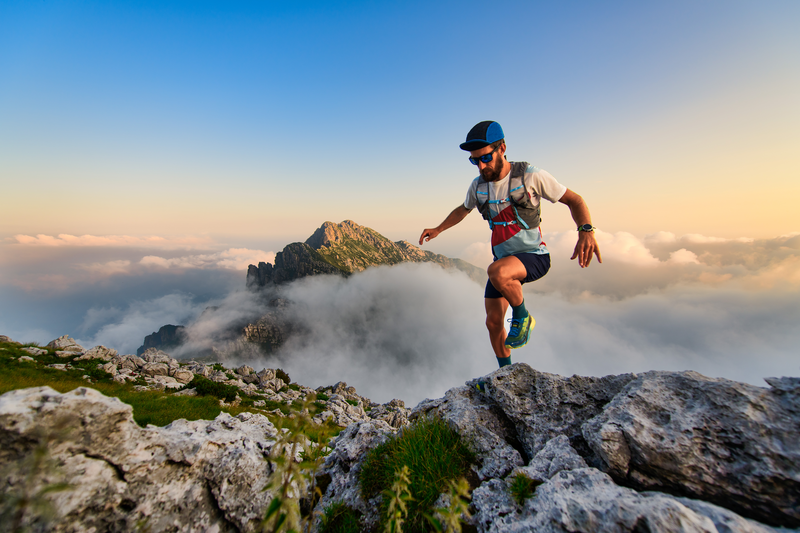
94% of researchers rate our articles as excellent or good
Learn more about the work of our research integrity team to safeguard the quality of each article we publish.
Find out more
MINI REVIEW article
Front. Immunol. , 16 May 2017
Sec. Inflammation
Volume 8 - 2017 | https://doi.org/10.3389/fimmu.2017.00552
This article is part of the Research Topic New Insights in Anaphylaxis View all 11 articles
Prevalence of food-induced anaphylaxis increases progressively and occurs in an unpredictable manner, seriously affecting the quality of life of patients. Intrinsic factors including age, physiological, and genetic features of the patient as well as extrinsic factors such as the intake of drugs and exposure to environmental agents modulate this disorder. It has been proven that diseases, such as mastocytosis, defects in HLA, or filaggrin genes, increase the risk of severe allergic episodes. Certain allergen families such as storage proteins, lipid transfer proteins, or parvalbumins have also been linked to anaphylaxis. Environmental factors such as inhaled allergens or sensitization through the skin can exacerbate or trigger acute anaphylaxis. Moreover, the effect of dietary habits such as the early introduction of certain foods in the diet, and the advantage of the breastfeeding remain as yet unresolved. Interaction of allergens with the intestinal cell barrier together with a set of effector cells represents the primary pathways of food-induced anaphylaxis. After an antigen cross-links the IgEs on the membrane of effector cells, a complex intracellular signaling cascade is initiated, which leads cells to release preformed mediators stored in their granules that are responsible for the acute symptoms of anaphylaxis. Afterward, they can also rapidly synthesize lipid compounds such as prostaglandins or leukotrienes. Cytokines or chemokines are also released, leading to the recruitment and activation of immune cells in the inflammatory microenvironment. Multiple factors that affect food-induced anaphylaxis are discussed in this review, paying special attention to dietary habits and environmental and genetic conditions.
The widely used definition of anaphylaxis as “an adverse allergic reaction that is rapid in onset and may cause death” is also accompanied by clinical criteria for diagnosis (1). Other definitions of anaphylaxis have been formulated to aid its diagnosis and management (2). It constitutes an alarming medical emergency (1, 3, 4), not only for the patient and members of the family, but sometimes also for the healthcare professionals involved. Death usually occurs because of respiratory or cardiac arrest as an aftershock of an anaphylactic attack (5). Although life-threatening episodes are uncommon, these events constitute an unpredictable risk and their prevalence is steadily increasing affecting up to 2% of the population (6). Hospital and critical care unit admissions are not common but continue to increase, doubling in frequency between 1998 and 2012 (7, 8). An accurate population-based estimate is difficult to obtain due to underdiagnosis and underreporting, as well as by the use of different clinical definitions for anaphylaxis and methods of case diagnosis in populations under study (9).
Molecules such as histamine (HIS), tryptase, leukotrienes, and prostaglandins, among others, mediate the clinical manifestations of anaphylaxis. Secretion of these mediators occurs after an allergen cross-links the IgEs bound to mast cells (MCs) and basophils. However, IgE-independent immune mechanisms may also be involved (3). Physiological state (3), as well as certain diseases and medications (10), are risk factors for anaphylaxis. Cofactors such as drugs or exercise that can exacerbate or trigger acute anaphylactic episodes have been described (11–13). Specialist physicians and patients need to be aware of the relevant risk factors and cofactors in the context of long-term management and treatment of this condition. In this mini review, we summarize the physiological, genetic, and environmental aspects in the field of food allergy (FA) focusing special attention on anaphylactic reactions.
Food allergy is a serious and often life-threatening health concern that is increasing in frequency especially in the vulnerable pediatric population affecting 4% of children and 2–3% of the adult population worldwide (14). The treatment requires changes in dietary habits and social behavior (15). FA is originated by a reaction of the immune system that results in non-tolerance of specific foods. In most patients, IgE mediates this immune disorder, although there are also IgE-independent cell-mediated allergies that are accompanied by gastrointestinal symptoms (16), but they are not going to be analyzed in this review.
More than 170 foods have been associated with type I allergies, the most common of which are milk, egg, wheat, fish, shellfish, peanuts, soy, and tree nuts, although the prevalence varies geographically (17). The two most frequent food allergens that induce severe and potentially lethal anaphylaxis are milk and egg, while the third differs between countries (18) being peanuts in the USA and Switzerland, wheat in Germany and Japan, tree nuts in Spain, and sesame in Israel (16). Foods and meals containing hidden allergens at restaurants are a serious source of risk for patients with food allergies (19). The prevalence of food-induced anaphylaxis seems to vary with the population selected and the region where they are recruited. The point prevalence of food challenge-confirmed allergy is under 1% (20–23).
Food allergies can be caused by primary sensitization to an eliciting food allergen (class I allergen), or they can be triggering by a primary sensitization to an inhalant allergen and later IgE cross-reactivity to a homologous protein in food (class II allergen) (24). Almost all plant food allergens are either storage or defense-related proteins and three dominating plant allergenic protein superfamilies have been identified as being involved in triggering severe reactions. These are prolamins that include the 2S albumins, lipid transfer proteins (LTPs), and α-amylase/trypsin inhibitors, cupins that comprise the major globulin storage proteins, mainly found in legumes and nuts and the Bet v 1-related protein family (25, 26). Among animal food allergen families, parvalbumins, tropomyosins, and caseins are the three dominant groups (25, 26). The most significant molecular characteristic of these allergens is their resistance to proteolysis, which increases the probability of reaching intact the intestinal mucosa and triggering an immune response (27).
