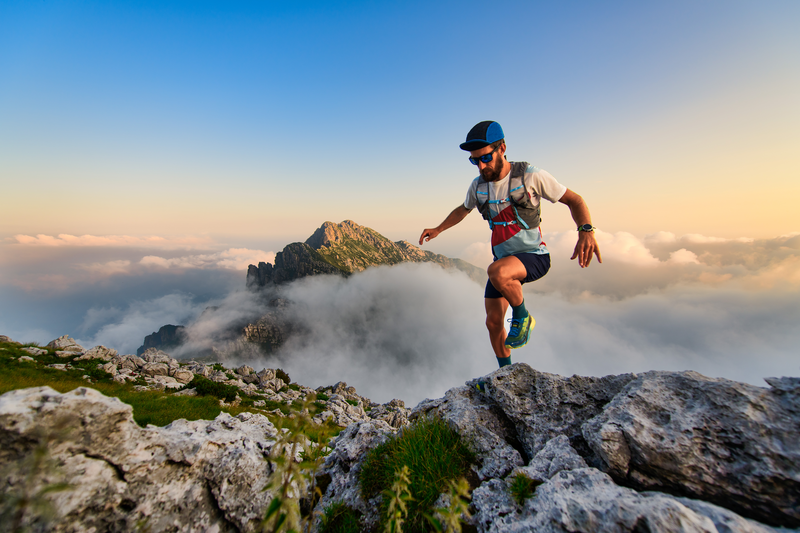
95% of researchers rate our articles as excellent or good
Learn more about the work of our research integrity team to safeguard the quality of each article we publish.
Find out more
MINI REVIEW article
Front. Immunol. , 12 October 2017
Sec. Immunological Tolerance and Regulation
Volume 8 - 2017 | https://doi.org/10.3389/fimmu.2017.01282
This article is part of the Research Topic Therapeutic Potential of Gene-Modified Regulatory T Cells View all 10 articles
The development of novel approaches to control immune responses to self- and allogenic tissues/organs represents an ambitious goal for the management of autoimmune diseases and in transplantation. Regulatory T cells (Tregs) are recognized as key players in the maintenance of peripheral tolerance in physiological and pathological conditions, and Treg-based cell therapies to restore tolerance in T cell-mediated disorders have been designed. However, several hurdles, including insufficient number of Tregs, their stability, and their antigen specificity, have challenged Tregs clinical applicability. In the past decade, the ability to engineer T cells has proven a powerful tool to redirect specificity and function of different cell types for specific therapeutic purposes. By using lentivirus-mediated gene transfer of the thymic-derived Treg transcription factor forkhead-box-P3 (FOXP3) in conventional CD4+ T cells, we converted effector T cells into Treg-like cells, endowed with potent in vitro and in vivo suppressive activity. The resulting CD4FOXP3 T-cell population displays stable phenotype and suppressive function. We showed that this strategy restores Treg function in T lymphocytes from patients carrying mutations in FOXP3 [immune-dysregulation, polyendocrinopathy, enteropathy, X-linked (IPEX)], in whom CD4FOXP3 T cell could be used as therapeutics to control autoimmunity. Here, we will discuss the potential advantages of using CD4FOXP3 T cells for in vivo application in inflammatory diseases, where tissue inflammation may undermine the function of natural Tregs. These findings pave the way for the use of engineered Tregs not only in IPEX syndrome but also in autoimmune disorders of different origin and in the context of stem cell and organ transplantation.
Regulatory T cells (Tregs) are a subset of T lymphocytes devoted to the modulation of immune responses and to the maintenance of immunological tolerance. They control aberrant immune responses toward a wide range of antigens (Ags), including self-, food-Ags, allergens, and tumors (1). Several subsets of Tregs have been identified. Among those, Tregs expressing the forkhead-box-P3 (FOXP3) transcription factor (FOXP3+-Tregs) (2, 3) and the IL-10-dependent T-regulatory-type-1 cells (4) are the best characterized. The latter will be the subject of a review by Gregori et al. in the present Research Topic, whereas the former subset and its application in the clinical practice will be discussed here.
FOXP3+-Tregs can originate either in the thymus [thymic-derived Tregs (tTregs)] or differentiate in the periphery from naïve T cells (pTregs) (5, 6). Regardless of their origin, both subsets are characterized by constitutive expression of FOXP3, a transcription factor critical for their function, as demonstrated by the devastating autoimmunity resulting from mutations of FOXP3 (7, 8). Impaired Treg function is the key pathogenic event leading to disruption of self-tolerance in patients with immune-dysregulation, polyendocrinopathy, enteropathy, X-linked (IPEX) syndrome (9, 10).
It is now well accepted that although FOXP3 expression is dispensable for thymic development of tTregs, mainly dictated by epigenetic remodeling occurring regardless of FOXP3, its expression becomes fundamental in later stages for the peripheral function and maintenance of Tregs (11). Indeed, high and stable FOXP3 expression allows the acquisition of full suppressive function and stability of the Treg lineage by orchestrating the expression or repression of multiple genes indispensable for Treg suppressive function (12–14).
In addition to FOXP3, the expression of several molecules, including high CD25 (IL2Rα chain) in the absence of CD127 (IL7Rα chain) (15), CTLA-4 (16), GITR (17), CD39 (18), Galectin 10 (19), latency-associated peptide (20), Helios (21), the T-cell immune receptor TIGIT (22), and glycoprotein-A repetitions predominant (23) has been associated with human FOXP3+-Tregs, although none of these molecules is exclusive for this subset, but shared with activated conventional T cells. To date, the most reliable feature unambiguously identifying FOXP3+-Tregs is the epigenetic remodeling of specific genomic regions within the FOXP3-locus (CNS2-TSDR) (24) or in Treg-related genes (11).
FOXP3+-Tregs modulate both innate and adaptive immune cells by various mechanisms. The inhibitory activity of Tregs is primarily dependent on contact with target cells, which allows modulation of antigen-presenting cells stimulatory capacity via CTLA-4 (25) or the killing of T effector (Teff) cells through the granzyme/perforin axis (26, 27). Additional mechanisms of suppression include the release of inhibitory cytokines, e.g., IL-10 (28), TGF-β (29, 30), and IL-35, at least in murine Tregs (31), cytokine deprivation (32), and generation of immunosuppressive metabolites, i.e., extracellular adenosine (33) and intracellular cAMP (34). FOXP3+-Tregs are not a homogeneous population but are rather constituted by a heterogeneous pool, including specialized subtypes (28, 35–39).
