- 1Department of Immunology and Oncology, Centro Nacional de Biotecnologia (CNB-CSIC), Madrid, Spain
- 2Department of Cellular and Molecular Medicine, Centro de Investigaciones Biologicas (CIB-CSIC), Madrid, Spain
Antibodies have proven their high value in antitumor therapy over the last two decades. They are currently being used as the first-choice to treat some of the most frequent metastatic cancers, like HER2+ breast cancers or colorectal cancers, currently treated with trastuzumab (Herceptin) and bevacizumab (Avastin), respectively. The impressive therapeutic success of antibodies inhibiting immune checkpoints has extended the use of therapeutic antibodies to previously unanticipated tumor types. These anti-immune checkpoint antibodies allowed the cure of patients devoid of other therapeutic options, through the recovery of the patient’s own immune response against the tumor. In this review, we describe how the antibody-based therapies will evolve, including the use of antibodies in combinations, their main characteristics, advantages, and how they could contribute to significantly increase the chances of success in cancer therapy. Indeed, novel combinations will consist of mixtures of antibodies against either different epitopes of the same molecule or different targets on the same tumor cell; bispecific or multispecific antibodies able of simultaneously binding tumor cells, immune cells or extracellular molecules; immunomodulatory antibodies; antibody-based molecules, including fusion proteins between a ligand or a receptor domain and the IgG Fab or Fc fragments; autologous or heterologous cells; and different formats of vaccines. Through complementary mechanisms of action, these combinations could contribute to elude the current limitations of a single antibody which recognizes only one particular epitope. These combinations may allow the simultaneous attack of the cancer cells by using the help of the own immune cells and exerting wider therapeutic effects, based on a more specific, fast, and robust response, trying to mimic the action of the immune system.
Introduction
Nowadays, the therapeutic activity of antibodies in oncology has been widely demonstrated (1–6), being these proteins, after chemotherapy, radiotherapy, and small molecule inhibitors, one of the most used drugs for oncological treatments (7, 8). Most of the antibodies used on antitumor immuno-therapies had positive health effects as long as the antibody is present in the patient’s blood. The clinical use of antibodies directed against antigens not present on the tumor cells, but on cells of the immune system (i.e., anti-immune checkpoint antibodies), evidenced the beneficial effects of the treatment, which persisted even after it was finished (9, 10). These findings allowed to demonstrate that the anti-checkpoint antibodies were able to reprogram the organism’s response, re-directing the antitumor immune response, and skewing the balance on the tumor microenvironment toward immune destruction of the tumor. Thus, allowing to envisage a cure for cancer.
The aim of this review is to discuss the information on the possible anti-cancer treatments using monoclonal antibodies (mAbs; in clinical trials or already in the market) in combinations, either with other antibodies or with other biological agents (11–18). The clinical trials are mentioned throughout this review only as examples of the different types of combinations being currently analyzed for cancer treatment. Thus, for most of the studies details and results will be shown on Supplementary Tables S1 and S2. The use of radiation therapy, chemotherapy, small-molecule compounds, or stem cell autotransplants will be mentioned only, if the information is strictly necessary in our attempt to dissect the reasons behind the use of these combinations. Similarly, there is no specific section describing combinations including checkpoint inhibitory mAbs, since this subject has been recently reviewed (19–24), including the reviews by Xu-Monette and Young and Aris et al., in this issue (25, 26). Similarly, combinations directed against cancer stem cells will not be discussed since they have been treated elsewhere in this research topic (27).
Antibodies are generated by the immune system’s adaptive arm to defend the organism from pathogens and malignant cells. The antibodies are basically secreted molecules involved in mediating interactions on the extracellular compartment; they are made by a variable part that gives its binding specificity, and a constant region that is able to interact with other molecules or cells of the innate and adaptive immune system, to give them molecular information regarding their interaction with antigen. Thus, it should come to no surprise that antibodies’ functions promote health and that treatments based on antibodies might, therefore, be curative (6, 28, 29).
Very few therapeutic antibodies are able to directly kill the tumor cells, either by interacting with a signal pathway (i.e., as a receptor antagonist or sequestering the ligand), or by direct triggering of apoptosis. Most of them kill the tumor cells through the interaction with other molecules or cells of the immune system, acting as molecules mediating interactions on the extracellular compartment. Although they originated as receptors on the surface of cells from the acquired immune system, they became secreted on mature B cells, and through either engagement with Fc-receptor-bearing cells or by interaction with the complement system, they can exert a broad spectrum of effector functions, coordinating the immune response (see Figure 1).
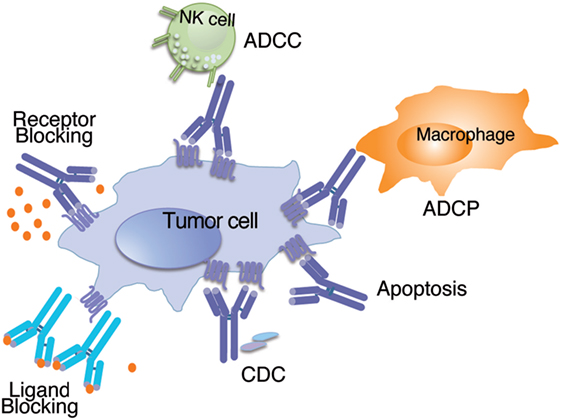
Figure 1. Schematic representation of the mechanisms of action used by naked antibodies to inhibit tumor growth. Naked antibodies can inhibit tumor growth through effector functions such as ADCC (antibody-dependent cell-cytotoxicity) where the antibody bound to the tumor antigen is recognized by the natural killer (NK) cell and triggers cytotoxic activity; can also trigger antibody-dependent cell-phagocytosis (ADCP) when the antibody bound to the tumor antigen opsonizes the cell and activates phagocytic cells; the antibody can also fix complement after binding to the tumor cell, and trigger complement-dependent cytotoxicity (CDC). Conversely, naked antibodies can kill the tumor cells by interfering with important signal pathways, either by binding to the ligand (Ligand Blocking) or by binding to the receptor (Receptor Blocking). In addition, they can trigger direct apoptosis after binding to an antigen on the tumor cell surface.
A current goal of antitumor immune therapies is to trigger, from the beginning, all the possible host body defense mechanisms. Aiming to destroy, as early as possible, the highest number of tumor cells, decreasing the possibilities of the tumor developing escape mechanisms, to obtain a more effective therapy. The defense mechanisms include (i) to directly kill the tumor cells; (ii) to switch the immune system from an antitumor immunosuppressed status to another that allows to attack the tumor, i.e., through stimulating the secretion of cytokines or modulating cell to cell interactions; (iii) to attract the immune system cells to the tumor; (iv) to decrease the tumor-directed neo-vascularization; and (v) to inhibit migration, metalloprotease secretion, and tumor cell invasion, among others.
The current trends for the use of antibodies in oncology as therapeutic agents are to employ them either alone or, more often, as combinations with (i) cytotoxic agents; (ii) radiotherapy; (iii) molecularly targeted drugs interfering with tumor cell survival or proliferation; (iv) other antibodies against the same target; (v) other antibodies against molecules implicated in the same signaling pathway; (vi) other antibodies, each one of them specific for unrelated targets (including targets in immune system cells and neo-vascularization); (vii) vaccines or oncolytic virus; (viii) cells that would either act as immunogens or as effector cells; or (ix) adjuvants, liposomes, nanoparticles, etc.
For the treatment of cancer, the FDA and the EMA (United States and European Union Drug Administrations), have approved (or are reviewing) a total of 32 therapeutic antibodies or their derivatives. Interestingly, the number has doubled between 2012 and 2017 (Tables 1 and 2), concomitant with a 100% increase in phase III clinical trials using mAb on a similar time-period (30). Twenty of these antibodies are indicated for treatment of patients with solid tumors (Table 1), identifying 13 different targets; whereas 12 are indicated for neoplasias of hematological origin (Table 2), identifying eight different targets. The targets identified by these antibodies are described in Table 3. Other therapeutic antibodies, not yet approved, but mentioned on this review are summarized on Table 4.
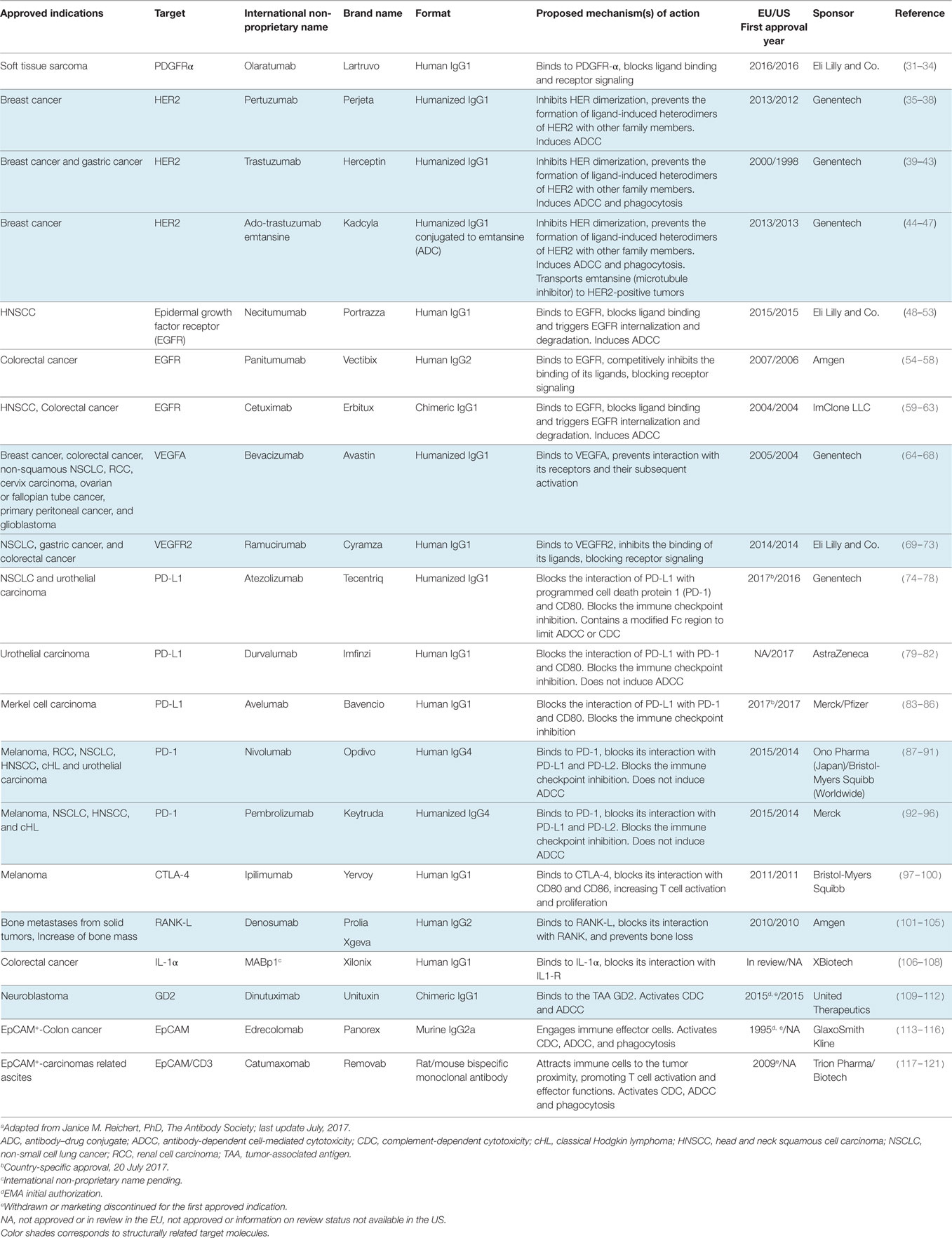
Table 1. Antibodies approved (or in review) by the FDA and/or EMA for the clinical treatment of solid tumors.a
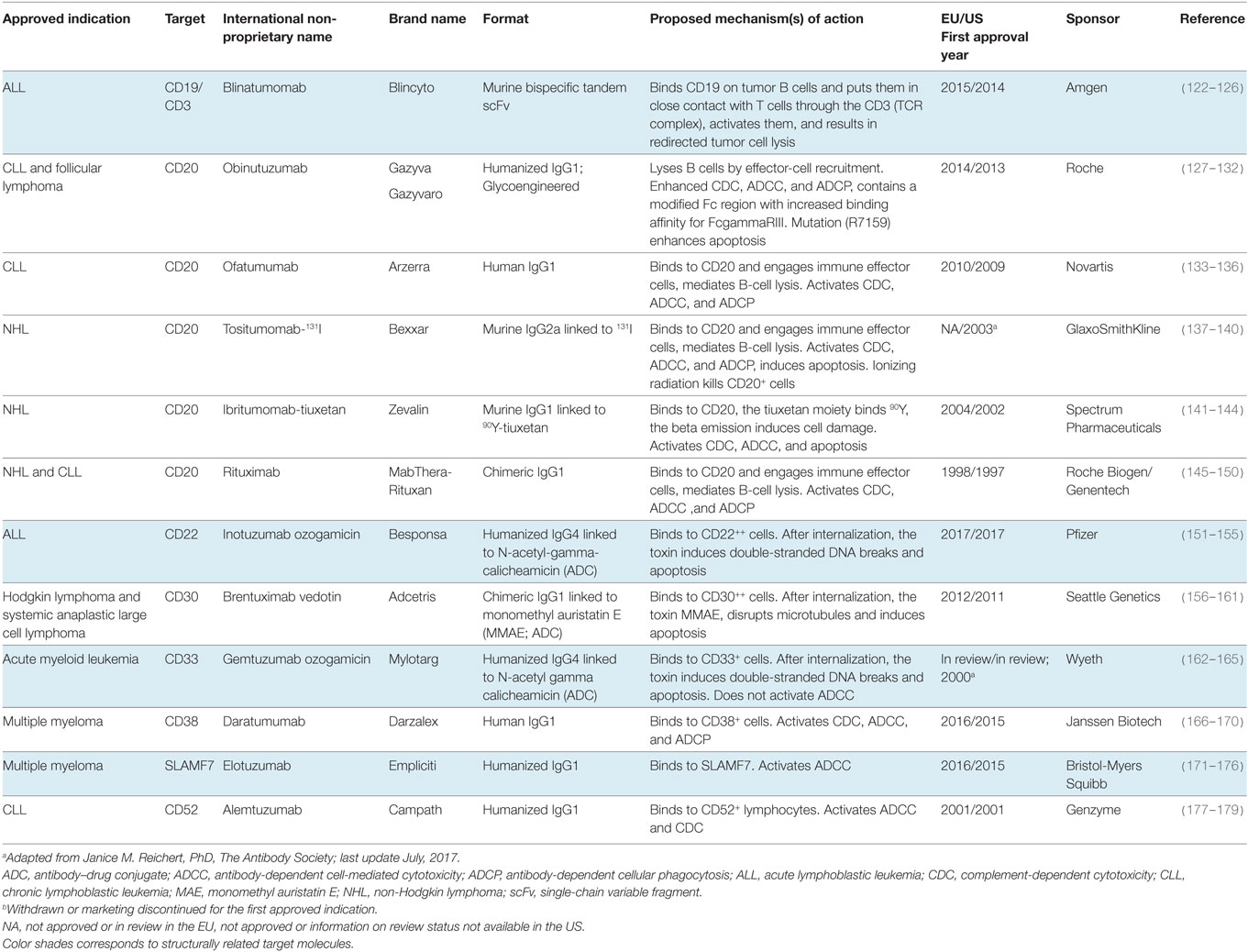
Table 2. Antibodies approved (or in review) by the FDA and/or EMA for the clinical treatment of hematologic neoplasias.a
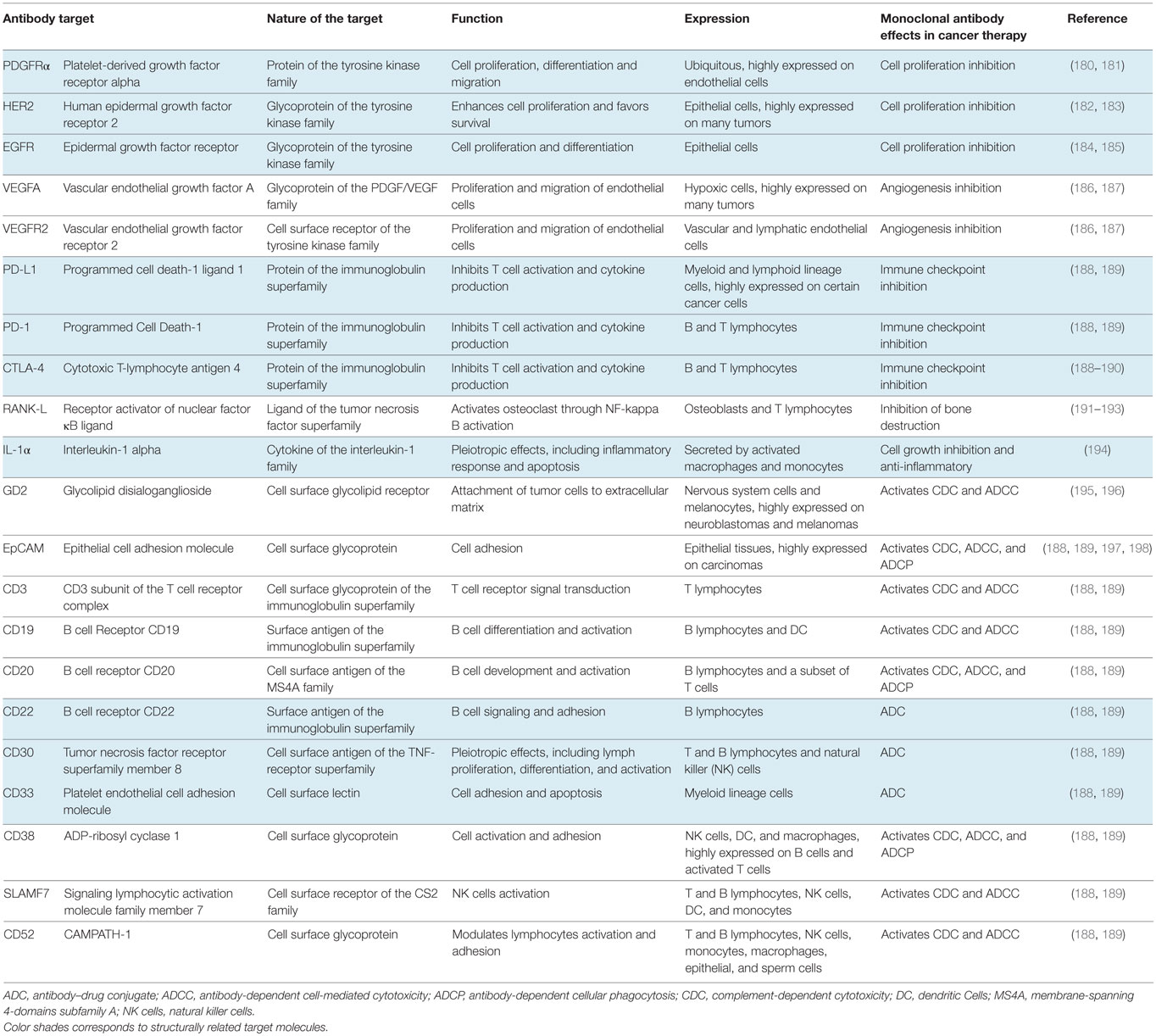
Table 3. Characteristics of the main target molecules identified by therapeutic antibodies used in oncology.
From the antibodies approved (or under review) for the treatment of solid tumors, seven of them recognize tumor cell surface tyrosine kinase receptors involved in proliferation and survival pathways. These receptors (see Table 3) are PDGFRα (targeted by the antibody olaratumab), HER2 (pertuzumab, trastuzumab and emtansine, ado-trastuzumab) and epidermal growth factor receptor (EGFR; necitumumab, panitumumab, and cetuximab). Two antibodies inhibit tumor angiogenesis by binding to the soluble ligand VEGF (bevacizumab) or to the endothelial cell receptor VEGFR2 (ramucirumab). Six of the mAb disrupt inhibitory immune checkpoint signals by binding to the programmed cell death protein 1 (PD-1) receptor on the T cells (nivolumab and pembrolizumab), to PD-L1 on the tumor cells (atezolizumab, durvalumab, and avelumab) or to CTLA-4 on T cells (ipilimumab). Two of these mAb block the binding of cytokines that are involved in the growth of some tumors, including antibodies against RANK-L (denosumab) and IL-1α (MABp1). The last three mAb recognize antigens overexpressed on the surface of tumor cells. They identify GD2 (dinutuximab) and EpCAM (edrecolomab and catumaxomab) (Table 1).
From the antibodies approved (or under review) for the treatment of hematopoietic neoplasias, eight antibodies recognize B cell antigens. Among those, one recognizes CD19 (blinatumomab), five mAb recognize CD20 (obinutuzumab, ofatumumab, rituximab, ibritumomab tiuxetan, and 131I tositumomab), one mAb binds to CD22 (inotuzumab ozogamicin), and the last one recognizes CD52 (alemtuzumab). Other two antibodies identify antigens expressed by B cells and by other cells of the immune system, including an anti-CD30 mAb (shared between B and T cells, brentuximab vedotin) and an anti-SLAMF7 (present on activated B cells and natural killer (NK) cells among others, elotuzumab). In addition, there are two antibodies against other immune cells, an anti-CD33 (myeloid lineage, gemtuzumab ozogamicin); and the non-lineage-restricted CD38 (daratumumab) (Table 2).
We will describe in the following paragraphs a set of clinical trials using antibodies in combination for oncological treatments, giving a systematic description of the antibody combinations with biological agents and their rationale. Describing the current aims of antibody-mediated cancer therapy and to envisage where its future lies. In addition, there will be a section where the therapeutic effects and toxicities for selected clinical trials will be discussed, which will help us to envisage the future of therapeutic antibodies for cancer treatments. Before starting with this systematic analysis, we will describe, with one example, in this case for the treatment of GD2+-neuroblastomas, the complexity of the clinical trials being carried out.
