- 1Health Sciences North Research Institute, Sudbury, ON, Canada
- 2Second Department of Internal Medicine, University of Tübingen Medical Center, Tübingen, Germany
- 3UConn Center on Aging, UConn Health, Farmington, CT, United States
The number of people over the age of 60 is expected to double by 2050 according to the WHO. This emphasizes the need to ensure optimized resilience to health stressors in late life. In older adults, influenza is one of the leading causes of catastrophic disability (defined as the loss of independence in daily living and self-care activities). Influenza vaccination is generally perceived to be less protective in older adults, with some studies suggesting that the humoral immune response to the vaccine is further impaired in cytomegalovirus (CMV)-seropositive older people. CMV is a β-herpes virus infection that is generally asymptomatic in healthy individuals. The majority of older adults possess serum antibodies against the virus indicating latent infection. Age-related changes in T-cell-mediated immunity are augmented by CMV infection and may be associated with more serious complications of influenza infection. This review focuses on the impact of aging and CMV on immune cell function, the response to influenza infection and vaccination, and how the current understanding of aging and CMV can be used to design a more effective influenza vaccine for older adults. It is anticipated that efforts in this field will address the public health need for improved protection against influenza in older adults, particularly with regard to the serious complications leading to loss of independence.
Introduction
Cytomegalovirus (CMV) is a β-herpes virus that infects fibroblasts, epithelial, endothelial, stromal, smooth muscle cells, but most importantly, monocytes and dendritic cells (DCs) (1). Depending on the country and its state of development, 25–90% of the worldwide population is CMV seropositive (2, 3) with prevalence higher in older adults (4). Once infected with CMV, the immune system is unable to eliminate the virus, resulting in persistent latent infection. While the contribution of CMV infection to features of immune senescence are well recognized (5, 6), the translation to predicting outcomes in older adults has been much more challenging. Earlier reports of the association between CMV seropositivity and prevalent frailty in community-dwelling older women (7, 8) (frailty determined based on a five component measure: unintentional weight loss, weak grip strength, exhaustion, slow walking speed, and low level of activity) have not always been replicated in more recent longitudinal studies of CMV seropositivity as a predictor of frailty as measured by grip strength (9). However, many other studies have reported an association between CMV seropositivity and frailty (5, 6, 8, 10, 11), and increased mortality (12–15), but these findings are not consistent across all age groups and under all conditions. For example, in the BELFRAIL study, CMV seropositivity was not associated with an increased risk for all-cause mortality in a cohort of very old people. This may have been the result of a survival effect, whereby CMV-seropositive subjects with high anti-CMV titers die at a younger age compared with other individuals. This may reflect CMV reactivation being more common in the end stages of life (15). In terms of the impact of CMV on immune function, CMV seropositivity has been linked to poor CD4+ T-cell responses to influenza internal proteins (16), while other studies have found no association between CMV pp65-reactive CD8+ T-cells and poor CD8+ T-cell responses to influenza internal proteins (17). Although CMV seropositivity in older adults has never been directly correlated with poor vaccine-mediated protection in older adults, high levels of CMV-reactive CD4+ T-cells have been associated with an increased risk of viral respiratory illness in elderly nursing home residents (18) and predict increased morbidity and mortality.
Cytomegalovirus and aging of the immune system are associated with oligoclonal expansions of CD8+ T-cells, possibly due to an increase in the frequency and magnitude of reactivation of CMV in older compared to young adults (19). At the same time, CMV IgG titers and viral load increase markedly with age (20). These findings suggest that while older adults are able to contain CMV, they do so at the cost of investing ever-increasing resources to control this single pathogen, with the result that immune responses to other challenges may be reduced (21).
Influenza is a single-stranded negative-sense RNA virus that is transmitted through the air by coughing and sneezing and infects epithelial cells, usually in the nose, throat, and lungs. The virus has a major impact on the aging population; ≥90% of annual influenza-related deaths occur in individuals ≥65 years of age (22). Often, influenza itself is not the cause of death, but rather it predisposes older adults to develop secondary bacterial infections and exacerbations of preexisting medical conditions (23, 24). Furthermore, older adults represent the majority of individuals hospitalized with influenza illness (25), which raises concern as hospitalization itself is often followed by a decline in the ability to perform activities of daily living for individuals in this age group (26). Additionally, influenza-related hospitalizations have a significant economic and social impact (27). Although antiviral drugs against influenza are available, vaccination continues to be the most effective method to control infection (28). Prevention of influenza illness through vaccination aids in reducing the burden on the health-care system and maintaining the quality of life of older adults. Hospitalization rates for influenza remain high (25) in spite of evidence that vaccination campaigns can reduce such events (29). Furthermore, multiple impairments associated with CMV and aging appear to lessen the effectiveness of influenza vaccination and reduce the ability to respond to influenza infection to prevent serious complications (30, 31). Recently, it has been shown that influenza vaccination provides good protection against influenza-related hospitalization, but vaccine effectiveness declines as frailty (using the Frailty Index) increases in older adults (32).
The Role of Antigen-Presenting Cells (APCs) in Influenza Infection and Vaccination
Macrophages and DCs play an important role in directing the immune response to the site of infection. These cells act as APCs and modulate the innate and adaptive immune response. Macrophages initiate the inflammatory responses, while activation of DCs is required for the induction of adaptive immunity.
There are two major categories of macrophages: M1 macrophages, which are induced by Th1 cytokines (IFN-γ and TNF-α); and M2 macrophages, induced by Th2 cytokines (including IL-4 and IL-13). M1 macrophages are characterized by the production of IL-1, IL-12, and TNF-α. In addition, M1 macrophages drive Th1 responses. Aging is characterized by an elevation in baseline inflammatory factors in blood, contributing to a skewed M1/M2 macrophage distribution (32, 33). Specifically, monocytes (macrophage precursors) obtained from older adults prior to influenza vaccination exhibit impaired function with decreased TNF-α and IL-6 secretion, but intact IL-10 responses (34). The dysregulation of IL-10 production from monocytes suggests its potential role in impaired influenza vaccine responses in older adults (34). This dysregulation of TNF-α and IL-6 vs. IL-10 response to influenza vaccination has been linked to the downregulation of the expression of the costimulatory molecules CD80 and CD86 by activated monocytes as a predictor of the antibody response to influenza vaccination (35). A link between CMV infection and dysregulation of DC function may be provided by the finding that CMV itself encodes an IL-10 ortholog, which is known to be expressed during latent infection of myeloid precursor cells (36). CMV-IL-10 inhibits DC function by hindering their maturation and functionality (37) and hence may also play a role in poorer responses to vaccination. Furthermore, plasmacytoid DCs from the elderly are also impaired and produce less TNF-α/IFN-γ in response to TLR7 and TLR9 stimulation (38), which has been associated with poor antibody response to influenza vaccination as well.
The Role of B-Cells in Influenza Infection and Vaccination
As noted above, CMV may act as an environmental amplifier of immunosenescence resulting in the accumulation of large amounts of late-differentiated CMV-specific effector T-cells (39, 40) and possibly contributing to inflammation. CMV seropositivity is also associated with intrinsically mediated increased levels of inflammatory cytokines in B-cells and diminished B-cell function that predicts poor antibody responses to influenza vaccination (41).
The B-cell response to vaccination decreases with age (42–44) and the compromised effector function of B-cells in the elderly results in lower antibody production and poor Ig class switching (45). While the intrinsic deficits found in B-cells as a result of aging are limited, they are mostly associated with lower levels of long-lived plasmablasts (46–48) and memory B-cells (46, 48, 49). Studies have shown that the age-related decrease in antibody response to influenza vaccination is correlated with extrinsic factors, including impaired T-cell help (39, 40), poor DC function (38), and high IL-10 production by monocytes/macrophages (34) as discussed above.
Influenza vaccines function by generating a B-cell and follicular helper T-cell (TFH) response, which in turn results in the proliferation of vaccine antigen-specific B-cells (50, 51). It is believed that IgA and IgM specific for viral hemagglutinin (HA) protect against the establishment of initial infection though neutralization of the virus, while IgG antibodies against nucleoprotein (NP) neutralize the virus if infection becomes established (52, 53).
B-cell defects associated with aging include reduced activation-induced cytidine deaminase (AID). AID is an enzyme required for class switch recombination as well as somatic hypermutation (54) and has been found to correlate with IgG production (55). Prior to vaccination, AID mRNA levels and switched memory B-cell frequencies in response to CpG stimulation correlate with the serum antibody response and are thus predictors of the response to both vaccination and infection. In this context, older adults who are seropositive for CMV show a reduction in both AID and switched memory B-cells relative to CMV seronegatives, and a correspondingly diminished antibody response to influenza A/H1N1 strains.
Another biomarker of B-cell functionality is intracellular TNF-α, which correlates with serum TNF-α levels, which are elevated in the elderly, and more so in CMV seropositives. TNF-α places B-cells in a status of preactivation, which impairs functionality (41). Although the exact mechanism involved in reduced B-cell function by CMV is not known, it may involve a TNF-α feedback loop. Specifically, CMV induces increased production of TNF-α in B-cells via NF-κB induction (56). This results in a systemic elevation of TNF-α levels and contributes to CMV-associated B-cell activation, systemic inflammation, and reduced function in these older individuals (41). The important role of TNF-α is further illustrated in B-cell cultures in which TNF-α is neutralized, resulting in improved antibody class switching in elderly individuals (57).
Ambiguity of the Role of CMV and Aging on the Antibody Response to Influenza Vaccination
Some reports indicate that CMV seropositivity may be associated with better antibody response to vaccination in younger adults (58). This is different in studies involving older adults, where CMV seropositivity has been variably found to be associated with beneficial (59), negative (41, 58–62), or negligible effects (58, 63). The overall impact of CMV infection on influenza vaccine responsiveness remains controversial, as it is depends on many variables. Different studies performed with different seasonal vaccines, tested in different populations, at different times, are difficult to compare directly. As such, there have been no studies that have directly linked CMV seropositivity with increased risk of influenza illness in vaccinated older adults.
In addition to lack of consensus on the impact of aging and CMV seropositivity on antibody responses to influenza vaccination, investigating this issue is further complicated by differing responses to strains of the virus. Vaccine efficacy in the elderly against H3N2 is particularly poor compared to H1N1 or B strains (64, 65). One explanation for an apparent lack of responsiveness in the elderly may reside in the manner in which antibody responses are quantified, which is dependent on the immunological history of the individual. Thus, older adults who already have a high-antibody titer prior to vaccination may be classified as non-responders if they do not further increase an already-protective titer. More importantly, comparisons of the antibody response to influenza vaccination in young and older adults have been confounded by the effects of age and exposure history related to prior vaccination (66). In addition, these differing observations may be explained in the context of original antigenic sin, which supports the notion that vaccination re-stimulates immunological memory of past exposure to a similar strain, and may explain the relative protection of older adults against the pandemic H1N1 (pH1N1) strains (67). The theory of vaccine re-stimulation has not been explored in the context of CMV, but highlights the importance of identifying which subtype of influenza is being studied and a consensus as regards to the definition of vaccine “responder.”
Contradictory observations of influenza strain-specific titers post-vaccination between CMV-seropositive and -negative individuals have also been identified. Specifically, CMV+ subjects were found to have higher antibody titer to H1N1 (58, 59, 61), while others have observed the opposite (41). Similarly, in some studies, no association was observed between CMV status and H3N2-directed antibodies (63), while others have reported lower H3N2 antibody responses in such subjects (62). Those identifying an improved response to vaccination have hypothesized that CMV infection is accompanied by a higher level of a low-grade chronic inflammation that in turn provides an ongoing stimulation to the immune system in older (68) and younger adults (58).
It should be noted that studies in this area have used different measures of the antibody response to vaccination as a correlate of protection. Specifically, some have reported peak antibody response, while others measured antibody persistence. Although peak antibody titers after vaccination depend mainly on short-lived plasma B-cells, antibody persistence depends on memory B-cells and long-lived plasma cells. As such, antibody persistence may be a more meaningful measure of clinical protection. Some apparent discrepancies in the literature could derive from such different measures.
Other possible reasons for the discrepancies reported in the literature may be related to confounding factors such as medications, as illustrated in a recent study by Reed et al. These investigators identified a poorer antibody response (quantified based on antibody persistence) to vaccination in CMV-seropositive older adults, but only if they were taking β-adrenergic-blocking drugs (69). β-adrenergic blockers, a class of drug commonly used for blood pressure control, may also influence immune responses (70) and could therefore represent a confounding factor resulting in non-consensus of previous studies of antibody responses in older adults. However, it should also be noted that the use of β-blockers may simply reflect non-specifically generally poorer health or could represent an association with other health conditions relating to immunosenescence. The potential impact of drug treatment on vaccine response is further illustrated in the case of statins and antibody responses to influenza vaccination in older adults (71) as well as vaccine efficacy (72). Statins are known to influence immune responses via multiple different mechanisms (73), indicating a need to investigate the relationship further, especially since this class of drug is used by a growing number of older adults (74, 75).
