- 1Health Sciences Research Centre (CICS-UBI), Universidade da Beira Interior, Covilhã, Portugal
- 2Faculty of Health Sciences (FCS-UBI), Universidade da Beira Interior, Covilhã, Portugal
Understanding the rationale for the generation of a pool of highly differentiated effector memory CD8+ T cells displaying a weakened capacity to scrutinize for peptides complexed with major histocompatibility class I molecules via their T cell receptor, lacking the “signal 2” CD28 receptor, and yet expressing a highly diverse array of innate receptors, from natural killer receptors, interleukin receptors, and damage-associated molecular pattern receptors, among others, is one of the most challenging issues in contemporary human immunology. The prevalence of these differentiated CD8+ T cells, also known as CD8+CD28−, CD8+KIR+, NK-like CD8+ T cells, or innate CD8+ T cells, in non-lymphoid organs and tissues, in peripheral blood of healthy elderly, namely centenarians, but also in stressful and chronic inflammatory conditions suggests that they are not merely end-of-the-line dysfunctional cells. These experienced CD8+ T cells are highly diverse and capable of sensing a variety of TCR-independent signals, which enables them to respond and fine-tune tissue homeostasis.
Preface
Thanks to their T cell antigen receptor (TCR), thymus-derived CD8+ T cells have the unique ability to scrutinize any cell of our body displaying at the cell surface peptides bound to major histocompatibility class I (MHC-I) molecules and respond by means of cell activation and proliferation whenever the MHC-I molecule looks different than usual. In this regard, the quote “Divide and Conquer” (from the Latin saying Divide et Impera, credited to Julius Caesar) is the name of an algorithm that solves a problem by breaking it sequentially into two or more sub-problems until these become simple enough to be solved (1). Paraphrasing the quote and the algorithm, it can be anticipated that the tendency of a thymus-derived CD8+ T cell to divide and generate a progeny of cells is meant to solve a problem, keep body homeostasis, by making conquerors that travel to distant injured/inflamed tissues (effector CD8+ T cells), some of which may mutiny and become a problem (inflammatory CD8+ T cells) and have to be restrained by peacekeepers (suppressor/regulator CD8+ T cells). At the end of the process, a mixture of the different subsets survives as sensors of any further change that may occur within the environment they visited and conquered (memory CD8+ T cells). Human CD8+ T cell differentiation is a complex process enfolded in contrasting views on the functional role of the memory CD8+ T cells under normal and diseased conditions. Hereby, we present a perspective on the function of these CD8+ T cells that focus on the relationship with their internal environment.
CD8+ T Cell Differentiation: One-Way Ticket to Pleiotropy
Before leaving the thymus to enter the circulation, CD8+ T cells survive two critical events that determine their fate in the periphery. First, they learn to trans-interact via their TCR clonotypic receptor with composites of a MHC-I heavy chain, a light chain (β2m), and a short peptide (2). These antigen-presenting MHC-I structures are also designated “closed conformers” to distinguish them from the “open conformers” that are constituted only by the MHC-I heavy chain after dissociation from the light chain and/or the peptide and that can exist at the cell surface in an ordered non-denatured form (3). Open conformers can interact in trans and cis with a variety of receptors, namely members of the natural killer receptor (NKR) family, with important functional implications, as discussed below. The recognition of closed MHC-I conformers gives naïve CD8+ T cells the capacity to survive in the periphery and eventually recognize and be activated by closed MHC-I conformers presenting an excess of unusual antigens (4). After activation, naïve CD8+ T cells enter differentiation programs that result in the generation of effector CD8+ T cells displaying different bioactivities (5). After the excess of antigen is neutralized and removed, homeostatic mechanisms are turned on to cease the effector function while keeping a small pool that remains in circulation as memory CD8+ T cells (6). Second, CD8+ T cells are genetically programed to express an array of receptors during the differentiation process, which allows them to receive activation and survival signals from receptors and ligands other than MHC class I closed conformers (3, 7–10).
As a result of the huge effort done during the last decades and based on the expression of CCR7, CD27, CD28, CD45RA, and others, we have now a close picture of the main differentiation stages of human CD8+ T cells (Figure 1). Thus, the recirculating peripheral CD8+ T cell compartment is a mixture of lymphocytes distributed among five major pools: naïve (Tn), stem-cell memory (Tscm), central memory (Tcm), effector memory (Tem), and effector memory CD45RA+ (Temra) (11–13). An additional pool of non-recirculating tissue-resident memory cells (Trm) has also been described (14). Despite certain phenotypic and functional overlap among these CD8+ T cell pools, this classification has been most useful to describe the level of differentiation that the CD8+ T cell compartment has endured under different inflammatory settings, such as autoimmunity, cancer, and acute and chronic viral responses (15–17). Yet, perhaps the most significant achievement has been the identification of genes differently expressed by these pools, allowing to envision novel roles for CD8+ T cells (7, 18–20).
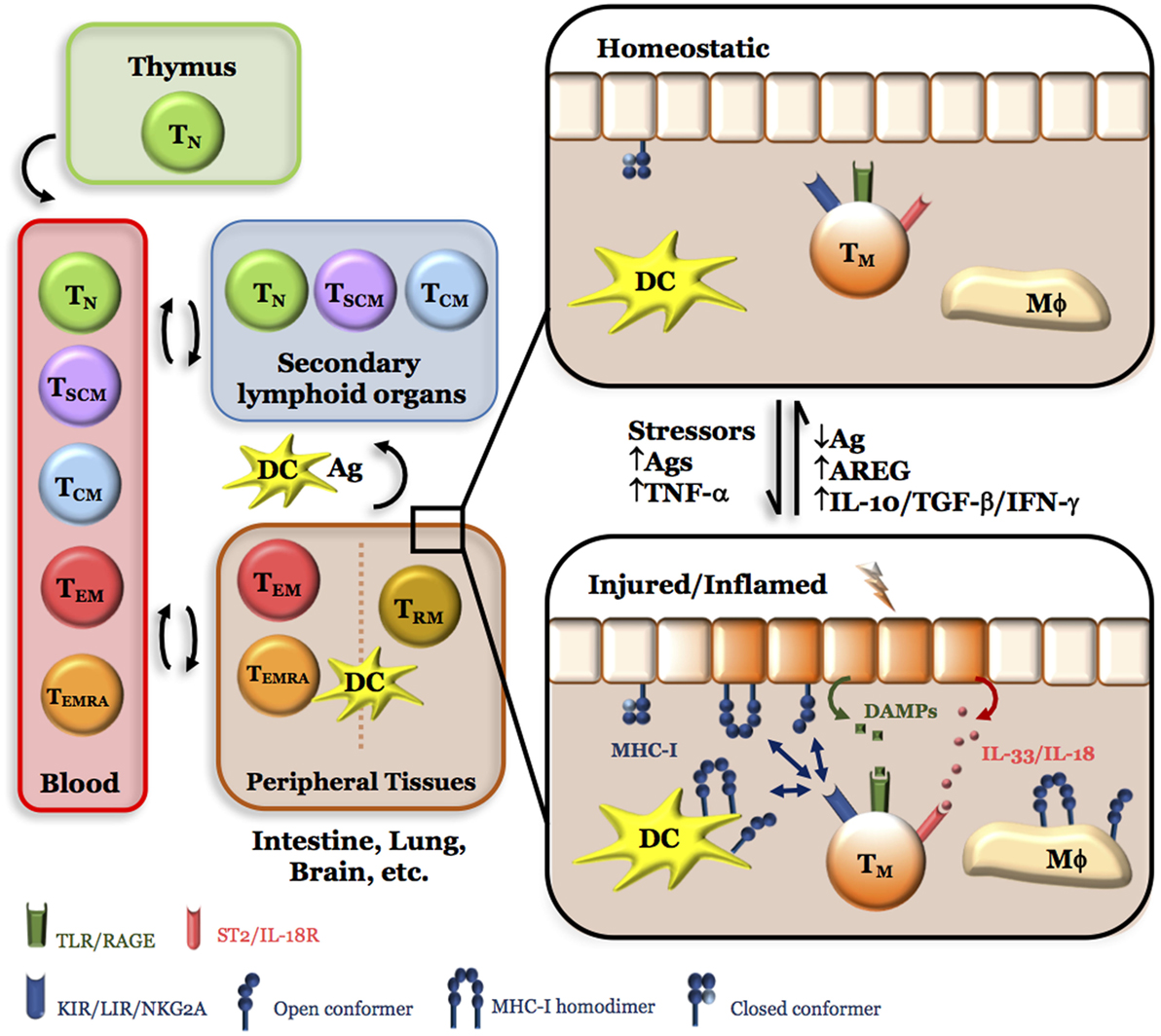
Figure 1. Simplified model for the role of NK-like CD8+ Tem cells in tissue integrity. Of the five major circulating CD8+ T cell pools, naive (Tn), stem-cell memory (Tscm), and central memory (Tcm) preferentially migrate to secondary lymphoid organs, where they can be activated by processed antigens presented by closed major histocompatibility class I (MHC-I) conformers expressed by dendritic cells (DC) recently arrived from peripheral tissues and differentiate into effector memory (Tem) and effector memory CD45RA+ (Temra). On the other hand, CD8+ Tem and Temra have preferential, but not exclusive, access to peripheral tissues under homeostatic (healthy) conditions where they can stay as CD8+ Trm. Under tissue stress and/or injury, a sudden increase in antigens (Ags) and/or inflammatory cytokines (TNF-α) results in the release of endogenous products [damage-associated molecular patterns (DAMP), IL-33, ATP, etc.] and expression of open MHC-I conformers by immune and non-immune cells. While tissue DCs could migrate to secondary lymphoid organs and induce more cycles of CD8+ T cell activation and differentiation, Tem, Temra, and Trm (denoted as Tm for simplicity) could directly sense these changes in loco; thanks to the expression of killer Ig-like receptor, leukocyte Ig-like receptor, NKG2A, DAMP receptors, IL-18/IL-33 receptors, purinergic receptors, and others. Thus, the presence of CD8+ Tm cells in peripheral tissues allows a faster response to harmful situations by secreting cytokines (IFN-γ, IL-10, TGF-β) and factors [amphiregulin (AREG)] that activate pathways leading to tissue repair and regeneration, and, therefore, to the homeostatic (healthy) state. Any imbalance in this equilibrium (e.g., overt tissue injury and necrosis, hypoxia, excess of antigen, high CD4/CD8 T cell ratios, low numbers or absence of CD8+ Tem, Temra, Trm cells, etc.) will result in a failure to resolve inflammation and chronic inflammation will ensue.
