- 1Department of Microbiology and Immunology, Medical University of South Carolina (MUSC), Charleston, SC, United States
- 2Hollings Cancer Center, Medical University of South Carolina (MUSC), Charleston, SC, United States
Chronic Inflammation in tumor microenvironments is not only associated with various stages of tumor development, but also has significant impacts on tumor immunity and immunotherapy. Inflammasome are an important innate immune pathway critical for the production of active IL-1β and interleukin 18, as well as the induction of pyroptosis. Although extensive studies have demonstrated that inflammasomes play a vital role in infectious and autoimmune diseases, their role in tumor progression remains elusive. Multiple studies using a colitis-associated colon cancer model show that inflammasome components provide protection against the development of colon cancer. However, very recent studies demonstrate that inflammasomes promote tumor progression in skin and breast cancer. These results indicate that inflammasomes can promote and suppress tumor development depending on types of tumors, specific inflammasomes involved, and downstream effector molecules. The complicated role of inflammasomes raises new opportunities and challenges to manipulate inflammasome pathways in the treatment of cancer.
Introduction
Emerging evidence indicates that chronic inflammation plays an important role at all stages of tumor development, including initiation, growth, invasion, and metastasis (1–7). As part of the immune surveillance system, various innate immune pathways may engage with cellular components released from dead tumor cells due to hypoxia, chemotherapy, radiotherapy, or an immune attack (8–11). The innate immune cells activated by tumors or tumor components may induce antitumor immunity through the recruitment of effector cells or promote tumor development by providing a pro-inflammatory environment (Figure 1). While there are numerous studies on the involvement of toll-like receptors (TLRs) or interferon (IFN) pathways in tumor development (9, 12–16), the role of inflammasomes in tumor development is poorly characterized. The inflammasome is a novel innate immune pathway involved in the production of active IL-1β and interleukin 18 (IL-18), which are potent inflammatory cytokines (11, 17–21). Extensive evidence indicates that inflammasomes play a vital role in pathogen infections and autoimmune diseases. However, their role in tumor progression remains unclear. Many published studies use colitis-induced colon cancer as an animal model to investigate the role of inflammasomes in cancer. Results from those studies indicate that inflammasome components provide protection against tumorigenesis in colitis-associated colon cancer (22–29). Yet recent studies from our group and others demonstrate that inflammasomes can promote tumor development in certain types of cancer (30–32).
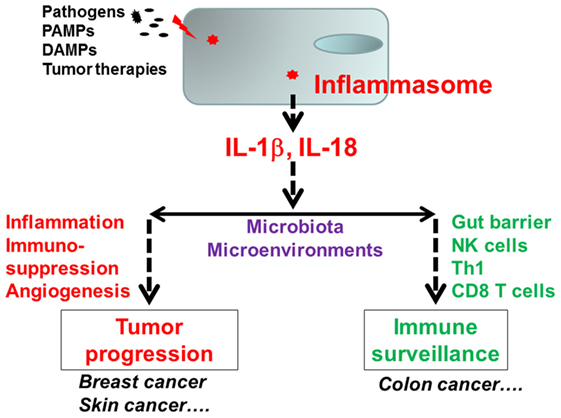
Figure 1. The dynamic role of the inflammasome in tumor development. The inflammasomes can promote or inhibit tumor progression depending on context. In colitis-associated colon cancer, inflammasome-derived interleukin 18 (IL-18) supports intestinal barrier function and induces tumor surveillance at the intestinal mucosal surface. For breast cancer and skin cancer, IL-1β-induced inflammation and immunosuppression promote tumor growth and metastasis. Tumor microenvironments and gut microbiota may also influence tumor progression and host antitumor immunity.
In this review, we will discuss how the inflammasome and its effector pathways influence the pathogenesis of various types of cancer (Figure 1). The first part of the paper provides basic information about inflammasomes. Subsequently, we use a colitis-associated colon cancer model to illustrate the protect role of inflammasomes against tumors. Then, we utilize skin and breast cancer models to demonstrate the tumor-promoting effects of inflammasomes. Finally, we list some questions about inflammasomes and cancer in the perspective section.
Inflammasomes
The inflammasome is a major component of the innate immune system that functions to induce maturation of inflammatory cytokines such as Interleukin 1 (IL-1β) and IL-18 in response to infection or autogenous danger signals (33–39). An inflammasome is a multimolecular complex, composed of a NOD-like protein (NLR), the adaptor apoptosis-associated speck-like protein containing a caspase recruitment domain (ASC), and caspase-1. NLRs belong to host pattern-recognition receptors (PRRs) that recognize pathogen-associated molecular patterns (PAMPs) from bacteria or viruses to initiate the innate immune response (11, 20, 40–42). These PRRs can be found on the membrane surface, e.g., TLRs and C-type lectin receptors, or intracellularly, e.g., NOD-like receptors (NLRs) and RIG-I-like receptors. Like TLRs, NLRPs also interact with endogenous ligands or damage-associated molecular patterns (DAMPs) from normal host tissues or tumor cells to induce autoimmune diseases or an antitumor response. In recent years, NLRP proteins have attracted lots of attention because some of them can form inflammasomes.
The NLRs generally comprise of a caspase recruitment domain (CARD), or pyrin (PYD) at the N-terminus, a nucleotide-binding and oligomerization domain (NBD or NACHT) in the middle, and leucine-rich repeat (LRR) at the C-terminus (23, 33, 43, 44). The CARD or PYD interacts with the PYD domain in ASC or other downstream signaling molecules, NACHT is involved in oligomerization and other regulatory functions, whereas LRR functions as a sensor or signal receiver from PAMPs and DAMPs. Production of mature or active IL-1β needs two signals: the first signal is initiated by TLR ligands or endogenous molecules to induce the expression of pro-IL-1β proteins; the second is triggered by very diverse stimuli activating inflammasomes, leading to caspase-1-dependent conversion of pro-IL-1β to mature IL-1β. Upon engagement of endogenous or exogenous stimuli, NLRP proteins interact with ASC and caspase-1 and undergo oligomerization, eventually forming a huge signaling complex. When pro-caspase-1 is associated with ASC and NLRPs, it undergoes self-cleavage to form an active form of caspase-1 enzyme, which in turn process pro-IL-1 and pro-IL-18 proteins into their active forms. Activation of inflammasomes also leads to a form of inflammatory cell death, termed as pyroptosis, through a pore-forming protein gasdermin D (45–48). NLRs have about 22 family members in the human genome and more than 30 members in the mouse genome. Many, but not all, NLRs can form inflammasomes. While the NLR, pyrin domain containing 3 (NLRP3) inflammasome is the most studied one in this group of signaling complexes, other inflammasomes, including NLRP1, NLRC4, and NLRP12 inflammasomes, have also been identified (33–39). In addition to NLRs, the HIN200 family members absent in myeloma 2 (AIM2) and IFN-inducible protein 16 (IFI16) can assemble inflammasomes with ASC and caspase-1 (49, 50). AIM2 has been shown to form an inflammasome in response to bacterial DNA, viral DNA, or endogenous DNA released during cellular damage (49–51). Similarly, IFI16 recruits ASC and caspase-1 to assemble an inflammasome upon sensing DNA from Kaposi sarcoma-associated herpes virus (52–55). Because of its potential to recognize host DNA, AIM2 may contribute to the development of autoimmune diseases, including systemic lupus erythematosus, psoriasis, and arthritis. Furthermore, recent studies show that AIM2 is required to mediate protection against colorectal cancer (56–61). Generally, each type of inflammasomes, except NLRP3, recognizes defined molecular patterns from pathogens. For example, NLRC4 senses cytoplasmic flagellin and bacterial type III secretion apparatus from Gram-negative bacteria such as Salmonella typhimurium. NLRP1 can sense muramyl dipeptide, and Bacillus anthracis lethal toxin. AIM2 recognizes cytoplasmic DNA from host or pathogens (33–39). Although many stimuli with very diverse and unrelated molecular structures can trigger the activation of the NLRP3 inflammasome, the underlying molecular mechanisms remain elusive.
