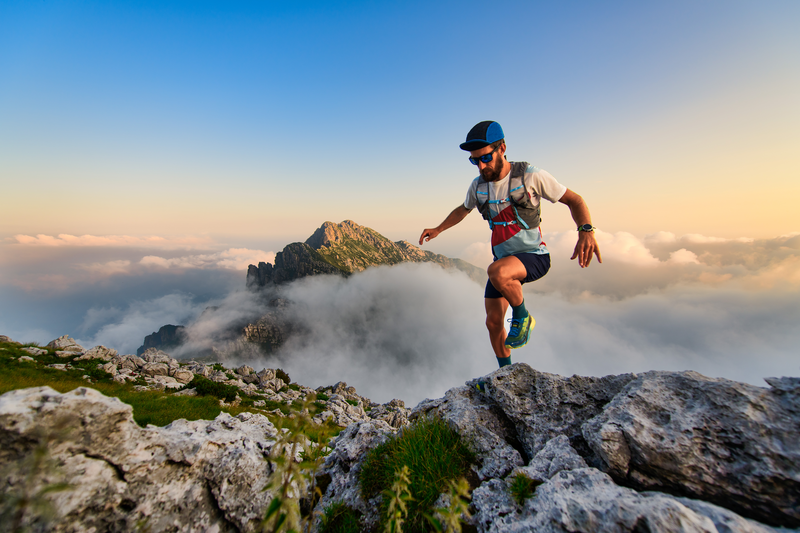
94% of researchers rate our articles as excellent or good
Learn more about the work of our research integrity team to safeguard the quality of each article we publish.
Find out more
MINI REVIEW article
Front. Immunol. , 03 November 2017
Sec. T Cell Biology
Volume 8 - 2017 | https://doi.org/10.3389/fimmu.2017.01467
This article is part of the Research Topic Membrane Lipids in T Cell Functions View all 8 articles
Spatiotemporal compartmentalization of signaling pathways and second messengers is pivotal for cell biology and membrane rafts are, therefore, required for several lymphocyte functions. On the other hand, T cells have the specific necessity of tuning signaling amplification depending on the context in which the antigen is presented. In this review, we discuss of membrane rafts in the context of T cell signaling, focusing on CD28-mediated costimulation.
In the late 1980 s, accumulating evidence set forth the existence of ordered lipid clusters in the cell membrane, named lipid rafts. According to the “lipid raft hypothesis,” forces between cholesterol and sphingolipids facilitate the dynamic assemblies of lipid domains in an unsaturated glycerophospholipid environment (1). Experimental evidence accumulated during the following years, indicated that in real cell membranes transmembrane, signaling, and cytoskeletal proteins play a key role in controlling raft generation and dynamics, and have suggested a new model based on protein–lipid interaction (2, 3). In 2006, at the end of a vibrant meeting, a consensus definition for membrane rafts was achieved and they were defined as “small (10–200 nm), heterogeneous, highly dynamic, sterol- and sphingolipid-enriched domains that compartmentalize cellular processes” (2, 4). Nonetheless, the size, density, molecular composition, and morphology of these domains in cellular membranes have remained controversial for a very long time.
As a matter of fact, for several years from their initial discovery, scientists were skeptical about the physiological existence of lipid platforms at the cell membrane, mainly because it was almost impossible at that time to probe their presence in living cells. A critical issue in this sense was the methodology employed to define a raft-integrated factor that was foremost based on detergent resistance; indeed, a hallmark of a raft component is the recovery in the low-density fraction after cold Triton extraction and sucrose density gradient centrifugation. However, this technique can produce multiple artifacts due to the type and concentration of the detergents, duration of extraction, and temperature (5, 6). Importantly, methyl-β-cyclodextrin (MβCD) that extracts cholesterol from cell membranes was largely exploited to discriminate whether a protein was a raft component and more to evaluate whether a biological process was raft dependent or independent. Even in this case, the use of this drug was matter of debate due to the multiple non-specific effects of MβCD in addition to cholesterol removal, as lateral protein immobilization, plasma membrane depolarization, and Ca2+ store depletion (7).
To overcome biochemical hurdles, alternative approaches with high temporal and spatial resolution have been optimized. Subczynski and Kusumi advanced and exploited pulse electron paramagnetic resonance spin labeling methods and single molecule optical imaging to track the dynamic entry and exit of molecules in membrane rafts (8). In addition, over the last decades a plethora of advanced imaging techniques have been applied to identify membrane rafts in live cells as single fluorophore tracking (9) and photonic force microscopy (10), fluorescence resonance energy transfer (11), fluorescence recovery after photobleaching (12), total internal reflection fluorescence (13), and stimulated emission depletion microscopy (14). For technically oriented readers, distinguished reviews in the field have illustrated advantages and limits of the different methodologies that have been exploited to identify and study lipid membrane microdomains (15).
Beyond conceptual and technical criticisms, it was clear that one of the most relevant features of membrane rafts is their intrinsic capacity to selectively include or exclude specific proteins. The partitioning of a single molecule in such liquid-ordered domains depends on different factors, as cell type and activation state, and more importantly it can be regulated by intra- and extracellular signals (8). Given their small size, the number of proteins that can localize in a single raft domain is limited, probably no more than 10–30 proteins. Controversial theories have been proposed on raft composition in terms of proteins and lipids and several studies have documented the coexistence of different membrane rafts with distinctly different proteins and/or lipids within the same cell type (16). Indeed, a simplified but still operating view of membrane rafts is that they can act as highly dynamic platforms at the plasma membrane, where effector enzymes, cofactors, adaptors, and scaffold proteins can trigger and organize multiple signaling cascades. In this sense, the existence of plasma membrane microdomains not only changes the structural organization of the Singer and Nicolson fluid mosaic model but, importantly, it also influences our knowledge on the mechanisms that connect receptor stimulation with the activation of an intracellular signaling process. Indeed, raft domains may control signal transduction in multiple ways. For example, receptor stimulation can rapidly and efficiently activate a signaling cascade thanks to the spatial juxtaposition of different interacting molecules within the same membrane domain. In addition, as for of tyrosine kinases, rafts can offer a protective micro-environment that isolates the activated signaling machinery from non-raft enzymes such as membrane phosphatases that could inhibit the process. By internalizing selective molecules, membrane rafts also play a role in signal termination (17).