The CD8+ Tn pool comprises polyclonal T cells recently emigrated from the thymus that express CD28, CCR7, and CD62L, the two latter allowing them to recirculate between blood and secondary lymphoid organs (21). The CD8+ Tem and CD8+ Temra pools (for easiness, both termed as Tem thereafter) are highly differentiated CD8+ T cells that differ in the expression of the tyrosine phosphatase isoform CD45RA. They were formerly described as lymphocytes lacking CD28, responding poorly to TCR-stimulation, displaying redirected cytotoxicity, containing oligoclonal T cells, and being able to migrate to non-lymphoid organs and tissues (21–23). CD8+ T cells with the Tem phenotype were reported to express receptors thought to be solely expressed by NK cells, including CD56, CD94/NKG2A, killer Ig-like receptors (KIR), and leukocyte Ig-like receptors (LIR), among others (24–26). CD8+ T cells with the Tem phenotype also express NKp46 (27), a member of the natural cytotoxicity receptor, akin to several inhibitory receptors, such as CTLA-4, PD1, TIM3, and LAG3 (28). Due to these distinguishing features, they have also been designated CD8+CD28–, CD8+KIR+, CD8+NKR+, NK-like CD8+ T cells, and more recently innate CD8+ T cells (29–32). The evidence gathered during recent years suggests that the human CD8+ Tem pool is very diverse and polyfunctional and contains cells endowed with suppressor, inflammatory, and cytotoxic features (25–35). Whether these polyfunctional CD8+ Tem cells reflect the existence of distinctive subsets or a pleiotropic CD8+ T cell population that displays its activities depending on the signals that receive in the different tissue environments, remains to be elucidated.
On the Origin of NK-Like CD8+ T Cells: Aging, Viruses, Cytokines, and More
Following the initial description of CD8+CD28− T cells in the late 1980s and early 1990s, high levels of these cells were described in peripheral blood of healthy elderly people, during viral infections (e.g., CMV, HIV, and EBV), cancer, and autoimmunity (8). Nowadays, alterations in CD8+CD28− T cells have been reported in almost every chronic inflammatory disease. Studies performed on CMV-seropositive elderly showed that a sizable fraction of CD8+CD28− T cells contains CMV-specific CD8+ T cells (17). The description in the elderly of an association between the accumulation of CD8+CD28− T cells, a phenomenon called memory CD8+ T cell inflation (36), CMV seropositivity, a decrease in survival rate and faulty in vivo humoral and cellular responses to vaccination, brought about the view that CD8+ Tem cells were terminally differentiated dysfunctional cells that contributed to immunosenescence and susceptibility to develop chronic inflammatory diseases (35–40). Recent studies are revealing that CMV-specific CD8+ Tem are not dysfunctional cells. Rather, they are polyfunctional in terms of cytokine secretion and proliferation (41–44), capable of surviving for longer periods of time (45, 46), and are only functionally restricted by the set of inhibitory receptors they express (28, 41). On the other hand, longitudinal studies comparing IgG titers and DNA viral load with CMV-specific CD8+ T cell frequencies suggest that CMV serology may not be a reliable indicator to study associations between chronic CVM infection and CD8+ Tem cell expansions (47). Thus, the association between CD8+ T cell expansions, CMV seropositivity, immunosenescence, and predisposition to disease remains an open question (48).
Besides chronic activation by viral antigens, there is solid evidence that cytokines such as IL-15, TNF-α, and TGF-β, as well as several cell types, regulate CD8+ T cell homeostasis. IL-15 displays multiple bioactivities, namely induction and maintenance of CD8+ Tem cells in vitro and in vivo (49–54), suggesting that memory CD8+ T cell inflation may also result from encounters with cytokines, thus increasing virtual memory CD8+ T cells (55). IL-15 is also involved in liver homeostasis and regeneration after hepatectomy (56). Whether this bioactivity is linked to the pro-survival activities of hepatocytes on CD8+ T cells and the presence of large amounts of CD8+ Tem cells in the liver, remains to be elucidated (27, 57). On the other hand, reconstitution studies in mice have shown that accumulation of CD8+ Tem cells depends on the presence of IL-15 and CD4+ T cells (9). Finally, intestinal epithelial cells and 4-1BBL+ B cells have also been shown to drive expansion and accumulation of CD8+ T cells with the Tem phenotype in vitro (58, 59) and in vivo (60), respectively, expanding the universe of factors that drive NK-like CD8+ T cell generation.
While unnoticed, expansions of CD8+ T cells with a Tem phenotype were described in conditions where oxidative stress is high, including HFE hemochromatosis, heavy alcohol consumption, hemodialysis, β-thalassemia, and during acute exercise (61–65). Although the molecular cues underlying CD8+CD28− Tem generation under stressful conditions are uncertain, PGE2, a byproduct of arachidonic acid catabolism produced under pro-oxidant and inflammatory conditions, induces expression of NKG2A and downregulates CD28 on CD8+ T cells, two features associated with the acquisition of the Tem phenotype (66–68). Oxidative stress has also been shown to regulate expression of Bach2, a transcription factor involved in the formation of CD8+ Tem cells through downregulation of genes associated with effector function, such as Blimp1 (69, 70). On the other hand, expression of the transcription factor HIF-1 by CD8+ T cells in vitro under low oxygen conditions, mimicking acute exercise, correlates with accumulation of CD8+ Tem cells (71), which is in agreement with the reported role of HFI-1 in modulating the balance between effector and memory CD8+ T cells in models of chronic activation (72). These data suggest that oxidative stress may play an important role in modulating the formation of CD8+ Tem cells.