Their great potential as modulators of immune responses, resulting from both preclinical models and clinical evidences, convinced investigators that Tregs could be used as tools to control unwanted immune responses in the context of transplantation or to treat autoimmune/inflammatory diseases (40, 41). A great effort has been devoted to the development of good-manufacturing practice-grade protocols to isolate/expand human Tregs in vitro allowing translation of Treg-based cell therapy to the clinical practice (42–45).
In this review, we will give an overview of the clinical trials that applied FOXP3+-Tregs as therapeutics for the control of graft-versus-host disease (GvHD) in the context of hematopoietic stem cell transplantation (HSCT) and for the modulation of autoimmune reactions and the challenges that these trials highlighted. We will discuss the innovative therapeutic approach based on adoptive transfer of engineered Treg-like cells that we are developing for the treatment of IPEX syndrome, whose application could potentially extend to reestablish tolerance in autoimmune diseases of different origin and in transplantation.
Several Phase I-clinical trials have been conducted to assess the effect of Treg-based cell therapy on GvHD following allogenic HSCT, organ transplantation, in patients with type 1 diabetes (T1D) and chronic inflammatory diseases. Overall, results obtained with different subsets of Tregs demonstrated favorable safety profiles (46, 47).
Regulatory T cell-based clinical trials in HSCT have preceded other indications because the timing of GvHD onset is known and can be monitored, the time needed for prevention is relatively short, the initial efficacy is likely to provide lifelong protection, and complications of GvHD can be lethal.
Several groups have applied polyclonal CD4+CD25+ Tregs containing a high proportion of FOXP3+ T cells, either freshly isolated or ex vivo expanded, with the aim of preventing GvHD after allogenic HSCT for onco-hematological diseases. The results showed that the overall procedure is feasible and safe (48–52). One trial reported decreased incidence of grade II–IV GvHD as compared with historical controls in patients receiving umbilical cord blood-derived Tregs, without increased infections (49). Data were confirmed in a more recent trial from the same group, in which the clinical outcome of patients receiving Treg-based cell therapy was compared with that of control patients who received the same conditioning regimen and immunosuppressive treatment but no Tregs. The incidence of grade II–IV acute-GvHD at 100 days was 9 vs 45% in controls, whereas chronic-GvHD at 1 year was 0 in treated patients (52).
In a third trial patients injected with freshly isolated peripheral Tregs showed low grade GvHD and no development of chronic-GvHD (50). More recently, the same group showed reduced incidence of relapse in Treg-treated patients (53).
These initial reassuring results encouraged a wider application of Tregs as therapy after solid organ transplantation. Several trials are currently ongoing, although final results are not currently available (47). Among those, in The-ONE-Study (http://www.onestudy.org/), a Phase I/II dose-escalation study, several subtypes of Tregs, including ex vivo expanded FOXP3+-Tregs, have been infused in patients undergoing kidney transplant with the goal of avoiding lifelong immunosuppression through the induction of active tolerance (NCT02129881) (47, 54). Similarly, a Treg-immunotherapy trial in the setting of liver transplantation, ThRIL (NCT02166177), has been initiated, although safety data are not yet available (44).
FOXP3+-Treg-based therapy was safely tested also in the context of autoimmune diseases. In a trial limited to few patients, ex vivo expanded CD4+CD25hiCD127− Tregs were administered to children with recent-onset T1D (55), and more recently to new-onset adult T1D patients (43). In both cases, the procedure appeared to be safe, although published data do not allow to draw conclusions on efficacy. Importantly, in the latter trial, safety was demonstrated for transfer of high number of Tregs (up to 2.6 × 109 cells) (43).
Overall, the data available support the feasibility and safety of the approach. These results convinced researchers to pursue adoptive Treg-cell therapy and much effort is currently devoted to address open issues in the field, such as the in vivo persistence and stability of the injected product and the need for Ag specificity to increase efficacy.
Immune-dysregulation, polyendocrinopathy, enteropathy, X-linked syndrome is the prototype poly-autoimmune disease caused by mutations in the gene encoding for the transcription factor FOXP3 (8). Affected patients develop early-onset multi-organ autoimmunity, which includes severe enteropathy, T1D, and eczema (9, 56). Beside the severely affected patients, many subjects manifest with a milder form of the disease, which is often misdiagnosed or diagnosed later due to the atypical presentation (57). FOXP3 mutations result in loss of functional Tregs, which is considered the primary cause of disease. FOXP3-mutated Tregs display defective in vitro suppressive function (58–60) and unstable behavior in inflammatory conditions, with conversion from a regulatory to an effector (i.e., IL-17-producing) phenotype (61). Defects in peripheral cells other than Tregs have also been described, e.g., conventional T cells (58, 61–63) and B cells (64). Those additional defects are likely to be an indirect consequence of Treg dysfunction, rather than a direct effect of the mutations, thus suggesting that therapies aimed at improving/restoring a functional Treg compartment should be beneficial to IPEX patients.
The treatment of IPEX syndrome currently relies on supportive therapy, immunosuppression, and HSCT. Allogenic HSCT has proven curative (9), but for patients who do not undergo HSCT the treatment is limited to nutritional support, replacement therapy for endocrine organ failure, and to multiple immunosuppressive drugs, with incomplete control of autoimmunity and burdensome side effects in young patients. Therefore, a therapy aimed at restoring Treg functions represents an unmet medical need. Furthermore, experimental evidence in scurfy mice, the murine model of FOXP3-deficiency, shows that adoptive Treg transfer improves lifespan (65). On the same line, experience from transplanted patients with partial donor chimerism (66–68) and the presence of a fully wild-type Treg compartment in healthy carriers of FOXP3 mutations (69) supports the idea that few functional Tregs are sufficient to control disease progression and induce remission.
The latter evidences convinced us that restoration of a functional Treg compartment in IPEX patients is a therapeutic option. We therefore designed an approach to genetically modify autologous T cells for adoptive transfer in these patients.
The genetic reprogramming of mammalian cells for clinical purposes has recently become an available option, with the completion of clinical trials for the treatment of genetic diseases (70–73) and cancer (74, 75) and their translation in market-authorized therapies (76). Gene-transfer technology has been applied also to the field of Treg-based cell therapy, with the aim of generating high numbers of functional Tregs. Ectopic overexpression of FOXP3 in conventional CD4+ T cells from healthy donors (3, 77–79), ectopic expression of T cell receptors (TCRs) with known specificity in polyclonal Tregs (80–82), and the use of chimeric antigen receptors (CARs) (83–86) are the approaches so far tested in preclinical settings (Table 1). While the former approach would maintain the Ag specificity of the starting population, the latter would redirect Treg specificity. In preclinical studies, expression of TCRs specific for tumor-Ags/allergens conferred human Tregs the ability to suppress Ag-specific responses (80, 81). More recently, Tregs-expressing CARs specific for HLA-Ags have proven effective in inhibiting xenogeneic GvHD and allograft rejection in preclinical models (84–86).