Evolution of Treatment Complexity with Antibody in Combinations
In this section, we will discuss, as an example, the use of anti GD2 antibodies for the treatment of GD2-positive solid tumors, including neuroblastoma (266–269). Near 50 clinical assays have been started using two different mouse antibodies and their corresponding chimeras or humanized antibodies. The clinical use of dinutuximab (ch14.18) was approved in 2015, whereas the therapeutic efficacy of the other antibody, 3F8 has been demonstrated with many patients (270–272). These antibodies, recognizing the neuroblastoma tumor-associated antigen GD2, are being used to (i) kill the tumor with either the naked antibody alone, apparently through Fc-mediated effector actions [antibody-dependent cell cytotoxicity (ADCC), complement-dependent cytotoxicity (CDC), and antibody-dependent cell phagocytosis (ADCP)] or apoptosis (NCT00002458, NCT00072358, NCT01418495, NCT01419834, NCT01704872, NCT02258815, NCT02743429); (ii) kill the tumor by the naked antibody in combination with chemotherapeutic agents (busulfan, carboplatin, cisplatin, cyclophosphamide, doxorubicin, etoposide, lomustine, melphalan, or vincristine), small molecule drugs (crizotinib), external radiation, and/or conventional surgery (NCT03098030, NCT03126916); (iii) directly transport a radioelement toward the tumor, by conjugating the radioelement to the anti-GD2 mAb. This will induce radiolysis of the tumor cells, minimizing the effects on normal cells (NCT00058370, NCT00445965, NCT03126916); (iv) use them in combination with agents that modify cell expression patterns, inhibiting proliferation and inducing cell differentiation and apoptosis [i.e., isotretinoin (13-cis retinoic acid or RA)] (NCT00003022, NCT00030719, NCT01183416, NCT01183429, NCT01183884, NCT01526603, NCT01711554, NCT02100930, NCT03033303); or (v) use them in combination with agents able to burst the host immune response against the tumor. These include, granulocyte-macrophage colony-stimulating factor (GM-CSF) or granulocyte colony-stimulating factor (G-CSF) (NCT01704716, NCT01767194, NCT02484443, NCT02502786, NCT03189706); which increase the number of innate immune response cells by triggering the proliferation of granulocytes and macrophages; increasing both innate and adaptive responses by inducing the maturation/proliferation of NK cells and T lymphocyte proliferation with interleukin-2 (IL-2) alone or in combination with GM-CSF and/or RA (NCT00005576, NCT00026312, NCT01041638, NCT01592045, NCT01662804, NCT02169609, NCT02641782); regulating the threshold of the immune response with an adjuvant, changing the secreted cytokine expression pattern of cells bearing certain pattern recognition receptors (i.e., beta glucan that binds the C-type lectin receptor Dectin-1) (NCT00037011, NCT00492167, NCT00089258); increasing the pool of cytotoxic cells able to fight the tumor with allogeneic NK cells (NCT00877110, NCT01857934, NCT02573896, NCT02650648); using in vitro activated T cells coated with bispecific OKT3-hu3F8 mAb, together with IL-2 and GM-CSF to redirect T lymphocyte cell lysis (NCT02173093); and combining the anti-GD2 antibody with nivolumab, an anti-immune checkpoint (PD-1) mAb able to block the immunosuppressor activity induced by the tumor (NCT02914405).
From these “basic” aims further combinations arose, for example one where the aim is to induce radiolysis of the tumor cells with 131I-3F8, simultaneously bursting the innate immune response with filgastrim (G-CSF), inhibiting neo-vascularization with bevacizumab (anti-VEGF), together with autologous stem cell rescue of irradiated patients (NCT00450827).
We believe that this example gave a rough idea of the complexity that clinical trials for one antibody (two in this case) can reach. The chimeric, human-murine, anti-GD2 mAb dinutuximab has been approved in combination with GM-CSF, IL-2, and retinoic acid for the treatment of pediatric patients with high-risk neuroblastoma (273). Interestingly, the overall survival and event-free survival of patients treated with dinutuximab increased 2 years when compared to standard treatment during phase III clinical trials (273).
Combination of Antibodies with Non-Biological Agents
Chemotherapeutic drugs are cytotoxic agents affecting unspecifically cell proliferation and survival, which inhibit topoisomerases I or II (doxorubicin, etoposide, irinotecan, topotecan, etc.), produce DNA breaks interfering with DNA replication, RNA transcription and cell division through changes in DNA alkylation, DNA methylation, and DNA cross-linking or intercalating between base pairs in the DNA helix (busulfan, melphalan, cyclophosphamide, carboplatin, cisplatin, lomustine, thiotepa, etc.). These chemotherapeutic drugs are being used in combination with mAbs for many cancer treatments (274).
In addition to surgery, treatment with antibodies and external irradiation has also been used. Localized external irradiation allows, by destroying tumor cells, better exposure of the tumor antigens to the immune system cells, this combination is also working well and is being used in numerous clinical trials (275–279).
Small molecule drugs that inhibit molecular interactions or enzymatic activity of proteins involved in cell signaling, or inhibitors of protein kinases overexpressed in tumor cells (including erlotinib, ibrutinib, imatinib, lapatinib, olaparib, regorafenib, ruxolitinib, sorafenib, sunitinib, etc.), are also being used in combination with antibodies (280, 281). There are numerous examples of treatments with this type of combinations that, by simultaneously inhibiting ligand–receptor interactions and kinases belonging to the same signaling pathway, have led to very positive therapeutic results (282–286).
Combination of Antibodies with Biological Agents
These are therapies that use a combination of antibodies or antibody-based molecules with other biological substances, for example, recombinant proteins, genetic material, virus, bacteria, and cells (16). Most of these strategies are designed to stimulate the host immune system to act against the cancer cells.
In the following paragraphs, we describe antibodies in combinations, where (i) one of the antibodies identifies a tumor-associated antigen (an antigen overexpressed in tumor cells), used either naked, as an antibody–drug conjugate (ADC) or as an immunotoxin; (ii) antibodies against the tumor cell are used in combination with cytokines or immunocytokines to burst the immune response against the tumor, or conversely use anti-cytokine antibodies when the expressed cytokines can be harmful for the antitumor response, aiming to disrupt their balance; (iii) the antibodies directly target the angiogenesis process, aiming to inhibit new vascularization required for tumor growth; (iv) the mAb can also be combined with effector cells to increase the immune response against the tumor; or (v) combined with antibodies against immunomodulatory or immunostimulatory proteins to disrupt the inhibitory signals sent by the tumor to the host immune system to inhibit the antitumor response. Although several of the examples we will describe could be included more than one subheading, each one of them is described only in one of them.
Antibodies Against Tumor-Associated Antigens
The rationale of using antibodies as therapeutic agents was to kill the tumor cells either directly or through activating the patient’s immune system effector functions (ADCC, CDC, or phagocytosis) with antibodies specific for tumor-associated antigens. Mucin 1 (MUC-1), an antigen present on the surface of many adenocarcinomas, which is recognized by mAb m170 (237). This mAb has been used radiolabeled as 111In-m170 or 90Y-m170, in combination with chemotherapy and the immunosuppressor cyclosporine to treat patients with metastatic prostate cancer that did not respond to hormone therapy. This treatment was followed by peripheral stem cell transplantation (NCT00009750). The rationale is to kill the tumor with the combination of chemotherapy and the mAb coupled to the radioisotope in the presence of cyclosporine. Afterward peripheral stem cell transplantation will allow to refurbish the hematopoietic compartment.
Other approaches have been used on hematological neoplasias, one of them, a combination of two anti-transferrin receptor (TfR) antibodies A27.15 and E2.3 (202, 287) was used for the treatment of chronic myeloproliferative disorders (NCT00003082). The anti-TfR mAb block the binding of (Fe3+)2-transferrin to TfR, resulting in decreased tumor cell growth. Other targets used in hematopoietic malignancies are CD20 and CD74. In this case, a combination of the anti-CD20 mAb veltuzumab (IMMU-106) (288) and milatuzumab (anti-CD74) (239) was used to treat relapsed or refractory B cell non-Hodgkin lymphoma (NCT00989586). CD74, a surface receptor of the pro-inflammatory cytokine macrophage migration inhibitory factor (MIF) (289–291), is an MHC class II chaperone and an accessory-signaling molecule (292). Milatuzumab induces apoptosis, related to inhibition of CD74 activation by MIF, ADCC, or CDC (293), while veltuzumab triggers CDC and ADCC in cells that overexpress CD20 (288). Other combinations include rituximab (anti-CD20) in combination with CC-90002 (anti-CD47) (294, 295) for the treatment of advanced solid and hematologic cancers (NCT02367196). CC-90002 selectively binds to CD47 expressed on tumor cells, blocks CD47 interaction with signal regulatory protein alpha (SIRPa), a protein expressed on phagocytic cells, which prevents CD47/SIRPa-mediated signaling and abrogates the CD47/SIRPa-mediated inhibition of phagocytosis. The result is an induction of pro-phagocytic signaling, resulting in macrophage activation and the specific phagocytosis of tumor cells. In addition, CD47 signaling blockade activates both, an antitumor T lymphocyte immune response and T cell-mediated killing of CD47-expressing tumor cells. CD47, also called integrin-associated protein (IAP), is a tumor-associated antigen (TAA) expressed on normal, healthy hematopoietic stem cells (HSC) and overexpressed on the surface of a variety of cancer cells. Expression of CD47 and its interaction with SIRPa leads to the inhibition of macrophage activation and protects cancer cells from phagocytosis, resulting in cancer cell proliferation (294, 296–298).
ADC and Immunotoxins
Some antitumor treatments use, rather than naked antibodies, antibody–toxin fusion proteins (immunotoxins) or antibodies linked to drugs (299–302). In some cases, to increase the cell-killing potential of antibodies, they can be covalently linked to potent cytotoxic or cytostatic agents, including small molecule drugs or inactive forms of a biological toxin. The antibody directs the toxin toward the tumor cell. When the cell endocytoses the ADC, it undergoes enzymatic cleavage and the drug is released, gets activated, and exerts its cytotoxic action, killing the tumor cell. The endocytic process works for antigens that can be internalized. Most of the current antibodies that are being used as ADC identify cell surface receptors that are efficiently endocytosed. However, many cell surface proteins are not internalized and a large amount of work is being carried out to develop alternatives, such as making an ADC where the antibody is coupled to the drug through a linker that can be cleaved by tumor cell-surface proteases, when in close contact with the tumor cell; conversely the antibody may carry a tumor receptor antagonist to direct it toward the tumor cell surface (303, 304).
One example includes a combination of an antitumor-associated antigen mAb and an ADC. Trastuzumab in combination with an antibody against the zinc transporter LIV-1 (SLC39A6) conjugated to the cytotoxic agent monomethyl auristatin E (MMAE) (257) for the treatment of patients with metastatic breast cancer (NCT01969643). In this particular combination, trastuzumab inhibits the tyr-kinase receptor HER2, while through the potent microtubule disrupting agent MMAE, which is coupled to the anti-LIV-1 antibody, induces cell cycle arrest in the G2/M phase and apoptosis of LIV-1+ cells (257). This type of combination can be made more potent by adding to the equation, antibodies against immune-checkpoints to burst antitumor immune responses. For example, the combination of nivolumab, ipilimumab, and rovalpituzumab tesirine has been used in extensive-stage small cell lung cancer (SCLC) (NCT03026166). Rovalpituzumab tesirine is an anti-delta-like protein 3 (DLL3) antibody conjugated to the cytotoxic pyrrolobenzodiazepine dimer D6.5 (256, 305). This antibody recognizes the membrane protein DLL3, which is overexpressed in certain tumors, binds to Notch receptors, and regulates Notch-mediated signaling (256). Thus, this combination should kill the cells overexpressing DLL3, while the anti-checkpoint antibodies nivolumab and ipilimumab redirect the host immune response to attack the tumor.
Another example shows the combination of mAb CR011, against the transmembrane protein GPNMB (glycoprotein non-metastatic B) coupled to MMAE (306). This ADC was used in combination with anti-PD-1 mAb nivolumab or pembrolizumab and varlilumab (307, 308), an agonistic anti-CD27 mAb, for the treatment of advanced melanoma (NCT02302339). The rationale is, in addition to targeting the GPNMB+ cells with the antibody-coupled to the toxin, the anti-PD-1 antibodies suppress the tumor-promoted inhibition of the antitumor immune response, while the anti-CD27 triggers an activation of the cytotoxic T lymphocytes (CTL).
The following example combines the ADC mirvetuximab soravtansine with either bevacizumab (VEGF) or pembrolizumab (PD-1) in primary peritoneal, fallopian tube, or endometrial cancer (NCT02606305). Mirvetuximab soravtansine is an immunoconjugate consisting of a folate receptor 1 (FOLR1) mAb (M9346A) conjugated to the cytotoxic maytansinoid DM4 (240). DM4 is released after internalization, binds to tubulin, and disrupts microtubule dynamics. FOLR1 is a member of the folate receptor family, overexpressed on a variety of epithelial-derived cancer cells (240, 309). Other studies combine nivolumab (anti-PD-1) with the ADC BMS-986148 (208, 310), composed of a mAb against the cell surface glycoprotein mesothelin (MSLN), conjugated to an as of yet undisclosed cytotoxic drug, for the treatment of mesothelioma, NSCLC, ovarian cancer, pancreatic cancer, and gastric cancer (NCT02341625). The rationale here is to block with the anti-PD-1 mAb the binding of PD-L1 (present on the tumor cells) to its receptor PD-1 (present on T cells), avoiding the suppression of antitumor responses triggered by the PD-L1/PD-1 interaction, while targeting mesothelin+ cells with the BMS-986148 mAb. Since the mAb is an ADC, upon internalization the cytotoxic agent kills the tumor cells. The mAb also activates ADCC. Another combination, for the treatment of MSLN-expressing NSCLC uses a combination of the anti-VEGF antibody bevacizumab with the single-chain anti-MSLN mAb SS1 (dsFv) linked to the exotoxin PE-38 from Pseudomonas (258) (NCT01051934). Since MSLN is not shed in significant amounts into the bloodstream, the dsFv-toxin can be concentrated onto the tumor cell surface. Once the dsFv toxin is internalized, the toxin is released and inactivates eukaryotic translation elongation factor 2, disrupting tumor cell protein synthesis. Concomitantly, the anti-VEGF antibody inhibits angiogenesis (258).
Antibodies Combined with Cytokines and Immunocytokines
Another way to burst the host immune response against the tumor involves the use of either exogenous cytokines or fusion proteins that include a cytokine, administered either systemically or directly in the tumor. In some cases, due to cytokine toxicity, it could be envisaged to directly couple the cytokine to a mAb specific for a tumor-associated antigen, as a recombinant fusion protein (311, 312). These combinations allow, decreasing the dose, to reach higher local concentrations at the tumor site and to exert its therapeutic function avoiding systemic toxicity, while increasing the cytokine’s half-life, since it is coupled to the antibody, which prevents renal clearance (313).
In another example for the treatment of advanced or metastatic solid tumors, a combination of nivolumab and the Aldesleukin Prodrug NKTR-214 was used (NCT02983045). NKTR-214 is a recombinant human IL-2 conjugated to six releasable polyethylene glycol chains (PEG) (314). When the cytokine is released, binds to CD122 (IL-2 receptor beta subunit) and the mAb may act synergistically with NKTR-214 by blocking PD-1 activation through the mAb and simultaneously stimulating growth and cytotoxic activity against the tumor of the patient’s T and NK cells by the exogenous IL-2. The advantages of using this conjugated form of the IL-2 are that, on the one side, is released in a controlled way in the tumor’s proximity, avoiding systemic toxic effects; and on the other side, PEG conjugation prevents IL-2 binding to the IL2Ralpha subunit (and the subsequent activation of CD4-positive regulatory immunosuppressive T cells), while IL2Rbeta activation plays a key role on the proliferation and activation of effector T cells (314). In another clinical trial, the NKTR-214 immunocytokine was also administered, using a similar therapeutic strategy for the treatment of patients with metastatic urothelial bladder cancer or metastatic NSCLC, in combination with atezolizumab (74–76) (NCT03138889). Another trial for advanced or metastatic solid tumors expressing the carcinoembryonic antigen (CEA), combined atezolizumab and cergutuzumab amunaleukin [CEA-IL-2 variant (IL2v)] (218), alone or together with a pretreatment with the anti-CD20 mAb obinutuzumab (NCT02350673). The immunocytokine CEA-IL2v is a fusion protein between a recombinant IL2v, unable to bind CD25, fused to the C-terminus of a high affinity, bivalent CEA-specific antibody (218). The strategy is to inhibit the PD-1/PD-1L checkpoint, increasing locally IL-2 activity on the tumor cells, allowing its binding to CD122. A similar strategy uses nivolumab in combination with the superagonist ALT-803 for the treatment of advanced and unresectable NSCLC (NCT02523469). ALT-803 is a fusion protein containing a mutated IL-15 (IL-15N72D) cytokine and a soluble, dimeric IL-15 receptor alpha Fc fusion protein (IL-15Ra-Fc) (315). The rationale of this study is to suppress the signaling through negative checkpoints, while activating and increasing NK levels and memory CD8+ T cells, through the binding of ALT-803 to the IL-2/IL-15 receptor beta gamma chain, strengthening the patient’s immune response.
Another example is the anti-HER2 mAb trastuzumab, which has been used in combination with IL-12 in treating patients with recurrent solid tumors [breast cancer, endometrial carcinoma, gastric cancer, non-small cell lung cancer (NSCLC), SCLC, and ovarian epithelial cancer] (NCT00028535). IL-12 stimulates IFN-gamma production and enhances T- and NK-cell proliferation, differentiation, and activation (316). A more complex example combines two mAb–cytokine fusion proteins, consisting of L19, a human single-chain variable fragment directed against the extra-domain B (ED-B) of fibronectin, linked to either the human pro-inflammatory cytokine tumor necrosis factor alpha (TNFa, L19-TNF) or to human IL-2 (L19-IL-2). These combinations have been used on patients with malignant melanoma (317–320) (NCT02076633). The rationale of the trial is that the L19 moiety binds to the ED-B domain of a fibronectin isoform selectively expressed in the tumor neovasculature during neo-angiogenesis. TNFa may locally induce an immune response against ED-B+ tumor cells, while the IL-2 moiety may locally activate CTL, NK cells, and macrophages.
In another clinical trial, atezolizumab was combined with (i) ipilimumab, (ii) interferon alfa-2b, (iii) PEG-interferon Alfa-2a, (iv) bevacizumab and PEG-interferon alfa-2a, and (v) obinutuzumab, for the treatment of locally advanced or metastatic solid tumors (NCT02174172). The aim is to compare on the same trial the results from these different strategies. The rationale is to restore the antitumor immune response by blocking immune checkpoints, while inducing cell cycle arrest, apoptosis or differentiation, which will led to tumor growth inhibition, concomitant with T cell and NK cell activation, inhibition of angiogenesis and induction of cytokine expression, through the administration of interferon alpha (321).
Anti-Cytokine Antibodies
On the previous section, the aim was to provide exogenous cytokines to burst the antitumor immune response. Here, the aim is to disrupt the balance of other cytokines such as IL-17 or IL-1, that may hinder the antitumor immune response.
An example, for the treatment of patients with multiple myeloma, uses the anti PD-1 mAb PDR001 in combination with the mAb CJM112 (targeting IL-17) or with the Smac Mimetic LCL161 drug (an IAP inhibitor) (NCT03111992). This strategy aims to restore the cellular immune response inhibiting checkpoint signaling, changing the cytokine balance by decreasing the available IL-17 and favoring tumor cell apoptosis. A more complex clinical trial was used for the treatment of colorectal cancer, triple-negative breast cancer, NSCLC and adenocarcinoma, where the anti-PD-1 mAb PDR001 (13) was used in combination with either (i) the anti-interleukin-1 beta (IL-1b) mAb canakinumab (214), (ii) the anti-IL-17 mAb CJM112 (219), (iii) the small molecule inhibitor trametinib (MAPKK1 and MAPKK2 inhibitor) or (iv) the EGFR antagonist nazartinib (NCT02900664). The aim is to inhibit the immune checkpoint, while either simultaneously suppressing the inflammatory responses (blocking IL-1b or IL-17), or inhibiting tumor cell proliferation with mitogen-activated protein kinase and EGFR inhibitors.
Antibodies Targeting Angiogenesis
Unlike to what happens with hematologic tumors, the growth of a solid tumor is concomitant with a local increase in nutrient and oxygen consumption and secretion of metabolites, requiring neo-vascularization for its growth. Therefore, some of the antitumor therapies aim to interfere with the neo-vascularization process, either by including antibodies against the soluble ligands, or against their receptors present in the cell surface of endothelial cells (322).
The anti-VEGF-A mAb bevacizumab, able to inhibit angiogenesis is currently being tested in combination with other therapeutic agents to determine its usefulness for cancer treatment. These combinations include: cetuximab, in advanced lung cancer (NCT00368992); MEDI3617 (anti-Ang-2) (238), for advanced solid malignancies (NCT01248949); the mAb drozitumab (PRO95780) (223) against death receptor 5 (DR5/TRAIL-R2), in metastatic colorectal cancer (NCT00851136); NK immunotherapy, in recurrent solid tumors (NCT02857920); and atezolizumab (NCT03038100, NCT02659384), nivolumab (NCT02873962), or pembrolizumab (NCT02853318) for the treatment of ovarian, fallopian tube, or primary peritoneal cancer. Bevacizumab has also been combined with MNRP1685A (242, 323), a mAb against membrane-bound endothelial cell co-receptor neuropilin-1 (NRP1), overexpressed in certain tumor cells, for advanced or metastatic solid tumors (NCT00954642). MNRP1685A prevents angiogenesis by blocking binding of VEGF, VEGF-B, and placental growth factor 2 to neuropilin-1, resulting in vessel immaturity. Other combinations of bevacizumab include parsatuzumab (247), a mAb against the vascular-restricted extracellular matrix protein epidermal growth factor-like domain multiple 7 (EGFL7), upregulated during angiogenesis and overexpressed on the cell surface of different solid tumors, for the treatment of metastatic colorectal cancer (NCT01399684). Parsatuzumab inhibits vascular development regulated by EGFL7, affecting to the survival and migration of endothelial cells during angiogenesis. An additional combination used bevacizumab and anti-VEGFC/Flt4 (VGX-100) (265) for metastatic solid tumors (NCT01514123). The rationale is to simultaneously inhibit vascular and lymphatic endothelial cell proliferation and angiogenesis.
A different approach is to target angiogenesis with the Anti-VEGFR-2 mAb ramucirumab, in combination with the anti-c-MET [hepatocyte growth factor receptor (HGFR)] mAb emibetuzumab (227), on advanced refractary solid tumors (NCT02082210). The rationale of the trial is to inhibit angiogenesis and MET signaling on the tumor cells (227). A similar strategy has been used where instead of using an anti-HGFR mAb, uses ficlatuzumab (229, 324), a mAb against the c-MET ligand (HGF), in combination with cetuximab (NCT02277197).
A similar strategy targets molecules with an expression highly restricted to the vascular endothelium. An example is the combination of pembrolizumab with demcizumab (222), a mAb that blocks the interaction of anti-delta-like ligand 4 (DLL4) with Notch-1 and Notch-4, inhibiting Notch-mediated signaling and gene transcription, impairing the productive growth of new blood vessels (325) (NCT02722954). Pembrolizumab avoids the immunosuppression by immune checkpoint signaling while demicizumab prevents angiogenesis.
In K-ras wild-type metastatic colorectal cancers, cetuximab mAb was used in combination with the alphaVbeta3 (vitronectin receptor) integrin inhibitor EMD 525797, an anti-alphaV integrin subunit mAb (226) (NCT01008475). AlphaVbeta3 integrin is a cell adhesion and signaling receptor expressed on the surface of tumor endothelial cells, with a crucial role in their adhesion and migration. The aim of the trial is to inhibit angiogenesis and endothelial cell interaction(s) with other cells or with the extracellular matrix, required for tumor angiogenesis and metastasis. A similar study in solid tumors combines bevacizumab with intetumumab (235, 326), a pan alpha-v human mAb that blocks both alpha-v beta-3 and alpha-v beta-5 integrins, resulting in inhibition of integrin-mediated tumor angiogenesis and tumor growth (NCT00888043).