NOD-like receptor proteins have been shown to be associated with various autoimmune or inflammatory diseases. For example, NOD1 and NOD2 have been implicated in the pathogenesis of inflammatory bowel disease (IBD) (62, 63). Mutations in the human NLRP3 gene is associated with a group of autoimmune diseases termed as cold-induced auto-inflammatory syndrome, including familial cold-induced auto-inflammatory syndrome, Muckle–Wells syndrome, and NOMID/CINCA (neonatal onset multisystem inflammatory disorder or chronic infantile neurologic cutaneous and articular syndrome) (34, 64–67). Accumulating evidence shows that NLRP3 inflammasome is involved in a wide array of autoimmune and inflammatory diseases, such as IBD, liver steatosis, cardiovascular disease, rheumatoid arthritis, type one diabetes, and neurologic diseases (21, 23, 38, 43, 64, 68–71).
IL-1β and IL-18 are potent inflammatory cytokines that trigger various signaling pathways, including NF-κB, MAPK, and PI3K pathways (72–76). They are members of the IL-1 family of cytokines produced by macrophages and other cells during an immune response. Previous clinical studies also reveal that increased IL-1β in tumor tissues is associated with poorer prognosis (72, 77–80). Although inflammasomes are essential for host defense against pathogens and contribute to autoimmune diseases, their roles in tumor progression remain controversial. Results from published studies have shown that inflammasomes can inhibit or promote tumor growth and progression. Currently, we have very limited knowledge of the mechanisms responsible for inflammasome activation during tumor development and therapies.
Inflammasomes Inhibit Cancer Development in Colitis-Associated Colon Cancer
A series of studies using various NLRP or caspase-1-deficient mice have reported that inflammasome activities protect mice from colitis-associated colon cancer (CAC) induced by azoxymethane/dextran sodium sulfate (AOM/DSS) (22–29). In this model, DSS in drinking water causes damage to the epithelial barrier, resulting in massive inflammation induced by gut microflora. AOM is a potent carcinogen causing DNA damage in epithelial cells. Repeating DSS administration cause chronic inflammation, which promotes colorectal cancer development in cells harboring mutations elicited by AOM. Results from these studies show that mice deficient for inflammasome components, including NLRP3, caspase-1, NLRP1, NLRP6, and NLRC4, are highly susceptible to colitis-associated colon cancer induced by AOM/DSS by displaying severe intestinal inflammation, and increased number of colon polyps (22–29).
While inflammasome-deficient mice are generally susceptible to DSS-induced colitis, the results on a particular NLRP may vary. For instance, Hu et al. showed NLRC4 and caspase-1 control colitis-associated tumorigenesis (81). In the AOM/DSS-induced colorectal cancer model, NLRC4 and caspase-1 KO mice exhibited increased tumor load and tumor number per mice. However, the authors found no difference in colitis-associated colon cancer between the NLRP3-deficient and WT mice (81). In contrast, Allen et al. found that NLRC4 had no protective role in tumorigenesis, compared to WT mice. Instead, NLRP3 expression in hematopoietic cell compartment is essential for protection against colon cancer (25). This discrepancy may be due to experimental conditions or micro biota associated with mouse colonies.
Surprisingly, the results from these studies indicate that IL-18, but not IL-1β, plays a major role in suppressing colitis. Further mechanistic studies suggest that inflammasome-mediated IL-18 is critical for intestinal tissue repair and remodeling as discussed below. Moreover, injection of recombinant IL-18 could ameliorate the severity of DSS-induced colitis in inflammasome-deficient mice. These results suggest that during this chemically induced inflammation, IL-18 produced during inflammasome activation is vital for the homeostasis of the epithelial barrier in the intestinal tissues. This conclusion is further supported by studies showing that IL-18 KO or IL-18R KO mice are also highly susceptible to DSS-induced colitis and AOM/DSS-induced colon cancer (82, 83). All these studies highlight the importance of inflammasome-dependent IL-18 production in suppressing colorectal tumorigenesis.
Role of IL-18 and Microbiota in Inflammation-Associated Colon Cancer
The interaction of microbiota and the intestinal system is essential for maintaining host homeostasis and development of mucosal immunity (84–87). The human body, especially the gastrointestinal tract, is colonized by trillions of bacteria. While the commensal microorganisms are essential for the homeostasis of intestinal system and the development of host immune system, altered community representation and function of microbial species in the gut ecosystem, a state called dysbiosis, could induce intestinal inflammation and epithelial neoplasia (85, 87–95).
The commensal microbiota and bacterial products are sensed and monitored by epithelial cells and innate immune cells via innate receptors, including TLRs and NLRs. As mentioned above, inflammasome-mediated IL-18 is critical for intestinal tissue remodeling and barrier function, which has significant impacts on intestinal inflammation, gut microbiota, and even the systemic immunity. At steady state, commensal bacteria and their products induce inflammasome activation and IL-18 production in the colon that supports intestinal barrier function and prevents commensal dysbiosis (11, 25, 82, 96–98). Deficiency in inflammasome components leads to reduced production of IL-18, resulting in impaired epithelial remodeling and regeneration. Loss of barrier function causes increased commensal bacteria penetration, and enhanced inflammation, which may promote tumorigenesis and tumor growth. Dysbiosis has been observed in mice deficient for inflammasome components, including NLRP6, ASC, caspase-1, and IL-18 (25, 82, 96–99). For example, it has been proposed that NLRP6 is required for the maintenance of both composition and distribution of commensal bacteria in the gut. NLRP6 KO mice show altered microbial composition, exacerbated colitis upon chemically induced damage to the epithelial barrier, and inceased incidence of inflammation-associated colon cancer (100–102). While solid evidence has demonstrated the protective roles of inflammasomes and IL-18 in AOM/DSS-induced colon cancer, it would be informative to determine whether inflammasomes, IL-1β or IL-18 inhibit or promote colon cancer development in chronic or genetic colon cancer models as well as in human colorectal cancer patients.
Although several studies show that inflammasome deficiency leads to aberrations in microbial ecology or dysbiosis, a recent report by Mamantopoulos et al. challenges the role of inflammasomes in gut microbiota and colitis (103). Mamantopoulos et al. tested whether inflammasomes shape gut ecology by carefully analyzing NLRP6-deficient mice and littermate controls. Their results show that NLRP6 inflammasome deficiency does not affect gut microbiota composition and DSS-induced colitis when controlling for non-genetic confounders (103). This finding raises questions about previous publications related to the role of inflammasomes in controlling intestinal ecology. Therefore, further studies are required to verify whether inflammasomes shape intestinal microbiota ecology, and how the dysbiotic microbiota associated with inflammasome deficiency affect tumorigenesis and tumor progression.
Cross Talk of Inflammasome Mediators in Colitis and Colon Cancer
While numerous studies indicate the protective role of inflammasomes and IL-18 in DSS-induced colitis, recent studies demonstrate inflammasome-mediated IL-1β drives chronic inflammation in IL-10 KO mice and in human patients with IL-10R deficiency (38, 104, 105). It seems that IL-1β and IL-18 may have different roles in intestinal inflammation. As IL-1 and IL-18 are main inflammatory mediators processed by inflammasomes, it remains unclear how the host immune system integrates IL-1 and IL-18 signals during colitis and inflammation-associated colon cancer. Currently, there are a few studies on this topic. One possible mechanism is the differential transcriptional regulation of those two cytokines. Zhu and Kanneganti found that IL-18 expression was increased and sustained after TLR stimulation, whereas IL-1β expression was upregulated but not sustained upon stimulation in vitro. Furthermore, induction of IL-18 in macrophages requires type I IFN signaling (106). Interestingly, previous studies demonstrate that type I IFN suppresses transcription of IL-1β gene in macrophages (107). Additionally, IL-1β and IL-18 may influence intestinal inflammation through exerting distinct effects on T cell subsets. Accumulating evidence suggests that IL-1 drives the generation of pathogenic Th17 cells in experimental autoimmune diseases, including colitis (108–111). In contrast, Harrison et al. show that epithelial-derived IL-18 acts directly on CD4 T cells to limit colonic Th17 cell differentiation, partially through antagonizing IL-1R signaling (112). They also found that IL-18R1 signaling was critical for Foxp3 Treg cell-mediated control of colitis. These findings imply that IL-18 reduces intestinal inflammation by suppressing Th17 cells and promoting Treg function. Finally, in addition to producing active IL-1 and IL-18, inflammasome activation induces pyroptosis, the inflammatory form of programmed cell death, through gasdermin D. Currently it is unknown whether pyroptosis is involved in tumorigenesis. Recently, Wang et al. show that chemotherapy drugs induce pyroptosis through caspase-3 cleavage of gasdermin E (113). It would be interesting to know the role of pyroptosis and gasdermin D, and their relationship with IL-1 and IL-18 in tumor development and therapies.
