- 1Fundação Oswaldo Cruz, Fiocruz Rondônia, Porto Velho, Rondônia, Brazil
- 2Departamento de Medicina da Universidade Federal de Rondônia, UNIR, Porto Velho, Rondônia, Brazil
- 3Fundação Oswaldo Cruz, Fiocruz Ceará, Fortaleza, Ceará, Brazil
- 4Plataforma Bi-Institucional de Medicina Translacional (Fiocruz-USP), Ribeirão Preto, São Paulo, Brazil
Due mainly to properties such as high affinity and antigen specificity, antibodies have become important tools for biomedical research, diagnosis, and treatment of several human diseases. When the objective is to administer them for therapy, strategies are used to reduce the heterologous protein immunogenicity and to improve pharmacokinetic and pharmacodynamic characteristics. Size minimization contributes to ameliorate these characteristics, while preserving the antigen–antibody interaction site. Since the discovery that camelids produce functional antibodies devoid of light chains, studies have proposed the use of single domains for biosensors, monitoring and treatment of tumors, therapies for inflammatory and neurodegenerative diseases, drug delivery, or passive immunotherapy. Despite an expected increase in antibody and related products in the pharmaceutical market over the next years, few research initiatives are related to the development of alternatives for helping to manage neglected tropical diseases (NTDs). In this review, we summarize developments of camelid single-domain antibodies (VHH) in the field of NTDs. Particular attention is given to VHH-derived products, i.e., VHHs fused to nanoparticles, constructed for the development of rapid diagnostic kits; fused to oligomeric matrix proteins for viral neutralization; and conjugated with proteins for the treatment of human parasites. Moreover, paratransgenesis technology using VHHs is an interesting approach to control parasite development in vectors. With enormous biotechnological versatility, facility and low cost for heterologous production, and greater ability to recognize different epitopes, VHHs have appeared as an opportunity to overcome challenges related to the prevention, detection, and control of human diseases, especially NTDs.
Monoclonal Antibodies (mAbs): From Biotechnological Assays to Biopharmaceutical Market
The advent of hybridoma technology provided a great development in antibody engineering (1). Using this technology, mAbs with high specificity for proteins, nucleic acids, carbohydrates, and haptens have been produced satisfactorily over time (2). The specificity and affinity of mAbs, added to their homogeneity and unlimited availability, are essential to their applications in the biological sciences (3–5). However, for clinical application, these structures may have some disadvantages, such as low tissue distribution, adverse reactions related to non-human origin proteins (6, 7), and high cost of scale-up production.
Thus, the humanization of heterologous immunoglobulins (Igs), either by fusing the variable regions of mouse antibodies with the constant domains of human Igs or by inserting the murine CDRs into human variable chains, has been an alternative for increasing the effectiveness and safety of immunotherapy. Furthermore, recombinant DNA technology has allowed for the production of different antibody formats for use in biomedical studies, construction of biosensors, and/or therapy (8).
The strategy to minimize antibody size preserves antigen–antibody binding sites generating monovalent [fragment antigen binding (Fab)] fragments from IgGs, single-chain variable fragments (scFv), as well as single-domain antibodies (9). This approach aims to improve bioavailability and reduce immunogenicity, when the objective is to use them for pharmacological treatment. When these fragments are designed for radioimmunotherapy or in vivo imaging, the lack of a constant region [fragment crystallizable (Fc)] allows for better renal clearance (10). For diagnostic methods, like immunohistochemistry, the lack of Fc ensures, in addition to better tissue distribution, reduced non-specific binding.
It is important to mention that antibody fragments normally lack glycosylation, allowing for their production in prokaryotic expression systems, saving time and money (9). However, fragments lacking an Fc domain possess a shorter half-life than intact IgGs and are unable to elicit Fc-mediated cytotoxic processes (11), which require optimizations for antibody fragment products.
Since the introduction of the first therapeutic mAb (Orthoclone OKT3) in the biopharmaceutical market in 1986, several monoclonal antibody therapeutics have been approved for the treatment of diseases (2). After 30 years, the global sales revenue of biopharmaceutical products is nearly $150 billion. From these, about 50% are related to the sales of therapeutic mAbs or related products, including antibody fragments, antibody-drug conjugates, and Fc-fusion proteins (12).
Currently, about 50 monoclonal antibody products have been approved by the Food and Drug Administration, in the US, or European Medicines Agency in Europe, for the treatment of several diseases (12). Among the products, most of them were developed to treat chronic inflammatory diseases and cancer, but there are also products in the market for neurodegenerative disorders and for infectious diseases (13).
Advances in the understanding of disease physiopathology at a molecular level, together with recombinant DNA technology, and the intrinsic characteristics of antibodies, point to significant growth in this sector of the pharmaceutical market over the next few years (14). A rate of four to six new products per year is expected in the market, reaching about 70 monoclonal products in 2020, with worldwide sales of approximately $ 125 billion (12, 15).
Camelid Heavy-Chain Antibodies (HCAbs) and VHH Domain
The discovery that the Camelidae family produces a significant proportion of functional antibodies lacking L chains, along with molecular technologies, consolidated at the beginning of twenty-first century brought new perspectives for the antibody-bioengineering field (16, 17).
Referred to as camelid HCAbs (IgG2 and IgG3), these molecules have approximately 90 kDa, and the antigen recognition site is formed by a single domain, termed VHH (variable domain of camelid heavy-chain-only antibody) or nanobody (16, 18). The percentage of HCAbs in the total IgG of these animals represents 10–80%, indicating the importance of these antibodies in the immune protection of camelids (18, 19).
VHHs present a similar organization to that of VHs from conventional IgGs (1, 20). Both are composed of three variable CDRs interspersed with four conserved FRs, but with notable differences. To improve the molecular stability of VHHs, hydrophobic amino acid residues present in conventional VHs (FR2 regions) were substituted for smaller or more hydrophilic amino acids in VHHs (21–24). Besides that, CDRs 1 and 3 are usually larger, providing a compensatory antigen interaction surface in the absence of the VL domain (18, 25). This difference is observed mainly in the CDR3, which possesses an average of 18 amino acid residues, in contrast with about 14 residues in the VH orthologous region. Additional disulfide bonds formed frequently between CDR3 and CDR1 or FR2 restricts the flexibility of the prominent loop, ensuring greater stability for these molecules (26) (Figures 1A,B).