Although originally thought as lipid-based free-floating platforms—“rafts,” indeed—it became clear that membrane rafts are tightly connected with the cell cytoskeleton through actin-binding proteins such as ezrin and filamin (3) and, therefore, more similar to “flying kites” than “floating rafts” (2). The cross-talk between membrane rafts and the actin cytoskeleton is very complex: membrane rafts are indeed enriched in actin-binding proteins (18, 19); they contain phosphatidylinositol-4,5-bisphosphate (PIP2) (20, 21), which is a key regulator of the actin-polymerization machinery that, on the other hand, regulates raft assembly and dynamics (22, 23).
As for membrane rafts in immunity, over the last decades it has become evident that these microdomains actively modulate immune signaling, contributing to orchestrate innate and acquired immune responses (24). Compartmentalization of signaling molecules represents indeed an essential strategy exploited by immune cells to integrate multiple extracellular cues and trigger and direct complex functions (25). In T lymphocytes, this is primarily achieved by the allocation of selected proteins into well-organized structures, such as the immunological synapse (IS)—during antigen recognition—or the leading and rear edges—during cell migration—and, importantly, by the clustering of key signaling players into membrane rafts.
This review will describe how membrane rafts support T cell activation, focusing in particular on CD28-mediated costimulation.
In order to become fully activated, T cells require a double signal. The first signal is T cell receptor (TCR) ligation by peptide-major histocompatibility complex on antigen-presenting cells (APCs); the second signal derives from the interaction between a coreceptor on the T cell with counter-receptors expressed by APCs. The major coreceptor molecule expressed by T cells is CD28, whose ligands on professional APCs are CD80/B7.1 and CD86/B7.2 (26–28).
The combination of antigen recognition and costimulation results in the formation of a mature IS (29), a dynamic structure whose role is to regulate T cell function by integrating different signals and, thus, determining the fate of an antigenic stimulation. The IS, also defined as supramolecular activation cluster (SMAC), is described as a “bull’s eye structure,” organized in a central region (c-SMAC) and a peripheral region (p-SMAC) (30). Several proteins involved in T cell signaling, such as TCR/CD3, CD28, protein kinase Cθ (PKCθ), lymphocyte specific protein-tyrosine kinase (Lck), and zeta-chain-associated protein kinase 70 (ZAP-70) are located in the c-SMAC, surrounded by a ring of cytoskeletal or adhesion molecules, forming the p-SMAC, which provides structural support to the IS. Antigen recognition occurs in the c-SMAC, while the p-SMAC maintains the architecture of the IS and stabilizes the T cell–APC conjugation. The spatial organization of the IS is intimately correlated to its function and ensures a fine regulation of T cell activation: indeed, the integration of different signals occurring at the level of the IS ensures both the sensitivity and the specificity of T cell responses.
The IS is characterized by a peculiar lipid composition, which differs from the lipid composition of other membrane regions (31). Live-cell imaging experiments have elegantly shown that raft lipid localization is regulated during the IS formation: rafts first enrich in the center of the synapse and then move to the periphery (31, 32).
Importantly, the functional relevance of membrane rafts is supported by the observation that several signaling molecules involved in T cell activation can be found in raft domains. The Src kinase Lck, which is activated immediately after TCR triggering, is acylated on two cysteine residues on its N-terminus, thus being targeted to membrane rafts (33). This is crucial for Lck-mediated tyrosine phosphorylation, as demonstrated by the fact that Lck mutants resistant to acylation do not localize to the plasma membrane and do not fully activate downstream signaling (34). Also the linker for activation of T cells (LAT) localizes to raft domains. Upon TCR engagement, LAT is phosphorylated by ZAP-70 and, consequently, activates several signaling pathways (35, 36). Mutations preventing LAT palmitoylation inhibit LAT phosphorylation by ZAP-70 and hinder TCR signal transduction (37, 38). Also PKCθ is localized to membrane rafts and clusters at the IS in CD3/CD28-stimulated T cells; PKCθ raft translocation depends on Lck and is crucial for downstream NF-κB activation (39). In addition, the activation of phosphoinositide 3-kinase (PI3K)/Akt signaling pathway, which is crucial for T cell activation, has been shown to be tightly connected to the presence of raft nanodomains (40).
Altogether, these results support a model in which lipid rafts act as a docking platform, where the actors involved in T cell activation can organize and integrate downstream signaling events.
In T cells, the coupling of the outer and inner lipid microdomains is mediated by cholesterol. By contrast, the crosslinking of TCR/CD3 triggers the formation of large, stable rafts independently of cholesterol (41). These results are explained by the role of CD28 in the generation of membrane rafts.
The costimulatory molecule CD28 plays a crucial role in determining T cell sensitivity. Its ligands CD80 and CD86 are highly expressed by pathogen-activated professional APCs, such as mature dendritic cells, macrophages, and activated B cells. As a consequence, CD28 costimulation occurs in the context of infection and/or inflammation, thus representing a possible control mechanism for avoiding excessive T cell responses. Accordingly, CD28 has been indicated as an important regulator of autoimmune diseases (42). Interestingly, genome-wide studies on patients have associated single nucleotide polymorphisms in the CD28 gene to increased risk for autoimmune disease (43). Notably, two recent works appointed CD28 signaling pathway as the direct target of PD-1 based-therapy, thus providing intriguing insights for the improvement of current immune-therapies against cancer and viral infections (44, 45).
