 weeks gestation, a three-dose vaccine series of HBV induces protective antibody (Ab) titers in only ~45–85% of patients as opposed to 90–100% in more mature infants (11).
Consideration of vaccine adjuvantation in early life must take into account that newborn innate and adaptive immune cells exhibit distinct activation profiles in response to pattern-recognition receptor (PRR) agonists. However, activation of some PRRs in newborns, such as toll-like receptors (TLRs) 7/8, can induce an adult-like response (12, 13). For example, the germinal center reaction that drives the magnitude and persistence of the Ab response is impaired early in life but can be enhanced with certain TLR agonists (14, 15). Of note, preterm innate and adaptive immunity is distinct from that of both term newborns and adults. For example, preterm monocytes exhibit attenuated PRR-mediated Th1 and Th17-cytokine responses (16). Herein, we assessed whether a live-attenuated vaccine Bacille Calmette–Guérin (BCG), known to activate multiple PRRs (17, 18), might exert adjuvant activity in the context of neonatal HBV immunization.
BCG is the most commonly administered vaccine worldwide and when administered at birth is safe and effective in reducing the rates of infantile tuberculous (TB), meningitis, and disseminated miliary disease (19). It is the only routinely administered neonatal vaccine that induces a Th1-polarized immune response (20). BCG administration may also, in an age-dependent manner, induce beneficial heterologous (“non-specific” or “trained”) immunity against unrelated pathogens and stimuli (21–25), impact responses to other vaccines (20, 26–28), and immune-modulate in the context of allergic diseases as well (29).
Some limited clinical studies have suggested that BCG may enhance responses to other, vaccines such as Oral Polio Vaccine and HBV (20). We posited that co-administration of BCG with HBV could induce greater innate and adaptive immune responses, including acute cytokine induction and HBV-specific Ab production. Employing in vitro human blood and mononuclear cell assays we demonstrated that (BCG + HBV) enhanced cytokine and chemokine production on both the protein and mRNA level. Strikingly, in vivo immunization of neonatal [day of life (DOL)-0 and -7] and adult mice demonstrated that combined (BCG + HBV) vaccination induced anti-HBV-specific Ab titers in all three age groups at 21 days post-immunization relative to immunization with HBV alone. Overall, our studies provide fresh insight into a vaccine–vaccine interaction that may be the basis of enhanced immunization strategies for vulnerable preterm and term newborn populations.
Materials and Methods
Cord Blood Collection
Moderate to late preterm (28 2/7–34 6/7 weeks GA) and term cord blood was collected at The Brigham and Women’s Hospital and the Beth Israel Deaconess Medical Center, both tertiary care centers for delivery and postnatal care of the preterm and term newborns. The details of our preterm study cohort are outlined in Table 1. The de-identified newborn cord blood (~15–60 ml) was collected immediately after caesarian section or vaginal delivery of the placenta from a larger placental or umbilical vein under sterile conditions, as previously described (30). No cord blood samples from newborns born to human-immunodeficiency virus-positive mothers were included. Samples were collected from both male and female newborns. Blood and blood-derived products were handled per applicable biohazard policies. As the type of anti-coagulant and length of storage prior to assay can affect cytokine production (31), we have established a routine standard of procedure in which blood was anti-coagulated with 15–20 U/ml pyrogen-free heparin sodium (Sagent Pharmaceuticals, Inc.; Schaumberg, IL, USA), and then kept at room temperature (RT) and processed within 4 hours (h) of collection (typically 1–2 h). Each preterm placenta was histologically examined for signs of chorioamnionitis, and information on the timing of prenatal steroid administration, as well antibiotic administration was collected. Peripheral blood was collected from healthy adult male and female volunteers employed at BCH.
Animals
C57BL/6 mice were obtained from Charles River Laboratories and housed in specific pathogen-free conditions in the animal research facilities at BCH. To obtain newborn mice, pregnant dams were purchased on pre-determined days of pregnancy and cages checked twice daily (~every 12 h) to assess for the presence of pups. Both male and female pups were used for experiments.
Vaccines and Whole Blood Assay
~8 ml of fresh blood was processed for the whole blood assay as previously described (32). Briefly, neonatal cord (preterm and/or term) or adult whole blood was mixed 1:1 with sterile pre-warmed (37°C) RPMI 1640 medium (Invitrogen) and 180 µl of the 1:1 suspension added to each well of a 96-well U-bottom plate (Becton Dickinson) containing 20 µl freshly prepared HBV, BCG, (BCG + HBV) at 10× final concentration, testing stimuli at a 5-point concentration–response curve based on published data (12, 33). As sources of BCG and HBV, we used the Danish Strain 1331 (Statens Serum Institut, Copenhagen, Denmark) and Recombivax® HB (Merck and Co, Inc.), respectively. Suspensions containing 200 μl/well were gently mixed by pipetting and incubated at 37°C in a humidified incubator at 5% CO2 for 6 h.
ELISA and Multiplex Cytokine Analysis
After treatment of the preterm, term, and adult blood with the described vaccines for 6 h, the plates were centrifuged (10 min, RT, 500 g), and supernatants collected and stored in three aliquots at −80°C for subsequent TNF (BD Biosciences Human TNF ELISA) and IL-1β ELISA (eBioscience Human IL-1β ELISA) and for subsequent multiplexing assays for Th1 (TNF, IL-1β, IL-12p70, IFNα, and IFNγ) and Th2 (IL-6, IL-10, and IL-12p40), and Th17 (IL-6, IL-1β) polarizing cytokines (Milliplex Human Magnetic Bead Panel; Millipore; Chicago, IL, USA). Data were analyzed on the Luminex® 100/200™ System using xPOTENT® software (Luminex; Austin, TX, USA).
Isolation of Cord Blood Mononuclear Cells (CBMCs) and Peripheral Blood Mononuclear Cells (PBMCs) and In Vitro Stimulation
From each whole blood sample collected, matched PBMCs and CBMCs were isolated using Ficoll density gradient methodologies and cryopreserved for further downstream stimulation experiments (12, 33). MCs were stored at 50 million cells per vial in 1 ml RPMI containing 20% autologous plasma and 10% DMSO at −80°C until use. After a standardized thawing procedure, PBMCs and CBMCs isolated from human donors were resuspended at a concentration of 2 × 106 cells/1000 µl of RPMI supplemented with 10% of autologous platelet-poor plasma. Cells were stimulated for 4 h with either HBV, BCG, or (BCG + HBV) (each at 1:1,000, 1:100, 1:10 vol/vol) and cells washed with ice cold PBS prior to addition of RLT buffer (RNeasy Lysis Buffer, Qiagen, MD, USA) and storage at −80°C for subsequent RNA isolation.
Gene Expression Analysis by Quantitative Real-time PCR Array
Total RNA was extracted from lysates of vaccine-stimulated PBMCs and CBMCs using the Qiagen RNeasy Minikit and DNAse treatment performed using the Qiagen RNAase Free DNAase set all per the manufacturer’s instructions. RNA concentrations were determined using the Nanodrop 1000 and cDNA generated using the Qiagen RT2 First Strand Kit. 96-well PCR array analysis was performed using the Qiagen standardized Innate and Adaptive Immune Reponses PCR Array (PAHS-0522A) and RT2 qPCR roxSYBR green kit. Web-based PCR array analyses (RT2 Profiler PCR Array Data Analysis version 3.5) was used and normalized to five reference genes (B2M, HPRT1, RPL13A, GAPDH, and ACTB). Relative quantification of gene expression was calculated by the ΔCt (relative expression × 104). Multivariate biplots of principal component analyses were performed in R 3.4.2 using ggplot2, ggord, and vegan packages using log-fold transcript abundance of gene arrays in each group. Genes were sorted using unsupervised hierarchical heatmap clustering of log-fold changes using the heatmap2 package.
Immunization and Anti-Recombinant HBV Surface Antigen (rHBsAg)-Specific Ab Quantification, Subtype Classification, and Avidity Determination
For immunization experiments, mice of three age groups were used: the first group of mice were given their first immunization (prime immunization) on DOL0; the second group of mice on DOL7, and in the third group at 6–8 weeks of life. Each of the three age groups were divided into five immunization groups: saline; BCG (Organon Teknika/Merck, Durham, NC, USA) alone; HBV vaccine alone; (BCG + HBV) as a combined admixed injection; and BCG with HBV vaccine administered separately. All immunizations were injected subcutaneously (s.c.). If one injection was performed per animal, it was performed into the right posterior thigh, if two separate injections were performed they were performed either into the right and left posterior thighs (DOL7 mice; adult mice) or in DOL0 mice into the right posterior thigh (HBV) and the scruff (BCG). The injection volumes were 50 µl of vaccine (or vaccine combination)/injection in the adult animals and 25 µl of vaccine (or vaccine combination)/injection in the newborn animals (DOL0 and DOL7). The injection dose of Recombivax® was 0.25 µg of rHBsAg for the adult animals and 0.125 µg in the newborn pups (DOL0 and DOL7) diluted in 0.9% NaCl Inj (USP). We chose to administer half HBV doses in the newborn as this is an established approach in human clinical vaccinology (ACIP recommendations for hepatitis B immunization). The injection dose for BCG was 0.4 × 106 CFU for the adult animals and 0.2 × 106 CFU for the pups (DOL0 and DOL7) diluted in 0.9% NaCL Inj (USP). We selected the BCG dose based on published literature in neonatal mice (12, 26). The selected dose of HBV was slightly lower than that routinely used in other murine studies (34) and reflected the volume limitations inherent to administration of two vaccines. We conducted preliminary experiments to confirm that we could obtain measurable Ab titers with the chosen concentration of HBV in all age groups. Mice were immunized with a prime-boost schedule; a primary immunization; and a secondary (booster) immunization, 2 weeks apart. Serum samples were obtained from blood collected via tail vein or artery nick as indicated for Ab detection. rHBsAg-specific IgG were quantified by ELISA. High binding flat bottom 96-well plates (Corning Life Sciences) were coated with Recombivax® diluted to 1 µg/ml in carbonate buffer pH 9.6, incubated overnight at 4°C, and blocked with PBS + BSA 1% (Sigma-Aldrich) for 1 h at RT. Then, sera from immunized mice were added with an initial dilution of 1:100 and 1:3 serial dilutions in PBS + BSA 1% and incubated for 2 h at RT. Plates were then washed and incubated for 1 h at RT with HRP-conjugated anti-mouse IgG, IgG1, IgG2c (Southern Biotech). At the end of the incubation, plates were washed again and developed with tetramethylbenzidine (BD Biosciences) for 5 min, then stopped with 2N H2SO4. The optical density was read at 450 nm on a Versamax microplate reader with SoftMax Pro Version 5 (both from Molecular Devices), and endpoint titers were calculated using as cutoff two times the optical density of the background (35). For assessing Ab avidity, plates were incubated 15 min with ammonium thiocyanate 0.5 M before the addition of HRP-conjugated Abs. Avidity was expressed as the LogEC50 ratio of corresponding plates treated with or without ammonium thiocyanate (36).
