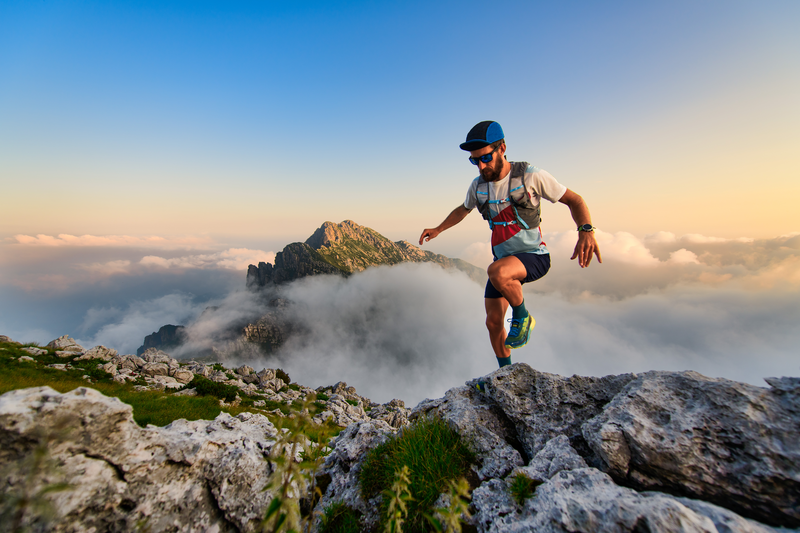
95% of researchers rate our articles as excellent or good
Learn more about the work of our research integrity team to safeguard the quality of each article we publish.
Find out more
MINI REVIEW article
Front. Immunol. , 28 September 2017
Sec. Inflammation
Volume 8 - 2017 | https://doi.org/10.3389/fimmu.2017.01224
This article is part of the Research Topic Sexual Dimorphism of the Immune Inflammatory Response in Infectious and Non-infectious Diseases View all 9 articles
The human immunodeficiency virus (HIV)-1 epidemic continues to represent a global health problem that is over-proportionally affecting women from sub-Saharan Africa. Besides social and environmental factors, the modulation of immunological pathways by sex hormones and gene dosage effects of X chromosomal-encoded genes have been suggested to lead to differential outcomes in HIV-1 disease. Women present with lower HIV-1 loads early in infection. However, the progression to AIDS for the same level of viremia is faster in women than in men. Type I interferons (IFNs) play a prominent role in the control of HIV-1 transmission and replication. Continuous stimulation of type I IFNs in chronic viral infections can lead to increased levels of immune activation, which can be higher in HIV-1-infected women than in men. A role of steroid hormone signaling in regulating viral replication has been postulated, which might further account for sex differences observed in HIV-1 infections. Here, we review recent findings and current knowledge on sex-specific differences in HIV-1 infections.
Infections with human immunodeficiency virus (HIV)-1 still represent a global health problem with especially women and girls in sub-Saharan Africa being severely affected (1). The prevalence of HIV-1-infected girls and young women is twice as high as in men of same ages (2). While the overall survival does not differ between infected males and females (3, 4), women are at increased risk to acquire HIV-1 via heterosexual contact (5). It is now well established that sex-related biological factors affect HIV-1 disease manifestations and progression. During acute infection, HIV-1-infected females have lower HIV-1 viral load levels in comparison to men (6–9). Plasma viral load levels predict the size of the HIV-1 reservoir (10, 11), hence lower viral load levels in HIV-1-infected females might contribute to a smaller size of the HIV-1 DNA reservoir (12, 13). Type I interferons (IFNs) play a critical role in restricting viral replication through induction of host restriction factors and might contribute to this initial control of HIV-1 viremia. On the other hand, type I IFNs have been shown to increase systemic immune activation upon chronic viral infection (14, 15).
Several factors have been suggested to influence the sex bias observed in the manifestations of HIV-1 infections, including sex and gender differences in comorbidities as well as socioeconomic factors (16, 17). Dissecting biological mechanisms from socioeconomic factors represents a frequently challenging process. The elucidation of the biological mechanisms that underlie immunological differences between the sexes has become an emerging area of interest aimed at understanding the involvement of steroid hormones (18–20), the direct effects of X and Y chromosomal-linked factors (21–23) or epigenetic modifications that influence the composition and phenotype of immune cells, and the role of the microbiome (24). Recent reviews have already covered several aspects of sex-based differences in HIV-1 infection (16, 25–27). This review will focus on the latest findings contributing to our understanding of the impact of sex-based differences in type I IFNs on HIV-1 disease pathogenesis.