CD28 costimulation is indeed fundamental for full T cell activation, as it lowers the stimulation threshold of naïve T cells, in terms of number of triggered TCRs (28), preventing anergy and enhancing cytokine production, such as interleukin-2 (IL-2), and lymphocyte proliferation (46). T lymphocytes from CD28 knockout mice can be activated by increasing antigen doses, showing that the signals delivered by CD28 can be replaced, at least in part, by stronger TCR signaling (27, 47). These results suggest that CD28 functions as a general amplifier of early TCR signaling, thereby determining the sensitivity of the adaptive immune response. However, several studies have highlighted that CD28 regulates T cell activation not only quantitatively but also qualitatively (48).
Numerous works have demonstrated that CD28 costimulation regulates T cell function, by enhancing several signaling pathways and, finally, tuning gene transcription (49, 50). This can be achieved thanks to the presence of highly conserved tyrosine- and proline-rich signaling motifs on CD28 cytosolic tail, which are phosphorylated following CD3/CD28 stimulation and act as docking sites for the recruitment through their SH2 and/or SH3 domains of several downstream factors (51), such as PI3K (52, 53), Tec and Itk protein-tyrosine kinases (54–56), growth factor receptor-bound protein 2 (Grb2) (57), Grb2-related adaptor protein (Gads) (58), Lck (59), PKCθ (60, 61), Filamin A and associated NF-κB inducing kinase (22, 62), the guanine nucleotide exchange factor Vav1, and associated phosphatidylinositol 4,5-biphosphate kinases (PIP5Ks) α and β (23, 63).
Importantly, CD28-mediated costimulation is intrinsically linked to the reorganization of membrane rafts at T lymphocyte plasma membrane.
We and others have shown that CD28 coengagement results in membrane rafts clustering at the site of TCR triggering (22, 64–69). Rafts recruitment into the IS requires indeed CD28 signaling and is not observed in T cells stimulated by CD3-specific antibodies or through the TCR only (22, 64, 69). In addition, CD28-induced membrane raft assembly may precede TCR triggering and prepare a signaling microdomain for the upcoming IS (70).
CD28-mediated raft recruitment is a central event for the orchestration of TCR signaling. Indeed, CD28 coengagement by Abs or natural ligands protects the action of TCR-activated kinases from the inhibition mediated by phosphatases. Consequently, tyrosine phosphorylation of TCR signaling targets is stabilized and can be observed for several minutes, while being transient and unstable in the absence of CD28 costimulation (64). Quantitative phosphoproteomic analysis confirmed that CD3/CD28 stimulation enhances the phosphorylation of sites that are usually modified by CD3 stimulation (71).
As mentioned before, several proteins involved in TCR signaling have been found associated with membrane rafts and, at least for some of them, the recruitment to the IS depends on CD28-triggered organization of lipid domains. Lck, for example, accumulates at the IS in a CD28-dependent way and this process requires the CD28 COOH-terminal PxxPP motif and Vav1 (69).
Also PKCθ clusters at the IS upon CD3/CD28 engagement translocating to membrane rafts (39). Its association with CD28 is necessary for the activation of downstream signaling as NF-κB pathway and plays a crucial role for the differentiation of Th2 and Th17 subtypes, but not Th1 (61, 72, 73). The lymphoid cell-specific actin-uncapping protein Rltpr binds to CD28 and is required for the relocation of PKCθ and CARD-containing MAGUK protein 1 (Carma1) at the central region of the IS (74, 75).
The group of Tasken has described a further CD28-dependent mechanism of T cell regulation, involving cyclic adenosine monophosphate (cAMP) and protein kinase A (PKA). It has been known for a long time that TCR ligation induces an increase in cAMP production (76), which in turns results in PKA recruitment to lipid rafts via ezrin and leads to the inhibition of T cell function and proliferation (77). However, when the costimulatory signal delivered by CD28 is triggered, the phosphodiesterase enzyme PDE4 is recruited to lipid rafts; PDE4 locally degrades the TCR-induced cAMP pool, thus counteracting cAMP-mediated inhibition of T cell functions (78, 79). The recruitment of PDE4 to lipid rafts depends on CD28 (78) and involves PI3K activity and PIP3 production (79).
The dynamic and functional assembly of the IS depends on actin cytoskeleton rearrangements (80). The actin cytoskeleton drives the contact between the T cell and the APC not only mechanically but also directly interacting with IS-associated signaling molecules (81). As stated above, several components of the actin-based cytoskeleton are enriched in lipid rafts purified from T cells, as highlighted by mass-spectrometry-based proteomic analysis (3, 18, 19).
One of the most characterized functions of CD28 is the ability to organize the cytoskeleton. At the immune synapse, the CD3/CD28-activated polymerization of actin is regulated by the activity of the guanine nucleotide-exchange factor Vav1 (82, 83), the small Rho GTPase cell division control protein 42 (Cdc42) (84), the Wiskott–Aldrich Syndrome protein (WASP) (66) and the actin-related protein 2/3 complex (ARP2/3). The ARP2/3 complex interacts with filamins, elongated proteins that crosslink F-actin thus generating and organizing dynamic cytoskeletal networks (85). We demonstrated that, after physiological stimulation, CD28 recruits Filamin A at the IS (22), where Filamin A regulates TCR and CD28 signaling (22, 86). Interestingly, the absence of Filamin A results in decreased membrane raft clustering at the IS along with impaired CD28-dependent costimulation (22). Furthermore, Filamin A seems to contribute to the Vav1-dependent actin-polymerization pathway to regulate raft dynamics by confining them at the IS (3, 22).
Vav1 is a key regulator of cytoskeletal dynamics and T cell responses: indeed, Vav1-deficient cells present impaired membrane raft clustering at the IS, which correlates with defects in cytoskeletal reorganization and T cell activation (67, 87, 88). CD28, via the adaptor protein Grb2, mediates the formation of Vav1/SLP-76 complex, which is crucial for initiating downstream signaling (82, 83).
The role of Vav1 in CD28-mediated signaling is crucial: T cells lacking the adaptor molecule Cbl-b display enhanced Vav1 activation and are independent of CD28 for their triggering (89), as well as for membrane raft clustering at the IS (90). In the absence of Cbl-b, the deregulated activation of the Vav1/WASP pathway induces receptor clustering irrespective of CD28 signaling and enhances TCR signaling (90). In agreement, WASP-deficient T cells show defects in membrane raft reorganization in response to CD28 costimulation (66).