Statistical Analyses and Graphics
Data were analyzed and graphed using Prism for MacIntosh v. 7.0 (GraphPad Software). Tests used for statistical comparisons are indicated in figure legends. p value < 0.05 was considered significant. An adaptation of the Loewe method of additivity (37) was used to assess whether cytokine production after stimulation with (BCG + HBV) was synergistic, additive, or antagonistic. Concentration–response curves were subjected to regression analysis to determine the slope and y-intercept of each curve in the exponential phase. The formula D = [Ac]/[Ae] + [Bc]/[Be] was used, where [Ac] = the concentration of (HBV) used in the combination of (HBV + BCG) that results in half the maximal TNF production measured with the combination of both vaccines; [Ae] = the concentration of HBV used alone that results in half the maximal TNF production measured with the combination of (HBV + BCG); [Bc] = the concentration of BCG used in the combination of (HBV + BCG) that results in half the maximal TNF production measured with the combination of vaccines; and [Be] = the concentration of BCG used alone that results in half the maximal TNF production measured with the combination of (BCG + HBV). If D = 1: (HBV + BCG) act additively, if D > 1: (HBV + BCG) act antagonistically, and if D < 1: (HBV + BCG) act synergistically. Our laboratory has employed this interaction analysis method in other recently published studies (13, 38, 39).
Results
(BCG + HBV) Synergistically Enhances IL-1β Production in Preterm, Term, and Adult Whole Blood
The in vitro whole blood assay is a useful tool to characterize the effects of vaccines on cytokine production as it enables testing of moderate number of different vaccine formulations, at multiple concentrations in a sample from a single individual (13, 38, 40). To assess whether (BCG + HBV) may enhance NF-κB and inflammasome-mediated cytokine induction compared to HBV alone, we compared (BCG + HBV) with identical concentrations of BCG and HBV in its ability to stimulate TNF and IL-1β production in preterm (Figure 1A,D) or term cord (Figure 1B,E) or adult peripheral blood (Figure 1C,F). In all three age groups, (BCG + HBV) significantly increased TNF and IL-1β secretion relative to RPMI controls and also secretion of IL-1β relative to HBV alone. Using the Berenbaum equation to assess drug–drug interactions, the interaction between HBV and BCG with regard to TNF was additive or antagonistic (Table 2). However, with respect to IL-1β, the combined vaccine effect was synergistic, defined as a D-value < 1, especially so in the term followed by the preterm, and least pronounced in the adult (Table 2). Of note, IL-1β is important to immunogencity of ALUM-adjuvanted vaccines (41), has a role in neutrophil recruitment and in Ab production, and has been used as an adjuvant (42–45). Figure 1 also demonstrates that BCG alone is a potent inducer of both TNF and IL-1β in whole blood of all age groups and in this context is likely the driving component behind this vaccine interaction.
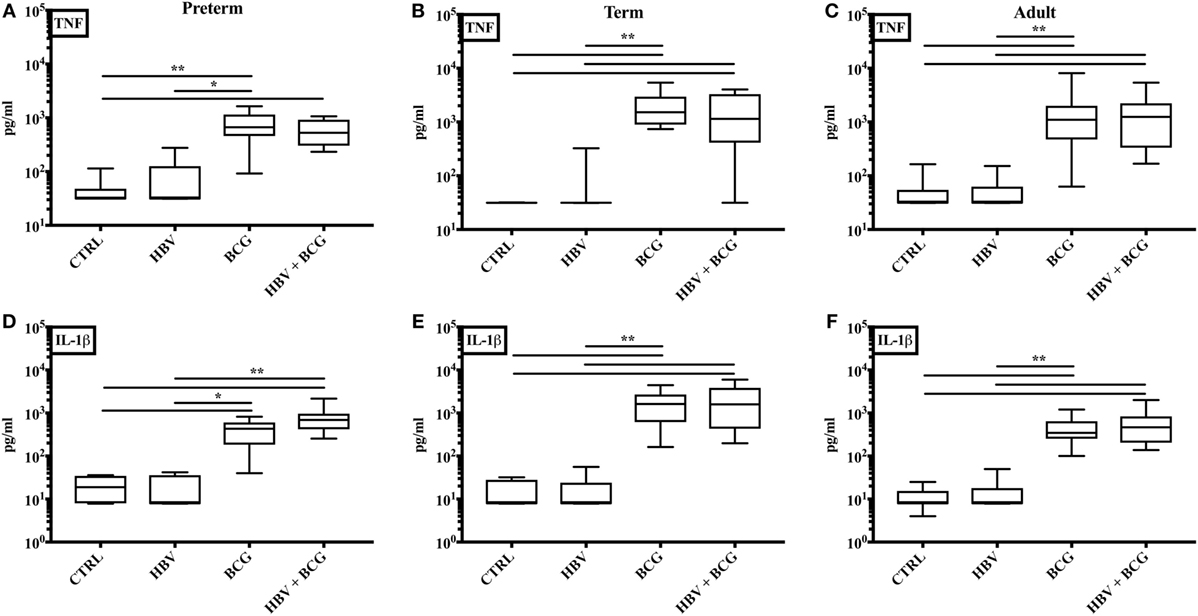
Figure 1. Concurrent stimulation with [Bacille Calmette–Guérin (BCG) + hepatitis B vaccine (HBV)] synergistically enhances IL-1β production in human preterm, term, and adult whole blood. Preterm (A, D), term (B, E), and adult (C, F) whole blood was stimulated with HBV alone, BCG alone, or (BCG + HBV) at 1:10 v/v. After 6 h, supernatants were analyzed for TNF (A–C) and IL-1β (D–F) cytokine production by ELISA. Statistical significance was determined by Kruskal–Wallis with Dunn post hoc test. *p < 0.05, **p < 0.01. N = 7–8 preterm, N = 10–11 term newborns, and N = 10–11 adults.
The Combination of BCG and HBV Vaccine Stimulates Secretion of Numerous Cytokines and Chemokines in Preterm, Term, and Adult Whole Blood
Having established that (BCG + HBV) enhance TNF and IL-1β production by preterm and term whole blood, we next characterized the cytokine profiles induced by these vaccine treatments in more detail employing multiplex cytokine analysis on the isolated supernatants. As shown in Figure 2, cytokines significantly induced in preterm or term cord blood by the vaccine combination relative to RPMI control and/or HBV vaccine alone included CSF2 (GM-CSF), IL-6, IL-10, CXCL8, CCL2, and CCL3. Interestingly, IL-12p70 was significantly induced by the vaccine combination in the preterm. Of note, the similarity of the BCG cytokine/chemokine profile to that induced by (BCG + HBV) suggested that the vaccine combination effect was mainly driven by BCG.