With the ultimate goal of controlling the devastating autoimmunity resulting from mutations of FOXP3 in IPEX syndrome, we envisaged the possibility of performing adoptive transfer of functional autologous Tregs generated in vitro. To this aim, the human FOXP3 coding sequence was cloned under the control of a constitutive promoter in a bidirectional lentiviral vector (LV) construct (88) allowing simultaneous expression of full-length FOXP3 and of a cell-surface marker (ΔNGFR) for the identification/selection of transduced T cells (79) (LV-FOXP3) (Figure 1A). Transduction of peripheral CD4+ T lymphocytes with LV-FOXP3 and in vitro expansion of transduced cells lead to the generation of a homogeneous pool of T cells constitutively overexpressing FOXP3 (Figure 1B). The resulting CD4FOXP3 T cells behave as functional and stable FOXP3+-Treg-like cells, with potent in vitro suppressive activity, reduced proliferative capacity, and limited cytokine production (79, 87). CD4FOXP3 T cells stably express FOXP3 in steady-state and inflammatory conditions, especially when generated from naïve T cells, and maintain inhibitory functions in vivo in a model of xenogeneic GvHD (87). Furthermore, we demonstrated that fully functional CD4FOXP3 T cells can be generated from T cells of IPEX patients (87), regardless of the underlying FOXP3 mutation and co-expression of mutated protein, thus demonstrating the feasibility of our approach and paving the way for the development of alternative therapies based on the adoptive transfer of autologous genetically modified Treg-like cells for the control of autoimmunity in IPEX syndrome.
Figure 1. (A) Schematic representation of control and FOXP3-expressing lentiviral vector constructs. (B) Protocol for the generation of CD4FOXP3 Treg-like cells and control transduced T cells (CD4NGFR) from conventional CD4+ T cells (either naïve or total CD4+ T cells). MOI, multiplicity of infection; FOXP3, forkhead-box-P3; Treg, regulatory T cell.
The fact that CD4FOXP3 T cells can be obtained from CD4+ T cells renders the manufacturing process easy and cost-effective. CD4FOXP3 T cells do not require extensive in vitro expansion with high cytokine concentration. The current preclinical small-scale method for the generation of CD4FOXP3 T cells leads up to 10-fold expansion in 3/4-week culture. This guarantees the feasibility of the production for infusion into patients, taking into consideration that the starting conventional CD4+ T cells can be available in large numbers. In addition, the clinical use of LV platforms does not pose a limitation, since it has proven to be safe in cancer patients and pediatric patients who received HSC gene therapy (72, 73, 89, 90).
Although in principle, the use of CAR-Tregs or TCR-transgenic Tregs would allow the generation of Ag-specific Tregs suitable for the treatment of autoimmune diseases, the Ag target of the autoimmune damage is still unknown in many diseases. The fact that CD4FOXP3 originate from polyclonal CD4+ T cells may constitute an advantage for such diseases. Indeed, the CD4+ T cells obtained from a patient suffering with autoimmunity would most likely comprise the pathogenic T cells with TCRs specific for the target Ags. Therefore, in specific disease context, CD4FOXP3 cells may find a broader and more effective use, as compared with the TCR-transgenic-/CAR-Tregs.
Despite their promising results, the initial trials of Treg-based cell therapy raised some concern on issues related to FOXP3+-Treg biology. Due to their intrinsic anergic and terminally differentiated phenotype, one open issue is the in vivo lifespan of the infused product. Initial data on in vivo infused Tregs showed 2-week survival post-injection (49). We obtained similar results when CD4FOXP3 T cells were injected in immune-deficient mice (87). Surprisingly, data from a Treg-cell therapy trial in T1D patients demonstrated that, although the majority of ex vivo expanded autologous Tregs persists for 2 weeks post-infusion, a fraction of the injected cells is detectable after 1 year, suggesting that Tregs might contribute to tolerance maintenance long term (43).
Several evidences demonstrated that FOXP3+-Tregs are intrinsically plastic and that under inflammatory conditions Tregs can downmodulate FOXP3 and secrete pro-inflammatory cytokines (91–93). Therefore, the risk of loss of regulatory functions by infused Tregs could be worrisome. To address this issue, culture with rapamycin, to favor the generation of stable Treg products, has been developed (94–96). In this context, CD4FOXP3 T cells represent the ideal Treg product: constant FOXP3 expression is warranted by a constitutive promoter-driven transcription, and stability has been demonstrated in steady-state and inflammatory conditions, both in vitro and in vivo (87). Stability is especially maintained when CD4FOXP3 T cells are generated from naïve T cells. In the case of memory-derived CD4FOXP3 T cells, FOXP3 expression appeared slightly reduced with inflammatory cytokines, resulting in weaker suppressive function and increased proliferation, as compared with naïve T cell-derived products (87), most likely due to posttranscriptional regulatory mechanisms.
Finally, the possibility of a generalized effect of immunosuppression that injection of suppressor cells may cause, as well as concerns about the dose required for injection of polyclonal Tregs has prompted investigators to design more targeted therapies. Methods to expand human Ag-specific Tregs have been developed (42, 97, 98). These protocols well apply to allo-Ag-specific Tregs. Of note, encouraging safety and efficacy results come from a recently published Treg-based cell therapy trial, in which Tregs induced in the presence of donor-irradiated PBMCs were infused after liver transplantation. Despite low doses of Tregs, in 7/10 patients, immunosuppression was stopped, and operational tolerance to the graft was induced (99). Currently, ongoing trials in solid organ transplantation, which foresee the injection of donor-specific Tregs, will lead to further progresses (47).
We believe that in the case of IPEX syndrome and diseases with multiple autoimmune manifestations, the need for Ag specificity is unlikely to be necessary. The use of patients’ Teff cells as source of CD4FOXP3 cells will potentially allow the generation of Treg-like cells enriched for autoreactive specificities. Still, the infusion of polyclonal potent suppressor cells may result in a generalized effect of immunosuppression, which could potentially interfere with protective responses to common pathogens. Although the results of the clinical trials using polyclonal Tregs were reassuring, we are currently establishing a protocol to generate CD4FOXP3 T cells from Ag-experienced T cells with known specificity, which should restrict their suppressive effect to the target Ag. Briefly, the protocol foresees pre-activation of T cells with a target Ag; Ag-specific T cells activating in response to their cognate Ag will be preferentially transduced. Subsequent in vitro expansion allows generation of a T-cell population enriched of FOXP3-overexpressing cells with known Ag specificity (Passerini and Bacchetta, unpublished results). This method could be used to extend the application of the CD4FOXP3 T-cell product beyond IPEX syndrome, to treat autoimmune/inflammatory diseases with known target Ags or in the context of transplantation tolerance.