Also cofilin has been proposed as a linker between the actin cytoskeleton and T cell costimulation. Indeed, TCR/CD28 engagement, but not TCR stimulation alone, induces cofilin dephosphorylation and its consequent association with actin (91). In T cells, cofilin dephosphorylation can be mediated by the protein phosphatases PP1 and PP2A (92); interestingly, PP2A has been reported to interact with CD28 (93), but the functional significance of this interaction has not been elucidated yet.
Cytoskeletal dynamics are also regulated by PIP2, produced by PIP5K enzymes. It has been estimated that half of the PIP2 pool is localized in membrane rafts (20) and is important, for example, for the recruitment of WASP (94). Recent studies show that upon CD28 costimulation, PIP5Kβ translocates to the IS where it organizes both the recruitment of filamin A and membrane raft clustering. Moreover, in collaboration with PIP5Kα and Vav1, PIP5Kβ promotes actin polymerization and CD28 signaling in human T cells (23, 63, 95, 96).
Since the first demonstration in 1999 for a role of CD28 in controlling membrane rafts assembly at the IS (64), several studies have contributed to identify the molecular mechanisms responsible for the formation of these selective and dynamic signaling microdomains. Today, we know that CD28-dependent recruitment of membrane rafts at the IS depends on specific residues of the CD28 cytoplasmic domain that allow CD28 to recruit Vav1 and initiate signaling pathways involving PIP5Ks and actin-binding proteins (Figure 1). This massive actin reorganization allows recruitment and trapping of membrane rafts in the IS and is required to sustain and amplify TCR-induced signaling when costimulation is provided. Thus, CD28-mediated assembly of membrane rafts represents an extraordinary strategy for spatiotemporal compartmentalization and context-specific amplification of signal transduction.
Figure 1. CD28 orchestrates membrane raft trapping at the immunological synapse (IS). The engagement of both the T cell receptor (TCR) and CD28 by the interaction with a professional antigen-presenting cell (APC) results in the formation of a mature IS, characterized by the clustering of membrane rafts. CD28 cytosolic tail binds Filamin A and the nucleotide-exchange factor Vav1, thus regulating the action of cell division control protein 42 (Cdc42), Wiskott–Aldrich Syndrome protein (WASP) and actin-related protein 2/3 complex (ARP2/3) proteins, which are responsible for increased actin polymerization at the IS. CD28, through Vav1, recruits phosphatidylinositol 4,5-biphosphate kinases (PIP5Ks) to the IS and promotes PIP5K-dependent production of phosphatidylinositol-4,5-bisphosphate (PIP2). PIP5Ks are pivotal for the recruitment of Filamin A and membrane rafts and sustain actin polymerization. The molecular interactions initiated by CD28 result in the cytoskeletal reorganization associated with membrane raft clustering at the IS.
SZ, BM, and AV conceived and wrote the manuscript and realized the figure.
The authors declare that the research was conducted in the absence of any commercial or financial relationships that could be construed as a potential conflict of interest.
The authors wish to thank the European Union’s Seventh Framework Programme for research, technological development, and demonstration under grant agreement no. 602363 and the ERC Advance Grant under grant agreement no. 322823 to AV.
1. Simons K, Ikonen E. Functional rafts in cell membranes. Nature (1997) 387:569–72. doi:10.1038/42408
2. Mayor S, Viola A, Stan RV, del Pozo MA. Flying kites on slippery slopes at keystone. Symposium on lipid rafts and cell function. EMBO Rep (2006) 7:1089–93. doi:10.1038/sj.embor.7400836
3. Viola A, Gupta N. Tether and trap: regulation of membrane-raft dynamics by actin-binding proteins. Nat Rev Immunol (2007) 7:889–96. doi:10.1038/nri2193
4. Pike LJ. Rafts defined: a report on the keystone symposium on lipid rafts and cell function. J Lipid Res (2006) 47:1597–8. doi:10.1194/jlr.E600002-JLR200
5. Lichtenberg D, Goñi FM, Heerklotz H. Detergent-resistant membranes should not be identified with membrane rafts. Trends Biochem Sci (2005) 30:430–6. doi:10.1016/j.tibs.2005.06.004
6. Lingwood D, Simons K. Detergent resistance as a tool in membrane research. Nat Protoc (2007) 2:2159–65. doi:10.1038/nprot.2007.294
7. Pizzo P, Giurisato E, Tassi M, Benedetti A, Pozzan T, Viola A. Lipid rafts and T cell receptor signaling: a critical re-evaluation. Eur J Immunol (2002) 32:3082–91. doi:10.1002/1521-4141(200211)32:11<3082:AID-IMMU3082>3.0.CO;2-2
8. Subczynski WK, Kusumi A. Dynamics of raft molecules in the cell and artificial membranes: approaches by pulse EPR spin labeling and single molecule optical microscopy. Biochim Biophys Acta (2003) 1610:231–43. doi:10.1016/S0005-2736(03)00021-X