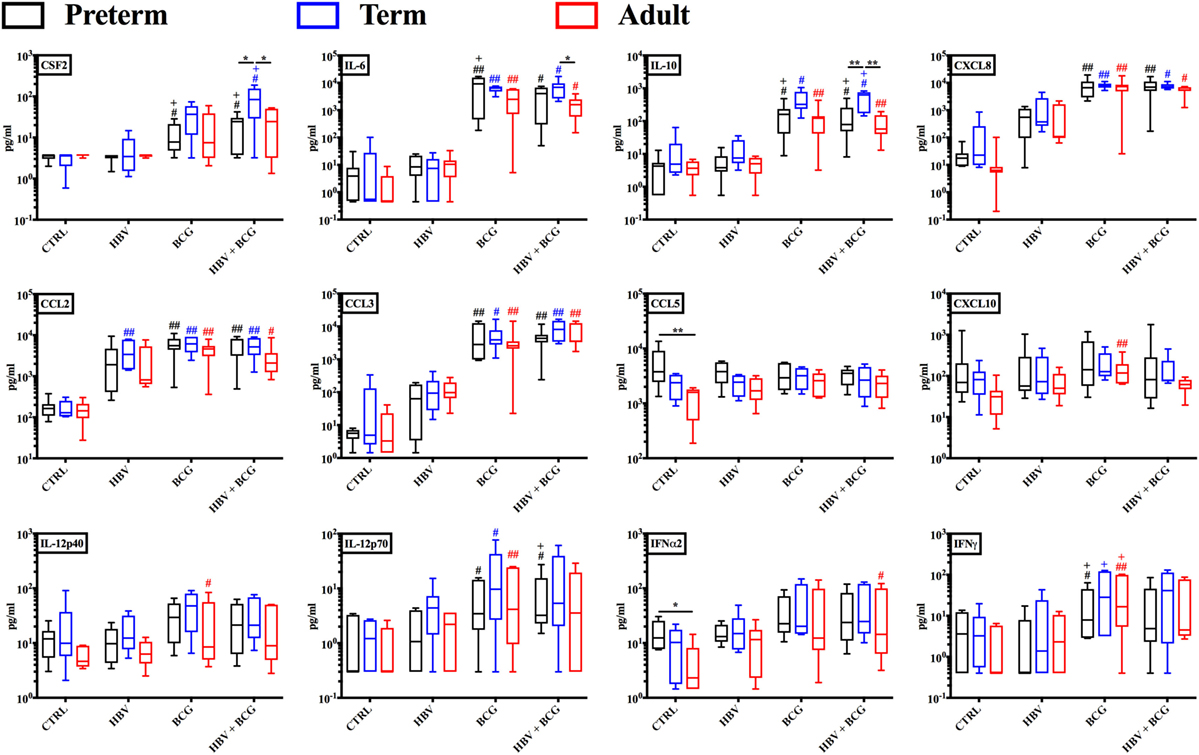
Figure 2. Cytokine and chemokine profiles induced by hepatitis B vaccine (HBV) and Bacille Calmette–Guérin (BCG) in human preterm, term, and adult whole blood. Preterm, term, and adult whole blood was stimulated for 6 h with either HBV, BCG, or (BCG + HBV) and supernatants analyzed via Multiplex Cytokine Analysis. Statistical significance was determined by repeated measure or ordinary one-way ANOVA with Holm-Sidak post hoc test (or their non-parametric equivalent Friedman or Kruskal–Wallis with Dunn post hoc test). *p < 0.05, **p < 0.01 of preterm vs. term vs. adult; #,+p < 0.05, ##,++p < 0.01 of groups indicated by the corresponding color, respectively, vs. saline or HBV. N = 7 preterm newborns, N = 6 term newborns, N = 7 adults.
HBV, BCG, and (BCG + HBV) Induce Distinct RNA Transcription Clusters in CBMCs/PBMCs Isolated from Preterm, Term, and Adult Individuals
To investigate how addition of BCG to HBV vaccination alters gene expression patterns at the mRNA level, RNA isolated from neonatal CBMCs (term and preterm) or adult PBMCs stimulated for 4 h with vehicle (control), BCG, HBV, or (BCG + HBV) was subjected to quantitative real-time PCR array comprised of 84 genes in human innate and adaptive immune pathways. mRNA levels were quantified in 4–5 individuals/group. Figure 3 shows that there were increases in expression of several cytokine and chemokine transcripts upon mononuclear cell stimulation with (BCG + HBV) compared to unstimulated or HBV-treated cells. Some of them reached statistical significance in all (i.e., CSF2) or some age groups (i.e., CXCL8 in preterms and adults). Interestingly, some of these genes encode proteins with defined roles in vaccine efficacy (13, 42–48). Figure 4A demonstrates treatment-driven segregation of age groups by a principle component biplot of mRNA gene expression data. The points representing age and treatment group (open circles) approximate gene expression patterns between groupings. The unsupervised hierarchical heat map in Figure 4B demonstrates similar clustering by treatment and outlines in a red to blue scale high to low gene expression per gene.
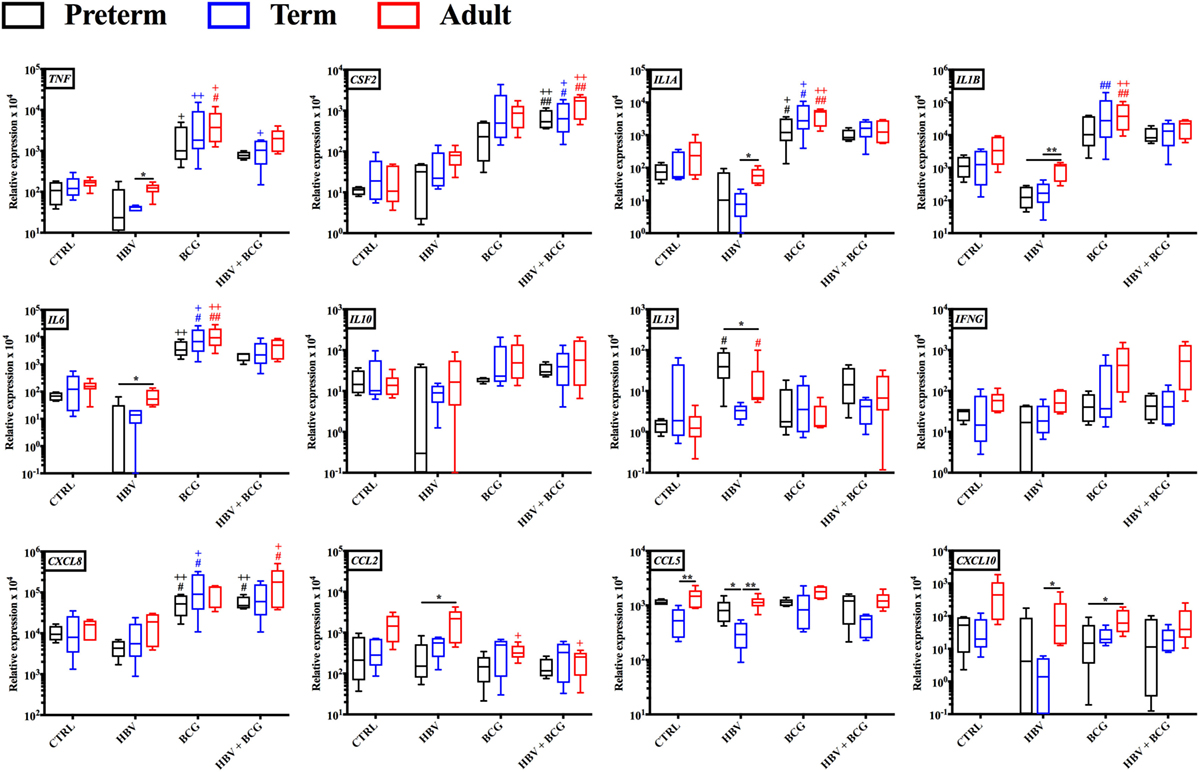
Figure 3. Gene expression profiles induced by hepatitis B vaccine (HBV) and Bacille Calmette–Guérin (BCG) in human preterm, term, and adult mononuclear cells. Preterm and term cord blood mononuclear cells, and adult peripheral blood mononuclear cells was stimulated for 4 h with either HBV, BCG or (BCG + HBV) and cells were harvested for quantitative real-time PCR analysis. Statistical significance was determined by repeated measure or ordinary one-way ANOVA with Holm-Sidak post hoc test (or their non-parametric equivalent Friedman or Kruskal–Wallis with Dunn post hoc test). *p < 0.05, **p < 0.01 of preterm vs. term vs. adult; #,+p < 0.05, ##,++p < 0.01 of groups indicated by the corresponding color, respectively, vs. saline or HBV. N = 4–5/group.