Finally, the CD4/CD8 T cell ratio is a factor that appears to influence the extent of the CD8+CD28− T cell expansions. Early studies in HFE hemochromatosis and heavy alcohol drinkers reported a positive correlation between the size of the CD8+CD28− T cell expansions and the size of the CD8+ T cell pool, regardless of age (61, 62). Importantly, the regression curve had a much higher slope and correlation coefficient in the patients than in the control group, implying that under stressful/adverse conditions there is a hastened formation of CD8+CD28− T cells (8, 61, 62). Similar results were observed when the percentage of CD8+CD56+ T cells, which is increased in the elderly, was analyzed (73), strongly suggesting that the expansions of CD8+CD28− T cells are constrained by the size of the CD8+ T cell pool in relation to the CD4+, which are both under the control of major autosomal recessive genes (74). The importance of this influence is illustrated by two sets of studies. First, studies in infants with overt CD4+ T cell lymphopenia and reversed CD4/CD8 T cell ratios, due to deficiency in the tyrosine kinase p56lck, showed that the peripheral CD8+ T cell pool was made up almost entirely of CD8+ T cells with the Tem phenotype (75–77). Second, a recent cross-sectional study in elderly people, including centenarians, showed that the heterogeneity found in the CD8+ Tem pool could be explained by variations in the size of the CD8+ T cell compartment (78). Although it is difficult to discern what is cause and what is effect, we favor the view that the CD4/CD8 T cell ratio influences the extent of the CD8+CD28− T cell expansions. In this context, it is worth mentioning that the expansions CD8+CD28− T cells reported in HFE hemochromatosis patients were paralleled by a defective CD8-associated p56lck (61, 79). In view of these data and studies in mice showing that CD8-associated Lck is dispensable for maintenance of memory CD8+ T cells (80), it is tempting to speculate that in humans a deficient CD8-p56lck signaling and expansion of CD8+CD28− T cells could be intertwined processes.
On the Function of NK-Like CD8+ T Cells: There is Life Beyond Closed MHC-I Conformers
The accumulated evidence indicates that loss of CD28, shrinkage of the TCR repertoire, gain of a variety of NKR, and expression of tissue homing receptors are interdependent events that end up in the formation of polyfunctional human CD8+ Tem cells that migrate to peripheral tissues where a fraction stays as a pool of non-recirculating CD8+ Trm cells upon expression of CD69 and CD103 (14). A series of recent studies using tissue samples from otherwise healthy infant and adult organ donors have shown that CD8+ Tem cells are predominant within non-lymphoid tissues and organs, including the brain, and this prevalence increases from childhood to adultness (81–84). CD8+ Tem predominance also occurs in the healthy bone marrow, stomach, and gingiva (85–87). Interestingly, lower CD4/CD8 T cell ratios within these tissues are associated with a larger CD8+ Tem pool (81), pointing again to the importance of the molecular cues that regulate this setting. Although recirculating and non-recirculating CD8+ Tem present in non-lymphoid tissues confer local immune protection against infections (88–90), it is also true that CD8+ Tem cells adapt to the new environment and may participate in the resolution of inflammation followed by tissue regeneration and repair after injury through complex networks involving cross-talk with other tissue environmental cells (91–99).
The picture emerges where CD28 loss and expression KIR, LIR and other NKR allows CD8+ Tem cells to engage in a cross-talk with other cells in their environment (8, 100, 101). But how this acquired skill is conveyed in terms of control of tissue integrity and organ function? As already mentioned, cell surface MHC-I molecules can exist in equilibrium between closed and open conformers, a process that is regulated by endocytosis and phosphorylation of a conserved tyrosine residue in the cytoplasmic tail of MHC-I heavy chains, and that allows the open conformers to self-associate and form novel structures called class I homodimers (3, 102–104). In this context, a series of recent reports are unveiling the many lives of MHC class I molecules (105), by showing that besides interacting with closed conformers in a peptide-independent manner (106, 107), KIR and LIR also interact with open conformers and homodimers (108–114). These results are of upmost importance if we consider that open conformers are expressed and/or released by metabolically active and stressed immune and non-immune cells, including neurons (103, 115–119). Thus, expression of NKR by CD8+ Tem cells allows them to sense changes in the level of closed and open MHC-I conformers, i.e., in the stressful/inflammatory state of the environment. The impact that KIR/LIR/NKG2A engagement has on CD8+ T cell survival and cytokine secretion (29, 52, 120), is of the foremost importance for tissue homeostasis under normal or pathological conditions. Thus, IFN-γ and other cytokines released by CD8+ Tem upon NKR triggering could mediate resolution of inflammation and subsequent tissue healing by modulating growth and proliferation of epithelial and other tissue cells (121, 122). Importantly, by inducing upregulation of classical and non-classical MHC-I molecules on epithelial/endothelial cells, IFN-γ may further promote survival and proliferation signals upon MHC-I reverse signaling by their cognate NKR expressed by CD8+ Tem cells (123), thus harnessing the healing process.
Although expression of NKR allows CD8+ Tem cells located in peripheral tissues to sense changes in the closed ↔ open MHC-I equilibrium, this is likely not enough to cope with the fluctuations that occur within an ever changing internal environment (Figure 1). In this respect, there is evidence that CD8+ Tem cells also express receptors for damage-associated molecular patterns (DAMP), which are specialized in recognizing endogenous products released by cell stress, injury, or dead (124). DAMP receptors include toll-like receptors (TLR), advance glycosylation end products receptors (RAGE), receptors for IL-1 family members (e.g., IL-18 and IL-33), purinergic receptors (P2YR), and β2-adrenergic receptors (19, 125–129), to cite some. Although most of the studies on these receptors have focused on innate cells, there is growing evidence that DAMP receptor expression by CD8+ Tem cells could broaden their capacity to sense the disruption of tissue homeostasis and respond by secreting regulatory cytokines and healing factors. Thus, ligation of TLR on CD8+ Tem cells is known to augment IFN-γ in in vitro and in vivo settings (130, 131), which in barrier tissues such as the lung, where a large fraction of CD8+ T cells are Temra (81) may exacerbate tissue pathology (132). RAGE encompasses multiple ligands, including glycated proteins, nuclear high-mobility group box 1 (HMGB1), S100 proteins, and β-amyloid, among others, which transmit intracellular signals associated with tissue repair and regeneration (133, 134). RAGE+CD8+ T cells were described more than two decades ago and proposed to participate in the regulation of tissue homeostasis through secretion of IFN-γ (135). HMGB1 can also bind to TIM3, an inhibitory receptor expressed on CD8+ Temra cells, whose inhibitory function depends on the co-expression of CEACAM-1 (28, 136). Although expression of the IL-18 and IL-33 receptors by CD8+ T cells is known for some time (127), their importance in the regulation of tissue repair by T cells has only recently emerged (137–139). Thus, binding of IL-18 and IL-33 to regulatory T cells triggers the secretion of amphiregulin (AREG), a ligand for the EGF receptor involved in suppression of inflammation and tissue repair (140, 141). Although formal proof for the secretion of AREG by CD8+ Tem cells is lacking, there is evidence that CD8+ T cells express this tissue repair factor (141, 142). Finally, CD8+ Tem cells also express purinergic receptors (19), as well as the β2-adrenergic receptor (129). While the former can sense environmental nucleotides released under adverse conditions and induce suppressive signals on T cells, thus downplaying inflammation (128, 143), the latter allows the sympathetic nervous system to communicate under stressful conditions with CD8+ T cells (144, 145).