The process of HIV-1 transmission through the mucosa of the female genital tract is comparatively unproductive (28). The innate immune system plays a major role in sensing incoming HIV-1 to initiate antiviral responses (29). HIV-1 can be recognized by multiple nucleic acid sensors, including toll-like receptors (TLRs) 3, 7, 8, and 9 (30), IFN-inducible protein (IFI) 16 (31), and cyclic GMP-AMP synthase (32). Recognition of HIV-1 by pattern recognition receptors leads to induction of antiviral and proinflammatory responses via JAK/STAT signaling, NF-κB, and interferon regulatory factors (IRFs) (33), ultimately resulting in the production of cytokines, chemokines, and type I IFNs. Type I IFNs are the first line of defense against viral infections, as they are capable of mediating immunregulatory, growth-inhibitory, and antiviral activities (34), through the induction of a multitude of interferon-stimulated genes (ISGs) that encode for antiviral proteins. In humans, the type I IFN family of genes consists of 13 highly homologous IFNα subtypes, IFNβ, IFNε, IFNκ, and IFNω (35). All type I IFNs bind to the same IFNα/β receptor (IFNAR) composed of two subunits IFNAR1 and IFNAR2, with varying affinities for the two subunits (36, 37). IFNARs are expressed on most cell types and their activation induces the transcription of ISGs. Type I IFNs play an expanding role in restricting HIV-1 replication (38). Productive infections in a new host were shown to be established by HIV-1 strains (transmitted founder viruses) that were much less susceptible to the antiviral effects of type I IFNs in vitro (39–41). In line with these antiviral effects of type I IFNs that have to be overcome by transmitted founder viruses, one recent study demonstrated that vaginal IFNβ administration in rhesus macaques upregulated the expression of ISGs in vaginal suspensions and prevented SHIV-1 acquisition (42). The distinct capacities of the 13 different IFNα subtypes in controlling retroviral infections have been studied using humanized mouse models, suggesting that IFNα8 and IFNα14 suppress HIV-1 replication significantly better than other IFNα subtypes (43). Garcia-Minambres et al. recently described another, less prominent, type I IFN important in HIV-1 restriction (44). IFNε is expressed in the female genital tract of mice and humans and induces several HIV-1 restriction factors in T cells, with levels and antiviral effects similar to IFNα-mediated induction. IFNε reduced HIV-1 replication in vitro and HIV-1 strains that were grown in the presence of IFNε were less infectious than HIV-1 that was produced in the absence of IFNε (44). Interestingly, IFNε expression in the female reproductive tract is hormone dependent, with the highest expression during the proliferative phase of the menstrual cycle, when estrogen levels are peaking (45). During the secretory phase, which is characterized by a peak in progesterone, IFNε levels decline (45). This decrease in IFNε in the presence of high progesterone might play a role in the suggested increase in HIV-1 acquisition in females that take long-acting contraceptives (46–50). Taken together, type I IFN levels that are increased within the first weeks of HIV-1 infection induce expression of HIV-1 restriction factors, which play an important role in restricting HIV-1 spread and replication early in infection.
Plasmacytoid dendritic cells (pDCs) are innate immune cells that are specialized to produce type I IFNs and mediate cross-talk between innate and adaptive immunity. Multiple studies have contributed important knowledge on the role of pDCs in mediating sex-specific, HIV-1-associated immune activation. pDCs from females produce higher levels of IFNα upon TLR7 stimulation using HIV-1 derived ssRNA (20) or synthetic ligands (18, 19, 51, 52) in comparison to men. In addition, we recently showed that pDCs from females express significantly higher mRNA levels of all 13 IFNα subtypes and IFNβ after TLR7-stimulation of peripheral blood mononuclear cells (PBMCs) (52). The mRNA expression levels of IFNα in pDCs were correlated with the expression levels of IFNβ in pDCs after TLR7 stimulation, but were independent from secondary signaling via IFNAR (52), as reported for TLR9 signaling or type I IFN production by other cell types than pDCs (53–55). Although IFNα produced by pDCs is able to inhibit HIV-1 replication by exerting growth-inhibitory and antiviral activities, the continuous exposure of pDCs to HIV-1 has opposing effects. HIV-1 recognition by pDCs leads to the expression of low levels of maturation molecules on pDCs and therefore skews pDCs toward a partially matured, persistently IFNα-secreting phenotype stimulating only weak T cell responses (56). The effect of chronic exposure to IFNα was recently studied in mice, showing that chronic IFNα stimulation was sufficient to suppress specific CD8 T cell responses to vaccinia virus infection by inducing the accumulation of suppressive Ly6Chi monocytes (57). HIV-1 in turn has been suggested to impair TLR7-mediated IFNα production by pDCs, potentially by binding to CD303 receptors expressed on pDCs (58).
TLR7 is encoded on the X chromosome (22). X chromosome inactivation (XCI) of one of the two female X chromosomes ensures dosage compensation in female mammals, in contrast to male mammals harboring only one X chromosome. Most genes on the X chromosome are assumed to be transcriptionally silent; however, the process of XCI is dynamic and relies on dosage-dependent activators. A number of genes are known to escape XCI and are expressed from both the active and inactive X chromosome (59–62). So far, there is no evidence for a higher expression of TLR7 genes in pDCs from women in humans (51, 63), though TLR7 gene duplication has been associated with autoreactive B cell responses in mice (64) and increased levels of TLR7 and TLR8 have been found in PBMCs of patients with the primary Sjögren’s syndrome, an autoimmune disease with a female sex imbalance (65). Taken together, further research investigating the molecular mechanisms underlying TLR7 expression and regulation in pDCs will help to dissect the role of TLR7 signaling and its contribution to the sex bias in type I IFN production in HIV-1-infected individuals.
Steroid hormones have also been shown to modulate TLR-dependent responses of pDCs in humans. Estrogens and estrogen receptor-dependent regulations play key roles in dendritic cell development and function (66). pDCs from postmenopausal women display reduced TLR7-responsiveness and IFNα production in comparison to women of reproductive age. Estrogen replacement therapy of postmenopausal women increased the percentage of IFNα-producing pDCs after TLR7 and TLR9 stimulation (18). In vitro blockage of ER signaling during pDC-differentiation dampened the IFNα response of pDCs after TLR7 stimulation (63). IFNα-induction by pDCs is regulated by IRFs at the transcriptional level (67). IRF5 is one of the central mediators of TLR7 signaling (68, 69) and has recently been shown to be expressed at higher basal levels in pDCs from females in comparison to males (19). While upregulation of IRF5 levels in pDCs resulted in increased IFNα secretion by pDCs, genetic ablation of the Esr1 gene reduced IRF5 mRNA expression in pDCs and subsequent IFNα production in response to TLR7 stimulation (19).