The remaining clinical trials on this section, all of them combine an anti-checkpoint antibody (either anti-CTLA-4, PD-1 or PD-L1) with anti-angiogenic antibodies such as the anti-Angiopoietin 2 (Ang-2) mAb (MEDI3617) in metastatic melanoma (NCT02141542); with the antibody carotuximab (TRC105) (215) that recognizes the endothelial cell surface protein endoglin, essential for angiogenesis, in metastatic NSCLC (NCT03181308); with antibodies specific for Ang-1 and Ang-2, which prevent their interaction with their target tie2 receptors (NCT00861419); with vanucizumab (bispecific anti-VEGF/Ang-2 antibody) (263), in advanced or metastatic solid tumors (NCT01688206). The bispecific mAb targets both VEGF-A and Ang-2, which are upregulated in a variety of tumor cell types, play key roles in tumor cell proliferation, angiogenesis and metastasis. The anti-VEGF-A arm is based on bevacizumab and the anti-Ang-2 arm is based on the anti-Ang-2 antibody LC06 (263). It simultaneously binds and neutralizes both VEGF-A and Ang-2. This prevents the activation of both VEGF-A/VEGFR- and Ang-2/Tie2-mediated signaling pathways, resulting in the inhibition of proliferation of VEGF-A- and/or Ang-2-overexpressing tumor cells (263, 327).
Another strategy is to combine the anti-EGFR mAb panitumumab with an anti-hepatocyte growth factor mAb rilotumumab (252) or ganitumab (230), an anti-insulin-like growth factor 1 receptor (IGF-1R) mAb in metastatic colorectal cancer with wild-type KRAS (NCT00788957) (328). The rationale here is to simultaneously inhibit strong proliferative signals triggered by EGFR and c-MET.
Antibodies Combined with Effector Cells
In some patients, the number of cells from the innate or adaptive immune system could be decreased by the effects of previous treatments. In these cases, treatments with antibodies, whose mechanisms of action depend on immune system cell effector functions (i.e., ADCC, ADCP, etc.), could be compromised. In these cases, either autologous (harvested prior to the treatment) or allogeneic cells (NK cells, T cells, CTL cells, dendritic cells (DC), etc.) can be administered concomitantly with the therapeutic antibodies.
A combination of mAb and cells for the treatment of hematological malignancies combines the anti-CD22 mAb epratuzumab (228) with haploidentical NK cells and low-dose exogenous IL-2, for the treatment of relapsed acute lymphoblastic leukemia (NCT00941928). CD22 is a cell surface glycoprotein present on mature B cells and on many B cell malignancies. In this example, since epratuzumab action involves ADDC, the exogenous administered haploidentical NK cells strengthens its effects. The exogenous IL-2 induces NK cell proliferation, activates cytotoxic immune responses against the tumor and induces expression of certain cytotoxic cytokines, such as interferon-gamma (IFNgamma) and transforming growth factor-beta. In another example, NK cells were used in combination with nivolumab for the treatment of recurrent solid tumors (NCT02843204), strengthening the endogenous immune response with the anti-checkpoint antibodies and increasing the NK cell load. Another combination used for the treatment of recurrent solid tumors uses NK cells in combination with bevacizumab (NCT02857920), increasing the NK cell load and simultaneously targeting tumor neo-vascularization. The last combinations to be mentioned with effector cells use pembrolizumab, administrated with autologous dendritic cells-cytokine induced killer cell (DC-CIK), for advanced solid tumors (NCT03190811), or the anti-PD-1 mAb, that was used in vitro to activate and expand DC-CIK from the patient’s peripheral blood, before infusion (NCT02886897). These clinical trials aim to target the immune checkpoint and increasing the load of cytolytic cells with the DC-CIK.
Bispecific Antibodies
Nowadays the FDA and EMA allow clinical trials where the therapeutic agent is a combination of two antibodies. Bispecific antibodies may be considered as a particular combination where both antibodies are in a single molecule. This type of antibodies allows to put in close proximity the tumor cell with an effector cell, a cytokine, etc., or to re-direct the immune response of cytotoxic T cells bypassing antigen recognition through the TCR (329).
On all the trials on hematological tumors using bispecific mAb reported here, the same bispecific mAb blinatumomab (biespecific CD19-CD3) was used in different combinations. An example combines blinatumomab with the anti-CD20 mAb rituximab, for non-receptor tyrosine kinase (ABL)-negative B lineage acute lymphoblastic leukemia (NCT02003222). The rationale is to target the CD20+ B cells, while putting in close contact T cells (CD3+) with CD19+ B cells, to mount a strong cytotoxic T cell response. The rest of the trials from this group, all of them combine mAb targeting immune-checkpoints (CTLA-4, PD-1, or PD-L1) with the bispecific antibodies. These include blinatumomab in relapsed or refractory precursor B-lymphoblastic leukemia (NCT02879695) or for relapsed or refractory B cell acute lymphoblastic leukemia (NCT03160079). The rationale for these trials is to avoid the suppression of antitumor responses, while putting in close contact T cells (CD3+) with CD19+ B cells.
Bispecific antibodies in combination have also been used for the treatment of solid tumors. For most of the examples, one of the arms of the bispecific mAb identifies a tumor-associated antigen, such as the bispecific antibody RO6958688 (bispecific CD3-CEA) combined with atezolizumab, for the treatment of advanced and metastatic solid tumors (NCT02650713). The bispecific antibody RO6958688 (253, 254) recognizes, on the one side, the CD3 molecule of the TCR and, on the other side, the CEA, an antigen overexpressed in several tumors. The rationale of the trial is to block the binding of PD-L1 to its receptor avoiding the suppression of antitumor responses, while putting in close contact T cells (CD3+) with the CEA+ tumor cells, inducing a strong T cell activation which may result in a potent antitumor CTL response. Similarly, solid tumors were treated with atezolizumab in combination with bevacizumab or with vanucizumab, a bispecific mAb that simultaneously targets VEGF and Ang-2 (NCT02715531). This treatment aims to block the immune checkpoint suppression while inhibiting angiogenesis.
Another example of targeting tumor-associated antigens is the use of in vitro-activated T cells armed with GD2Bi-aATC, a bispecific antibody that recognizes CD3 and GD2 (231, 232), in combination with IL-2 and GM-CSF in patients with neuroblastoma or osteosarcoma (NCT02173093). The rationale of this study is to generate in vitro activated T cells that are infused in the patient after binding to the bispecific mAb, which will direct them to the tumor, generating a potent CTL response to kill tumor cells. Exogenous IL-2 and GM-CSF are added to maintain these cells and generate an inflammatory environment surrounding the tumor. In addition, another approach that has been used is to combine the anti-HER2 antibody trastuzumab with a bispecific MM-11 mAb anti-ErbB2/anti-ErbB3 mAb (241) for the treatment of HER2+ breast cancer (NCT01097460). The aim is to simultaneously inhibit signaling through this family of tyrosine kinase receptors.
Another approach is to use bispecific mAb to inhibit angiogenesis in solid tumors. The two examples we describe use the bispecific mAb vanucizumab (VEGF-A/Ang-2). In one of them, it is used in combination with RO7009789 (255), an antibody with immunostimulatory effects that recognizes CD40, a member of the TNF receptor superfamily, for the treatment of metastatic solid tumors (NCT02665416). In the other example, it is used either alone or in combination with atezolizumab (PD-L1) in advanced or metastatic solid tumors (NCT01688206). The rationale for both cases is to either use an anti-CD40 agonist or an anti-checkpoint antibody to burst the antitumor immune response, while inhibiting simultaneously angiogenesis by blocking the VEGF-A/VEGFR- and Ang-2/Tie2-signaling pathways.
Antibodies with Immunomodulatory Effects
In a clinical trial for metastatic colorectal cancer, imalumab (BAX69) (233), a mAb that identifies MIF is used in combination with panitumumab (NCT02448810). BAX69 abrogates MIF signaling and MIF-mediated secretion of cytokines (IL-1β, TNF-α, etc.) and inhibits proliferation of MIF overexpressing tumor cells, together with the antiproliferative effects of panitumumab (anti-EGFR mAb).
Other examples, used for solid tumors, combine nivolumab with cabiralizumab, an anti-colony-stimulating factor 1 receptor (CSF1R) mAb, which inhibits binding of its ligands (CSF-1 and IL-34), blocking the production of inflammatory mediators by macrophages and monocytes and preventing osteoclast activation (NCT02526017; NCT03158272). The aim is to inhibit the tumor-induced immune suppression with nivolumab, and to block with cabiralizumab the recruitment of CSF1R-dependent tumor-associated macrophages (TAMs). Cabiralizumab also enhances T cell infiltration and antitumor T cell immune responses.
Another immunomodulatory antibody, anti-CD73 (BMS-986179) (209–211), has been used in combination with nivolumab on advanced or spread solid cancers (NCT02754141). In this case, the use of an antibody against the cell surface enzyme CD73 turns out to be very interesting. CD73 is overexpressed in many tumors and catalyzes the conversion of extracellular nucleotides into nucleosides, generating adenosine (211). The anti-CD73 antibody prevents the conversion of AMP to adenosine, which releases the inhibition of T cell, DC, and NK activities, induces the activation of macrophages, and reduces the activity of both myeloid-derived suppressor cells and regulatory T cells (209–211). This treatment was designed to abrogate the immunosuppressor effects of both, the immune checkpoint with nivolumab and the metabolic checkpoint with BMS-986179.
In addition to antibodies that release the inhibitory effects of immune-checkpoints, there are other antibodies that are able to directly activate the immune response. The following examples represent clinical trials where these immunostimulatory antibodies are used. One of them combines avelumab, which suppresses the signaling through negative immune checkpoints, with either the anti-cytokine antibody PD-0360324 (anti-CSF-1 mAb) (248); or with the immunostimulatory antibodies PF-04518600 (anti-OX40 mAb), or utomilumab (an anti-CD137 mAb) (262) (NCT02554812); whereas another combines the anti-OX40 mAb (MOXR0916) with atezolizumab in locally advanced or metastatic solid tumors. (NCT02410512). The aim is to inhibit the PD-1/PD-L1 axis (avelumab or atezolizumab), simultaneously bursting the immune response through OX-40, CD137, or blocking TAMs generation with the anti-CFS-1 (Aspelagh, 2016). A similar strategy uses atezolizumab in combination with varlilumab (an agonistic anti-CD27 mAb), which results in an increase of the CTL response against CD27 ligands expressed on tumor cells (NCT02543645).
The anti-CD40 mAb RO7009789 activates and triggers proliferation of antigen-presenting cells (APC) and activates B and T cells, resulting in an enhanced immune response. When CD40 is expressed in solid tumor cells, RO7009789 leads to apoptosis and decreased tumor growth. This antibody in combination with the CSF1R inhibitory antibody emactuzumab has been used for the treatment of advanced solid tumors (225) (NCT02760797). Related examples combine anti-CD40 mAb either with nivolumab for the treatment of metastatic pancreatic adenocarcinoma (NCT03214250) or with the bispecific antibody vanucizumab (anti-VEGF-A and anti-Ang-2) (NCT02665416). The rationale is to activate the immune response through CD40 while inhibiting the angiogenesis blocking the binding of VEGF-A and Ang-2 to their receptors.
Adjuvants and other Immunostimulatory Agents
Another possible strategy is to combine therapeutic antibodies with molecules carrying repeated structural motifs that cannot be synthesized by vertebrates, and bind to pattern recognition receptors present in cells from the innate arm of the immune system (i.e., beta glucan that binds the C-type lectin receptor Dectin-1). These molecules are able to regulate the threshold of the immune response as an adjuvant, changing the secreted cytokine expression pattern. A particular example of this type of agents is the use of an attenuated preparation of the BCG (Bacille Calmette–Guerin) strain of Mycobacterium bovis, with potential immunostimulatory activity for the treatment of patients with bladder cancer (330).
An example, combining mAb and adjuvants is the use of BTH1704 (212), an mAb against MUC1, an aberrantly glycosylated antigen overexpressed on the surface of a variety of cancer cells, in combination with a polysaccharide beta 1,3/1,6 glucan derived from the cell wall of Saccharomyces cerevisiae (PGG Beta-Glucan), for the treatment of patients with advanced pancreatic cancer (NCT02132403). The rationale of this trial is to directly target the tumor with the anti-MUC1 mAb, while unspecifically stimulate the immune response with beta glucan by binding to an alternate site on the neutrophil complement receptor 3 (CR3), priming the neutrophil to become cytotoxic after binding to complement on tumor cells via CR3. In addition, this agent may induce hematopoietic progenitor cell mobilization.
Antibodies in Combination with Vaccines
Tumor cells carry antigens which can be recognized as non-self by the immune system. In some cases, however, the microenvironment in which these tumor antigens are presented do not allow to evoke an immune response. There is a plethora of possibilities to burst the antitumor immune response, one of them is to use tumor antigens as a vaccine. In the context of antitumor therapies, anti-idiotipic antibodies represent a particular type of vaccines.
Since the interaction of antibodies with other molecules is based on structural complementarity, antibodies that recognize the region of an antibody that interacts with its antigen (anti-idiotipic antibodies) might mimic the structure of this antigen (i.e., a tumor marker). Thus, the anti-idiotipic antibodies might act as an antitumor vaccine able to trigger a host immune response to kill tumor cells. Combining chemotherapy and radiation therapy with vaccine therapy may help to kill tumor cells more effectively.
An example of anti-idiotipic antibodies used as vaccines in antitumor therapy is abagovomab, an IgG1 anti-idiotype mAb, that functionally mimics the 3D structure of a specific epitope on the ovarian cancer tumor-associated antigen CA-125 (203). Its variable region acts as a surrogate antigen for CA-125, bestowing potential antineoplastic activity; it has been used in ovarian epithelial, fallopian tube, or peritoneal cancer (NCT00058435). Another example of vaccine therapy combines two anti-idiotipic antibodies, 11D10 (201) an mAb that mimics an epitope of the high molecular weight human milk fat globule glycoprotein, expressed at high levels by human breast and other tumor cells and 3H1 an mAb that mimics an epitope of the tumor-associated protein CEA (199). This combination has been used for the treatment of colorectal cancer metastatic to the liver (NCT00033748). The 11D10 mAb has also been used in other combinations, for example, with a GD2 anti-idiotype mAb vaccine, together with chemotherapy and radiotherapy, for the treatment of limited-stage SCLC (NCT00045617).
A study combining the anti-idiotipic mAb abagovomab, which mimics a specific epitope on CA-125 with stereotactic body radiation therapy (SBRT), chemotherapy, and the synthetic antiviral agent nelfinavir mesylate, which selectively binds to and inhibits human immunodeficiency virus protease, has been used for the treatment of locally advanced pancreatic cancer (NCT01959672).
Currently, other types of vaccines are being used with mAb in combination with agents that allow to evoke an immune response against tumor antigens. The mAb in these combinations may strengthen the evoked immune response. For the examples described below, the combination contains antibodies that either disrupt the PD-1/PDL-1 axis or that block the binding of B7-1 and B7-2 to CTLA-4 allowing T cell co-stimulatory signals and activation, unless otherwise specified. One example is the treatment with a peptide from Wilms tumor 1 antigen on recurrent ovarian cancer (NCT02737787). Another, more complex example is to administrate the peptide vaccine PVX-410 (derived from X-box-binding protein 1-unspliced XBP1-US, XBP1-spliced syndecan-1, and CS1), to treat triple-negative breast cancer tumors (NCT02826434). A third example uses a HER2 intracellular domain peptide in combination with the polysaccharide-K as adjuvant, in HER2+ recurrent breast cancer patients, which are receiving pertuzumab or trastuzumab (NCT01922921). The rationale for this trial is to combine the effects of the anti-HER2 mAb with using a HER2 peptide to switch the B and T cell responses through APC activation. Otherwise, peptides could be presented as a fusion protein, such as in CIMAvax vaccine (EGF-rP64K/Montanide ISA 51), which triggers a strong humoral immune response against EGF and has been used in NSCLC (NCT02955290). There are also personalized neoantigen cancer vaccines, such as NeoVax for the treatment of high-risk renal cell carcinoma (NCT02950766).
Another approach to generate vaccines is to use modified virus such as Ad-CEA vaccine, an oncolytic adenovirus encoding an epitope of human CEA (331), used for the treatment of patients with previously untreated metastatic colorectal cancer (NCT03050814). This vaccine may induce both humoral and cellular immune responses against CEA+ tumor cells.
A different example uses the CV301 (CEA-MUC-1-TRICOM Vaccine) viral vaccine, which contains a version of the recombinant vaccinia viral vector and a recombinant fowlpox viral vector encoding both CEA and MUC-1, in combination with TRICOM [co-stimulatory molecules, B7-1, intracellular adhesion molecule 1 (ICAM-1), and LFA-3] (332). It may enhance presentation of CEA and MUC-1 to APC and subsequently a CTL response against the tumor cells, it has been used in previously treated NSCLC (NCT02840994). A similar approach has been evaluated for the treatment of prostate cancer using a recombinant vaccinia virus encoding a modified peptide of the prostate-specific antigen and TRICOM (NCT00113984). This viral vaccine may enhance antigen presentation and may activate a CTL response.
Attenuated bacteria have also been used as carriers for antitumor vaccines. For example, the attenuated Listeria ADXS11-001 encoding a papillomavirus type 16 E7 fused to a non-hemolytic listeriolysin O protein, it has been used for the treatment of cervical and Head and Neck Cancer (HNSCC) (NCT02291055). The rationale is to mount a CTL response against cancer cells overexpressing the cell surface glycoprotein HPV 16 E7, overexpressed in the majority of cervical cancer cells.
More sophisticated approaches use tumor vaccines, such as GM.CD40L, which is a cell-based vaccine composed of irradiated tumor cells transduced with GM-CSF and CD40-ligand (CD40L) genes (333). Upon administration, this vaccine may stimulate an antitumoral DC-mediated immune response, it has been used in lung adenocarcinomas (NCT02466568). Another example that does not use anti-checkpoint antibodies but combines the anti-HER2 mAb trastuzumab with a cell-based vaccine, consisting of two irradiated allogeneic mammary carcinoma cell lines genetically modified to secrete human GM-CSF, has been used for the treatment of HER2+ metastatic breast tumors (NCT00399529). An additional example of cell-based vaccines uses GVAX (334), a pancreatic cancer vaccine and IMC-CS4, a macrophage targeting mAb (CSF1R inhibitor) for the treatment of pancreatic adenocarcinomas (234). GVAX is composed of irradiated, whole tumor cells (autologous or allogeneic), genetically modified to secrete GM-CSF (NCT03153410).
The immune response against the tumor can be busted using also DNA, RNA, or liposome-based vaccines. As an example, triple-negative breast cancers have been treated with neoantigen DNA vaccine combined with anti-immune-checkpoint antibodies (NCT03199040). Other vaccines use autologous DC loaded in vitro with Cytomegalovirus pp65-lysosomal-associated membrane protein mRNA as a vaccine, in combination with the anti-IL2R alpha mAb basiliximab (207), in glioblastoma multiform (NCT00626483). The rationale is to restore the number of immunosuppressive T regulatory cells during recovery from therapeutic temozolomide-induced lymphopenia, together with a synergistic enhancement of vaccine-driven CTL responses. Another study describes the use of the immuno-modulating mAb varlilumab (anti-CD27, TNFR family) in combination with a liposome-based vaccine consisting of two peptides from MUC1 and the toll-like receptor 4 encapsulated in liposomes (NCT02270372). This immunization stimulates both cellular and humoral responses.
Another clinical trial uses trastuzumab and an allogeneic GM-CSF-secreting whole cell breast cancer vaccine for HER-2+ breast tumors. This study will also test whether cyclophosphamide can eliminate the suppressive influence of regulatory T cells. The vaccine consists of two irradiated allogeneic mammary carcinoma cell lines genetically modified to secrete human GM-CSF (NCT00399529).
Pidilizumab (anti-PD-1) (250) in combination with a DC fusion vaccine, following autologous stem cell transplantation has been used on multiple myeloma (NCT01067287). The rationale is to disrupt the PD-1/PD-L1 axis, while vaccinating the patients with de DC fusion vaccine, which consists of DC fused to the patient’s myeloma, where the myeloma antigens will be presented by HLA class I to CD8+ T cells, allowing their activation and mounting a CTL response. This is done on patients after an autologous transplantation with HSCs. A similar approach uses nivolumab vaccine with autologous DCs pulsed with tumor lysate antigen, in patients with recurrent glioblastoma (NCT03014804).
Another study combines lymphodepletion with anti-CD45 mAb with a vaccine generated from autologous DC and Epstein Barr virus (EBV)-infected lymphoblastoid cell lines transduced with an LMP1/LMP2-expressing adenoviral vector, which are irradiated, and then used to stimulate and expand autologous CTL to produce LMP1-/LMP2-specific CTL ex vivo, for the treatment of EBV+-nasopharyngeal carcinoma (NCT00515957). The rationale of this trial is to deplete autologous CD45+ cells, then generate a cell-based vaccine to activate ex vivo specific CTL that are then infused into the patient.
Other Strategies
In some approaches, a fusion protein between the antibody and a tumor antigen is used in combination with other therapies. For example, on the treatment of NY-ESO 1+ NSCLC, where atezolizumab was combined with both the adjuvant poly-ICLC (a synthetic complex of carboxymethylcellulose, polyinosinic-polycytidylic acid and poly-L-lysine double-stranded RNA) and DEC-205/NY-ESO-1 [CDX-1401, a fusion protein between a mAb directed against the endocytic DC receptor DEC-205, linked to the tumor-associated antigen (NY-ESO-1)] (NCT02495636) (217). Atezolizumab will inhibit immune checkpoints negative signals, while the internalization by DC of the mAb–antigen fusion protein may specifically deliver the NY-ESO-1 molecule and trigger a CTL response against cancer cells expressing this antigen. Simultaneously, the adjuvant may stimulate the release of cytotoxic cytokines by inducing IFNgamma production. A similar approach, for the treatment of melanoma patients, using CDX-1401 combined with a neoantigen-based melanoma-poly-ICLC vaccine and a recombinant Flt3 Ligand (CDX-301) (NCT02129075). This treatment should boost the immune system to mount a CTL response against cancer cells expressing NY-ESO-1. In addition, the adjuvant may induce IFNgamma production and the recombinant Flt3 ligand may stimulate the proliferation and mobilization of bone marrow precursor cells, including CD34+ cells, and DCs.
An additional strategy would be to use scavengers of the ligand with low immunogenicity. For example, sEphB4-HAS, a human serum albumin (HAS) fused with the extracellular domain of tyrosine kinase ephrin type-B receptor 4 (sEphB4) is combined with pembrolizumab, for the treatment of NSCLC or HNSCC (NCT03049618). Pembrolizumab inhibits negative immune checkpoint signals, whereas EphB4-HSA is expected to decrease angiogenesis and cell growth of Efnb2 and/or EphB4 overexpressing tumor cells, while the albumin moiety will avoid renal clearance of the fusion protein, increasing its half-life without affecting immunogenicity.