The prevalence of FA is growing at an alarming rate (28), and it is not easy to know with precision the reasons behind this growth. Factors such as a sterile pathogen-free environment, changing dietary habits, vitamin D deficiency, intestinal microbiota composition, stability properties of certain allergens, or alcohol consumption may play a role in this increase (29) (Figure 1).
Figure 1. Diagram showing genetic, environmental, dietary habits, and allergen-related risk factors for food allergy.
Breast milk provides an abundant source of soluble IgA and prebiotic glycans that promote the expansion of species of Lachnospira, Veillonella, and Rothia, which are adapted to this food source (30, 31). In contrast, formula feeding may not be sufficient for neonates with poor immunity, exacerbating the allergic reactions and only amino acid-based formulas should be considered as non-allergenic. Pre- or probiotic supplemented infant formulas are also used but they might not fully replicate the beneficial effect of breast milk.
The correct time to incorporate certain allergenic foods in the diet of infants has also been controversial topic. It has been demonstrated that the early introduction of peanut to high-risk 7-month-old babies reduces the incidence of peanut allergy (32). Moreover, the LEAP study showed that in high-risk infants, sustained consumption of peanut beginning in the first 11 months of life was highly effective in preventing the development of peanut allergy (33).
Nowadays, food processing is an important aspect in our daily diet. The stability of the allergenic proteins during these procedures (34) and the resistance to gastric and intestinal digestion (35) are properties that preserve the integrity of IgE-specific epitopes, as occurs in LTPs allergic patients (36). These processes can alter structural characteristics of allergens and therefore modify their allergenic capacity (37–40). Patients, who possess specific IgE to linear epitopes, have a stronger response to cooked and partially digested antigens, while those patients who recognize conformational or three-dimensional epitopes have a milder clinical response (41, 42).
It has also been proved that some nutrients, such as vitamin D, influence the regulation of the immune system (43–45). In fact, the frequency of food anaphylaxis increases in areas where the exposure to UVB radiation is low and vitamin D is not synthesized in adequate concentrations (46). Moreover, vitamin D deficiency is associated with challenge-proven FA in infants (47). The deficit of this vitamin promotes Th2 responses by reducing the number of Th1 cells and inducing Th2 cell proliferation. However, in correct doses, vitamin D is responsible for reducing the allergic response by promoting Treg and suppressing Th17 cells, demonstrating the importance of a correct balance (48).
A few specific gene mutations have been related to food anaphylaxis. Several studies have identified an association of HLA genes with peanut allergy (49–51). Moreover, filaggrin is a key protein in the function of the epidermic epithelial barrier, and Cabanillas and Novak (52) have shown that the development of peanut allergy in children that carry one or more mutations in the filaggrin genes is provoked even when they are exposed to very small quantities of allergen, a risk that increased with higher dose exposure.
However, exclusively genetic susceptibility cannot explain the rapidly increasing prevalence of food allergies, suggesting that something in our environment is promoting this disease. There is increasing evidence that an early sensitization is occurring in individuals through the skin or even through breast milk and the amniotic fluid. Atopic dermatitis, multiplies by a factor of 10 the risk of suffering peanut allergy (53). The exposure to peanut particles suspended in the environment (54), the absorption of active allergenic components present in topical lotions (55), the damage induced by scratching, or the presence of Staphylococcal enterotoxin B in the areas surrounding a skin lesion have all been associated to peanut allergy (56). Moreover, workers exposed to occupational food allergens can be sensitized through the skin or by inhalation (57, 58).
Allergic reactions are increasingly associated with demographic variables, which are becoming every day more widespread in the twenty-first century such as cesarean births. Delivery by cesarean section may predispose the newborn baby to FA, presumably due to modifications in the establishment of gut microbiota caused by a different initial exposure to microbes (59, 60). Continuous intake of antibiotics has a profound influence on the bacterial composition that colonize the intestinal track, which may affect the allergic response to food and which seems to be essential for the maintenance of homeostasis (61, 62). In a healthy gastrointestinal tract, the epithelium develops immune tolerance to antigens present in the diet, fights invading microorganisms, and limits their presence near the mucosa (63, 64). Alterations in gut microbiota (dysbiosis) and consequent disruption of homeostasis have been linked to the occurrence of allergic reactions (65). However, whether an incremented permeability in the intestine is a cause or a consequence of an allergic reaction is still an open question (66). The finding that Clostridia strains can suppress allergy in mice (67, 68) suggests the potential use of microbial therapies to enhance the development of tolerance when given with allergen immunotherapy (69, 70).
A broad variety of other factors may also contribute to the increased risk of food-induced anaphylaxis. A prospective study reported an association between anaphylaxis and exercise, drug use, acute infection, premenstrual status, or psychological stress in 20% of patients (13). Food-dependent exercise-induced anaphylaxis has also been observed, although it is found more often in adults than in children (71–73). Studies have also shown that epigenetic variants, primarily in the pattern of DNA methylation, are associated with FA (74). Increased severity of anaphylaxis has also been reported for the elderly, patients with pre-existing cardiovascular disease, MC disorders, or undergoing concomitant treatment with a beta-adrenergic blocker and/or angiotensin converting enzyme inhibitor (75). Non-steroidal anti-inflammatory drugs also seem to enhance some food-allergic reactions (76).
The gastrointestinal tract has a large surface area that is responsible for the digestion and absorption of food. It is formed by the intestinal epithelium, a cell monolayer that creates a selective barrier separating both outside and inside environments (77) (Figure 2). This epithelium is the first line of defense forming a physical and biochemical barrier where the mutualistic relationship between commensal microbial communities and immune cells are in a state of homeostasis (78), thereby preventing the colonization by other pathogenic microorganisms (79). This delicate balance depends on the functions of intestinal epithelial cells (IECs), maintaining a state of non-responsiveness (tolerance) or releasing an antipathogenic immunity.
Figure 2. Key features in the immunological mechanisms of intestinal mucosa involved in food allergy and food-induced anaphylaxis. CBP, carboxipeptidase; CMA, chymase; Cys-LT, cysteinyl leukotriene; HIS, histamine; IECs, intestinal epithelial cells; PAF, platelet-activating factor; PG, prostaglandins; TRY, tryptase.