Concluding Remarks
Although one important facet of CD8+ T cells has to do with tissue damage and injury resulting from coping with infections, this should not overshadow other facets of CD8+ T cells related with the maintenance of tissue integrity and homeostasis (Figure 1). Since the description of CD8+CD28− T cells about 25 years ago, a huge amount of information has been obtained on the functional phenotype and localization of these lymphocytes. Their capacity to migrate and reside in peripheral tissues in parallel with the expression of receptors for unconventional MHC-I molecules and endogenous products released by injured, inflamed, and necrotic cells may endow these cells with the capacity to fine-tune tissue repair, regeneration, and homeostasis by a number of ways, namely by inducing epithelial, endothelial, and mesenchymal cells to grow and proliferate and inhibiting inflammatory responses. All these bioactivities will likely involve active crosstalk with environmental cells and complex loops between secreted cytokines.
Author Contributions
FA and EC wrote the manuscript. EC drew the figure. AE and CP read and edited the manuscript.
Conflict of Interest Statement
The authors declare that the research was conducted in the absence of any commercial or financial relationships that could be construed as a potential conflict of interest.
Acknowledgments
The authors would like to thank the people who made this manuscript possible.
Funding
The work by the authors was supported by FEDER funds through the POCI—COMPETE 2020—Operational Program Competitiveness and Internationalization in Axis I—strengthening research, technological development, and innovation (Project No. 007491), and National Funds by FCT—Foundation for Science and Technology (Project UID/Multi/00709).
References
2. Li XL, Teng MK, Reinherz EL, Wang JH. Strict major histocompatibility complex molecule class-specific binding by co-receptors enforces MHC-restricted αβ TCR recognition during T lineage subset commitment. Front Immunol (2013) 4:383. doi: 10.3389/fimmu.2013.00383
3. Arosa FA, Santos SG, Powis SJ. Open conformers: the hidden face of MHC-I molecules. Trends Immunol (2007) 28(3):115–23. doi:10.1016/j.it.2007.01.002
4. Freitas AA, Rocha B. Population biology of lymphocytes: the flight for survival. Annu Rev Immunol (2000) 18:83–111. doi:10.1146/annurev.immunol.18.1.83
5. Shrikant PA, Rao R, Li Q, Kesterson J, Eppolito C, Mischo A, et al. Regulating functional cell fates in CD8 T cells. Immunol Res (2010) 46(1–3):12–22. doi:10.1007/s12026-009-8130-9
6. Sprent J, Surh CD. Normal T cell homeostasis: the conversion of naive cells into memory-phenotype cells. Nat Immunol (2011) 12(6):478–84. doi:10.1038/ni.2018
7. Weng NP, Araki Y, Subedi K. The molecular basis of the memory T cell response: differential gene expression and its epigenetic regulation. Nat Rev Immunol (2012) 12(4):306–15. doi:10.1038/nri3173
8. Arosa FA. CD8+CD28- T cells: certainties and uncertainties of a prevalent human T-cell subset. Immunol Cell Biol (2002) 80(1):1–13. doi:10.1046/j.1440-1711.2002.01057.x
9. Zaragoza B, Evaristo C, Kissenpfennig A, Libri V, Malissen B, Rocha B, et al. Cell-to-cell interactions and signals involved in the reconstitution of peripheral CD8 T(CM) and T(em) cell pools. PLoS One (2011) 6(3):e17423. doi:10.1371/journal.pone.0017423
10. Heikamp EB, Powell JD. Sensing the immune microenvironment to coordinate T cell metabolism, differentiation & function. Semin Immunol (2012) 24(6):414–20. doi:10.1016/j.smim.2012.12.003
11. Appay V, van Lier RA, Sallusto F, Roederer M. Phenotype and function of human T lymphocyte subsets: consensus and issues. Cytometry A (2008) 73(11):975–83. doi:10.1002/cyto.a.20643
12. Mahnke YD, Brodie TM, Sallusto F, Roederer M, Lugli E. The who’s who of T-cell differentiation: human memory T-cell subsets. Eur J Immuno (2013) 43(11):2797–809. doi:10.1002/eji.201343751
13. Restifo NP, Gattinoni L. Lineage relationship of effector and memory T cells. Curr Opin Immunol (2013) 25(5):556–63. doi:10.1016/j.coi.2013.09.003
14. Thome JJ, Farber DL. Emerging concepts in tissue-resident T cells: lessons from humans. Trends Immunol (2015) 36(7):428–35. doi:10.1016/j.it.2015.05.003
15. Mayerl C, Prelog M. Immunosenescence and juvenile idiopathic arthritis. Autoimmun Rev (2012) 11(5):297–300. doi:10.1016/j.autrev.2010.02.015
16. Reiser J, Banerjee A. Effector, memory, and dysfunctional CD8(+) T cell fates in the antitumor immune response. J Immunol Res (2016) 2016:8941260. doi:10.1155/2016/8941260
17. van Aalderen MC, Remmerswaal EB, Verstegen NJ, Hombrink P, ten Brinke A, Pircher H, et al. Infection history determines the differentiation state of human CD8+ T cells. J Virol (2015) 89(9):5110–23. doi:10.1128/JVI.03478-14
18. Duraiswamy J, Ibegbu CC, Masopust D, Miller JD, Araki K, Doho GH, et al. Phenotype, function, and gene expression profiles of programmed death-1(hi) CD8 T cells in healthy human adults. J Immunol (2011) 186(7):4200–12. doi:10.4049/jimmunol.1001783
19. Gattinoni L, Lugli E, Ji Y, Pos Z, Paulos CM, Quigley MF, et al. A human memory T cell subset with stem cell-like properties. Nat Med (2011) 17(10):1290–7. doi:10.1038/nm.2446
20. Yang C, Khanniche A, DiSpirito JR, Ji P, Wang S, Wang Y, et al. Transcriptome signatures reveal rapid induction of immune-responsive genes in human memory CD8(+) T cells. Sci Rep (2016) 6:27005. doi:10.1038/srep27005
21. Sallusto F, Geginat J, Lanzavecchia A. Central memory and effector memory T cell subsets: function, generation, and maintenance. Annu Rev Immunol (2004) 22:745–63. doi:10.1146/annurev.immunol.22.012703.104702
22. Azuma M, Phillips JH, Lanier LL. CD28− T lymphocytes. Antigenic and functional properties. J Immunol (1993) 150(4):1147–59.
23. Posnett DN, Sinha R, Kabak S, Russo C. Clonal populations of T cells in normal elderly humans: the T cell equivalent to “benign monoclonal gammapathy.” J Exp Med (1994) 179(2):609–18. doi:10.1084/jem.179.2.609
24. Mingari MC, Ponte M, Vitale C, Bellomo R, Moretta L. Expression of HLA class I-specific inhibitory receptors in human cytolytic T lymphocytes: a regulated mechanism that controls T-cell activation and function. Hum Immunol (2000) 61(1):44–50. doi:10.1016/S0198-8859(99)00158-5
25. Speiser DE, Valmori D, Rimoldi D, Pittet MJ, Lienard D, Cerundolo V, et al. CD28 negative cytolytic effector T cells frequently express NK receptors and are present at variable proportions in circulating lymphocytes from healthy donors and melanoma patients. Eur J Immunol (1999) 29(6):1990–9. doi:10.1002/(SICI)1521-4141(199906)29:06<1990::AID-IMMU1990>3.0.CO;2-9