Another strategy would be to target matrix enzymes required for tumor invasiveness. An example is the use of nivolumab combined with andecaliximab (GS-5745) (204, 205), an inhibitory mAb of matrix metalloproteinase 9 (MMP-9) in recurrent gastric or gastroesophageal junction adenocarcinomas (NCT02864381). Since MMP-9 activity is associated with tumor invasion and metastasis (335), the rationale is that andecaliximab will inhibit extracellular matrix protein degradation and angiogenesis, while nivolumab will interfere with the PD-1/PD-L1 axis.
Pembrolizumab (PD-1) was used in combination with CVA21 (CAVATAK™), coxsackievirus A21, a naturally occurring enterovirus with potential antitumor activity. This combination was used for advanced NSCLC (NCT02824965). CVA21, intra-tumor administered, targets and binds the ICAM-1 and decay acceleration factor, cell surface molecules, both overexpressed on certain malignant cells (336, 337). After entering the cells, the virus replicates causing cell lysis. This, together with the inhibition of the immune checkpoint, results in a reduction of tumor cell growth.
Another strategy combines conatumumab (220), an agonist mAb directed against the extracellular domain of human tumor necrosis factor-related apoptosis-inducing ligand (TRAIL) receptor 2 (TRAIL-R2), also known as DR5, with the anti-IGF-1R mAb ganitumab, in patients with advanced solid tumors without disease progression whose previous studies were closed (NCT01327612). TRAIL-2 and IGF-1R are expressed by a variety of solid tumors and cancers of hematopoietic origin. Conatumumab mimics TRAIL activity, activating caspase cascades and inducing tumor cell apoptosis, while ganitumab inhibits IGF-1 binding and, therefore, the PI3K/Akt pathway. This treatment may result in the inhibition of tumor cell proliferation and the induction of tumor cell apoptosis. Another clinical trial combining tigatuzumab, a mAb targeting the death receptor TRAIL-R2 with abraxane, an albumin-stabilized nanoparticle containing paclitaxel, non-covalently coated with the anti-CD20 mAb rituximab, in patients with metastatic, triple-negative breast cancer (NCT01307891). The relevance of the trial is that combines an anti-TRAIL-R2 mAb that induces death, while albumin stabilizes the complex, whereas rituximab allows to target paclitaxel to CD20+ cells, minimizing toxicity on normal cells. A strategy being used on EGFR+ tumors is to combine an anti-immune checkpoint antibody with the anti-EGFR mAb necitumumab (NCT02451930) or nimotuzumab (245) (NCT02947386). Or the same strategy, but using B-701, a neutralizing mAb directed against the fibroblast growth factor receptor type 3, in combination with atezolizumab in urothelial cell carcinoma (NCT03123055).
One of the most sophisticated clinical trials combines chemotherapy, bevacizumab, avelumab, ALT-803 (IL-15 super agonist), aNK (allogenic human NK-92 cell line, expressing CD16 and IL-2), and GI-4000 (a heat-killed recombinant Saccharomyces cerevisiae yeast transfected with mutated forms of Ras) with the NANT pancreatic cancer vaccine (ETBX-011) containing a replication-defective adenoviral vector encoding a CEA epitope Ad5-CEA(6D), used for pancreatic cancer (NCT03136406). A clinical trial for colorectal cancer uses a similar combination of chemotherapy, nivolumab, avelumab bevacizumab, cetuximab, SBRT, haNK, ALT-803, and a cocktail of vaccines: ETBX-011, ETBX-021, ETBX-051, ETBX-061, GI-4000, GI-6207, and GI-6301 (NCT03169777). The rationale for these two clinical trials is to hit the tumor simultaneously with a wide spectrum of the available tools against the tumor, where the concentration of each one of the agents can be decreased to minimize the unwanted effects on normal cells.
Questions and Queries Raised by the Combinations
We hope that we have been able to depict up to here, the huge complexity inherent to the use of therapeutic antibodies in combination with other biological agents for the treatment of cancer. Since most of the clinical trials described in this review are relatively recent (started during the last 7 years), many of them lack results in public databases, including the clinical trials database from NCI, or as published scientific manuscripts. This burst of clinical trials using antibodies in combinations with other biologicals is based on the positive results found by some combinations (anti-HER2 or anti-GD2 mAb), although the complexity increase in these combinations also implies an increase in the possibility of adverse side effects/increased toxicity or lack of additive or synergistic effects of the therapeutic agents. Initially, the therapeutic doses used for combinations were taken from the monotherapeutic trials, although in many cases, the non-toxic concentrations used in monotherapy, turn to be toxic in combinations, generating new toxicity profiles (338). This is of particular relevance when antibodies able to burst the antitumor immune response are used (either to inhibit the immune checkpoint proteins, to block inhibitory NK receptors or to trigger NK cells through activating receptors, etc.), which might lead to a dis-regulation of the immune response, an uncontrolled inflammatory response, and autoimmunity. This problem can also be related to an apparent lack of additive or synergistic effects of the therapeutic agents, where the potential clinical benefits of the combination could be overlooked by the initial toxicity of the mixture. Dose and schedule changes, however, can overcome the toxicity effects, allowing to demonstrate the enhanced clinical benefits of a particular combination (339). The use of CTLA-4 and PD-1 inhibitor antibodies in combination (nivolumab and ipilimumab), improved the treatment efficacy in advanced melanoma, as compared to monotherapies (340). Indeed, this combination has been approved in 2016 by the US FDA for the treatment of metastatic melanoma (341), despite the higher frequency and severity of adverse reactions of the combination, as compared to the corresponding monotherapies (340, 342–344).
It is interesting to note that a combination of TRC105 (carotuximab, anti-endoglin antibody) with bevacizumab was used on a clinical trial for the treatment of patients with advanced cancer, where the combination was well tolerated and clinical activity was observed in a VEGF inhibitor-refractory population (NCT01332721) (345), the same combination failed to improve progression-free survival on patients with refractory metastatic renal cell cancer (NCT01727089) (346). These data clearly suggest that the problem does not strictly lie with the antibody combination, but rather it might be related to the tumor microenvironment, tumor type, the therapeutic approach used, or the clinical history of the patient.
Several examples of clinical trials where antibodies that have been used in combination with other biologicals, which were well tolerated and showed additive or synergistic therapeutic responses have been selected. These include a clinical trial for melanoma patients with low tumor infiltrating lymphocytes, an anti-PD-1 non-responsive phenotype. The combination of pembrolizumab with an intratumoral electroporation of a plasmid coding for interleukin 12 cDNA (pIL-12) showed a 40% clinical response with associated positive immune-based biomarker data and a safety profile (NCT02493361) (347), where the combination of pembrolizumab with the plasmid pIL-12 renders half of the patients responsive to the anti-PD-1. Another example that combines mAb with cytokines is a study of immune activation and antitumor activity in renal cancer of PEGylated human IL-10 (AM0010) in combination with pembrolizumab or nivolumab, the combinations were well tolerated, and CD8+ T cell activation was detected (NCT02009449) (348).
Other examples include a phase Ib study, otlertuzumab (TRU-016, an anti-CD37 mAb) in combination with rituximab and bendamustine, which was well tolerated and induced therapeutic responses in the majority of patients with relapsed indolent B-non-Hodgkin lymphoma (NCT01317901) (349). Similarly, the combination of pidilizumab plus rituximab was well tolerated and therapeutically active in patients with relapsed follicular lymphoma (350).
On a phase Ib study of utomilumab (PF-05082566, a 4-1BB/CD137 agonist), in combination with pembrolizumab (MK-3475) in patients with advanced solid tumors had a confirmed complete or partial response in 26.1% of them. Pharmacokinetics and immunogenicity of both mAb were similar when administered alone or in combination. A trend toward higher levels of activated memory/effector peripheral blood CD8+ T cells was observed in responders versus non-responders, supporting further investigation of this combination (NCT02179918) (262).
Other combinations include the anti-checkpoint antibody nivolumab in combination with an antibody that blocks the KIR inhibitory receptors in NK cells. On a phase I/II study of the NK-targeted antibody lirilumab (a fully human mAb that blocks inhibitory KIRs on NK cells) in combination with nivolumab in advanced HNSCC demonstrated, in preliminary results, clinical benefit, with deep and durable responses in some patients. This combination demonstrated a manageable safety profile similar to that observed with nivolumab monotherapy (NCT01714739) (347).
Another combination that might be interesting for the future of the field is the combination of oncolytic virus with anti-checkpoint antibodies. Indeed, preliminary results from several clinical trials using the oncolytic virus coxsackievirus A21 (CVA21, CAVATAK) in combination with ipilimumab (NCT01636882) (351) or pembrolizumab (NCT02043665) (348), for the treatment of patients with advanced cancer, showed that these combinations were generally well tolerated and induced antitumor activity. A phase II trial using intratumoral injection of the HF10 oncolytic virus, an attenuated, replication-competent mutant strain of herpes simplex virus type 1, and ipilimumab in patients with unresectable or metastatic melanoma showed therapeutic activity and the treatment was well tolerated (NCT02272855) (352).
For other clinical trials, on early phases, the combination is well tolerated, such as a dose escalation study of the OX40 agonist MOXR0916 and atezolizumab (anti-PD-L1 mAb) in patients with advanced solid tumors, using each agent at its recommended monotherapy dose, was well tolerated. (NCT02410512) (353).
Other clinical trials, including anti-checkpoint antibodies detect clear tumor regression, although with a toxicity higher than reasonable. This is the case for a clinical trial were BMS-986016 (anti-LAG-3 mAb) in combination with nivolumab was administered to patients with hematologic and solid malignancies. Preliminary results demonstrated objective tumor regressions, concomitant with the toxicity characteristic of immune checkpoint blockers (NCT02061761, NCT01968109) (348).
On another group of clinical trials, the main characteristic is that although they are well tolerated in general, they failed to provide significant additive/synergistic therapeutic effects. This is the case of a clinical trial where urelumab (a CD137 agonist), in combination with nivolumab was used for the treatment of hematologic and solid tumor malignancies. This combination did not provide significant additive/synergistic clinical benefits at the doses evaluated (NCT01471210, NCT02253992) (351). In another, urelumab in combination with rituximab or cetuximab was used in patients with refractory lymphoma or selected advanced solid tumors. Although the combinations were safe and well tolerated, with minimal evidence of liver toxicity, they did not demonstrate substantial enhancement of clinical responses or lead to intratumoral immune modulation in these tumor settings (NCT01775631, NCT02110082) (348).
Finally, there are a couple of selected clinical trials using combinations of antibodies and other biologicals that were toxic or had to be terminated on overall benefit–risk assessment. These include a clinical trial with patients with advanced solid tumors, which were treated with a combination of MDX-447 [a bispecific mAb directed to FcγRI (CD64) and EGFR] with G-CSF, although the bispecific mAb alone was well tolerated, the combination was not well tolerated and precluded meaningful dose escalation on a phase I clinical trial (354). A second example is a phase II study of imalumab [BAX69, an anti-oxidized macrophage MIF (oxMIF)] and 5-FU/Leucovorin or Panitumumab (anti-EGRF mAb), versus the standard of care in metastatic colorectal cancer patients, which was terminated (February, 2017) based on overall benefit–risk assessment (NCT02448810), although it was initially reported that this combination was generally safe and well tolerated (355).
The problems that arose with using therapeutic antibodies in combinations has led the Society for Immunotherapy of Cancer to name a Combination Immunotherapy Task Force to identify and prioritize the most promising prospects for combinatorial approaches as well as to address the challenges associated with developing these strategies (339). Furthermore, it seems clear by now, that an improved understanding of pharmacodynamic effects of each agent within a combination will support the rational development of immune-based combinations for cancer treatment (356).
Conclusion
The broad variety of clinical trials summarized here presents the overwhelming complexity of the use of antibody in combinations for cancer treatments. The antibodies used either interfere with a ligand–receptor interaction, blocking a signaling pathway relevant for tumor growth, or identify tumor-associated antigens, where they somehow induce the death of the tumor cells by ADCC, CDC, and ADCP or directly inducing apoptosis. If the antibodies by themselves cannot kill the tumor cells, they can be conjugated to cytotoxic drugs to exert this function, or directly coupled to radio-labeled agents, where they trigger radiolysis. These antibodies can be combined with other antibodies that rather than directed against the tumor cells, identify targets on the tumor environment. These include antibodies that inhibit tumor-induced vascularization, or antibodies against cells or molecules involved in the immune response. A turning point was the use of mAb that block PD-L1/PD-1 interaction, or anti-CTLA-4 mAb; all of them disrupting the tumor-induced suppression of antitumor immune responses. In addition, mAb are used in combination with vaccines with the aim of evoking an antitumor response, either against a single tumor antigen or against a broad spectrum of antigens, for example when using irradiated tumor cells expressing pro-inflammatory cytokines.
It is obvious that there are many challenges to be solved regarding antibodies in combinations as antitumor therapeutic agents. One of the most relevant challenges is the possible increase of toxicity of the combinations, concomitant with an increased complexity of the trials. This has to be carefully evaluated for each combination to identify the conditions giving the highest efficacy/toxicity ratio. This is of particular interest when the aim is to burst the antitumor host immune response, where a small response could fail to kill the tumor, but an over-response could lead to unwanted inflammatory or autoimmune processes. Another relevant challenge to be solved is the identification of new/additional biomarkers that would allow a personalized follow-up of the patient’s status, which would be required due to the tumor and the patient’s genotypic and phenotypic differences.
The current clinical trials suggest that in the future anti-cancer therapies will combine antibodies that block signaling cascades, or identify tumor-associated antigens with others that disrupt the tumor-induced immuno-suppression, together with vaccines that evoke an antitumor immune response, activated effector cells or CAR T cells. These combinations could also contain anti-cytokine antibodies, or cytokines, to burst the immune response. The aim will be to directly target tumor antigens or signal pathways, and at the same time interfere with the “immune history” of the organism, to make the patient’s own body aware of the tumor presence and simultaneously help it to make a strong antitumor response. Thus, it will be the patient’s immune response against the tumor the ultimate mechanism responsible for the cure of cancer.
Author Contributions
All authors contributed to drafting, revising, and approving the final article.
Conflict of Interest Statement
The authors declare that the research was conducted in the absence of any commercial or financial relationships that could be construed as a potential conflict of interest.
Acknowledgments
The authors thank all the members of their laboratories for critical reading of the manuscript and helpful suggestions.
Funding
The work in the author’s laboratories was partially supported by grants from the CSIC (PIE-201420E109, to LK), the PN2014-A from the ISCIII (PI14/00703, co-financed by FEDER funds from the EU, Operative program on Intelligent Growth 2014-2020, to LK), and from the Spanish Ministry of Economy, Industry and Competitiveness (RTC-2015-3786-1 to LK and JG-S, and RTC-2015-3846-1 to JG-S), both co-financed by FEDER funds from the EU.
Supplementary Material
The Supplementary Material for this article can be found online at http://www.frontiersin.org/articles/10.3389/fimmu.2017.01804/full#supplementary-material.
References
1. Ayyar BV, Arora S, O’Kennedy R. Coming-of-age of antibodies in cancer therapeutics. Trends Pharmacol Sci (2016) 37(12):1009–28. doi:10.1016/j.tips.2016.09.005
2. Chiavenna SM, Jaworski JP, Vendrell A. State of the art in anti-cancer mAbs. J Biomed Sci (2017) 24(1):15. doi:10.1186/s12929-016-0311-y
3. Farkona S, Diamandis EP, Blasutig IM. Cancer immunotherapy: the beginning of the end of cancer? BMC Med (2016) 14:73. doi:10.1186/s12916-016-0623-5
4. Leavy O. Therapeutic antibodies: past, present and future. Nat Rev Immunol (2010) 10(5):297. doi:10.1038/nri2763
5. Scott AM, Wolchok JD, Old LJ. Antibody therapy of cancer. Nat Rev Cancer (2012) 12(4):278–87. doi:10.1038/nrc3236
6. Weiner LM, Surana R, Wang S. Monoclonal antibodies: versatile platforms for cancer immunotherapy. Nat Rev Immunol (2010) 10(5):317–27. doi:10.1038/nri2744
7. Adler MJ, Dimitrov DS. Therapeutic antibodies against cancer. Hematol Oncol Clin North Am (2012) 26(3):447–81,vii. doi:10.1016/j.hoc.2012.02.013
8. Martin-Liberal J, Ochoa de Olza M, Hierro C, Gros A, Rodon J, Tabernero J. The expanding role of immunotherapy. Cancer Treat Rev (2017) 54:74–86. doi:10.1016/j.ctrv.2017.01.008
9. Schadendorf D, Hodi FS, Robert C, Weber JS, Margolin K, Hamid O, et al. Pooled analysis of long-term survival data from phase II and phase III trials of ipilimumab in unresectable or metastatic melanoma. J Clin Oncol (2015) 33(17):1889–94. doi:10.1200/JCO.2014.56.2736
10. Callahan MK, Wolchok JD. At the bedside: CTLA-4- and PD-1-blocking antibodies in cancer immunotherapy. J Leukoc Biol (2013) 94(1):41–53. doi:10.1189/jlb.1212631
11. Schuurman J, Parren PW. Editorial overview: special section: new concepts in antibody therapeutics: what’s in store for antibody therapy? Curr Opin Immunol (2016) 40:vii–xiii. doi:10.1016/j.coi.2016.04.001
12. Melero I, Berman DM, Aznar MA, Korman AJ, Perez Gracia JL, Haanen J. Evolving synergistic combinations of targeted immunotherapies to combat cancer. Nat Rev Cancer (2015) 15(8):457–72. doi:10.1038/nrc3973
13. Mahoney KM, Rennert PD, Freeman GJ. Combination cancer immunotherapy and new immunomodulatory targets. Nat Rev Drug Discov (2015) 14(8):561–84. doi:10.1038/nrd4591
14. Henricks LM, Schellens JH, Huitema AD, Beijnen JH. The use of combinations of monoclonal antibodies in clinical oncology. Cancer Treat Rev (2015) 41(10):859–67. doi:10.1016/j.ctrv.2015.10.008
15. Vilgelm AE, Johnson DB, Richmond A. Combinatorial approach to cancer immunotherapy: strength in numbers. J Leukoc Biol (2016) 100(2):275–90. doi:10.1189/jlb.5RI0116-013RR
16. Morrissey KM, Yuraszeck TM, Li CC, Zhang Y, Kasichayanula S. Immunotherapy and novel combinations in oncology: current landscape, challenges, and opportunities. Clin Transl Sci (2016) 9(2):89–104. doi:10.1111/cts.12391
17. Sathyanarayanan V, Neelapu SS. Cancer immunotherapy: strategies for personalization and combinatorial approaches. Mol Oncol (2015) 9(10):2043–53. doi:10.1016/j.molonc.2015.10.009
18. Khalil DN, Smith EL, Brentjens RJ, Wolchok JD. The future of cancer treatment: immunomodulation, CARs and combination immunotherapy. Nat Rev Clin Oncol (2016) 13(5):273–90. doi:10.1038/nrclinonc.2016.25
19. Ni L, Dong C. New checkpoints in cancer immunotherapy. Immunol Rev (2017) 276(1):52–65. doi:10.1111/imr.12524
20. Romano G, Gawlinski A. New frontiers in oncology: immune checkpoint inhibitors in combination therapy. Drugs Today (Barc) (2017) 53(2):103–15. doi:10.1358/dot.2017.53.2.2592798
21. Granier C, De Guillebon E, Blanc C, Roussel H, Badoual C, Colin E, et al. Mechanisms of action and rationale for the use of checkpoint inhibitors in cancer. ESMO Open (2017) 2(2):e000213. doi:10.1136/esmoopen-2017-000213
22. Pianko MJ, Liu Y, Bagchi S, Lesokhin AM. Immune checkpoint blockade for hematologic malignancies: a review. Stem Cell Investig (2017) 4:32. doi:10.21037/sci.2017.03.04
23. Goodman A, Patel SP, Kurzrock R. PD-1-PD-L1 immune-checkpoint blockade in B-cell lymphomas. Nat Rev Clin Oncol (2017) 14(4):203–20. doi:10.1038/nrclinonc.2016.168
24. Balar AV, Weber JS. PD-1 and PD-L1 antibodies in cancer: current status and future directions. Cancer Immunol Immunother (2017) 66(5):551–64. doi:10.1007/s00262-017-1954-6
25. Aris M, Mordoh J, Barrio MM. Immunomodulatory monoclonal antibodies in combined immunotherapy trials for cutaneous melanoma. Front Immunol (2017) 8:1024. doi:10.3389/fimmu.2017.01024
26. Xu-Monette ZY, Zhang M, Li J, Young KH. PD-1/PD-L1 blockade: have we found the key to unleash the antitumor immune response? Front Immunol (2017) 8:(1597). doi:10.3389/fimmu.2017.01597
27. Santamaria S, Delgado M, Kremer L, Garcia-Sanz JA. Will a mAb-based immunotherapy directed against cancer stem cells be feasible? Front Immunol (2017) 8:1509. doi:10.3389/fimmu.2017.01509
28. Papaioannou NE, Beniata OV, Vitsos P, Tsitsilonis O, Samara P. Harnessing the immune system to improve cancer therapy. Ann Transl Med (2016) 4(14):261. doi:10.21037/atm.2016.04.01
29. Brody J, Kohrt H, Marabelle A, Levy R. Active and passive immunotherapy for lymphoma: proving principles and improving results. J Clin Oncol (2011) 29(14):1864–75. doi:10.1200/JCO.2010.33.4623
30. Reichert JM. Antibodies to watch in 2017. MAbs (2017) 9(2):167–81. doi:10.1080/19420862.2016.1269580
31. Andrick BJ, Gandhi A. Olaratumab: a novel platelet-derived growth factor receptor alpha-inhibitor for advanced soft tissue sarcoma. Ann Pharmacother (2017) 51(12):1090–8. doi:10.1177/1060028017723935
32. Chiorean EG, Sweeney C, Youssoufian H, Qin A, Dontabhaktuni A, Loizos N, et al. A phase I study of olaratumab, an anti-platelet-derived growth factor receptor alpha (PDGFRalpha) monoclonal antibody, in patients with advanced solid tumors. Cancer Chemother Pharmacol (2014) 73(3):595–604. doi:10.1007/s00280-014-2389-9
33. Deshpande HA, Cecchini M, Ni Choileain S, Jones R. Olaratumab for the treatment of soft tissue sarcoma. Drugs Today (Barc) (2017) 53(4):247–55. doi:10.1358/dot.2017.53.4.2560077
34. van der Graaf WT. Olaratumab in soft-tissue sarcomas. Lancet (2016) 388(10043):442–4. doi:10.1016/S0140-6736(16)30788-7
35. Agus DB, Gordon MS, Taylor C, Natale RB, Karlan B, Mendelson DS, et al. Phase I clinical study of pertuzumab, a novel HER dimerization inhibitor, in patients with advanced cancer. J Clin Oncol (2005) 23(11):2534–43. doi:10.1200/JCO.2005.03.184
36. Gerratana L, Bonotto M, Bozza C, Ongaro E, Fanotto V, Pelizzari G, et al. Pertuzumab and breast cancer: another piece in the anti-HER2 puzzle. Expert Opin Biol Ther (2017) 17(3):365–74. doi:10.1080/14712598.2017.1282944
37. Jhaveri K, Esteva FJ. Pertuzumab in the treatment of HER2+ breast cancer. J Natl Compr Canc Netw (2014) 12(4):591–8. doi:10.6004/jnccn.2014.0059
38. Araki K, Fukada I, Yanagi H, Kobayashi K, Shibayama T, Horii R, et al. First report of eribulin in combination with pertuzumab and trastuzumab for advanced HER2-positive breast cancer. Breast (2017) 35:78–84. doi:10.1016/j.breast.2017.06.015
39. Goldenberg MM. Trastuzumab, a recombinant DNA-derived humanized monoclonal antibody, a novel agent for the treatment of metastatic breast cancer. Clin Ther (1999) 21(2):309–18. doi:10.1016/S0149-2918(00)88288-0
40. Molina MA, Codony-Servat J, Albanell J, Rojo F, Arribas J, Baselga J. Trastuzumab (herceptin), a humanized anti-Her2 receptor monoclonal antibody, inhibits basal and activated Her2 ectodomain cleavage in breast cancer cells. Cancer Res (2001) 61(12):4744–9.