Enterocytes form the dominant cell population in the epithelial barrier, constituting more than 80% of all IECs with functions that are metabolic and digestive, as well as secretory. Globet and paneth cells, also present in the barrier, release numerous hormone regulators such as mucins and antimicrobial proteins and protect the host from infection (80, 81). The most abundant mucins, MUC2, control macrophage and adaptive T cell responses during inflammation and inhibit pathogen microorganism chemotaxis (82, 83). Microfold cells (M cells) that form concentrations in the epithelium above Peyer’s patches mediate the presentation of antigens and microorganisms to the mucosal immune system, appearing as efficient mechanisms of receptor-mediated transport (84). In addition, among the cells of the immune system, dendritic cells and macrophages play an important role as antigen presenting cells. They are able to capture, process, and present the antigen to T lymphocytes, and induce a specific immune response. On the other hand, MCs located in the lamina propria and cell barrier detect antigens through the specific IgEs attached to their membrane and induce inflammatory reactions by secreting cytokines and immune mediators.
Mast cells and basophils represent the primary effector cells in the pathophysiological process of food-induced anaphylaxis (85, 86), although it has been speculated that they may also participate in late-phase and chronic allergic reactions (87). They express complementary and overlapping roles in both regulatory and effector activities (88) and due to the similarities in histochemical characteristics, IgE receptor expression and the mediators produced, MCs and basophils were supposed to be related. However, transcriptional analysis has shown minimal similarity between MCs and basophils (89), and in recent years, several studies have indicated that although derived from unique hematopoietic progenitors, they are not closely related (90, 91). Both cell types also differ in their development (92). Basophils complete their differentiation within the bone marrow, while MCs circulate in the blood as progenitor cells and enter the tissues to proliferate and maturate (93). Tissue microenvironment regulates the mast cell expression of proteases (94, 95). Accordingly, humans MCs are classified based on their protease composition as MCs containing both tryptase and chymase, typically present in the bowel submucosa within the connective tissue, and those containing only tryptase, which are predominant on mucosal and epithelial surfaces such as bowel mucosa (96). Chymase-only-positive MCs have also been described but they appear to be very infrequent (97).
To understand the mechanisms of effector cell activation, it is essential to comprehend the regulation of intracellular signaling pathways that lead cells to release their mediators during the acute phase of anaphylaxis. In the last years, these events have been extensively studied and reviewed in the literature (98–104). However, given the complexity of these processes, here we present only a brief overview of the current state of affairs, focusing primarily on FcεRI. Signaling initiated by FcεRI aggregation triggers phosphorylation of immunoglobulin receptor activation motifs, which is mediated by the Src family tyrosine kinase Lyn and Syk. Subsequently, two major signaling enzymes, phosphoinositide phospholipase C-gamma and phosphoinositide 3-kinase, are activated which causes the release of calcium from intracellular stores, leading to cell degranulation, arachidonic acid metabolism, and activation of transcription factors with the consequent production and release of lipid mediators and the production of cytokine and chemokine. Effector cells also possess receptors characterized by a conserved immunoreceptor tyrosine-based inhibitory motif that when phosphorylated, recruits protein (SHP-1) and lipid phosphatases (SHIP1, SHIP2) that inhibit protein interactions and therefore inhibit the signaling cascade. In the end, despite all these complex cellular signaling pathways, the status of effector cell activation is really a balance between the pathways that upregulate these processes and those that downregulate them.
Both MCs and basophils participate in clinical manifestations of anaphylaxis that typically involve the skin and the respiratory and gastrointestinal tracts (105). They release potent inflammatory mediators after an antigen cross-links the specific IgE attached to their surface receptors. Tissue-derived MCs and circulating basophils can immediately release preformed mediators stored in their granules such as HIS, heparin, or proteases that are responsible for many of the acute symptoms such as vascular leak or bronchoconstriction (106, 107). HIS, the main biogenic amine released upon activation of effector cells, has long been proven to be a short half-life factor triggering a variety of symptoms of anaphylaxis, including inflammation, itchiness, and mucus production (108). Recent studies have shown a correlation between plasma HIS levels and anaphylaxis severity (109). The efficiency of blocking HIS H1 and H4 receptors to suppress intestinal anaphylaxis in peanut allergy has also been proved, being this effect mediated through the limitation of mesenteric lymph node and intestinal dendritic cell accumulation and function (110). Tryptase is currently one of the biomarkers to assess MCs activation and levels are enhanced after onset of anaphylaxis (111). Basal tryptase levels in serum may predict moderate to severe anaphylaxis in children with FA (112) and they have also been described as good markers for the diagnosis of food-induced anaphylaxis (113). Chymase and carboxypeptidase levels in serum of patients with anaphylaxis have been found to be significantly greater than those found in healthy subjects, although there is no correlation with tryptase levels (114).
After activation, MCs and basophils can also rapidly synthesize lipid compounds in their membrane. These include prostaglandins, leukotrienes, and platelet-activating factor (PAF) that are mediators of hypotension, bronchospasm, mucus secretion, as well as leukocyte and dendritic cell recruitment during anaphylaxis (115). Increased urinary concentrations of leukotrienes and prostaglandins have been found in patients with food-induced anaphylaxis (caused by seafood, nuts, and soybean milk) compared to healthy individuals, showing good correlation between both mediators (116). PAF correlates better than either HIS or tryptase with severity of symptoms (117), and a deficiency of PAF-AH, the enzyme that inactivates PAF, predisposes patients to severe anaphylaxis (118). Moreover, it has been proven that detection of malfunction of PAF-AH may help identify individuals at risk of anaphylaxis (119).
Upon activation of MCs and basophils, cytokines or chemokines are newly synthesized and released, which are involved in the recruitment and activation of several cells in the inflammatory microenvironment (106). Many cytokines, chemokines, and growth factors are released from MCs (120) and basophils (121). IL-2, IL-4, IL-5, IL-6, IL-10, IL-13, and IFN-γ are elevated in anaphylactic patients (106), but only IL-2, IL-6, and IL-10 have been correlated with initial reaction severity (106, 109), although it is difficult to determine their origin because they are not exclusively produced by effector cells.