Inflammasomes Promote Cancer Development
Skin Cancer
Several reports indicate that inflammasomes and IL-1 promote inflammation-induced skin cancer in a two-stage carcinogenesis-induced papilloma model. Drexler et al. found that IL-1R- and caspase-1-deficient mice were partially protected against skin cancer induced by 7,12-dimethylbenz(a)anthracene (DMBA)/12-O-tetradecanoylphorbol-13-acetate (TPA) treatments (114). Caspase-1 and IL-1R KO mice have a later onset and reduced incidence of tumors after DMBA/TPA treatments, compared with WT mice. In an independent study, Chow et al. also found that NLRP3 KO mice exhibited reduced skin papilloma lesions in the inflammation-induced skin cancer model (115). These data suggest that inflammasome-dependent IL-1β production contributes to the development of epithelial skin cancer. Surprisingly, Drexler et al. found that mice fully deficient for ASC displayed a similar incidence of skin papilloma as WT mice. To dissect the role of the ASC molecule, they generated conditional KO of ASC in myeloid cells and keratinocytes. Results show that mice specifically deficient for ASC in myeloid cells are protected from DMBA/TPA-induced skin cancer, suggesting a tumor-promoting role of inflammasomes and IL-1R signaling in myeloid cells. In contrast, mice specifically deficient for ASC in keratinocytes develop more tumors, compared with WT controls, suggesting that ASC suppresses tumor development in keratinocytes. Furthermore, expression of ASC protein is lost in human cutaneous squamous cell carcinoma (SCC). These results indicate that ASC has complicated roles in tumor development: ASC functions as a tumor suppressor in keratinocytes, but a tumor-promoter in myeloid cells (114). Interestingly, Okamoto et al. demonstrated that late stage human melanoma cell lines constitutively synthesize and secrete IL-1β through activated NLRP3 inflammasome, which requires no exogenous stimulation. In contrast, early stage melanoma cells need a stimulation of IL-1R to induce the production of IL-1β (116). Moreover, the production of IL-1β by melanoma cells could be inhibited by the caspase-1 inhibitor or IL-1R blockade. The authors suggest that the inflammasome/IL-1 autocrine loop contributes to the phenotype and progression of human melanoma.
A recent study unveils a very exciting finding about genetic mutations of the NLRP1 inflammasome in some skin cancers (31). There are two overlapping genetic skin disorders: multiple self-healing palmoplantar carcinoma (MSPC) and familial keratosis lichenoides chronica (FKLC), whose symptoms include numerous ulcerative, hyperkeratotic nodular growths on plantar and palmar skin. FKLC and MSPC skin lesions clinically resemble rapidly growing benign proliferative epithelial skin lesions known as keratoacanthomas, but histologically display characteristics of well-differentiated SCCs (31, 117). Furthermore, affected patients have increased susceptibility to malignant SCCs. Elegant research work from Reversade’s lab establishes a connection between genetic mutations of NLRP1 gene and increased susceptibility to skin cancer in human patients (31). To identify the genetic mutations in these two skin disorders, Zhong et al. performed whole-exome sequencing on genomic DNA isolated from affected patients, and found gain-of-mutations within the NLRP1 locus. Previous work showed that that NLRP1 is the most prominently expressed inflammasome in human skin. Functional studies of these mutations reveal that gain-of-function mutations in NLRP1 increase susceptibility to skin cancer, and a unique regulatory auto-inhibition mechanism in the NLRP1 inflammasome. In WT NLRP1, the Pyrin (PYD) and LRR domains interact with each other to inhibit its self-oligomerization. However, MSPC and FKLC associated mutations change the structure of these two domains, leading to constitutive NLRP1 self-oligomerization and inflammasome activation (31). As a result, keratinocytes from affected patients display spontaneous inflammasome activation and IL-1 secretion. Therefore, NLRP1 mutants cause skin hyperplasia via paracrine constitutive active inflammatory signaling. Together, these studies demonstrate that germline, gain-of-function NLRP1 mutations cause skin cancer and skin disorders. This is also the first study that provides genetic and functional evidence linking inflammasome mutations with cancer development.
Breast Cancer
Our recent study has demonstrated that inflammasome and IL-1β play a critical role in promoting tumor growth and metastasis in breast cancer (30). Our results show that tumor growth is associated with elevated levels of IL-1β in tumor microenvironments in mouse mammary tumor models and human breast cancer tissues. To evaluate the impact of inflammasome activities on tumor growth and metastasis, we utilized an orthotopic mammary gland tumor model in WT and inflammasome deficient mice. Compared to WT mice, primary tumor growth and lung metastasis in inflammasome-deficient mice were significantly reduced. Similarly, we found that IL-1R KO mice had reduced tumor growth after injection of breast cancer cells. Collectively, these results suggest that the inflammasome and IL-1 pathway promote tumor growth and metastases of breast cancer. Our data also show that inflammasome activation promotes the infiltration of myeloid cells such as tumor-associated macrophages (TAMs) and myeloid-derived suppressor cells. Furthermore, blocking IL-1R signaling with an IL-1R antagonist or anti-IL-1R antibody inhibits tumor growth and metastasis accompanied by decreased myeloid cell recruitment. We also found that targeting the inflammasome/IL-1 pathway leads to reduced tumor growth and metastasis in the xenograft mouse model of human breast cancer cells (30). Our results suggest that inflammasome activation and IL-1β production in TAMs provides an inflammatory microenvironment promoting breast cancer progression.
In another study, Kolb et al. show that obesity-induced NLRC4 inflammasome activation contributes to breast cancer progression (32). Obesity is one of the major risk factors for tumor development, including breast cancer. It causes metabolic and inflammatory changes that favor tumor growth and progression. Kolb et al. found that the obesity-associated tumor growth depended on caspase-1, as caspase-1 KO mice had significantly reduced tumor growth under experimental obesity conditions. In contrast, caspase-1 KO and WT mice displayed similar tumor growth under normal weight conditions. The authors further show that obesity is associated with NLRC4 inflammasome activation and IL-1β production in myeloid cells, which in turn induces VEGF and angiogenesis. Furthermore, tumor-bearing mice treated with metformin inhibit obesity-associated tumor growth (32). Currently, it is unclear how obesity is linked to NLRC4 activation in this tumor model. Nevertheless, this study establishes a causal link between obesity, inflammasome activation, and breast cancer progression.
The Complicated Roles of Inflammasomes in Other Types of Cancer
NOD-like receptors have many family members in human or mouse genome. It is not surprising that different inflammasomes may have different or opposite roles in tumor development depending on their expression patterns and tumor types. In addition to the tumors we discussed above, studies on other types of tumors are starting to emerge. For example, Daley et al. show that NLRP3 signaling drives macrophage-induced adaptive immune suppression in pancreatic carcinoma (118). Pancreatic ductal adenocarcinoma (PDA) is associated with very high mortality rate with no effective therapy options. PDA is also characterized with inflammatory and immunosuppressive tumor microenvironments (119–121). Daley et al. found that NLRP3 deletion was protective against PDA (118). Their results show that NLRP3 promotes the expansion of immunosuppressive macrophages in PDA, which inhibit antitumor T cell response. Inflammasome activation and IL-1 signaling are also implicated in the development of asbestos-induced mesothelioma (122, 123). On the other hand, Wei et al. found that inflammasomes might suppress the development of human liver cancer (124). Emerging evidence indicates that inflammasomes play an important role in non-alcoholic fatty liver disease, a spectrum of metabolic disorders ranging from steatosis (NAFL) to steatohepatitis (NASH) to cirrhosis. Moreover, hepatic steatosis or cirrhosis is strongly associated with the development of hepatocellular carcinoma (125, 126). While many studies demonstrate that inflammasomes contribute to the pathogenesis of liver diseases, some reports show that inflammasome activities can inhibit liver steatosis (99, 127, 128). Moreover, the role of inflammasomes in HCC is not well studied. By analyzing the expression of NLRP3 components in HCC tissues and corresponding non-cancerous liver tissues, Wei et al. showed that the expression of inflammasome components was significantly decreased or completely lost in human HCC tissues, and that the deficiency in inflammasome expression is positively correlated with the advanced stages of HCC (124). This correlative analysis suggests that inflammasomes may suppress the development of human liver cancer. However, further clinical and experimental studies are needed to determine the role of inflammasomes and effector cytokines in liver cancer initiation and progression.