41. Petricevic B, Laengle J, Singer J, Sachet M, Fazekas J, Steger G, et al. Trastuzumab mediates antibody-dependent cell-mediated cytotoxicity and phagocytosis to the same extent in both adjuvant and metastatic HER2/neu breast cancer patients. J Transl Med (2013) 11:307. doi:10.1186/1479-5876-11-307
42. Valabrega G, Montemurro F, Aglietta M. Trastuzumab: mechanism of action, resistance and future perspectives in HER2-overexpressing breast cancer. Ann Oncol (2007) 18(6):977–84. doi:10.1093/annonc/mdl475
43. Gall VA, Philips AV, Qiao N, Clise-Dwyer K, Perakis AA, Zhang M, et al. Trastuzumab increases HER2 uptake and cross-presentation by dendritic cells. Cancer Res (2017) 77(19):5374–83. doi:10.1158/0008-5472.CAN-16-2774
44. Barok M, Joensuu H, Isola J. Trastuzumab emtansine: mechanisms of action and drug resistance. Breast Cancer Res (2014) 16(2):209. doi:10.1186/bcr3621
45. Lewis Phillips GD, Li G, Dugger DL, Crocker LM, Parsons KL, Mai E, et al. Targeting HER2-positive breast cancer with trastuzumab-DM1, an antibody-cytotoxic drug conjugate. Cancer Res (2008) 68(22):9280–90. doi:10.1158/0008-5472.CAN-08-1776
46. Recondo G Jr, de la Vega M, Galanternik F, Diaz-Canton E, Leone BA, Leone JP. Novel approaches to target HER2-positive breast cancer: trastuzumab emtansine. Cancer Manag Res (2016) 8:57–65. doi:10.2147/CMAR.S104447
47. Krop IE, Kim SB, Martin AG, LoRusso PM, Ferrero JM, Badovinac-Crnjevic T, et al. Trastuzumab emtansine versus treatment of physician’s choice in patients with previously treated HER2-positive metastatic breast cancer (TH3RESA): final overall survival results from a randomised open-label phase 3 trial. Lancet Oncol (2017) 18(6):743–54. doi:10.1016/S1470-2045(17)30313-3
48. Dienstmann R, Tabernero J. Necitumumab, a fully human IgG1 mAb directed against the EGFR for the potential treatment of cancer. Curr Opin Investig Drugs (2010) 11(12):1434–41.
49. Genova C, Hirsch FR. Clinical potential of necitumumab in non-small cell lung carcinoma. Onco Targets Ther (2016) 9:5427–37. doi:10.2147/OTT.S114039
50. Li S, Kussie P, Ferguson KM. Structural basis for EGF receptor inhibition by the therapeutic antibody IMC-11F8. Structure (2008) 16(2):216–27. doi:10.1016/j.str.2007.11.009
51. Thakur MK, Wozniak AJ. Spotlight on necitumumab in the treatment of non-small-cell lung carcinoma. Lung Cancer (Auckl) (2017) 8:13–9. doi:10.2147/LCTT.S104207
52. Tamura Y, Nokihara H, Honda K, Tanabe Y, Asahina H, Yamada Y, et al. Phase I study of the second-generation, recombinant, human EGFR antibody necitumumab in Japanese patients with advanced solid tumors. Cancer Chemother Pharmacol (2016) 78(5):995–1002. doi:10.1007/s00280-016-3154-z
53. Reck M, Thomas M, Kropf-Sanchen C, Mezger J, Socinski MA, Depenbrock H, et al. Necitumumab plus gemcitabine and cisplatin as first-line therapy in patients with stage IV EGFR- expressing squamous non-small-cell lung cancer: German subgroup data from an open-label, randomized controlled phase 3 study (SQUIRE). Oncol Res Treat (2016) 39(9):539–47. doi:10.1159/000448085
54. Battaglin F, Dadduzio V, Bergamo F, Manai C, Schirripa M, Lonardi S, et al. Anti-EGFR monoclonal antibody panitumumab for the treatment of patients with metastatic colorectal cancer: an overview of current practice and future perspectives. Expert Opin Biol Ther (2017) 17(10):1–12. doi:10.1080/14712598.2017.1356815
55. Cohenuram M, Saif MW. Panitumumab the first fully human monoclonal antibody: from the bench to the clinic. Anticancer Drugs (2007) 18(1):7–15. doi:10.1097/CAD.0b013e32800feecb
56. Tay RY, Wong R, Hawkes EA. Treatment of metastatic colorectal cancer: focus on panitumumab. Cancer Manag Res (2015) 7:189–98. doi:10.2147/CMAR.S71821
57. Yang XD, Jia XC, Corvalan JR, Wang P, Davis CG. Development of ABX-EGF, a fully human anti-EGF receptor monoclonal antibody, for cancer therapy. Crit Rev Oncol Hematol (2001) 38(1):17–23. doi:10.1016/S1040-8428(00)00134-7
58. Carrato A, Abad A, Massuti B, Gravalos C, Escudero P, Longo-Munoz F, et al. First-line panitumumab plus FOLFOX4 or FOLFIRI in colorectal cancer with multiple or unresectable liver metastases: a randomised, phase II trial (PLANET-TTD). Eur J Cancer (2017) 81:191–202. doi:10.1016/j.ejca.2017.04.024
59. Baselga J. The EGFR as a target for anticancer therapy – focus on cetuximab. Eur J Cancer (2001) 37(Suppl 4):S16–22. doi:10.1016/S0959-8049(01)00233-7
60. Humblet Y. Cetuximab: an IgG(1) monoclonal antibody for the treatment of epidermal growth factor receptor-expressing tumours. Expert Opin Pharmacother (2004) 5(7):1621–33. doi:10.1517/14656566.5.7.1621
61. Marzi L, Combes E, Vie N, Ayrolles-Torro A, Tosi D, Desigaud D, et al. FOXO3a and the MAPK p38 are activated by cetuximab to induce cell death and inhibit cell proliferation and their expression predicts cetuximab efficacy in colorectal cancer. Br J Cancer (2016) 115(10):1223–33. doi:10.1038/bjc.2016.313
62. Maseki S, Ijichi K, Nakanishi H, Hasegawa Y, Ogawa T, Murakami S. Efficacy of gemcitabine and cetuximab combination treatment in head and neck squamous cell carcinoma. Mol Clin Oncol (2013) 1(5):918–24. doi:10.3892/mco.2013.159
63. Venook AP, Niedzwiecki D, Lenz HJ, Innocenti F, Fruth B, Meyerhardt JA, et al. Effect of first-line chemotherapy combined with cetuximab or bevacizumab on overall survival in patients with KRAS wild-type advanced or metastatic colorectal cancer: a randomized clinical trial. JAMA (2017) 317(23):2392–401. doi:10.1001/jama.2017.7105
64. Ferrara N, Hillan KJ, Gerber HP, Novotny W. Discovery and development of bevacizumab, an anti-VEGF antibody for treating cancer. Nat Rev Drug Discov (2004) 3(5):391–400. doi:10.1038/nrd1381
65. Levin PA, Dowell JE. Spotlight on bevacizumab and its potential in the treatment of malignant pleural mesothelioma: the evidence to date. Onco Targets Ther (2017) 10:2057–66. doi:10.2147/OTT.S113598
66. Mortimer J, Zonder HB, Pal SK. Lessons learned from the bevacizumab experience. Cancer Control (2012) 19(4):309–16. doi:10.1177/107327481201900407
67. Roviello G, Bachelot T, Hudis CA, Curigliano G, Reynolds AR, Petrioli R, et al. The role of bevacizumab in solid tumours: a literature based meta-analysis of randomised trials. Eur J Cancer (2017) 75:245–58. doi:10.1016/j.ejca.2017.01.026
68. Zhao Z, Li J, Ye R, Wu X, Gao L, Niu B. A phase II clinical study of combining FOLFIRI and bevacizumab plus erlotinib in 2nd-line chemotherapy for patients with metastatic colorectal cancer. Medicine (Baltimore) (2017) 96(30):e7182. doi:10.1097/MD.0000000000007182
69. Arrieta O, Zatarain-Barron ZL, Cardona AF, Carmona A, Lopez-Mejia M. Ramucirumab in the treatment of non-small cell lung cancer. Expert Opin Drug Saf (2017) 16(5):637–44. doi:10.1080/14740338.2017.1313226
70. Krupitskaya Y, Wakelee HA. Ramucirumab, a fully human mAb to the transmembrane signaling tyrosine kinase VEGFR-2 for the potential treatment of cancer. Curr Opin Investig Drugs (2009) 10(6):597–605.
71. Spratlin J. Ramucirumab (IMC-1121B): monoclonal antibody inhibition of vascular endothelial growth factor receptor-2. Curr Oncol Rep (2011) 13(2):97–102. doi:10.1007/s11912-010-0149-5
72. Vennepureddy A, Singh P, Rastogi R, Atallah JP, Terjanian T. Evolution of ramucirumab in the treatment of cancer – a review of literature. J Oncol Pharm Pract (2016) 23(7):525–39. doi:10.1177/1078155216655474
73. Chau I, Peck-Radosavljevic M, Borg C, Malfertheiner P, Seitz JF, Park JO, et al. Ramucirumab as second-line treatment in patients with advanced hepatocellular carcinoma following first-line therapy with sorafenib: patient-focused outcome results from the randomised phase III REACH study. Eur J Cancer (2017) 81:17–25. doi:10.1016/j.ejca.2017.05.001
74. Lee HT, Lee JY, Lim H, Lee SH, Moon YJ, Pyo HJ, et al. Molecular mechanism of PD-1/PD-L1 blockade via anti-PD-L1 antibodies atezolizumab and durvalumab. Sci Rep (2017) 7(1):5532. doi:10.1038/s41598-017-06002-8
75. Krishnamurthy A, Jimeno A. Atezolizumab: a novel PD-L1 inhibitor in cancer therapy with a focus in bladder and non-small cell lung cancers. Drugs Today (Barc) (2017) 53(4):217–37. doi:10.1358/dot.2017.53.4.2589163
76. Aydin AM, Woldu SL, Hutchinson RC, Boegemann M, Bagrodia A, Lotan Y, et al. Spotlight on atezolizumab and its potential in the treatment of advanced urothelial bladder cancer. Onco Targets Ther (2017) 10:1487–502. doi:10.2147/OTT.S109453
77. Cha E, Wallin J, Kowanetz M. PD-L1 inhibition with MPDL3280A for solid tumors. Semin Oncol (2015) 42(3):484–7. doi:10.1053/j.seminoncol.2015.02.002
78. Balar AV, Galsky MD, Rosenberg JE, Powles T, Petrylak DP, Bellmunt J, et al. Atezolizumab as first-line treatment in cisplatin-ineligible patients with locally advanced and metastatic urothelial carcinoma: a single-arm, multicentre, phase 2 trial. Lancet (2017) 389(10064):67–76. doi:10.1016/S0140-6736(16)32455-2
79. Jeanson A, Barlesi F. MEDI 4736 (durvalumab) in non-small cell lung cancer. Expert Opin Biol Ther (2017) 17(10):1317–23. doi:10.1080/14712598.2017.1351939
80. Stewart R, Morrow M, Hammond SA, Mulgrew K, Marcus D, Poon E, et al. Identification and characterization of MEDI4736, an antagonistic anti-PD-L1 monoclonal antibody. Cancer Immunol Res (2015) 3(9):1052–62. doi:10.1158/2326-6066.CIR-14-0191
81. Tan S, Liu K, Chai Y, Zhang CW, Gao S, Gao GF, et al. Distinct PD-L1 binding characteristics of therapeutic monoclonal antibody durvalumab. Protein Cell (2017). doi:10.1007/s13238-017-0412-8
82. Lee JM, Cimino-Mathews A, Peer CJ, Zimmer A, Lipkowitz S, Annunziata CM, et al. Safety and clinical activity of the programmed death-ligand 1 inhibitor durvalumab in combination with poly (ADP-ribose) polymerase inhibitor olaparib or vascular endothelial growth factor receptor 1-3 inhibitor cediranib in women’s cancers: a dose-escalation, phase I study. J Clin Oncol (2017) 35(19):2193–202. doi:10.1200/JCO.2016.72.1340
83. Boyerinas B, Jochems C, Fantini M, Heery CR, Gulley JL, Tsang KY, et al. Antibody-dependent cellular cytotoxicity activity of a novel anti-PD-L1 antibody avelumab (MSB0010718C) on human tumor cells. Cancer Immunol Res (2015) 3(10):1148–57. doi:10.1158/2326-6066.CIR-15-0059
84. Hamilton G, Rath B. Avelumab: combining immune checkpoint inhibition and antibody-dependent cytotoxicity. Expert Opin Biol Ther (2017) 17(4):515–23. doi:10.1080/14712598.2017.1294156
85. Liu K, Tan S, Chai Y, Chen D, Song H, Zhang CW, et al. Structural basis of anti-PD-L1 monoclonal antibody avelumab for tumor therapy. Cell Res (2017) 27(1):151–3. doi:10.1038/cr.2016.102
86. Apolo AB, Infante JR, Balmanoukian A, Patel MR, Wang D, Kelly K, et al. Avelumab, an anti-programmed death-ligand 1 antibody, in patients with refractory metastatic urothelial carcinoma: results from a multicenter, phase Ib study. J Clin Oncol (2017) 35(19):2117–24. doi:10.1200/JCO.2016.71.6795
87. Brahmer JR, Drake CG, Wollner I, Powderly JD, Picus J, Sharfman WH, et al. Phase I study of single-agent anti-programmed death-1 (MDX-1106) in refractory solid tumors: safety, clinical activity, pharmacodynamics, and immunologic correlates. J Clin Oncol (2010) 28(19):3167–75. doi:10.1200/JCO.2009.26.7609
88. Guo L, Zhang H, Chen B. Nivolumab as programmed death-1 (PD-1) inhibitor for targeted immunotherapy in tumor. J Cancer (2017) 8(3):410–6. doi:10.7150/jca.17144
89. Venur VA, Joshi M, Nepple KG, Zakharia Y. Spotlight on nivolumab in the treatment of renal cell carcinoma: design, development, and place in therapy. Drug Des Devel Ther (2017) 11:1175–82. doi:10.2147/DDDT.S110209
90. Wang C, Thudium KB, Han M, Wang XT, Huang H, Feingersh D, et al. In vitro characterization of the anti-PD-1 antibody nivolumab, BMS-936558, and in vivo toxicology in non-human primates. Cancer Immunol Res (2014) 2(9):846–56. doi:10.1158/2326-6066.CIR-14-0040
91. Kudo T, Hamamoto Y, Kato K, Ura T, Kojima T, Tsushima T, et al. Nivolumab treatment for oesophageal squamous-cell carcinoma: an open-label, multicentre, phase 2 trial. Lancet Oncol (2017) 18(5):631–9. doi:10.1016/S1470-2045(17)30181-X
92. Longoria TC, Tewari KS. Evaluation of the pharmacokinetics and metabolism of pembrolizumab in the treatment of melanoma. Expert Opin Drug Metab Toxicol (2016) 12(10):1247–53. doi:10.1080/17425255.2016.1216976
93. McDermott J, Jimeno A. Pembrolizumab: PD-1 inhibition as a therapeutic strategy in cancer. Drugs Today (Barc) (2015) 51(1):7–20. doi:10.1358/dot.2015.51.1.2250387
94. Poole RM. Pembrolizumab: first global approval. Drugs (2014) 74(16):1973–81. doi:10.1007/s40265-014-0314-5
95. Rihawi K, Gelsomino F, Sperandi F, Melotti B, Fiorentino M, Casolari L, et al. Pembrolizumab in the treatment of metastatic non-small cell lung cancer: a review of current evidence. Ther Adv Respir Dis (2017) 11(9):353–73. doi:10.1177/1753465817725486
96. Zinzani PL, Ribrag V, Moskowitz CH, Michot JM, Kuruvilla J, Balakumaran A, et al. Safety and tolerability of pembrolizumab in patients with relapsed/refractory primary mediastinal large B-cell lymphoma. Blood (2017) 130(3):267–70. doi:10.1182/blood-2016-12-758383
97. Letendre P, Monga V, Milhem M, Zakharia Y. Ipilimumab: from preclinical development to future clinical perspectives in melanoma. Future Oncol (2017) 13(7):625–36. doi:10.2217/fon-2016-0385
98. Movva S, Verschraegen C. The monoclonal antibody to cytotoxic T lymphocyte antigen 4, ipilimumab (MDX-010), a novel treatment strategy in cancer management. Expert Opin Biol Ther (2009) 9(2):231–41. doi:10.1517/14712590802643347
99. Wolchok JD, Hodi FS, Weber JS, Allison JP, Urba WJ, Robert C, et al. Development of ipilimumab: a novel immunotherapeutic approach for the treatment of advanced melanoma. Ann N Y Acad Sci (2013) 1291:1–13. doi:10.1111/nyas.12180
100. Williams NL, Wuthrick EJ, Kim H, Palmer JD, Garg S, Eldredge-Hindy H, et al. Phase 1 study of ipilimumab combined with whole brain radiation therapy or radiosurgery for melanoma patients with brain metastases. Int J Radiat Oncol Biol Phys (2017) 99(1):22–30. doi:10.1016/j.ijrobp.2017.05.028
101. Body JJ, Facon T, Coleman RE, Lipton A, Geurs F, Fan M, et al. A study of the biological receptor activator of nuclear factor-kappaB ligand inhibitor, denosumab, in patients with multiple myeloma or bone metastases from breast cancer. Clin Cancer Res (2006) 12(4):1221–8. doi:10.1158/1078-0432.CCR-05-1933
102. Burkiewicz JS, Scarpace SL, Bruce SP. Denosumab in osteoporosis and oncology. Ann Pharmacother (2009) 43(9):1445–55. doi:10.1345/aph.1M102
103. Prommer E. Palliative oncology: denosumab. Am J Hosp Palliat Care (2015) 32(5):568–72. doi:10.1177/1049909114539035
104. Savvidou OD, Bolia IK, Chloros GD, Papanastasiou J, Koutsouradis P, Papagelopoulos PJ. Denosumab: current use in the treatment of primary bone tumors. Orthopedics (2017) 40(4):204–10. doi:10.3928/01477447-20170627-04
105. Saad F, Carles J, Gillessen S, Heidenreich A, Heinrich D, Gratt J, et al. Radium-223 and concomitant therapies in patients with metastatic castration-resistant prostate cancer: an international, early access, open-label, single-arm phase 3b trial. Lancet Oncol (2016) 17(9):1306–16. doi:10.1016/S1470-2045(16)30173-5
106. Hong EE, Erickson H, Lutz RJ, Whiteman KR, Jones G, Kovtun Y, et al. Design of coltuximab ravtansine, a CD19-targeting antibody-drug conjugate (ADC) for the treatment of B-cell malignancies: structure-activity relationships and preclinical evaluation. Mol Pharm (2015) 12(6):1703–16. doi:10.1021/acs.molpharmaceut.5b00175
107. O’Sullivan Coyne G, Burotto M. MABp1 for the treatment of colorectal cancer. Expert Opin Biol Ther (2017) 17(9):1155–61. doi:10.1080/14712598.2017.1347631
108. Hickish T, Andre T, Wyrwicz L, Saunders M, Sarosiek T, Kocsis J, et al. MABp1 as a novel antibody treatment for advanced colorectal cancer: a randomised, double-blind, placebo-controlled, phase 3 study. Lancet Oncol (2017) 18(2):192–201. doi:10.1016/S1470-2045(17)30006-2
109. Bartholomew J, Washington T, Bergeron S, Nielson D, Saggio J, Quirk L. Dinutuximab: a novel immunotherapy in the treatment of pediatric patients with high-risk neuroblastoma. J Pediatr Oncol Nurs (2016) 34(1):5–12. doi:10.1177/1043454216659448
110. Hoy SM. Dinutuximab: a review in high-risk neuroblastoma. Target Oncol (2016) 11(2):247–53. doi:10.1007/s11523-016-0420-2
111. Soman G, Kallarakal AT, Michiel D, Yang X, Saptharish N, Jiang H, et al. Analytical characterization of ch14.18: a mouse-human chimeric disialoganglioside-specific therapeutic antibody. MAbs (2012) 4(1):84–100. doi:10.4161/mabs.4.1.18566
112. Marachelian A, Desai A, Balis F, Katzenstein H, Qayed M, Armstrong M, et al. Comparative pharmacokinetics, safety, and tolerability of two sources of ch14.18 in pediatric patients with high-risk neuroblastoma following myeloablative therapy. Cancer Chemother Pharmacol (2016) 77(2):405–12. doi:10.1007/s00280-015-2955-9
113. Adkins JC, Spencer CM. Edrecolomab (monoclonal antibody 17-1A). Drugs (1998) 56(4):619–26; discussion 27–8. doi:10.2165/00003495-199856040-00011
114. Frodin JE, Biberfeld P, Christensson B, Pihlstedt P, Shetye J, Makower J, et al. Treatment of gastrointestinal tumors with MAb17-1A. J Chemother (1989) 1(4 Suppl):1209–11.