Molecular Genetics As a Tool to Investigate B-Cell Function in the Context of Aging and CMV
Sequencing of the immunoglobulin heavy chain has been conducted to study B-cell receptor (BCR) repertories. It was found that V (variable), D (diversity), and J (joining) usage is consistent between age groups, although mutations in V genes are associated with CMV seropositivity. Furthermore, mutations in IgM and IgG sequences are higher in the elderly, and more so in those who are CMV seropositive (76). This suggests that repeated antigen exposure with aging and CMV reactivation induces B-cell proliferation and IgG gene mutations.
In a groundbreaking study, de Bourcy et al. used next-generation sequencing technology to study BCR diversity. They showed that while BCR repertoires become more specialized over the lifespan, they also demonstrate decreased capacity for plasticity or adaptability. Relative to the young, older adults have a smaller naïve repertoire and lower intra-lineage diversity, resulting in a reduced ability to mount a diverse response to novel antigens (77). This suggests that annual updates of the strains of influenza contained in the vaccine are less likely to induce antibody responses to new viral variants in older adults.
The association of leukocyte telomere shortening with some aspects of aging has been well documented. In a seminal study of the correlation between telomere length of B-cells and humoral immune responses to influenza vaccination in adults over the age of 70, it was shown that those with longer telomeres (6.3 kb) had superior antibody responses (based on fold-increase of influenza-specific antibody titers) relative to those with shorter telomeres (5.6 kb) (17). While the mechanism involved is still not well understood, telomere length could be used as a marker for immune function and vaccine responsiveness.
The Role of T-Cells in Influenza Infection and Vaccination
The antibody response to influenza virus plays a vital role in protection against influenza infection, but epitope-specific T-cells are also critical (78–80). Although assessment of antibody responses to influenza vaccines is mainly used as a measure of efficacy, studies continue to show that humoral immunity by itself does not provide sterilizing immunity against infection in older adults, and that T-cell responses are critically important when antibody-mediated protection fails (81). Furthermore, T-cell responses are cross-reactive within the strains of influenza A or influenza B, allowing for broad protection against drifted strains of influenza (82).
Decreased output of naïve T-cells resulting from thymic involution after puberty results in a reduced ability to respond to novel antigens (83) and has been linked to poor response to influenza vaccination in the elderly (84). Studies have shown that telomere length of T-cells specific for CMV are longer on average than those specific for influenza and may suggest that CMV continues to recruit cells from the naïve T-cell pool over time (85).
Aging is correlated with a loss of naïve CD8+ T-cells, more so than naïve CD4+ T-cells. This loss in naïve cells is not associated with CMV seropositivity (86). The loss of naïve CD4+ T-cells is associated with an increase in effector and effector memory CD4+ T-cells and is observed essentially only in CMV-seropositive individuals (86). These findings illustrate the distinct, and sometimes additive effect of aging and CMV in different T-cell populations.
Helper T-Cells
Influenza infection induces HA-specific CD4+ T-helper cells (87), resulting in a diverse antibody response (88). Th2 responses stimulate antibody production that is driven by the production of cytokines, including IL-4, IL-5, IL-10, IL-13, IL-31, and IL-33 by mast cells and eosinophils, which are responsible for a Th2 response, thereby leading to the activation of B-cell clones and production of influenza-specific IgG1 and IgE (89, 90). The regulatory T-cell (Treg) response results in the expression of IL-10 and TGF-β, which further hinders a Th1 response (91).
Aging is associated with an increasing acquisition of a Th2 bias, specifically with an increase in CD4+CD294+ (Th2) cells (92). Furthermore, some studies have reported a decline in the total number of CD8+ T-cells and increases in T-helper cells reflected in a lower Th1:Th2 ratio (92). IL-10 and other cytokines produced by Th2 or Treg have been associated with reduced cytotoxic T-lymphocyte (CTL) activity in older adults (93) and against ex vivo influenza virus challenge (94). Although Th2-associated cytokines do not help in the recovery from influenza infection (95), these cytokines continue to be expressed at high levels at the site of influenza infection and may be a factor in inflammation and lung damage associated with infection (78).
Role of TFH Cells during Influenza Infection and Vaccination in the Elderly
IL-12 production by activated DCs induces naïve CD4+ T-cells to differentiate into IL-21-producing TFH cells (96, 97). Elevated IL-21 is positively correlated with CMV seropositivity (98) as it is believed to be required for the maintenance of latent infection (99–101) as well as clearance of acute viral infections (102, 103).
TFH cells are a separate type of helper T-cells that are known to promote germinal center formation, B-cell survival, proliferation, class switching, plasma cell differentiation, and somatic hypermutation (104–108) and are hence found in germinal centers (GC TFH) and in peripheral blood (pTFH). Studies have found a direct relationship between the frequency of activated pTFH cells following vaccination and influenza vaccine-induced antibody responses (109–111). It has also been shown that pTFH cells isolated post-influenza vaccination are better able than other CD4+ T-cell subsets to support B-cell differentiation and to stimulate influenza-specific antibody secretion (110).
A study of older women found a high frequency of activated TFH cells (CD38+HLA-DR+Ki-67+). The presence of activated pTFH cells in older women prior to influenza vaccination was negatively correlated with antibody titers post-vaccination and suggests that activated pTFH cells are less capable of providing help to B-cells when faced with new antigens (112).
Cytotoxic T-Lymphocytes
Aging creates specific challenges to effective CTL activity against infection: (a) T-cell receptor (TCR) diversity reduction, (b) reduced effector function of cells, (c) cell type frequency changes, and (d) general inflammation.
Cytomegalovirus elicits CD4+ and CD8+ T-cell responses (113, 114), which play an important role in maintaining latency of CMV infection (115). The immune response necessary to maintain latency has two major consequences: driving T-cells to a late-differentiated state associated with immunosenescence (6, 14, 116) and memory inflation (117). The latter develops from chronic antigen exposure as a result of CMV infection (118, 119), but interestingly Epstein–Barr virus does not have the same impact on inflation (120). CMV-specific memory T-cells account for 0.1–40% of the total memory population in the periphery (113, 114). The high frequency of CMV-specific T-cells develop during the first year after infection and either gradually increases or remains constant long term (121–123). Over a lifetime, a large group of T-cells recognizing CMV epitopes emerges, the majority of which may be dysfunctional (124). This contributes to the concept of a restricted “immunological space,” whereby the T-cell populations consisting of dysfunctional clonally expanded and anergic cells are targeted toward a small number of epitopes (125). In addition, aging results in a decrease of the TCR repertoire, which is associated with a poor response to influenza vaccination (126–129). Furthermore, the repertoire may become oligoclonal due to extended lifespan and homeostatic turnover of naïve T-cells (130).
On infection, viral epitopes bind to the major histocompatibility complex (MHC) molecules of APCs, and through the interaction with TCRs activate naïve or memory T-cells to become effector CTLs (131, 132). The majority of the CD8+ T-cell epitopes derived from influenza virus are contained within the NP and matrix 1 (M1) proteins. As a result of the homology of these internal proteins and highly conserved epitopes across the different subtypes (A/H1N1 and A/H3N2), the CD8+ T-cell response to influenza is cross-reactive among all of the strains of influenza A. Activation of T-cells leads to their migration to the infection site where they recognize influenza virus-infected cells and eliminate them via lytic activity. While CTL killing of influenza-infected cells can be mediated through Fas- (132), and TRAIL- (133) associated pathways, the dominant mechanism appears to be perforin-mediated killing (134). Recent studies suggest that perforin-mediated killing through granzyme B (GrB) apoptotic pathways is the most critical for viral clearance (135).
Role of GrB in Protection against Influenza Infection
A correlation between low GrB prior to H3N2 infection, fever, and lack of seroconversion is indicative of the association of cell-mediated immunity and illness severity (136). GrB levels increase in response to H3N2 infection independently of serological responses (136), with a deficiency in the production of GrB and IFN-γ in CD8+ T-cells observed in vaccinated older adults (137, 138).
Granzyme B has been associated with clinical protection from influenza (139) and is produced by both CD4+ and CD8+ T-cells (140). Late-differentiated T-cells (CD45RA+GrB+Perforin−) particularly CD8+ subsets are abundant (with as many as 50% of these cells producing GrB in the resting state) and are associated with poor CD8+ T-cell cytolytic activity following influenza vaccination (137, 141). Of note, the cytolytic activity of CD8+ T-cells dramatically declines by 10 weeks post-vaccination, and while this occurs to a lesser degree in CD4+ T-cells, their cytolytic potential is relatively minor compared to CD8+ T-cells (137). However, this discordant change in CD4+ and CD8+ vaccine-specific T-cells suggests that CD4+ CTLs in older adults could be targeted to promote cell-mediated immune protection via vaccination.
CD4+ T-cells in the lung expressing GrB and perforin have cytolytic activity against influenza in mice (142, 143). Using influenza M1 peptide stimulation, it has been shown that the proportion of subjects mounting a CD4+ T-cell response was lower in CMV-seropositive than seronegative individuals (16).
Granzyme B and perforin-expressing CD4+ T-cells also produce IFN-γ, suggesting a Th1 lineage (143, 144). Many CD4+ T-cells responding to influenza in the lung produce IL-10, largely in cells also producing IFN-γ (78, 145). This IL-10 production by influenza-specific CD4+ T-cells results in reduced protection (146) by suppressing cytokine production in Th17 cells (78, 145), but also plays an important role in limiting immunopathology (78).
Cytomegalovirus-seropositive older adults have higher levels of GrB in resting T-cells, the majority of which have a late-differentiated T-cell phenotype (CD45RA+) or are CD28− (147). The accumulation of GrB in putatively terminally differentiated CD8+ T-cells in the absence of perforin in vivo (147) suggests that GrB may be released into and accumulate in the extracellular space, resulting in inflammation and tissue damage (148–150). It has also been shown that ex vivo live influenza virus challenge results in a lower GrB response in CMV-seropositive compared to -seronegative older adults (63), further suggesting an impairment of CTL response to influenza mediated by CMV.
Impact of Reduced CD28+ T-Cells in Elderly and CMV Seropositivity
CD28 has an important function as a costimulator in the activation of T-cells and influences their susceptibility to apoptosis (151). It is required for optimal T-cell activation, but its level of expression by CD8+ T-cells decreases with age (152, 153). This cell phenotype has been associated with a poor response to influenza vaccination (39, 40, 154), and it has been suggested that it has some similarities with replicative senescence (155). It has also been shown that CMV infection contributes to the accumulation of these cells (156, 157). In addition to CD8+CD28− cells impacting vaccine response, late-stage differentiated CD4+ T-cells, lacking CCR7, CD27, and CD28 and re-expressing CD45RA are also found in CMV-seropositive subjects and correlated with poor vaccination response (158). A low frequency of CD45RA re-expressing late-differentiated CD4+ T-cells are found in CMV-seropositive individuals, independent of age (159–161), with the majority of these cells being CMV specific (158, 160).
CD4:CD8 Ratio As a Biomarker of CTL Function
CD4:CD8 T-cell ratios contribute to immune risk profiles with a ratio of less than 1 being predictive of 2-year mortality in some studies (162, 163). CMV infection has been found to be associated with a CD4:CD8 of <1 (13, 164). These findings are consistent with the notion that the clonal expansions of CD8+ T-cells observed in the elderly (165–167) are to a large extent CMV-specific (124) and associated with mortality (168). Changes in the ratio were found to be the result of an increase in CD8+ T-cell populations, specifically, CD27−, CD28−, CD56+, and CD57+, CD45RA+, and CD45RA+/RO+ cells (13), markers that indicate reduced effector functionality and provides support for the finding of poor humoral response to influenza vaccination in those with a low CD4:CD8 ratio (169). Furthermore, the majority of the CMV-specific CD8+ T-cells in the elderly have reduced functionality, supported by the finding that the fraction of cells producing IFN-γ in response to peptide stimulation in the elderly was significantly lower than in the young (124). Elderly with a CD4:CD8 ratio <1 had about 10% of total CD8+ T-cells specific for a single CMV epitope (170), but in the group with a CD4:CD8 ratio >1, the frequency was similar to the middle-aged group (124).
Alterations to Vaccine Design
In addition to the aging immune system and CMV seropositivity both potentially hindering the immune response to influenza vaccination, other challenges to effective vaccine design are also in play. Challenges faced particularly in influenza vaccine development lie in the high level of strain divergence from season to season resulting from error-prone replication of the influenza virus via RNA-dependent RNA polymerase, recombination, and genetic drift (171). While the plasticity of the virus is sustained when variability accumulates in the HA and neuraminidase (NA) proteins, internal proteins are not as capable of maintaining functionality in the face of mutation accumulation, resulting in much less variation in the matrix and NP (172). The current vaccine strategy is designed to stimulate the development of antibodies against the HA and NA proteins, resulting in the ongoing need for annual vaccine modification to account for viral strain variation, which may be obviated to some extent through vaccines designed to also target internal proteins.
While antibody titers are the generally accepted standard for the testing of influenza vaccination protection, it has been suggested that it is a poor measure if used alone to assess protection in the elderly (173). The limitations of antibody titers are apparent when examining the strain-specific differences in the elderly. For example, vaccine efficacy against H3N2 in the elderly is particularly poor compared to H1N1 or B strains (64, 65), while concurrently, others have found higher antibody titers against the H3N2 strain post-vaccination in this group (174–176).