Sex hormones have also been reported to directly modulate host factors that play a role in HIV-1 acquisition (25). Generally, progesterone increases susceptibility to viral infections whereas estrogen protects against HIV-1 acquisition (70, 71). Studies of rhesus macaques support this general concept, as simian immunodeficiency virus (SIV) susceptibility was highest in the luteal phase of the menstrual cycle (high progesterone) compared with the follicular phase (high estrogen) (72). Several studies report an increase in expression of HIV-1 receptors on cervical CD4 T cells mediated by progesterone (73–75), supporting the reported increase in HIV-1 acquisition. Nevertheless, one in vitro study suggests that progesterone decreases the upregulation of CCR5 on CD4 T cells in peripheral blood (76). Furthermore, Meditz et al. showed that postmenopausal women, who have lower levels of progesterone, have elevated cervical CCR5 expression, which may increase their risk for HIV-1 acquisition (77). Estrogen exerts several biphasic effects on cells of the immune system, including modulation of T helper 1 versus T helper 2 cell differentiation (22) and expansion of regulatory T cells (78). Animal studies have shown that topical estrogen protects against vaginal SIV transmission in rhesus macaques (79). Estrogen was shown to downregulate the susceptibility of CD4 T cells and macrophages to HIV-1 in vitro (80), yet some studies suggest that in vivo hormone treatment with estrogens and antiandrogens in men to female transsexuals upregulates CCR5 on CD4 T cells over time (81), and also in mice (82).
The direct influence of steroid hormones on viral replication capacity of HIV-1 has been investigated in a few studies. Estradiol and progesterone can potentially regulate HIV-1 replication by directly altering HIV-1 transcriptional activation (83); however, the current results are controversial. In one study, in vitro estradiol treatment was shown to inhibit production of HIV-1 (84), while another study suggested an estrogen-based increase of transcriptional activity of HIV-1 LTRs (85). In addition, hormonal effects on HIV-1 replication were shown to be donor and HIV-1 subtype specific (86). Further studies are required to determine how the hormonal milieu might shape the outcome of HIV-1 infection in vivo and to identify the underlying molecular mechanisms.
It is now well established that systemic immune activation is a strong predictor for HIV-1 disease progression to AIDS. Consistent with the finding that innate immune function is linked to higher responses to TLR7 stimulation of pDCs and subsequent type I IFN production, HIV-1-infected women have higher expression levels of ISGs than men for the same level of viral load (87). Furthermore, the surface expression levels of the common IFN-α/β receptor subunit 2 (IFNAR2) are significantly higher on pDCs from females in comparison to males and tends to be higher on other antigen-presenting cells from females as well (52). The increase in ISG expression in female HIV-1-infected individuals might explain increased levels of T cell activation (CD4/8+ CD38+ HLA-DR+) in HIV-1-infected females in comparison to male HIV-1-infected individuals for the same level of viral load (20). Higher expression levels of IFNAR2 might contribute to the increased levels of ISG expression in females in comparison to males. In addition, higher levels of IFNα have been associated with decreased CD4 counts (88), which is in line with one study showing that increased expression of ISGs in CD4 T cells was associated with CD4 T cell depletion in HIV-1-infected individuals (89). The importance of type I IFNs in HIV-1 infections, as well as the timing of their effects, was studied in SIV infections of rhesus macaques (90). Blockage of IFN α/β receptors in rhesus macaques reduced antiviral gene expression and reservoir size during acute infections; however, continued IFNα2a treatment led to a desensitisation toward type I IFNs, resulting in decreased antiviral gene expression, increased reservoir size and accelerated CD4 T cell loss (90). Several mechanisms that underlie the association of immune activation and progression to AIDS have been proposed (14). Overall, it appears that the timing of type I IFN-induced innate response to HIV/SIV infection largely influences overall disease pathogenesis.
Several markers of inflammation and immune activation, such as C-reactive protein, sCD14, IL-6, and TNFα, have been associated with increased progression and mortality in HIV-1 infections (91–93). A novel non-human primate model for the investigation of sex differences in HIV-1 disease progression was recently used to study sex differences in local innate immune activation and gut microbiota (94). Viral load, disease progression, microbiota, and immunological parameters were studied in rhesus macaques infected with SHIV-1. This non-human primate model showed the same sex bias in SHIV-1 infection as observed for HIV-1 in humans and linked an earlier and more robust proinflammatory immune response as well as increased expansion of Proteobacteria in the female rectal mucosa to the increased disease susceptibility in female macaques (94).
A recent longitudinal study investigated sex differences in inflammatory and immune activation markers in HIV-1-infected women and men after initiation of combination antiretroviral treatment (cART). After cART initiation, women experienced less cART-associated reduction in inflammation and immune activation, indicated by higher levels of IFNγ and TNFα 48 weeks after initiation of therapy (95). Another study analyzed sex differences in soluble markers in plasma and cerebrospinal fluid (CSF) in HIV-1-infected treatment-naive individuals with or without cognitive impairment from Thailand. Soluble markers were quantified before and 48 weeks after cART initiation to identify variations in markers that may contribute to differences in disease progression (96). In chronic untreated HIV-1 infection, up to 50% of infected individuals have been reported to have cognitive impairment, commonly termed HIV-1-associated neurological disorders (97). Treatment naive, HIV-1-infected women with impaired cognition had elevated levels of neopterin and TNF-RII, both correlative markers for HIV-1 progression and efficiency of cART, compared to women with normal cognition in both plasma and CSF, whereas no associations were observed between these markers and cognition in men. Furthermore, sex-specific differences in the levels of a number of markers, including MCP-1, IL-8, IL-10, I-FABP, and sCD14, were detected in response to treatment (96). This study therefore proposed sex-specific differences in markers that were associated with cognitive impairment and chronic inflammation. Overall, these data suggest that changes in soluble markers vary before and after initiation of antiretroviral therapy between chronically HIV-1-infected women and men and might be predictive for HIV-1 disease outcomes.