115. Gires O, Bauerle PA. EpCAM as a target in cancer therapy. J Clin Oncol (2010) 28(15):e239–40; author reply e241–2. doi:10.1200/JCO.2009.26.8540
116. Schmoll HJ, Arnold D. When wishful thinking leads to a misty-eyed appraisal: the story of the adjuvant colon cancer trials with edrecolomab. J Clin Oncol (2009) 27(12):1926–9. doi:10.1200/JCO.2008.20.6284
117. Chelius D, Ruf P, Gruber P, Ploscher M, Liedtke R, Gansberger E, et al. Structural and functional characterization of the trifunctional antibody catumaxomab. MAbs (2010) 2(3):309–19. doi:10.4161/mabs.2.3.11791
118. Eskander RN, Tewari KS. Epithelial cell-adhesion molecule-directed trifunctional antibody immunotherapy for symptom management of advanced ovarian cancer. Clin Pharmacol (2013) 5(Suppl 1):55–61. doi:10.2147/CPAA.S45885
119. Sebastian M, Passlick B, Friccius-Quecke H, Jager M, Lindhofer H, Kanniess F, et al. Treatment of non-small cell lung cancer patients with the trifunctional monoclonal antibody catumaxomab (anti-EpCAM x anti-CD3): a phase I study. Cancer Immunol Immunother (2007) 56(10):1637–44. doi:10.1007/s00262-007-0310-7
120. Seimetz D, Lindhofer H, Bokemeyer C. Development and approval of the trifunctional antibody catumaxomab (anti-EpCAM x anti-CD3) as a targeted cancer immunotherapy. Cancer Treat Rev (2010) 36(6):458–67. doi:10.1016/j.ctrv.2010.03.001
121. Kurbacher CM, Horn O, Kurbacher JA, Herz S, Kurbacher AT, Hildenbrand R, et al. Outpatient intraperitoneal catumaxomab therapy for malignant ascites related to advanced gynecologic neoplasms. Oncologist (2015) 20(11):1333–41. doi:10.1634/theoncologist.2015-0076
122. Friberg G, Reese D. Blinatumomab (Blincyto(R)); lessons learned from the bispecific t-cell engager (BiTE(R)) in acute lymphocytic leukemia (ALL). Ann Oncol (2017) 28(8):2009–12. doi:10.1093/annonc/mdx150
123. Kaplan JB, Grischenko M, Giles FJ. Blinatumomab for the treatment of acute lymphoblastic leukemia. Invest New Drugs (2015) 33(6):1271–9. doi:10.1007/s10637-015-0289-4
124. Nagorsen D, Kufer P, Baeuerle PA, Bargou R. Blinatumomab: a historical perspective. Pharmacol Ther (2012) 136(3):334–42. doi:10.1016/j.pharmthera.2012.07.013
125. Sanders S, Stewart DA. Targeting non-Hodgkin lymphoma with blinatumomab. Expert Opin Biol Ther (2017) 17(8):1013–7. doi:10.1080/14712598.2017.1334053
126. Goebeler ME, Knop S, Viardot A, Kufer P, Topp MS, Einsele H, et al. Bispecific T-cell engager (BiTE) antibody construct blinatumomab for the treatment of patients with relapsed/refractory non-Hodgkin lymphoma: final results from a phase I study. J Clin Oncol (2016) 34(10):1104–11. doi:10.1200/JCO.2014.59.1586
127. Al-Sawaf O, Fischer K, Engelke A, Pflug N, Hallek M, Goede V. Obinutuzumab in chronic lymphocytic leukemia: design, development and place in therapy. Drug Des Devel Ther (2017) 11:295–304. doi:10.2147/DDDT.S104869
128. Klein C, Lammens A, Schafer W, Georges G, Schwaiger M, Mossner E, et al. Epitope interactions of monoclonal antibodies targeting CD20 and their relationship to functional properties. MAbs (2013) 5(1):22–33. doi:10.4161/mabs.22771
129. Mossner E, Brunker P, Moser S, Puntener U, Schmidt C, Herter S, et al. Increasing the efficacy of CD20 antibody therapy through the engineering of a new type II anti-CD20 antibody with enhanced direct and immune effector cell-mediated B-cell cytotoxicity. Blood (2010) 115(22):4393–402. doi:10.1182/blood-2009-06-225979
130. Owen C, Stewart DA. Obinutuzumab for the treatment of lymphoproliferative disorders. Expert Opin Biol Ther (2012) 12(3):343–51. doi:10.1517/14712598.2012.657622
131. Tobinai K, Klein C, Oya N, Fingerle-Rowson GA. Review of obinutuzumab (GA101), a novel type II anti-CD20 monoclonal antibody, for the treatment of patients with B-cell malignancies. Adv Ther (2017) 34(2):324–56. doi:10.1007/s12325-016-0451-1
132. Cheson BD, Trask PC, Gribben JG, Dimier N, Kimby E, Lugtenburg PJ, et al. Health-related quality of life and symptoms in patients with rituximab-refractory indolent non-Hodgkin lymphoma treated in the phase III GADOLIN study with obinutuzumab plus bendamustine versus bendamustine alone. Ann Hematol (2017) 96(2):253–9. doi:10.1007/s00277-016-2878-5
133. Castillo J, Milani C, Mendez-Allwood D. Ofatumumab, a second-generation anti-CD20 monoclonal antibody, for the treatment of lymphoproliferative and autoimmune disorders. Expert Opin Investig Drugs (2009) 18(4):491–500. doi:10.1517/13543780902832679
134. Karlin L, Coiffier B. Ofatumumab in the treatment of non-Hodgkin’s lymphomas. Expert Opin Biol Ther (2015) 15(7):1085–91. doi:10.1517/14712598.2015.1055241
135. Laurenti L, Innocenti I, Autore F, Sica S, Efremov DG. New developments in the management of chronic lymphocytic leukemia: role of ofatumumab. Onco Targets Ther (2016) 9:421–9. doi:10.2147/OTT.S72845
136. Flinn IW, Ruppert AS, Harwin W, Waterhouse D, Papish S, Jones JA, et al. A phase II study of two dose levels of ofatumumab induction followed by maintenance therapy in symptomatic, previously untreated chronic lymphocytic leukemia. Am J Hematol (2016) 91(10):1020–5. doi:10.1002/ajh.24468
137. Prasad V. The withdrawal of drugs for commercial reasons: the incomplete story of tositumomab. JAMA Intern Med (2014) 174(12):1887–8. doi:10.1001/jamainternmed.2014.5756
138. Srinivasan A, Mukherji SK. Tositumomab and iodine I 131 tositumomab (Bexaar). AJNR Am J Neuroradiol (2011) 32(4):637–8. doi:10.3174/ajnr.A2593
139. Zelenetz AD. A clinical and scientific overview of tositumomab and iodine I 131 tositumomab. Semin Oncol (2003) 30(2 Suppl 4):22–30. doi:10.1053/sonc.2003.23803
140. Quackenbush RC, Horner TJ, Williams VC, Giampietro P, Lin TS. Patients with relapsed follicular lymphoma treated with rituximab versus tositumomab and iodine I-131 tositumomab. Leuk Lymphoma (2015) 56(3):779–81. doi:10.3109/10428194.2014.927461
141. Grillo-Lopez AJ. Zevalin: the first radioimmunotherapy approved for the treatment of lymphoma. Expert Rev Anticancer Ther (2002) 2(5):485–93. doi:10.1586/14737140.2.5.485
142. Krasner C, Joyce RM. Zevalin: 90yttrium labeled anti-CD20 (ibritumomab tiuxetan), a new treatment for non-Hodgkin’s lymphoma. Curr Pharm Biotechnol (2001) 2(4):341–9. doi:10.2174/1389201013378545
143. Rizzieri D. Zevalin((R)) (ibritumomab tiuxetan): after more than a decade of treatment experience, what have we learned? Crit Rev Oncol Hematol (2016) 105:5–17. doi:10.1016/j.critrevonc.2016.07.008
144. Hertzberg M, Gandhi MK, Trotman J, Butcher B, Taper J, Johnston A, et al. Early treatment intensification with R-ICE and 90Y-ibritumomab tiuxetan (Zevalin)-BEAM stem cell transplantation in patients with high-risk diffuse large B-cell lymphoma patients and positive interim PET after 4 cycles of R-CHOP-14. Haematologica (2017) 102(2):356–63. doi:10.3324/haematol.2016.154039
145. Anderson DR, Grillo-Lopez A, Varns C, Chambers KS, Hanna N. Targeted anti-cancer therapy using rituximab, a chimaeric anti-CD20 antibody (IDEC-C2B8) in the treatment of non-Hodgkin’s B-cell lymphoma. Biochem Soc Trans (1997) 25(2):705–8. doi:10.1042/bst0250705
146. Boross P, Leusen JH. Mechanisms of action of CD20 antibodies. Am J Cancer Res (2012) 2(6):676–90.
147. Pavanello F, Zucca E, Ghielmini M. Rituximab: 13 open questions after 20years of clinical use. Cancer Treat Rev (2017) 53:38–46. doi:10.1016/j.ctrv.2016.11.015
148. Scott SD. Rituximab: a new therapeutic monoclonal antibody for non-Hodgkin’s lymphoma. Cancer Pract (1998) 6(3):195–7. doi:10.1046/j.1523-5394.1998.006003195.x
149. Weiner GJ. Rituximab: mechanism of action. Semin Hematol (2010) 47(2):115–23. doi:10.1053/j.seminhematol.2010.01.011
150. Larsen JT, Shanafelt TD, Leis JF, LaPlant B, Call T, Pettinger A, et al. Akt inhibitor MK-2206 in combination with bendamustine and rituximab in relapsed or refractory chronic lymphocytic leukemia: results from the N1087 alliance study. Am J Hematol (2017) 92(8):759–63. doi:10.1002/ajh.24762
151. Betts AM, Haddish-Berhane N, Tolsma J, Jasper P, King LE, Sun Y, et al. Preclinical to clinical translation of antibody-drug conjugates using PK/PD modeling: a retrospective analysis of inotuzumab ozogamicin. AAPS J (2016) 18(5):1101–16. doi:10.1208/s12248-016-9929-7
152. DiJoseph JF, Armellino DC, Boghaert ER, Khandke K, Dougher MM, Sridharan L, et al. Antibody-targeted chemotherapy with CMC-544: a CD22-targeted immunoconjugate of calicheamicin for the treatment of B-lymphoid malignancies. Blood (2004) 103(5):1807–14. doi:10.1182/blood-2003-07-2466
153. Lamb YN. Inotuzumab ozogamicin: first global approval. Drugs (2017) 77(14):1603–10. doi:10.1007/s40265-017-0802-5
154. Wong BY, Dang NH. Inotuzumab ozogamicin as novel therapy in lymphomas. Expert Opin Biol Ther (2010) 10(8):1251–8. doi:10.1517/14712598.2010.498418
155. Goy A, Forero A, Wagner-Johnston N, Christopher Ehmann W, Tsai M, Hatake K, et al. A phase 2 study of inotuzumab ozogamicin in patients with indolent B-cell non-Hodgkin lymphoma refractory to rituximab alone, rituximab and chemotherapy, or radioimmunotherapy. Br J Haematol (2016) 174(4):571–81. doi:10.1111/bjh.14094
156. Ansell SM. Brentuximab vedotin: delivering an antimitotic drug to activated lymphoma cells. Expert Opin Investig Drugs (2011) 20(1):99–105. doi:10.1517/13543784.2011.542147
157. Deng C, Pan B, O’Connor OA. Brentuximab vedotin. Clin Cancer Res (2013) 19(1):22–7. doi:10.1158/1078-0432.CCR-12-0290
158. Furtado M, Rule S. Emerging pharmacotherapy for relapsed or refractory Hodgkin’s lymphoma: focus on brentuximab vedotin. Clin Med Insights Oncol (2012) 6:31–9. doi:10.4137/CMO.S6637
159. Scott LJ. Brentuximab vedotin: a review in CD30-positive Hodgkin lymphoma. Drugs (2017) 77(4):435–45. doi:10.1007/s40265-017-0705-5
160. Ramsey SD, Nademanee A, Masszi T, Holowiecki J, Abidi M, Chen A, et al. Quality of life results from a phase 3 study of brentuximab vedotin consolidation following autologous haematopoietic stem cell transplant for persons with Hodgkin lymphoma. Br J Haematol (2016) 175(5):860–7. doi:10.1111/bjh.14316
161. Borate U, Mehta A, Reddy V, Tsai M, Josephson N, Schnadig I. Treatment of CD30-positive systemic mastocytosis with brentuximab vedotin. Leuk Res (2016) 44:25–31. doi:10.1016/j.leukres.2016.02.010
162. Breccia M, Lo-Coco F. Gemtuzumab ozogamicin for the treatment of acute promyelocytic leukemia: mechanisms of action and resistance, safety and efficacy. Expert Opin Biol Ther (2011) 11(2):225–34. doi:10.1517/14712598.2011.543895
163. Godwin CD, Gale RP, Walter RB. Gemtuzumab ozogamicin in acute myeloid leukemia. Leukemia (2017) 31(9):1855–68. doi:10.1038/leu.2017.187
164. Hamann PR, Hinman LM, Hollander I, Beyer CF, Lindh D, Holcomb R, et al. Gemtuzumab ozogamicin, a potent and selective anti-CD33 antibody-calicheamicin conjugate for treatment of acute myeloid leukemia. Bioconjug Chem (2002) 13(1):47–58. doi:10.1021/bc0100206
165. Hutter-Kronke ML, Benner A, Dohner K, Krauter J, Weber D, Moessner M, et al. Salvage therapy with high-dose cytarabine and mitoxantrone in combination with all-trans retinoic acid and gemtuzumab ozogamicin in acute myeloid leukemia refractory to first induction therapy. Haematologica (2016) 101(7):839–45. doi:10.3324/haematol.2015.141622
166. de Weers M, Tai YT, van der Veer MS, Bakker JM, Vink T, Jacobs DC, et al. Daratumumab, a novel therapeutic human CD38 monoclonal antibody, induces killing of multiple myeloma and other hematological tumors. J Immunol (2011) 186(3):1840–8. doi:10.4049/jimmunol.1003032
167. Laubach JP, Paba Prada CE, Richardson PG, Longo DL. Daratumumab, elotuzumab, and the development of therapeutic monoclonal antibodies in multiple myeloma. Clin Pharmacol Ther (2017) 101(1):81–8. doi:10.1002/cpt.550
168. McKeage K. Daratumumab: first global approval. Drugs (2016) 76(2):275–81. doi:10.1007/s40265-015-0536-1
169. Touzeau C, Moreau P. Daratumumab for the treatment of multiple myeloma. Expert Opin Biol Ther (2017) 17(7):887–93. doi:10.1080/14712598.2017.1322578
170. Dimopoulos MA, Oriol A, Nahi H, San-Miguel J, Bahlis NJ, Usmani SZ, et al. Daratumumab, lenalidomide, and dexamethasone for multiple myeloma. N Engl J Med (2016) 375(14):1319–31. doi:10.1056/NEJMoa1607751
171. Chen WC, Kanate AS, Craig M, Petros WP, Hazlehurst LA. Emerging combination therapies for the management of multiple myeloma: the role of elotuzumab. Cancer Manag Res (2017) 9:307–14. doi:10.2147/CMAR.S117477
172. Liu YC, Szmania S, van Rhee F. Profile of elotuzumab and its potential in the treatment of multiple myeloma. Blood Lymphat Cancer (2014) 2014(4):15–27. doi:10.2147/BLCTT.S49780
173. Lonial S, Kaufman J, Laubach J, Richardson P. Elotuzumab: a novel anti-CS1 monoclonal antibody for the treatment of multiple myeloma. Expert Opin Biol Ther (2013) 13(12):1731–40. doi:10.1517/14712598.2013.847919
174. Magen H, Muchtar E. Elotuzumab: the first approved monoclonal antibody for multiple myeloma treatment. Ther Adv Hematol (2016) 7(4):187–95. doi:10.1177/2040620716652862
175. Weisel K. Spotlight on elotuzumab in the treatment of multiple myeloma: the evidence to date. Onco Targets Ther (2016) 9:6037–48. doi:10.2147/OTT.S94531
176. Jakubowiak A, Offidani M, Pegourie B, De La Rubia J, Garderet L, Laribi K, et al. Randomized phase 2 study: elotuzumab plus bortezomib/dexamethasone vs bortezomib/dexamethasone for relapsed/refractory MM. Blood (2016) 127(23):2833–40. doi:10.1182/blood-2016-01-694604
177. Ferrajoli A, O’Brien S, Keating MJ. Alemtuzumab: a novel monoclonal antibody. Expert Opin Biol Ther (2001) 1(6):1059–65. doi:10.1517/14712598.1.6.1059
178. Warner JL, Arnason JE. Alemtuzumab use in relapsed and refractory chronic lymphocytic leukemia: a history and discussion of future rational use. Ther Adv Hematol (2012) 3(6):375–89. doi:10.1177/2040620712458949
179. Wei G, Wang J, Huang H, Zhao Y. Novel immunotherapies for adult patients with B-lineage acute lymphoblastic leukemia. J Hematol Oncol (2017) 10(1):150. doi:10.1186/s13045-017-0516-x
180. Westermark B, Claesson-Welsh L, Heldin CH. Structural and functional aspects of platelet-derived growth factor and its receptors. Ciba Found Symp (1990) 150:6–14; discussion 14–22.
181. Joglekar-Javadekar M, Van Laere S, Bourne M, Moalwi M, Finetti P, Vermeulen PB, et al. Characterization and targeting of platelet-derived growth factor receptor alpha (PDGFRA) in inflammatory breast cancer (IBC). Neoplasia (2017) 19(7):564–73. doi:10.1016/j.neo.2017.03.002
182. Yang WJ, Shen XJ, Ma XX, Tan ZG, Song Y, Guo YT, et al. Correlation of human epidermal growth factor receptor protein expression and colorectal cancer. World J Gastroenterol (2015) 21(28):8687–96. doi:10.3748/wjg.v21.i28.8687
183. Iqbal N, Iqbal N. Human epidermal growth factor receptor 2 (HER2) in cancers: overexpression and therapeutic implications. Mol Biol Int (2014) 2014:852748. doi:10.1155/2014/852748
184. Bregman MD, Sipes NJ. Transformation-related growth factors and their receptors. Int J Cell Cloning (1986) 4(4):224–36. doi:10.1002/stem.5530040401
185. Normanno N, De Luca A, Bianco C, Strizzi L, Mancino M, Maiello MR, et al. Epidermal growth factor receptor (EGFR) signaling in cancer. Gene (2006) 366(1):2–16. doi:10.1016/j.gene.2005.10.018
186. Holmes K, Roberts OL, Thomas AM, Cross MJ. Vascular endothelial growth factor receptor-2: structure, function, intracellular signalling and therapeutic inhibition. Cell Signal (2007) 19(10):2003–12. doi:10.1016/j.cellsig.2007.05.013
187. Shibuya M. Vascular endothelial growth factor (VEGF) and its receptor (VEGFR) signaling in angiogenesis: a crucial target for anti- and pro-angiogenic therapies. Genes Cancer (2011) 2(12):1097–105. doi:10.1177/1947601911423031
188. NCBI. Available from: http://www.ncbi.nlm.nih.gov/protein.
189. HCDM. Available from: http://www.hcdm.org/index.php/molecule-information
190. Kosmaczewska A, Ciszak L, Bocko D, Frydecka I. Expression and functional significance of CTLA-4, a negative regulator of T cell activation. Arch Immunol Ther Exp (Warsz) (2001) 49(1):39–46.
191. Wei S, Teitelbaum SL, Wang MW, Ross FP. Receptor activator of nuclear factor-kappa b ligand activates nuclear factor-kappa b in osteoclast precursors. Endocrinology (2001) 142(3):1290–5. doi:10.1210/endo.142.3.8031
192. Boyce BF, Xing L. Biology of RANK, RANKL, and osteoprotegerin. Arthritis Res Ther (2007) 9(Suppl 1):S1. doi:10.1186/ar2165
193. Roux S, Amazit L, Meduri G, Guiochon-Mantel A, Milgrom E, Mariette X. RANK (receptor activator of nuclear factor kappa B) and RANK ligand are expressed in giant cell tumors of bone. Am J Clin Pathol (2002) 117(2):210–6. doi:10.1309/BPET-F2PE-P2BD-J3P3
194. Schroder JM. Peptides and cytokines. Arch Dermatol Res (1992) 284(Suppl 1):S22–6. doi:10.1007/BF00638236
195. Navid F, Santana VM, Barfield RC. Anti-GD2 antibody therapy for GD2-expressing tumors. Curr Cancer Drug Targets (2010) 10(2):200–9. doi:10.2174/156800910791054167
196. Modak S, Gerald W, Cheung NK. Disialoganglioside GD2 and a novel tumor antigen: potential targets for immunotherapy of desmoplastic small round cell tumor. Med Pediatr Oncol (2002) 39(6):547–51. doi:10.1002/mpo.10151
197. Patriarca C, Macchi RM, Marschner AK, Mellstedt H. Epithelial cell adhesion molecule expression (CD326) in cancer: a short review. Cancer Treat Rev (2012) 38(1):68–75. doi:10.1016/j.ctrv.2011.04.002
198. Trzpis M, McLaughlin PM, de Leij LM, Harmsen MC. Epithelial cell adhesion molecule: more than a carcinoma marker and adhesion molecule. Am J Pathol (2007) 171(2):386–95. doi:10.2353/ajpath.2007.070152
199. Pervin S, Chakraborty M, Bhattacharya-Chatterjee M, Zeytin H, Foon KA, Chatterjee SK. Induction of antitumor immunity by an anti-idiotype antibody mimicking carcinoembryonic antigen. Cancer Res (1997) 57(4):728–34.
200. Wu L, Huang P, Wang F, Li D, Xie E, Zhang Y, et al. Relationship between serum CA19-9 and CEA levels and prognosis of pancreatic cancer. Ann Transl Med (2015) 3(21):328. doi:10.3978/j.issn.2305-5839.2015.11.17
201. Reece DE, Foon KA, Bhattarcharya-Chatterjee M, Adkins D, Broun ER, Connaghan DG, et al. Use of the anti-idiotype breast cancer vaccine 11D10 in conjunction with autologous stem cell transplantation in patients with metastatic breast cancer. Clin Breast Cancer (2003) 3(Suppl 4):S152–7. doi:10.3816/CBC.2003.s.005
202. White S, Taetle R, Seligman PA, Rutherford M, Trowbridge IS. Combinations of anti-transferrin receptor monoclonal antibodies inhibit human tumor cell growth in vitro and in vivo: evidence for synergistic antiproliferative effects. Cancer Res (1990) 50(19):6295–301.