The focus on humoral immune protection against influenza has its limitations, as circulating strains may not match the vaccine (177). Current vaccines do not induce sufficient cross-reactive CD8+ T-cells to provide protection against non-homologous influenza A virus challenge (178), but this would be an advantageous characteristic of future vaccine candidates.
Dosage
Studies comparing dosage of trivalent inactivated influenza virus vaccines in older adults have found that people who received high dose vaccinations had significantly higher antibody titers for all three strains (to varying degrees) than those who received standard dose vaccinations (179, 180). High dose vaccine recipients had a greater frequency of pTFH cells post-vaccination than those receiving a standard dose. Specifically, the expression of CD278 (also referred to as inducible T-cell costimulator: ICOS) on the pTFH cells was elevated (181), suggesting an increase in their ability to provide B-cell help. Furthermore, as mentioned previously, the frequency of pTFH cells was a predictor of seroconversion for all three vaccine strains (181). It has also been shown that the longevity of the antibody response is not influenced by vaccine dose (182). An assessment of the impact of vaccine dose on the cellular immune response has been limited to analysis of IFN-γ response, which found no significant difference (182, 183). However, this important issue requires further study.
While the high dose vaccine has been shown to deliver better clinical protection in older adults (184), further investigation as to the mechanism is required. Furthermore, the impact of vaccine dose in more vulnerable older adults, including those who are CMV seropositive also requires investigation.
Adjuvants
The elderly might benefit from the increased application of adjuvanted vaccines. For example, glucopyranosyl lipid adjuvant (GLA) is a TLR4 agonist that has been found to be safe and well tolerated (185). GLA, when combined with Fluzone, a split virus vaccine, showed enhanced antibody response as well as a shift to a Th1 cytokine profile in mice (185). In vivo, GLA produces a shift toward a Th1 cell-mediated response to influenza challenge by reducing IL-10 expression along with an increase in GrB activity (186). It has also been shown in vitro that GLA can induce the maturation of human DCs with an associated release of Th1-inducing cytokine and chemokine constellation (187).
Older adults seropositive for CMV have been found to have lower levels of activated DCs than young CMV+ adults, as it is believed that the older group has a greater control of infection due to higher CMV-specific antibodies. Due to a lower preactivated state, the DCs of older adults, regardless of CMV status, were also relatively more responsive to TLR4 antagonist (in this case LPS) and were able to produce TNF and IL-6 at the same level, and same cell frequency as younger adults who were CMV seronegative (188). Thus, the use of a TLR4 agonist in influenza vaccines for the CMV-seropositive elderly may be a potential adjuvant to improve vaccine efficacy in this group.
Vaccine Antigen
Conserved proteins, NP and M1, share high levels of homology among influenza strains (189, 190) and contain immunodominant MHC Class I and II epitopes (191). At the present time, influenza vaccines are designed to have specific quantities of HA protein, but the quantity of the internal proteins are believed to be low and are not quantified as part of vaccine formulation quality control (192). Evidence suggests that the inclusion of NP and M1 proteins in influenza vaccines would develop a Th1 memory and provide more effective protection in older adults.
Clinical studies of mRNA-based vaccines demonstrated their ability to elicit functional antibodies and T-cell responses (193, 194). Unlike previously considered DNA-based vaccines, there is no concern regarding genome integration, or the need to design nuclear transport mechanisms for mRNA vaccines. RNA-based vaccines allow for cell entry, viral protein translation, and broad immune responses, while also acting as an adjuvant (TLR7/8) (195). Two different forms of RNA-based vaccines are currently being developed against influenza: non-amplifying mRNA (196) and self-amplifying mRNA molecules (197, 198). A modified vaccinia virus Ankara-based vaccine against influenza has been developed which consists of non-replicating RNA encoding both NP and M1 proteins (199). This vaccine was found to be safe and able to stimulate T-cell responses in older adults similar in magnitude to young adults (200). Amplifying mRNA (SAM1 technology) is based on non-viral delivery of antigen-encoding RNA by modified alphavirus single-stranded RNA, which allows for viral RNA replication and greater viral protein translation (193, 194, 197). In a comparison of replicating and non-replicating vaccines, it was found that self-replicating vaccines elicit significantly stronger cellular and humoral immune responses, which was suggested to be the result of greater antigen presentation (201). These findings suggest that self-replicating influenza RNA vaccines may be used to overcome the effects of immune senescence and CMV seropositivity.
Vaccination Schedule
A study mapping the prevalence of influenza-specific antibodies in children found that by the age of 6, all children had seroconverted and thus had immunological memory for at least one influenza virus strain (202). Over time, this memory is boosted and diversified by subsequent infections with drifted influenza virus strains. In turn, this memory response (both humoral and cellular) can act to protect against infection with similar stain variants, referred to as cross-protection. Cross-protection was shown in the pH1N1 virus identified during the 2009–2010 and 2013–2014 influenza outbreaks which resulted in high rates of morbidity and mortality. Analysis of the CD8+ T-cells specific for the 2009 pH1N1 virus identified epitopes shared with the 1918 pH1N1 strain, as well as stains circulating prior to 1945 (203). These findings corroborate the observation that the severity of the influenza illness in the over-65 age group infected with pH1N1 was considerably lower than other influenza seasons (204), suggesting cross-protection. Furthermore, it was found that the majority of severe cases of pH1N1 infection occurred in young adults (205). This has led to suggestions that vaccines may only be effective due to their ability to act as a booster to memory CD8+ T-cells remaining from previous infections, rather than creating a memory response to new targets (137). Furthermore, evidence suggests that T-cells generated in youth can remain protective over decades (206, 207), while those derived later in life are severely impaired (208, 209). Thus, it could be postulated that the most effective way to ensure protection via vaccination in older adults would be to design a vaccine strategy targeted at youth.
Vaccine effectiveness against influenza declines with time after vaccination over the winter season and is most evident in older adults, with efficacy lasting 140 days for H3N2 and 200 days for influenza B (210). Interestingly, vaccine effectiveness appeared to be maintained for over 200 days for subtype H1N1 (210). Influenza vaccines are only administered once a year but the impact of a double dose or booster vaccines is being studied to determine whether efficacy and level of protection can be improved. At present, a clinical trial of vaccines administered bi-yearly in older adults is underway (http://ClinicalTrials.gov: NCT02655874). The benefit of two-dose influenza vaccines has been shown under somewhat different circumstances in studies of solid organ transplant recipients where a second influenza vaccine dose after 5 weeks resulted in higher rates of seroconversion and seroprotection (defined as titer ≥1:40) (211). Frail older adults who were non-responders to an initial dose of influenza vaccine showed a decline in antibody titers to the A/H3N2 strain following a booster dose of influenza vaccine and derived no clinical benefit from this booster strategy (212). These results are consistent with our earlier studies showing a significant IL-10 response to a booster dose vaccination and a decline in the antibody and GrB response to influenza challenge following vaccination, compared to those older adults who received a single dose of influenza vaccine (213). Studies of H5N1 have shown that a two-dose approach using heterosubtypic H5 antigen results in a greater magnitude of T-cell cytokine response with heterosubtypic protection, but this does not seem to be the case in older adults (214). As such, the impact of a two-dose influenza vaccine is not yet clear and requires further investigation to determine effectiveness.
Reducing the Impact of Immunosenescence through Anti-CMV Strategies
One possible method to improve influenza vaccine efficacy might be an anti-CMV strategy via vaccination or treatment. While the majority of CMV treatment strategies have been developed for the prevention of congenital CMV infection or for the immunocompromised, there is potential for its application in the wider population.
The challenge in CMV vaccine design lays in the extensive genetic diversity of CMV strains due to recombination (215, 216). Several multi-component subunit (217), recombinant live-attenuated (218), and DNA (219) vaccine candidates aimed at achieving broad cross-neutralizing humoral and cellular immune responses are currently under investigation. One clear caveat for the CMV vaccine strategy to be effective is the need for its administration at a young age, and the ability for the vaccine to impart long-lasting immunity. Drug treatments may be a more practical approach in the short-term including those with anti-CMV activity in vitro currently being tested in clinical trials: a protein kinase inhibitor with specific activity against CMV (220); a CMV terminase inhibitor (221); and a broad spectrum antiviral agent (220).
Conclusion
Aging, along with CMV seropositivity, impacts the immune response to influenza infection and vaccination as a result of many interacting mechanisms (Table 1; Figure 1). With the increased risk of influenza-associated morbidity and mortality in the over-65 population, it is critical to take these impairments into consideration when developing the next generation of influenza vaccines.
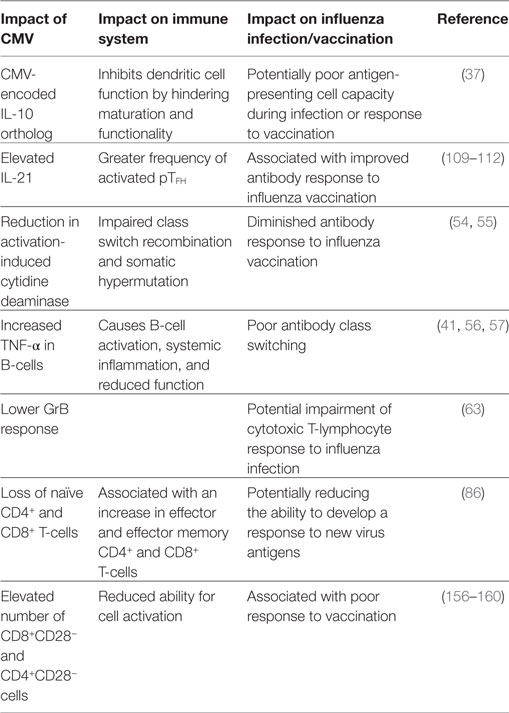
Table 1. Summary of the impact of cytomegalovirus (CMV) in the elderly and resulting influence on the response to influenza infection and vaccination.
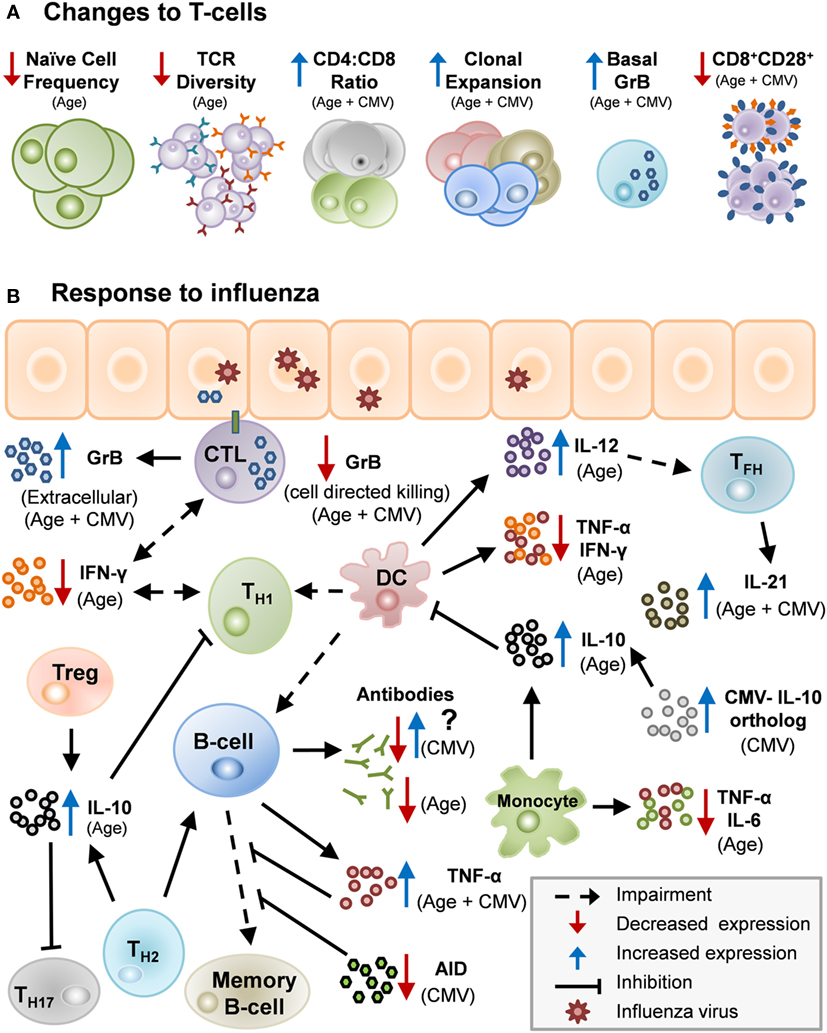
Figure 1. Potential mechanisms by which age and cytomegalovirus (CMV) may cause changes in human immunity. (A) Age and CMV can act in unison to impair aspects of the immune system: lower numbers of naïve T-cells, decrease in T-cell receptor (TCR) repertoire, heightened CD4:CD8 ratio, clonal expansion, increased levels of basal granzyme B (GrB) in resting T-cells and decrease in CD8+CD28+ cells. (B) There are several potential mechanisms by which age and CMV may cause changes in human immunity. Influenza infection stimulates Th1/Th2 although impaired in older adults due to diminished antigen-presenting cell function in the elderly. Th1 response with IFN-γ activating memory cytotoxic T-lymphocyte (CTL) which clears virus from the lungs. Age-related changes drive a Th2/regulatory T-cell (Treg) response to infection, and IL-10 production suppresses the CTL response. CMV infection further impairs the response to influenza infection by contributing to age-related impairments and by other mechanisms.