The precise role of type I IFNs in HIV-1 disease progression still remains insufficiently understood. The outcomes of type I IFNs on disease outcome are multifactorial and appear to depend on timing and extend of the induction (local versus systemic), as well as on the specific IFNα subtypes that are induced. Sex differences in type I IFN responses are now widely accepted; however, the underlying mechanisms that lead to sex-based differences in type I IFN production and their impact on infectious diseases such as HIV-1, but also on autoimmune diseases, remain less well understood. The frequent under-representation of women in clinical studies furthermore impedes research progress in that area. Thoughtful designed experimental and clinical studies will have to fill in the current gaps in knowledge concerning the impact of chromosomal effects and/or hormonal influences on innate immune responses and the subsequent consequences for HIV-1 disease progression.
All authors have made a substantial, direct, and intellectual contribution to the work and approved it for publication.
The authors declare that the research was conducted in the absence of any commercial or financial relationships that could be construed as a potential conflict of interest.
We thank Sven Hagen for critical reading of the manuscript.
1. WHO. HIV/AIDS Fact sheet. (2017). Available from: http://www.who.int/mediacentre/factsheets/fs360/en/
2. UNAIDS. Report on the Global AIDS Epidemic 2013. (2017). Available from: http://www.unaids.org/sites/default/files/media_asset/UNAIDS_Global_Report_2013_en_1.pdf
3. Lemp GF, Hirozawa AM, Cohen JB, Derish PA, McKinney KC, Hernandez SR. Survival for women and men with AIDS. J Infect Dis (1992) 166(1):74–9. doi:10.1093/infdis/166.1.74
4. Chaisson RE, Keruly JC, Moore RD. Race, sex, drug use, and progression of human immunodeficiency virus disease. N Engl J Med (1995) 333(12):751–6. doi:10.1056/NEJM199509213331202
5. Nicolosi A, Correa Leite ML, Musicco M, Arici C, Gavazzeni G, Lazzarin A. The efficiency of male-to-female and female-to-male sexual transmission of the human immunodeficiency virus: a study of 730 stable couples. Italian Study Group on HIV Heterosexual Transmission. Epidemiology (1994) 5(6):570–5. doi:10.1097/00001648-199411000-00003
6. Anastos K, Gange SJ, Lau B, Weiser B, Detels R, Giorgi JV, et al. Association of race and gender with HIV-1 RNA levels and immunologic progression. J Acquir Immune Defic Syndr (2000) 24(3):218–26. doi:10.1097/00126334-200007010-00004
7. Farzadegan H, Hoover DR, Astemborski J, Lyles CM, Margolick JB, Markham RB, et al. Sex differences in HIV-1 viral load and progression to AIDS. Lancet (1998) 352(9139):1510–4. doi:10.1016/S0140-6736(98)02372-1
8. Sterling TR, Lyles CM, Vlahov D, Astemborski J, Margolick JB, Quinn TC. Sex differences in longitudinal human immunodeficiency virus type 1 RNA levels among seroconverters. J Infect Dis (1999) 180(3):666–72. doi:10.1086/314967
9. Sterling TR, Vlahov D, Astemborski J, Hoover DR, Margolick JB, Quinn TC. Initial plasma HIV-1 RNA levels and progression to AIDS in women and men. N Engl J Med (2001) 344(10):720–5. doi:10.1056/NEJM200103083441003
10. Ghosn J, Deveau C, Chaix ML, Goujard C, Galimand J, Zitoun Y, et al. Despite being highly diverse, immunovirological status strongly correlates with clinical symptoms during primary HIV-1 infection: a cross-sectional study based on 674 patients enrolled in the ANRS CO 06 PRIMO cohort. J Antimicrob Chemother (2010) 65(4):741–8. doi:10.1093/jac/dkq035
11. Ngo-Giang-Huong N, Deveau C, Da Silva I, Pellegrin I, Venet A, Harzic M, et al. Proviral HIV-1 DNA in subjects followed since primary HIV-1 infection who suppress plasma viral load after one year of highly active antiretroviral therapy. AIDS (2001) 15(6):665–73. doi:10.1097/00002030-200104130-00001
12. Cuzin L, Pugliese P, Saune K, Allavena C, Ghosn J, Cottalorda J, et al. Levels of intracellular HIV-DNA in patients with suppressive antiretroviral therapy. AIDS (2015) 29(13):1665–71. doi:10.1097/QAD.0000000000000723
13. Fourati S, Flandre P, Calin R, Carcelain G, Soulie C, Lambert-Niclot S, et al. Factors associated with a low HIV reservoir in patients with prolonged suppressive antiretroviral therapy. J Antimicrob Chemother (2014) 69(3):753–6. doi:10.1093/jac/dkt428
14. Utay NS, Douek DC. Interferons and HIV infection: the good, the bad, and the ugly. Pathog Immun (2016) 1(1):107–16. doi:10.20411/pai.v1i1.125
15. Odorizzi PM, Wherry EJ. Immunology. An interferon paradox. Science (2013) 340(6129):155–6. doi:10.1126/science.1237568
16. Griesbeck M, Scully E, Altfeld M. Sex and gender differences in HIV-1 infection. Clin Sci (Lond) (2016) 130(16):1435–51. doi:10.1042/CS20160112