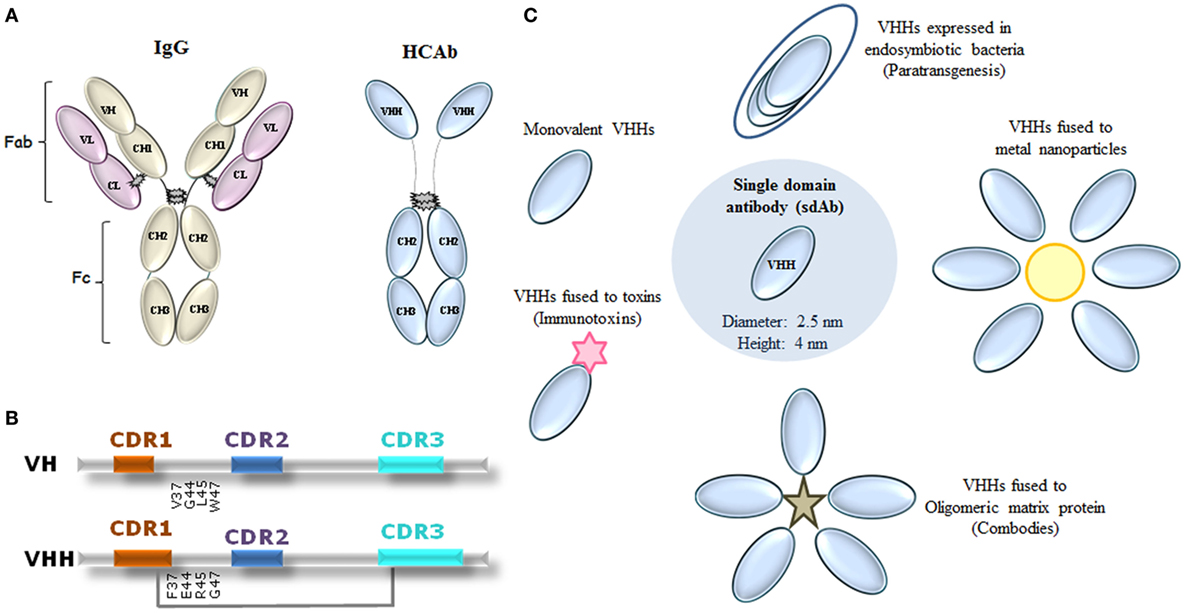
Figure 1. Representation of immunoglobulin (Ig) G molecules and uses of VHHs in neglected tropical diseases (NTDs). (A) Conventional IgG showing heavy and light domains that perform fragment antigen binding (Fab) and fragment crystallizable (Fc) regions, and camelid heavy chain [heavy-chain antibody (HCAb)] IgG. (B) Comparison between VH and VHH domains on the amino acid sequence level. CDR1 and CDR3 of VHHs are often larger than the respective VH regions. Amino acid substitutions in framework 2 are given, as well as an eventual extra disulfide bond between CDR1 and CDR3 (gray line). CDR1 indicated as red; CDR2 as blue, and CDR3 as light blue. Adapted from Ref. (24, 27–29). (C) Proposed uses of VHHs in NTDs. VHHs have been proposed in the following formats: monovalent, fused to proteins (immunotoxins), fused to oligomeric matrix proteins (combodies), conjugated to metal nanoparticles, and expressed by endosymbiotic bacteria in NTD vectors for a paratransgenic approach.
When compared to scFv or disulfide-stabilized Fv antibody fragment, VHHs present higher thermal stability, maintaining binding activity between 80 and 100% (30). Paving the biotechnological way, at high temperatures, conventional antibody fragments expose hydrophobic interfaces between VH and VL, inducing aggregation and precipitation. VHH possess a great thermal stability, and it may be related to the replacement of the hydrophobic amino acids (31).
With one-tenth the size of IgGs, nanometer dimensions, and extended CDRs, VHHs are capable of penetrating dense tissues and interacting with weakly antigenic epitopes for human or murine antibodies. A similarity greater than 80% with the FRs of human VH regions and high renal clearance justify their low immunogenicity (20, 32). Additionally, their high solubility, affinity, and specificity for molecular targets, stability, flexibility related to the construction of different formats (monomers, dimers—mono- or bispecifics, fused to drugs, etc.), multiple routes of administration, humanized construction possibilities, and low cost of production make VHHs important biotechnological tools.
Combining the advantages of mAbs with properties of small molecules, the possibilities of VHH applications in health are vast. Beyond the development of drugs for inflammatory and neurodegenerative diseases, along with antitumor and viral neutralizing agents, VHHs are useful for drug delivery and are interesting agents in diagnosing diseases (33–37). Furthermore, these fragments could be used as intrabody, directed to specific intracellular target proteins, aiming, for example, to inhibit viral replication (38, 39). In the field of serum therapy, VHHs have emerged as tools for antivenom development (40–43).
Although no therapeutic agent is currently available, several VHH-based products are under development. The company Ablynx, in collaboration with multiple pharmaceutical industries, has 40 product candidates for the treatment of cancer, inflammatory, and neurodegenerative diseases. Five products are in clinical development, and the first therapeutic agent based on VHH, anti-vWF, is expected to be sold in 2018 (44). Despite the expected increase in antibody development over the next years, many diseases remain neglected because drugs or diagnostic tools would not generate monetary profits for the big pharmaceutical companies (45, 46). Recently, public–private partnerships have emerged to develop medicines and health technologies to circumvent the challenges faced in NTDs (47).
Diagnostic and Treatment Challenges in NTDs
Neglected tropical diseases are a group of infectious diseases, caused by parasites, viruses, and bacteria, prevalent in 149 countries affecting more than one billion people (48). Most of these people live in extreme poverty without adequate sanitation and in close contact with infectious vectors and domestic animals and livestock. Causing relatively low mortality but high morbidity, NTDs generate serious consequences for individuals or entire communities in terms of lifestyle quality, loss of productivity and poverty aggravation, and cost billions of dollars every year to developing economies (49).
Today, 19 diseases are classified as NTDs, including Buruli ulcer, Chagas disease (CD), dengue, chikungunya, dracunculiasis, echinococcosis, foodborne trematodiases, human African trypanosomiasis (HAT), leishmaniasis, leprosy, lymphatic filariasis, onchocerciasis, rabies, schistosomiasis, soli-transmitted helminthiases, taeniasis/cysticercosis, trachoma, yaws, and mycetoma. Moreover, WHO recognizes that there are still many tropical and poverty-related diseases or conditions that remain neglected and also require adequate prevention and control approaches (48).