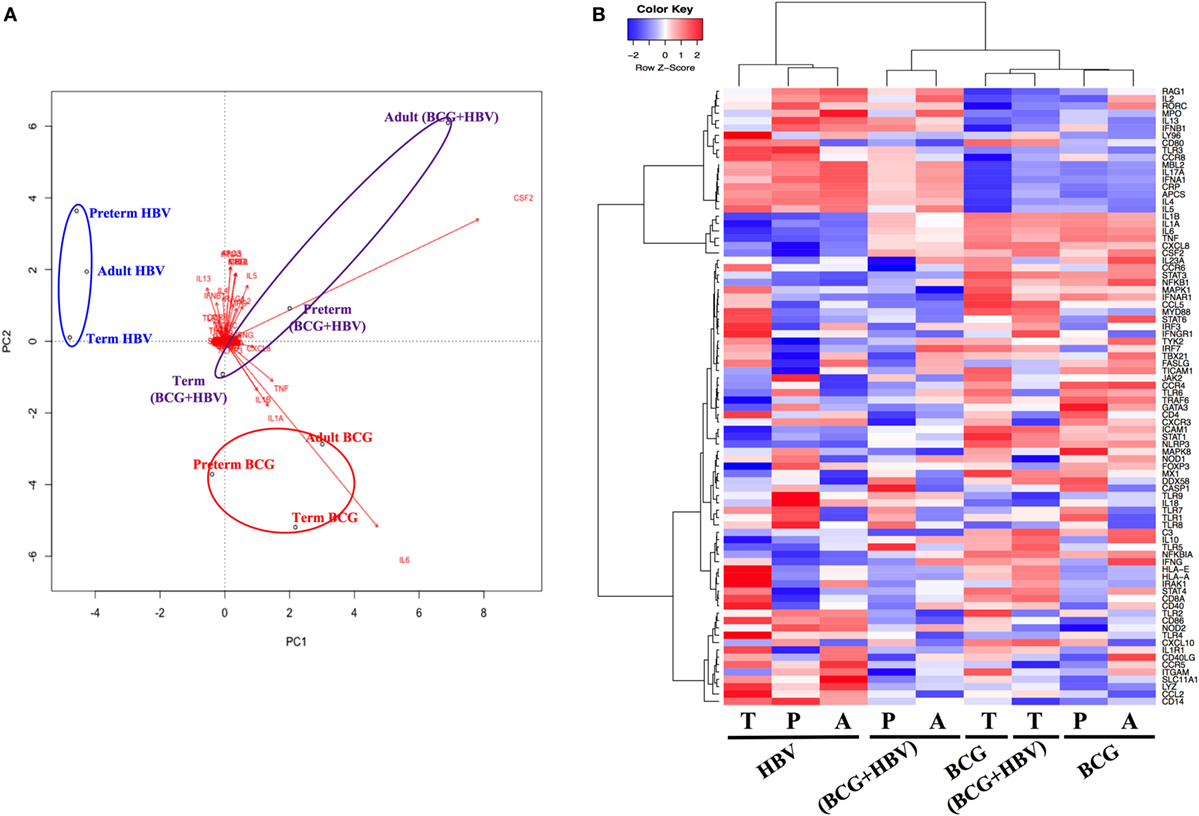
Figure 4. Principal component analysis of gene expression data reveals treatment-specific segregation of different age groups. Gene expression data were generated as outlined in Figure 3. (A) Principal components analysis biplot of mRNA gene expression data where observations (samples) are points and gene expression profiles are arrows shows dominant clustering of profiles by treatment. The distance between points approximates gene expression pattern differences among groupings. Arrows indicate genes that have greater biplot scores and drives the differences between groups. Arrowheads close to a particular group indicate genes are expressed at a greater relative abundance differences in those samples. (B) Unsupervised hierarchical heatmap shows clustering of treatments demonstrating log2-fold changes, expression values of genes in each sample; red to blue scale represent intensity of fold changes per genes (red indicates up, blue indicates down). Each row means individual gene and each column indicates groupings of age and treatment. P, preterm; T, term; A, adult.
(BCG + HBV) Significantly Enhance the Level of Anti-rHBsAg-Specific IgG Titers in Neonatal Mice (Immunized on DOL0 and DOL7) but Not Adult Mice
To assess the impact of (BCG + HBV) in vivo, we turned to a murine model. Mice at DOL 0–5 have been utilized as a model for preterm innate and adaptive immunity (49, 50) while mice at DOL7 have been used to model the term newborn (4, 15, 51–53). We made use of these age-specific models to investigate whether BCG can enhance early-life immunization with HBV, for which anti-Hepatitis B sAg Ab titers are the established correlate of protection (54). Figure 5A demonstrates the schedule according to which the mice of all three age groups were prime-immunized and booster immunized 2 weeks later. The age at prime immunization was DOL0 (the “preterm” group, n = 9–14), DOL7 (the “term” group, n = 14–16) and 6–8 weeks for the adult group (n = 15–17). These data were acquired in two separate experiments, each of which included all three age groups and within each age group all five treatment groups. The linear graphs represent median anti-rHBsAg IgG titers over time post-prime immunization (Figure 5B). The box-and-whisker plots depict Ab titers 21 and 42 days post-prime immunization. To assess whether the potential beneficial effect of addition of BCG to HBV depends on co-administration of both vaccines into the same site, we differentiated two combined treatment groups: one in which (BCG + HBV) were combined (i.e., admixed) and injected into the right flank s.c. and one in which HBV was injected into the right thigh and BCG was injected either into the scruff (neonatal mice on DOL0) or left thigh (neonatal mice on DOL7 and adult mice). Addition of BCG to HBV significantly enhanced Ab responses at 21 days post-prime immunization in neonatal mice immunized on DOL0 and DOL7 but not in adult mice. Whereas in DOL0 mice, it was the separate injection of BCG and HBV that significantly enhanced anti-rHBsAg IgG titers, the combined injection was the administration technique that lead to enhanced Ab titers in DOL7 mice. While this effect was sustained at D42 post-prime immunization in mice immunized on DOL0, it was no longer evident in neonatal mice prime-immunized on DOL7 (Figure 5C). Interestingly, switching toward IgG2c was observed only in adult mice immunized with (BCG + HBV) (Figure S1 in Supplementary Material). In addition, BCG did not significantly modulate Ab avidity, suggesting that BCG did not affect affinity maturation of anti-rHBsAg Abs (Figure S2 in Supplementary Material).
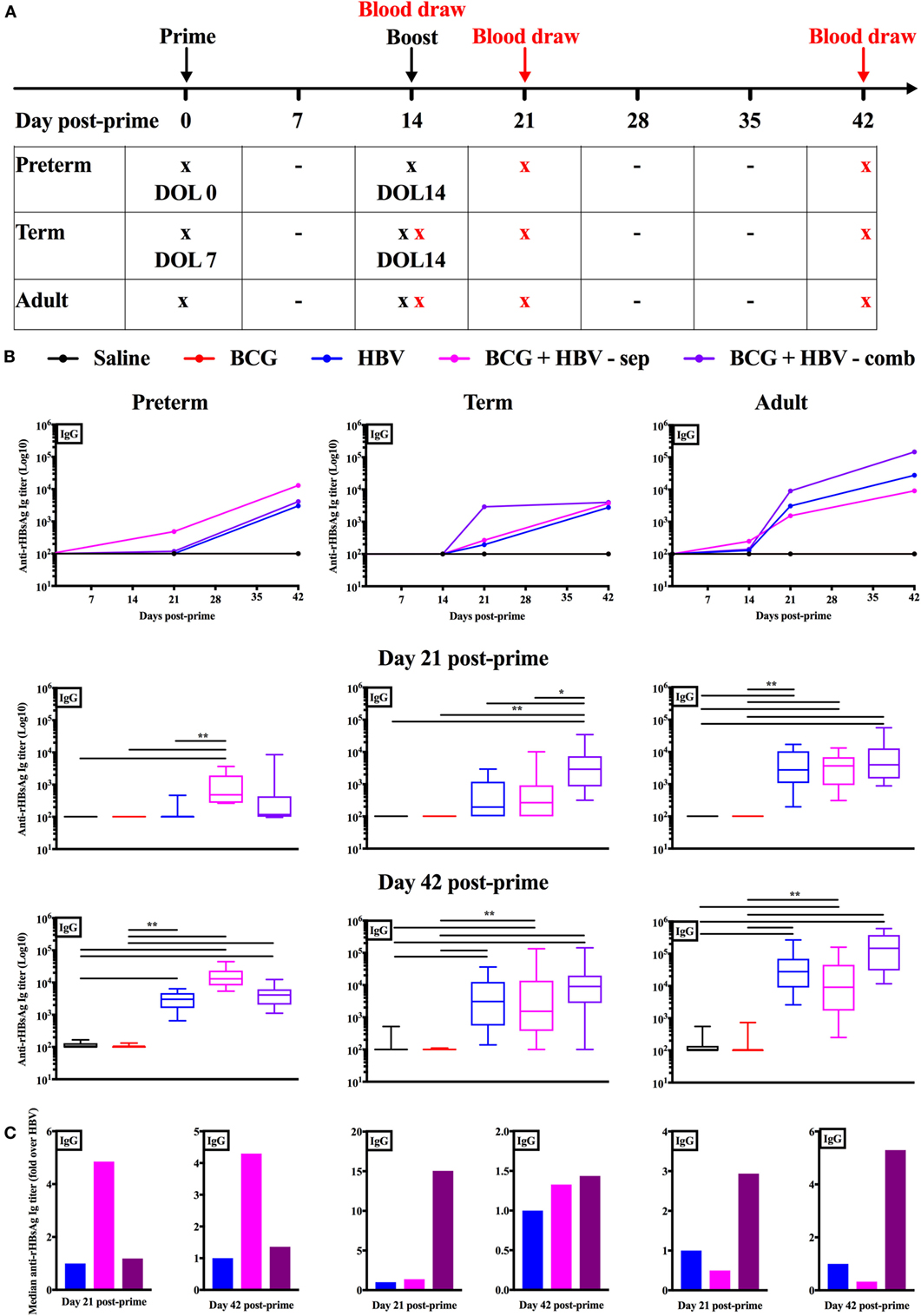
Figure 5. Combined immunization of newborn mice with Bacille Calmette–Guérin (BCG) and hepatitis B vaccine (HBV) in vivo enhances anti-recombinant HBV surface antigen (rHBsAg) IgG titers 21 days post-immunization. (A) Schematic representation of the immunization and blood draw schedule for the in vivo experiments: prime immunization was administered either on day of life (DOL) 0, DOL7, or at 6–8 weeks of life with either saline, BCG, HBV vaccine, BCG and HBV in a combined s.c. injection or BCG and HBV vaccine injected in separate sites. Booster immunization was performed with either saline in the mice prime-immunized with saline or BCG, or with HBV vaccine in the mice prime-immunized with either HBV vaccine alone, or BCG and HBV (either in combined or separate injection). Blood draws in the adult mice were obtained prior to prime immunization, prior to booster immunization, at 21 days and at 42 days post-prime immunization. Blood draws in the neonatal mice immunized on DOL7 were performed prior to booster immunization, at 21 days and at 42 days post-prime immunization. Blood draws in the mice immunized on DOL0 were obtained at 21 days and at 42 days post-prime immunization. Some neonatal mice from both the group immunized on DOL0 as well as DOL7 were sacrificed for a baseline blood draw prior to prime immunization. (B) Anti-rHBsAg IgG titers in mice immunized on DOL0, mice immunized on DOL7 and at 6–8 weeks of life. (C) Fold change over the HBV vaccine immunized group of the median anti-rHBsAg IgG titers. N = 9–14/group for the mice immunized on DOL0; N = 14–16/group for the mice immunized on DOL7; N = 15–17/group for the mice immunized at 6–8 weeks of life. Data are representative of two independent experiments each of which included all three age groups and within each age group all five treatment groups. Statistical analysis of differences between the treatment groups was performed via Kruskal–Wallis test with Dunn’s post hoc test. *p < 0.05, **p < 0.01.