The Inflammasome-Independent Role of AIMs and ASC in Cancer
Recent progress also indicates that there is an inflammasome-independent role of inflammasome components, particularly AIM2 and ASC, in tumor development. Man et al. showed that AIM2 suppressed colon cancer development by regulating intestinal stem cell proliferation (26). AIM2 was initially identified as a tumor suppressor in melanoma (129). Man et al. found that Aim2-deficient mice were hypersusceptible to colon cancer development, but inflammasome-associated cytokine levels were generally not affected. The results further demonstrate that deletion of AIM2 leads to the expansion of tumor-initiating stem cells through aberrant Wnt/β-catenin pathway (26). Similarly, Wilson et al. also found that there was an inflammasome-independent role of AIM2 in suppressing colon cancer development through DNA-PK and Akt signal pathways (130).
It has been shown that ASC is overexpressed or silenced in some tumors. Previous studies report aberrant methylation of ASC in breast, gastric, and prostate cancer (131, 132). There have been also studies showing that ASC inhibits tumorigenesis in primary melanoma cells by NF-κB suppression and p53/p21-related cell apoptosis (132–134). This may allude to an antitumor role behind ASC. Treating primary mouse keratinocytes or the human keratinocyte cell line with UVB-induced ASC-dependent phosphorylation of p53 and expression of p53 target genes (31). These results suggest that ASC may suppress tumor cells through induction of apoptosis or via p53 activation. It also suggests that ASC could be a novel target for anticancer therapy.
Perspectives
Although inflammasomes are essential for host defense against pathogens and contribute to autoimmune disease, their roles in tumor progression are much more complicated. The inflammasome has been shown to be either tumor promoting or tumor suppressive in various cancers. Thus, inflammasomes function as a double-edged sword in tumorigenesis as well as the anticancer immunity. We hypothesize that the exact role of an inflammasome depends on multiple factors, including its expression patterns, effector molecules, tumor types, and stages of tumor development. Tumor microenvironments and gut microbiota may also influence the function of inflammasomes and host immunity, eventually tumor progression (Figure 1). To better understand the critical role that inflammasomes in cancer, and to develop novel therapeutics, a number of questions need to be addressed: (1) how inflammasomes, such as NLRP1, NLRP3, and NLRC4, are activated during tumor growth and progression; (2) the regulation of inflammasomes by other signaling molecules or pathways and the implication of such interactions in tumor development; (3) effects of inflammasome activation in different cell types on tumor progression; (4) effects of each inflammasome pathway on host antitumor immunity and immunotherapy; and (5) dysregulation or mutations of inflammasome components in human cancer.
Our results suggest that targeting the inflammasome/IL-1 pathway in tumor microenvironments may provide a novel approach for the treatment of certain cancer, such as breast cancer. On the other hand, the immunomodulatory of inflammasome activities, particularly IL-18, can be harnessed for immunotherapy against cancer. IL-18 has been shown to play an important role in the induction of IFNγ production, increasing NK cell activity and T cell proliferation (135–140). Notably, those immunoregulatory activities of IL-18 are overlapped with that of IL-12. In fact, IL-18 and IL-12 can cooperate to induce optimal IFNγ production, Th1 responses, and NK cell activation in response to pathogens (141–143). As such, systemic injection of IL-18 protein and over-expressing IL-18 have been reported to enhance T cell response and NK cell function in several tumor models such as B16 melanoma (135–137, 141, 142, 144, 145). IL-18 also has been tested in clinical trials to treat different human tumors (146). Therefore, IL-18 alone or in combination with other therapeutic drugs may hold a promising potential for cancer treatment. Furthermore, IL-18-activated NK cells or Th1 cells could be developed as immune cell therapies against cancer.
Author Contributions
Conception, writing, and review: MK and BG.
Conflict of Interest Statement
The authors declare that the research was conducted in the absence of any commercial or financial relationships that could be construed as a potential conflict of interest.
Acknowledgments
This work was supported by the American Cancer Society Research Scholar Award (15040530), MUSC COBRE in Lipidomics and Pathobiology Pilot Grant, MUSC Hollings Cancer Center Pilot PPG, and NIH 1P01CA203628 (BG). The work was also supported in part by Hollings Cancer Center, Medical University of South Carolina (P30 CA138313).
References
1. Hanahan D, Weinberg RA. Hallmarks of cancer: the next generation. Cell (2011) 144:646–74. doi:10.1016/j.cell.2011.02.013
2. McAllister SS, Weinberg RA. Tumor-host interactions: a far-reaching relationship. J Clin Oncol (2010) 28:4022–8. doi:10.1200/JCO.2010.28.4257
3. Munn DH, Bronte V. Immune suppressive mechanisms in the tumor microenvironment. Curr Opin Immunol (2016) 39:1–6. doi:10.1016/j.coi.2015.10.009
4. Massague J, Obenauf AC. Metastatic colonization by circulating tumour cells. Nature (2016) 529:298–306. doi:10.1038/nature17038
5. Klement GL. Eco-evolution of cancer resistance. Sci Transl Med (2016) 8:327fs5. doi:10.1126/scitranslmed.aaf3802
6. Finn OJ, Beatty PL. Cancer immunoprevention. Curr Opin Immunol (2016) 39:52–8. doi:10.1016/j.coi.2016.01.002
7. Solinas G, Marchesi F, Garlanda C, Mantovani A, Allavena P. Inflammation-mediated promotion of invasion and metastasis. Cancer Metastasis Rev (2010) 29:243–8. doi:10.1007/s10555-010-9227-2
8. Hagerling C, Casbon AJ, Werb Z. Balancing the innate immune system in tumor development. Trends Cell Biol (2015) 25:214–20. doi:10.1016/j.tcb.2014.11.001
9. Gajewski TF, Schreiber H, Fu YX. Innate and adaptive immune cells in the tumor microenvironment. Nat Immunol (2013) 14:1014–22. doi:10.1038/ni.2703
10. Gonzalez-Gugel E, Saxena M, Bhardwaj N. Modulation of innate immunity in the tumor microenvironment. Cancer Immunol Immunother (2016) 65:1261–8. doi:10.1007/s00262-016-1859-9
11. Lamkanfi M, Dixit VM. Mechanisms and functions of inflammasomes. Cell (2014) 157:1013–22. doi:10.1016/j.cell.2014.04.007
12. Fuertes MB, Woo SR, Burnett B, Fu YX, Gajewski TF. Type I interferon response and innate immune sensing of cancer. Trends Immunol (2013) 34:67–73. doi:10.1016/j.it.2012.10.004
13. Nowarski R, Gagliani N, Huber S, Flavell RA. Innate immune cells in inflammation and cancer. Cancer Immunol Res (2013) 1:77–84. doi:10.1158/2326-6066.CIR-13-0081