Author Contributions
SM developed the initial outline and draft of the manuscript. GP, GK, and JM revised the manuscript critically for important intellectual content. All the authors read and approved the final manuscript.
Conflict of Interest Statement
SM, GP, and GK have no conflict of interest to declare. JM has travel support and her Institute has received honoraria for her participation in advisory boards or presentations at meetings from GSK, Sanofi, and Pfizer.
Funding
GP is supported by the German Research Foundation (DFG Pa 361/22-1) and the Croeni Foundation. GK and JM are supported by NIH AG048023, P01AG021600. JM is supported by Northern Ontario Heritage Fund Corporation, Canadian Immunization Research Network, and Northern Ontario Academic Medical Association.
Abbreviations
AID, activation-induced cytidine deaminase; APC, antigen-presenting cell; BCR, B-cell receptor; CMV, cytomegalovirus; CTL, cytotoxic T-lymphocyte; DC, dendritic cell; EBV, Epstein–Barr virus; GrB, granzyme B; HA, hemagglutinin; M1, matrix 1; MHC, major histocompatibility complex; NA, neuraminidase; NP, nucleoprotein; pH1N1, pandemic H1N1; pTFH, peripheral T-follicular helper cell; TCR, T-cell receptor; Treg, regulatory T-cell.
References
1. Haspot F, Lavault A, Sinzger C, Laib Sampaio K, Stierhof Y, Pilet P, et al. Human cytomegalovirus entry into dendritic cells occurs via a macropinocytosis-like pathway in a pH-independent and cholesterol-dependent manner. PLoS One (2012) 7(4):e34795. doi:10.1371/journal.pone.0034795
2. Antona D, Lepoutre A, Fonteneau L, Baudon C, Halftermeyer-Zhou F, LE Strat Y, et al. Seroprevalence of cytomegalovirus infection in France in 2010. Epidemiol Infect (2017) 145(7):1471–8. doi:10.1017/S0950268817000103
3. Vilibic-Cavlek T, Kolaric B, Beader N, Vrtar I, Tabain I, Mlinaric-Galinovic G. Seroepidemiology of cytomegalovirus infections in Croatia. Wien Klin Wochenschr (2016) 129(3–4):129–35. doi:10.1007/s00508-016-1069-7
4. Staras SA, Dollard SC, Radford KW, Flanders WD, Pass RF, Cannon MJ. Seroprevalence of cytomegalovirus infection in the United States, 1988–1994. Clin Infect Dis (2006) 43(9):1143–51. doi:10.1086/508173
5. Pawelec G, McElhaney JE, Aiello AE, Derhovanessian E. The impact of CMV infection on survival in older humans. Curr Opin Immunol (2012) 24(4):507–11. doi:10.1016/j.coi.2012.04.002
6. Pawelec G, Derhovanessian E. Role of CMV in immune senescence. Virus Res (2011) 157(2):175–9. doi:10.1016/j.virusres.2010.09.010
7. Schmaltz HN, Fried LP, Xue Q-L, Walston J, Leng SX, Semba RD. Chronic cytomegalovirus infection and inflammation are associated with prevalent frailty in community-dwelling older women. J Am Geriatr Soc (2005) 53(5):747–54. doi:10.1111/j.1532-5415.2005.53250.x
8. Wang GC, Kao WHL, Murakami P, Xue Q-L, Chiou RB, Detrick B, et al. Cytomegalovirus infection and the risk of mortality and frailty in older women: a prospective observational cohort study. Am J Epidemiol (2010) 171(10):1144–52. doi:10.1093/aje/kwq062
9. Savva GM, Pachnio A, Kaul B, Morgan K, Huppert FA, Brayne C, et al. Cytomegalovirus infection is associated with increased mortality in the older population. Aging Cell (2013) 12(3):381–7. doi:10.1111/acel.12059
10. Moro-García MA, Alonso-Arias R, López-Vázquez A, Suárez-García FM, Solano-Jaurrieta JJ, Baltar J, et al. Relationship between functional ability in older people, immune system status, and intensity of response to CMV. Age (2012) 34(2):479–95. doi:10.1007/s11357-011-9240-6
11. Aiello AE, Haan MN, Pierce CM, Simanek AM, Liang J. Persistent infection, inflammation, and functional impairment in older Latinos. J Gerontol A Biol Sci Med Sci (2008) 63(6):610–8. doi:10.1093/gerona/63.6.610
12. Boxer R, Kleppinger A, Ahmad A, Annis K, Hager D, Kenny A. The 6-minute walk is associated with frailty and predicts mortality in older adults with heart failure. Congest Heart Fail (2010) 16(5):208–13. doi:10.1111/j.1751-7133.2010.00151.x
13. Wikby A, Johansson B, Olsson J, Löfgren S, Nilsson B-O, Ferguson F. Expansions of peripheral blood CD8 T-lymphocyte subpopulations and an association with cytomegalovirus seropositivity in the elderly: the Swedish NONA immune study. Exp Gerontol (2002) 37(2–3):445–53. doi:10.1016/S0531-5565(01)00212-1
14. Derhovanessian E, Larbi A, Pawelec G. Biomarkers of human immunosenescence: impact of cytomegalovirus infection. Curr Opin Immunol (2009) 21(4):440–5. doi:10.1016/j.coi.2009.05.012
15. Matheï C, Adriaensen W, Vaes B, Van Pottelbergh G, Wallemacq P, Degryse J. No relation between CMV infection and mortality in the oldest old: results from the BELFRAIL study. Age Ageing (2015) 44(1):130–5. doi:10.1093/ageing/afu094
16. Derhovanessian E, Maier AB, Hähnel K, McElhaney JE, Slagboom EP, Pawelec G. Latent infection with cytomegalovirus is associated with poor memory CD4 responses to influenza a core proteins in the elderly. J Immunol (2014) 193(7):3624–31. doi:10.4049/jimmunol.1303361
17. Najarro K, Nguyen H, Chen G, Xu M, Alcorta S, Yao X, et al. Telomere length as an indicator of the robustness of B- and T-cell response to influenza in older adults. J Infect Dis (2015) 212(8):1261–9. doi:10.1093/infdis/jiv202
18. Johnstone J, Parsons R, Botelho F, Millar J, McNeil S, Fulop T, et al. Immune biomarkers predictive of respiratory viral infection in elderly nursing home residents. PLoS One (2014) 9(10):e108481. doi:10.1371/journal.pone.0108481
19. Stowe RP, Kozlova EV, Yetman DL, Walling DM, Goodwin JS, Glaser R. Chronic herpesvirus reactivation occurs in aging. Exp Gerontol (2007) 42(6):563–70. doi:10.1016/j.exger.2007.01.005
20. Parry HM, Zuo J, Frumento G, Mirajkar N, Inman C, Edwards E, et al. Cytomegalovirus viral load within blood increases markedly in healthy people over the age of 70 years. Immun Ageing (2016) 13:1. doi:10.1186/s12979-015-0056-6
21. Wills MR, Poole E, Lau B, Krishna B, Sinclair JH. The immunology of human cytomegalovirus latency: could latent infection be cleared by novel immunotherapeutic strategies? Cell Mol Immunol (2015) 12(2):128–38. doi:10.1038/cmi.2014.75
22. Thompson WW, Shay DK, Weintraub E, Brammer L, Cox N, Anderson LJ, et al. Mortality associated with influenza and respiratory syncytial virus in the United States. JAMA (2003) 289(2):1333–40. doi:10.1001/jama.289.2.179
23. Zimmerman RK, Lauderdale DS, Tan SM, Wagener DK. Prevalence of high-risk indications for influenza vaccine varies by age, race, and income. Vaccine (2010) 28(39):6470–7. doi:10.1016/j.vaccine.2010.07.037
24. Loerbroks A, Stock C, Bosch JA, Litaker DG, Apfelbacher CJ. Influenza vaccination coverage among high-risk groups in 11 European countries. Eur J Public Health (2012) 22(4):562–8. doi:10.1093/eurpub/ckr094
25. Thompson WW, Shay DK, Weintraub E. Influenza-associated hospitalizations in the United States. JAMA (2004) 292(11):1333–40. doi:10.1001/jama.292.11.1333
26. Ferrucci L, Guralnik JM, Pahor M, Corti M, Havlik RJ. Hospital diagnoses, Medicare charges, and nursing home admissions in the year when older persons become severely disabled. JAMA (1997) 277(9):728–34. doi:10.1001/jama.1997.03540330050034
27. Monto AS, Ansaldi F, Aspinall R, McElhaney JE, Montaño LF, Nichol KL, et al. Influenza control in the 21st century: optimizing protection of older adults. Vaccine (2009) 27(37):5043–53. doi:10.1016/j.vaccine.2009.06.032
28. Finco O, Rappuoli R. Designing vaccines for the twenty-first century society. Front Immunol (2014) 5:12. doi:10.3389/fimmu.2014.00012
29. Nichol KL, Margolis KL, Wuorenma J, Von Sternberg T. The efficacy and cost effectiveness of vaccination against influenza among elderly persons living in the community. N Engl J Med (1994) 331(12):778–84. doi:10.1056/NEJM199409223311206
30. Goodwin K, Viboud C, Simonsen L. Antibody response to influenza vaccination in the elderly: a quantitative review. Vaccine (2006) 24(8):1159–69. doi:10.1016/j.vaccine.2005.08.105
31. Simonsen L, Taylor RJ, Viboud C, Miller MA, Jackson LA. Mortality benefits of influenza vaccination in elderly people: an ongoing controversy. Lancet Infect Dis (2007) 7(10):658–66. doi:10.1016/S1473-3099(07)70236-0
32. Andrew M, Shinde V, Ye L, Hatchette T, Haguinet F, Dos Santos G, et al. The importance of frailty in the assessment of trivalent inactivated influenza vaccine effectiveness against influenza-related hospitalization in elderly people. J Infect Dis (2017) (in press).