9. Schütz GJ, Kada G, Pastushenko VP, Schindler H. Properties of lipid microdomains in a muscle cell membrane visualized by single molecule microscopy. EMBO J (2000) 19:892–901. doi:10.1093/emboj/19.5.892
10. Pralle A, Keller P, Florin EL, Simons K, Hörber JK. Sphingolipid-cholesterol rafts diffuse as small entities in the plasma membrane of mammalian cells. J Cell Biol (2000) 148:997–1008. doi:10.1083/jcb.148.5.997
11. Varma R, Mayor S. GPI-anchored proteins are organized in submicron domains at the cell surface. Nature (1998) 394:798–801. doi:10.1038/29563
12. Kenworthy AK. Fluorescence recovery after photobleaching studies of lipid rafts. Methods Mol Biol (2007) 398:179–92. doi:10.1007/978-1-59745-513-8_13
13. Asanov A, Zepeda A, Vaca L. A novel form of total internal reflection fluorescence microscopy (LG-TIRFM) reveals different and independent lipid raft domains in living cells. Biochim Biophys Acta (2010) 1801:147–55. doi:10.1016/j.bbalip.2009.10.004
14. Eggeling C, Ringemann C, Medda R, Schwarzmann G, Sandhoff K, Polyakova S, et al. Direct observation of the nanoscale dynamics of membrane lipids in a living cell. Nature (2009) 457:1159–62. doi:10.1038/nature07596
15. Simons K, Toomre D. Lipid rafts and signal transduction. Nat Rev Mol Cell Biol (2000) 1:31–9. doi:10.1038/35036052
16. Pike LJ. Lipid rafts: bringing order to chaos. J Lipid Res (2003) 44:655–67. doi:10.1194/jlr.R200021-JLR200
17. Head BP, Patel HH, Insel PA. Interaction of membrane/lipid rafts with the cytoskeleton: impact on signaling and function: membrane/lipid rafts, mediators of cytoskeletal arrangement and cell signaling. Biochim Biophys Acta (2014) 1838:532–45. doi:10.1016/j.bbamem.2013.07.018
18. von Haller PD, Donohoe S, Goodlett DR, Aebersold R, Watts JD. Mass spectrometric characterization of proteins extracted from Jurkat T cell detergent-resistant membrane domains. Proteomics (2001) 1:1010–21. doi:10.1002/1615-9861(200108)1:8<1010:AID-PROT1010>3.0.CO;2-L
19. Billadeau DD, Burkhardt JK. Regulation of cytoskeletal dynamics at the immune synapse: new stars join the actin troupe. Traffic (2006) 7:1451–60. doi:10.1111/j.1600-0854.2006.00491.x
20. Liu Y, Casey L, Pike LJ. Compartmentalization of phosphatidylinositol 4,5-bisphosphate in low-density membrane domains in the absence of caveolin. Biochem Biophys Res Commun (1998) 245:684–90. doi:10.1006/bbrc.1998.8329
21. Pike LJ, Miller JM. Cholesterol depletion delocalizes phosphatidylinositol bisphosphate and inhibits hormone-stimulated phosphatidylinositol turnover. J Biol Chem (1998) 273:22298–304. doi:10.1074/jbc.273.35.22298
22. Tavano R, Contento RL, Baranda SJ, Soligo M, Tuosto L, Manes S, et al. CD28 interaction with filamin-A controls lipid raft accumulation at the T-cell immunological synapse. Nat Cell Biol (2006) 8:1270–6. doi:10.1038/ncb1492
23. Kallikourdis M, Trovato AE, Roselli G, Muscolini M, Porciello N, Tuosto L, et al. Phosphatidylinositol 4-phosphate 5-kinase β controls recruitment of lipid rafts into the immunological synapse. J Immunol (2016) 196:1955–63. doi:10.4049/jimmunol.1501788
24. Varshney P, Yadav V, Saini N. Lipid rafts in immune signalling: current progress and future perspective. Immunology (2016) 149:13–24. doi:10.1111/imm.12617
25. Russell S, Oliaro J. Compartmentalization in T-cell signalling: membrane microdomains and polarity orchestrate signalling and morphology. Immunol Cell Biol (2006) 84:107–13. doi:10.1111/j.1440-1711.2005.01415.x
26. June CH, Ledbetter JA, Linsley PS, Thompson CB. Role of the CD28 receptor in T-cell activation. Immunol Today (1990) 11:211–6. doi:10.1016/0167-5699(90)90085-N
27. Shahinian A, Pfeffer K, Lee KP, Kündig TM, Kishihara K, Wakeham A, et al. Differential T cell costimulatory requirements in CD28-deficient mice. Science (1993) 261:609–12. doi:10.1126/science.7688139
28. Viola A, Lanzavecchia A. T cell activation determined by T cell receptor number and tunable thresholds. Science (1996) 273:104–6. doi:10.1126/science.273.5271.104
29. Davis DM, Dustin ML. What is the importance of the immunological synapse? Trends Immunol (2004) 25:323–7. doi:10.1016/j.it.2004.03.007
30. Dustin ML. The immunological synapse. Cancer Immunol Res (2014) 2:1023–33. doi:10.1158/2326-6066.CIR-14-0161
31. Gaus K, Chklovskaia E, Fazekas de St Groth B, Jessup W, Harder T. Condensation of the plasma membrane at the site of T lymphocyte activation. J Cell Biol (2005) 171:121–31. doi:10.1083/jcb.200505047
32. Owen DM, Oddos S, Kumar S, Davis DM, Neil MAA, French PMW, et al. High plasma membrane lipid order imaged at the immunological synapse periphery in live T cells. Mol Membr Biol (2010) 27:178–89. doi:10.3109/09687688.2010.495353