14. Goldberg JL, Sondel PM. Enhancing cancer immunotherapy via activation of innate immunity. Semin Oncol (2015) 42:562–72. doi:10.1053/j.seminoncol.2015.05.012
15. Iannello A, Thompson TW, Ardolino M, Marcus A, Raulet DH. Immunosurveillance and immunotherapy of tumors by innate immune cells. Curr Opin Immunol (2016) 38:52–8. doi:10.1016/j.coi.2015.11.001
16. Berraondo P, Minute L, Ajona D, Corrales L, Melero I, Pio R. Innate immune mediators in cancer: between defense and resistance. Immunol Rev (2016) 274:290–306. doi:10.1111/imr.12464
17. Broz P, Monack DM. Molecular mechanisms of inflammasome activation during microbial infections. Immunol Rev (2011) 243:174–90. doi:10.1111/j.1600-065X.2011.01041.x
18. Gross O, Thomas CJ, Guarda G, Tschopp J. The inflammasome: an integrated view. Immunol Rev (2011) 243:136–51. doi:10.1111/j.1600-065X.2011.01046.x
19. Rathinam VA, Vanaja SK, Fitzgerald KA. Regulation of inflammasome signaling. Nat Immunol (2012) 13:333–42. doi:10.1038/ni.2237
20. Zitvogel L, Kepp O, Galluzzi L, Kroemer G. Inflammasomes in carcinogenesis and anticancer immune responses. Nat Immunol (2012) 13:343–51. doi:10.1038/ni.2224
21. Wen H, Ting JP, O’Neill LA. A role for the NLRP3 inflammasome in metabolic diseases – did Warburg miss inflammation? Nat Immunol (2012) 13:352–7. doi:10.1038/ni.2228
22. Zaki MH, Lamkanfi M, Kanneganti TD. The Nlrp3 inflammasome: contributions to intestinal homeostasis. Trends Immunol (2011) 32:171–9. doi:10.1016/j.it.2011.02.002
23. Davis BK, Wen H, Ting JP. The inflammasome NLRs in immunity, inflammation, and associated diseases. Annu Rev Immunol (2011) 29:707–35. doi:10.1146/annurev-immunol-031210-101405
24. Chen GY, Nunez G. Inflammasomes in intestinal inflammation and cancer. Gastroenterology (2011) 141:1986–99. doi:10.1053/j.gastro.2011.10.002
25. Allen IC, TeKippe EM, Woodford RM, Uronis JM, Holl EK, Rogers AB, et al. The NLRP3 inflammasome functions as a negative regulator of tumorigenesis during colitis-associated cancer. J Exp Med (2010) 207:1045–56. doi:10.1084/jem.20100050
26. Man SM, Zhu Q, Zhu L, Liu Z, Karki R, Malik A, et al. Critical role for the DNA sensor AIM2 in stem cell proliferation and cancer. Cell (2015) 162:45–58. doi:10.1016/j.cell.2015.06.001
27. Dupaul-Chicoine J, Arabzadeh A, Dagenais M, Douglas T, Champagne C, Morizot A, et al. The Nlrp3 inflammasome suppresses colorectal cancer metastatic growth in the liver by promoting natural killer cell tumoricidal activity. Immunity (2015) 43:751–63. doi:10.1016/j.immuni.2015.08.013
28. Hu B, Elinav E, Huber S, Booth CJ, Strowig T, Jin C, et al. Inflammation-induced tumorigenesis in the colon is regulated by caspase-1 and NLRC4. Proc Natl Acad Sci U S A (2010) 107:21635–40. doi:10.1073/pnas.1016814108
29. Dupaul-Chicoine J, Yeretssian G, Doiron K, Bergstrom KS, McIntire CR, LeBlanc PM, et al. Control of intestinal homeostasis, colitis, and colitis-associated colorectal cancer by the inflammatory caspases. Immunity (2010) 32:367–78. doi:10.1016/j.immuni.2010.02.012
30. Guo B, Fu S, Zhang J, Liu B, Li Z. Targeting inflammasome/IL-1 pathways for cancer immunotherapy. Sci Rep (2016) 6:36107. doi:10.1038/srep36107
31. Zhong FL, Mamai O, Sborgi L, Boussofara L, Hopkins R, Robinson K, et al. Germline NLRP1 mutations cause skin inflammatory and cancer susceptibility syndromes via inflammasome activation. Cell (2016) 167:187–202.e17. doi:10.1016/j.cell.2016.09.001
32. Kolb R, Phan L, Borcherding N, Liu Y, Yuan F, Janowski AM, et al. Obesity-associated NLRC4 inflammasome activation drives breast cancer progression. Nat Commun (2016) 7:13007. doi:10.1038/ncomms13007
33. Ting JP, Duncan JA, Lei Y. How the noninflammasome NLRs function in the innate immune system. Science (2010) 327:286–90. doi:10.1126/science.1184004
34. Gross O, Yazdi AS, Thomas CJ, Masin M, Heinz LX, Guarda G, et al. Inflammasome activators induce interleukin-1alpha secretion via distinct pathways with differential requirement for the protease function of caspase-1. Immunity (2012) 36:388–400. doi:10.1016/j.immuni.2012.01.018
35. Ghiringhelli F, Apetoh L, Tesniere A, Aymeric L, Ma Y, Ortiz C, et al. Activation of the NLRP3 inflammasome in dendritic cells induces IL-1beta-dependent adaptive immunity against tumors. Nat Med (2009) 15:1170–8. doi:10.1038/nm.2028
36. Cassel SL, Joly S, Sutterwala FS. The NLRP3 inflammasome: a sensor of immune danger signals. Semin Immunol (2009) 21:194–8. doi:10.1016/j.smim.2009.05.002
37. Lukens JR, Kanneganti TD. Beyond canonical inflammasomes: emerging pathways in IL-1-mediated autoinflammatory disease. Semin Immunopathol (2014) 36(5):595–609. doi:10.1007/s00281-014-0434-8
38. Zhang J, Fu S, Sun S, Li Z, Guo B. Inflammasome activation has an important role in the development of spontaneous colitis. Mucosal Immunol (2014) 7(5):1139–50. doi:10.1038/mi.2014.1
39. Guo B, Li Z. Endoplasmic reticulum stress in hepatic steatosis and inflammatory bowel diseases. Front Genet (2014) 5:242. doi:10.3389/fgene.2014.00242
40. Kawai T, Akira S. Toll-like receptors and their crosstalk with other innate receptors in infection and immunity. Immunity (2011) 34:637–50. doi:10.1016/j.immuni.2011.05.006
41. Barton GM, Medzhitov R. Toll-like receptor signaling pathways. Science (2003) 300:1524–5. doi:10.1126/science.1085536
42. Lamkanfi M. Emerging inflammasome effector mechanisms. Nat Rev Immunol (2011) 11:213–20. doi:10.1038/nri2936
43. Rathinam VA, Fitzgerald KA. Inflammasome complexes: emerging mechanisms and effector functions. Cell (2016) 165:792–800. doi:10.1016/j.cell.2016.03.046
44. Leemans JC, Cassel SL, Sutterwala FS. Sensing damage by the NLRP3 inflammasome. Immunol Rev (2011) 243:152–62. doi:10.1111/j.1600-065X.2011.01043.x
45. Kayagaki N, Warming S, Lamkanfi M, Vande Walle L, Louie S, Dong J, et al. Non-canonical inflammasome activation targets caspase-11. Nature (2011) 479:117–21. doi:10.1038/nature10558
46. Liu X, Zhang Z, Ruan J, Pan Y, Magupalli VG, Wu H, et al. Inflammasome-activated gasdermin D causes pyroptosis by forming membrane pores. Nature (2016) 535:153–8. doi:10.1038/nature18629
47. Ding J, Wang K, Liu W, She Y, Sun Q, Shi J, et al. Pore-forming activity and structural autoinhibition of the gasdermin family. Nature (2016) 535:111–6. doi:10.1038/nature18590
48. Kayagaki N, Stowe IB, Lee BL, O’Rourke K, Anderson K, Warming S, et al. Caspase-11 cleaves gasdermin D for non-canonical inflammasome signalling. Nature (2015) 526:666–71. doi:10.1038/nature15541
49. Hornung V, Ablasser A, Charrel-Dennis M, Bauernfeind F, Horvath G, Caffrey DR, et al. AIM2 recognizes cytosolic dsDNA and forms a caspase-1-activating inflammasome with ASC. Nature (2009) 458:514–8. doi:10.1038/nature07725