33. Mahbub S, Deburghgraeve CR, Kovacs EJ. Advanced age impairs macrophage polarization. J Interferon Cytokine Res (2012) 32(1):18–26. doi:10.1089/jir.2011.0058
34. Mohanty S, Joshi SR, Ueda I, Wilson J, Blevins TP, Siconolfi B, et al. Prolonged proinflammatory cytokine production in monocytes modulated by interleukin 10 after influenza vaccination in older adults. J Infect Dis (2015) 211(7):1174–84. doi:10.1093/infdis/jiu573
35. van Duin D, Allore HG, Mohanty S, Ginter S, Newman FK, Belshe RB, et al. Prevaccine determination of the expression of costimulatory B7 molecules in activated monocytes predicts influenza vaccine responses in young and older adults. J Infect Dis (2007) 195(11):1590–7. doi:10.1086/516788
36. Cheung AKL, Gottlieb DJ, Plachter B, Pepperl-Klindworth S, Avdic S, Cunningham AL, et al. The role of the human cytomegalovirus UL111A gene in down-regulating CD4+ T-cell recognition of latently infected cells: implications for virus elimination during latency. Blood (2009) 114(1):4128–37. doi:10.1182/blood-2008-12-197111
37. Chang WLW, Baumgarth N, Yu D, Barry PA. Human cytomegalovirus-encoded interleukin-10 homolog inhibits maturation of dendritic cells and alters their functionality. J Virol (2004) 78(16):8720–31. doi:10.1128/JVI.78.16.8720-8731.2004
38. Panda A, Qian F, Mohanty S, van Duin D, Newman FK, Zhang L, et al. Age-associated decrease in TLR Function in primary human dendritic cells predicts influenza vaccine response. J Immunol (2010) 184(5):2518–27. doi:10.4049/jimmunol.0901022
39. Goronzy JJ, Fulbright JW, Crowson CS, Poland GA, O’Fallon WM, Weyand CM. Value of immunological markers in predicting responsiveness to influenza vaccination in elderly individuals. J Virol (2001) 75(24):12182–7. doi:10.1128/JVI.75.24.12182-12187.2001
40. Saurwein-Teissl M, Lung TL, Marx F, Gschösser C, Asch E, Blasko I, et al. Lack of antibody production following immunization in old age: association with CD8+CD28− T cell clonal expansions and an imbalance in the production of Th1 and Th2 cytokines. J Immunol (2002) 168(11):5893–9. doi:10.4049/jimmunol.168.11.5893
41. Frasca D, Diaz A, Romero M, Landin AM, Blomberg BB. Cytomegalovirus (CMV) seropositivity decreases B cell responses to the influenza vaccine. Vaccine (2015) 33(12):1433–9. doi:10.1016/j.vaccine.2015.01.071
42. Frasca D, Diaz A, Romero M, Landin AM, Phillips M, Lechner SC, et al. Intrinsic defects in B cell response to seasonal influenza vaccination in elderly humans. Vaccine (2010) 28(51):8077–84. doi:10.1016/j.vaccine.2010.10.023
43. Frasca D, Diaz A, Romero M, Phillips M, Mendez NV, Landin AM, et al. Unique biomarkers for B-cell function predict the serum response to pandemic H1N1 influenza vaccine. Int Immunol (2012) 24(3):175–82. doi:10.1093/intimm/dxr123
44. Khurana S, Frasca D, Blomberg B, Golding H. AID activity in B cells strongly correlates with polyclonal antibody affinity maturation in-vivo following pandemic 2009-H1N1 vaccination in humans. PLoS Pathog (2012) 8(9):e1002920. doi:10.1371/journal.ppat.1002920
45. Frasca D, Landin AM, Lechner SC, Ryan JG, Schwartz R, Riley RL, et al. Aging down-regulates the transcription factor E2A, activation-induced cytidine deaminase, and Ig class switch in human B cells. J Immunol (2008) 180(8):5283–90. doi:10.4049/jimmunol.180.8.5283
46. Sasaki S, Sullivan M, Narvaez CF, Holmes TH, Furman D, Zheng N-Y, et al. Limited efficacy of inactivated influenza vaccine in elderly individuals is associated with decreased production of vaccine-specific antibodies. J Clin Invest (2011) 121(8):3109–19. doi:10.1172/JCI57834
47. Pritz T, Lair J, Ban M, Keller M, Weinberger B, Krismer M, et al. Plasma cell numbers decrease in bone marrow of old patients. Eur J Immunol (2015) 45(3):738–46. doi:10.1002/eji.201444878
48. Frasca D, Diaz A, Romero M, Blomberg BB. The generation of memory B cells is maintained, but the antibody response is not, in the elderly after repeated influenza immunizations. Vaccine (2016) 34(25):2834–40. doi:10.1016/j.vaccine.2016.04.023
49. Andrews SF, Kaur K, Pauli NT, Huang M, Huang Y, Wilson PC. High preexisting serological antibody levels correlate with diversification of the influenza vaccine response. J Virol (2015) 89(6):3308–17. doi:10.1128/JVI.02871-14
50. Eto D, Lao C, DiToro D, Barnett B, Escobar TC, Kageyama R, et al. IL-21 and IL-6 are critical for different aspects of B cell immunity and redundantly induce optimal follicular helper CD4 T cell (Tfh) differentiation. PLoS One (2011) 6(3):e17739. doi:10.1371/journal.pone.0017739
51. Kroenke MA, Eto D, Locci M, Cho M, Davidson T, Haddad EK, et al. Bcl6 and Maf cooperate to instruct human follicular helper CD4 T cell differentiation. J Immunol (2012) 188(8):3734–44. doi:10.4049/jimmunol.1103246
52. Kaur K, Sullivan M, Wilson PC. Targeting B cell responses in universal influenza vaccine design. Trends Immunol (2011) 32(11):524–31. doi:10.1016/j.it.2011.08.007
53. Burlington DB, Clements ML, Meiklejohn G, Phelan M, Murphy BR. Hemagglutinin-specific antibody responses in immunoglobulin G, A, and M isotypes as measured by enzyme-linked immunosorbent assay after primary or secondary infection of humans with influenza A virus. Infect Immun (1983) 41(2):540–5.
54. Frasca D, Blomberg BB. Aging, cytomegalovirus (CMV) and influenza vaccine responses. Hum Vaccin Immunother (2016) 12(3):682–90. doi:10.1080/21645515.2015.1105413
55. Frasca D, Landin AM, Alvarez JP, Blackshear PJ, Riley RL, Blomberg BB. Tristetraprolin, a negative regulator of mRNA stability, is increased in old B cells and is involved in the degradation of E47 mRNA. J Immunol (2007) 179(2):918–27. doi:10.4049/jimmunol.179.2.918
56. Prösch S, Staak K, Stein J, Liebenthal C, Stamminger T, Volk H-D, et al. Stimulation of the human cytomegalovirus IE enhancer/promoter in HL-60 cells by TNFα is mediated via induction of NF-κB. Virology (1995) 208(1):197–206. doi:10.1006/viro.1995.1143
57. Frasca D, Diaz A, Romero M, Landin AM, Blomberg BB. High TNF-α levels in resting B cells negatively correlate with their response. Exp Gerontol (2014) 54:116–22. doi:10.1016/j.exger.2014.01.004
58. Furman D, Jojic V, Sharma S, Shen-Orr S, Angel CJL, Onengut-Gumuscu S, et al. Cytomegalovirus infection improves immune responses to influenza. Sci Transl Med (2015) 7(281):281ra43. doi:10.1126/scitranslmed.aaa2293
59. McElhaney JE, Garneau H, Camous X, Dupuis G, Pawelec G, Baehl S, et al. Predictors of the antibody response to influenza vaccination in older adults with type 2 diabetes. BMJ Open Diabetes Res Care (2015) 3:1. doi:10.1136/bmjdrc-2015-000140
60. Trzonkowski P, Myśliwska J, Szmit E, Wickiewicz J, Łukaszuk K, Brydak LB, et al. Association between cytomegalovirus infection, enhanced proinflammatory response and low level of anti-hemagglutinins during the anti-influenza vaccination – an impact of immunosenescence. Vaccine (2003) 21(22–26):3826–36. doi:10.1016/S0264-410X(03)00309-8
61. Wald A, Selke S, Magaret A, Boeckh M. Impact of human cytomegalovirus (CMV) infection on immune response to pandemic 2009 H1N1 influenza vaccine in healthy adults. J Med Virol (2013) 85(9):1557–60. doi:10.1002/jmv.23642
62. den Elzen WPJ, Vossen ACMT, Cools HJM, Westendorp RGJ, Kroes ACM, Gussekloo J. Cytomegalovirus infection and responsiveness to influenza vaccination in elderly residents of long-term care facilities. Vaccine (2011) 29(29–30):4869–74. doi:10.1016/j.vaccine.2011.03.086
63. Haq K, Fulop T, Tedder G, Gentleman B, Garneau H, Meneilly GS, et al. Cytomegalovirus seropositivity predicts a decline in the T cell but not the antibody response to influenza in accinated older adults independent of type 2 diabetes status. J Gerontol A Biol Sci Med Sci (2016):glw216. doi:10.1093/gerona/glw216
64. Bragstad K, Emborg H, Fischer TK, Voldstedlund M, Gubbels S, Andersen B, et al. Low vaccine effectiveness against influenza A(H3N2) virus among elderly people in Denmark in 2012/13 – a rapid epidemiological and virological assessment. Euro Surveill (2013) 18(6):11–7.
65. Remschmidt C, Rieck T, Bödeker B, Wichmann O. Application of the screening method to monitor influenza vaccine effectiveness among the elderly in Germany. BMC Infect Dis (2015) 15:137. doi:10.1186/s12879-015-0882-3
66. Höpping AM, McElhaney J, Fonville JM, Powers DC, Beyer WEP, Smith DJ. The confounded effects of age and exposure history in response to influenza vaccination. Vaccine (2016) 34(4):540–6. doi:10.1016/j.vaccine.2015.11.058
67. Boon ACM, deBeauchamp J, Krauss S, Rubrum A, Webb AD, Webster RG, et al. Cross-reactive neutralizing antibodies directed against pandemic H1N1 2009 virus are protective in a highly sensitive DBA/2 mouse influenza model. J Virol (2010) 84(15):7662–7. doi:10.1128/JVI.02444-09
68. Collerton J, Martin-Ruiz C, Davies K, Hilkens CM, Isaacs J, Kolenda C, et al. Frailty and the role of inflammation, immunosenescence and cellular ageing in the very old: cross-sectional findings from the Newcastle 85+ Study. Mech Ageing Dev (2012) 133(6):456–66. doi:10.1016/j.mad.2012.05.005
69. Reed RG, Greenberg RN, Segerstrom SC. Cytomegalovirus serostatus, inflammation, and antibody response to influenza vaccination in older adults: the moderating effect of beta blockade. Brain Behav Immun (2016) 61:14–20. doi:10.1016/j.bbi.2016.09.025
70. Holen E, Elsayed S. Effects of β2 adrenoceptor agonists on T-cell subpopulations. APMIS (1998) 106(9):849–57. doi:10.1111/j.1699-0463.1998.tb00231.x
71. Black S, Nicolay U, Del Giudice G, Rappuoli R. Influence of statins on influenza vaccine response in elderly individuals. J Infect Dis (2016) 213(8):1224–8. doi:10.1093/infdis/jiv456
72. McLean HQ, Chow BDW, VanWormer JJ, King JP, Belongia EA. Effect of statin use on influenza vaccine effectiveness. J Infect Dis (2016) 214(8):11150–8. doi:10.1093/infdis/jiw335
73. Huemer HP. Possible immunosuppressive effects of drug exposure and environmental and nutritional effects on infection and vaccination. Mediators Inflamm (2015) 2015. doi:10.1155/2015/349176
74. Salami JA, Warraich H, Valero-Elizondo J, Spatz ES, Desai NR, Rana JS, et al. National trends in statin use and expenditures in the US adult population from 2002 to 2013: insights from the medical expenditure panel survey. JAMA Cardiol (2017) 2(1):56–65. doi:10.1001/jamacardio.2016.4700
75. Qato DM, Alexander GC, Conti RM, Johnson M, Schumm P, Lindau ST. Use of prescription and over-the-counter medications and dietary supplements among older adults in the United States. JAMA (2008) 300(24):2867–78. doi:10.1001/jama.2008.892
76. Wang C, Liu Y, Xu LT, Jackson KJL, Roskin KM, Pham TD, et al. Effects of aging, CMV infection, and EBV infection on human B cell repertoires. J Immunol (2014) 192(2):603–11. doi:10.4049/jimmunol.1301384
77. de Bourcy CF, Angel CJ, Vollmers C, Dekker CL, Davis MM, Quake SR. Phylogenetic analysis of the human antibody repertoire reveals quantitative signatures of immune senescence and aging. Proc Natl Acad Sci U S A (2017) 114(5):1105–10. doi:10.1073/pnas.1617959114
78. Sun J, Madan R, Karp CL, Braciale TJ. Effector T cells control lung inflammation during acute influenza virus infection by producing IL-10. Nat Med (2009) 15(3):277–84. doi:10.1038/nm.1929
79. Wahl A, McCoy W, Schafer F, Bardet W, Buchli R, Fremont DH, et al. T-cell tolerance for variability in an HLA class I-presented influenza a virus epitope. J Virol (2009) 83(18):9209–14. doi:10.1128/JVI.00932-09
80. McMichael AJ, Gotch FM, Noble GR, Beare PAS. Cytotoxic T-cell immunity to influenza. N Engl J Med (1983) 309:13–7. doi:10.1056/NEJM198307073090103
81. Hsu JP, Phoon MC, Koh GCH, Chen MIC, Lee VJ, Wu Y, et al. Comparison of neutralizing antibody and cell-mediated immune responses to pandemic H1N1 2009 influenza virus before and after H1N1 2009 influenza vaccination of elderly subjects and healthcare workers. Int J Infect Dis (2012) 16(8):e621–7. doi:10.1016/j.ijid.2012.04.010
82. Wilkinson TM, Li CKF, Chui CSC, Huang AKY, Perkins M, Liebner JC, et al. Preexisting influenza-specific CD4+ T cells correlate with disease protection against influenza challenge in humans. Nat Med (2012) 18(2):274–80. doi:10.1038/nm.2612
83. Schulz AR, Mälzer JN, Domingo C, Jürchott K, Grützkau A, Babel N, et al. Low thymic activity and dendritic cell numbers are associated with the immune response to primary viral infection in elderly humans. J Immunol (2015) 195(10):4699–711. doi:10.4049/jimmunol.1500598
84. Vallejo AN. CD28 extinction in human T cells: altered functions and the program of T-cell senescence. Immunol Rev (2005) 205(1):158–69. doi:10.1111/j.0105-2896.2005.00256.x
85. O’Bryan JM, Woda M, Co M, Mathew A, Rothman AL. Telomere length dynamics in human memory T cells specific for viruses causing acute or latent infections. Immun Ageing (2013) 10:37. doi:10.1186/1742-4933-10-37
86. Wertheimer AM, Bennett MS, Park B, Uhrlaub JL, Martinez C, Pulko V, et al. Aging and cytomegalovirus infection differentially and jointly affect distinct circulating T cell subsets in humans. J Immunol (2014) 192(5):2143–55. doi:10.4049/jimmunol.1301721
87. Novak EJ, Liu AW, Nepom GT, Kwok WW. MHC class II tetramers identify peptide-specific human CD4(+) T cells proliferating in response to influenza A antigen. J Clin Invest (1999) 104(12):R63–7. doi:10.1172/JCI8476
88. Marshall D, Sealy R, Sangster M, Coleclough C. Cells primed during influenza virus infection provide help for qualitatively distinct antibody responses to subsequent immunization. J Immunol (1999) 163(9):4673–82.