Despite efforts to prevent FA and anaphylaxis and to elucidate the fundamental underlying mechanisms that link allergen exposure to symptoms, the incidence is increasing in developed countries. Individual genetic predisposition is an important determinant, although the features of the allergen itself and a variety of environmental factors, including diet and intestinal microbiota may have an important impact on sensitization, severity, and persistence of FA. Preventive strategies, such as dietary interventions, the use of probiotics, or prolonged exclusive breast-feeding, among others, have recently come into question and therefore it is important to identify and study how to control the risk factors to reduce the development of FA. The complex network of factors involved in these disorders makes research in this field difficult and much more investigation needs to be done to identify and manipulate the modifiable risk factors to offer significant benefits to mitigate this disease in the future.
SB, MG-A, LM-P, AD-P, and MV contributed to writing and critically revised the paper. CB contributed to designing and drawing the figures.
The authors declare that the research was conducted in the absence of any commercial or financial relationships that could be construed as a potential conflict of interest.
This work was supported by the Ministry of Economy and Competitiveness (project number SAF2014-53209-R) and the thematic network and cooperative research centers ARADyAL RD16/0006/0014 and RD16/0006/0003. Grant project BIO2013-41403R from Ministerio de Ciencia e Innovación (Spain) and Thematic Networks and Cooperative Research Centers: RIRAAF (RD12/0013/0014). SB acknowledges the financial support from MINECO through the “Juan de la Cierva” program (FJCI-2014-21696). MG-A is supported by a fellowship from Ministerio de Ciencia e Innovación (Spain) and Thematic Networks and Cooperative Research Centers ARADyAL (RD16/0006/0003). LM-P is supported by a fellowship from Ministerio de Ciencia e Innovación (Spain) and Thematic Networks and Cooperative Research Centers RIRAAF (RD12/0013/0014). CB is supported by an FPU fellowship from the Spanish Ministry of Education, Culture, and Sport.
FA, food allergy; HIS, histamine; IECs, intestinal epithelial cells; LTP, lipid transfer protein; MCs, mast cells; PAF, platelet-activating factor.
1. Sampson HA, Munoz-Furlong A, Campbell RL, Adkinson NF, Bock SA, Branum A, et al. Second symposium on the definition and management of anaphylaxis: summary report-second. National institute of allergy and infectious disease/food allergy and anaphylaxis network symposium. J Allergy Clin Immunol (2006) 117:391–7. doi:10.1016/j.jaci.2005.12.1303
2. Panesar SS, Javad S, de Silva D, Nwaru BI, Hickstein L, Muraro A, et al. The epidemiology of anaphylaxis in Europe: a systematic review. Allergy (2013) 68:1353–61. doi:10.1111/all.12272
3. Simons FER, Ardusso LRF, Bilo MB, El-Gamal YM, Ledford DK, Ring J, et al. World Allergy Organization guidelines for the assessment and management of anaphylaxis. J Allergy Clin Immunol (2011) 127:587–93.e1–22. doi:10.1016/j.jaci.2011.01.038
4. Soar J, Pumphrey R, Cant A, Clarke S, Corbett A, Dawson P, et al. Emergency treatment of anaphylactic reactions-guidelines for healthcare providers. Resuscitation (2008) 77:157–69. doi:10.1016/j.resuscitation.2008.02.001
5. Pumphrey R. Anaphylaxis: can we tell who is at risk of a fatal reaction? Curr Opin Allergy Clin Immunol (2004) 4:285–90. doi:10.1097/01.all.0000136762.89313.0b
6. Lieberman P, Camargo CA, Bohlke K, Jick H, Miller RL, Sheikh A, et al. Epidemiology of anaphylaxis: findings of the American College of Allergy, Asthma and Immunology Epidemiology of Anaphylaxis Working Group. Ann Allergy Asthma Immunol (2006) 97:596–602. doi:10.1016/S1081-1206(10)61086-1
7. Turner PJ, Baumert JL, Beyer K, Boyle RJ, Chan CH, Clark AT, et al. Can we identify patients at risk of life-threatening allergic reactions to food? Allergy (2016) 71:1241–55. doi:10.1111/all.12924
8. Gibbison B, Sheikh A, McShane P, Haddow C, Soar J. Anaphylaxis admissions to UK critical care units between 2005 and 2009. Anaesthesia (2012) 67:833–8. doi:10.1111/j.1365-2044.2012.07159.x
9. Sheikh A, Hippisley-Cox J, Newton J, Fenty J. Trends in national incidence, lifetime prevalence and adrenaline prescribing for anaphylaxis in England. J R Soc Med (2008) 101:139–43. doi:10.1258/jrsm.2008.070306
10. Muñoz-Cano R, Picado C, Valero A, Bartra J. Mechanisms of anaphylaxis beyond IgE. J Investig Allergol Clin Immunol (2016) 26:73–82. doi:10.18176/jiaci.0046
11. Keet CA, Frischmeyer-Guerrerio PA, Thyagarajan A, Schroeder JT, Hamilton RG, Boden S, et al. The safety and efficacy of sublingual and oral immunotherapy for milk allergy. J Allergy Clin Immunol (2012) 129:448–55, 455.e1–5. doi:10.1016/j.jaci.2011.10.023
12. Simons FER, Ardusso LRF, Bilo MB, Dimov V, Ebisawa M, El-Gamal YM, et al. Update: World Allergy Organization guidelines for the assessment and management of anaphylaxis. Curr Opin Allergy Clin Immunol (2012) 12:389–99. doi:10.1097/ACI.0b013e328355b7e4
13. Hompes S, Kohli A, Nemat K, Scherer K, Lange L, Rueff F. Provoking allergens and treatment of anaphylaxis in children and adolescents data from the anaphylaxis registry of German-speaking countries. Pediatr Allergy Immunol (2011) 22:568–74. doi:10.1111/j.1399-3038.2011.01154.x
14. Branum AM, Lukacs SL. Food allergy among children in the United States. Pediatrics (2009) 124:1549–55. doi:10.1542/peds.2009-1210
15. Rona RJ, Keil T, Summers C, Gislason D, Zuidmeer L, Sodergren E, et al. The prevalence of food allergy: a meta-analysis. J Allergy Clin Immunol (2007) 120:638–46. doi:10.1016/j.jaci.2007.05.026
16. Fiocchi A, Brozek J, Schunemann H, Bahna SL, von Berg A, Beyer K, et al. World allergy organization (WAO) diagnosis and rationale for action against cow’s milk allergy (DRACMA) guidelines. Pediatr Allergy Immunol (2010) 21:1–125. doi:10.1111/j.1399-3038.2010.01068.x
17. Longo G, Berti I, Burks AW, Krauss B, Barbi E. IgE-mediated food allergy in children. Lancet (2013) 382:1656–64. doi:10.1016/S0140-6736(13)60309-8
18. Colver AF, Nevantaus H, Macdougall CF, Cant AJ. Severe food allergic reactions in children across the UK and Ireland, 1998–2000. Acta Paediatr (2005) 94:689–95. doi:10.1111/j.1651-2227.2005.tb01966.x
19. Añíbarro B, Seoane FJ, Múgica MV. Involvement of hidden allergens in food allergic reactions. J Investig Allergol Clin Immunol (2007) 17:168–72.