17. Santelli JS, Edelstein ZR, Mathur S, Wei Y, Zhang W, Orr MG, et al. Behavioral, biological, and demographic risk and protective factors for new HIV infections among youth in Rakai, Uganda. J Acquir Immune Defic Syndr (2013) 63(3):393–400. doi:10.1097/QAI.0b013e3182926795
18. Seillet C, Laffont S, Tremollieres F, Rouquie N, Ribot C, Arnal JF, et al. The TLR-mediated response of plasmacytoid dendritic cells is positively regulated by estradiol in vivo through cell-intrinsic estrogen receptor alpha signaling. Blood (2012) 119(2):454–64. doi:10.1182/blood-2011-08-371831
19. Griesbeck M, Ziegler S, Laffont S, Smith N, Chauveau L, Tomezsko P, et al. Sex differences in plasmacytoid dendritic cell levels of IRF5 drive higher IFN-alpha production in women. J Immunol (2015) 195(11):5327–36. doi:10.4049/jimmunol.1501684
20. Meier A, Chang JJ, Chan ES, Pollard RB, Sidhu HK, Kulkarni S, et al. Sex differences in the Toll-like receptor-mediated response of plasmacytoid dendritic cells to HIV-1. Nat Med (2009) 15(8):955–9. doi:10.1038/nm.2004
21. Libert C, Dejager L, Pinheiro I. The X chromosome in immune functions: when a chromosome makes the difference. Nat Rev Immunol (2010) 10(8):594–604. doi:10.1038/nri2815
22. Fish EN. The X-files in immunity: sex-based differences predispose immune responses. Nat Rev Immunol (2008) 8(9):737–44. doi:10.1038/nri2394
23. Markle JG, Fish EN. SeXX matters in immunity. Trends Immunol (2014) 35(3):97–104. doi:10.1016/j.it.2013.10.006
24. Yurkovetskiy L, Burrows M, Khan AA, Graham L, Volchkov P, Becker L, et al. Gender bias in autoimmunity is influenced by microbiota. Immunity (2013) 39(2):400–12. doi:10.1016/j.immuni.2013.08.013
25. Hagen S, Altfeld M. The X awakens: multifactorial ramifications of sex-specific differences in HIV-1 infection. J Virus Erad (2016) 2(2):78–81.
26. Addo MM, Altfeld M. Sex-based differences in HIV type 1 pathogenesis. J Infect Dis (2014) 209(Suppl 3):S86–92. doi:10.1093/infdis/jiu175
27. Ziegler S, Altfeld M. Sex differences in HIV-1-mediated immunopathology. Curr Opin HIV AIDS (2016) 11(2):209–15. doi:10.1097/COH.0000000000000237
28. Wawer MJ, Gray RH, Sewankambo NK, Serwadda D, Li X, Laeyendecker O, et al. Rates of HIV-1 transmission per coital act, by stage of HIV-1 infection, in Rakai, Uganda. J Infect Dis (2005) 191(9):1403–9. doi:10.1086/429411
29. Altfeld M, Gale M Jr. Innate immunity against HIV-1 infection. Nat Immunol (2015) 16(6):554–62. doi:10.1038/ni.3157
30. Blasius AL, Beutler B. Intracellular toll-like receptors. Immunity (2010) 32(3):305–15. doi:10.1016/j.immuni.2010.03.012
31. Jakobsen MR, Bak RO, Andersen A, Berg RK, Jensen SB, Tengchuan J, et al. IFI16 senses DNA forms of the lentiviral replication cycle and controls HIV-1 replication. Proc Natl Acad Sci U S A (2013) 110(48):E4571–80. doi:10.1073/pnas.1311669110
32. Gao D, Wu J, Wu YT, Du F, Aroh C, Yan N, et al. Cyclic GMP-AMP synthase is an innate immune sensor of HIV and other retroviruses. Science (2013) 341(6148):903–6. doi:10.1126/science.1240933
33. Mogensen TH, Melchjorsen J, Larsen CS, Paludan SR. Innate immune recognition and activation during HIV infection. Retrovirology (2010) 7:54. doi:10.1186/1742-4690-7-54
34. Goodbourn S, Didcock L, Randall RE. Interferons: cell signalling, immune modulation, antiviral response and virus countermeasures. J Gen Virol (2000) 81(Pt 10):2341–64. doi:10.1099/0022-1317-81-10-2341
35. Pestka S, Krause CD, Walter MR. Interferons, interferon-like cytokines, and their receptors. Immunol Rev (2004) 202:8–32. doi:10.1111/j.0105-2896.2004.00204.x
36. Schreiber G, Piehler J. The molecular basis for functional plasticity in type I interferon signaling. Trends Immunol (2015) 36(3):139–49. doi:10.1016/j.it.2015.01.002
37. Gibbert K, Schlaak JF, Yang D, Dittmer U. IFN-alpha subtypes: distinct biological activities in anti-viral therapy. Br J Pharmacol (2013) 168(5):1048–58. doi:10.1111/bph.12010
38. Kent SJ, Kelleher AD. Expanding role for type I interferons in restricting HIV growth. Immunol Cell Biol (2017) 95(5):417–8. doi:10.1038/icb.2017.15
39. Iyer SS, Bibollet-Ruche F, Sherrill-Mix S, Learn GH, Plenderleith L, Smith AG, et al. Resistance to type 1 interferons is a major determinant of HIV-1 transmission fitness. Proc Natl Acad Sci U S A (2017) 114(4):E590–9. doi:10.1073/pnas.1620144114
40. Parrish NF, Gao F, Li H, Giorgi EE, Barbian HJ, Parrish EH, et al. Phenotypic properties of transmitted founder HIV-1. Proc Natl Acad Sci U S A (2013) 110(17):6626–33. doi:10.1073/pnas.1304288110
41. Fenton-May AE, Dibben O, Emmerich T, Ding H, Pfafferott K, Aasa-Chapman MM, et al. Relative resistance of HIV-1 founder viruses to control by interferon-alpha. Retrovirology (2013) 10:146. doi:10.1186/1742-4690-10-146