33. Resh MD. Myristylation and palmitylation of Src family members: the fats of the matter. Cell (1994) 76:411–3. doi:10.1016/0092-8674(94)90104-X
34. Kabouridis PS, Magee AI, Ley SC. S-acylation of LCK protein tyrosine kinase is essential for its signalling function in T lymphocytes. EMBO J (1997) 16:4983–98. doi:10.1093/emboj/16.16.4983
35. Zhang W, Sloan-Lancaster J, Kitchen J, Trible RP, Samelson LE. LAT: the ZAP-70 tyrosine kinase substrate that links T cell receptor to cellular activation. Cell (1998) 92:83–92. doi:10.1016/S0092-8674(00)80901-0
36. Sherman E, Barr V, Manley S, Patterson G, Balagopalan L, Akpan I, et al. Functional nanoscale organization of signaling molecules downstream of the T cell antigen receptor. Immunity (2011) 35:705–20. doi:10.1016/j.immuni.2011.10.004
37. Zhang W, Trible RP, Samelson LE. LAT palmitoylation: its essential role in membrane microdomain targeting and tyrosine phosphorylation during T cell activation. Immunity (1998) 9:239–46. doi:10.1016/S1074-7613(00)80606-8
38. Harder T, Kuhn M. Selective accumulation of raft-associated membrane protein LAT in T cell receptor signaling assemblies. J Cell Biol (2000) 151:199–208. doi:10.1083/jcb.151.2.199
39. Bi K, Tanaka Y, Coudronniere N, Sugie K, Hong S, van Stipdonk MJ, et al. Antigen-induced translocation of PKC-theta to membrane rafts is required for T cell activation. Nat Immunol (2001) 2:556–63. doi:10.1038/88765
40. Lasserre R, Guo X-J, Conchonaud F, Hamon Y, Hawchar O, Bernard A-M, et al. Raft nanodomains contribute to Akt/PKB plasma membrane recruitment and activation. Nat Chem Biol (2008) 4:538–47. doi:10.1038/nchembio.103
41. Gri G, Molon B, Manes S, Pozzan T, Viola A. The inner side of T cell lipid rafts. Immunol Lett (2004) 94:247–52. doi:10.1016/j.imlet.2004.05.012
42. Salomon B, Lenschow DJ, Rhee L, Ashourian N, Singh B, Sharpe A, et al. B7/CD28 costimulation is essential for the homeostasis of the CD4+CD25+ immunoregulatory T cells that control autoimmune diabetes. Immunity (2000) 12:431–40. doi:10.1016/S1074-7613(00)80195-8
43. Raychaudhuri S, Thomson BP, Remmers EF, Eyre S, Hinks A, Guiducci C, et al. Genetic variants at CD28, PRDM1 and CD2/CD58 are associated with rheumatoid arthritis risk. Nat Genet (2009) 41:1313–8. doi:10.1038/ng.479
44. Hui E, Cheung J, Zhu J, Su X, Taylor MJ, Wallweber HA, et al. T cell costimulatory receptor CD28 is a primary target for PD-1-mediated inhibition. Science (2017) 355:1428–33. doi:10.1126/science.aaf1292
45. Kamphorst AO, Wieland A, Nasti T, Yang S, Zhang R, Barber DL, et al. Rescue of exhausted CD8 T cells by PD-1-targeted therapies is CD28-dependent. Science (2017) 355:1423–7. doi:10.1126/science.aaf0683
46. Harding FA, McArthur JG, Gross JA, Raulet DH, Allison JP. CD28-mediated signalling co-stimulates murine T cells and prevents induction of anergy in T-cell clones. Nature (1992) 356:607–9. doi:10.1038/356607a0
47. Kündig TM, Shahinian A, Kawai K, Mittrücker HW, Sebzda E, Bachmann MF, et al. Duration of TCR stimulation determines costimulatory requirement of T cells. Immunity (1996) 5:41–52. doi:10.1016/S1074-7613(00)80308-8
48. Acuto O, Michel F. CD28-mediated co-stimulation: a quantitative support for TCR signalling. Nat Rev Immunol (2003) 3:939–51. doi:10.1038/nri1248
49. Viola A, Contento RL, Molon B. Signaling amplification at the immunological synapse. Curr Top Microbiol Immunol (2010) 340:109–22. doi:10.1007/978-3-642-03858-7_6
50. Porciello N, Tuosto L. CD28 costimulatory signals in T lymphocyte activation: emerging functions beyond a qualitative and quantitative support to TCR signalling. Cytokine Growth Factor Rev (2016) 28:11–9. doi:10.1016/j.cytogfr.2016.02.004
51. Esensten JH, Helou YA, Chopra G, Weiss A, Bluestone JA. CD28 costimulation: from mechanism to therapy. Immunity (2016) 44:973–88. doi:10.1016/j.immuni.2016.04.020
52. Pagès F, Ragueneau M, Rottapel R, Truneh A, Nunes J, Imbert J, et al. Binding of phosphatidylinositol-3-OH kinase to CD28 is required for T-cell signalling. Nature (1994) 369:327–9. doi:10.1038/369327a0
53. Harada Y, Ohgai D, Watanabe R, Okano K, Koiwai O, Tanabe K, et al. A single amino acid alteration in cytoplasmic domain determines IL-2 promoter activation by ligation of CD28 but not inducible costimulator (ICOS). J Exp Med (2003) 197:257–62. doi:10.1084/jem.20021305
54. August A, Gibson S, Kawakami Y, Kawakami T, Mills GB, Dupont B. CD28 is associated with and induces the immediate tyrosine phosphorylation and activation of the Tec family kinase ITK/EMT in the human Jurkat leukemic T-cell line. Proc Natl Acad Sci U S A (1994) 91:9347–51. doi:10.1073/pnas.91.20.9347
55. Marengère LE, Okkenhaug K, Clavreul A, Couez D, Gibson S, Mills GB, et al. The SH3 domain of Itk/Emt binds to proline-rich sequences in the cytoplasmic domain of the T cell costimulatory receptor CD28. J Immunol (1997) 159:3220–9.