50. Fernandes-Alnemri T, Yu JW, Datta P, Wu J, Alnemri ES. AIM2 activates the inflammasome and cell death in response to cytoplasmic DNA. Nature (2009) 458:509–13. doi:10.1038/nature07710
51. Fernandes-Alnemri T, Yu JW, Juliana C, Solorzano L, Kang S, Wu J, et al. The AIM2 inflammasome is critical for innate immunity to Francisella tularensis. Nat Immunol (2010) 11:385–93. doi:10.1038/ni.1859
52. Connolly DJ, Bowie AG. The emerging role of human PYHIN proteins in innate immunity: implications for health and disease. Biochem Pharmacol (2014) 92:405–14. doi:10.1016/j.bcp.2014.08.031
53. Diner BA, Lum KK, Cristea IM. The emerging role of nuclear viral DNA sensors. J Biol Chem (2015) 290:26412–21. doi:10.1074/jbc.R115.652289
54. Luecke S, Paludan SR. Innate recognition of alphaherpesvirus DNA. Adv Virus Res (2015) 92:63–100. doi:10.1016/bs.aivir.2014.11.003
55. Schattgen SA, Fitzgerald KA. The PYHIN protein family as mediators of host defenses. Immunol Rev (2011) 243:109–18. doi:10.1111/j.1600-065X.2011.01053.x
56. Choubey D. Absent in melanoma 2 proteins in the development of cancer. Cell Mol Life Sci (2016) 73:4383–95. doi:10.1007/s00018-016-2296-9
57. Choubey D, Panchanathan R. Absent in melanoma 2 proteins in SLE. Clin Immunol (2017) 176:42–8. doi:10.1016/j.clim.2016.12.011
58. Man SM, Karki R, Kanneganti TD. AIM2 inflammasome in infection, cancer, and autoimmunity: role in DNA sensing, inflammation, and innate immunity. Eur J Immunol (2016) 46:269–80. doi:10.1002/eji.201545839
59. Masters SL. Specific inflammasomes in complex diseases. Clin Immunol (2013) 147:223–8. doi:10.1016/j.clim.2012.12.006
60. Smith S, Jefferies C. Role of DNA/RNA sensors and contribution to autoimmunity. Cytokine Growth Factor Rev (2014) 25:745–57. doi:10.1016/j.cytogfr.2014.07.019
61. Xiao TS. The nucleic acid-sensing inflammasomes. Immunol Rev (2015) 265:103–11. doi:10.1111/imr.12281
62. Ogura Y, Bonen DK, Inohara N, Nicolae DL, Chen FF, Ramos R, et al. A frameshift mutation in NOD2 associated with susceptibility to Crohn’s disease. Nature (2001) 411:603–6. doi:10.1038/35079114
63. Hugot JP, Chamaillard M, Zouali H, Lesage S, Cezard JP, Belaiche J, et al. Association of NOD2 leucine-rich repeat variants with susceptibility to Crohn’s disease. Nature (2001) 411:599–603. doi:10.1038/35079107
64. Schroder K, Zhou R, Tschopp J. The NLRP3 inflammasome: a sensor for metabolic danger? Science (2010) 327:296–300. doi:10.1126/science.1184003
65. Agostini L, Martinon F, Burns K, McDermott MF, Hawkins PN, Tschopp J. NALP3 forms an IL-1beta-processing inflammasome with increased activity in Muckle-Wells autoinflammatory disorder. Immunity (2004) 20:319–25. doi:10.1016/S1074-7613(04)00046-9
66. Papin S, Cuenin S, Agostini L, Martinon F, Werner S, Beer HD, et al. The SPRY domain of pyrin, mutated in familial mediterranean fever patients, interacts with inflammasome components and inhibits proIL-1beta processing. Cell Death Differ (2007) 14:1457–66. doi:10.1038/sj.cdd.4402142
67. Petrilli V, Dostert C, Muruve DA, Tschopp J. The inflammasome: a danger sensing complex triggering innate immunity. Curr Opin Immunol (2007) 19:615–22. doi:10.1016/j.coi.2007.09.002
68. Broderick L, De Nardo D, Franklin BS, Hoffman HM, Latz E. The inflammasomes and autoinflammatory syndromes. Annu Rev Pathol (2015) 10:395–424. doi:10.1146/annurev-pathol-012414-040431
69. Stienstra R, Tack CJ, Kanneganti TD, Joosten LA, Netea MG. The inflammasome puts obesity in the danger zone. Cell Metab (2012) 15:10–8. doi:10.1016/j.cmet.2011.10.011
70. Lamkanfi M, Walle LV, Kanneganti TD. Deregulated inflammasome signaling in disease. Immunol Rev (2011) 243:163–73. doi:10.1111/j.1600-065X.2011.01042.x
71. Papatriantafyllou M. Mucosal immunology: inflammasome shapes the microbiota. Nat Rev Immunol (2011) 11:439. doi:10.1038/nri3012
72. Weber A, Wasiliew P, Kracht M. Interleukin-1 (IL-1) pathway. Sci Signal (2010) 3:cm1. doi:10.1126/scisignal.3105cm1
73. Lopalco G, Cantarini L, Vitale A, Iannone F, Anelli MG, Andreozzi L, et al. Interleukin-1 as a common denominator from autoinflammatory to autoimmune disorders: premises, perils, and perspectives. Mediators Inflamm (2015) 2015:194864. doi:10.1155/2015/194864
74. van de Veerdonk FL, Netea MG. New insights in the immunobiology of IL-1 family members. Front Immunol (2013) 4:167. doi:10.3389/fimmu.2013.00167
75. Palomo J, Dietrich D, Martin P, Palmer G, Gabay C. The interleukin (IL)-1 cytokine family – balance between agonists and antagonists in inflammatory diseases. Cytokine (2015) 76:25–37. doi:10.1016/j.cyto.2015.06.017
76. Mora J, Weigert A. IL-1 family cytokines in cancer immunity – a matter of life and death. Biol Chem (2016) 397:1125–34. doi:10.1515/hsz-2016-0215
77. Jin L, Yuan RQ, Fuchs A, Yao Y, Joseph A, Schwall R, et al. Expression of interleukin-1beta in human breast carcinoma. Cancer (1997) 80:421–34. doi:10.1002/(SICI)1097-0142(19970801)80:3<421::AID-CNCR10>3.0.CO;2-Z
78. Pantschenko AG, Pushkar I, Anderson KH, Wang Y, Miller LJ, Kurtzman SH, et al. The interleukin-1 family of cytokines and receptors in human breast cancer: implications for tumor progression. Int J Oncol (2003) 23:269–84. doi:10.3892/ijo.23.2.269
79. Perrier S, Caldefie-Chezet F, Vasson MP. IL-1 family in breast cancer: potential interplay with leptin and other adipocytokines. FEBS Lett (2009) 583:259–65. doi:10.1016/j.febslet.2008.12.030
80. Valdivia-Silva JE, Franco-Barraza J, Silva AL, Pont GD, Soldevila G, Meza I, et al. Effect of pro-inflammatory cytokine stimulation on human breast cancer: implications of chemokine receptor expression in cancer metastasis. Cancer Lett (2009) 283:176–85. doi:10.1016/j.canlet.2009.03.040
81. Hu B, Elinav E, Flavell RA. Inflammasome-mediated suppression of inflammation-induced colorectal cancer progression is mediated by direct regulation of epithelial cell proliferation. Cell Cycle (2011) 10:1936–9. doi:10.4161/cc.10.12.16008
82. Zaki MH, Vogel P, Body-Malapel M, Lamkanfi M, Kanneganti TD. IL-18 production downstream of the Nlrp3 inflammasome confers protection against colorectal tumor formation. J Immunol (2010) 185:4912–20. doi:10.4049/jimmunol.1002046
83. Salcedo R, Worschech A, Cardone M, Jones Y, Gyulai Z, Dai RM, et al. MyD88-mediated signaling prevents development of adenocarcinomas of the colon: role of interleukin 18. J Exp Med (2010) 207:1625–36. doi:10.1084/jem.20100199
84. Belkaid Y, Harrison OJ. Homeostatic immunity and the microbiota. Immunity (2017) 46:562–76. doi:10.1016/j.immuni.2017.04.008
85. Grigg JB, Sonnenberg GF. Host-microbiota interactions shape local and systemic inflammatory diseases. J Immunol (2017) 198:564–71. doi:10.4049/jimmunol.1601621
86. Gorjifard S, Goldszmid RS. Microbiota-myeloid cell crosstalk beyond the gut. J Leukoc Biol (2016) 100:865–79. doi:10.1189/jlb.3RI0516-222R