Discussion
In this study, we have demonstrated for the first time that BCG, alone or when coadministered with HBV in a neonatal context, can enhance human innate cytokine responses in vitro. Moreover, we show that BCG can enhance hepatitis B antigen-specific murine adaptive responses in vivo. These observations are important in that newborns and young infants are highly susceptible to infection with intracellular pathogens including viruses, such as hepatitis B virus. Acquisition of hepatitis B virus during the newborn period carries risks of developing both hepatocellular carcinoma and liver cirrhosis. Moreover, most licensed vaccines, including HBV, are not optimally effective at birth and require multiple booster immunizations later in life despite the fact that hepatitis B immunization in newborns induces higher primary and memory Ab responses than in adults (55). Under the current immunization schedule with an ALUM adjuvanted HBV vaccine, the term newborn does not reach a status of immunological protection against HBV, as measured by titers of anti-rHBsAg Abs, until 6 months of life. For the preterm newborn with a birth weight <2 kg the unmet need to enhance responses to HBV is exacerbated by the fact that a birth dose is not recommended as priming has been inefficient in this population. With age-specific differences in the quantity and quality of cellular and soluble factors playing a role (56), the neonatal immune system is distinct from that of infants and adults, with bias toward induction of regulatory T cell and Th2-type T cell responses. Distinct early-life immunity limits the efficacy of adjuvants that activate newborn DCs to produce Th1-polarizing cytokines. The preterm newborn, in addition to demonstrating low innate Th1 support, demonstrates a low TLR-mediated production of Th17-supporting cytokines, with robust anti-inflammatory IL-10 levels (57–60). Combined stimulation of newborn cells through certain combinations of PRRs may potentially overcome the early-life bias against Th1 responses (30, 38, 61). In summary, there is an unmet need for early-life vaccine strategies that provide earlier protection against HBV infection for the term and in particular for the preterm infant.
While HBV immunization of the term infant effectively reduces chronic Hepatitis B infection in countries with universal neonatal immunization, the window of vulnerability in term and preterm infants at birth (into infancy) is still a major concern. This concern could be addressed by maternal or an optimized (one dose) HBV immunization of the newborn at birth. The common goal among maternal vaccination programs is temporary protection of the young infant against severe illness and death by ensuring sufficient and timely transfer of protective antibodies from the mother (62). Unfortunately, while some attempt have been made, clinical studies of vaccination against hepatitis B have showed lower immunogenicity in pregnant women than in nonpregnant women (63, 64). Regarding improved immunization of the newborn, as the Center for Disease Control outlines, worldwide, most people with chronic Hepatitis B are infected at birth or during early childhood. While a positive mother is a significant source of risk for Hepatitis B infection as a newborn, the recommendation to administer Hepatitis B vaccination at birth in many countries including the United States is based on the understanding that asymptomatic chronic carriers within the family and family contacts can be a significant risk for infection in the neonatal period (ACIP, recommendations for Hepatitis B immunization).
BCG is the most commonly administered vaccine in world history with billions of doses given globally at or soon after birth to protect against disseminated TB during infancy (65). In addition to its protective effects against TB, for which there is no established correlate of protection, BCG administration may also enhance Ab responses to unrelated pathogens in human newborns and infants (20, 27, 28) and in newborn mice (26). Protective heterologous effects of certain live-attenuated vaccines including BCG have been demonstrated that reduce morbidity and mortality beyond what can be attributable to prevention of the target disease (23, 25). BCG activates TLRs, including TLR2 and TLR4 (66), as well as C-type lectin receptors (CLRs) such as Dectin-1 and macrophage-inducible C-type lectin (Mincle) (67–70), resulting in strong Th1-biased immune responses. Of note, combined TLR and CLR activation synergistically enhances Th1- and Th17-cytokine induction in human newborn monocyte-derived dendritic cells (38), raising the possibility that engagement of multiple PRRs by BCG may contribute to the observed enhancement of HBV immunogenicity. Accordingly, we examined the cytokine profiles induced by (BCG + HBV) relative to HBV alone in term and moderate to late preterm human infants on the protein and mRNA levels in vitro and then to examine the impact of cytokine polarization on the adaptive anti-rHBsAg-specific Ab production in vivo utilizing both mice on DOL0 as well as DOL7 to mirror different levels of immune ontogeny.
Our study characterized (BCG + HBV)-induced human leukocyte cytokine profiles at different GAs at the protein and mRNA level. Of these IL1B, IL6, CSF2, and TNF were key drivers of gene clustering in the principal component analysis by treatment instead of by age. The interleukins induced are particularly noteworthy as they have been associated with enhanced adaptive immunity: (a) IL-1β, whose production was synergistically induced by (BCG + HBV) in human preterm and term cord blood as well as peripheral adult blood in vitro, is an inflammasome-produced cytokine that may improve vaccine immunogenicity (42–45), having a role in neutrophil recruitment (71) and in Ab production (72) and has been used directly as an adjuvant (73). Indeed, IL-1β has been implicated as important to immunogenicity of HBV, in particular anti-HBsAg Ab responses (74–76) and (b) IL-6, a Th17-polarizing cytokine that stimulates differentiation and maturation of B cells to Ab-producing plasma cells, stimulates T cell proliferation, and is a murine adjuvant (46, 47, 77).
In neonatal mice, compared to HBV alone, we found that (BCG + HBV) induced significantly higher anti-rHBsAg-specific IgG levels at 21 days after prime immunization. In DOL0 mice, this effect was significant when BCG and HBV were injected into separate sites, in DOL7 mice this effect was significant when both vaccines were injected in combination. Although it should be recognized that the kinetics of immune ontogeny are distinct in mice compared to humans, this relatively early effect of BCG co-administration is intriguing. If such enhancing effects of BCG would extend to humans, they may fall within the window of susceptibility inherent to current term infant immunization schedules, prior to completion of HBV booster immunization. While in adult mice, the (BCG + HBV) group demonstrated higher Ab titers, the difference relative to HBV alone did not reach statistical significance. A surprising finding of this study was the observation that in the DOL0 mouse the separate injection of BCG and HBV compared to a combined injection in the same age group was the potent route of administration whereas in both the DOL7 mouse as well as in the adult mouse, the combined injection was more effective than the separate administration. This observation could reflect age-specific immunity and deserves further investigation. In summary, our study demonstrates that (BCG + HBV) synergistically induced IL-1β in vitro and enhanced neonatal anti-rHBsAg-specific Ab titers at an early stage post prime and first booster immunization in vivo. Given evidence in mice and humans that IL-1β production enhances the magnitude of HBV-induced Ab responses (74–76), we speculate that robust (BCG + HBV)-induced inflammasome activation may contribute to the observed enhancement in HBV immunogenicity.
Our study features many strengths including, to our knowledge, multiple novel aspects: (a) an age-specific approach to characterizing vaccine–vaccine interactions including study of preterm humans and newborn DOL0 mice, reflecting aspects of preterm humans who represent ~11% of all global live births and are particularly susceptible to infection (3); (b) human in vitro modeling of (BCG + HBV) effects, in a way that reflects immune ontological differences present in these age groups in vivo; (c) evaluating the impact of vaccine–vaccine interactions on innate cytokine induction using mathematical and bioinformatic approaches, and (d) characterizing age- and administration- (e.g., combined vs. separate injections) specific BCG-HBV interactions in newborn mice in vivo.
Our study also has a number of limitations. With regard to our in vitro systems, although providing potentially valuable human data that have predicted adjuvantation effects in vivo (13), they may not optimally reflect vaccine effects in vivo. The use of a whole blood assay aims to reflect in vivo conditions including differences in cell quantity and immunphenotype between the neonate and the adult. Consequently, whole blood data are limited with regard to the ability to ascribe cytokine differences to single cell function or cell composition (78). Moreover, there are differences in the functionality and composition of mononuclear cells from adult individuals and mononuclear cells derived from neonatal cord blood (e.g., more predominantly lymphocytic) (78). Potential confounders in the use of preterm cord blood include maternal disease such as preeclampsia, a disease not captured in our collection of data for the preterm cord blood samples. We were able to limit the effects of steroid exposure on our sample collection for a group of preterm cord blood samples as steroids were known to have been given over 48 h prior to cord blood collection (Table 1). We also recognize that attention must by paid to the type of anti-coagulant used for peripheral or cord blood collections as the type of cytokine used can affect cytokine production. We chose pyrogen-free anti-coagulant heparin sodium because it is certified to be endotoxin-free. Future studies may need to compare the results obtained with other methods of anti-coagulation, such as EDTA. Our in vivo studies feature distinct species (mouse) and route of administration (subcutaneous) from human newborns (intradermal). Indeed, we were not able to demonstrate higher primary anti-HBV Ab responses in our neonatal mice relative to our adult mice as has been previously demonstrated in humans (55). Nevertheless, the cogent pattern of enhanced age-dependent HBV responses in the presence of BCG, mirroring those observed in some clinical cohorts (20) suggests that our data may be relevant to the effects of these vaccines in human newborns in vivo.