42. Veazey RS, Pilch-Cooper HA, Hope TJ, Alter G, Carias AM, Sips M, et al. Prevention of SHIV transmission by topical IFN-beta treatment. Mucosal Immunol (2016) 9(6):1528–36. doi:10.1038/mi.2015.146
43. Lavender KJ, Gibbert K, Peterson KE, Van Dis E, Francois S, Woods T, et al. Interferon alpha subtype-specific suppression of HIV-1 infection in vivo. J Virol (2016) 90(13):6001–13. doi:10.1128/JVI.00451-16
44. Garcia-Minambres A, Eid SG, Mangan NE, Pade C, Lim SS, Matthews AY, et al. Interferon epsilon promotes HIV restriction at multiple steps of viral replication. Immunol Cell Biol (2017) 95(5):478–83. doi:10.1038/icb.2016.123
45. Fung KY, Mangan NE, Cumming H, Horvat JC, Mayall JR, Stifter SA, et al. Interferon-epsilon protects the female reproductive tract from viral and bacterial infection. Science (2013) 339(6123):1088–92. doi:10.1126/science.1233321
46. Morrison CS, Chen PL, Kwok C, Baeten JM, Brown J, Crook AM, et al. Hormonal contraception and the risk of HIV acquisition: an individual participant data meta-analysis. PLoS Med (2015) 12(1):e1001778. doi:10.1371/journal.pmed.1001778
47. Morrison CS, Chen PL, Kwok C, Richardson BA, Chipato T, Mugerwa R, et al. Hormonal contraception and HIV acquisition: reanalysis using marginal structural modeling. AIDS (2010) 24(11):1778–81. doi:10.1097/QAD.0b013e32833a2537
48. Blish CA, Baeten JM. Hormonal contraception and HIV-1 transmission. Am J Reprod Immunol (2011) 65(3):302–7. doi:10.1111/j.1600-0897.2010.00930.x
49. Baeten JM, Benki S, Chohan V, Lavreys L, McClelland RS, Mandaliya K, et al. Hormonal contraceptive use, herpes simplex virus infection, and risk of HIV-1 acquisition among Kenyan women. AIDS (2007) 21(13):1771–7. doi:10.1097/QAD.0b013e328270388a
50. Heffron R, Donnell D, Rees H, Celum C, Mugo N, Were E, et al. Use of hormonal contraceptives and risk of HIV-1 transmission: a prospective cohort study. Lancet Infect Dis (2012) 12(1):19–26. doi:10.1016/S1473-3099(11)70247-X
51. Berghofer B, Frommer T, Haley G, Fink L, Bein G, Hackstein H. TLR7 ligands induce higher IFN-alpha production in females. J Immunol (2006) 177(4):2088–96. doi:10.4049/jimmunol.177.4.2088
52. Ziegler SM, Beisel C, Sutter K, Griesbeck M, Hildebrandt H, Hagen SH, et al. Human pDCs display sex-specific differences in type I interferon subtypes and interferon alpha/beta receptor expression. Eur J Immunol (2017) 47(2):251–6. doi:10.1002/eji.201646725
53. Honda K, Yanai H, Negishi H, Asagiri M, Sato M, Mizutani T, et al. IRF-7 is the master regulator of type-I interferon-dependent immune responses. Nature (2005) 434(7034):772–7. doi:10.1038/nature03464
54. Marie I, Durbin JE, Levy DE. Differential viral induction of distinct interferon-alpha genes by positive feedback through interferon regulatory factor-7. EMBO J (1998) 17(22):6660–9. doi:10.1093/emboj/17.22.6660
55. Prakash A, Smith E, Lee CK, Levy DE. Tissue-specific positive feedback requirements for production of type I interferon following virus infection. J Biol Chem (2005) 280(19):18651–7. doi:10.1074/jbc.M501289200
56. O’Brien M, Manches O, Sabado RL, Baranda SJ, Wang Y, Marie I, et al. Spatiotemporal trafficking of HIV in human plasmacytoid dendritic cells defines a persistently IFN-alpha-producing and partially matured phenotype. J Clin Invest (2011) 121(3):1088–101. doi:10.1172/JCI44960
57. Taleb K, Auffray C, Villefroy P, Pereira A, Hosmalin A, Gaudry M, et al. Chronic type I IFN is sufficient to promote immunosuppression through accumulation of myeloid-derived suppressor cells. J Immunol (2017) 198(3):1156–63. doi:10.4049/jimmunol.1502638
58. Dhamanage A, Thakar M, Paranjape R. Human immunodeficiency virus-1 impairs IFN-alpha production induced by TLR-7 agonist in plasmacytoid dendritic cells. Viral Immunol (2017) 30(1):28–34. doi:10.1089/vim.2016.0084
59. Augui S, Nora EP, Heard E. Regulation of X-chromosome inactivation by the X-inactivation centre. Nat Rev Genet (2011) 12(6):429–42. doi:10.1038/nrg2987
60. Carrel L, Willard HF. X-inactivation profile reveals extensive variability in X-linked gene expression in females. Nature (2005) 434(7031):400–4. doi:10.1038/nature03479
61. Carrel L, Cottle AA, Goglin KC, Willard HF. A first-generation X-inactivation profile of the human X chromosome. Proc Natl Acad Sci U S A (1999) 96(25):14440–4. doi:10.1073/pnas.96.25.14440
62. Carrel L, Willard HF. Heterogeneous gene expression from the inactive X chromosome: an X-linked gene that escapes X inactivation in some human cell lines but is inactivated in others. Proc Natl Acad Sci U S A (1999) 96(13):7364–9. doi:10.1073/pnas.96.13.7364
63. Laffont S, Rouquie N, Azar P, Seillet C, Plumas J, Aspord C, et al. X-Chromosome complement and estrogen receptor signaling independently contribute to the enhanced TLR7-mediated IFN-alpha production of plasmacytoid dendritic cells from women. J Immunol (2014) 193(11):5444–52. doi:10.4049/jimmunol.1303400