These diseases can be preventable and treatable by combining public political strategies. Besides provision of safe water, sanitation and hygiene (WASH), personal protection measures, and rigorous implementation of vector control are required in reducing disease transmission (47). Integrative actions between NTD and WASH programs, in addition to the mass drug administration (MDA), create conditions for more effective control of many NTDs (50).
However, for most NTDs, the diagnostic methods are limited, the MDA treatment regimen presents limitations, and the medications used are archaic presenting high toxicity or inadequate efficacy (51). Thus, safe and effective drugs, vaccines, and diagnostic devices remain important challenges to prevent or control the course of NTDs. Given the necessity to develop fast and effective diagnostic methods, as well as innovative approaches to treat NTDs, and the increasing use of antibody-based products in health, research groups have invested in the development of these alternatives to enhance the fight against NTDs (52–54). Although promising, the high cost of mAb production compromises its large-scale manufacture, especially for NTDs. Moreover, VHHs’ superior ability to recognize and neutralize antigens as compared to VHs of Fabs or scFvs, in addition to their lower production cost, instigates the development of VHH-based products for these diseases.
Versatile VHH Approaches for NTDs
Like lego bricks, VHH technology has used the modular concept for several antibody-based applications. Among the proposed uses of VHHs for NTDs are monovalent or conjugated VHH structures for recognizing or inhibiting selected targets (Table 1). As conjugated structures, VHHs could be fused to metal nanoparticles, especially gold, for construction of fast immunochromatographic tests; fused to oligomeric matrix proteins (Combodies), to amplify the affinity and ability in neutralizing viruses; conjugated with proteins able to lyse infectious agents, so-called immunotoxins; and expressed by endosymbiotic bacteria in NTD vectors, as potential in vivo drug delivery systems, a paratransgenic approach (Figure 1C).
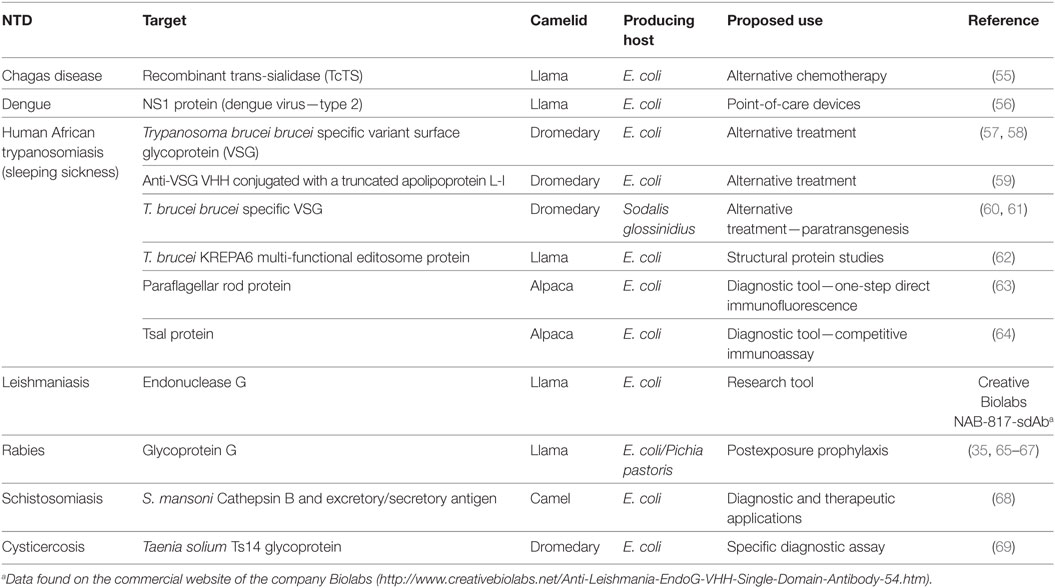
Table 1. Overview of published camelid single-domain antibodies developed against neglected tropical disease (NTD).
VHHs As a Tool against CD
Trypanosoma cruzi is the etiological agent of CD, an anthropozoonosis endemic to the American continent (70, 71), that affects about 10–12 million people worldwide (72). One of the mechanisms used by T. cruzi to survive in the mammalian host is related to the presence of highly diverse glycosylated mucins in parasite membrane (73, 74). Membrane anchored trans-sialidase (TcTS) participates in the addition of sialic acid in mucin coat, and so it is an important protein involved in the pathogenesis of T. cruzi (55, 75–77). TcTS activity was neutralized by antibodies found in patients with chronic CD, as well as in animals infected with T. cruzi (78–80). Passive transfer of anti-TcTS mAbs to infected animals prevents thrombocytopenia induced by the enzyme (81). Given the VHHs are often potent enzyme inhibitors, Ratier and colleagues (55) produced anti-TcTS VHHs (55). Despite inhibiting recombinant TcTS, the selected VHHs failed to neutralize purified TcTS from T. cruzi parasites. These results point to the relevance of diversity among members of the TcTS family (55, 82), which may be required for T. cruzi’s strategy to evade the host immune system.
VHHs As a Tool against Dengue
Belonging to the Flavivirus genus, the dengue virus (DENV) can cause visceral and central nervous system disease in humans. Each year, about 390 million people are infected with DENV and more than 3.6 billion people live in at-risk areas (83, 84). Currently, four DENV serotypes have been identified (83). Besides humans, the main vectors of the virus in nature are mosquitoes from the genus Aedes (73). These vectors contribute not only to Dengue outbreak but also to the spread of other mosquito-borne diseases, like Zika and chikungunya (85, 86). Vaccines for these diseases are at different stages of development, no specific treatment is available, and diagnostic methods are limited. Among the initiatives for the development of rapid diagnostic kits, Fatima and coworkers (56) produced VHHs and mAbs against DENV (type 2) NS1 non-structural protein aiming to construct two diagnostic kits based on an immunochromatographic assay (56). Comparing both devices, the VHH-based kit demonstrated better sensitivity and specificity against the antigen than the mAb-immobilized kit. Although the two antibodies recognize the same protein epitope, better results related to VHH devices might be due to VHHs’ longer CDR3 and their capability to bind to the cleft of the targeted antigen. This study demonstrated the viability of VHH-based point-of-care tests for the detection of DENV infection.