Finally, a relevant open issue on the way to the clinical application of CD4FOXP3 T cells is definitely their in vivo lifespan, difficult to assess in preclinical models. Short-lived cells would likely be safer, although they may imply clinical protocols with multiple infusions of the therapeutic product. Long-lived CD4FOXP3 T cells would allow single infusion but would likely require an additional safety layer, such as addition of a suicide gene in the construct used for their generation. The use of a suicide gene may also be considered as a safety measure to contrast the consequences of possible insertional mutagenesis, although it has been demonstrated that the use of LV-mediated gene transfer is not associated with selective integrations near oncogenes (100). However, for any type of genetically modified cellular product, analysis of the integration sites is recommended during preclinical assessments.
Thanks to the successfully completed trials, the use of adoptive Treg-cell therapy to control undesired immune responses has become applicable. The next challenge for researchers is the tailoring of the Treg-based therapy for specific diseases. We envisaged an approach based on the use of FOX3+-Treg-like cells electively designed to restore immune regulation in IPEX syndrome. Once safety and proof-of-concept will be completed in IPEX patients, the use of these autologous Treg-like cells could become the future standard of care for certain autoimmune diseases, akin to how CAR-T cells will become the standard of care in hematologic malignancies.
The authors (LP and RB) equally contributed to discuss the topic and write the manuscript.
The authors declare that the research was conducted in the absence of any commercial or financial relationships that could be construed as a potential conflict of interest.
The authors thank Dr. Silvia Gregori and Francesca Romana Santoni de Sio (Mechanisms of Peripheral Tolerance Unit, SR-TIGET, San Raffaele Scientific Institute, Milan, Italy) for critical reading of this manuscript and continuous helpful scientific discussion. This work was supported by grants to RB from the Italian Telethon Foundation (TGT11A04) and to LP from the Italian Ministry of Health (GR-11-103).
1. Schmetterer KG, Neunkirchner A, Pickl WF. Naturally occurring regulatory T cells: markers, mechanisms, and manipulation. FASEB J (2012) 26(6):2253–76. doi:10.1096/fj.11-193672
2. Sakaguchi S, Sakaguchi N, Asano M, Itoh M, Toda M. Immunologic self-tolerance maintained by activated T cells expressing IL-2 receptor alpha-chains (CD25). Breakdown of a single mechanism of self-tolerance causes various autoimmune diseases. J Immunol (1995) 155(3):1151–64.
3. Yagi H, Nomura T, Nakamura K, Yamazaki S, Kitawaki T, Hori S, et al. Crucial role of FOXP3 in the development and function of human CD25+CD4+ regulatory T cells. Int Immunol (2004) 16(11):1643–56. doi:10.1093/intimm/dxh165
4. Groux H, O’Garra A, Bigler M, Rouleau M, Antonenko S, de Vries JE, et al. A CD4+ T-cell subset inhibits antigen-specific T-cell responses and prevents colitis. Nature (1997) 389(6652):737–42. doi:10.1038/39614
5. Sakaguchi S, Miyara M, Costantino CM, Hafler DA. FOXP3+ regulatory T cells in the human immune system. Nat Rev Immunol (2010) 10(7):490–500. doi:10.1038/nri2785
6. Abbas AK, Benoist C, Bluestone JA, Campbell DJ, Ghosh S, Hori S, et al. Regulatory T cells: recommendations to simplify the nomenclature. Nat Immunol (2013) 14(4):307–8. doi:10.1038/ni.2554
7. Bennett CL, Ochs HD. IPEX is a unique X-linked syndrome characterized by immune dysfunction, polyendocrinopathy, enteropathy, and a variety of autoimmune phenomena. Curr Opin Pediatr (2001) 13(6):533–8. doi:10.1097/00008480-200112000-00007
8. Wildin RS, Ramsdell F, Peake J, Faravelli F, Casanova JL, Buist N, et al. X-linked neonatal diabetes mellitus, enteropathy and endocrinopathy syndrome is the human equivalent of mouse scurfy. Nat Genet (2001) 27(1):18–20. doi:10.1038/83707
9. Barzaghi F, Passerini L, Bacchetta R. Immune dysregulation, polyendocrinopathy, enteropathy, x-linked syndrome: a paradigm of immunodeficiency with autoimmunity. Front Immunol (2012) 3:211. doi:10.3389/fimmu.2012.00211
10. Bacchetta R, Barzaghi F, Roncarolo MG. From IPEX syndrome to FOXP3 mutation: a lesson on immune dysregulation. Ann N Y Acad Sci (2016). doi:10.1111/nyas.13011
11. Ohkura N, Hamaguchi M, Morikawa H, Sugimura K, Tanaka A, Ito Y, et al. T cell receptor stimulation-induced epigenetic changes and Foxp3 expression are independent and complementary events required for Treg cell development. Immunity (2012) 37(5):785–99. doi:10.1016/j.immuni.2012.09.010
12. Hill JA, Feuerer M, Tash K, Haxhinasto S, Perez J, Melamed R, et al. Foxp3 transcription-factor-dependent and -independent regulation of the regulatory T cell transcriptional signature. Immunity (2007) 27(5):786–800. doi:10.1016/j.immuni.2007.09.010
13. Fu W, Ergun A, Lu T, Hill JA, Haxhinasto S, Fassett MS, et al. A multiply redundant genetic switch ‘locks in’ the transcriptional signature of regulatory T cells. Nat Immunol (2012) 13(10):972–80. doi:10.1038/ni.2420
14. Passerini L, Santoni de Sio FR, Roncarolo MG, Bacchetta R. Forkhead box P3: the peacekeeper of the immune system. Int Rev Immunol (2014) 33(2):129–45. doi:10.3109/08830185.2013.863303
15. Liu W, Putnam AL, Xu-Yu Z, Szot GL, Lee MR, Zhu S, et al. CD127 expression inversely correlates with FoxP3 and suppressive function of human CD4+ T reg cells. J Exp Med (2006) 203(7):1701–11. doi:10.1084/jem.20060772