26. Anfossi N, Pascal V, Vivier E, Ugolini S. Biology of T memory type 1 cells. Immunol Rev (2001) 181:269–78.
27. Correia MP, Cardoso EM, Pereira CF, Neves R, Uhrberg M, Arosa FA. Hepatocytes and IL-15: a favorable microenvironment for T cell survival and CD8+ T cell differentiation. J Immunol (2009) 182(10):6149–59. doi:10.4049/jimmunol.0802470
28. Fuertes Marraco SA, Neubert NJ, Verdeil G, Speiser DE. Inhibitory receptors beyond T cell exhaustion. Front Immunol (2015) 6:310. doi:10.3389/fimmu.2015.00310
29. Vivier E, Anfossi N. Inhibitory NK-cell receptors on T cells: witness of the past, actors of the future. Nat Rev Immunol (2004) 4(3):190–8. doi:10.1038/nri1306
30. Abedin S, Michel JJ, Lemster B, Vallejo AN. Diversity of NKR expression in aging T cells and in T cells of the aged: the new frontier into the exploration of protective immunity in the elderly. Exp Gerontol (2005) 40(7):537–48. doi:10.1016/j.exger.2005.04.012
31. Vallejo AN, Mueller RG, Hamel DL Jr, Way A, Dvergsten JA, Griffin P, et al. Expansions of NK-like αβT cells with chronologic aging: novel lymphocyte effectors that compensate for functional deficits of conventional NK cells and T cells. Ageing Res Rev (2011) 10(3):354–61. doi:10.1016/j.arr.2010.09.006
32. Jacomet F, Cayssials E, Basbous S, Levescot A, Piccirilli N, Desmier D, et al. Evidence for eomesodermin-expressing innate-like CD8(+) KIR/NKG2A(+) T cells in human adults and cord blood samples. Eur J Immunol (2015) 45(7):1926–33. doi:10.1002/eji.201545539
33. Vlad G, Cortesini R, Suciu-Foca N. CD8+ T suppressor cells and the ILT3 master switch. Hum Immunol (2008) 69(11):681–6. doi:10.1016/j.humimm.2008.08.286
34. Dinesh RK, Skaggs BJ, La Cava A, Hahn BH, Singh RP. CD8+ Tregs in lupus, autoimmunity, and beyond. Autoimmun Rev (2010) 9(8):560–8. doi:10.1016/j.autrev.2010.03.006
35. Sansoni P, Vescovini R, Fagnoni F, Biasini C, Zanni F, Zanlari L, et al. The immune system in extreme longevity. Exp Gerontol (2008) 43(2):61–5. doi:10.1016/j.exger.2007.06.008
36. O’Hara GA, Welten SP, Klenerman P, Arens R. Memory T cell inflation: understanding cause and effect. Trends Immunol (2012) 33(2):84–90. doi:10.1016/j.it.2011.11.005
37. Koch S, Larbi A, Ozcelik D, Solana R, Gouttefangeas C, Attig S, et al. Cytomegalovirus infection: a driving force in human T cell immunosenescence. Ann N Y Acad Sci (2007) 1114:23–35. doi:10.1196/annals.1396.043
38. Weng NP, Akbar AN, Goronzy J. CD28(-) T cells: their role in the age-associated decline of immune function. Trends Immunol (2009) 30(7):306–12. doi:10.1016/j.it.2009.03.013
39. Pera A, Campos C, López N, Hassouneh F, Alonso C, Tarazona R, et al. Immunosenescence: implications for response to infection and vaccination in older people. Maturitas (2015) 82(1):50–5. doi:10.1016/j.maturitas.2015.05.004
40. Fulop T, Larbi A, Kotb R, de Angelis F, Pawelec G. Aging, immunity, and cancer. Discov Med (2011) 11(61):537–50.
41. van der Veken LT, Diez Campelo M, van der Hoorn MA, Hagedoorn RS, van Egmond HM, van Bergen J, et al. Functional analysis of killer Ig-like receptor-expressing cytomegalovirus-specific CD8+ T cells. J Immunol (2009) 182(1):92–101. doi:10.4049/jimmunol.182.1.92
42. Lachmann R, Bajwa M, Vita S, Smith H, Cheek E, Akbar A, et al. Polyfunctional T cells accumulate in large human cytomegalovirus-specific T cell responses. J Virol (2012) 86(2):1001–9. doi:10.1128/JVI.00873-11
43. Riddell NE, Griffiths SJ, Rivino L, King DC, Teo GH, Henson SM, et al. Multifunctional cytomegalovirus (CMV)-specific CD8(+) T cells are not restricted by telomere-related senescence in young or old adults. Immunology (2015) 144(4):549–60. doi:10.1111/imm.12409
44. Giménez E, Blanco-Lobo P, Muñoz-Cobo B, Solano C, Amat P, Pérez-Romero P, et al. Role of cytomegalovirus (CMV)-specific polyfunctional CD8+ T-cells and antibodies neutralizing virus epithelial infection in the control of CMV infection in an allogeneic stem-cell transplantation setting. J Gen Virol (2015) 96(9):2822–31. doi:10.1099/vir.0.000203
45. Cush SS, Flaño E. KLRG1+NKG2A+ CD8 T cells mediate protection and participate in memory responses during γ-herpesvirus infection. J Immunol (2011) 186(7):4051–8. doi:10.4049/jimmunol.1003122
46. Vasconcelos JR, Dominguez MR, Araújo AF, Ersching J, Tararam CA, Bruna-Romero O, et al. Relevance of long-lived CD8(+) T effector memory cells for protective immunity elicited by heterologous prime-boost vaccination. Front Immunol (2012) 3:358. doi:10.3389/fimmu.2012.00358
47. Li H, Weng P, Najarro K, Xue QL, Semba RD, Margolick JB, et al. Chronic CMV infection in older women: longitudinal comparisons of CMV DNA in peripheral monocytes, anti-CMV IgG titers, serum IL-6 levels, and CMV pp65 (NLV)-specific CD8(+) T-cell frequencies with twelve-year follow-up. Exp Gerontol (2014) 54:84–9. doi:10.1016/j.exger.2014.01.010
48. Weltevrede M, Eilers R, de Melker HE, van Baarle D. Cytomegalovirus persistence and T-cell immunosenescence in people aged fifty and older: a systematic review. Exp Gerontol (2016) 77:87–95. doi:10.1016/j.exger.2016.02.005
49. Alves NL, Hooibrink B, Arosa FA, van Lier RA. IL-15 induces antigen-independent expansion and differentiation of human naive CD8+ T cells in vitro. Blood (2003) 102(7):2541-6. doi:10.1182/blood-2003-01-0183
50. Chiu WK, Fann M, Weng NP. Generation and growth of CD28nullCD8+ memory T cells mediated by IL-15 and its induced cytokines. J Immunol (2006) 177(11):7802–10. doi:10.4049/jimmunol.177.11.7802
51. Overwijk WW, Schluns KS. Functions of γc cytokines in immune homeostasis: current and potential clinical applications. Clin Immunol (2009) 132(2):153–65. doi:10.1016/j.clim.2009.03.512
52. Correia MP, Costa AV, Uhrberg M, Cardoso EM, Arosa FA. IL-15 induces CD8+ T cells to acquire functional NK receptors capable of modulating cytotoxicity and cytokine secretion. Immunobiology (2011) 216(5):604-12. doi:10.1016/j.imbio.2010.09.012
53. Mueller YM, Petrovas C, Bojczuk PM, Dimitriou ID, Beer B, Silvera P, et al. Interleukin-15 increases effector memory CD8+ t cells and NK Cells in simian immunodeficiency virus-infected macaques. J Virol (2005) 79(8):4877–85. doi:10.1128/JVI.79.8.4877-4885.2005