Future work will be necessary to elucidate the immunological mechanisms involved in the BCG adjuvantation phenomenon described here, and hence enable design of a new generation of vaccines that recapitulate desirable features of the live vaccine BCG as (a) a single dose effectiveness and (b) induction of both adaptive and trained immunity. At this point, it is unclear whether the observed BCG-driven phenomena relate mechanistically to “heterologous” effects that could be mediated by trained immunity (79). In addition to informing optimization of the use of BCG vaccine together with other vaccines, characterizing BCG-induced enhancement of Ab titers in response to unrelated vaccines may inform development of “BCG-like” adjuvantation systems (12). Furthermore, of importance to global health, these findings support the hypothesis, that in the appropriate context in countries in which neonatal immunization with BCG is recommended, concurrent administration of (BCG + HBV) at birth to the moderate to late preterm and term newborn may enhance the protective response to HBV immunization. Of note, in relatively small preterm studies thus far, BCG has been immunogenic and safe when administered to the moderately to late preterm infant [31–33 weeks GA] (80). Further studies of the safety, efficacy and mechanism of action of the combination of (BCG + HBV) compared to each alone in newborn animals, including humans, will shed further light into this important area crucial to the protection of the most vulnerable among us.
Ethics Statement
Local institutional review boards at The Brigham and Women’s Hospital (Protocol #2000P000117/BWH) and the Beth Israel Deaconess Medical Center (Protocol #2011P-000118/BIDMC) have approved the cord blood collection protocols. Patient information concerning the collected cord blood samples was collected in a de-identified manner as approved by the onsite IRB. Peripheral blood draws were conducted at Boston Children’s Hospital (BCH) from healthy adult volunteers, employed at BCH after written informed consent with approval from the Ethics Committee of BCH (protocol number X07-05-0223). All experiments involving animals were approved by the Animal Care and Use Committee of BCH and Harvard Medical School (protocol numbers 15-11-3011 and 16-02-3130).
Author Contributions
AS, FB, DD, and OL designed the study. AS, DD, IB, and HC collected cord blood samples. AS, DD, MP, SJ, NL, and IB conducted the in vitro experiments. AS, FB, and CP conducted the in vivo experiments. JD-A performed the RNA data analysis. TK shared knowledge in the design of whole blood assays. AS and FB wrote the manuscript. DD and OL provided overall mentorship and assisted in writing and editing the manuscript. FB, CP, JD-A, MP, HC, SJ, NL, IB, and TK contributed to helpful discussions and review of the final manuscript. All the authors have given final approval for the version submitted for publication.
Conflict of Interest Statement
The authors declare that the research was conducted in the absence of any commercial or financial relationships that could be construed as a potential conflict of interest. The Levy Lab has received past sponsored research support from MedImmune, Crucell (Johnson & Johnson), and 3M Drug Delivery Systems that is not directly relevant to the content of this manuscript.
Acknowledgments
The authors are grateful for the mentorship and support of Drs. Terrie Inder, Linda Van Marter, Christiane Dammann, Jonathan Davis, Heber Nielsen, Camilia Martin, Michael Wessels, and Gary R. Fleisher. The authors thank the members of OL’s Laboratory for helpful discussions and assistance with phlebotomies.
Funding
OL’s laboratory is supported by US National Institutes of Health (NIH) grants 1R01AI100135-01 and 3R01AI067353-05S1, the National Institutes of Allergy and Infectious Diseases (NIAID), NIH, Department of Health and Human Services, NIH UO1 award Molecular Mechanisms of Combination Adjuvants (1U01AI124284-01), Adjuvant Discovery Program Contract No. HHSN272201400052C, the NIH (NIAID) Human Immunology Project Consortium award U19AI118608, as well as Global Health (OPPGH5284) and Grand Challenges Explorations (OPP1035192) awards from the Bill & Melinda Gates Foundation and an internal BCH award to the Precision Vaccines Program. FB was supported by UniNA and Compagnia di San Paolo, in the frame of Programme STAR. CP was supported by the scholarship “J. Miglierina,” Fondazione Comunitaria del Varesotto, Varese, Italy.
Supplementary Material
The Supplementary Material for this article can be found online at http://www.frontiersin.org/articles/10.3389/fimmu.2018.00029/full#supplementary-material.
References
1. Liu L, Johnson HL, Cousens S, Perin J, Scott S, Lawn JE, et al. Global, regional, and national causes of child mortality: an updated systematic analysis for 2010 with time trends since 2000. Lancet (2012) 379:2151–61. doi:10.1016/S0140-6736(12)60560-1
2. Blencowe H, Cousens S, Oestergaard MZ, Chou D, Moller AB, Narwal R, et al. National, regional, and worldwide estimates of preterm birth rates in the year 2010 with time trends since 1990 for selected countries: a systematic analysis and implications. Lancet (2012) 379:2162–72. doi:10.1016/S0140-6736(12)60820-4
3. Miller JE, Hammond GC, Strunk T, Moore HC, Leonard H, Carter KW, et al. Association of gestational age and growth measures at birth with infection-related admissions to hospital throughout childhood: a population-based, data-linkage study from Western Australia. Lancet Infect Dis (2016) 16:952–61. doi:10.1016/S1473-3099(16)00150-X
4. Dowling DJ, Levy O. Ontogeny of early life immunity. Trends Immunol (2014) 35:299–310. doi:10.1016/j.it.2014.04.007
5. Sanchez-Schmitz G, Levy O. Development of newborn and infant vaccines. Sci Transl Med (2011) 3:90ps27. doi:10.1126/scitranslmed.3001880
6. Dowling DJ, Levy O. Pediatric vaccine adjuvants: components of the modern vaccinologist’s toolbox. Pediatr Infect Dis J (2015) 34:1395–8. doi:10.1097/INF.0000000000000893
7. D’Angio CT. Active immunization of premature and low birth-weight infants: a review of immunogenicity, efficacy, and tolerability. Paediatr Drugs (2007) 9:17–32. doi:10.2165/00148581-200709010-00003
8. Esposito S, Serra D, Gualtieri L, Cesati L, Principi N. Vaccines and preterm neonates: why, when, and with what. Early Hum Dev (2009) 85:S43–5. doi:10.1016/j.earlhumdev.2009.08.011
9. Baxter D. Impaired functioning of immune defenses to infection in premature and term infants and their implications for vaccination. Hum Vaccin (2010) 6:494–505. doi:10.4161/hv.6.6.12008
10. Baxter D. Vaccine responsiveness in premature infants. Hum Vaccin (2010) 6:506–11. doi:10.4161/hv.6.6.12083
11. Linder N, Vishne TH, Levin E, Handsher R, Fink-Kremer I, Waldman D, et al. Hepatitis B vaccination: long-term follow-up of the immune response of preterm infants and comparison of two vaccination protocols. Infection (2002) 30:136–9. doi:10.1007/s15010-002-2068-3
12. Dowling DJ, Scott EA, Scheid A, Bergelson I, Joshi S, Pietrasanta C, et al. Toll-like receptor 8 agonist nanoparticles mimic immunomodulating effects of the live BCG vaccine and enhance neonatal innate and adaptive immune responses. J Allergy Clin Immunol (2017) 140(5):1339–50. doi:10.1016/j.jaci.2016.12.985
13. Dowling DJ, Van Haren SD, Scheid A, Bergelson I, Kim D, Mancuso CJ, et al. TLR7/8 adjuvant overcomes newborn hyporesponsiveness to pneumococcal conjugate vaccine at birth. JCI Insight (2017) 2:e91020. doi:10.1172/jci.insight.91020
14. Siegrist CA, Aspinall R. B-cell responses to vaccination at the extremes of age. Nat Rev Immunol (2009) 9:185–94. doi:10.1038/nri2508
15. Mastelic B, Kamath AT, Fontannaz P, Tougne C, Rochat AF, Belnoue E, et al. Environmental and T cell-intrinsic factors limit the expansion of neonatal follicular T helper cells but may be circumvented by specific adjuvants. J Immunol (2012) 189:5764–72. doi:10.4049/jimmunol.1201143