VHHs As a Tool against HAT (Sleeping Sickness)
About 300,000 human beings suffer from HAT, more than 60 million people live in areas with risk of infection (87). While Trypanosoma brucei gambiense causes the chronic form of the disease in western and central Africa, T. brucei rhodesiense is the etiological agent of an acute disease in eastern and southern Africa (88). Although the parasite and its vectors (tsetse flies, Glossina spp.) were identified more than a century ago, control of the disease remains elusive. With few drugs available, often causing toxicity, along with increasing drug resistance, the search for alternatives to treat the disease has become urgent (88, 89). Antigen variation is a defense mechanism adopted by trypanosomes to escape from the host–immune system. These parasites express numerous copies of the variant surface glycoprotein (VSG) in their membrane (88). Taking advantage of VHH properties, Stijlemans et al. (57) showed that anti-VSG VHHs were able to penetrate the VSG coat to target their epitope (57). Furthermore, Baral et al. (59) fused VHHs with truncated human trypanolytic factor [apolipoprotein L-I (apoL-I)] and demonstrated that the immunotoxins were capable of trypanolysis (59). Treatment with apoL-I–anti-VSG VHH conjugates resulted in curative and alleviating effects on acute and chronic infections in mice with both resistant and sensitive trypanosome strains. Caljon et al. (58) indicated that anti-VSG VHHs could penetrate the brain–blood barrier, especially in pathological conditions (58). Another strategy suggested to handle HAT is related to the control of trypanosome development in tsetse flies. Thus, De Vooght et al. (60), exploring paratransgenesis, expressed anti-VSG VHHs in the bacteria Sodalis glossinidius, an endosymbiotic microorganism of the fly (60). Subsequently, the group demonstrated that recombinant S. glossinidius can release anti-VSG VHHs in different tissues of Glossina morsitans morsitans (61). Beyond effective treatment, HAT requires efficient diagnostic devices. So, anti-paraflagellar rod protein (PRP) VHHs of trypanosomes or anti-Tsal protein, a biomarker present in tsetse fly saliva, seem to be alternatives for diagnosing the disease. While anti-PRP VHHs are suggested for the development of IFA assays, a T-sal VHH-based competitive immunoassay could be used to identify tsetse fly exposure (63, 64). Furthermore, anti-KREPA6 multifunctional protein of editosome VHHs of T. brucei has been proposed for use in structural protein studies (62).
VHHs As a Tool against Rabies
Rabies virus (RABV) causes approximately 59,000 human deaths per year (90). Shortly after exposure, patients should receive anti-rabies prophylaxis, which consists of passive immunization, with rabies-specific Igs, and a vaccine (65, 91). Issues related to the possibility of infectious agent transmission, high cost, and limited production, as well as the need for special storage conditions, instigate the development of new strategies for the disease. In 2015, the WHO and other groups announced a goal to eliminate rabies deaths worldwide by 2030. They call for cheaper and faster treatment for people and improved vaccinations in domestic dogs (90).
Since the outer envelope RABV glycoprotein was identified as the most significant surface antigen for generating virus-neutralizing antibodies, researchers have selected this target as a strategy to build broadened neutralizing antiviral molecules. In 2011, Hultberg and coworkers developed the first anti-RABV VHHs (35). VHH constructs improved the cross neutralization against viral strains, as well the viral neutralization potencies up to 1,500-fold, which was similar to or better than the best performing mAbs. Boruah and coworkers (66) fused anti-RABV VHHs with a peptide derived from the human cartilage oligomeric matrix protein (COMP48) to construct pentavalent multimers, called combodies (66). These combodies conferred protection for mice infected with lethal doses of RABV. Posteriorly, Terryn et al. (67) demonstrated the protective effect of VHH-based constructs against rabies induced in mice (67). Besides prolonging animal survival, anti-rabies VHHs were able to rescue mice from the disease. Construction of bivalent or biparatopic VHHs resulted in increased neutralizing potency, reaching a picomolar range. Recently, the same group showed that PEP using anti-RABV VHHs associated with vaccine administration improves protection of mice from lethal rabies infection. It is important to note that the vaccine alone, as well as the association of anti-RABV Igs with the vaccine, was unable to rescue mice from the lethal disease (65).
VHHs As a Tool against Schistosomiasis
Schistosomiasis, caused by a parasite of the genus Schistosoma, affects almost 240 million people worldwide. Infection is acquired when parasitic larvae (cercariae) penetrate the skin of people in contact with infested water. Based on the need to develop new approaches to schistosomiasis diagnosis and treatment, Sallam (68) developed an anti-schistosoma VHH–nanoparticle conjugate, using S. mansoni Cathepsin B and execratory secretory proteins, able of recognizing antigens from this parasite (68).
VHHs As a Tool against Taeniasis/Cysticercosis
Cysticercosis is a parasitic infection caused when humans ingest eggs of the pork tapeworm Taenia solium, and the larval form moves through the body and forms cysts in tissues, including the brain. With an objective of creating a more efficient diagnostic method, Deckers et al. (69) developed a specific VHH capable of discriminating the T. solium parasite from other Taenia species (69). The VHH recognizes a 14 kDa glycoprotein (Ts14) with subnanomolar affinity, using an antigen extract in the immunization of the animal. Purified polypeptides for immunization and panning could obtain VHHs with higher affinities, increasing the diagnostic/therapeutic use of anti-taenia VHHs, mainly in countries with critical sanitation problems.
Conclusion and Perspectives
Prophylactic and therapeutic aspects of various NTDs need more effective, cheaper, and faster approaches since currently there are limited methods for diagnosis, in some cases no vaccines, and the usual medications can cause systemic toxicity, besides constantly increasing drug resistance. Camelid VHHs conserve characteristics such as affinity and specificity of conventional IgGs, possess superior thermal stability, low immunogenicity, interesting pharmacokinetic and pharmacodynamic properties, biotechnological versatility, and can be cost-effectively produced in microorganisms.
All these attributes make VHHs valuable candidates for the development of alternative diagnostic tools, such as point-of-care devices, or safe drugs for a diverse number of diseases. Furthermore, bioinformatic algorithms, strategies of protein-driven evolution, and synthetic biology favor obtaining antibody molecules with improved properties. While researchers, mainly from Europe, are focused on the development of VHH-based products for inflammatory, cancer, and neurodegenerative disorders, the Oswaldo Cruz Foundation (Fiocruz) in Brazil established a VHH-development platform to produce these products aiming at the diagnosis or treatment of tropical diseases, mainly emerging arboviruses, as Zika, chikungunya, and yellow fever. To transform research results into products with high aggregate, social value is the big challenge in the area, since NTDs affect populations of the world’s smallest economies.