20. Rasmussen TA, Jorgensen MRS, Bjerrum S, Jensen-Fangel S, Stovring H, Ostergaard L, et al. Use of population based background rates of disease to assess vaccine safety in childhood and mass immunization in Denmark: nationwide population based cohort study. BMJ (2012) 345:e5823. doi:10.1136/bmj.e5823
21. Worm M, Edenharter G, Rueff F, Scherer K, Pfohler C, Mahler V, et al. Symptom profile and risk factors of anaphylaxis in central Europe. Allergy (2012) 67:691–8. doi:10.1111/j.1398-9995.2012.02795.x
22. Lynch RM, Robertson R. Anaphylactoid reactions to intravenous N-acetylcysteine: a prospective case controlled study. Accid Emerg Nurs (2004) 12:10–5. doi:10.1016/j.aaen.2003.07.001
23. Muraro A, Roberts G, Worm M, Bilo MB, Brockow K, Fernández Rivas M, et al. Anaphylaxis: guidelines from the European Academy of Allergy and Clinical Immunology. Allergy (2014) 69:1026–45. doi:10.1111/all.12437
24. Lorenz AR, Scheurer S, Vieths S. Food allergens: molecular and immunological aspects, allergen databases and cross-reactivity. Chem Immunol Allergy (2015) 101:18–29. doi:10.1159/000371647
25. Kumar S, Verma AK, Das M, Dwivedi PD. Allergenic diversity among plant and animal food proteins. Food Rev Int (2012) 28:277–98. doi:10.1080/87559129.2011.635391
26. Masilamani M, Commins S, Shreffler W. Determinants of food allergy. Immunol Allergy Clin North Am (2012) 32:11–33. doi:10.1016/j.iac.2011.12.003
27. Stanley JS, Bannon GA. Biochemistry of food allergens. Clin Rev Allergy Immunol (1999) 17:279–91. doi:10.1007/BF02737612
28. Prescott S, Allen KJ. Food allergy: riding the second wave of the allergy epidemic. Pediatr Allergy Immunol (2011) 22:155–60. doi:10.1111/j.1399-3038.2011.01145.x
29. Sicherer SH, Sampson HA. Food allergy: epidemiology, pathogenesis, diagnosis, and treatment. J Allergy Clin Immunol (2014) 133:291–307. doi:10.1016/j.jaci.2013.11.020
30. Barile D, Rastall RA. Human milk and related oligosaccharides as prebiotics. Curr Opin Biotechnol (2013) 24:214–9. doi:10.1016/j.copbio.2013.01.008
31. Coppa GV, Bruni S, Morelli L, Soldi S, Gabrielli O. The first prebiotics in humans: human milk oligosaccharides. J Clin Gastroenterol (2004) 38:S80–3. doi:10.1097/01.mcg.0000128926.14285.25
32. Ricci G, Caffarelli C. Early or not delayed complementary feeding? This is the question. J Allergy Clin Immunol (2016) 137:334–5. doi:10.1016/j.jaci.2015.09.038
33. Du Toit G, Roberts G, Sayre PH, Bahnson HT, Radulovic S, Santos AF, et al. Randomized trial of peanut consumption in infants at risk for peanut allergy. N Engl J Med (2015) 372:803–13. doi:10.1056/NEJMoa1414850
34. Jiménez-Saiz R, Benedé S, Molina E, López-Expósito I. Effect of processing technologies on the allergenicity of food products. Crit Rev Food Sci Nutr (2015) 55:1902–17. doi:10.1080/10408398.2012.736435
35. Benedé S, López-Expósito I, Molina E, López-Fandiño R. Egg proteins as allergens and the effects of the food matrix and processing. Food Funct (2015) 6:694–713. doi:10.1039/C4FO01104J
36. Van Winkle RR, Chang C. The biochemical basis and clinical evidence of food allergy due to lipid transfer proteins: a comprehensive review. Clin Rev Allergy Immunol (2014) 46:211–24. doi:10.1007/s12016-012-8338-7
37. Lepski S, Brockmeyer J. Impact of dietary factors and food processing on food allergy. Mol Nutr Food Res (2013) 57:145–52. doi:10.1002/mnfr.201200472
38. Maleki SJ, Hurlburt BK. Structural and functional alterations in major peanut allergens caused by thermal processing. J AOAC Int (2004) 87:1475–9.