87. Baumler AJ, Sperandio V. Interactions between the microbiota and pathogenic bacteria in the gut. Nature (2016) 535:85–93. doi:10.1038/nature18849
88. Lane ER, Zisman TL, Suskind DL. The microbiota in inflammatory bowel disease: current and therapeutic insights. J Inflamm Res (2017) 10:63–73. doi:10.2147/JIR.S116088
89. Blander JM, Longman RS, Iliev ID, Sonnenberg GF, Artis D. Regulation of inflammation by microbiota interactions with the host. Nat Immunol (2017) 18:851–60. doi:10.1038/ni.3780
90. Roy S, Trinchieri G. Microbiota: a key orchestrator of cancer therapy. Nat Rev Cancer (2017) 17:271–85. doi:10.1038/nrc.2017.13
91. Ni J, Wu GD, Albenberg L, Tomov VT. Gut microbiota and IBD: causation or correlation? Nat Rev Gastroenterol Hepatol (2017). doi:10.1038/nrgastro.2017.88
92. Honda K, Littman DR. The microbiota in adaptive immune homeostasis and disease. Nature (2016) 535:75–84. doi:10.1038/nature18848
93. Chen J, Domingue JC, Sears CL. Microbiota dysbiosis in select human cancers: evidence of association and causality. Semin Immunol (2017). doi:10.1016/j.smim.2017.08.001
94. Kang M, Martin A. Microbiome and colorectal cancer: unraveling host-microbiota interactions in colitis-associated colorectal cancer development. Semin Immunol (2017). doi:10.1016/j.smim.2017.04.003
95. Pope JL, Tomkovich S, Yang Y, Jobin C. Microbiota as a mediator of cancer progression and therapy. Transl Res (2017) 179:139–54. doi:10.1016/j.trsl.2016.07.021
96. Chen GY, Liu M, Wang F, Bertin J, Nunez G. A functional role for Nlrp6 in intestinal inflammation and tumorigenesis. J Immunol (2011) 186:7187–94. doi:10.4049/jimmunol.1100412
97. Williams TM, Leeth RA, Rothschild DE, Coutermarsh-Ott SL, McDaniel DK, Simmons AE, et al. The NLRP1 inflammasome attenuates colitis and colitis-associated tumorigenesis. J Immunol (2015) 194:3369–80. doi:10.4049/jimmunol.1402098
98. Zaki MH, Boyd KL, Vogel P, Kastan MB, Lamkanfi M, Kanneganti TD. The NLRP3 inflammasome protects against loss of epithelial integrity and mortality during experimental colitis. Immunity (2010) 32:379–91. doi:10.1016/j.immuni.2010.03.003
99. Henao-Mejia J, Elinav E, Jin C, Hao L, Mehal WZ, Strowig T, et al. Inflammasome-mediated dysbiosis regulates progression of NAFLD and obesity. Nature (2012) 482:179–85. doi:10.1038/nature10809
100. Elinav E, Strowig T, Kau AL, Henao-Mejia J, Thaiss CA, Booth CJ, et al. NLRP6 inflammasome regulates colonic microbial ecology and risk for colitis. Cell (2011) 145:745–57. doi:10.1016/j.cell.2011.04.022
101. Levy M, Thaiss CA, Zeevi D, Dohnalova L, Zilberman-Schapira G, Mahdi JA, et al. Microbiota-modulated metabolites shape the intestinal microenvironment by regulating NLRP6 inflammasome signaling. Cell (2015) 163:1428–43. doi:10.1016/j.cell.2015.10.048
102. Levy M, Shapiro H, Thaiss CA, Elinav E. NLRP6: a multifaceted innate immune sensor. Trends Immunol (2017) 38:248–60. doi:10.1016/j.it.2017.01.001
103. Mamantopoulos M, Ronchi F, Van Hauwermeiren F, Vieira-Silva S, Yilmaz B, Martens L, et al. Nlrp6- and ASC-dependent inflammasomes do not shape the commensal gut microbiota composition. Immunity (2017) 47:339–48.e4. doi:10.1016/j.immuni.2017.07.011
104. Shouval DS, Biswas A, Kang YH, Griffith AE, Konnikova L, Mascanfroni ID, et al. Interleukin 1beta mediates intestinal inflammation in mice and patients with interleukin 10 receptor deficiency. Gastroenterology (2016) 151:1100–4. doi:10.1053/j.gastro.2016.08.055
105. Ip WKE, Hoshi N, Shouval DS, Snapper S, Medzhitov R. Anti-inflammatory effect of IL-10 mediated by metabolic reprogramming of macrophages. Science (2017) 356:513–9. doi:10.1126/science.aal3535
106. Zhu Q, Kanneganti TD. Cutting edge: distinct regulatory mechanisms control proinflammatory cytokines IL-18 and IL-1beta. J Immunol (2017) 198:4210–5. doi:10.4049/jimmunol.1700352
107. Chang EY, Guo B, Doyle SE, Cheng G. Cutting edge: involvement of the type I IFN production and signaling pathway in lipopolysaccharide-induced IL-10 production. J Immunol (2007) 178:6705–9. doi:10.4049/jimmunol.178.11.6705
108. Coccia M, Harrison OJ, Schiering C, Asquith MJ, Becher B, Powrie F, et al. IL-1beta mediates chronic intestinal inflammation by promoting the accumulation of IL-17A secreting innate lymphoid cells and CD4(+) Th17 cells. J Exp Med (2012) 209:1595–609. doi:10.1084/jem.20111453
109. Wilke CM, Wang L, Wei S, Kryczek I, Huang E, Kao J, et al. Endogenous interleukin-10 constrains Th17 cells in patients with inflammatory bowel disease. J Transl Med (2011) 9:217. doi:10.1186/1479-5876-9-217
110. Koenders MI, Devesa I, Marijnissen RJ, Abdollahi-Roodsaz S, Boots AM, Walgreen B, et al. Interleukin-1 drives pathogenic Th17 cells during spontaneous arthritis in interleukin-1 receptor antagonist-deficient mice. Arthritis Rheum (2008) 58:3461–70. doi:10.1002/art.23957
111. Guo B. IL-10 modulates Th17 pathogenicity during autoimmune diseases. J Clin Cell Immunol (2016) 7:400. doi:10.4172/2155-9899.1000400
112. Harrison OJ, Srinivasan N, Pott J, Schiering C, Krausgruber T, Ilott NE, et al. Epithelial-derived IL-18 regulates Th17 cell differentiation and Foxp3(+) Treg cell function in the intestine. Mucosal Immunol (2015) 8:1226–36. doi:10.1038/mi.2015.13
113. Wang Y, Gao W, Shi X, Ding J, Liu W, He H, et al. Chemotherapy drugs induce pyroptosis through caspase-3 cleavage of a gasdermin. Nature (2017) 547:99–103. doi:10.1038/nature22393
114. Drexler SK, Bonsignore L, Masin M, Tardivel A, Jackstadt R, Hermeking H, et al. Tissue-specific opposing functions of the inflammasome adaptor ASC in the regulation of epithelial skin carcinogenesis. Proc Natl Acad Sci U S A (2012) 109:18384–9. doi:10.1073/pnas.1209171109
115. Chow MT, Tschopp J, Moller A, Smyth MJ. NLRP3 promotes inflammation-induced skin cancer but is dispensable for asbestos-induced mesothelioma. Immunol Cell Biol (2012) 90:983–6. doi:10.1038/icb.2012.46
116. Okamoto M, Liu W, Luo Y, Tanaka A, Cai X, Norris DA, et al. Constitutively active inflammasome in human melanoma cells mediating autoinflammation via caspase-1 processing and secretion of interleukin-1beta. J Biol Chem (2010) 285:6477–88. doi:10.1074/jbc.M109.064907
117. Mamai O, Boussofara L, Denguezli M, Escande-Beillard N, Kraeim W, Merriman B, et al. Multiple self-healing palmoplantar carcinoma: a familial predisposition to skin cancer with primary palmoplantar and conjunctival lesions. J Invest Dermatol (2015) 135:304–8. doi:10.1038/jid.2014.311
118. Daley D, Mani VR, Mohan N, Akkad N, Pandian G, Savadkar S, et al. NLRP3 signaling drives macrophage-induced adaptive immune suppression in pancreatic carcinoma. J Exp Med (2017) 214:1711–24. doi:10.1084/jem.20161707
119. Weledji EP, Enoworock G, Mokake M, Sinju M. How grim is pancreatic cancer? Oncol Rev (2016) 10:294. doi:10.4081/oncol.2016.294