Author Contributions
CF made substantial contributions to the conception, design, and drafting of the manuscript; SP contributed to the preparation and revision of the manuscript; ML participated in drafting the article; JZ contributed to the writing and revision of the manuscript; GF made substantial contributions to design, acquisition of data, and revision of the manuscript; and RS participated in drafting the article and reviewed it critically for intellectual content.
Conflict of Interest Statement
The authors declare that the research was conducted in the absence of any commercial or financial relationships that could be construed as a potential conflict of interest.
The reviewer, JA, and handling editor declared their shared affiliation, and the handling editor states that the process nevertheless met the standards of a fair and objective review.
Acknowledgments
This work was supported in whole or part by Conselho Nacional de Desenvolvimento Científico e Tecnológico (CNPq), COBIO 420576/2013-4, and Coordenação de Aperfeiçoamento de Pessoal de Nível Superior (CAPES). The authors thank Amy Nicole Grabner for the English review of the manuscript.
References
1. Kohler G, Milstein C. Continuous cultures of fused cells secreting antibody of predefined specificity. Nature (1975) 256(5517):495–7. doi: 10.1038/256495a0
2. Liu JK. The history of monoclonal antibody development – progress, remaining challenges and future innovations. Ann Med Surg (2014) 3(4):113–6. doi:10.1016/j.amsu.2014.09.001
3. Huang L, Muyldermans S, Saerens D. Nanobodies®: proficient tools in diagnostics. Expert Rev Mol Diagn (2010) 10(6):777–85. doi:10.1586/erm.10.62
4. Sharma S, Byrne H, O’Kennedy RJ. Antibodies and antibody-derived analytical biosensors. Essays Biochem (2016) 60(1):9–18. doi:10.1042/EBC20150002
5. Siddiqui MZ. Monoclonal antibodies as diagnostics; an appraisal. Indian J Pharm Sci (2010) 72(1):12–7. doi:10.4103/0250-474X.62229
6. de Marco A. Biotechnological applications of recombinant single-domain antibody fragments. Microb Cell Fact (2011) 10:44. doi:10.1186/1475-2859-10-44
7. Hansel TT, Kropshofer H, Singer T, Mitchell JA, George AJ. The safety and side effects of monoclonal antibodies. Nat Rev Drug Discov (2010) 9(4):325–38. doi:10.1038/nrd3003
8. Ducancel F, Muller BH. Molecular engineering of antibodies for therapeutic and diagnostic purposes. MAbs (2012) 4(4):445–57. doi:10.4161/mabs.20776
10. Jain RK. Physiological barriers to delivery of monoclonal antibodies and other macromolecules in tumors. Cancer Res (1990) 50(3 Suppl):814s–9s.
11. Sanz L, Cuesta AM, Compte M, Alvarez-Vallina L. Antibody engineering: facing new challenges in cancer therapy. Acta Pharmacol Sin (2005) 26(6):641–8. doi:10.1111/j.1745-7254.2005.00135.x
12. Ecker DM, Jones SD, Levine HL. The therapeutic monoclonal antibody market. MAbs (2015) 7(1):9–14. doi:10.4161/19420862.2015.989042
13. Elgundi Z, Reslan M, Cruz E, Sifniotis V, Kayser V. The state-of-play and future of antibody therapeutics. Adv Drug Deliv Rev (2016). doi:10.1016/j.addr.2016.11.004
14. Bilello JA. The agony and ecstasy of “OMIC” technologies in drug development. Curr Mol Med (2005) 5(1):39–52. doi:10.2174/1566524053152898
15. Walsh G. Biopharmaceutical benchmarks 2014. Nat Biotechnol (2014) 32(10):992–1000. doi:10.1038/nbt.3040
16. Hamers-Casterman C, Atarhouch T, Muyldermans S, Robinson G, Hamers C, Songa EB, et al. Naturally occurring antibodies devoid of light chains. Nature (1993) 363(6428):446–8. doi:10.1038/363446a0
17. Smith GP. Filamentous fusion phage: novel expression vectors that display cloned antigens on the virion surface. Science (1985) 228(4705):1315–7. doi:10.1126/science.4001944
18. Muyldermans S. Nanobodies: natural single-domain antibodies. Annu Rev Biochem (2013) 82:775–97. doi:10.1146/annurev-biochem-063011-092449
19. Blanc MR, Anouassi A, Ahmed Abed M, Tsikis G, Canepa S, Labas V, et al. A one-step exclusion-binding procedure for the purification of functional heavy-chain and mammalian-type gamma-globulins from camelid sera. Biotechnol Appl Biochem (2009) 54(4):207–12. doi:10.1042/BA20090208
20. Muyldermans S, Cambillau C, Wyns L. Recognition of antigens by single-domain antibody fragments: the superfluous luxury of paired domains. Trends Biochem Sci (2001) 26(4):230–5. doi:10.1016/S0968-0004(01)01790-X
21. Conrath KE, Wernery U, Muyldermans S, Nguyen VK. Emergence and evolution of functional heavy-chain antibodies in Camelidae. Dev Comp Immunol (2003) 27(2):87–103. doi:10.1016/S0145-305X(02)00071-X
22. Kolkman JA, Law DA. Nanobodies – from llamas to therapeutic proteins. Drug Discov Today Technol (2010) 7(2):e95–146. doi:10.1016/j.ddtec.2010.03.002
23. Nguyen VK, Muyldermans S, Hamers R. The specific variable domain of camel heavy-chain antibodies is encoded in the germline. J Mol Biol (1998) 275(3):413–8. doi:10.1006/jmbi.1997.1477
24. Desmyter A, Transue TR, Ghahroudi MA, Thi MH, Poortmans F, Hamers R, et al. Crystal structure of a camel single-domain VH antibody fragment in complex with lysozyme. Nat Struct Biol (1996) 3(9):803–11. doi:10.1038/nsb0996-803