56. Garçon F, Ghiotto M, Gérard A, Yang W-C, Olive D, Nunès JA. The SH3 domain of Tec kinase is essential for its targeting to activated CD28 costimulatory molecule. Eur J Immunol (2004) 34:1972–80. doi:10.1002/eji.200324777
57. Raab M, Cai YC, Bunnell SC, Heyeck SD, Berg LJ, Rudd CE. p56Lck and p59Fyn regulate CD28 binding to phosphatidylinositol 3-kinase, growth factor receptor-bound protein GRB-2, and T cell-specific protein-tyrosine kinase ITK: implications for T-cell costimulation. Proc Natl Acad Sci U S A (1995) 92:8891–5. doi:10.1073/pnas.92.19.8891
58. Watanabe R, Harada Y, Takeda K, Takahashi J, Ohnuki K, Ogawa S, et al. Grb2 and Gads exhibit different interactions with CD28 and play distinct roles in CD28-mediated costimulation. J Immunol (2006) 177:1085–91. doi:10.4049/jimmunol.177.2.1085
59. Holdorf AD, Green JM, Levin SD, Denny MF, Straus DB, Link V, et al. Proline residues in CD28 and the Src homology (SH)3 domain of Lck are required for T cell costimulation. J Exp Med (1999) 190:375–84. doi:10.1084/jem.190.3.375
60. Villalba M, Coudronniere N, Deckert M, Teixeiro E, Mas P, Altman A. A novel functional interaction between Vav and PKCtheta is required for TCR-induced T cell activation. Immunity (2000) 12:151–60. doi:10.1016/S1074-7613(00)80168-5
61. Yokosuka T, Kobayashi W, Sakata-Sogawa K, Takamatsu M, Hashimoto-Tane A, Dustin ML, et al. Spatiotemporal regulation of T cell costimulation by TCR-CD28 microclusters and protein kinase C theta translocation. Immunity (2008) 29:589–601. doi:10.1016/j.immuni.2008.08.011
62. Muscolini M, Sajeva A, Caristi S, Tuosto L. A novel association between filamin A and NF-κB inducing kinase couples CD28 to inhibitor of NF-κB kinase α and NF-κB activation. Immunol Lett (2011) 136:203–12. doi:10.1016/j.imlet.2011.01.011
63. Muscolini M, Camperio C, Porciello N, Caristi S, Capuano C, Viola A, et al. Phosphatidylinositol 4-phosphate 5-kinase α and Vav1 mutual cooperation in CD28-mediated actin remodeling and signaling functions. J Immunol (2015) 194:1323–33. doi:10.4049/jimmunol.1401643
64. Viola A, Schroeder S, Sakakibara Y, Lanzavecchia A. T lymphocyte costimulation mediated by reorganization of membrane microdomains. Science (1999) 283:680–2. doi:10.1126/science.283.5402.680
65. Martin M, Schneider H, Azouz A, Rudd CE. Cytotoxic T lymphocyte antigen 4 and CD28 modulate cell surface raft expression in their regulation of T cell function. J Exp Med (2001) 194:1675–81. doi:10.1084/jem.194.11.1675
66. Dupré L, Aiuti A, Trifari S, Martino S, Saracco P, Bordignon C, et al. Wiskott-Aldrich syndrome protein regulates lipid raft dynamics during immunological synapse formation. Immunity (2002) 17:157–66. doi:10.1016/S1074-7613(02)00360-6
67. Paccani SR, Boncristiano M, Patrussi L, Ulivieri C, Wack A, Valensin S, et al. Defective Vav expression and impaired F-actin reorganization in a subset of patients with common variable immunodeficiency characterized by T-cell defects. Blood (2005) 106:626–34. doi:10.1182/blood-2004-05-2051
68. Round JL, Tomassian T, Zhang M, Patel V, Schoenberger SP, Miceli MC. Dlgh1 coordinates actin polymerization, synaptic T cell receptor and lipid raft aggregation, and effector function in T cells. J Exp Med (2005) 201:419–30. doi:10.1084/jem.20041428
69. Tavano R, Gri G, Molon B, Marinari B, Rudd CE, Tuosto L, et al. CD28 and lipid rafts coordinate recruitment of Lck to the immunological synapse of human T lymphocytes. J Immunol (2004) 173:5392–7. doi:10.4049/jimmunol.173.9.5392
70. Byrum JN, Komen JSV, Rodgers W. CD28 sensitizes TCR Ca2+ signaling during Ag-independent polarization of plasma membrane rafts. J Immunol (2013) 191:3073–81. doi:10.4049/jimmunol.1300485
71. Kim J-E, White FM. Quantitative analysis of phosphotyrosine signaling networks triggered by CD3 and CD28 costimulation in Jurkat cells. J Immunol (2006) 176:2833–43. doi:10.4049/jimmunol.176.5.2833
72. Sanchez-Lockhart M, Graf B, Miller J. Signals and sequences that control CD28 localization to the central region of the immunological synapse. J Immunol (2008) 181:7639–48. doi:10.4049/jimmunol.181.11.7639
73. Kong K-F, Yokosuka T, Canonigo-Balancio AJ, Isakov N, Saito T, Altman A. A motif in the V3 domain of the kinase PKC-θ determines its localization in the immunological synapse and functions in T cells via association with CD28. Nat Immunol (2011) 12:1105–12. doi:10.1038/ni.2120
74. Liang Y, Cucchetti M, Roncagalli R, Yokosuka T, Malzac A, Bertosio E, et al. The lymphoid lineage-specific actin-uncapping protein Rltpr is essential for costimulation via CD28 and the development of regulatory T cells. Nat Immunol (2013) 14:858–66. doi:10.1038/ni.2634
75. Roncagalli R, Cucchetti M, Jarmuzynski N, Grégoire C, Bergot E, Audebert S, et al. The scaffolding function of the RLTPR protein explains its essential role for CD28 co-stimulation in mouse and human T cells. J Exp Med (2016) 213:2437–57. doi:10.1084/jem.20160579
76. Ledbetter JA, Parsons M, Martin PJ, Hansen JA, Rabinovitch PS, June CH. Antibody binding to CD5 (Tp67) and Tp44 T cell surface molecules: effects on cyclic nucleotides, cytoplasmic free calcium, and cAMP-mediated suppression. J Immunol (1986) 137:3299–305.