16. Takahashi T, Tagami T, Yamazaki S, Uede T, Shimizu J, Sakaguchi N, et al. Immunologic self-tolerance maintained by CD25(+)CD4(+) regulatory T cells constitutively expressing cytotoxic T lymphocyte-associated antigen 4. J Exp Med (2000) 192(2):303–10. doi:10.1084/jem.192.2.303
17. McHugh RS, Whitters MJ, Piccirillo CA, Young DA, Shevach EM, Collins M, et al. CD4(+)CD25(+) immunoregulatory T cells: gene expression analysis reveals a functional role for the glucocorticoid-induced TNF receptor. Immunity (2002) 16(2):311–23. doi:10.1016/S1074-7613(02)00280-7
18. Borsellino G, Kleinewietfeld M, Di Mitri D, Sternjak A, Diamantini A, Giometto R, et al. Expression of ectonucleotidase CD39 by Foxp3+ Treg cells: hydrolysis of extracellular ATP and immune suppression. Blood (2007) 110(4):1225–32. doi:10.1182/blood-2006-12-064527
19. Kubach J, Lutter P, Bopp T, Stoll S, Becker C, Huter E, et al. Human CD4+CD25+ regulatory T cells: proteome analysis identifies galectin-10 as a novel marker essential for their anergy and suppressive function. Blood (2007) 110(5):1550–8. doi:10.1182/blood-2007-01-069229
20. Tran DQ, Andersson J, Hardwick D, Bebris L, Illei GG, Shevach EM. Selective expression of latency-associated peptide (LAP) and IL-1 receptor type I/II (CD121a/CD121b) on activated human FOXP3+ regulatory T cells allows for their purification from expansion cultures. Blood (2009) 113(21):5125–33. doi:10.1182/blood-2009-01-199950
21. Thornton AM, Korty PE, Tran DQ, Wohlfert EA, Murray PE, Belkaid Y, et al. Expression of Helios, an Ikaros transcription factor family member, differentiates thymic-derived from peripherally induced Foxp3+ T regulatory cells. J Immunol (2010) 184(7):3433–41. doi:10.4049/jimmunol.0904028
22. Joller N, Lozano E, Burkett PR, Patel B, Xiao S, Zhu C, et al. Treg cells expressing the coinhibitory molecule TIGIT selectively inhibit proinflammatory Th1 and Th17 cell responses. Immunity (2014) 40(4):569–81. doi:10.1016/j.immuni.2014.02.012
23. Tran DQ, Andersson J, Wang R, Ramsey H, Unutmaz D, Shevach EM. GARP (LRRC32) is essential for the surface expression of latent TGF-beta on platelets and activated FOXP3+ regulatory T cells. Proc Natl Acad Sci U S A (2009) 106(32):13445–50. doi:10.1073/pnas.0901944106
24. Wieczorek G, Asemissen A, Model F, Turbachova I, Floess S, Liebenberg V, et al. Quantitative DNA methylation analysis of FOXP3 as a new method for counting regulatory T cells in peripheral blood and solid tissue. Cancer Res (2009) 69(2):599–608. doi:10.1158/0008-5472.CAN-08-2361
25. Wing K, Onishi Y, Prieto-Martin P, Yamaguchi T, Miyara M, Fehervari Z, et al. CTLA-4 control over Foxp3+ regulatory T cell function. Science (2008) 322(5899):271–5. doi:10.1126/science.1160062
26. Grossman WJ, Verbsky JW, Barchet W, Colonna M, Atkinson JP, Ley TJ. Human T regulatory cells can use the perforin pathway to cause autologous target cell death. Immunity (2004) 21(4):589–601. doi:10.1016/j.immuni.2004.09.002
27. Yamaguchi T, Wing JB, Sakaguchi S. Two modes of immune suppression by Foxp3(+) regulatory T cells under inflammatory or non-inflammatory conditions. Semin Immunol (2011) 23(6):424–30. doi:10.1016/j.smim.2011.10.002
28. Ito T, Hanabuchi S, Wang YH, Park WR, Arima K, Bover L, et al. Two functional subsets of FOXP3+ regulatory T cells in human thymus and periphery. Immunity (2008) 28(6):870–80. doi:10.1016/j.immuni.2008.03.018
29. Nakamura K, Kitani A, Fuss I, Pedersen A, Harada N, Nawata H, et al. TGF-beta 1 plays an important role in the mechanism of CD4+CD25+ regulatory T cell activity in both humans and mice. J Immunol (2004) 172(2):834–42. doi:10.4049/jimmunol.172.2.834
30. Stockis J, Colau D, Coulie PG, Lucas S. Membrane protein GARP is a receptor for latent TGF-beta on the surface of activated human Treg. Eur J Immunol (2009) 39(12):3315–22. doi:10.1002/eji.200939684
31. Collison LW, Workman CJ, Kuo TT, Boyd K, Wang Y, Vignali KM, et al. The inhibitory cytokine IL-35 contributes to regulatory T-cell function. Nature (2007) 450(7169):566–9. doi:10.1038/nature06306
32. Pandiyan P, Zheng L, Ishihara S, Reed J, Lenardo MJ. CD4+CD25+Foxp3+ regulatory T cells induce cytokine deprivation-mediated apoptosis of effector CD4+ T cells. Nat Immunol (2007) 8(12):1353–62. doi:10.1038/ni1536
33. Deaglio S, Dwyer KM, Gao W, Friedman D, Usheva A, Erat A, et al. Adenosine generation catalyzed by CD39 and CD73 expressed on regulatory T cells mediates immune suppression. J Exp Med (2007) 204(6):1257–65. doi:10.1084/jem.20062512
34. Bopp T, Becker C, Klein M, Klein-Hessling S, Palmetshofer A, Serfling E, et al. Cyclic adenosine monophosphate is a key component of regulatory T cell-mediated suppression. J Exp Med (2007) 204(6):1303–10. doi:10.1084/jem.20062129
35. Baecher-Allan C, Wolf E, Hafler DA. MHC class II expression identifies functionally distinct human regulatory T cells. J Immunol (2006) 176(8):4622–31. doi:10.4049/jimmunol.176.8.4622
36. Beriou G, Costantino CM, Ashley CW, Yang L, Kuchroo VK, Baecher-Allan C, et al. IL-17-producing human peripheral regulatory T cells retain suppressive function. Blood (2009) 113(18):4240–9. doi:10.1182/blood-2008-10-183251
37. Miyara M, Yoshioka Y, Kitoh A, Shima T, Wing K, Niwa A, et al. Functional delineation and differentiation dynamics of human CD4+ T cells expressing the FoxP3 transcription factor. Immunity (2009) 30(6):899–911. doi:10.1016/j.immuni.2009.03.019