25. Vu KB, Ghahroudi MA, Wyns L, Muyldermans S. Comparison of llama VH sequences from conventional and heavy chain antibodies. Mol Immunol (1997) 34(16–17):1121–31. doi:10.1016/S0161-5890(97)00146-6
26. Govaert J, Pellis M, Deschacht N, Vincke C, Conrath K, Muyldermans S, et al. Dual beneficial effect of interloop disulfide bond for single domain antibody fragments. J Biol Chem (2012) 287(3):1970–9. doi:10.1074/jbc.M111.242818
27. Schroeder HW Jr, Cavacini L. Structure and function of immunoglobulins. J Allergy Clin Immunol (2010) 125(2 Suppl 2):S41–52. doi:10.1016/j.jaci.2009.09.046
28. Wesolowski J, Alzogaray V, Reyelt J, Unger M, Juarez K, Urrutia M, et al. Single domain antibodies: promising experimental and therapeutic tools in infection and immunity. Med Microbiol Immunol (2009) 198(3):157–74. doi:10.1007/s00430-009-0116-7
29. Muyldermans S, Baral TN, Retamozzo VC, De Baetselier P, De Genst E, Kinne J, et al. Camelid immunoglobulins and nanobody technology. Vet Immunol Immunopathol (2009) 128(1–3):178–83. doi:10.1016/j.vetimm.2008.10.299
30. Arbabi Ghahroudi M, Desmyter A, Wyns L, Hamers R, Muyldermans S. Selection and identification of single domain antibody fragments from camel heavy-chain antibodies. FEBS Lett (1997) 414(3):521–6. doi:10.1016/S0014-5793(97)01062-4
31. Dumoulin M, Conrath K, Van Meirhaeghe A, Meersman F, Heremans K, Frenken LG, et al. Single-domain antibody fragments with high conformational stability. Protein Sci (2002) 11(3):500–15. doi:10.1110/ps.34602
32. Cortez-Retamozo V, Lauwereys M, Hassanzadeh GG, Gobert M, Conrath K, Muyldermans S, et al. Efficient tumor targeting by single-domain antibody fragments of camels. Int J Cancer (2002) 98(3):456–62. doi:10.1002/ijc.10212
33. Conrad U, Plagmann I, Malchow S, Sack M, Floss DM, Kruglov AA, et al. ELPylated anti-human TNF therapeutic single-domain antibodies for prevention of lethal septic shock. Plant Biotechnol J (2011) 9(1):22–31. doi:10.1111/j.1467-7652.2010.00523.x
34. Coppieters K, Dreier T, Silence K, de Haard H, Lauwereys M, Casteels P, et al. Formatted anti-tumor necrosis factor alpha VHH proteins derived from camelids show superior potency and targeting to inflamed joints in a murine model of collagen-induced arthritis. Arthritis Rheum (2006) 54(6):1856–66. doi:10.1002/art.21827
35. Hultberg A, Temperton NJ, Rosseels V, Koenders M, Gonzalez-Pajuelo M, Schepens B, et al. Llama-derived single domain antibodies to build multivalent, superpotent and broadened neutralizing anti-viral molecules. PLoS One (2011) 6(4):e17665. doi:10.1371/journal.pone.0017665
36. Strokappe N, Szynol A, Aasa-Chapman M, Gorlani A, Forsman Quigley A, Hulsik DL, et al. Llama antibody fragments recognizing various epitopes of the CD4bs neutralize a broad range of HIV-1 subtypes A, B and C. PLoS One (2012) 7(3):e33298. doi:10.1371/journal.pone.0033298
37. D’Huyvetter M, Xavier C, Caveliers V, Lahoutte T, Muyldermans S, Devoogdt N. Radiolabeled nanobodies as theranostic tools in targeted radionuclide therapy of cancer. Expert Opin Drug Deliv (2014) 11(12):1939–54. doi:10.1517/17425247.2014.941803
38. Pereira SS, Moreira-Dill LS, Morais MS, Prado ND, Barros ML, Koishi AC, et al. Novel camelid antibody fragments targeting recombinant nucleoprotein of Araucaria hantavirus: a prototype for an early diagnosis of hantavirus pulmonary syndrome. PLoS One (2014) 9(9):e108067. doi:10.1371/journal.pone.0108067
39. Serruys B, Van Houtte F, Farhoudi-Moghadam A, Leroux-Roels G, Vanlandschoot P. Production, characterization and in vitro testing of HBcAg-specific VHH intrabodies. J Gen Virol (2010) 91:643–52. doi:10.1099/vir.0.016063-0
40. Hmila I, Saerens D, Ben Abderrazek R, Vincke C, Abidi N, Benlasfar Z, et al. A bispecific nanobody to provide full protection against lethal scorpion envenoming. FASEB J (2010) 24(9):3479–89. doi:10.1096/fj.09-148213
41. Richard G, Meyers AJ, McLean MD, Arbabi-Ghahroudi M, MacKenzie R, Hall JC. In vivo neutralization of alpha-cobratoxin with high-affinity llama single-domain antibodies (VHHs) and a VHH-Fc antibody. PLoS One (2013) 8(7):e69495. doi:10.1371/journal.pone.0069495
42. Hmila I, Abdallah RB, Saerens D, Benlasfar Z, Conrath K, Ayeb ME, et al. VHH, bivalent domains and chimeric heavy chain-only antibodies with high neutralizing efficacy for scorpion toxin AahI’. Mol Immunol (2008) 45(14):3847–56. doi:10.1016/j.molimm.2008.04.011
43. Prado ND, Pereira SS, da Silva MP, Morais MS, Kayano AM, Moreira-Dill LS, et al. Inhibition of the myotoxicity induced by Bothrops jararacussu venom and isolated phospholipases A2 by specific camelid single-domain antibody fragments. PLoS One (2016) 11(3):e0151363. doi:10.1371/journal.pone.0151363
44. Reichert JM. Antibodies to watch in 2017. MAbs (2017) 9(2):167–81. doi:10.1080/19420862.2016.1269580
45. Morel CM. Neglected diseases: under-funded research and inadequate health interventions. Can we change this reality? EMBO Rep (2003) 4:S35–8. doi:10.1038/sj.embor.embor851
46. Morel CM, Acharya T, Broun D, Dangi A, Elias C, Ganguly NK, et al. Health innovation networks to help developing countries address neglected diseases. Science (2005) 309(5733):401–4. doi:10.1126/science.1115538