54. Younes SA, Freeman ML, Mudd JC, Shive CL, Reynaldi A, Panigrahi S, et al. IL-15 promotes activation and expansion of CD8+ T cells in HIV-1 infection. J Clin Invest (2016) 126(7):2745–56. doi:10.1172/JCI85996
55. Van Kaer L. Innate and virtual memory T cells in man. Eur J Immunol (2015) 45(7):1916–20. doi:10.1002/eji.201545761
56. Suzuki A, McCall S, Choi SS, Sicklick JK, Huang J, Qi Y, et al. Interleukin-15 increases hepatic regenerative activity. J Hepatol (2006) 45(3):410–8. doi:10.1016/j.jhep.2006.04.008
57. Nemeth E, Baird AW, O’Farrelly C. Microanatomy of the liver immune system. Semin Immunopathol (2009) 31(3):333–43. doi:10.1007/s00281-009-0173-4
58. Arosa FA, Irwin C, Mayer L, de Sousa M, Posnett DN. Interactions between peripheral blood CD8 T lymphocytes and intestinal epithelial cells (iEC). Clin Exp Immunol (1998) 112(2):226–36. doi:10.1046/j.1365-2249.1998.00566.x
59. Allez M, Brimnes J, Dotan I, Mayer L. Expansion of CD8+ T cells with regulatory function after interaction with intestinal epithelial cells. Gastroenterology (2002) 123(5):1516–26. doi:10.1053/gast.2002.36588
60. Lee-Chang C, Bodogai M, Moritoh K, Olkhanud PB, Chan AC, Croft M, et al. Accumulation of 4-1BBL+ B cells in the elderly induces the generation of granzyme-B+ CD8+ T cells with potential antitumor activity. Blood (2014) 124(9):1450–9. doi:10.1182/blood-2014-03-563940
61. Arosa FA, Oliveira L, Porto G, da Silva BM, Kruijer W, Veltman J, et al. Anomalies of the CD8+ T cell pool in hemochromatosis: HLA-A3-linked expansions of CD8+CD28- T cells. Clin Exp Immunol (1997) 107(3):548–54. doi:10.1046/j.1365-2249.1997.d01-967.x
62. Arosa FA, Porto G, Cabeda JM, Lacerda R, Resende D, Cruz E, et al. Expansions of CD8+CD28- and CD8+TcRVbeta5.2+ T cells in peripheral blood of heavy alcohol drinkers. Alcohol Clin Exp Res (2000) 24(4):519–27. doi:10.1097/00000374-200004000-00027
63. Trzonkowski P, Debska-Slizien A, Myśliwski A, Rutkowski B. Treatment with recombinant human erythropoietin is associated with rejuvenation of CD8+ T cell compartment in chronic renal failure patients. Nephrol Dial Transplant (2007) 22(11):3221–7. doi:10.1093/ndt/gfm361
64. Gharagozloo M, Bagherpour B, Tahanian M, Oreizy F, Amirghofran Z, Sadeghi HM, et al. Premature senescence of T lymphocytes from patients with beta-thalassemia major. Immunol Lett (2009) 122(1):84–8. doi:10.1016/j.imlet.2008.12.003
65. Campbell JP, Riddell NE, Burns VE, Turner M, van Zanten JJ, Drayson MT, et al. Acute exercise mobilises CD8+ T lymphocytes exhibiting an effector-memory phenotype. Brain Behav Immun (2009) 23(6):767–75. doi:10.1016/j.bbi.2009.02.011
66. Zeddou M, Greimers R, de Valensart N, Nayjib B, Tasken K, Boniver J, et al. Prostaglandin E2 induces the expression of functional inhibitory CD94/NKG2A receptors in human CD8+ T lymphocytes by a cAMP-dependent protein kinase A type I pathway. Biochem Pharmacol (2005) 70(5):714–24. doi:10.1016/j.bcp.2005.05.015
67. Ahmadi M, Emery DC, Morgan DJ. Prevention of both direct and cross-priming of antitumor CD8+ T-cell responses following overproduction of prostaglandin E2 by tumor cells in vivo. Cancer Res (2008) 68(18):7520–9. doi:10.1158/0008-5472.CAN-08-1060
68. Chou JP, Ramirez CM, Ryba DM, Koduri MP, Effros RB. Prostaglandin E2 promotes features of replicative senescence in chronically activated human CD8+ T cells. PLoS One (2014) 9(6):e99432. doi:10.1371/journal.pone.0099432
69. Roychoudhuri R, Clever D, Li P, Wakabayashi Y, Quinn KM, Klebanoff CA, et al. BACH2 regulates CD8(+) T cell differentiation by controlling access of AP-1 factors to enhancers. Nat Immunol (2016) 17(7):851–60. doi:10.1038/ni.3441
70. Richer MJ, Lang ML, Butler NS. T cell fates zipped up: how the bach2 basic leucine zipper transcriptional repressor directs T cell differentiation and function. J Immunol (2016) 197(4):1009–15. doi:10.4049/jimmunol.1600847
71. Larbi A, Zelba H, Goldeck D, Pawelec G. Induction of HIF-1 alpha and the glycolytic pathway alters apoptotic and differentiation profiles of activated human T cells. J Leukoc Biol (2010) 87(2):265–73. doi:10.1189/jlb.0509304
72. Doedens AL, Phan AT, Stradner MH, Fujimoto JK, Nguyen JV, Yang E, et al. Hypoxia-inducible factors enhance the effector responses of CD8(+) T cells to persistent antigen. Nat Immunol (2013) 14(11):1173–82. doi:10.1038/ni.2714
73. Lemster BH, Michel JJ, Montag DT, Paat JJ, Studenski SA, Newman AB, et al. Induction of CD56 and TCR-independent activation of T cells with aging. J Immunol (2008) 180(3):1979–90. doi:10.4049/jimmunol.180.3.1979
74. Clementi M, Forabosco P, Amadori A, Zamarchi R, De Silvestro G, Di Gianantonio E, et al. CD4 and CD8 T lymphocyte inheritance. Evidence for major autosomal recessive genes. Hum Genet (1999) 105(4):337–42.
75. Goldman FD, Ballas ZK, Schutte BC, Kemp J, Hollenback C, Noraz N, et al. Defective expression of p56lck in an infant with severe combined immunodeficiency. J Clin Invest (1998) 102(2):421–9. doi:10.1172/JCI3205
76. Kutukculer N, Aksu G, Genel F, Ozturk C. Idiopathic CD4+ T cell lymphocytopenia with the absence of B cells and CD8+28+ cells in peripheral blood. Clin Exp Med (2002) 2(3):143–6. doi:10.1007/s102380200020
77. Hauck F, Randriamampita C, Martin E, Gerart S, Lambert N, Lim A, et al. Primary T-cell immunodeficiency with immunodysregulation caused by autosomal recessive LCK deficiency. J Allergy Clin Immunol (2012) 130(5):1144.e–52.e. doi:10.1016/j.jaci.2012.07.029
78. Vescovini R, Fagnoni FF, Telera AR, Bucci L, Pedrazzoni M, Magalini F, et al. Naïve and memory CD8 T cell pool homeostasis in advanced aging: impact of age and of antigen-specific responses to cytomegalovirus. Age (Dordr) (2014) 36(2):625–40. doi:10.1007/s11357-013-9594-z
79. Arosa FA, da Silva AJ, Godinho IM, ter Steege JC, Porto G, Rudd CE, et al. Decreased CD8-p56lck activity in peripheral blood T-lymphocytes from patients with hereditary haemochromatosis. Scand J Immunol (1994) 39(5):426–32.