120. Khan MA, Azim S, Zubair H, Bhardwaj A, Patel GK, Khushman M, et al. Molecular drivers of pancreatic cancer pathogenesis: looking inward to move forward. Int J Mol Sci (2017) 18:E779. doi:10.3390/ijms18040779
121. Khalafalla FG, Khan MW. Inflammation and epithelial-mesenchymal transition in pancreatic ductal adenocarcinoma: fighting against multiple opponents. Cancer Growth Metastasis (2017) 10:1179064417709287. doi:10.1177/1179064417709287
122. Kadariya Y, Menges CW, Talarchek J, Cai KQ, Klein-Szanto AJ, Pietrofesa RA, et al. Inflammation-related IL1beta/IL1R signaling promotes the development of asbestos-induced malignant mesothelioma. Cancer Prev Res (Phila) (2016) 9:406–14. doi:10.1158/1940-6207.CAPR-15-0347
123. Thompson JK, MacPherson MB, Beuschel SL, Shukla A. Asbestos-induced mesothelial to fibroblastic transition is modulated by the inflammasome. Am J Pathol (2017) 187:665–78. doi:10.1016/j.ajpath.2016.11.008
124. Wei Q, Mu K, Li T, Zhang Y, Yang Z, Jia X, et al. Deregulation of the NLRP3 inflammasome in hepatic parenchymal cells during liver cancer progression. Lab Invest (2014) 94:52–62. doi:10.1038/labinvest.2013.126
125. Musso G, Cassader M, Gambino R. Non-alcoholic steatohepatitis: emerging molecular targets and therapeutic strategies. Nat Rev Drug Discov (2016) 15:249–74. doi:10.1038/nrd.2015.3
126. Machado MV, Diehl AM. Pathogenesis of nonalcoholic steatohepatitis. Gastroenterology (2016) 150:1769–77. doi:10.1053/j.gastro.2016.02.066
127. Zhang J, Zhang K, Li Z, Guo B. ER stress-induced inflammasome activation contributes to hepatic inflammation and steatosis. J Clin Cell Immunol (2016) 7:457. doi:10.4172/2155-9899.1000457
128. Imaeda AB, Watanabe A, Sohail MA, Mahmood S, Mohamadnejad M, Sutterwala FS, et al. Acetaminophen-induced hepatotoxicity in mice is dependent on Tlr9 and the Nalp3 inflammasome. J Clin Invest (2009) 119:305–14. doi:10.1172/JCI35958
129. DeYoung KL, Ray ME, Su YA, Anzick SL, Johnstone RW, Trapani JA, et al. Cloning a novel member of the human interferon-inducible gene family associated with control of tumorigenicity in a model of human melanoma. Oncogene (1997) 15:453–7. doi:10.1038/sj.onc.1201206
130. Wilson JE, Petrucelli AS, Chen L, Koblansky AA, Truax AD, Oyama Y, et al. Inflammasome-independent role of AIM2 in suppressing colon tumorigenesis via DNA-PK and Akt. Nat Med (2015) 21:906–13. doi:10.1038/nm.3908
131. Das PM, Ramachandran K, Vanwert J, Ferdinand L, Gopisetty G, Reis IM, et al. Methylation mediated silencing of TMS1/ASC gene in prostate cancer. Mol Cancer (2006) 5:28. doi:10.1186/1476-4598-5-28
132. Levine JJ, Stimson-Crider KM, Vertino PM. Effects of methylation on expression of TMS1/ASC in human breast cancer cells. Oncogene (2003) 22:3475–88. doi:10.1038/sj.onc.1206430
133. Wu L, Zhang C, Wang X, Ding X, Deng J, Liang H. Methylation of ASC/TMS1 promoter is associated with poor prognosis of patients with gastric cancer. Clin Transl Oncol (2016) 18:296–303. doi:10.1007/s12094-015-1367-y
134. Liu W, Luo Y, Dunn JH, Norris DA, Dinarello CA, Fujita M. Dual role of apoptosis-associated speck-like protein containing a CARD (ASC) in tumorigenesis of human melanoma. J Invest Dermatol (2013) 133:518–27. doi:10.1038/jid.2012.317
135. Xu B, Dong CY, Zhang F, Lin YM, Wu KF, Ma XT. Synergistic antileukemia effect of combinational gene therapy using murine beta-defensin 2 and IL-18 in L1210 murine leukemia model. Gene Ther (2007) 14:1181–7. doi:10.1038/sj.gt.3302966
136. Tse BW, Russell PJ, Lochner M, Forster I, Power CA. IL-18 inhibits growth of murine orthotopic prostate carcinomas via both adaptive and innate immune mechanisms. PLoS One (2011) 6:e24241. doi:10.1371/journal.pone.0024241
137. Wong JL, Berk E, Edwards RP, Kalinski P. IL-18-primed helper NK cells collaborate with dendritic cells to promote recruitment of effector CD8+ T cells to the tumor microenvironment. Cancer Res (2013) 73:4653–62. doi:10.1158/0008-5472.CAN-12-4366
138. Akira S. The role of IL-18 in innate immunity. Curr Opin Immunol (2000) 12:59–63. doi:10.1016/S0952-7915(99)00051-5
139. Chaix J, Tessmer MS, Hoebe K, Fuseri N, Ryffel B, Dalod M, et al. Cutting edge: priming of NK cells by IL-18. J Immunol (2008) 181:1627–31. doi:10.4049/jimmunol.181.3.1627
140. Takeda K, Tsutsui H, Yoshimoto T, Adachi O, Yoshida N, Kishimoto T, et al. Defective NK cell activity and Th1 response in IL-18-deficient mice. Immunity (1998) 8:383–90. doi:10.1016/S1074-7613(00)80543-9
141. Balkow S, Loser K, Krummen M, Higuchi T, Rothoeft T, Apelt J, et al. Dendritic cell activation by combined exposure to anti-CD40 plus interleukin (IL)-12 and IL-18 efficiently stimulates anti-tumor immunity. Exp Dermatol (2009) 18:78–87. doi:10.1111/j.1600-0625.2008.00800.x
142. Mirjacic Martinovic K, Babovic N, Dzodic R, Jurisic V, Matkovic S, Konjevic G. Favorable in vitro effects of combined IL-12 and IL-18 treatment on NK cell cytotoxicity and CD25 receptor expression in metastatic melanoma patients. J Transl Med (2015) 13:120. doi:10.1186/s12967-015-0479-z
143. Nielsen CM, Wolf AS, Goodier MR, Riley EM. Synergy between common gamma chain family cytokines and IL-18 potentiates innate and adaptive pathways of NK cell activation. Front Immunol (2016) 7:101. doi:10.3389/fimmu.2016.00101
144. Choi IK, Lee JS, Zhang SN, Park J, Sonn CH, Lee KM, et al. Oncolytic adenovirus co-expressing IL-12 and IL-18 improves tumor-specific immunity via differentiation of T cells expressing IL-12Rbeta2 or IL-18Ralpha. Gene Ther (2011) 18:898–909. doi:10.1038/gt.2011.37
145. Wong JL, Muthuswamy R, Bartlett DL, Kalinski P. IL-18-based combinatorial adjuvants promote the intranodal production of CCL19 by NK cells and dendritic cells of cancer patients. Oncoimmunology (2013) 2:e26245. doi:10.4161/onci.26245
Keywords: inflammasome, tumor microenvironments, NOD-like receptors, IL-1, tumor, inflammation, immunotherapy
Citation: Kantono M and Guo B (2017) Inflammasomes and Cancer: The Dynamic Role of the Inflammasome in Tumor Development. Front. Immunol. 8:1132. doi: 10.3389/fimmu.2017.01132
Received: 29 June 2017; Accepted: 28 August 2017;
Published: 12 September 2017
Edited by:
Masoud H. Manjili, Virginia Commonwealth University, United StatesReviewed by:
Tsan Sam Xiao, Case Western Reserve University, United StatesKyle K. Payne, Wistar Institute, United States
Copyright: © 2017 Kantono and Guo. This is an open-access article distributed under the terms of the Creative Commons Attribution License (CC BY). The use, distribution or reproduction in other forums is permitted, provided the original author(s) or licensor are credited and that the original publication in this journal is cited, in accordance with accepted academic practice. No use, distribution or reproduction is permitted which does not comply with these terms.
*Correspondence: Beichu Guo, guobe@musc.edu