47. Watts G. Drug development partnerships look set to grow. Lancet (2016) 388(10039):16–8. doi:10.1016/S0140-6736(16)30937-0
48. WHO. Neglected Tropical Diseases. (2017). Available from: http://www.who.int/neglected_diseases/diseases/en/
49. WHO. Neglected Tropical Diseases, Hidden Successes, Emerging Opportunities. (2009). Available from: http://apps.who.int/iris/bitstream/10665/44214/1/9789241598705_eng.pdf
50. Johnston EA, Teague J, Graham JP. Challenges and opportunities associated with neglected tropical disease and water, sanitation and hygiene intersectoral integration programs. BMC Public Health (2015) 15:547. doi:10.1186/s12889-015-1838-7
51. Hotez PJ, Pecoul B. “Manifesto” for advancing the control and elimination of neglected tropical diseases. PLoS Negl Trop Dis (2010) 4(5):e718. doi:10.1371/journal.pntd.0000718
52. Aavula SM, Nimmagadda SV, Biradhar N, Sula S, Chandran D, Lingala R, et al. Generation and characterization of an scFv directed against site II of rabies glycoprotein. Biotechnol Res Int (2011) 2011:652147. doi:10.4061/2011/652147
53. Hosking CG, McWilliam HE, Driguez P, Piedrafita D, Li Y, McManus DP, et al. Generation of a novel bacteriophage library displaying scFv antibody fragments from the natural buffalo host to identify antigens from adult Schistosoma japonicum for diagnostic development. PLoS Negl Trop Dis (2015) 9(12):e0004280. doi:10.1371/journal.pntd.0004280
54. Li PC, Liao MY, Cheng PC, Liang JJ, Liu IJ, Chiu CY, et al. Development of a humanized antibody with high therapeutic potential against dengue virus type 2. PLoS Negl Trop Dis (2012) 6(5):e1636. doi:10.1371/journal.pntd.0001636
55. Ratier L, Urrutia M, Paris G, Zarebski L, Frasch AC, Goldbaum FA. Relevance of the diversity among members of the Trypanosoma cruzi trans-sialidase family analyzed with camelids single-domain antibodies. PLoS One (2008) 3(10):e3524. doi:10.1371/journal.pone.0003524
56. Fatima A, Wang H, Kang K, Xia L, Wang Y, Ye W, et al. Development of VHH antibodies against dengue virus type 2 NS1 and comparison with monoclonal antibodies for use in immunological diagnosis. PLoS One (2014) 9(4):e95263. doi:10.1371/journal.pone.0095263
57. Stijlemans B, Conrath K, Cortez-Retamozo V, Van Xong H, Wyns L, Senter P, et al. Efficient targeting of conserved cryptic epitopes of infectious agents by single domain antibodies. African trypanosomes as paradigm. J Biol Chem (2004) 279(2):1256–61. doi:10.1074/jbc.M307341200
58. Caljon G, Caveliers V, Lahoutte T, Stijlemans B, Ghassabeh GH, Van Den Abbeele J, et al. Using microdialysis to analyse the passage of monovalent nanobodies through the blood-brain barrier. Br J Pharmacol (2012) 165(7):2341–53. doi:10.1111/j.1476-5381.2011.01723.x
59. Baral TN, Magez S, Stijlemans B, Conrath K, Vanhollebeke B, Pays E, et al. Experimental therapy of African trypanosomiasis with a nanobody-conjugated human trypanolytic factor. Nat Med (2006) 12(5):580–4. doi:10.1038/nm1395
60. De Vooght L, Caljon G, Stijlemans B, De Baetselier P, Coosemans M, Van Den Abbeele J. Expression and extracellular release of a functional anti-trypanosome nanobody (R) in Sodalis glossinidius, a bacterial symbiont of the tsetse fly. Microb Cell Fact (2012) 11:23. doi:10.1186/1475-2859-11-23
61. De Vooght L, Caljon G, De Ridder K, Van Den Abbeele J. Delivery of a functional anti-trypanosome nanobody in different tsetse fly tissues via a bacterial symbiont, Sodalis glossinidius. Microb Cell Fact (2014) 13:156. doi:10.1186/s12934-014-0156-6
62. Wu M, Park YJ, Pardon E, Turley S, Hayhurst A, Deng J, et al. Structures of a key interaction protein from the Trypanosoma brucei editosome in complex with single domain antibodies. J Struct Biol (2011) 174(1):124–36. doi:10.1016/j.jsb.2010.10.007
63. Obishakin E, Stijlemans B, Santi-Rocca J, Vandenberghe I, Devreese B, Muldermans S, et al. Generation of a nanobody targeting the paraflagellar rod protein of trypanosomes. PLoS One (2014) 9(12):e115893. doi:10.1371/journal.pone.0115893
64. Caljon G, Hussain S, Vermeiren L, Van Den Abbeele J. Description of a nanobody-based competitive immunoassay to detect tsetse fly exposure. PLoS Negl Trop Dis (2015) 9(2):e0003456. doi:10.1371/journal.pntd.0003456
65. Terryn S, Francart A, Rommelaere H, Stortelers C, Van Gucht S. Post-exposure treatment with anti-rabies VHH and vaccine significantly improves protection of mice from lethal rabies infection. PLoS Negl Trop Dis (2016) 10(8):e0004902. doi:10.1371/journal.pntd.0004902
66. Boruah BM, Liu DW, Ye D, Gu TJ, Jiang CL, Qu MS, et al. Single domain antibody multimers confer protection against rabies infection. PLoS One (2013) 8(8):e71383. doi:10.1371/journal.pone.0071383
67. Terryn S, Francart A, Lamoral S, Hultberg A, Rommelaere H, Wittelsberger A, et al. Protective effect of different anti-rabies virus VHH constructs against rabies disease in mice. PLoS One (2014) 9(10):e109367. doi:10.1371/journal.pone.0109367
68. Sallam MA. Anti-Schistoma Single-Domain Antibody-Nanoparticles Conjugate: A Novel Tool for Diagnostic and Therapeutic Applications. Cairo: The American University of Cairo (2012).