38. Zheng Y, Chaudhry A, Kas A, deRoos P, Kim JM, Chu TT, et al. Regulatory T-cell suppressor program co-opts transcription factor IRF4 to control T(H)2 responses. Nature (2009) 458(7236):351–6. doi:10.1038/nature07674
39. Kordasti S, Costantini B, Seidl T, Perez Abellan P, Martinez Llordella M, McLornan D, et al. Deep phenotyping of Tregs identifies an immune signature for idiopathic aplastic anemia and predicts response to treatment. Blood (2016) 128(9):1193–205. doi:10.1182/blood-2016-03-703702
40. Roncarolo MG, Battaglia M. Regulatory T-cell immunotherapy for tolerance to self antigens and alloantigens in humans. Nat Rev Immunol (2007) 7(8):585–98. doi:10.1038/nri2138
41. Tang Q, Bluestone JA, Kang SM. CD4(+)Foxp3(+) regulatory T cell therapy in transplantation. J Mol Cell Biol (2012) 4(1):11–21. doi:10.1093/jmcb/mjr047
42. Putnam AL, Safinia N, Medvec A, Laszkowska M, Wray M, Mintz MA, et al. Clinical grade manufacturing of human alloantigen-reactive regulatory T cells for use in transplantation. Am J Transplant (2013) 13(11):3010–20. doi:10.1111/ajt.12433
43. Bluestone JA, Buckner JH, Fitch M, Gitelman SE, Gupta S, Hellerstein MK, et al. Type 1 diabetes immunotherapy using polyclonal regulatory T cells. Sci Transl Med (2015) 7(315):315ra189. doi:10.1126/scitranslmed.aad4134
44. Safinia N, Vaikunthanathan T, Fraser H, Thirkell S, Lowe K, Blackmore L, et al. Successful expansion of functional and stable regulatory T cells for immunotherapy in liver transplantation. Oncotarget (2016) 7(7):7563–77. doi:10.18632/oncotarget.6927
45. McKenna DH Jr, Sumstad D, Kadidlo DM, Batdorf B, Lord CJ, Merkel SC, et al. Optimization of cGMP purification and expansion of umbilical cord blood-derived T-regulatory cells in support of first-in-human clinical trials. Cytotherapy (2017) 19(2):250–62. doi:10.1016/j.jcyt.2016.10.011
46. Gitelman SE, Bluestone JA. Regulatory T cell therapy for type 1 diabetes: may the force be with you. J Autoimmun (2016) 71:78–87. doi:10.1016/j.jaut.2016.03.011
47. Vaikunthanathan T, Safinia N, Boardman D, Lechler RI, Lombardi G. Regulatory T cells: tolerance induction in solid organ transplantation. Clin Exp Immunol (2017) 189(2):197–210. doi:10.1111/cei.12978
48. Trzonkowski P, Bieniaszewska M, Juscinska J, Dobyszuk A, Krzystyniak A, Marek N, et al. First-in-man clinical results of the treatment of patients with graft versus host disease with human ex vivo expanded CD4+CD25+CD127- T regulatory cells. Clin Immunol (2009) 133(1):22–6. doi:10.1016/j.clim.2009.06.001
49. Brunstein CG, Miller JS, Cao Q, McKenna DH, Hippen KL, Curtsinger J, et al. Infusion of ex vivo expanded T regulatory cells in adults transplanted with umbilical cord blood: safety profile and detection kinetics. Blood (2011) 117(3):1061–70. doi:10.1182/blood-2010-07-293795
50. Di Ianni M, Falzetti F, Carotti A, Terenzi A, Castellino F, Bonifacio E, et al. Tregs prevent GVHD and promote immune reconstitution in HLA-haploidentical transplantation. Blood (2011) 117(14):3921–8. doi:10.1182/blood-2010-10-311894
51. Edinger M, Hoffmann P. Regulatory T cells in stem cell transplantation: strategies and first clinical experiences. Curr Opin Immunol (2011) 23(5):679–84. doi:10.1016/j.coi.2011.06.006
52. Brunstein CG, Miller JS, McKenna DH, Hippen KL, DeFor TE, Sumstad D, et al. Umbilical cord blood-derived T regulatory cells to prevent GVHD: kinetics, toxicity profile, and clinical effect. Blood (2016) 127(8):1044–51. doi:10.1182/blood-2015-06-653667
53. Martelli MF, Di Ianni M, Ruggeri L, Falzetti F, Carotti A, Terenzi A, et al. HLA-haploidentical transplantation with regulatory and conventional T cell adoptive immunotherapy prevents acute leukemia relapse. Blood (2014) 124:638–44. doi:10.1182/blood-2014-03-564401
54. Leslie M. Immunology. Regulatory T cells get their chance to shine. Science (2011) 332(6033):1020–1. doi:10.1126/science.332.6033.1020
55. Marek-Trzonkowska N, Mysliwiec M, Dobyszuk A, Grabowska M, Techmanska I, Juscinska J, et al. Administration of CD4+CD25highCD127- regulatory T cells preserves beta-cell function in type 1 diabetes in children. Diabetes Care (2012) 35(9):1817–20. doi:10.2337/dc12-0038
56. Ochs HD, Gambineri E, Torgerson TR. IPEX, FOXP3 and regulatory T-cells: a model for autoimmunity. Immunol Res (2007) 38(1–3):112–21. doi:10.1007/s12026-007-0022-2
57. Bin Dhuban K, Piccirillo CA. The immunological and genetic basis of immune dysregulation, polyendocrinopathy, enteropathy, X-linked syndrome. Curr Opin Allergy Clin Immunol (2015) 15(6):525–32. doi:10.1097/ACI.0000000000000214
58. Bacchetta R, Passerini L, Gambineri E, Dai M, Allan SE, Perroni L, et al. Defective regulatory and effector T cell functions in patients with FOXP3 mutations. J Clin Invest (2006) 116(6):1713–22. doi:10.1172/JCI25112
59. d’Hennezel E, Ben-Shoshan M, Ochs HD, Torgerson TR, Russell LJ, Lejtenyi C, et al. FOXP3 forkhead domain mutation and regulatory T cells in the IPEX syndrome. N Engl J Med (2009) 361(17):1710–3. doi:10.1056/NEJMc0907093
60. Moes N, Rieux-Laucat F, Begue B, Verdier J, Neven B, Patey N, et al. Reduced expression of FOXP3 and regulatory T-cell function in severe forms of early-onset autoimmune enteropathy. Gastroenterology (2010) 139(3):770–8. doi:10.1053/j.gastro.2010.06.006
61. Passerini L, Olek S, Di Nunzio S, Barzaghi F, Hambleton S, Abinun M, et al. Forkhead box protein 3 (FOXP3) mutations lead to increased TH17 cell numbers and regulatory T-cell instability. J Allergy Clin Immunol (2011) 128(6):1376–9.e1. doi:10.1016/j.jaci.2011.09.010