39. Hilmenyuk T, Bellinghausen I, Heydenreich B, Ilchmann A, Toda M, Grabbe S, et al. Effects of glycation of the model food allergen ovalbumin on antigen uptake and presentation by human dendritic cells. Immunology (2010) 129:437–45. doi:10.1111/j.1365-2567.2009.03199.x
40. Ilchmann A, Burgdorf S, Scheurer S, Waibler Z, Nagai R, Wellner A, et al. Glycation of a food allergen by the Maillard reaction enhances its T-cell immunogenicity: role of macrophage scavenger receptor class A type I and II. J Allergy Clin Immunol (2010) 125:175–83. doi:10.1016/j.jaci.2009.08.013
41. Perrier C, Corthesy B. Gut permeability and food allergies. Clin Exp Allergy (2011) 41:20–8. doi:10.1111/j.1365-2222.2010.03639.x
42. Vila L, Beyer K, Järvinen KM, Chatchatee P, Bardina L, Sampson HA. Role of conformational and linear epitopes in the achievement of tolerance in cow’s milk allergy. Clin Exp Allergy (2001) 31:1599–606. doi:10.1046/j.1365-2222.2001.01218.x
43. Nwaru B I, Ahonen S, Kaila M, Erkkola M, Haapala AM, Kronberg-Kippilä C, et al. Maternal diet during pregnancy and allergic sensitization in the offspring by 5 years of age: a prospective cohort study. Pediatr Allergy Immunol (2010) 21:29–37. doi:10.1111/j.1399-3038.2009.00949.x
44. Muehleisen B, Gallo RL. Vitamin D in allergic disease: shedding light on a complex problem. J Allergy Clin Immunol (2013) 131:324–9. doi:10.1016/j.jaci.2012.12.1562
45. Vassallo MF, Camargo CA. Potential mechanisms for the hypothesized link between sunshine, vitamin D, and food allergy in children. J Allergy Clin Immunol (2010) 126:217–22. doi:10.1016/j.jaci.2010.06.011
46. Mullins RJ, Camargo CA. Latitude, sunlight, vitamin D, and childhood food allergy/anaphylaxis. Curr Allergy Asthma Rep (2012) 12:64–71. doi:10.1007/s11882-011-0230-7
47. Allen KJ, Koplin JJ, Ponsonby AL, Gurrin LC, Wake M, Vuillermin P, et al. Vitamin D insufficiency is associated with challenge-proven food allergy in infants. J Allergy Clin Immunol (2013) 131:1109–16. doi:10.1016/j.jaci.2013.01.017
48. Baek JH, Shin YH, Chung IH, Kim HJ, Yoo EG, Yoon JW, et al. The link between serum vitamin D level, sensitization to food allergens, and the severity of atopic dermatitis in infancy. J Pediatr (2014) 165:849–54. doi:10.1016/j.jpeds.2014.06.058
49. Hong X, Hao K, Ladd-Acosta C, Hansen KD, Tsai HJ, Liu X, et al. Genome-wide association study identifies peanut allergy-specific loci and evidence of epigenetic mediation in US children. Nat Commun (2015) 6:6304. doi:10.1038/ncomms7304
50. Madore AM, Vaillancourt VT, Asai Y, Alizadehfar R, Ben-Shoshan M, Michelet DL, et al. HLA-DQB1*02 and DQB1*06:03P are associated with peanut allergy. Eur J Hum Genet (2013) 21:1181–4. doi:10.1038/ejhg.2013.13
51. Howell WM, Turner SJ, Hourihane JO, Dean TP, Warner JO. HLA class IIDRB1, DQB1 and DPB1 genotypic associations with peanut allergy: evidence from a family-based and case–control study. Clin Exp Allergy (1998) 28:156–62. doi:10.1046/j.1365-2222.1998.00224.x
52. Cabanillas B, Novak N. Atopic dermatitis and filaggrin. Curr Opin Immunol (2016) 42:1–8. doi:10.1016/j.coi.2016.05.002
53. Brough HA, Liu AH, Sicherer S, Makinson K, Douiri A, Brown SJ, et al. Atopic dermatitis increases the effect of exposure to peanut antigen in dust on peanut sensitization and likely peanut allergy. J Allergy Clin Immunol (2015) 135:164–70. doi:10.1016/j.jaci.2014.10.007
54. Ramirez DA, Bahna SL. Food hypersensitivity by inhalation. Clin Mol Allergy (2009) 7:4. doi:10.1186/1476-7961-7-4
55. Fox AT. Peanut Allergy: Routes of Pre-natal and Post-natal Exposure. Cambridge: University of Cambridge (2012).
56. Boguniewicz M, Leung DY. Atopic dermatitis: a disease of altered skin barrier and immune dysregulation. Immunol Rev (2011) 242:233–46. doi:10.1111/j.1600-065X.2011.01027.x
57. Siracusa A, Folletti I, van Wijk RG, Jeebhay MF, Moscato G, Quirce S, et al. Occupational anaphylaxis – an EAACI task force consensus statement. Allergy (2015) 70:141–52. doi:10.1111/all.12541
58. Leonardi S, Pecoraro R, Filippelli M, Miraglia del Giudice M, Marseglia G, Salpietro C, et al. Allergic reactions to foods by inhalation in children. Allergy Asthma Proc (2014) 35:288–94. doi:10.2500/aap.2014.35.3755
59. Papathoma E, Triga M, Fouzas S, Dimitriou G. Cesarean section delivery and development of food allergy and atopic dermatitis in early childhood. Pediatr Allergy Immunol (2016) 27:419–24. doi:10.1111/pai.12552
60. Pantoja-Feliciano IG, Clemente JC, Costello EK, Perez ME, Blaser MJ, Knight R, et al. Biphasic assembly of the murine intestinal microbiota during early development. ISME J (2013) 7:1112–5. doi:10.1038/ismej.2013.15
61. Cho I, Blaser MJ. The human microbiome: at the interface of health and disease. Nat Rev Genet (2012) 13:260–70. doi:10.1038/nrg3182
62. Feehley T, Stefka AT, Cao S, Nagler CR. Microbial regulation of allergic responses to food. Semin Immunophatol (2012) 34:671–88. doi:10.1007/s00281-012-0337-5
63. Faria A, Weiner HL. Oral tolerance. Immunol Rev (2005) 206:232–59. doi:10.1111/j.0105-2896.2005.00280.x
64. Vickery BP, Scurlock AM, Jones SM, Burks AW. Mechanisms of immune tolerance relevant to food allergy. J Allergy Clin Immunol (2011) 127:576–84. doi:10.1016/j.jaci.2010.12.1116
65. Berin MC, Sampson HA. Mucosal immunology of food allergy. Curr Biol (2013) 23:R389–400. doi:10.1016/j.cub.2013.02.043
66. Ventura MT, Polimeno L, Amoruso AC, Gatti F, Annoscia E, Marinaro M, et al. Intestinal permeability in patients with adverse reactions to food. Dig Liver Dis (2006) 38:732–6. doi:10.1016/j.dld.2006.06.012
67. Stefka AT, Feehley T, Tripathi P, Qiu J, Mccoy K, Mazmanian SK, et al. Commensal bacteria protect against food allergen sensitization. Proc Natl Acad Sci U S A (2014) 111:13145–50. doi:10.1073/pnas.1412008111