16. Lavoie PM, Huang Q, Jolette E, Whalen M, Nuyt AM, Audibert F, et al. Profound lack of interleukin (IL)-12/IL-23p40 in neonates born early in gestation is associated with an increased risk of sepsis. J Infect Dis (2010) 202:1754–63. doi:10.1086/657143
17. Schreibelt G, Klinkenberg LJ, Cruz LJ, Tacken PJ, Tel J, Kreutz M, et al. The C-type lectin receptor CLEC9A mediates antigen uptake and (cross-)presentation by human blood BDCA3+ myeloid dendritic cells. Blood (2012) 119:2284–92. doi:10.1182/blood-2011-08-373944
18. Kleinnijenhuis J, Van Crevel R, Netea MG. Trained immunity: consequences for the heterologous effects of BCG vaccination. Trans R Soc Trop Med Hyg (2015) 109:29–35. doi:10.1093/trstmh/tru168
19. Ritz N, Casalaz D, Donath S, Tebruegge M, Dutta B, Connell TG, et al. Comparable CD4 and CD8 T cell responses and cytokine release after at-birth and delayed BCG immunisation in infants born in Australia. Vaccine (2016) 34:4132–9. doi:10.1016/j.vaccine.2016.06.077
20. Ota MO, Vekemans J, Schlegel-Haueter SE, Fielding K, Sanneh M, Kidd M, et al. Influence of Mycobacterium bovis bacillus Calmette-Guerin on antibody and cytokine responses to human neonatal vaccination. J Immunol (2002) 168:919–25. doi:10.4049/jimmunol.168.2.919
21. Aaby P, Roth A, Ravn H, Napirna BM, Rodrigues A, Lisse IM, et al. Randomized trial of BCG vaccination at birth to low-birth-weight children: beneficial nonspecific effects in the neonatal period? J Infect Dis (2011) 204:245–52. doi:10.1093/infdis/jir240
22. Flanagan KL, Van Crevel R, Curtis N, Shann F, Levy O, Optimmunize N. Heterologous (“nonspecific”) and sex-differential effects of vaccines: epidemiology, clinical trials, and emerging immunologic mechanisms. Clin Infect Dis (2013) 57:283–9. doi:10.1093/cid/cit209
23. Aaby P, Kollmann TR, Benn CS. Nonspecific effects of neonatal and infant vaccination: public-health, immunological and conceptual challenges. Nat Immunol (2014) 15:895–9. doi:10.1038/ni.2961
24. Levy O, Netea MG. Innate immune memory: implications for development of pediatric immunomodulatory agents and adjuvanted vaccines. Pediatr Res (2014) 75:184–8. doi:10.1038/pr.2013.214
25. de Castro MJ, Pardo-Seco J, Martinon-Torres F. Nonspecific (heterologous) protection of neonatal BCG vaccination against hospitalization due to respiratory infection and sepsis. Clin Infect Dis (2015) 60:1611–9. doi:10.1093/cid/civ144
26. Brynjolfsson SF, Bjarnarson SP, Mori E, Del Giudice G, Jonsdottir I. Concomitant administration of Mycobacterium bovis BCG with the meningococcal C conjugate vaccine to neonatal mice enhances antibody response and protective efficacy. Clin Vaccine Immunol (2011) 18:1936–42. doi:10.1128/CVI.05247-11
27. Ritz N, Mui M, Balloch A, Curtis N. Non-specific effect of Bacille Calmette-Guerin vaccine on the immune response to routine immunisations. Vaccine (2013) 31:3098–103. doi:10.1016/j.vaccine.2013.03.059
28. Blakney AK, Tchakoute CT, Hesseling AC, Kidzeru EB, Jones CE, Passmore JA, et al. Delayed BCG vaccination results in minimal alterations in T cell immunogenicity of acellular pertussis and tetanus immunizations in HIV-exposed infants. Vaccine (2015) 33:4782–9. doi:10.1016/j.vaccine.2015.07.096
29. Curtis N. Potential role for BCG in treatment of autoimmune diseases. BMJ (2016) 355:i6091. doi:10.1136/bmj.i6091
30. Philbin VJ, Dowling DJ, Gallington LC, Cortes G, Tan Z, Suter EE, et al. Imidazoquinoline toll-like receptor 8 agonists activate human newborn monocytes and dendritic cells through adenosine-refractory and caspase-1-dependent pathways. J Allergy Clin Immunol (2012) 130:195–204.e9. doi:10.1016/j.jaci.2012.02.042
31. Blimkie D, Fortuno ES III, Yan H, Cho P, Ho K, Turvey SE, et al. Variables to be controlled in the assessment of blood innate immune responses to toll-like receptor stimulation. J Immunol Methods (2011) 366:89–99. doi:10.1016/j.jim.2011.01.009
32. Dowling DJ, Tan Z, Prokopowicz ZM, Palmer CD, Matthews MA, Dietsch GN, et al. The ultra-potent and selective TLR8 agonist VTX-294 activates human newborn and adult leukocytes. PLoS One (2013) 8:e58164. doi:10.1371/journal.pone.0058164
33. Dowling DJ, Sanders H, Cheng WK, Joshi S, Brightman S, Bergelson I, et al. A meningococcal outer membrane vesicle vaccine incorporating genetically attenuated endotoxin dissociates inflammation from immunogenicity. Front Immunol (2016) 7:562. doi:10.3389/fimmu.2016.00562
34. Weeratna RD, Brazolot Millan CL, Mccluskie MJ, Siegrist CA, Davis HL. Priming of immune responses to hepatitis B surface antigen in young mice immunized in the presence of maternally derived antibodies. FEMS Immunol Med Microbiol (2001) 30:241–7. doi:10.1111/j.1574-695X.2001.tb01577.x
35. Mastelic Gavillet B, Eberhardt CS, Auderset F, Castellino F, Seubert A, Tregoning JS, et al. MF59 mediates its B cell adjuvanticity by promoting T follicular helper cells and thus germinal center responses in adult and early life. J Immunol (2015) 194:4836–45. doi:10.4049/jimmunol.1402071
36. Lofano G, Mancini F, Salvatore G, Cantisani R, Monaci E, Carrisi C, et al. Oil-in-water emulsion MF59 increases germinal center B cell differentiation and persistence in response to vaccination. J Immunol (2015) 195:1617–27. doi:10.4049/jimmunol.1402604
37. Berenbaum MC. Correlations between methods for measurement of synergy. J Infect Dis (1980) 142:476–80. doi:10.1093/infdis/142.3.476
38. van Haren SD, Dowling DJ, Foppen W, Christensen D, Andersen P, Reed SG, et al. Age-specific adjuvant synergy: dual TLR7/8 and mincle activation of human newborn dendritic cells enables Th1 polarization. J Immunol (2016) 197:4413–24. doi:10.4049/jimmunol.1600282
39. Speer EM, Dowling DJ, Ozog LS, Xu J, Yang J, Kennady G, et al. Pentoxifylline inhibits TLR- and inflammasome-mediated in vitro inflammatory cytokine production in human blood with greater efficacy and potency in newborns. Pediatr Res (2017) 81:806–16. doi:10.1038/pr.2017.6
40. van Haren SD, Ganapathi L, Bergelson I, Dowling DJ, Banks M, Samuels RC, et al. In vitro cytokine induction by TLR-activating vaccine adjuvants in human blood varies by age and adjuvant. Cytokine (2016) 83:99–109. doi:10.1016/j.cyto.2016.04.001
41. Harte C, Gorman AL, Mccluskey S, Carty M, Bowie AG, Scott CJ, et al. Alum activates the bovine NLRP3 inflammasome. Front Immunol (2017) 8:1494. doi:10.3389/fimmu.2017.01494
42. Ben-Sasson SZ, Hu-Li J, Quiel J, Cauchetaux S, Ratner M, Shapira I, et al. IL-1 acts directly on CD4 T cells to enhance their antigen-driven expansion and differentiation. Proc Natl Acad Sci U S A (2009) 106:7119–24. doi:10.1073/pnas.0902745106
43. Ben-Sasson SZ, Hogg A, Hu-Li J, Wingfield P, Chen X, Crank M, et al. IL-1 enhances expansion, effector function, tissue localization, and memory response of antigen-specific CD8 T cells. J Exp Med (2013) 210:491–502. doi:10.1084/jem.20122006
44. Schenten D, Nish SA, Yu S, Yan X, Lee HK, Brodsky I, et al. Signaling through the adaptor molecule MyD88 in CD4+ T cells is required to overcome suppression by regulatory T cells. Immunity (2014) 40:78–90. doi:10.1016/j.immuni.2013.10.023
45. Ritvo PG, Churlaud G, Quiniou V, Florez L, Brimaud F, Fourcade G, et al. Tfr cells lack IL-2Ralpha but express decoy IL-1R2 and IL-1Ra and suppress the IL-1-dependent activation of Tfh cells. Sci Immunol (2017) 2(15):eaan0368. doi:10.1126/sciimmunol.aan0368
46. Muraguchi A, Hirano T, Tang B, Matsuda T, Horii Y, Nakajima K, et al. The essential role of B cell stimulatory factor 2 (BSF-2/IL-6) for the terminal differentiation of B cells. J Exp Med (1988) 167:332–44. doi:10.1084/jem.167.2.332
47. Van Snick J. Interleukin-6: an overview. Annu Rev Immunol (1990) 8:253–78. doi:10.1146/annurev.iy.08.040190.001345
48. Clive KS, Tyler JA, Clifton GT, Holmes JP, Mittendorf EA, Ponniah S, et al. Use of GM-CSF as an adjuvant with cancer vaccines: beneficial or detrimental? Expert Rev Vaccines (2010) 9:519–25. doi:10.1586/erv.10.40
49. Kronforst KD, Mancuso CJ, Pettengill M, Ninkovic J, Power Coombs MR, Stevens C, et al. A neonatal model of intravenous Staphylococcus epidermidis infection in mice <24 h old enables characterization of early innate immune responses. PLoS One (2012) 7:e43897. doi:10.1371/journal.pone.0043897