62. Chatila TA, Blaeser F, Ho N, Lederman HM, Voulgaropoulos C, Helms C, et al. JM2, encoding a fork head-related protein, is mutated in X-linked autoimmunity-allergic disregulation syndrome. J Clin Invest (2000) 106(12):R75–81. doi:10.1172/JCI11679
63. Nieves DS, Phipps RP, Pollock SJ, Ochs HD, Zhu Q, Scott GA, et al. Dermatologic and immunologic findings in the immune dysregulation, polyendocrinopathy, enteropathy, X-linked syndrome. Arch Dermatol (2004) 140(4):466–72. doi:10.1001/archderm.140.4.466
64. Kinnunen T, Chamberlain N, Morbach H, Choi J, Kim S, Craft J, et al. Accumulation of peripheral autoreactive B cells in the absence of functional human regulatory T cells. Blood (2013) 121(9):1595–603. doi:10.1182/blood-2012-09-457465
65. Smyk-Pearson SK, Bakke AC, Held PK, Wildin RS. Rescue of the autoimmune scurfy mouse by partial bone marrow transplantation or by injection with T-enriched splenocytes. Clin Exp Immunol (2003) 133(2):193–9. doi:10.1046/j.1365-2249.2003.02217.x
66. Seidel MG, Fritsch G, Lion T, Jurgens B, Heitger A, Bacchetta R, et al. Selective engraftment of donor CD4+25high FOXP3-positive T cells in IPEX syndrome after nonmyeloablative hematopoietic stem cell transplantation. Blood (2009) 113(22):5689–91. doi:10.1182/blood-2009-02-206359
67. Kasow KA, Morales-Tirado VM, Wichlan D, Shurtleff SA, Abraham A, Persons DA, et al. Therapeutic in vivo selection of thymic-derived natural T regulatory cells following non-myeloablative hematopoietic stem cell transplant for IPEX. Clin Immunol (2011) 141(2):169–76. doi:10.1016/j.clim.2011.07.005
68. Horino S, Sasahara Y, Sato M, Niizuma H, Kumaki S, Abukawa D, et al. Selective expansion of donor-derived regulatory T cells after allogeneic bone marrow transplantation in a patient with IPEX syndrome. Pediatr Transplant (2014) 18(1):E25–30. doi:10.1111/petr.12184
69. Di Nunzio S, Cecconi M, Passerini L, McMurchy AN, Baron U, Turbachova I, et al. Wild-type FOXP3 is selectively active in CD4+CD25(hi) regulatory T cells of healthy female carriers of different FOXP3 mutations. Blood (2009) 114(19):4138–41. doi:10.1182/blood-2009-04-214593
70. Aiuti A, Cattaneo F, Galimberti S, Benninghoff U, Cassani B, Callegaro L, et al. Gene therapy for immunodeficiency due to adenosine deaminase deficiency. N Engl J Med (2009) 360(5):447–58. doi:10.1056/NEJMoa0805817
71. Gaspar HB, Aiuti A, Porta F, Candotti F, Hershfield MS, Notarangelo LD. How I treat ADA deficiency. Blood (2009) 114(17):3524–32. doi:10.1182/blood-2009-06-189209
72. Aiuti A, Biasco L, Scaramuzza S, Ferrua F, Cicalese MP, Baricordi C, et al. Lentiviral hematopoietic stem cell gene therapy in patients with Wiskott-Aldrich syndrome. Science (2013) 341(6148):1233151. doi:10.1126/science.1233151
73. Biffi A, Montini E, Lorioli L, Cesani M, Fumagalli F, Plati T, et al. Lentiviral hematopoietic stem cell gene therapy benefits metachromatic leukodystrophy. Science (2013) 341(6148):1233158. doi:10.1126/science.1233158
74. Ciceri F, Bonini C, Marktel S, Zappone E, Servida P, Bernardi M, et al. Antitumor effects of HSV-TK-engineered donor lymphocytes after allogeneic stem-cell transplantation. Blood (2007) 109(11):4698–707. doi:10.1182/blood-2006-05-023416
75. Kaufmann KB, Buning H, Galy A, Schambach A, Grez M. Gene therapy on the move. EMBO Mol Med (2013) 5(11):1642–61. doi:10.1002/emmm.201202287
76. Monaco L, Faccio L. Patient-driven search for rare disease therapies: the Fondazione Telethon success story and the strategy leading to Strimvelis. EMBO Mol Med (2017) 9(3):289–92. doi:10.15252/emmm.201607293
77. Oswald-Richter K, Grill SM, Shariat N, Leelawong M, Sundrud MS, Haas DW, et al. HIV infection of naturally occurring and genetically reprogrammed human regulatory T-cells. PLoS Biol (2004) 2(7):E198. doi:10.1371/journal.pbio.0020198
78. Aarts-Riemens T, Emmelot ME, Verdonck LF, Mutis T. Forced overexpression of either of the two common human Foxp3 isoforms can induce regulatory T cells from CD4(+)CD25(-) cells. Eur J Immunol (2008) 38(5):1381–90. doi:10.1002/eji.200737590
79. Allan SE, Alstad AN, Merindol N, Crellin NK, Amendola M, Bacchetta R, et al. Generation of potent and stable human CD4+ T regulatory cells by activation-independent expression of FOXP3. Mol Ther (2008) 16(1):194–202. doi:10.1038/sj.mt.6300341
80. Brusko TM, Koya RC, Zhu S, Lee MR, Putnam AL, McClymont SA, et al. Human antigen-specific regulatory T cells generated by T cell receptor gene transfer. PLoS One (2010) 5(7):e11726. doi:10.1371/journal.pone.0011726
81. Schmetterer KG, Haiderer D, Leb-Reichl VM, Neunkirchner A, Jahn-Schmid B, Kung HJ, et al. Bet v 1-specific T-cell receptor/forkhead box protein 3 transgenic T cells suppress Bet v 1-specific T-cell effector function in an activation-dependent manner. J Allergy Clin Immunol (2011) 127(1):238–45, 245.e231–3. doi:10.1016/j.jaci.2010.10.023
82. Hull CM, Nickolay LE, Estorninho M, Richardson MW, Riley JL, Peakman M, et al. Generation of human islet-specific regulatory T cells by TCR gene transfer. J Autoimmun (2017) 79:63–73. doi:10.1016/j.jaut.2017.01.001
83. Hombach AA, Kofler D, Rappl G, Abken H. Redirecting human CD4+CD25+ regulatory T cells from the peripheral blood with pre-defined target specificity. Gene Ther (2009) 16(9):1088–96. doi:10.1038/gt.2009.75