203. Sabbatini P, Dupont J, Aghajanian C, Derosa F, Poynor E, Anderson S, et al. Phase I study of abagovomab in patients with epithelial ovarian, fallopian tube, or primary peritoneal cancer. Clin Cancer Res (2006) 12(18):5503–10. doi:10.1158/1078-0432.CCR-05-2670
204. Marshall DC, Lyman SK, McCauley S, Kovalenko M, Spangler R, Liu C, et al. Selective allosteric inhibition of MMP9 is efficacious in preclinical models of ulcerative colitis and colorectal cancer. PLoS One (2015) 10(5):e0127063. doi:10.1371/journal.pone.0127063
205. Appleby TC, Greenstein AE, Hung M, Liclican A, Velasquez M, Villasenor AG, et al. Biochemical characterization and structure determination of a potent, selective antibody inhibitor of human MMP9. J Biol Chem (2017) 292(16):6810–20. doi:10.1074/jbc.M116.760579
206. Bellmunt J, Orsola A, Sonpavde G. Precision and predictive medicine in urothelial cancer: are we making progress? Eur Urol (2015) 68(4):547–9. doi:10.1016/j.eururo.2015.04.025
207. Onrust SV, Wiseman LR. Basiliximab. Drugs (1999) 57(2):207–13; discussion 14. doi:10.2165/00003495-199957020-00006
208. Zhao XY, Subramanyam B, Sarapa N, Golfier S, Dinter H. Novel antibody therapeutics targeting mesothelin in solid tumors. Clin Cancer Drugs (2016) 3(2):76–86. doi:10.2174/2212697X03666160218215744
209. Antonioli L, Yegutkin GG, Pacher P, Blandizzi C, Hasko G. Anti-CD73 in cancer immunotherapy: awakening new opportunities. Trends Cancer (2016) 2(2):95–109. doi:10.1016/j.trecan.2016.01.003
210. Antonioli L, Blandizzi C, Malavasi F, Ferrari D, Hasko G. Anti-CD73 immunotherapy: a viable way to reprogram the tumor microenvironment. Oncoimmunology (2016) 5(9):e1216292. doi:10.1080/2162402X.2016.1216292
211. Beavis PA, Stagg J, Darcy PK, Smyth MJ. CD73: a potent suppressor of antitumor immune responses. Trends Immunol (2012) 33(5):231–7. doi:10.1016/j.it.2012.02.009
212. Goel G, Sun W. Novel approaches in the management of pancreatic ductal adenocarcinoma: potential promises for the future. J Hematol Oncol (2015) 8:44. doi:10.1186/s13045-015-0141-5
213. Cannarile MA, Weisser M, Jacob W, Jegg AM, Ries CH, Ruttinger D. Colony-stimulating factor 1 receptor (CSF1R) inhibitors in cancer therapy. J Immunother Cancer (2017) 5(1):53. doi:10.1186/s40425-017-0257-y
214. Dinarello CA. An expanding role for interleukin-1 blockade from gout to cancer. Mol Med (2014) 20(Suppl 1):S43–58. doi:10.2119/molmed.2014.00232
215. Rosen LS, Hurwitz HI, Wong MK, Goldman J, Mendelson DS, Figg WD, et al. A phase I first-in-human study of TRC105 (Anti-Endoglin Antibody) in patients with advanced cancer. Clin Cancer Res (2012) 18(17):4820–9. doi:10.1158/1078-0432.CCR-12-0098
216. Zheng B, Wong P, Yang W, Narla R, Burgess M, Escoubet L, et al. Abstract 2009: CC-90002 (anti-CD47 antibody) in vivo anti-tumor activity is associated with an increase in M1-polarized macrophages. Cancer Res (2017) 77(13 Suppl):2009. doi:10.1158/1538-7445.am2017-2009
217. Dhodapkar MV, Sznol M, Zhao B, Wang D, Carvajal RD, Keohan ML, et al. Induction of antigen-specific immunity with a vaccine targeting NY-ESO-1 to the dendritic cell receptor DEC-205. Sci Transl Med (2014) 6(232):232ra51. doi:10.1126/scitranslmed.3008068
218. Klein C, Waldhauer I, Nicolini VG, Freimoser-Grundschober A, Nayak T, Vugts DJ, et al. Cergutuzumab amunaleukin (CEA-IL2v), a CEA-targeted IL-2 variant-based immunocytokine for combination cancer immunotherapy: overcoming limitations of aldesleukin and conventional IL-2-based immunocytokines. Oncoimmunology (2017) 6(3):e1277306. doi:10.1080/2162402X.2016.1277306
219. Wasilewska A, Winiarska M, Olszewska M, Rudnicka L. Interleukin-17 inhibitors. A new era in treatment of psoriasis and other skin diseases. Postepy Dermatol Alergol (2016) 33(4):247–52. doi:10.5114/ada.2016.61599
220. Rosevear HM, Lightfoot AJ, Griffith TS. Conatumumab, a fully human mAb against death receptor 5 for the treatment of cancer. Curr Opin Investig Drugs (2010) 11(6):688–98.
221. Wagner K, Schulz P, Scholz A, Wiedenmann B, Menrad A. The targeted immunocytokine L19-IL2 efficiently inhibits the growth of orthotopic pancreatic cancer. Clin Cancer Res (2008) 14(15):4951–60. doi:10.1158/1078-0432.CCR-08-0157
222. Smith DC, Eisenberg PD, Manikhas G, Chugh R, Gubens MA, Stagg RJ, et al. A phase I dose escalation and expansion study of the anticancer stem cell agent demcizumab (anti-DLL4) in patients with previously treated solid tumors. Clin Cancer Res (2014) 20(24):6295–303. doi:10.1158/1078-0432.CCR-14-1373
223. Camidge DR, Herbst RS, Gordon MS, Eckhardt SG, Kurzrock R, Durbin B, et al. A phase I safety and pharmacokinetic study of the death receptor 5 agonistic antibody PRO95780 in patients with advanced malignancies. Clin Cancer Res (2010) 16(4):1256–63. doi:10.1158/1078-0432.CCR-09-1267
224. Taetle R, Dos Santos B, Ohsugi Y, Koishihara Y, Yamada Y, Messner H, et al. Effects of combined antigrowth factor receptor treatment on in vitro growth of multiple myeloma. J Natl Cancer Inst (1994) 86(6):450–5. doi:10.1093/jnci/86.6.450
225. Cassier PA, Italiano A, Gomez-Roca CA, Le Tourneau C, Toulmonde M, Cannarile MA, et al. CSF1R inhibition with emactuzumab in locally advanced diffuse-type tenosynovial giant cell tumours of the soft tissue: a dose-escalation and dose-expansion phase 1 study. Lancet Oncol (2015) 16(8):949–56. doi:10.1016/S1470-2045(15)00132-1
226. Wirth M, Heidenreich A, Gschwend JE, Gil T, Zastrow S, Laniado M, et al. A multicenter phase 1 study of EMD 525797 (DI17E6), a novel humanized monoclonal antibody targeting alphav integrins, in progressive castration-resistant prostate cancer with bone metastases after chemotherapy. Eur Urol (2014) 65(5):897–904. doi:10.1016/j.eururo.2013.05.051
227. Rosen LS, Goldman JW, Algazi AP, Turner PK, Moser B, Hu T, et al. A first-in-human phase I study of a bivalent MET antibody, emibetuzumab (LY2875358), as monotherapy and in combination with erlotinib in advanced cancer. Clin Cancer Res (2017) 23(8):1910–9. doi:10.1158/1078-0432.CCR-16-1418
228. Leonard JP, Goldenberg DM. Preclinical and clinical evaluation of epratuzumab (anti-CD22 IgG) in B-cell malignancies. Oncogene (2007) 26(25):3704–13. doi:10.1038/sj.onc.1210370
229. D’Arcangelo M, Cappuzzo F. Focus on the potential role of ficlatuzumab in the treatment of non-small cell lung cancer. Biologics (2013) 7:61–8. doi:10.2147/BTT.S28908
230. Beltran PJ, Chung YA, Moody G, Mitchell P, Cajulis E, Vonderfecht S, et al. Efficacy of ganitumab (AMG 479), alone and in combination with rapamycin, in Ewing’s and osteogenic sarcoma models. J Pharmacol Exp Ther (2011) 337(3):644–54. doi:10.1124/jpet.110.178400
231. Trivedi A, Stienen S, Zhu M, Li H, Yuraszeck T, Gibbs J, et al. Clinical pharmacology and translational aspects of bispecific antibodies. Clin Transl Sci (2017) 10(3):147–62. doi:10.1111/cts.12459
232. Fan G, Wang Z, Hao M, Li J. Bispecific antibodies and their applications. J Hematol Oncol (2015) 8:130. doi:10.1186/s13045-015-0227-0
233. Savva A, Brouwer MC, Roger T, Valls Seron M, Le Roy D, Ferwerda B, et al. Functional polymorphisms of macrophage migration inhibitory factor as predictors of morbidity and mortality of pneumococcal meningitis. Proc Natl Acad Sci U S A (2016) 113(13):3597–602. doi:10.1073/pnas.1520727113
234. Fauvel B, Yasri A. Antibodies directed against receptor tyrosine kinases: current and future strategies to fight cancer. MAbs (2014) 6(4):838–51. doi:10.4161/mabs.29089
235. Chu FM, Picus J, Fracasso PM, Dreicer R, Lang Z, Foster B. A phase 1, multicenter, open-label study of the safety of two dose levels of a human monoclonal antibody to human alpha(v) integrins, intetumumab, in combination with docetaxel and prednisone in patients with castrate-resistant metastatic prostate cancer. Invest New Drugs (2011) 29(4):674–9. doi:10.1007/s10637-010-9388-4
236. Kohrt HE, Thielens A, Marabelle A, Sagiv-Barfi I, Sola C, Chanuc F, et al. Anti-KIR antibody enhancement of anti-lymphoma activity of natural killer cells as monotherapy and in combination with anti-CD20 antibodies. Blood (2014) 123(5):678–86. doi:10.1182/blood-2013-08-519199
237. Richman CM, Denardo SJ, O’Donnell RT, Yuan A, Shen S, Goldstein DS, et al. High-dose radioimmunotherapy combined with fixed, low-dose paclitaxel in metastatic prostate and breast cancer by using a MUC-1 monoclonal antibody, m170, linked to indium-111/yttrium-90 via a cathepsin cleavable linker with cyclosporine to prevent human anti-mouse antibody. Clin Cancer Res (2005) 11(16):5920–7. doi:10.1158/1078-0432.CCR-05-0211
238. Leow CC, Coffman K, Inigo I, Breen S, Czapiga M, Soukharev S, et al. MEDI3617, a human anti-angiopoietin 2 monoclonal antibody, inhibits angiogenesis and tumor growth in human tumor xenograft models. Int J Oncol (2012) 40(5):1321–30. doi:10.3892/ijo.2012.1366
239. Alinari L, Yu B, Christian BA, Yan F, Shin J, Lapalombella R, et al. Combination anti-CD74 (milatuzumab) and anti-CD20 (rituximab) monoclonal antibody therapy has in vitro and in vivo activity in mantle cell lymphoma. Blood (2011) 117(17):4530–41. doi:10.1182/blood-2010-08-303354
240. Ponte JF, Ab O, Lanieri L, Lee J, Coccia J, Bartle LM, et al. Mirvetuximab soravtansine (IMGN853), a folate receptor alpha-targeting antibody-drug conjugate, potentiates the activity of standard of care therapeutics in ovarian cancer models. Neoplasia (2016) 18(12):775–84. doi:10.1016/j.neo.2016.11.002
241. McDonagh CF, Huhalov A, Harms BD, Adams S, Paragas V, Oyama S, et al. Antitumor activity of a novel bispecific antibody that targets the ErbB2/ErbB3 oncogenic unit and inhibits heregulin-induced activation of ErbB3. Mol Cancer Ther (2012) 11(3):582–93. doi:10.1158/1535-7163.MCT-11-0820
242. Patnaik A, LoRusso PM, Messersmith WA, Papadopoulos KP, Gore L, Beeram M, et al. A Phase Ib study evaluating MNRP1685A, a fully human anti-NRP1 monoclonal antibody, in combination with bevacizumab and paclitaxel in patients with advanced solid tumors. Cancer Chemother Pharmacol (2014) 73(5):951–60. doi:10.1007/s00280-014-2426-8
243. Aspeslagh S, Postel-Vinay S, Rusakiewicz S, Soria JC, Zitvogel L, Marabelle A. Rationale for anti-OX40 cancer immunotherapy. Eur J Cancer (2016) 52:50–66. doi:10.1016/j.ejca.2015.08.021
244. Lee D, Kim D, Choi YB, Kang K, Sung ES, Ahn JH, et al. Simultaneous blockade of VEGF and DLL4 by HD105, a bispecific antibody, inhibits tumor progression and angiogenesis. MAbs (2016) 8(5):892–904. doi:10.1080/19420862.2016.1171432
245. Takeda M, Okamoto I, Nishimura Y, Nakagawa K. Nimotuzumab, a novel monoclonal antibody to the epidermal growth factor receptor, in the treatment of non-small cell lung cancer. Lung Cancer (Auckl) (2011) 2:59–67. doi:10.2147/LCTT.S16440
246. Cerquozzi S, Owen C. Clinical role of obinutuzumab in the treatment of naive patients with chronic lymphocytic leukemia. Biologics (2015) 9:13–22. doi:10.2147/BTT.S61600
247. Garcia-Carbonero R, van Cutsem E, Rivera F, Jassem J, Gore I Jr, Tebbutt N, et al. Randomized phase II trial of parsatuzumab (anti-EGFL7) or placebo in combination with FOLFOX and bevacizumab for first-line metastatic colorectal cancer. Oncologist (2017) 22(4):375–e30. doi:10.1634/theoncologist.2016-0133
248. Masek-Hammerman K, Peeva E, Ahmad A, Menon S, Afsharvand M, Peng Qu R, et al. Monoclonal antibody against macrophage colony-stimulating factor suppresses circulating monocytes and tissue macrophage function but does not alter cell infiltration/activation in cutaneous lesions or clinical outcomes in patients with cutaneous lupus erythematosus. Clin Exp Immunol (2016) 183(2):258–70. doi:10.1111/cei.12705
249. Diab A, El-Khoueiry A, Eskens FA, Ros W, Thompson JA, Konto C, et al. A first-in-human (FIH) study of PF-04518600 (PF-8600) OX40 agonist in adult patients (pts) with select advanced malignancies. Ann Oncol (2016) 27(Suppl_6):1053D. doi:10.1093/annonc/mdw378.08
250. Lu J, Lee-Gabel L, Nadeau MC, Ferencz TM, Soefje SA. Clinical evaluation of compounds targeting PD-1/PD-L1 pathway for cancer immunotherapy. J Oncol Pharm Pract (2015) 21(6):451–67. doi:10.1177/1078155214538087
251. Sundar R, Cho BC, Brahmer JR, Soo RA. Nivolumab in NSCLC: latest evidence and clinical potential. Ther Adv Med Oncol (2015) 7(2):85–96. doi:10.1177/1758834014567470
252. Giordano S. Rilotumumab, a mAb against human hepatocyte growth factor for the treatment of cancer. Curr Opin Mol Ther (2009) 11(4):448–55.
253. Bacac M, Klein C, Umana P. CEA TCB: a novel head-to-tail 2:1 T cell bispecific antibody for treatment of CEA-positive solid tumors. Oncoimmunology (2016) 5(8):e1203498. doi:10.1080/2162402X.2016.1203498
254. Bacac M, Fauti T, Sam J, Colombetti S, Weinzierl T, Ouaret D, et al. A novel carcinoembryonic antigen T-cell bispecific antibody (CEA TCB) for the treatment of solid tumors. Clin Cancer Res (2016) 22(13):3286–97. doi:10.1158/1078-0432.CCR-15-1696
255. Ellmark P, Mangsbo SM, Furebring C, Totterman TH, Norlen P. Kick-starting the cancer-immunity cycle by targeting CD40. Oncoimmunology (2015) 4(7):e1011484. doi:10.1080/2162402X.2015.1011484
256. Rudin CM, Pietanza MC, Bauer TM, Ready N, Morgensztern D, Glisson BS, et al. Rovalpituzumab tesirine, a DLL3-targeted antibody-drug conjugate, in recurrent small-cell lung cancer: a first-in-human, first-in-class, open-label, phase 1 study. Lancet Oncol (2017) 18(1):42–51. doi:10.1016/S1470-2045(16)30565-4
257. Sussman D, Smith LM, Anderson ME, Duniho S, Hunter JH, Kostner H, et al. SGN-LIV1A: a novel antibody-drug conjugate targeting LIV-1 for the treatment of metastatic breast cancer. Mol Cancer Ther (2014) 13(12):2991–3000. doi:10.1158/1535-7163.MCT-13-0896
258. Hassan R, Lerner MR, Benbrook D, Lightfoot SA, Brackett DJ, Wang QC, et al. Antitumor activity of SS(dsFv)PE38 and SS1(dsFv)PE38, recombinant antimesothelin immunotoxins against human gynecologic cancers grown in organotypic culture in vitro. Clin Cancer Res (2002) 8(11):3520–6.
259. Forero-Torres A, Varley KE, Abramson VG, Li Y, Vaklavas C, Lin NU, et al. TBCRC 019: a phase II trial of nanoparticle albumin-bound paclitaxel with or without the anti-death receptor 5 monoclonal antibody tigatuzumab in patients with triple-negative breast cancer. Clin Cancer Res (2015) 21(12):2722–9. doi:10.1158/1078-0432.CCR-14-2780
260. Forero-Torres A, Shah J, Wood T, Posey J, Carlisle R, Copigneaux C, et al. Phase I trial of weekly tigatuzumab, an agonistic humanized monoclonal antibody targeting death receptor 5 (DR5). Cancer Biother Radiopharm (2010) 25(1):13–9. doi:10.1089/cbr.2009.0673
261. Sanmamed MF, Rodriguez I, Schalper KA, Onate C, Azpilikueta A, Rodriguez-Ruiz ME, et al. Nivolumab and urelumab enhance antitumor activity of human T lymphocytes engrafted in Rag2-/-IL2Rgammanull immunodeficient mice. Cancer Res (2015) 75(17):3466–78. doi:10.1158/0008-5472.CAN-14-3510
262. Tolcher AW, Sznol M, Hu-Lieskovan S, Papadopoulos KP, Patnaik A, Rasco DW, et al. Phase Ib study of utomilumab (PF-05082566), a 4-1BB/CD137 agonist, in combination with pembrolizumab (MK-3475) in patients with advanced solid tumors. Clin Cancer Res (2017) 23(18):5349–57. doi:10.1158/1078-0432.CCR-17-1243
263. Kienast Y, Klein C, Scheuer W, Raemsch R, Lorenzon E, Bernicke D, et al. Ang-2-VEGF-A CrossMab, a novel bispecific human IgG1 antibody blocking VEGF-A and Ang-2 functions simultaneously, mediates potent antitumor, antiangiogenic, and antimetastatic efficacy. Clin Cancer Res (2013) 19(24):6730–40. doi:10.1158/1078-0432.CCR-13-0081
264. Goldenberg DM, Morschhauser F, Wegener WA. Veltuzumab (humanized anti-CD20 monoclonal antibody): characterization, current clinical results, and future prospects. Leuk Lymphoma (2010) 51(5):747–55. doi:10.3109/10428191003672123
265. Tampellini M, Sonetto C, Scagliotti GV. Novel anti-angiogenic therapeutic strategies in colorectal cancer. Expert Opin Investig Drugs (2016) 25(5):507–20. doi:10.1517/13543784.2016.1161754
266. Mora J. Dinutuximab for the treatment of pediatric patients with high-risk neuroblastoma. Expert Rev Clin Pharmacol (2016) 9(5):647–53. doi:10.1586/17512433.2016.1160775
267. Dobrenkov K, Cheung NK. GD2-targeted immunotherapy and radioimmunotherapy. Semin Oncol (2014) 41(5):589–612. doi:10.1053/j.seminoncol.2014.07.003
268. Suzuki M, Cheung NK. Disialoganglioside GD2 as a therapeutic target for human diseases. Expert Opin Ther Targets (2015) 19(3):349–62. doi:10.1517/14728222.2014.986459
269. Perez Horta Z, Goldberg JL, Sondel PM. Anti-GD2 mAbs and next-generation mAb-based agents for cancer therapy. Immunotherapy (2016) 8(9):1097–117. doi:10.2217/imt-2016-0021
270. Parsons K, Bernhardt B, Strickland B. Targeted immunotherapy for high-risk neuroblastoma – the role of monoclonal antibodies. Ann Pharmacother (2013) 47(2):210–8. doi:10.1345/aph.1R353
271. Cheung NK, Cheung IY, Kushner BH, Ostrovnaya I, Chamberlain E, Kramer K, et al. Murine anti-GD2 monoclonal antibody 3F8 combined with granulocyte-macrophage colony-stimulating factor and 13-cis-retinoic acid in high-risk patients with stage 4 neuroblastoma in first remission. J Clin Oncol (2012) 30(26):3264–70. doi:10.1200/JCO.2011.41.3807
272. Dhillon S. Dinutuximab: first global approval. Drugs (2015) 75(8):923–7. doi:10.1007/s40265-015-0399-5
273. Ploessl C, Pan A, Maples KT, Lowe DK. Dinutuximab: an anti-GD2 monoclonal antibody for high-risk neuroblastoma. Ann Pharmacother (2016) 50(5):416–22. doi:10.1177/1060028016632013
274. Mehmood RK. Review of cisplatin and oxaliplatin in current immunogenic and monoclonal antibody treatments. Oncol Rev (2014) 8(2):256. doi:10.4081/oncol.2014.256
275. Sridharan V, Schoenfeld JD. Immune effects of targeted radiation therapy for cancer. Discov Med (2015) 19(104):219–28.