89. Reiner SL, Sallusto F, Lanzavecchia A. Division of labor with a workforce of one: challenges in specifying effector and memory T cell fate. Science (2007) 317(5838):622–5. doi:10.1126/science.1143775
90. Sakaguchi S, Powrie F. Emerging challenges in regulatory T cell function and biology. Science (2007) 317(5838):627–9. doi:10.1126/science.1142331
91. Wahl SM, Chen W. Transforming growth factor-β-induced regulatory T cells referee inflammatory and autoimmune diseases. Arthritis Res Ther (2005) 7(2):62–8. doi:10.1186/ar1504
92. Mansfield AS, Nevala WK, Dronca RS, Leontovich AA, Shuster L, Markovic SN. Normal ageing is associated with an increase in Th2 cells, MCP-1 (CCL1) and RANTES (CCL5), with differences in sCD40L and PDGF-AA between sexes. Clin Exp Immunol (2012) 170(2):186–93. doi:10.1111/j.1365-2249.2012.04644.x
93. Hsu H-C, Scott DK, Mountz JD. Impaired apoptosis and immune senescence – cause or effect? Immunol Rev (2005) 205(1):130–46. doi:10.1111/j.0105-2896.2005.00270.x
94. Taylor SF, Cottey RJ, Zander DS, Bender BS. Influenza infection of beta 2-microglobulin-deficient (beta 2m-/-) mice reveals a loss of CD4+ T cell functions with aging. J Immunol (1997) 159(7):3453–9.
95. Graham M, Braciale V, Braciale T. Influenza virus-specific CD4+ T helper type 2 T lymphocytes do not promote recovery from experimental virus infection. J Exp Med (1994) 180(4):1273–82. doi:10.1084/jem.180.4.1273
96. Schmitt N, Morita R, Bourdery L, Bentebibel SE, Zurawski SM, Banchereau J, et al. Human dendritic cells induce the differentiation of interleukin-21-producing T follicular helper-like cells through interleukin-12. Immunity (2009) 31(1):158–69. doi:10.1016/j.immuni.2009.04.016
97. Ma CS, Suryani S, Avery DT, Chan A, Nanan R, Santner-Nanan B, et al. Early commitment of naive human CD4+ T cells to the T follicular helper (TFH) cell lineage is induced by IL-12. Immunol Cell Biol (2009) 87:590–600. doi:10.1038/icb.2009.64
98. Agrawal A, Su H, Chen J, Osann K, Agrawal S, Gupta S. Increased IL-21 secretion by aged CD4+T cells is associated with prolonged STAT-4 activation and CMV seropositivity. Aging (2012) 4(9):648–59. doi:10.18632/aging.100490
99. Fröhlich A, Kisielow J, Schmitz I, Freigang S, Shamshiev AT, Weber J, et al. IL-21R on T cells is critical for sustained functionality and control of chronic viral infection. Science (2009) 324(5934):1576–80. doi:10.1126/science.1172815
100. Yi JS, Du M, Zajac AJ. A vital role for IL-21 in the control of a chronic viral infection. Science (2009) 324(5934):1572–6. doi:10.1126/science.1175194
101. Elsaesser H, Sauer K, Brooks DG. IL-21 is required to control chronic viral infection. Science (2009) 324(5934):1569–72. doi:10.1126/science.1174182
102. Publicover J, Goodsell A, Nishimura S, Vilarinho S, Wang Z, Avanesyan L, et al. IL-21 is pivotal in determining age-dependent effectiveness of immune responses in a mouse model of human hepatitis B. J Clin Invest (2011) 121(3):1154–62. doi:10.1172/JCI44198
103. Dodd JS, Clark D, Muir R, Korpis C, Openshaw PJM. Endogenous IL-21 regulates pathogenic mucosal CD4 T-cell responses during enhanced RSV disease in mice. Mucosal Immunol (2013) 6(4):704–17. doi:10.1038/mi.2012.108
104. Boyden AW, Legge KL, Waldschmidt TJ. Pulmonary infection with influenza a virus induces site-specific germinal center and T follicular helper cell responses. PLoS One (2012) 7(7):e40733. doi:10.1371/journal.pone.0040733
105. Crotty S. A brief history of T cell help to B cells. Nat Rev Immunol (2015) 15(3):185–9. doi:10.1038/nri3803
106. Swain SL, McKinstry KK, Strutt TM. Expanding roles for CD4+ T cells in immunity to viruses. Nat Rev Immunol (2012) 12(2):136–48. doi:10.1038/nri3152
107. Breitfeld D, Ohl L, Kremmer E, Ellwart J, Sallusto F, Lipp M, et al. Follicular B helper T cells express CXC chemokine receptor 5, localize to B cell follicles, and support immunoglobulin production. J Exp Med (2000) 192(11):1545–52. doi:10.1084/jem.192.11.1545
108. Ozaki K, Spolski R, Feng CG, Qi C-F, Cheng J, Sher A, et al. A critical role for IL-21 in regulating immunoglobulin production. Science (2002) 298(5598):1630–4. doi:10.1126/science.1077002
109. Spensieri F, Borgogni E, Zedda L, Bardelli M, Buricchi F, Volpini G, et al. Human circulating influenza-CD4+ ICOS1+IL-21+ T cells expand after vaccination, exert helper function, and predict antibody responses. Proc Natl Acad Sci U S A (2013) 110(35):14330–5. doi:10.1073/pnas.1311998110
110. Bentebibel S-E, Lopez S, Obermoser G, Schmitt N, Mueller C, Harrod C, et al. Induction of ICOS+CXCR3+CXCR5+ TH cells correlates with antibody responses to influenza vaccination. Sci Transl Med (2013) 5(176):176ra32. doi:10.1126/scitranslmed.3005191
111. Herati RS, Reuter MA, Dolfi DV, Mansfield KD, Aung H, Badwan OZ, et al. Circulating CXCR5+PD-1+ response predicts influenza vaccine antibody responses in young adults but not elderly adults. J Immunol (2014) 193(7):3528–37. doi:10.4049/jimmunol.1302503
112. Parmigiani A, Alcaide ML, Freguja R, Pallikkuth S, Frasca D, Fischl MA, et al. Impaired antibody response to influenza vaccine in HIV-infected and uninfected aging women is associated with immune activation and inflammation. PLoS One (2013) 8(11):e79816. doi:10.1371/journal.pone.0079816
113. Sylwester AW, Mitchell BL, Edgar JB, Taormina C, Pelte C, Ruchti F, et al. Broadly targeted human cytomegalovirus-specific CD4+ and CD8+ T cells dominate the memory compartments of exposed subjects. J Exp Med (2005) 202(5):673–85. doi:10.1084/jem.20050882
114. Vescovini R, Biasini C, Fagnoni FF, Telera AR, Zanlari L, Pedrazzoni M, et al. Massive load of functional effector CD4+ and CD8+ T cells against cytomegalovirus in very old subjects. J Immunol (2007) 179(6):4283–91. doi:10.4049/jimmunol.179.6.4283
115. Polić B, Hengel H, Krmpotić A, Trgovcich J, Pavić I, Lučin P, et al. Hierarchical and redundant lymphocyte subset control precludes cytomegalovirus replication during latent infection. J Exp Med (1998) 188(6):1047–54. doi:10.1084/jem.188.6.1047
116. Pera A, Campos C, López N, Hassouneh F, Alonso C, Tarazona R, et al. Immunosenescence: implications for response to infection and vaccination in older people. Maturitas (2015) 82(1):50–5. doi:10.1016/j.maturitas.2015.05.004
117. Waller ECP, Day E, Sissons JGP, Wills MR. Dynamics of T cell memory in human cytomegalovirus infection. Med Microbiol Immunol (2008) 197(2):83–98. doi:10.1007/s00430-008-0082-5
118. Almanzar G, Schwaiger S, Jenewein B, Keller M, Herndler-Brandstetter D, Würzner R, et al. Long-term cytomegalovirus infection leads to significant changes in the composition of the CD8+ T-Cell repertoire, which may be the basis for an imbalance in the cytokine production profile in elderly persons. J Virol (2005) 79(6):3675–83. doi:10.1128/JVI.79.6.3675-3683.2005
119. O’Hara GA, Welten SPM, Klenerman P, Arens R. Memory T cell inflation: understanding cause and effect. Trends Immunol (2012) 33(2):84–90. doi:10.1016/j.it.2011.11.005
120. Sansoni P, Vescovini R, Fagnoni F, Biasini C, Zanni F, Zanlari L, et al. The immune system in extreme longevity. Exp Gerontol (2008) 43(2):61–5. doi:10.1016/j.exger.2007.06.008
121. Gamadia LE, Remmerswaal EBM, Weel JF, Bemelman F, van Lier RAW, Ten Berge IJM. Primary immune responses to human CMV: a critical role for IFN-γ-producing CD4+ T cells in protection against CMV disease. Blood (2003) 101(7):2686–92. doi:10.1182/blood-2002-08-2502
122. Gibson L, Piccinini G, Lilleri D, Revello MG, Wang Z, Markel S, et al. Human cytomegalovirus proteins pp65 and immediate early protein 1 are common targets for CD8+ T cell responses in children with congenital or postnatal human cytomegalovirus infection. J Immunol (2004) 172(4):2256–64. doi:10.4049/jimmunol.172.4.2256
123. Munks MW, Cho KS, Pinto AK, Sierro S, Klenerman P, Hill AB. Four distinct patterns of memory CD8 T cell responses to chronic murine cytomegalovirus infection. J Immunol (2006) 177(1):450–8. doi:10.4049/jimmunol.177.1.450
124. Ouyang Q, Wikby A, Walter S, Aubert G, Dodi AI, Travers P, et al. Large numbers of dysfunctional CD8+ T lymphocytes bearing receptors for a single dominant CMV epitope in the very old. J Clin Immunol (2003) 23(4):247–57. doi:10.1023/A:1024580531705
125. Franceschi C, Bonafè M, Valensin S. Human immunosenescence: the prevailing of innate immunity, the failing of clonotypic immunity, and the filling of immunological space. Vaccine (2000) 18(16):1717–20. doi:10.1016/S0264-410X(99)00513-7
126. Čičin-Šain L, Messaoudi I, Park B, Currier N, Planer S, Fischer M, et al. Dramatic increase in naïve T cell turnover is linked to loss of naïve T cells from old primates. Proc Natl Acad Sci U S A (2007) 104(50):19960–5. doi:10.1073/pnas.0705905104
127. Ahmed M, Lanzer KG, Yager EJ, Adams PS, Johnson LL, Blackman MA. Clonal expansions and loss of receptor diversity in the naive CD8 T cell repertoire of aged mice. J Immunol (2009) 182(2):784–92. doi:10.4049/jimmunol.182.2.784
128. Tsukamoto H, Clise-Dwyer K, Huston GE, Duso DK, Buck AL, Johnson LL, et al. Age-associated increase in lifespan of naïve CD4 T cells contributes to T-cell homeostasis but facilitates development of functional defects. Proc Natl Acad Sci U S A (2009) 106(43):18333–8. doi:10.1073/pnas.0910139106
129. Kilpatrick RD, Rickabaugh T, Hultin LE, Hultin P, Hausner MA, Detels R, et al. Homeostasis of the naive CD4(+) T cell compartment during aging. J Immunol (2008) 180(3):1499–507. doi:10.4049/jimmunol.180.10.6437-a
130. Gil A, Yassai MB, Naumov YN, Selin LK. Narrowing of human influenza a virus-specific T cell receptor α and β repertoires with increasing age. J Virol (2015) 89(8):4102–16. doi:10.1128/JVI.03020-14
131. van de Sandt CE, Kreijtz JHCM, Rimmelzwaan GF. Evasion of influenza a viruses from innate and adaptive immune responses. Viruses (2012) 4(9):1438–76. doi:10.3390/v4091438
132. Topham DJ, Tripp RA, Doherty PC. CD8+ T cells clear influenza virus by perforin or Fas-dependent processes. J Immunol (1997) 159(11):5197–200.