84. MacDonald KG, Hoeppli RE, Huang Q, Gillies J, Luciani DS, Orban PC, et al. Alloantigen-specific regulatory T cells generated with a chimeric antigen receptor. J Clin Invest (2016) 126(4):1413–24. doi:10.1172/JCI82771
85. Boardman DA, Philippeos C, Fruhwirth GO, Ibrahim MA, Hannen RF, Cooper D, et al. Expression of a chimeric antigen receptor specific for donor HLA class I enhances the potency of human regulatory T cells in preventing human skin transplant rejection. Am J Transplant (2017) 17(4):931–43. doi:10.1111/ajt.14185
86. Noyan F, Zimmermann K, Hardtke-Wolenski M, Knoefel A, Schulde E, Geffers R, et al. Prevention of allograft rejection by use of regulatory T cells with an MHC-specific chimeric antigen receptor. Am J Transplant (2017) 17(4):917–30. doi:10.1111/ajt.14175
87. Passerini L, Rossi Mel E, Sartirana C, Fousteri G, Bondanza A, Naldini L, et al. CD4(+) T cells from IPEX patients convert into functional and stable regulatory T cells by FOXP3 gene transfer. Sci Transl Med (2013) 5(215):215ra174. doi:10.1126/scitranslmed.3007320
88. Amendola M, Venneri MA, Biffi A, Vigna E, Naldini L. Coordinate dual-gene transgenesis by lentiviral vectors carrying synthetic bidirectional promoters. Nat Biotechnol (2005) 23(1):108–16. doi:10.1038/nbt1049
89. Bonini C, Ciceri F, Marktel S, Bordignon C. Suicide-gene-transduced T-cells for the regulation of the graft-versus-leukemia effect. Vox Sang (1998) 74(Suppl 2):341–3. doi:10.1111/j.1423-0410.1998.tb05440.x
90. Sessa M, Lorioli L, Fumagalli F, Acquati S, Redaelli D, Baldoli C, et al. Lentiviral haemopoietic stem-cell gene therapy in early-onset metachromatic leukodystrophy: an ad-hoc analysis of a non-randomised, open-label, phase 1/2 trial. Lancet (2016) 388(10043):476–87. doi:10.1016/S0140-6736(16)30374-9
91. Zhou X, Bailey-Bucktrout S, Jeker LT, Bluestone JA. Plasticity of CD4(+) FoxP3(+) T cells. Curr Opin Immunol (2009) 21(3):281–5. doi:10.1016/j.coi.2009.05.007
92. da Silva Martins M, Piccirillo CA. Functional stability of Foxp3+ regulatory T cells. Trends Mol Med (2012) 18(8):454–62. doi:10.1016/j.molmed.2012.06.001
93. MacDonald KG, Orban PC, Levings MK. T regulatory cell therapy in transplantation: stability, localization and functional specialization. Curr Opin Organ Transplant (2012) 17(4):343–8. doi:10.1097/MOT.0b013e328355aaaf
94. Battaglia M, Stabilini A, Roncarolo MG. Rapamycin selectively expands CD4+CD25+FoxP3+ regulatory T cells. Blood (2005) 105(12):4743–8. doi:10.1182/blood-2004-10-3932
95. Battaglia M, Stabilini A, Migliavacca B, Horejs-Hoeck J, Kaupper T, Roncarolo MG. Rapamycin promotes expansion of functional CD4+CD25+FOXP3+ regulatory T cells of both healthy subjects and type 1 diabetic patients. J Immunol (2006) 177(12):8338–47. doi:10.4049/jimmunol.177.12.8338
96. Hoffmann P, Eder R, Boeld TJ, Doser K, Piseshka B, Andreesen R, et al. Only the CD45RA+ subpopulation of CD4+CD25high T cells gives rise to homogeneous regulatory T-cell lines upon in vitro expansion. Blood (2006) 108(13):4260–7. doi:10.1182/blood-2006-06-027409
97. Sagoo P, Ali N, Garg G, Nestle FO, Lechler RI, Lombardi G. Human regulatory T cells with alloantigen specificity are more potent inhibitors of alloimmune skin graft damage than polyclonal regulatory T cells. Sci Transl Med (2011) 3(83):83ra42. doi:10.1126/scitranslmed.3002076
98. Noyan F, Lee YS, Zimmermann K, Hardtke-Wolenski M, Taubert R, Warnecke G, et al. Isolation of human antigen-specific regulatory T cells with high suppressive function. Eur J Immunol (2014) 44(9):2592–602. doi:10.1002/eji.201344381
99. Todo S, Yamashita K, Goto R, Zaitsu M, Nagatsu A, Oura T, et al. A pilot study of operational tolerance with a regulatory T-cell-based cell therapy in living donor liver transplantation. Hepatology (2016) 64(2):632–43. doi:10.1002/hep.28459
Keywords: regulatory T cells, forkhead box P3, tolerance, regulatory T cell-based cell therapy, gene transfer, antigen specificity, autoimmunity, immune dysregulation
Citation: Passerini L and Bacchetta R (2017) Forkhead-Box-P3 Gene Transfer in Human CD4+ T Conventional Cells for the Generation of Stable and Efficient Regulatory T Cells, Suitable for Immune Modulatory Therapy. Front. Immunol. 8:1282. doi: 10.3389/fimmu.2017.01282
Received: 02 August 2017; Accepted: 25 September 2017;
Published: 12 October 2017
Edited by:
Christine Happle, Hannover Medical School, GermanyReviewed by:
Lianjun Zhang, University of Lausanne, SwitzerlandCopyright: © 2017 Passerini and Bacchetta. This is an open-access article distributed under the terms of the Creative Commons Attribution License (CC BY). The use, distribution or reproduction in other forums is permitted, provided the original author(s) or licensor are credited and that the original publication in this journal is cited, in accordance with accepted academic practice. No use, distribution or reproduction is permitted which does not comply with these terms.
*Correspondence: Rosa Bacchetta, cm9zYWJAc3RhbmZvcmQuZWR1
Disclaimer: All claims expressed in this article are solely those of the authors and do not necessarily represent those of their affiliated organizations, or those of the publisher, the editors and the reviewers. Any product that may be evaluated in this article or claim that may be made by its manufacturer is not guaranteed or endorsed by the publisher.
Research integrity at Frontiers
Learn more about the work of our research integrity team to safeguard the quality of each article we publish.