276. Vatner RE, Cooper BT, Vanpouille-Box C, Demaria S, Formenti SC. Combinations of immunotherapy and radiation in cancer therapy. Front Oncol (2014) 4:325. doi:10.3389/fonc.2014.00325
277. Hu ZI, McArthur HL, Ho AY. The abscopal effect of radiation therapy: what is it and how can we use it in breast cancer? Curr Breast Cancer Rep (2017) 9(1):45–51. doi:10.1007/s12609-017-0234-y
278. Kaur P, Asea A. Radiation-induced effects and the immune system in cancer. Front Oncol (2012) 2:191. doi:10.3389/fonc.2012.00191
279. Formenti SC, Demaria S. Systemic effects of local radiotherapy. Lancet Oncol (2009) 10(7):718–26. doi:10.1016/S1470-2045(09)70082-8
280. Baker SJ, Reddy EP. Targeted inhibition of kinases in cancer therapy. Mt Sinai J Med (2010) 77(6):573–86. doi:10.1002/msj.20220
281. Prieto PA, Reuben A, Cooper ZA, Wargo JA. Targeted therapies combined with immune checkpoint therapy. Cancer J (2016) 22(2):138–46. doi:10.1097/PPO.0000000000000182
282. Gross S, Rahal R, Stransky N, Lengauer C, Hoeflich KP. Targeting cancer with kinase inhibitors. J Clin Invest (2015) 125(5):1780–9. doi:10.1172/JCI76094
283. Robak T, Blonski JZ, Robak P. Antibody therapy alone and in combination with targeted drugs in chronic lymphocytic leukemia. Semin Oncol (2016) 43(2):280–90. doi:10.1053/j.seminoncol.2016.02.010
284. Parakh S, Gan HK, Parslow AC, Burvenich IJG, Burgess AW, Scott AM. Evolution of anti-HER2 therapies for cancer treatment. Cancer Treat Rev (2017) 59:1–21. doi:10.1016/j.ctrv.2017.06.005
285. Saygin C, Carraway HE. Emerging therapies for acute myeloid leukemia. J Hematol Oncol (2017) 10(1):93. doi:10.1186/s13045-017-0463-6
286. Konecny GE. Emerging strategies for the dual inhibition of HER2-positive breast cancer. Curr Opin Obstet Gynecol (2013) 25(1):55–65. doi:10.1097/GCO.0b013e32835c5e90
287. Jones DT, Trowbridge IS, Harris AL. Effects of transferrin receptor blockade on cancer cell proliferation and hypoxia-inducible factor function and their differential regulation by ascorbate. Cancer Res (2006) 66(5):2749–56. doi:10.1158/0008-5472.CAN-05-3857
288. Stein R, Qu Z, Chen S, Rosario A, Shi V, Hayes M, et al. Characterization of a new humanized anti-CD20 monoclonal antibody, IMMU-106, and Its use in combination with the humanized anti-CD22 antibody, epratuzumab, for the therapy of non-Hodgkin’s lymphoma. Clin Cancer Res (2004) 10(8):2868–78. doi:10.1158/1078-0432.CCR-03-0493
289. Gordon-Weeks AN, Lim SY, Yuzhalin AE, Jones K, Muschel R. Macrophage migration inhibitory factor: a key cytokine and therapeutic target in colon cancer. Cytokine Growth Factor Rev (2015) 26(4):451–61. doi:10.1016/j.cytogfr.2015.03.002
290. Bifulco C, McDaniel K, Leng L, Bucala R. Tumor growth-promoting properties of macrophage migration inhibitory factor. Curr Pharm Des (2008) 14(36):3790–801. doi:10.2174/138161208786898608
291. Nishihira J, Ishibashi T, Fukushima T, Sun B, Sato Y, Todo S. Macrophage migration inhibitory factor (MIF): its potential role in tumor growth and tumor-associated angiogenesis. Ann N Y Acad Sci (2003) 995:171–82. doi:10.1111/j.1749-6632.2003.tb03220.x
292. Stein R, Mattes MJ, Cardillo TM, Hansen HJ, Chang CH, Burton J, et al. CD74: a new candidate target for the immunotherapy of B-cell neoplasms. Clin Cancer Res (2007) 13(18 Pt 2):5556s–63s. doi:10.1158/1078-0432.CCR-07-1167
293. Berkova Z, Tao RH, Samaniego F. Milatuzumab – a promising new immunotherapeutic agent. Expert Opin Investig Drugs (2010) 19(1):141–9. doi:10.1517/13543780903463854
294. Weiskopf K. Cancer immunotherapy targeting the CD47/SIRPalpha axis. Eur J Cancer (2017) 76:100–9. doi:10.1016/j.ejca.2017.02.013
295. Huang Y, Ma Y, Gao P, Yao Z. Targeting CD47: the achievements and concerns of current studies on cancer immunotherapy. J Thorac Dis (2017) 9(2):E168–74. doi:10.21037/jtd.2017.02.30
296. Barclay AN, Van den Berg TK. The interaction between signal regulatory protein alpha (SIRPalpha) and CD47: structure, function, and therapeutic target. Annu Rev Immunol (2014) 32:25–50. doi:10.1146/annurev-immunol-032713-120142
297. Matlung HL, Szilagyi K, Barclay NA, van den Berg TK. The CD47-SIRPalpha signaling axis as an innate immune checkpoint in cancer. Immunol Rev (2017) 276(1):145–64. doi:10.1111/imr.12527
298. Weiskopf K, Weissman IL. Macrophages are critical effectors of antibody therapies for cancer. MAbs (2015) 7(2):303–10. doi:10.1080/19420862.2015.1011450
299. Kamath AV, Iyer S. Challenges and advances in the assessment of the disposition of antibody-drug conjugates. Biopharm Drug Dispos (2016) 37(2):66–74. doi:10.1002/bdd.1957
300. Kamath AV, Iyer S. Preclinical pharmacokinetic considerations for the development of antibody drug conjugates. Pharm Res (2015) 32(11):3470–9. doi:10.1007/s11095-014-1584-z
301. Bouchard H, Viskov C, Garcia-Echeverria C. Antibody-drug conjugates-a new wave of cancer drugs. Bioorg Med Chem Lett (2014) 24(23):5357–63. doi:10.1016/j.bmcl.2014.10.021
302. de Goeij BE, Lambert JM. New developments for antibody-drug conjugate-based therapeutic approaches. Curr Opin Immunol (2016) 40:14–23. doi:10.1016/j.coi.2016.02.008
303. Polakis P. Antibody drug conjugates for cancer therapy. Pharmacol Rev (2016) 68(1):3–19. doi:10.1124/pr.114.009373
304. Rossin R, van Duijnhoven SM, Ten Hoeve W, Janssen HM, Kleijn LH, Hoeben FJ, et al. Triggered drug release from an antibody-drug conjugate using fast “click-to-release” chemistry in mice. Bioconjug Chem (2016) 27(7):1697–706. doi:10.1021/acs.bioconjchem.6b00231
305. Rossi A. Rovalpituzumab tesirine and DLL3: a new challenge for small-cell lung cancer. Lancet Oncol (2017) 18(1):3–5. doi:10.1016/S1470-2045(16)30575-7
306. Pollack VA, Alvarez E, Tse KF, Torgov MY, Xie S, Shenoy SG, et al. Treatment parameters modulating regression of human melanoma xenografts by an antibody-drug conjugate (CR011-vcMMAE) targeting GPNMB. Cancer Chemother Pharmacol (2007) 60(3):423–35. doi:10.1007/s00280-007-0490-z
307. Ramakrishna V, Sundarapandiyan K, Zhao B, Bylesjo M, Marsh HC, Keler T. Characterization of the human T cell response to in vitro CD27 costimulation with varlilumab. J Immunother Cancer (2015) 3:37. doi:10.1186/s40425-015-0080-2
308. Burris HA, Infante JR, Ansell SM, Nemunaitis JJ, Weiss GR, Villalobos VM, et al. Safety and activity of varlilumab, a novel and first-in-class agonist anti-CD27 antibody, in patients with advanced solid tumors. J Clin Oncol (2017) 35(18):2028–36. doi:10.1200/JCO.2016.70.1508
309. Ab O, Whiteman KR, Bartle LM, Sun X, Singh R, Tavares D, et al. IMGN853, a folate receptor-alpha (FRalpha)-targeting antibody-drug conjugate, exhibits potent targeted antitumor activity against fralpha-expressing tumors. Mol Cancer Ther (2015) 14(7):1605–13. doi:10.1158/1535-7163.MCT-14-1095
310. Hassan R, Thomas A, Alewine C, Le DT, Jaffee EM, Pastan I. Mesothelin immunotherapy for cancer: ready for prime time? J Clin Oncol (2016) 34(34):4171–9. doi:10.1200/JCO.2016.68.3672
311. List T, Neri D. Immunocytokines: a review of molecules in clinical development for cancer therapy. Clin Pharmacol (2013) 5:29–45. doi:10.2147/CPAA.S49231
312. Neri D, Sondel PM. Immunocytokines for cancer treatment: past, present and future. Curr Opin Immunol (2016) 40:96–102. doi:10.1016/j.coi.2016.03.006
313. Pasche N, Neri D. Immunocytokines: a novel class of potent armed antibodies. Drug Discov Today (2012) 17(11–12):583–90. doi:10.1016/j.drudis.2012.01.007
314. Charych DH, Hoch U, Langowski JL, Lee SR, Addepalli MK, Kirk PB, et al. NKTR-214, an engineered cytokine with biased IL2 receptor binding, increased tumor exposure, and marked efficacy in mouse tumor models. Clin Cancer Res (2016) 22(3):680–90. doi:10.1158/1078-0432.CCR-15-1631
315. Wong HC, Jeng EK, Rhode PR. The IL-15-based superagonist ALT-803 promotes the antigen-independent conversion of memory CD8+ T cells into innate-like effector cells with antitumor activity. Oncoimmunology (2013) 2(11):e26442. doi:10.4161/onci.26442
316. Lasek W, Zagozdzon R, Jakobisiak M. Interleukin 12: still a promising candidate for tumor immunotherapy? Cancer Immunol Immunother (2014) 63(5):419–35. doi:10.1007/s00262-014-1523-1
317. Danielli R, Patuzzo R, Di Giacomo AM, Gallino G, Maurichi A, Di Florio A, et al. Intralesional administration of L19-IL2/L19-TNF in stage III or stage IVM1a melanoma patients: results of a phase II study. Cancer Immunol Immunother (2015) 64(8):999–1009. doi:10.1007/s00262-015-1704-6
318. Danielli R, Patuzzo R, Ruffini PA, Maurichi A, Giovannoni L, Elia G, et al. Armed antibodies for cancer treatment: a promising tool in a changing era. Cancer Immunol Immunother (2015) 64(1):113–21. doi:10.1007/s00262-014-1621-0
319. Spitaleri G, Berardi R, Pierantoni C, De Pas T, Noberasco C, Libbra C, et al. Phase I/II study of the tumour-targeting human monoclonal antibody-cytokine fusion protein L19-TNF in patients with advanced solid tumours. J Cancer Res Clin Oncol (2013) 139(3):447–55. doi:10.1007/s00432-012-1327-7
320. Schwager K, Hemmerle T, Aebischer D, Neri D. The immunocytokine L19-IL2 eradicates cancer when used in combination with CTLA-4 blockade or with L19-TNF. J Invest Dermatol (2013) 133(3):751–8. doi:10.1038/jid.2012.376
321. Asmana Ningrum R. Human interferon alpha-2b: a therapeutic protein for cancer treatment. Scientifica (Cairo) (2014) 2014:970315. doi:10.1155/2014/970315
322. Zhao Y, Adjei AA. Targeting angiogenesis in cancer therapy: moving beyond vascular endothelial growth factor. Oncologist (2015) 20(6):660–73. doi:10.1634/theoncologist.2014-0465
323. Bumbaca D, Xiang H, Boswell CA, Port RE, Stainton SL, Mundo EE, et al. Maximizing tumour exposure to anti-neuropilin-1 antibody requires saturation of non-tumour tissue antigenic sinks in mice. Br J Pharmacol (2012) 166(1):368–77. doi:10.1111/j.1476-5381.2011.01777.x
324. Gozdzik-Spychalska J, Szyszka-Barth K, Spychalski L, Ramlau K, Wojtowicz J, Batura-Gabryel H, et al. C-MET inhibitors in the treatment of lung cancer. Curr Treat Options Oncol (2014) 15(4):670–82. doi:10.1007/s11864-014-0313-5
325. Hellstrom M, Phng LK, Hofmann JJ, Wallgard E, Coultas L, Lindblom P, et al. DLL4 signalling through Notch1 regulates formation of tip cells during angiogenesis. Nature (2007) 445(7129):776–80. doi:10.1038/nature05571
326. Chen Q, Manning CD, Millar H, McCabe FL, Ferrante C, Sharp C, et al. CNTO 95, a fully human anti alphav integrin antibody, inhibits cell signaling, migration, invasion, and spontaneous metastasis of human breast cancer cells. Clin Exp Metastasis (2008) 25(2):139–48. doi:10.1007/s10585-007-9132-4
327. Kloepper J, Riedemann L, Amoozgar Z, Seano G, Susek K, Yu V, et al. Ang-2/VEGF bispecific antibody reprograms macrophages and resident microglia to anti-tumor phenotype and prolongs glioblastoma survival. Proc Natl Acad Sci U S A (2016) 113(16):4476–81. doi:10.1073/pnas.1525360113
328. Van Cutsem E, Eng C, Nowara E, Swieboda-Sadlej A, Tebbutt NC, Mitchell E, et al. Randomized phase Ib/II trial of rilotumumab or ganitumab with panitumumab versus panitumumab alone in patients with wild-type KRAS metastatic colorectal cancer. Clin Cancer Res (2014) 20(16):4240–50. doi:10.1158/1078-0432.CCR-13-2752
329. Zhukovsky EA, Morse RJ, Maus MV. Bispecific antibodies and CARs: generalized immunotherapeutics harnessing T cell redirection. Curr Opin Immunol (2016) 40:24–35. doi:10.1016/j.coi.2016.02.006
330. Gan C, Mostafid H, Khan MS, Lewis DJ. BCG immunotherapy for bladder cancer – the effects of substrain differences. Nat Rev Urol (2013) 10(10):580–8. doi:10.1038/nrurol.2013.194
331. Salucci V, Lena AM, Ciliberto G, Scarselli E, La Monica N. Adenovirus transduction and culture conditions affect the immunogenicity of murine dendritic cells. Scand J Immunol (2005) 62(3):206–17. doi:10.1111/j.1365-3083.2005.01658.x
332. Gulley JL, Arlen PM, Tsang KY, Yokokawa J, Palena C, Poole DJ, et al. Pilot study of vaccination with recombinant CEA-MUC-1-TRICOM poxviral-based vaccines in patients with metastatic carcinoma. Clin Cancer Res (2008) 14(10):3060–9. doi:10.1158/1078-0432.CCR-08-0126
333. Dessureault S, Noyes D, Lee D, Dunn M, Janssen W, Cantor A, et al. A phase-I trial using a universal GM-CSF-producing and CD40L-expressing bystander cell line (GM.CD40L) in the formulation of autologous tumor cell-based vaccines for cancer patients with stage IV disease. Ann Surg Oncol (2007) 14(2):869–84. doi:10.1245/s10434-006-9196-4
335. Mehner C, Hockla A, Miller E, Ran S, Radisky DC, Radisky ES. Tumor cell-produced matrix metalloproteinase 9 (MMP-9) drives malignant progression and metastasis of basal-like triple negative breast cancer. Oncotarget (2014) 5(9):2736–49. doi:10.18632/oncotarget.1932
336. Bradley S, Jakes AD, Harrington K, Pandha H, Melcher A, Errington-Mais F. Applications of coxsackievirus A21 in oncology. Oncolytic Virother (2014) 3:47–55. doi:10.2147/OV.S56322
337. Skelding KA, Barry RD, Shafren DR. Systemic targeting of metastatic human breast tumor xenografts by coxsackievirus A21. Breast Cancer Res Treat (2009) 113(1):21–30. doi:10.1007/s10549-008-9899-2
338. Kroschinsky F, Stolzel F, von Bonin S, Beutel G, Kochanek M, Kiehl M, et al. New drugs, new toxicities: severe side effects of modern targeted and immunotherapy of cancer and their management. Crit Care (2017) 21(1):89. doi:10.1186/s13054-017-1678-1
339. Ott PA, Hodi FS, Kaufman HL, Wigginton JM, Wolchok JD. Combination immunotherapy: a road map. J Immunother Cancer (2017) 5:16. doi:10.1186/s40425-017-0218-5
340. Wolchok JD, Kluger H, Callahan MK, Postow MA, Rizvi NA, Lesokhin AM, et al. Nivolumab plus ipilimumab in advanced melanoma. N Engl J Med (2013) 369(2):122–33. doi:10.1056/NEJMoa1302369
341. Boutros C, Tarhini A, Routier E, Lambotte O, Ladurie FL, Carbonnel F, et al. Safety profiles of anti-CTLA-4 and anti-PD-1 antibodies alone and in combination. Nat Rev Clin Oncol (2016) 13(8):473–86. doi:10.1038/nrclinonc.2016.58
342. Helissey C, Vicier C, Champiat S. The development of immunotherapy in older adults: new treatments, new toxicities? J Geriatr Oncol (2016) 7(5):325–33. doi:10.1016/j.jgo.2016.05.007
343. Hassel JC, Heinzerling L, Aberle J, Bahr O, Eigentler TK, Grimm MO, et al. Combined immune checkpoint blockade (anti-PD-1/anti-CTLA-4): evaluation and management of adverse drug reactions. Cancer Treat Rev (2017) 57:36–49. doi:10.1016/j.ctrv.2017.05.003
344. Spain L, Larkin J. Combination immune checkpoint blockade with ipilimumab and nivolumab in the management of advanced melanoma. Expert Opin Biol Ther (2016) 16(3):389–96. doi:10.1517/14712598.2016.1141195
345. Gordon MS, Robert F, Matei D, Mendelson DS, Goldman JW, Chiorean EG, et al. An open-label phase Ib dose-escalation study of TRC105 (anti-endoglin antibody) with bevacizumab in patients with advanced cancer. Clin Cancer Res (2014) 20(23):5918–26. doi:10.1158/1078-0432.CCR-14-1143
346. Dorff TB, Longmate JA, Pal SK, Stadler WM, Fishman MN, Vaishampayan UN, et al. Bevacizumab alone or in combination with TRC105 for patients with refractory metastatic renal cell cancer. Cancer (2017) 123(23):4566–73. doi:10.1002/cncr.30942
347. Althammer S, Steele K, Rebelatto M, Tan TH, Wiestler T, Schmidt G, et al. 31st annual meeting and associated programs of the society for immunotherapy of cancer (SITC 2016): late breaking abstracts: National Harbor, MD, USA. 9-13 November 2016. J Immunother Cancer (2016) 4(Suppl 2):91. doi:10.1186/s40425-016-0191-4
348. Ager C, Reilley M, Nicholas C, Bartkowiak T, Jaiswal A, Curran M, et al. 31st annual meeting and associated programs of the society for immunotherapy of cancer (SITC 2016): part two. J Immunother Cancer (2016) 4(1):73. doi:10.1186/s40425-016-0173-6
349. Gopal AK, Tarantolo SR, Bellam N, Green DJ, Griffin M, Feldman T, et al. Phase 1b study of otlertuzumab (TRU-016), an anti-CD37 monospecific ADAPTIR therapeutic protein, in combination with rituximab and bendamustine in relapsed indolent lymphoma patients. Invest New Drugs (2014) 32(6):1213–25. doi:10.1007/s10637-014-0125-2
350. Westin JR, Chu F, Zhang M, Fayad LE, Kwak LW, Fowler N, et al. Safety and activity of PD1 blockade by pidilizumab in combination with rituximab in patients with relapsed follicular lymphoma: a single group, open-label, phase 2 trial. Lancet Oncol (2014) 15(1):69–77. doi:10.1016/S1470-2045(13)70551-5
351. Lundqvist A, van Hoef V, Zhang X, Wennerberg E, Lorent J, Witt K, et al. 31st annual meeting and associated programs of the society for immunotherapy of cancer (SITC 2016): part one. J Immunother Cancer (2016) 4(Suppl 1):1–106. doi:10.1186/s40425-016-0172-7
352. Eissa IR, Naoe Y, Bustos-Villalobos I, Ichinose T, Tanaka M, Zhiwen W, et al. Genomic signature of the natural oncolytic herpes simplex virus HF10 and its therapeutic role in preclinical and clinical trials. Front Oncol (2017) 7:149. doi:10.3389/fonc.2017.00149
353. Infante JR, Hansen AR, Pishvaian MJ, Man Chow LQ, McArthur GA, Bauer TM. A phase lb dose escalation study of the OX40 agonist MOXR0916 and the PD-L1 inhibitor atezolizumab in patients with advanced solid tumors. J Clin Oncol (2016) 34(15_Suppl):abstr101. doi:10.1200/JCO.2016.34.15_suppl.101
354. Fury MG, Lipton A, Smith KM, Winston CB, Pfister DG. A phase-I trial of the epidermal growth factor receptor directed bispecific antibody MDX-447 without and with recombinant human granulocyte-colony stimulating factor in patients with advanced solid tumors. Cancer Immunol Immunother (2008) 57(2):155–63. doi:10.1007/s00262-007-0357-5
355. Mahalingam D, Patel M, Sachdev J, Hart L, Halama N, Ramanathan R, et al. Safety and efficacy analysis of imalumab, an anti-oxidized macrophage migration inhibitory factor (oxMIF) antibody, alone or in combination with 5-fluorouracil/leucovorin (5-FU/LV) or panitumumab, in patients with metastatic colorectal cancer (mCRC). Ann Oncol (2016) 27(Suppl_2):ii105. doi:10.1093/annonc/mdw200.11
356. Das R, Verma R, Sznol M, Boddupalli CS, Gettinger SN, Kluger H, et al. Combination therapy with anti-CTLA-4 and anti-PD-1 leads to distinct immunologic changes in vivo. J Immunol (2015) 194(3):950–9. doi:10.4049/jimmunol.1401686
Appendix
Key Concepts
Antibody-dependent cell cytotoxicity (ADCC): the binding of an antibody to a cell surface antigen promotes the interaction of the natural killer cell with the Fc antibody fragment and triggers its cytotoxic response.
Adaptive immune response: its main function is to eliminate pathogens and fight cancer. Specifically recognizes antigen though receptors on the surface of T and B lymphocytes. It creates immunological memory, after the initial response to a given antigen, leads to an exacerbated response to subsequent encounters with the same antigen. This is the basis of vaccination.
Antibody-dependent phagocytosis (ADCP): the binding of an antibody to a cell surface antigen allows the opsonization of the cell and promotes its phagocytosis.
Combined therapy: therapy that combines different therapeutic approaches (i.e., chemotherapy, radiotherapy, small molecule drugs, vaccines, antibodies, etc.).
Complement-dependent cytotoxicity (CDC): the binding of an antibody to a cell surface antigen activates the complement cascade, resulting in the cell’s death.
Immune checkpoint: there are checkpoints that receive negative signals from the tumor, inhibiting the antitumor immune response. These include the PD-1/PD-L1 axis (PD-1 receptor on the T cells and to PD-L1 on the tumor cells) and CTLA-4 (on T cells). Antibodies against these molecules (nivolumab, pembrolizumab, atezolizumab, durvalumab, avelumab, and ipilimumab) are able to inhibit these negative signals and promote the antitumor immune response.
Immunotherapy: therapeutic treatment that takes advantage of the immune system response.
Innate immune response: provides immediate defense against infection, on a non-specific manner, is not long-lasting and recognizes pathogens through generic receptors.
Therapeutic antibody: an antibody that can be used for therapeutic purposes, which is able to either act as antagonist, guide a drug toward the tumor cell, where it will act, or kill the tumor cell either directly or by activating immune response mechanisms (ADCC, CDC, and ADCP).
Vaccine: compounds inoculated in an organism to evoke a primary immune response, generating immunological memory able to protect against the antigen.
Keywords: cancer, antibody combinations, oncology, therapeutic antibodies, immunotherapy
Citation: Corraliza-Gorjón I, Somovilla-Crespo B, Santamaria S, Garcia-Sanz JA and Kremer L (2017) New Strategies Using Antibody Combinations to Increase Cancer Treatment Effectiveness. Front. Immunol. 8:1804. doi: 10.3389/fimmu.2017.01804
Received: 11 September 2017; Accepted: 30 November 2017;
Published: 21 December 2017
Edited by:
Nurit Hollander, Tel Aviv University, IsraelReviewed by:
Daniel Olive, Institut National de la Santé et de la Recherche Médicale, FranceEstrella Mariel Levy, Consejo Nacional de Investigaciones Científicas y Técnicas (CONICET), Argentina
William L. Redmond, Earle A. Chiles Research Institute, United States
Copyright: © 2017 Corraliza-Gorjón, Somovilla-Crespo, Santamaria, Garcia-Sanz and Kremer. This is an open-access article distributed under the terms of the Creative Commons Attribution License (CC BY). The use, distribution or reproduction in other forums is permitted, provided the original author(s) or licensor are credited and that the original publication in this journal is cited, in accordance with accepted academic practice. No use, distribution or reproduction is permitted which does not comply with these terms.
*Correspondence: Jose A. Garcia-Sanz, amFzYW56QGNpYi5jc2ljLmVz;
Leonor Kremer, bGtyZW1lckBjbmIuY3NpYy5lcw==
†Both authors contributed equally to this work and should be regarded as senior co-authors.