77. Ruppelt A, Mosenden R, Grönholm M, Aandahl EM, Tobin D, Carlson CR, et al. Inhibition of T cell activation by cyclic adenosine 5’-monophosphate requires lipid raft targeting of protein kinase A type I by the A-kinase anchoring protein ezrin. J Immunol (2007) 179:5159–68. doi:10.4049/jimmunol.179.8.5159
78. Abrahamsen H, Baillie G, Ngai J, Vang T, Nika K, Ruppelt A, et al. TCR- and CD28-mediated recruitment of phosphodiesterase 4 to lipid rafts potentiates TCR signaling. J Immunol (2004) 173:4847–58. doi:10.4049/jimmunol.173.8.4847
79. Bjørgo E, Solheim SA, Abrahamsen H, Baillie GS, Brown KM, Berge T, et al. Cross talk between phosphatidylinositol 3-kinase and cyclic AMP (cAMP)-protein kinase a signaling pathways at the level of a protein kinase B/beta-arrestin/cAMP phosphodiesterase 4 complex. Mol Cell Biol (2010) 30:1660–72. doi:10.1128/MCB.00696-09
80. Monks CR, Freiberg BA, Kupfer H, Sciaky N, Kupfer A. Three-dimensional segregation of supramolecular activation clusters in T cells. Nature (1998) 395:82–6. doi:10.1038/25764
81. Comrie WA, Burkhardt JK. Action and traction: cytoskeletal control of receptor triggering at the immunological synapse. Front Immunol (2016) 7:68. doi:10.3389/fimmu.2016.00068
82. Raab M, Pfister S, Rudd CE. CD28 signaling via VAV/SLP-76 adaptors: regulation of cytokine transcription independent of TCR ligation. Immunity (2001) 15:921–33. doi:10.1016/S1074-7613(01)00248-5
83. Dennehy KM, Elias F, Na S-Y, Fischer K-D, Hünig T, Lühder F. Mitogenic CD28 signals require the exchange factor Vav1 to enhance TCR signaling at the SLP-76-Vav-Itk signalosome. J Immunol (2007) 178:1363–71. doi:10.4049/jimmunol.178.3.1363
84. Salazar-Fontana LI, Barr V, Samelson LE, Bierer BE. CD28 engagement promotes actin polymerization through the activation of the small Rho GTPase Cdc42 in human T cells. J Immunol (2003) 171:2225–32. doi:10.4049/jimmunol.171.5.2225
85. Nakamura F, Stossel TP, Hartwig JH. The filamins: organizers of cell structure and function. Cell Adh Migr (2011) 5:160–9. doi:10.4161/cam.5.2.14401
86. Hayashi K, Altman A. Filamin A is required for T cell activation mediated by protein kinase C-theta. J Immunol (2006) 177:1721–8. doi:10.4049/jimmunol.177.3.1721
87. Fischer KD, Kong YY, Nishina H, Tedford K, Marengère LE, Kozieradzki I, et al. Vav is a regulator of cytoskeletal reorganization mediated by the T-cell receptor. Curr Biol (1998) 8:554–62. doi:10.1016/S0960-9822(98)70224-6
88. Villalba M, Bi K, Rodriguez F, Tanaka Y, Schoenberger S, Altman A. Vav1/Rac-dependent actin cytoskeleton reorganization is required for lipid raft clustering in T cells. J Cell Biol (2001) 155:331–8. doi:10.1083/jcb.200107080
89. Chiang YJ, Kole HK, Brown K, Naramura M, Fukuhara S, Hu RJ, et al. Cbl-b regulates the CD28 dependence of T-cell activation. Nature (2000) 403:216–20. doi:10.1038/35003235
90. Krawczyk C, Bachmaier K, Sasaki T, Jones RG, Snapper SB, Bouchard D, et al. Cbl-b is a negative regulator of receptor clustering and raft aggregation in T cells. Immunity (2000) 13:463–73. doi:10.1016/S1074-7613(00)00046-7
91. Lee KH, Meuer SC, Samstag Y. Cofilin: a missing link between T cell co-stimulation and rearrangement of the actin cytoskeleton. Eur J Immunol (2000) 30:892–9. doi:10.1002/1521-4141(200003)30:3<892:AID-IMMU892>3.0.CO;2-U
92. Ambach A, Saunus J, Konstandin M, Wesselborg S, Meuer SC, Samstag Y. The serine phosphatases PP1 and PP2A associate with and activate the actin-binding protein cofilin in human T lymphocytes. Eur J Immunol (2000) 30:3422–31. doi:10.1002/1521-4141(2000012)30:12<3422::AID-IMMU3422>3.0.CO;2-J
93. Chuang E, Fisher TS, Morgan RW, Robbins MD, Duerr JM, Vander Heiden MG, et al. The CD28 and CTLA-4 receptors associate with the serine/threonine phosphatase PP2A. Immunity (2000) 13:313–22. doi:10.1016/S1074-7613(00)00031-5
94. Golub T, Caroni P. PI(4,5)P2-dependent microdomain assemblies capture microtubules to promote and control leading edge motility. J Cell Biol (2005) 169:151–65. doi:10.1083/jcb.200407058
95. Muscolini M, Camperio C, Capuano C, Caristi S, Piccolella E, Galandrini R, et al. Phosphatidylinositol 4-phosphate 5-kinase α activation critically contributes to CD28-dependent signaling responses. J Immunol (2013) 190:5279–86. doi:10.4049/jimmunol.1203157
Keywords: T lymphocyte, CD28 costimulation, membrane rafts, immunological synapse, cytoskeleton
Citation: Zumerle S, Molon B and Viola A (2017) Membrane Rafts in T Cell Activation: A Spotlight on CD28 Costimulation. Front. Immunol. 8:1467. doi: 10.3389/fimmu.2017.01467
Received: 13 September 2017; Accepted: 19 October 2017;
Published: 03 November 2017
Edited by:
Chenqi Xu, Chinese Academy of Sciences, ChinaReviewed by:
Jacques A. Nunes, Centre de Recherche en Cancerologie de Marseille, FranceCopyright: © 2017 Zumerle, Molon and Viola. This is an open-access article distributed under the terms of the Creative Commons Attribution License (CC BY). The use, distribution or reproduction in other forums is permitted, provided the original author(s) or licensor are credited and that the original publication in this journal is cited, in accordance with accepted academic practice. No use, distribution or reproduction is permitted which does not comply with these terms.
*Correspondence: Antonella Viola, YW50b25lbGxhLnZpb2xhQHVuaXBkLml0
Disclaimer: All claims expressed in this article are solely those of the authors and do not necessarily represent those of their affiliated organizations, or those of the publisher, the editors and the reviewers. Any product that may be evaluated in this article or claim that may be made by its manufacturer is not guaranteed or endorsed by the publisher.
Research integrity at Frontiers
Learn more about the work of our research integrity team to safeguard the quality of each article we publish.