68. Atarashi K, Tanoue T, Oshima K, Suda W, Nagano Y, Nishikawa H, et al. Treg induction by a rationally selected mixture of clostridia strains from the human microbiota. Nature (2013) 500:232–6. doi:10.1038/nature12331
69. Blázquez AB, Berin MC. Microbiome and food allergy. Transl Res (2017) 179:199–203. doi:10.1016/j.trsl.2016.09.003
70. Benedé S, Blázquez AB, Chiang D, Tordesillas L, Berin MC. The rise of food allergy: environmental factors and emerging treatments. EBioMedicine (2016) 7:27–34. doi:10.1016/j.ebiom.2016.04.012
71. Dascola CP, Caffarelli C. Exercise-induced anaphylaxis: a clinical view. Ital J Pediatr (2012) 38:43. doi:10.1186/1824-7288-38-43
72. Tewari A, Du TG, Lack G. The difficulties of diagnosing food-dependent exercise induced anaphylaxis in childhood – a case study and review. Pediatr Allergy Immunol (2006) 17:157–60. doi:10.1111/j.1399-3038.2005.00374.x
73. Aihara Y, Takahashi Y, Kotoyori T, Mitsuda T, Ito R, Aihara M, et al. Frequency of food-dependent, exercise-induced anaphylaxis in Japanese junior-high-school students. J Allergy Clin Immunol (2001) 108:1035–9. doi:10.1067/mai.2001.119914
74. Martino DJ, Saffery R, Allen KJ, Prescott SL. Epigenetic modifications: mechanisms of disease and biomarkers of food allergy. Curr Opin Immunol (2016) 42:9–15. doi:10.1016/j.coi.2016.05.005
75. Simons FE, Ebisawa M, Sanchez-Borges M, Thong T, Worm M, Tanno LK, et al. 2015 update of the evidence base: World Allergy Organization anaphylaxis guidelines. World Allergy Organ J (2015) 8:32. doi:10.1186/s40413-015-0080-1
76. Cardona V, Luengo O, Garriga T, Labrador-Horrillo M, Sala-Cunill A, Izquierdo A, et al. Co-factor-enhanced food allergy. Allergy (2012) 67:1316–8. doi:10.1111/j.1398-9995.2012.02877.x
77. Wesemann DR, Nagler CR. The microbiome, timing, and barrier function in the context of allergic disease. Immunity (2016) 44:728–38. doi:10.1016/j.immuni.2016.02.002
78. Natividad JM, Verdu EF. Modulation of intestinal barrier by intestinal microbiota: pathological and therapeutic implications. Pharmacol Res (2013) 69:42–51. doi:10.1016/j.phrs.2012.10.007
79. Peterson LW, Artis D. Intestinal epithelial cells: regulators of barrier function and immune homeostasis. Nat Rev Immunol (2014) 14:141–53. doi:10.1038/nri3608
80. Gallo RL, Hooper LV. Epithelial antimicrobial defense of the skin and intestine. Nat Rev Immunol (2012) 12:503–16. doi:10.1038/nri3228
81. Kim YS, Ho SB. Intestinal goblet cells and mucins in health and disease: recent insights and progress. Curr Gastroenterol Rep (2010) 12:319–30. doi:10.1007/s11894-010-0131-2
82. Nair MG, Guild KJ, Du Y, Zaph C, Yancopoulos GD, Valenzuela DM, et al. Goblet cell-derived resistin-like molecule β augments CD4+ T cell production of IFN-γ and infection-induced intestinal inflammation. J Immunol (2008) 181:4709–15. doi:10.4049/jimmunol.181.7.4709
83. Artis D, Wang ML, Keilbaugh SA, He W, Brenes M, Swain GP, et al. RELMβ/FIZZ2 is a goblet cell-specific immune-effector molecule in the gastrointestinal tract. Proc Natl Acad Sci U S A (2004) 101:13596–600. doi:10.1073/pnas.0404034101
84. McDole JR, Wheeler LW, McDonald KG, Wang B, Konjufca V, Knoop KA, et al. Goblet cells deliver luminal antigen to CD103+ dendritic cells in the small intestine. Nature (2012) 483:345–9. doi:10.1038/nature10863
85. Galli SJ, Tsai M. IgE and mast cells in allergic disease. Nat Med (2012) 18:693–704. doi:10.1038/nm.2755
86. Reber LL, Marichal T, Mukai K, Kita Y, Tokuoka SM, Roers A, et al. Selective ablation of mast cells or basophils reduces peanut-induced anaphylaxis in mice. J Allergy Clin Immunol (2013) 132:881–8.e1–11. doi:10.1016/j.jaci.2013.06.008
87. Crivellato E, Ribatti D. Cooperation of mast cells and basophils in allergy. J Allergy Ther (2013) 4:135. doi:10.4172/2155-6121.1000135
88. Galli SJ, Grimbaldeston M, Tsai M. Immunomodulatory mast cells: negative, as well as positive, regulators of immunity. Nat Rev Immunol (2008) 8:478–86. doi:10.1038/nri2327
89. Dwyer DF, Barrett NA, Austen KF. The immunological genome project consortium. Expression profiling of constitutive mast cells reveals a unique identity within the immune system. Nat Immunol (2016) 17:878–87. doi:10.1038/ni.3445
90. Huang H, Li Y. Mechanisms controlling mast cell and basophil lineage decisions. Curr Allergy Asthma Rep (2014) 14:457. doi:10.1007/s11882-014-0457-1
91. Kocabas CN, Yavuz AS, Lipsky PE, Metcalfe DD, Akin C. Analysis of the lineage relationship between mast cells and basophils using the c-kit D816V mutation as a biologic signature. J Allergy Clin Immunol (2005) 115:1155–61. doi:10.1016/j.jaci.2005.02.030
93. Okayama Y, Kawakami T. Development, migration, and survival of mast cells. Immunol Res (2006) 34:97–115. doi:10.1385/IR:34:2:97
94. Gurish MF, Austen KF. Developmental origin and functional specialization of mast cell subsets. Immunity (2012) 37:25–33. doi:10.1016/j.immuni.2012.07.003
95. Xing W, Austen KF, Gurish MF, Jones TG. Protease phenotype of constitutive connective tissue and of induced mucosal mast cells in mice is regulated by the tissue. Proc Natl Acad Sci U S A (2011) 108:14210–5. doi:10.1073/pnas.1111048108
96. Moon TC, St Laurent CD, Morris KE, Marcet C, Yoshimura T, Sekar Y, et al. Advances in mast cell biology: new understanding of heterogeneity and function. Mucosal Immunol (2010) 3:111–28. doi:10.1038/mi.2009.136
97. Weidner N, Austen KF. Heterogeneity of mast cells at multiple body sites. Fluorescent determination of avidin binding and immunofluorescent determination of chymase, tryptase, and carboxypeptidase content. Pathol Res Pract (1993) 189:156–62. doi:10.1016/S0344-0338(11)80086-5
98. Bulfone-Paus S, Nilsson G, Draber P, Blank U, Levi-Schaffer F. Positive and negative signals in mast cell activation. Trends Immunol (2017). doi:10.1016/j.it.2017.01.008