133. Brincks EL, Katewa A, Kucaba TA, Griffith TS, Legge KL. CD8 T cells utilize TRAIL to control influenza virus infection. J Immunol (2008) 181(7):4918–25. doi:10.4049/jimmunol.181.7.4918
134. Metkar SS, Menaa C, Pardo J, Wang B, Wallich R, Freudenberg M, et al. Human and mouse granzyme a induce a proinflammatory cytokine response. Immunity (2008) 29(5):720–33. doi:10.1016/j.immuni.2008.08.014
135. Brown DM, Lampe AT, Workman AM. The differentiation and protective function of cytolytic CD4 T cells in influenza infection. Front Immunol (2016) 7:93. doi:10.3389/fimmu.2016.00093
136. Shahid Z, Kleppinger A, Gentleman B, Falsey AR, McElhaney JE. Clinical and immunologic predictors of influenza illness among vaccinated older adults. Vaccine (2010) 28(38):6145–51. doi:10.1016/j.vaccine.2010.07.036
137. Zhou X, McElhaney JE. Age-related changes in memory and effector T cells responding to influenza A/H3N2 and pandemic A/H1N1 strains in humans. Vaccine (2011) 29(11):6145–51. doi:10.1016/j.vaccine.2010.12.029
138. Lee JB, Oelke M, Ramachandra L, Canaday DH, Schneck JP. Decline of influenza-specific CD8+ T cell repertoire in healthy geriatric donors. Immun Ageing (2011) 8:1. doi:10.1186/1742-4933-8-6
139. McElhaney JE, Ewen C, Zhou X, Kane KP, Xie D, Hager WD, et al. Granzyme B: correlates with protection and enhanced CTL response to influenza vaccination in older adults. Vaccine (2009) 27(18):2418–24. doi:10.1016/j.vaccine.2009.01.136
140. McElhaney JE, Xie D, Hager WD, Barry MB, Wang Y, Kleppinger A, et al. T cell responses are better correlates of vaccine protection in the elderly. J Immunol (2006) 176(1):6333–9. doi:10.4049/jimmunol.176.10.6333
141. Nowacki TM, Kuerten S, Zhang W, Shive CL, Kreher CR, Boehm BO, et al. Granzyme B production distinguishes recently activated CD8(+) memory cells from resting memory cells. Cell Immunol (2007) 247(1):36–48. doi:10.1016/j.cellimm.2007.07.004
142. Brown DM, Dilzer AM, Meents DL, Swain SL. CD4 T cell-mediated protection from lethal influenza: perforin and antibody-mediated mechanisms give a one-two punch. J Immunol (2006) 177(5):2888–98. doi:10.4049/jimmunol.177.5.2888
143. Brown DM, Lee S, Garcia-Hernandez M, Swain SL. Multifunctional CD4 cells expressing gamma interferon and perforin mediate protection against lethal influenza virus infection. J Virol (2012) 86(12):6792–803. doi:10.1128/JVI.07172-11
144. Strutt TM, McKinstry KK, Kuang Y, Bradley LM, Swain SL. Memory CD4(+) T-cell-mediated protection depends on secondary effectors that are distinct from and superior to primary effectors. Proc Natl Acad Sci U S A (2012) 109(38):E2551–60. doi:10.1073/pnas.1205894109
145. McKinstry KK, Strutt TM, Buck A, Curtis JD, Dibble JP, Huston G, et al. IL-10 deficiency unleashes an influenza-specific Th17 response and enhances survival against high dose challenge. J Immunol (2009) 182(12):7353–63. doi:10.4049/jimmunol.0900657
146. Sun K, Torres L, Metzger DW. A detrimental effect of interleukin-10 on protective pulmonary humoral immunity during primary influenza A virus infection. J Virol (2010) 84(10):5007–14. doi:10.1128/JVI.02408-09
147. McElhaney JE, Zhou X, Talbot HK, Soethout E, Bleackley RC, Granville DJ, et al. The unmet need in the elderly: how immunosenescence, CMV infection, co-morbidities and frailty are a challenge for the development of more effective influenza vaccines. Vaccine (2012) 30(12):2060–7. doi:10.1016/j.vaccine.2012.01.015
148. Hiebert PR, Boivin WA, Abraham T, Pazooki S, Zhao H, Granville DJ. Granzyme B contributes to extracellular matrix remodeling and skin aging in apolipoprotein E knockout mice. Exp Gerontol (2011) 46(6):489–99. doi:10.1016/j.exger.2011.02.004
149. Afonina IS, Tynan GA, Logue SE, Cullen SP, Bots M, Lüthi AU, et al. Granzyme B-dependent proteolysis acts as a switch to enhance the pro-inflammatory activity of IL-1α. Mol Cell (2011) 44(2):265–78. doi:10.1016/j.molcel.2011.07.037
150. Granville DJ. Granzymes in disease: bench to bedside. Cell Death Differ (2010) 17(4):565–6. doi:10.1038/cdd.2009.218
151. McLeod JD. Apoptotic capability in ageing T cells. Mech Ageing Dev (2001) 121(1–3):151–9. doi:10.1016/S0047-6374(00)00206-2
152. Effros RB, Boucher N, Porter V, Zhu X, Spaulding C, Walford RL, et al. Decline in CD28+ T cells in centenarians and in long-term T cell cultures: a possible cause for both in vivo and in vitro immunosenescence. Exp Gerontol (1994) 29(6):601–9. doi:10.1016/0531-5565(94)90073-6
153. Nociari MM, Telford W, Russo C. Postthymic development of CD28−CD8+ T cell subset: age-associated expansion and shift from memory to naive phenotype. J Immunol (1999) 162(6):3327–35.
154. Xie D, McElhaney JE. Lower GrB+CD62L(high) CD8 T(CM) effector lymphocyte response to influenza virus in older adults is associated with increased CD28(null) CD8 T lymphocytes. Mech Ageing Dev (2007) 128(5–6):392–400. doi:10.1016/j.mad.2007.05.001
155. Tortorella C, Loria MP, Piazzolla G, Schulze-Koops H, Lipsky PE, Jirillo E, et al. Age-related impairment of T cell proliferative responses related to the decline of CD28+ T cell subsets. Arch Gerontol Geriatr (1997) 26(1):55–70. doi:10.1016/S0167-4943(97)00033-2
156. Di Benedetto S, Derhovanessian E, Steinhagen-Thiessen E, Goldeck D, Muller L, Pawelec G. Impact of age, sex and CMV-infection on peripheral T cell phenotypes: results from the Berlin BASE-II Study. Biogerontology (2015) 16(5):631–43. doi:10.1007/s10522-015-9563-2
157. van der Heiden M, van Zelm MC, Bartol SJW, de Rond LGH, Berbers GAM, Boots AMH, et al. Differential effects of Cytomegalovirus carriage on the immune phenotype of middle-aged males and females. Sci Rep (2016) 6:26892. doi:10.1038/srep26892
158. Derhovanessian E, Theeten H, Hähnel K, Van Damme P, Cools N, Pawelec G. Cytomegalovirus-associated accumulation of late-differentiated CD4 T-cells correlates with poor humoral response to influenza vaccination. Vaccine (2013) 31(4):685–90. doi:10.1016/j.vaccine.2012.11.041
159. Derhovanessian E, Maier AB, Hähnel K, Beck R, de Craen AJM, Slagboom EP, et al. Infection with cytomegalovirus but not herpes simplex virus induces the accumulation of late-differentiated CD4+ and CD8+ T-cells in humans. J Gen Virol (2011) 92(12):2746–56. doi:10.1099/vir.0.036004-0
160. Libri V, Azevedo RI, Jackson SE, Di Mitri D, Lachmann R, Fuhrmann S, et al. Cytomegalovirus infection induces the accumulation of short-lived, multifunctional CD4(+) CD45RA(+) CD27(−) T cells: the potential involvement of interleukin-7 in this process. Immunology (2011) 132(3):326–39. doi:10.1111/j.1365-2567.2010.03386.x
161. Derhovanessian E, Maier AB, Hähnel K, Zelba H, de Craen AJM, Roelofs H, et al. Lower proportion of naïve peripheral CD8+ T cells and an unopposed pro-inflammatory response to human cytomegalovirus proteins in vitro are associated with longer survival in very elderly people. Age (2013) 35(4):1387–99. doi:10.1007/s11357-012-9425-7
162. Wang Y, Zhang J, Qiao F, Zhu J, Yin F, Han H. Correlation between T lymphocyte subsets in peripheral blood lymphocytes and 2-year all-cause mortality in an apparently healthy elderly Chinese cohort. Chin Med J (Engl) (2012) 125(6):1121–6.
163. Huppert FA, Pinto EM, Morgan K, Brayne C. Survival in a population sample is predicted by proportions of lymphocyte subsets. Mech Ageing Dev (2003) 124(4):449–51. doi:10.1016/S0047-6374(03)00021-6
164. Olsson J, Wikby A, Johansson B, Löfgren S, Nilsson B-O, Ferguson FG. Age-related change in peripheral blood T-lymphocyte subpopulations and cytomegalovirus infection in the very old: the Swedish longitudinal OCTO immune study. Mech Ageing Dev (2001) 121(1–3):157–201. doi:10.1016/S0047-6374(00)00210-4
165. Fagnoni FF, Vescovini R, Mazzola M, Bologna G, Nigro E, Lavagetto G, et al. Expansion of cytotoxic CD8+ CD28- T cells in healthy ageing people, including centenarians. Immunology (1996) 88(4):501–7. doi:10.1046/j.1365-2567.1996.d01-689.x
166. Wack A, Cossarizza A, Heltai S, Barbieri D, D’Addato S, Fransceschi C, et al. Age-related modifications of the human alphabeta T cell repertoire due to different clonal expansions in the CD4+ and CD8+ subsets. Int Immunol (1998) 10(9):1281–8. doi:10.1093/intimm/10.9.1281
167. Posnett DN, Edinger JW, Manavalan JS, Irwin C, Marodon G. Differentiation of human CD8 T cells: implications for in vivo persistence of CD8+ CD28- cytotoxic effector clones. Int Immunol (1999) 11(2):229–41. doi:10.1093/intimm/11.2.229
168. Hadrup SR, Strindhall J, Køllgaard T, Seremet T, Johansson B, Pawelec G, et al. Longitudinal studies of clonally expanded CD8 T cells reveal a repertoire shrinkage predicting mortality and an increased number of dysfunctional cytomegalovirus-specific T cells in the very elderly. J Immunol (2006) 176(4):2645–53. doi:10.4049/jimmunol.176.4.2645
169. Strindhall J, Ernerudh J, Mörner A, Waalen K, Löfgren S, Matussek A, et al. Humoral response to influenza vaccination in relation to pre-vaccination antibody titres, vaccination history, cytomegalovirus serostatus and CD4/CD8 ratio. Infect Dis (2016) 48(6):436–42. doi:10.3109/23744235.2015.1135252
170. Johnstone J, Parsons R, Botelho F, Millar J, McNeil S, Fulop T, et al. T-cell phenotypes predictive of frailty and mortality in elderly nursing home residents. J Am Geriatr Soc (2017) 65(1):153–9. doi:10.1111/jgs.14507
171. Taubenberger JK, Kash JC. Influenza virus evolution, host adaptation and pandemic formation. Cell Host Microbe (2010) 7(6):440–51. doi:10.1016/j.chom.2010.05.009
172. Heiny AT, Miotto O, Srinivasan KN, Khan AM, Zhang GL, Brusic V, et al. Evolutionarily conserved protein sequences of influenza A viruses, avian and human, as vaccine targets. PLoS One (2007) 2(11):e1190. doi:10.1371/journal.pone.0001190
173. Effros RB. Role of T lymphocyte replicative senescence in vaccine efficacy. Vaccine (2007) 25(4):599–604. doi:10.1016/j.vaccine.2006.08.032
174. Ohmit SE, Victor JC, Rotthoff JR, Teich ER, Truscon RK, Baum LL, et al. Prevention of antigenically drifted influenza by inactivated and live attenuated vaccines. N Engl J Med (2006) 355(24):2513–22. doi:10.1056/NEJMoa061850
175. Sasaki S, He X-S, Holmes TH, Dekker CL, Kemble GW, Arvin AM, et al. Influence of prior influenza vaccination on antibody and B-cell responses. PLoS One (2008) 3(8):e2975. doi:10.1371/journal.pone.0002975
176. Camilloni B, Nunzi E, Basileo M, Iorio AM. Antibody responses after influenza vaccination in elderly people: useful information from a 27-year study (from 1988–1989 to 2014–2015). In: Baddour M, editor. The Steps Forward in Diagnosing and Controlling Influenza. InTech (2016).