69. Deckers N, Saerens D, Kanobana K, Conrath K, Victor B, Wernery U, et al. Nanobodies, a promising tool for species-specific diagnosis of Taenia solium cysticercosis. Int J Parasitol (2009) 39(5):625–33. doi:10.1016/j.ijpara.2008.10.012
70. Coura JR. Chagas disease: what is known and what is needed – a background article. Mem Inst Oswaldo Cruz (2007) 102(Suppl 1):113–22. doi:10.1590/S0074-02762007000900018
71. Rassi A Jr, Rassi A, Marin-Neto JA. Chagas disease. Lancet (2010) 375(9723):1388–402. doi:10.1016/S0140-6736(10)60061-X
72. Gascon J, Albajar P, Canas E, Flores M, Gomez i Prat J, Herrera RN, et al. Diagnosis, management and treatment of chronic Chagas’ heart disease in areas where Trypanosoma cruzi infection is not endemic. Rev Esp Cardiol (2007) 60(3):285–93. doi:10.1016/S1885-5857(07)60153-4
73. Barrett MP, Burchmore RJ, Stich A, Lazzari JO, Frasch AC, Cazzulo JJ, et al. The trypanosomiases. Lancet (2003) 362(9394):1469–80. doi:10.1016/S0140-6736(03)14694-6
74. Buscaglia CA, Campo VA, Frasch AC, Di Noia JM. Trypanosoma cruzi surface mucins: host-dependent coat diversity. Nat Rev Microbiol (2006) 4(3):229–36. doi:10.1038/nrmicro1351
75. Agusti R, Paris G, Ratier L, Frasch AC, de Lederkremer RM. Lactose derivatives are inhibitors of Trypanosoma cruzi trans-sialidase activity toward conventional substrates in vitro and in vivo. Glycobiology (2004) 14(7):659–70. doi:10.1093/glycob/cwh079
76. Mucci J, Risso MG, Leguizamon MS, Frasch AC, Campetella O. The trans-sialidase from Trypanosoma cruzi triggers apoptosis by target cell sialylation. Cell Microbiol (2006) 8(7):1086–95. doi:10.1111/j.1462-5822.2006.00689.x
77. Todeschini AR, Mendonca-Previato L, Previato JO, Varki A, van Halbeek H. Trans-sialidase from Trypanosoma cruzi catalyzes sialoside hydrolysis with retention of configuration. Glycobiology (2000) 10(2):213–21. doi:10.1093/glycob/10.2.213
78. Leguizamon MS, Campetella OE, Gonzalez Cappa SM, Frasch AC. Mice infected with Trypanosoma cruzi produce antibodies against the enzymatic domain of trans-sialidase that inhibit its activity. Infect Immun (1994) 62(8):3441–6.
79. Pereira-Chioccola VL, Schenkman S, Kloetzel JK. Sera from chronic chagasic patients and rodents infected with Trypanosoma cruzi inhibit trans-sialidase by recognizing its amino-terminal and catalytic domain. Infect Immun (1994) 62(7):2973–8.
80. Leguizamon MS, Campetella O, Russomando G, Almiron M, Guillen I, Ganzalez Cappa SM, et al. Antibodies inhibiting Trypanosoma cruzi trans-sialidase activity in sera from human infections. J Infect Dis (1994) 170(6):1570–4. doi:10.1093/infdis/170.6.1570
81. Tribulatti MV, Mucci J, Van Rooijen N, Leguizamon MS, Campetella O. The trans-sialidase from Trypanosoma cruzi induces thrombocytopenia during acute Chagas’ disease by reducing the platelet sialic acid contents. Infect Immun (2005) 73(1):201–7. doi:10.1128/IAI.73.1.201-207.2005
82. Frasch AC. Functional diversity in the trans-sialidase and mucin families in Trypanosoma cruzi. Parasitol Today (2000) 16(7):282–6. doi:10.1016/S0169-4758(00)01698-7
83. Diamond MS, Pierson TC. Molecular insight into dengue virus pathogenesis and its implications for disease control. Cell (2015) 162(3):488–92. doi:10.1016/j.cell.2015.07.005
84. Bhatt S, Gething PW, Brady OJ, Messina JP, Farlow AW, Moyes CL, et al. The global distribution and burden of dengue. Nature (2013) 496(7446):504–7. doi:10.1038/nature12060
85. Chouin-Carneiro T, Vega-Rua A, Vazeille M, Yebakima A, Girod R, Goindin D, et al. Differential susceptibilities of Aedes aegypti and Aedes albopictus from the Americas to Zika virus. PLoS Negl Trop Dis (2016) 10(3):e0004543. doi:10.1371/journal.pntd.0004543
86. Gautam R, Mishra S, Milhotra A, Nagpal R, Mohan M, Singhal A, et al. Challenges with mosquito-borne viral diseases: outbreak of the monsters. Curr Top Med Chem (2017) 17. doi:10.2174/1568026617666170130122921
87. Baral TN. Immunobiology of African trypanosomes: need of alternative interventions. J Biomed Biotechnol (2010) 2010:389153. doi:10.1155/2010/389153
88. Franco JR, Simarro PP, Diarra A, Jannin JG. Epidemiology of human African trypanosomiasis. Clin Epidemiol (2014) 6:257–75. doi:10.2147/CLEP.S39728
89. Kennedy PG. Human African trypanosomiasis of the CNS: current issues and challenges. J Clin Invest (2004) 113(4):496–504. doi:10.1172/JCI21052
Keywords: VHH, single-domain antibody, biotechnology, neglected tropical diseases, camelid antibody
Citation: Fernandes CFC, Pereira SS, Luiz MB, Zuliani JP, Furtado GP and Stabeli RG (2017) Camelid Single-Domain Antibodies As an Alternative to Overcome Challenges Related to the Prevention, Detection, and Control of Neglected Tropical Diseases. Front. Immunol. 8:653. doi: 10.3389/fimmu.2017.00653
Received: 05 April 2017; Accepted: 18 May 2017;
Published: 09 June 2017
Edited by:
Florian Krammer, Icahn School of Medicine at Mount Sinai, United StatesReviewed by:
Johannes S. Gach, University of California, Irvine, United StatesCarole Henry, University of Chicago, United States
Joseph Ashour, Icahn School of Medicine at Mount Sinai, United States
Copyright: © 2017 Fernandes, Pereira, Luiz, Zuliani, Furtado and Stabeli. This is an open-access article distributed under the terms of the Creative Commons Attribution License (CC BY). The use, distribution or reproduction in other forums is permitted, provided the original author(s) or licensor are credited and that the original publication in this journal is cited, in accordance with accepted academic practice. No use, distribution or reproduction is permitted which does not comply with these terms.
*Correspondence: Carla F. C. Fernandes, Y2FybGEuY2VsZWRvbmlvQGZpb2NydXouYnI=;
Rodrigo G. Stabeli, cm9kcmlnby5zdGFiZWxpQGZpb2NydXouYnI=