80. Tewari K, Walent J, Svaren J, Zamoyska R, Suresh M. Differential requirement for Lck during primary and memory CD8+ T cell responses. Proc Natl Acad Sci U S A (2006) 103(44):16388–93. doi:10.1073/pnas.0602565103
81. Sathaliyawala T, Kubota M, Yudanin N, Turner D, Camp P, Thome JJ, et al. Distribution and compartmentalization of human circulating and tissue-resident memory T cell subsets. Immunity (2013) 38(1):187–97. doi:10.1016/j.immuni.2012.09.020
82. Thome JJ, Yudanin N, Ohmura Y, Kubota M, Grinshpun B, Sathaliyawala T, et al. Spatial map of human T cell compartmentalization and maintenance over decades of life. Cell (2014) 159(4):814–28. doi:10.1016/j.cell.2014.10.026
83. Thome JJ, Bickham KL, Ohmura Y, Kubota M, Matsuoka N, Gordon C, et al. Early-life compartmentalization of human T cell differentiation and regulatory function in mucosal and lymphoid tissues. Nat Med (2016) 22(1):72–7. doi:10.1038/nm.4008
84. Smolders J, Remmerswaal EB, Schuurman KG, Melief J, van Eden CG, van Lier RA, et al. Characteristics of differentiated CD8(+) and CD4 (+) T cells present in the human brain. Acta Neuropathol (2013) 126(4):525–35. doi:10.1007/s00401-013-1155-0
85. Di Rosa F. T-lymphocyte interaction with stromal, bone and hematopoietic cells in the bone marrow. Immunol Cell Biol (2009) 87(1):20–9. doi:10.1038/icb.2008.84
86. Booth JS, Toapanta FR, Salerno-Goncalves R, Patil S, Kader HA, Safta AM, et al. Characterization and functional properties of gastric tissue-resident memory T cells from children, adults, and the elderly. Front Immunol (2014) 5:294. doi:10.3389/fimmu.2014.00294
87. Dutzan N, Konkel JE, Greenwell-Wild T, Moutsopoulos NM. Characterization of the human immune cell network at the gingival barrier. Mucosal Immunol (2016) 9(5):1163–72. doi:10.1038/mi.2015.136
88. Park CO, Kupper TS. The emerging role of resident memory T cells in protective immunity and inflammatory disease. Nat Med (2015) 21(7):688–97. doi:10.1038/nm.3883.82
89. Huang Y, Park Y, Wang-Zhu Y, Larange A, Arens R, Bernardo I, et al. Mucosal memory CD8+ T cells are selected in the periphery by an MHC class I molecule. Nat Immunol (2011) 12(11):1086–95. doi:10.1038/ni.2106
90. Shane HL, Klonowski KD. Every breath you take: the impact of environment on resident memory CD8 T cells in the lung. Front Immunol (2014) 5:320. doi:10.3389/fimmu.2014.00320
91. Wakim LM, Woodward-Davis A, Bevan MJ. Memory T cells persisting within the brain after local infection show functional adaptations to their tissue of residence. Proc Natl Acad Sci U S A (2010) 107(42):17872–9. doi:10.1073/pnas.1010201107
92. Ritzel RM, Crapser J, Patel AR, Verma R, Grenier JM, Chauhan A, et al. Age-associated resident memory CD8 T cells in the central nervous system are primed to potentiate inflammation after ischemic brain injury. J Immunol (2016) 196(8):3318–30. doi:10.4049/jimmunol.1502021
93. Bieber AJ, Kerr S, Rodriguez M. Efficient central nervous system remyelination requires T cells. Ann Neurol (2003) 53(5):680–4. doi:10.1002/ana.10578
94. Shichita T, Ito M, Yoshimura A. Post-ischemic inflammation regulates neural damage and protection. Front Cell Neurosci (2014) 8:319. doi:10.3389/fncel.2014.00319
95. Toulon A, Breton L, Taylor KR, Tenenhaus M, Bhavsar D, Lanigan C, et al. A role for human skin-resident T cells in wound healing. J Exp Med (2009) 206(4):743–50. doi:10.1084/jem.20081787
96. Fu Y, Yang J, Xiong N. Cutting edge: skin CCR10+ CD8+ T cells support resident regulatory T cells through the B7.2/receptor axis to regulate local immune homeostasis and response. J Immunol (2016) 196(12):4859–64. doi:10.4049/jimmunol.1502662
97. Liu Y, Wang L, Kikuiri T, Akiyama K, Chen C, Xu X, et al. Mesenchymal stem cell-based tissue regeneration is governed by recipient T lymphocytes via IFN-γ and TNF-α. Nat Med (2011) 17(12):1594–601. doi:10.1038/nm.2542
98. Schmidt-Bleek K, Schell H, Schulz N, Hoff P, Perka C, Buttgereit F, et al. Inflammatory phase of bone healing initiates the regenerative healing cascade. Cell Tissue Res (2012) 347(3):567–73. doi:10.1007/s00441-011-1205-7
99. Shashkova EV, Trivedi J, Cline-Smith AB, Ferris C, Buchwald ZS, Gibbs J, et al. Osteoclast-primed foxp3+ CD8 T cells induce T-bet, eomesodermin, and IFN-γ to regulate bone resorption. J Immunol (2016) 197(3):726–35. doi:10.4049/jimmunol.1600253
100. Seyda M, Elkhal A, Quante M, Falk CS, Tullius SGT. Cells going innate. Trends Immunol (2016) 37(8):546–56. doi:10.1016/j.it.2016.06.004
101. Mou D, Espinosa J, Lo DJ, Kirk AD. CD28 negative T cells: is their loss our gain? Am J Transplant (2014) 14(11):2460–6. doi:10.1111/ajt.12937
102. Santos SG, Powis SJ, Arosa FA. Misfolding of major histocompatibility complex class I molecules in activated T cells allows cis-interactions with receptors and signaling molecules and is associated with tyrosine phosphorylation. J Biol Chem (2004) 279(51):53062–70. doi:10.1074/jbc.M408794200
103. Santos SG, Antoniou AN, Sampaio P, Powis SJ, Arosa FA. Lack of tyrosine 320 impairs spontaneous endocytosis and enhances release of HLA-B27 molecules. J Immunol (2006) 176(5):2942–9. doi:10.4049/jimmunol.176.5.2942
104. Campbell EC, Antoniou AN, Powis SJ. The multi-faceted nature of HLA class I dimer molecules. Immunology (2012) 136(4):380–4. doi:10.1111/j.1365-2567.2012.03593
105. Bowness P, Caplan S, Edidin M. MHC molecules lead many lives. Workshop on MHC class I molecules at the interface between biology & medicine. EMBO Rep (2009) 10(1):30–4. doi:10.1038/embor.2008.215
106. Saunders PM, Vivian JP, O’Connor GM, Sullivan LC, Pymm P, Rossjohn J, et al. A bird’s eye view of NK cell receptor interactions with their MHC class I ligands. Immunol Rev (2015) 267(1):148–66. doi:10.1111/imr.12319
107. Hudson LE, Allen RL. Leukocyte Ig-like receptors – a model for MHC class I disease associations. Front Immunol (2016) 7:281. doi:10.3389/fimmu.2016.00281
108. Goodridge JP, Burian A, Lee N, Geraghty DE. HLA-F complex without peptide binds to MHC class I protein in the open conformer form. J Immunol (2010) 184(11):6199–208. doi:10.4049/jimmunol.1000078
109. Goodridge JP, Burian A, Lee N, Geraghty DE. HLA-F and MHC class I open conformers are ligands for NK cell Ig-like receptors. J Immunol (2013) 191(7):3553–62. doi:10.4049/jimmunol.1300081
110. Burian A, Wang KL, Finton KA, Lee N, Ishitani A, Strong RK, et al. HLA-F and MHC-I Open conformers bind natural killer cell Ig-like receptor KIR3DS1. PLoS One (2016) 11(9):e0163297. doi:10.1371/journal.pone.0163297
111. Jones DC, Kosmoliaptsis V, Apps R, Lapaque N, Smith I, Kono A, et al. HLA class I allelic sequence and conformation regulate leukocyte Ig-like receptor binding. J Immunol (2011) 186(5):2990–7. doi:10.4049/jimmunol.1003078
112. Zhang Z, Hatano H, Shaw J, Olde Nordkamp M, Jiang G, Li D, et al. The leukocyte immunoglobulin-like receptor family member LILRB5 binds to HLA-class I heavy chains. PLoS One (2015) 10(6):e0129063. doi:10.1371/journal.pone.0129063
113. Hatano H, Shaw J, Marquardt K, Zhang Z, Gauthier L, Chanteux S, et al. The D0 Ig-like domain plays a central role in the stronger binding of KIR3DL2 to B27 free H chain dimers. J Immunol (2015) 194(4):1591–601. doi:10.4049/jimmunol.1402214
114. Garcia-Beltran WF, Hölzemer A, Martrus G, Chung AW, Pacheco Y, Simoneau CR, et al. Open conformers of HLA-F are high-affinity ligands of the activating NK-cell receptor KIR3DS1. Nat Immunol (2016) 17(9):1067–74. doi:10.1038/ni.3513
115. Demaria S, DeVito-Haynes LD, Salter RD, Burlingham WJ, Bushkin Y. Peptide-conformed beta2m-free class I heavy chains are intermediates in generation of soluble HLA by the membrane-bound metalloproteinase. Hum Immunol (1999) 60(12):1216–26. doi:10.1016/S0198-8859(99)00113-5
116. Tsai WC, Chen CJ, Yen JH, Ou TT, Tsai JJ, Liu CS, et al. Free HLA class I heavy chain-carrying monocytes-a potential role in the pathogenesis of spondyloarthropathies. J Rheumatol (2002) 29(5):966–72.