99. Metcalfe DD, Mekori YA. Pathogenesis and pathology of mastocytosis. Annu Rev Pathol (2017) 12:487–514. doi:10.1146/annurev-pathol-052016-100312
100. Varshney P, Yadav V, Saini N. Lipid rafts in immune signalling: current progress and future perspective. Immunology (2016) 149:13–24. doi:10.1111/imm.12617
101. Ashmole I, Bradding P. Ion channels regulating mast cell biology. Clin Exp Allergy (2013) 43:491–502. doi:10.1111/cea.12043
102. Gilfillan AM, Austin SJ, Metcalfe DD. Mast cell biology: introduction and overview. Annu Rev Pathol (2011) 716:2–12. doi:10.1007/978-1-4419-9533-9_1
103. Metcalfe DD, Peavy RD, Gilfillan AM. Mechanisms of mast cell signaling in anaphylaxis. J Allergy Clin Immunol (2009) 124:639–46. doi:10.1016/j.jaci.2009.08.035
104. Peavy RD, Metcalfe DD. Understanding the mechanisms of anaphylaxis. Curr Opin Allergy Clin Immunol (2008) 8:310–5. doi:10.1097/ACI.0b013e3283036a90
105. Ahrens B, Niggemann B, Wahn U, Beyer K. Organ-specific symptoms during oral food challenge in children with food allergy. J Allergy Clin Immunol (2012) 130:549–51. doi:10.1016/j.jaci.2012.05.045
106. Stone SF, Cotterell C, Isbister GK, Holdgate A, Brown SG; Emergency Department Anaphylaxis Investigators. Elevated serum cytokines during human anaphylaxis: identification of potential mediators of acute allergic reactions. J Allergy Clin Immunol (2009) 124:786–92. doi:10.1016/j.jaci.2009.07.055
107. Oschatz C, Maas C, Lecher B, Jansen T, Björkqvist J, Tradler T, et al. Mast cells increase vascular permeability by heparin-initiated bradykinin formation in vivo. Immunity (2011) 34:258–68. doi:10.1016/j.immuni.2011.02.008
109. Brown SG, Stone SF, Fatovich DM, Burrows SA, Holdgate A, Celenza A, et al. Anaphylaxis: clinical patterns, mediator release, and severity. J Allergy Clin Immunol (2013) 132:1141–9.e5. doi:10.1016/j.jaci.2013.06.015
110. Wang M, Han J, Domenico J, Shin YS, Jia Y, Gelfand EW. Combined blockade of the histamine H1 and H4 receptor suppresses peanut-induced intestinal anaphylaxis by regulating dendritic cell function. Allergy (2016) 71:1561–74. doi:10.1111/all.12904
111. Sala-Cunill A, Cardona V, Labrador-Horrillo M, Luengo O, Esteso O, Garriga T, et al. Usefulness and limitations of sequential serum tryptase for the diagnosis of anaphylaxis in 102 patients. Int Arch Allergy Immunol (2013) 160:192–9. doi:10.1159/000339749
112. Sahiner UM, Yavuz ST, Buyuktiryaki B, Cavkaytar O, Yilmaz EA, Tuncer A, et al. Serum basal tryptase may be a good marker for predicting the risk of anaphylaxis in children with food allergy. Allergy (2014) 69:265–8. doi:10.1111/all.12317
113. Wongkaewpothong P, Pacharn P, Sripramong C, Boonchoo S, Piboonpocanun S, Visitsunthorn N, et al. The utility of serum tryptase in the diagnosis of food-induced anaphylaxis. Allergy Asthma Immunol Res (2014) 6:304–9. doi:10.4168/aair.2014.6.4.304
114. Zhou X, Whitworth HS, E-Khedr M, Brown TA, Goswami R, Eren E, et al. Mast cell chymase: a useful serum marker in anaphylaxis. J Allergy Clin Immunol (2011) 127:AB143. doi:10.1016/j.jaci.2010.12.566
115. Schauberger E, Peinhaupt M, Cazares T, Lindsley AW. Lipid mediators of allergic disease: pathways, treatments, and emerging therapeutic targets. Curr Allergy Asthma Rep (2016) 16:48. doi:10.1007/s11882-016-0628-3
116. Ono E, Taniguchi M, Mita H, Fukutomi Y, Higashi N, Miyazaki E, et al. Increased production of cysteinyl leukotrienes and prostaglandin D2 during human anaphylaxis. Clin Exp Allergy (2009) 39:72–80. doi:10.1111/j.1365-2222.2008.03104.x
117. Vadas P, Perelman B, Liss G. Platelet-activating factor, histamine, and tryptase levels in human anaphylaxis. J Allergy Clin Immunol (2013) 131:144–9. doi:10.1016/j.jaci.2012.08.016
118. Vadas P, Gold M, Perelman B, Liss GM, Lack G, Blyth T, et al. Platelet-activating factor, PAF acetylhydrolase, and severe anaphylaxis. N Engl J Med (2008) 358:28–35. doi:10.1056/NEJMoa070030
119. Oyoshi MK, Oettgen HC, Chatila TA, Geha RS, Bryce PJ. Food allergy: insights into etiology, prevention, and treatment provided by murine models. J Allergy Clin Immunol (2014) 133:309–17. doi:10.1016/j.jaci.2013.12.1045
120. Galli SJ, Nakae S, Tsai M. Mast cells in the development of adaptive immune responses. Nat Immunol (2005) 6:135–42. doi:10.1038/ni1158
Keywords: food-induced anaphylaxis, IgE, allergens, diet, mast cells, basophils
Citation: Benedé S, Garrido-Arandia M, Martín-Pedraza L, Bueno C, Díaz-Perales A and Villalba M (2017) Multifactorial Modulation of Food-Induced Anaphylaxis. Front. Immunol. 8:552. doi: 10.3389/fimmu.2017.00552
Received: 14 March 2017; Accepted: 25 April 2017;
Published: 16 May 2017
Edited by:
Toshiaki Kawakami, La Jolla Institute for Allergy and Immunology, USAReviewed by:
Annette Kuehn, Luxembourg Institute of Health, LuxembourgCopyright: © 2017 Benedé, Garrido-Arandia, Martín-Pedraza, Bueno, Díaz-Perales and Villalba. This is an open-access article distributed under the terms of the Creative Commons Attribution License (CC BY). The use, distribution or reproduction in other forums is permitted, provided the original author(s) or licensor are credited and that the original publication in this journal is cited, in accordance with accepted academic practice. No use, distribution or reproduction is permitted which does not comply with these terms.
*Correspondence: Mayte Villalba, bXZpbGxhbGJAdWNtLmVz
Disclaimer: All claims expressed in this article are solely those of the authors and do not necessarily represent those of their affiliated organizations, or those of the publisher, the editors and the reviewers. Any product that may be evaluated in this article or claim that may be made by its manufacturer is not guaranteed or endorsed by the publisher.
Research integrity at Frontiers
Learn more about the work of our research integrity team to safeguard the quality of each article we publish.