64. Pisitkun P, Deane JA, Difilippantonio MJ, Tarasenko T, Satterthwaite AB, Bolland S. Autoreactive B cell responses to RNA-related antigens due to TLR7 gene duplication. Science (2006) 312(5780):1669–72. doi:10.1126/science.1124978
65. Karlsen M, Jakobsen K, Jonsson R, Hammenfors D, Hansen T, Appel S. Expression of toll-like receptors in peripheral blood mononuclear cells of patients with primary Sjogren’s syndrome. Scand J Immunol (2017) 85(3):220–6. doi:10.1111/sji.12520
66. Laffont S, Seillet C, Guery JC. Estrogen receptor-dependent regulation of dendritic cell development and function. Front Immunol (2017) 8:108. doi:10.3389/fimmu.2017.00108
67. Honda K, Takaoka A, Taniguchi T. Type I interferon [corrected] gene induction by the interferon regulatory factor family of transcription factors. Immunity (2006) 25(3):349–60. doi:10.1016/j.immuni.2006.08.009
68. Schoenemeyer A, Barnes BJ, Mancl ME, Latz E, Goutagny N, Pitha PM, et al. The interferon regulatory factor, IRF5, is a central mediator of toll-like receptor 7 signaling. J Biol Chem (2005) 280(17):17005–12. doi:10.1074/jbc.M412584200
69. Takaoka A, Yanai H, Kondo S, Duncan G, Negishi H, Mizutani T, et al. Integral role of IRF-5 in the gene induction programme activated by Toll-like receptors. Nature (2005) 434(7030):243–9. doi:10.1038/nature03308
70. Mingjia L, Short R. How oestrogen or progesterone might change a woman’s susceptibility to HIV-1 infection. Aust N Z J Obstet Gynaecol (2002) 42(5):472–5. doi:10.1111/j.0004-8666.2002.00472.x
71. Kaushic C, Roth KL, Anipindi V, Xiu F. Increased prevalence of sexually transmitted viral infections in women: the role of female sex hormones in regulating susceptibility and immune responses. J Reprod Immunol (2011) 88(2):204–9. doi:10.1016/j.jri.2010.12.004
72. Sodora DL, Gettie A, Miller CJ, Marx PA. Vaginal transmission of SIV: assessing infectivity and hormonal influences in macaques inoculated with cell-free and cell-associated viral stocks. AIDS Res Hum Retroviruses (1998) 14(Suppl 1):S119–23.
73. Dominguez F, Galan A, Martin JJ, Remohi J, Pellicer A, Simon C. Hormonal and embryonic regulation of chemokine receptors CXCR1, CXCR4, CCR5 and CCR2B in the human endometrium and the human blastocyst. Mol Hum Reprod (2003) 9(4):189–98. doi:10.1093/molehr/gag024
74. Prakash M, Kapembwa MS, Gotch F, Patterson S. Oral contraceptive use induces upregulation of the CCR5 chemokine receptor on CD4(+) T cells in the cervical epithelium of healthy women. J Reprod Immunol (2002) 54(1–2):117–31. doi:10.1016/S0165-0378(01)00125-5
75. Sheffield JS, Wendel GD Jr, McIntire DD, Norgard MV. The effect of progesterone levels and pregnancy on HIV-1 coreceptor expression. Reprod Sci (2009) 16(1):20–31. doi:10.1177/1933719108325510
76. Vassiliadou N, Tucker L, Anderson DJ. Progesterone-induced inhibition of chemokine receptor expression on peripheral blood mononuclear cells correlates with reduced HIV-1 infectability in vitro. J Immunol (1999) 162(12):7510–8.
77. Meditz AL, Moreau KL, MaWhinney S, Gozansky WS, Melander K, Kohrt WM, et al. CCR5 expression is elevated on endocervical CD4+ T cells in healthy postmenopausal women. J Acquir Immune Defic Syndr (2012) 59(3):221–8. doi:10.1097/QAI.0b013e31823fd215
78. Arruvito L, Sanz M, Banham AH, Fainboim L. Expansion of CD4+CD25+and FOXP3+ regulatory T cells during the follicular phase of the menstrual cycle: implications for human reproduction. J Immunol (2007) 178(4):2572–8. doi:10.4049/jimmunol.178.4.2572
79. Smith SM, Mefford M, Sodora D, Klase Z, Singh M, Alexander N, et al. Topical estrogen protects against SIV vaginal transmission without evidence of systemic effect. AIDS (2004) 18(12):1637–43. doi:10.1097/01.aids.0000131393.76221.cc
80. Rodriguez-Garcia M, Biswas N, Patel MV, Barr FD, Crist SG, Ochsenbauer C, et al. Estradiol reduces susceptibility of CD4+ T cells and macrophages to HIV-infection. PLoS One (2013) 8(4):e62069. doi:10.1371/journal.pone.0062069
81. Giltay EJ, Fonk JC, von Blomberg BM, Drexhage HA, Schalkwijk C, Gooren LJ. In vivo effects of sex steroids on lymphocyte responsiveness and immunoglobulin levels in humans. J Clin Endocrinol Metab (2000) 85(4):1648–57. doi:10.1210/jcem.85.4.6562
82. Mo R, Chen J, Grolleau-Julius A, Murphy HS, Richardson BC, Yung RL. Estrogen regulates CCR gene expression and function in T lymphocytes. J Immunol (2005) 174(10):6023–9. doi:10.4049/jimmunol.174.10.6023
83. Asin SN, Heimberg AM, Eszterhas SK, Rollenhagen C, Howell AL. Estradiol and progesterone regulate HIV type 1 replication in peripheral blood cells. AIDS Res Hum Retroviruses (2008) 24(5):701–16. doi:10.1089/aid.2007.0108