117. Santos SG, Lynch S, Campbell EC, Antoniou AN, Powis SJ. Induction of HLA-B27 heavy chain homodimer formation after activation in dendritic cells. Arthritis Res Ther (2008) 10(4):R100. doi:10.1186/ar2492
118. Rysnik O, McHugh K, van Duivenvoorde L, van Tok M, Guggino G, Taurog J, et al. Non-conventional forms of HLA-B27 are expressed in spondyloarthritis joints and gut tissue. J Autoimmun (2016) 70:12–21. doi:10.1016/j.jaut.2016.03.009
119. Elmer BM, McAllister AK. Major histocompatibility complex class I proteins in brain development and plasticity. Trends Neurosci (2012) 35(11):660–70. doi:10.1016/j.tins.2012.08.001
120. Katz HR. Inhibition of inflammatory responses by leukocyte Ig-like receptors. Adv Immunol (2006) 91:251–72. doi:10.1016/S0065-2776(06)91007-4
121. Podolsky DK. Healing the epithelium: solving the problem from two sides. J Gastroenterol (1997) 32(1):122–6. doi:10.1007/BF01213309
122. Nathan C, Ding A. Nonresolving inflammation. Cell (2010) 140(6):871–82. doi:10.1016/j.cell.2010.02.029
123. Tsai EW, Reed EF. MHC class I signaling: new functional perspectives for an old molecule. Tissue Antigens (2014) 83(6):375–81. doi:10.1111/tan.12381
124. Kono H, Rock KL. How dying cells alert the immune system to danger. Nat Rev Immunol (2008) 8(4):279–89. doi:10.1038/nri2215
125. Hammond T, Lee S, Watson MW, Flexman JP, Cheng W, Fernandez S, et al. Toll-like receptor (TLR) expression on CD4+ and CD8+ T-cells in patients chronically infected with hepatitis C virus. Cell Immunol (2010) 264(2):150–5. doi:10.1016/j.cellimm.2010.06.001
126. Akirav EM, Preston-Hurlburt P, Garyu J, Henegariu O, Clynes R, Schmidt AM, et al. RAGE expression in human T cells: a link between environmental factors and adaptive immune responses. PLoS One (2012) 7(4):e34698. doi:10.1371/journal.pone.0034698
127. Chan WL, Pejnovic N, Lee CA, Al-Ali NA. Human IL-18 receptor and ST2L are stable and selective markers for the respective type 1 and type 2 circulating lymphocytes. J Immunol (2001) 167(3):1238–44. doi:10.4049/jimmunol.167.3.1238
128. Ohta A, Sitkovsky M. Extracellular adenosine-mediated modulation of regulatory T cells. Front Immunol (2014) 5:304. doi:10.3389/fimmu.2014.00304
129. Karaszewski JW, Reder AT, Anlar B, Kim WC, Arnason BG. Increased lymphocyte beta-adrenergic receptor density in progressive multiple sclerosis is specific for the CD8+, CD28- suppressor cell. Ann Neurol (1991) 30(1):42–7. doi:10.1002/ana.410300109
130. Tabiasco J, Devêvre E, Rufer N, Salaun B, Cerottini JC, Speiser D, et al. Human effector CD8+ T lymphocytes express TLR3 as a functional coreceptor. J Immunol (2006) 177(12):8708–13. doi:10.4049/jimmunol.177.12.8708
131. Cottalorda A, Mercier BC, Mbitikon-Kobo FM, Arpin C, Teoh DY, McMichael A, et al. TLR2 engagement on memory CD8(+) T cells improves their cytokine-mediated proliferation and IFN-gamma secretion in the absence of Ag. Eur J Immunol (2009) 39(10):2673–81. doi:10.1002/eji.200939627
132. Freeman CM, Martinez FJ, Han MK, Washko GR Jr, McCubbrey AL, Chensue SW, et al. Lung CD8+ T cells in COPD have increased expression of bacterial TLRs. Respir Res (2013) 14:13. doi:10.1186/1465-9921-14-13
133. Sorci G, Riuzzi F, Giambanco I, Donato R. RAGE in tissue homeostasis, repair and regeneration. Biochim Biophys Acta (2013) 1833(1):101–9. doi:10.1016/j.bbamcr.2012.10.021
134. Ott C, Jacobs K, Haucke E, Navarrete Santos A, Grune T, Simm A. Role of advanced glycation end products in cellular signaling. Redox Biol (2014) 2:411–29. doi:10.1016/j.redox.2013.12.016
135. Imani F, Horii Y, Suthanthiran M, Skolnik EY, Makita Z, Sharma V, et al. Advanced glycosylation endproduct-specific receptors on human and rat T-lymphocytes mediate synthesis of interferon gamma: role in tissue remodeling. J Exp Med (1993) 178(6):2165–72. doi:10.1084/jem.178.6.2165
136. Anderson AC, Joller N, Kuchroo VK. Lag-3, Tim-3, and TIGIT: co-inhibitory receptors with specialized functions in immune regulation. Immunity (2016) 44(5):989–1004. doi:10.1016/j.immuni.2016.05.001
137. Villarreal DO, Weiner DB. Interleukin 33: a switch-hitting cytokine. Curr Opin Immunol (2014) 28:102–6. doi:10.1016/j.coi.2014.03.004
138. Molofsky AB, Savage AK, Locksley RM. Interleukin-33 in tissue homeostasis, injury, and inflammation. Immunity (2015) 42(6):1005–19. doi:10.1016/j.immuni.2015.06.006
139. Lu J, Kang J, Zhang C, Zhang X. The role of IL-33/ST2L signals in the immune cells. Immunol Lett (2015) 164(1):11–7. doi:10.1016/j.imlet.2015.01.008
140. Zaiss DM, Gause WC, Osborne LC, Artis D. Emerging functions of amphiregulin in orchestrating immunity, inflammation, and tissue repair. Immunity (2015) 42(2):216–26. doi:10.1016/j.immuni.2015.01.020
141. Vasanthakumar A, Kallies A. The regulatory T cell: jack-of-all-trades. Trends Immunol (2015) 36(12):756–8. doi:10.1016/j.it.2015.10.002
142. Qi Y, Operario DJ, Georas SN, Mosmann TR. The acute environment, rather than T cell subset pre-commitment, regulates expression of the human T cell cytokine amphiregulin. PLoS One (2012) 7(6):e39072. doi:10.1371/journal.pone.0039072
143. Burnstock G, Boeynaems JM. Purinergic signalling and immune cells. Purinergic Signal (2014) 10(4):529–64. doi:10.1007/s11302-014-9427-2
144. Marino F, Cosentino M. Adrenergic modulation of immune cells: an update. Amino Acids (2013) 45(1):55–71. doi:10.1007/s00726-011-1186-6
Keywords: effector memory CD8+ T cells, NK-like T cells, natural killer receptors, innate receptors, open MHC-I conformers, IL-15, IFN-γ, tissue repair
Citation: Arosa FA, Esgalhado AJ, Padrão CA and Cardoso EM (2017) Divide, Conquer, and Sense: CD8+CD28− T Cells in Perspective. Front. Immunol. 7:665. doi: 10.3389/fimmu.2016.00665
Received: 21 October 2016; Accepted: 16 December 2016;
Published: 03 January 2017
Edited by:
Paulo Vieira, Institut Pasteur de Paris, FranceReviewed by:
Amit Awasthi, Translational Health Science and Technology Institute, IndiaAvery August, Cornell University, USA
Copyright: © 2017 Arosa, Esgalhado, Padrão and Cardoso. This is an open-access article distributed under the terms of the Creative Commons Attribution License (CC BY). The use, distribution or reproduction in other forums is permitted, provided the original author(s) or licensor are credited and that the original publication in this journal is cited, in accordance with accepted academic practice. No use, distribution or reproduction is permitted which does not comply with these terms.
*Correspondence: Fernando A. Arosa, YXJvc2EmI3gwMDA0MDtmY3NhdWRlLnViaS5wdA==