50. Bi D, Qiao L, Bergelson I, Ek CJ, Duan L, Zhang X, et al. Staphylococcus epidermidis bacteremia induces brain injury in neonatal mice via toll-like receptor 2-dependent and -independent pathways. J Infect Dis (2015) 212:1480–90. doi:10.1093/infdis/jiv231
51. Barrios C, Brandt C, Berney M, Lambert PH, Siegrist CA. Partial correction of the TH2/TH1 imbalance in neonatal murine responses to vaccine antigens through selective adjuvant effects. Eur J Immunol (1996) 26:2666–70. doi:10.1002/eji.1830261118
52. Siegrist CA. Neonatal and early life vaccinology. Vaccine (2001) 19:3331–46. doi:10.1016/S0264-410X(01)00028-7
53. Siegrist CA. The challenges of vaccine responses in early life: selected examples. J Comp Pathol (2007) 137(Suppl 1):S4–9. doi:10.1016/j.jcpa.2007.04.004
54. Plotkin SA. Complex correlates of protection after vaccination. Clin Infect Dis (2013) 56:1458–65. doi:10.1093/cid/cit048
55. Ota MO, Vekemans J, Schlegel-Haueter SE, Fielding K, Whittle H, Lambert PH, et al. Hepatitis B immunisation induces higher antibody and memory Th2 responses in new-borns than in adults. Vaccine (2004) 22:511–9. doi:10.1016/j.vaccine.2003.07.020
56. Pettengill MA, Van Haren SD, Levy O. Soluble mediators regulating immunity in early life. Front Immunol (2014) 5:457. doi:10.3389/fimmu.2014.00457
57. Strunk T, Richmond P, Simmer K, Currie A, Levy O, Burgner D. Neonatal immune responses to coagulase-negative staphylococci. Curr Opin Infect Dis (2007) 20:370–5. doi:10.1097/QCO.0b013e3281a7ec98
58. Strunk T, Power Coombs MR, Currie AJ, Richmond P, Golenbock DT, Stoler-Barak L, et al. TLR2 mediates recognition of live Staphylococcus epidermidis and clearance of bacteremia. PLoS One (2010) 5:e10111. doi:10.1371/journal.pone.0010111
59. Kollmann TR, Levy O, Montgomery RR, Goriely S. Innate immune function by toll-like receptors: distinct responses in newborns and the elderly. Immunity (2012) 37:771–83. doi:10.1016/j.immuni.2012.10.014
60. Strunk T, Prosser A, Levy O, Philbin V, Simmer K, Doherty D, et al. Responsiveness of human monocytes to the commensal bacterium Staphylococcus epidermidis develops late in gestation. Pediatr Res (2012) 72:10–8. doi:10.1038/pr.2012.48
61. Levy O, Suter EE, Miller RL, Wessels MR. Unique efficacy of toll-like receptor 8 agonists in activating human neonatal antigen-presenting cells. Blood (2006) 108:1284–90. doi:10.1182/blood-2005-12-4821
62. Marchant A, Sadarangani M, Garand M, Dauby N, Verhasselt V, Pereira L, et al. Maternal immunisation: collaborating with mother nature. Lancet Infect Dis (2017) 17:e197–208. doi:10.1016/S1473-3099(17)30229-3
63. Grosheide PM, Schalm SW, Van Os HC, Fetter WP, Heijtink RA. Immune response to hepatitis B vaccine in pregnant women receiving post-exposure prophylaxis. Eur J Obstet Gynecol Reprod Biol (1993) 50:53–8. doi:10.1016/0028-2243(93)90164-8
64. Gupta I, Ratho RK. Immunogenicity and safety of two schedules of hepatitis B vaccination during pregnancy. J Obstet Gynaecol Res (2003) 29:84–6. doi:10.1046/j.1341-8076.2002.00076.x
65. Gengenbacher M, Nieuwenhuizen NE, Kaufmann S. BCG – old workhorse, new skills. Curr Opin Immunol (2017) 47:8–16. doi:10.1016/j.coi.2017.06.007
66. Heldwein KA, Liang MD, Andresen TK, Thomas KE, Marty AM, Cuesta N, et al. TLR2 and TLR4 serve distinct roles in the host immune response against Mycobacterium bovis BCG. J Leukoc Biol (2003) 74:277–86. doi:10.1189/jlb.0103026
67. Yadav M, Schorey JS. The beta-glucan receptor dectin-1 functions together with TLR2 to mediate macrophage activation by mycobacteria. Blood (2006) 108:3168–75. doi:10.1182/blood-2006-05-024406
68. Matsunaga I, Moody DB. Mincle is a long sought receptor for mycobacterial cord factor. J Exp Med (2009) 206:2865–8. doi:10.1084/jem.20092533
69. Schoenen H, Bodendorfer B, Hitchens K, Manzanero S, Werninghaus K, Nimmerjahn F, et al. Cutting edge: mincle is essential for recognition and adjuvanticity of the mycobacterial cord factor and its synthetic analog trehalose-dibehenate. J Immunol (2010) 184:2756–60. doi:10.4049/jimmunol.0904013
70. Jensen KJ, Larsen N, Biering-Sorensen S, Andersen A, Eriksen HB, Monteiro I, et al. Heterologous immunological effects of early BCG vaccination in low-birth-weight infants in Guinea-Bissau: a randomized-controlled trial. J Infect Dis (2015) 211:956–67. doi:10.1093/infdis/jiu508
71. Oleszycka E, Moran HB, Tynan GA, Hearnden CH, Coutts G, Campbell M, et al. IL-1alpha and inflammasome-independent IL-1beta promote neutrophil infiltration following alum vaccination. FEBS J (2016) 283:9–24. doi:10.1111/febs.13546
72. Nakae S, Asano M, Horai R, Iwakura Y. Interleukin-1 beta, but not interleukin-1 alpha, is required for T-cell-dependent antibody production. Immunology (2001) 104:402–9. doi:10.1046/j.1365-2567.2001.01337.x
73. Staats HF, Ennis FA Jr. IL-1 is an effective adjuvant for mucosal and systemic immune responses when coadministered with protein immunogens. J Immunol (1999) 162:6141–7.
74. Kuriyama S, Tsujii T, Ishizaka S, Kikuchi E, Kinoshita K, Nishimura K, et al. Enhancing effects of oral adjuvants on anti-HBs responses induced by hepatitis B vaccine. Clin Exp Immunol (1988) 72:383–9.
75. Yucesoy B, Sleijffers A, Kashon M, Garssen J, De Gruijl FR, Boland GJ, et al. IL-1beta gene polymorphisms influence hepatitis B vaccination. Vaccine (2002) 20:3193–6. doi:10.1016/S0264-410X(02)00267-0
76. Sleijffers A, Yucesoy B, Kashon M, Garssen J, De Gruijl FR, Boland GJ, et al. Cytokine polymorphisms play a role in susceptibility to ultraviolet B-induced modulation of immune responses after hepatitis B vaccination. J Immunol (2003) 170:3423–8. doi:10.4049/jimmunol.170.6.3423
77. Larsen DL, Dybdahl-Sissoko N, Mcgregor MW, Drape R, Neumann V, Swain WF, et al. Coadministration of DNA encoding interleukin-6 and hemagglutinin confers protection from influenza virus challenge in mice. J Virol (1998) 72:1704–8.
78. Kollmann TR, Crabtree J, Rein-Weston A, Blimkie D, Thommai F, Wang XY, et al. Neonatal innate TLR-mediated responses are distinct from those of adults. J Immunol (2009) 183:7150–60. doi:10.4049/jimmunol.0901481
79. Smith SG, Kleinnijenhuis J, Netea MG, Dockrell HM. Whole blood profiling of bacillus Calmette-Guerin-induced trained innate immunity in infants identifies epidermal growth factor, IL-6, platelet-derived growth factor-AB/BB, and natural killer cell activation. Front Immunol (2017) 8:644. doi:10.3389/fimmu.2017.00644
Keywords: Bacille Calmette–Guérin, hepatitis B vaccine, preterm, newborn, innate cytokine profiles, HBV-specific antibodies
Citation: Scheid A, Borriello F, Pietrasanta C, Christou H, Diray-Arce J, Pettengill MA, Joshi S, Li N, Bergelson I, Kollmann T, Dowling DJ and Levy O (2018) Adjuvant Effect of Bacille Calmette–Guérin on Hepatitis B Vaccine Immunogenicity in the Preterm and Term Newborn. Front. Immunol. 9:29. doi: 10.3389/fimmu.2018.00029
Received: 15 October 2017; Accepted: 04 January 2018;
Published: 24 January 2018
Edited by:
Kirsty Le Doare, Imperial College London, United KingdomReviewed by:
Fabienne Willems, Université libre de Bruxelles, BelgiumJames Lawrence Wynn, University of Florida, United States
Copyright: © 2018 Scheid, Borriello, Pietrasanta, Christou, Diray-Arce, Pettengill, Joshi, Li, Bergelson, Kollmann, Dowling and Levy. This is an open-access article distributed under the terms of the Creative Commons Attribution License (CC BY). The use, distribution or reproduction in other forums is permitted, provided the original author(s) or licensor are credited and that the original publication in this journal is cited, in accordance with accepted academic practice. No use, distribution or reproduction is permitted which does not comply with these terms.
*Correspondence: Annette Scheid, YXNjaGVpZEBid2guaGFydmFyZC5lZHU=, YW5uZXR0ZS5zY2hlaWRAY2hpbGRyZW5zLmhhcnZhcmQuZWR1;
Ofer Levy, b2Zlci5sZXZ5QGNoaWxkcmVucy5oYXJ2YXJkLmVkdQ==
†These authors have contributed equally to this work.