177. Osterholm MT, Kelley NS, Sommer A, Belongia EA. Efficacy and effectiveness of influenza vaccines: a systematic review and meta-analysis. Lancet (2012) 12(1):36–44. doi:10.1016/S1473-3099(11)70295-X
178. Slütter B, Pewe LL, Lauer P, Harty JT. Rapid boosting of cross-reactive memory CD8 T-cells broadens the protective capacity of the Flumist(®) vaccine. J Immunol (2013) 190(8):3854–8. doi:10.4049/jimmunol.1202790
179. DiazGranados C, Dunning A, Jordanov E, Landolfi V, Denis M, Talbot H. High-dose trivalent influenza vaccine compared to standard dose vaccine in elderly adults: safety, immunogenicity and relative efficacy during the 2009–2010 season. Vaccine (2013) 31(6):861–6. doi:10.1016/j.vaccine.2012.12.013
180. Falsey A, Treanor J, Tornieporth N, Capellan J, Gorse G. Randomized, double-blind controlled phase 3 trial comparing the immunogenicity of high-dose and standard-dose influenza vaccine in adults 65 years of age and older. J Infect Dis (2009) 200(2):172–80. doi:10.1086/599790
181. Pilkinton MA, Nicholas KJ, Warren CM, Smith RM, Yoder SM, Talbot HK, et al. Greater activation of peripheral T follicular helper cells following high dose influenza vaccine in older adults forecasts seroconversion. Vaccine (2017) 35(2):329–36. doi:10.1016/j.vaccine.2016.11.059
182. Kim JH, Talbot HK, Mishina M, Zhu Y, Chen J, Cao W, et al. High-dose influenza vaccine favors acute plasmablast responses rather than long-term cellular responses. Vaccine (2016) 34(38):4594–601. doi:10.1016/j.vaccine.2016.07.018
183. Chen WH, Cross AS, Edelman R, Sztein MB, Blackwelder WC, Pasetti MF. Antibody and Th1-type cell-mediated immune responses in elderly and young adults immunized with the standard or a high dose influenza vaccine. Vaccine (2011) 29(16):2865–73. doi:10.1016/j.vaccine.2011.02.017
184. DiazGranados CA, Dunning AJ, Kimmel M, Kirby D, Treanor J, Collins A, et al. Efficacy of high-dose versus standard-dose influenza vaccine in older adults. N Engl J Med (2014) 371(7):635–45. doi:10.1056/NEJMoa1315727
185. Coler RN, Baldwin SL, Shaverdian N, Bertholet S, Reed SJ, Raman VS, et al. A synthetic adjuvant to enhance and expand immune responses to influenza vaccines. PLoS One (2010) 5(10):e13677. doi:10.1371/journal.pone.0013677
186. Behzad H, Huckriede ALW, Haynes L, Gentleman B, Coyle K, Wilschut JC, et al. GLA-SE, a synthetic toll-like receptor 4 agonist, enhances T-cell responses to influenza vaccine in older adults. J Infect Dis (2011) 205(3):466–73. doi:10.1093/infdis/jir769
187. Coler RN, Bertholet S, Moutaftsi M, Guderian JA, Windish HP, Baldwin SL, et al. Development and characterization of synthetic glucopyranosyl lipid adjuvant system as a vaccine adjuvant. PLoS One (2011) 6(1):e16333. doi:10.1371/journal.pone.0016333
188. Janssen N, Derhovanessian E, Demuth I, Arnaout F, Steinhagen-Thiessen E, Pawelec G. Responses of dendritic cells to TLR-4 stimulation are maintained in the elderly and resist the effects of CMV infection seen in the young. J Gerontol A Biol Sci Med Sci (2016) 71(9):1117–23. doi:10.1093/gerona/glv119
189. Lambe T, Carey JB, Li Y, Spencer AJ, van Laarhoven A, Mullarkey CE, et al. Immunity against heterosubtypic influenza virus induced by adenovirus and MVA expressing nucleoprotein and matrix protein-1. Sci Rep (2013) 3:1443. doi:10.1038/srep01443
190. Epstein SL, Tumpey TM, Misplon JA, Lo C-Y, Cooper LA, Subbarao K, et al. DNA vaccine expressing conserved influenza virus proteins protective against H5N1 challenge infection in mice. Emerg Infect Dis (2002) 8(8):796–801. doi:10.3201/eid0808.010476
191. Doucet J-D, Forget M-A, Grange C, Rouxel RN, Arbour N, von Messling V, et al. Endogenously expressed matrix protein M1 and nucleoprotein of influenza A are efficiently presented by class I and class II major histocompatibility complexes. J Gen Virol (2011) 92(5):1162–71. doi:10.1099/vir.0.029777-0
192. Minor DP. Assaying the potency of influenza vaccines. Vaccines (Basel) (2015) 3(1):90–104. doi:10.3390/vaccines3010090
193. Brito LA, Chan M, Shaw CA, Hekele A, Carsillo T, Schaefer M, et al. A cationic nanoemulsion for the delivery of next-generation RNA vaccines. Mol Ther (2014) 22(12):2118–29. doi:10.1038/mt.2014.133
194. Bogers WM, Oostermeijer H, Mooij P, Koopman G, Verschoor EJ, Davis D, et al. Potent immune responses in rhesus macaques induced by nonviral delivery of a self-amplifying RNA vaccine expressing HIV Type 1 envelope with a cationic nanoemulsion. J Infect Dis (2015) 211(6):647–55. doi:10.1093/infdis/jiu522
195. Edwards DK, Jasny E, Yoon H, Horscroft N, Schanen B, Geter T, et al. Adjuvant effects of a sequence-engineered mRNA vaccine: translational profiling demonstrates similar human and murine innate response. J Transl Med (2017) 15(1):798–801. doi:10.1186/s12967-016-1111-6
196. Petsch B, Schnee M, Vogel AB, Lange E, Hoffmann B, Voss D, et al. Protective efficacy of in vitro synthesized, specific mRNA vaccines against influenza A virus infection. Nat Biotechnol (2012) 30(12):1210–6. doi:10.1038/nbt.2436
197. Hekele A, Bertholet S, Archer J, Gibson DG, Palladino G, Brito LA, et al. Rapidly produced SAM(®) vaccine against H7N9 influenza is immunogenic in mice. Emerg Microbes Infect (2013) 2(8):e52. doi:10.1038/emi.2013.54
198. Brazzoli M, Magini D, Bonci A, Buccato S, Giovani C, Kratzer R, et al. Induction of broad-based immunity and protective efficacy by self-amplifying mRNA vaccines encoding influenza virus hemagglutinin. J Virol (2016) 90(1):332–44. doi:10.1128/JVI.01786-15
199. Berthoud TK, Hamill M, Lillie PJ, Hwenda L, Collins KA, Ewer KJ, et al. Potent CD8(+) T-cell immunogenicity in humans of a novel heterosubtypic influenza A vaccine, MVA-NP+M1. Clin Infect Dis (2011) 52(1):1–7. doi:10.1093/cid/ciq015
200. Antrobus RD, Lillie PJ, Berthoud TK, Spencer AJ, McLaren JE, Ladell K, et al. A T cell-inducing influenza vaccine for the elderly: safety and immunogenicity of MVA-NP+M1 in adults aged over 50 years. PLoS One (2012) 7(10):e48322. doi:10.1371/journal.pone.0048322
201. Peng B, Wang LR, Gómez-Román VR, Davis-Warren A, Montefiori DC, Kalyanaraman VS, et al. Replicating rather than nonreplicating adenovirus-human immunodeficiency virus recombinant vaccines are better at eliciting potent cellular immunity and priming high-titer antibodies. J Virol (2005) 79(16):10200–9. doi:10.1128/JVI.79.16.10200-10209.2005
202. Bodewes R, de Mutsert G, van der Klis FRM, Ventresca M, Wilks S, Smith DJ, et al. Prevalence of antibodies against seasonal influenza A and B viruses in children in Netherlands. Clin Vaccine Immunol (2011) 18(3):469–76. doi:10.1128/CVI.00396-10
203. Gras S, Kedzierski L, Valkenburg SA, Laurie K, Liu YC, Denholm JT, et al. Cross-reactive CD8(+) T-cell immunity between the pandemic H1N1-2009 and H1N1-1918 influenza A viruses. Proc Natl Acad Sci U S A (2010) 107(28):12599–604. doi:10.1073/pnas.1007270107
204. Webster RG. Immunity to influenza in the elderly. Vaccine (2000) 18(16):1686–9. doi:10.1016/S0264-410X(99)00507-1
205. Agrati C, Gioia C, Lalle E, Cimini E, Castilletti C, Armignacco O, et al. Association of profoundly impaired immune competence in H1N1v-infected patients with a severe or fatal clinical course. J Infect Dis (2010) 202(5):681–9. doi:10.1086/655469
206. Hammarlund E, Lewis MW, Hansen SG, Strelow LI, Nelson JA, Sexton GJ, et al. Duration of antiviral immunity after smallpox vaccination. Nat Med (2003) 9(9):1121–7. doi:10.1038/nm917
207. Hammarlund E, Lewis MW, Carter SV, Amanna I, Hansen SG, Strelow LI, et al. Multiple diagnostic techniques identify previously vaccinated individuals with protective immunity against monkeypox. Nat Med (2005) 11(9):1005–11. doi:10.1038/nm1273
208. Haynes L, Eaton SM, Burns EM, Randall TD, Swain SL. CD4 T cell memory derived from young naive cells functions well into old age, but memory generated from aged naive cells functions poorly. Proc Natl Acad Sci U S A (2003) 100(25):15053–8. doi:10.1073/pnas.2433717100
209. Valkenburg SA, Venturi V, Dang THY, Bird NL, Doherty PC, Turner SJ, et al. Early priming minimizes the age-related immune compromise of CD8(+) T cell diversity and function. PLoS Pathog (2012) 8(2):e1002544. doi:10.1371/journal.ppat.1002544
210. Kissling E, Nunes B, Robertson C, Valenciano M, Reuss A, Larrauri A, et al. I-MOVE multicentre case-control study 2010/11 to 2014/15: is there within-season waning of influenza type/subtype vaccine effectiveness with increasing time since vaccination? Euro Surveill (2016) 21:16. doi:10.2807/1560-7917.ES.2016.21.16.30201
211. Cordero E, Roca-Oporto C, Bulnes-Ramos A, Aydillo T, Gavaldà J, Moreno A, et al. Two doses of inactivated influenza vaccine improve immune response in solid organ transplant recipients: results of TRANSGRIPE 1-2, a randomized controlled clinical trial. Clin Infect Dis (2017) 64(7):829–38. doi:10.1093/cid/ciw855
212. Oxford JS, Al-Jabri AA, Lambkin R, Palache AM, Fleming DM. Non-responders to egg grown influenza vaccine seroconvert after booster immunization with MDCK cell grown vaccine. Vaccine (2003) 21(21–22):2743–6. doi:10.1016/S0264-410X(03)00214-7
213. McElhaney JE, Hooton JW, Hooton N, Bleackley RC. Comparison of single versus booster dose of influenza vaccination on humoral and cellular immune responses in older adults. Vaccine (2005) 23(25):3294–300. doi:10.1016/j.vaccine.2005.01.080
214. Hoft DF, Lottenbach K, Goll JB, Hill H, Winokur PL, Patel SM, et al. Priming vaccination with influenza virus H5 hemagglutinin antigen significantly increases the duration of T cell responses induced by a heterologous H5 booster vaccination. J Infect Dis (2016) 214(7):1020–9. doi:10.1093/infdis/jiw310
215. Sijmons S, Thys K, Mbong Ngwese M, Van Damme E, Dvorak J, Van Loock M, et al. High-throughput analysis of human cytomegalovirus genome diversity highlights the widespread occurrence of gene-disrupting mutations and pervasive recombination. J Virol (2015) 89(15):7673–95. doi:10.1128/JVI.00578-15
216. Ross SA, Novak Z, Pati S, Patro RK, Blumenthal J, Danthuluri VR, et al. Mixed infection and strain diversity in congenital cytomegalovirus infection. J Infect Dis (2011) 204(7):1003–7. doi:10.1093/infdis/jir457
217. Pass RF, Zhang C, Evans A, Simpson T, Andrews W, Huang M-L, et al. Vaccine prevention of maternal cytomegalovirus infection. N Engl J Med (2009) 360(12):1191–9. doi:10.1056/NEJMoa0804749
218. Schleiss MR, Bierle CJ, Swanson EC, McVoy MA, Wang JB, Al-Mahdi Z, et al. Vaccination with a live attenuated cytomegalovirus devoid of a protein kinase R inhibitory gene results in reduced maternal viremia and improved pregnancy outcome in a guinea pig congenital infection model. J Virol (2015) 89(19):9727–38. doi:10.1128/JVI.01419-15
219. Mori T, Kanda Y, Takenaka K, Okamoto S, Kato J, Kanda J, et al. Safety of ASP0113, a cytomegalovirus DNA vaccine, in recipients undergoing allogeneic hematopoietic cell transplantation: an open-label phase 2 trial. Int J Hematol (2017) 105(2):206–12. doi:10.1007/s12185-016-2110-3
220. Dropulic LK, Cohen JI. Update on new antivirals under development for the treatment of double-stranded DNA virus infections. Clin Pharmacol Ther (2010) 88(5):610–9. doi:10.1038/clpt.2010.178
Keywords: cytomegalovirus, influenza, vaccination, infection, older adult, aging, elderly, cytotoxic T-lymphocyte
Citation: Merani S, Pawelec G, Kuchel GA and McElhaney JE (2017) Impact of Aging and Cytomegalovirus on Immunological Response to Influenza Vaccination and Infection. Front. Immunol. 8:784. doi: 10.3389/fimmu.2017.00784
Received: 15 April 2017; Accepted: 21 June 2017;
Published: 17 July 2017
Edited by:
Jeffrey C. Nolz, Oregon Health & Science University, United StatesReviewed by:
Vaiva Vezys, University of Minnesota, United StatesTara Marlene Strutt, University of Central Florida, United States
Copyright: © 2017 Merani, Pawelec, Kuchel and McElhaney. This is an open-access article distributed under the terms of the Creative Commons Attribution License (CC BY). The use, distribution or reproduction in other forums is permitted, provided the original author(s) or licensor are credited and that the original publication in this journal is cited, in accordance with accepted academic practice. No use, distribution or reproduction is permitted which does not comply with these terms.
*Correspondence: Janet E. McElhaney, jmcelhaney@hsnri.ca