84. Szotek EL, Narasipura SD, Al-Harthi L. 17beta-Estradiol inhibits HIV-1 by inducing a complex formation between beta-catenin and estrogen receptor alpha on the HIV promoter to suppress HIV transcription. Virology (2013) 443(2):375–83. doi:10.1016/j.virol.2013.05.027
85. Katagiri D, Hayashi H, Victoriano AF, Okamoto T, Onozaki K. Estrogen stimulates transcription of human immunodeficiency virus type 1 (HIV-1). Int Immunopharmacol (2006) 6(2):170–81. doi:10.1016/j.intimp.2005.07.017
86. Ragupathy V, Devadas K, Tang S, Wood O, Lee S, Dastyer A, et al. Effect of sex steroid hormones on replication and transmission of major HIV subtypes. J Steroid Biochem Mol Biol (2013) 138:63–71. doi:10.1016/j.jsbmb.2013.03.002
87. Chang JJ, Altfeld M. Immune activation and the role of TLRs and TLR agonists in the pathogenesis of HIV-1 infection in the humanized mouse model. J Infect Dis (2013) 208(Suppl 2):S145–9. doi:10.1093/infdis/jit402
88. Hardy GA, Sieg S, Rodriguez B, Anthony D, Asaad R, Jiang W, et al. Interferon-alpha is the primary plasma type-I IFN in HIV-1 infection and correlates with immune activation and disease markers. PLoS One (2013) 8(2):e56527. doi:10.1371/journal.pone.0056527
89. Fernandez S, Tanaskovic S, Helbig K, Rajasuriar R, Kramski M, Murray JM, et al. CD4+ T-cell deficiency in HIV patients responding to antiretroviral therapy is associated with increased expression of interferon-stimulated genes in CD4+ T cells. J Infect Dis (2011) 204(12):1927–35. doi:10.1093/infdis/jir659
90. Sandler NG, Bosinger SE, Estes JD, Zhu RT, Tharp GK, Boritz E, et al. Type I interferon responses in rhesus macaques prevent SIV infection and slow disease progression. Nature (2014) 511(7511):601–5. doi:10.1038/nature13554
91. Ledwaba L, Tavel JA, Khabo P, Maja P, Qin J, Sangweni P, et al. Pre-ART levels of inflammation and coagulation markers are strong predictors of death in a South African cohort with advanced HIV disease. PLoS One (2012) 7(3):e24243. doi:10.1371/journal.pone.0024243
92. Kuller LH, Tracy R, Belloso W, De Wit S, Drummond F, Lane HC, et al. Inflammatory and coagulation biomarkers and mortality in patients with HIV infection. PLoS Med (2008) 5(10):e203. doi:10.1371/journal.pmed.0050203
93. Sandler NG, Wand H, Roque A, Law M, Nason MC, Nixon DE, et al. Plasma levels of soluble CD14 independently predict mortality in HIV infection. J Infect Dis (2011) 203(6):780–90. doi:10.1093/infdis/jiq118
94. Ren W, Ma Y, Yang L, Gettie A, Salas J, Russell K, et al. Fast disease progression in simian HIV-infected female macaque is accompanied by a robust local inflammatory innate immune and microbial response. AIDS (2015) 29(10):F1–8. doi:10.1097/QAD.0000000000000711
95. Mathad JS, Gupte N, Balagopal A, Asmuth D, Hakim J, Santos B, et al. Sex-related differences in inflammatory and immune activation markers before and after combined antiretroviral therapy initiation. J Acquir Immune Defic Syndr (2016) 73(2):123–9. doi:10.1097/QAI.0000000000001095
96. Krebs SJ, Slike BM, Sithinamsuwan P, Allen IE, Chalermchai T, Tipsuk S, et al. Sex differences in soluble markers vary before and after the initiation of antiretroviral therapy in chronically HIV-infected individuals. AIDS (2016) 30(10):1533–42. doi:10.1097/QAD.0000000000001096
Keywords: type I interferon, human immunodeficiency virus 1, sex hormones, immune activation, sex differences, toll-like receptor 7
Citation: Ziegler SM and Altfeld M (2017) Human Immunodeficiency Virus 1 and Type I Interferons—Where Sex Makes a Difference. Front. Immunol. 8:1224. doi: 10.3389/fimmu.2017.01224
Received: 26 July 2017; Accepted: 15 September 2017;
Published: 28 September 2017
Edited by:
Mustapha Chamekh, Free University of Brussels, BelgiumReviewed by:
Sally A. Huber, University of Vermont, United StatesCopyright: © 2017 Ziegler and Altfeld. This is an open-access article distributed under the terms of the Creative Commons Attribution License (CC BY). The use, distribution or reproduction in other forums is permitted, provided the original author(s) or licensor are credited and that the original publication in this journal is cited, in accordance with accepted academic practice. No use, distribution or reproduction is permitted which does not comply with these terms.
*Correspondence: Marcus Altfeld, bS5hbHRmZWxkQHVrZS5kZQ==
Disclaimer: All claims expressed in this article are solely those of the authors and do not necessarily represent those of their affiliated organizations, or those of the publisher, the editors and the reviewers. Any product that may be evaluated in this article or claim that may be made by its manufacturer is not guaranteed or endorsed by the publisher.
Research integrity at Frontiers
Learn more about the work of our research integrity team to safeguard the quality of each article we publish.