- 1Department of Clinical Immunology of the Third Affiliated Hospital at the Sun Yat-sen University, Guangzhou, China
- 2Division of Rheumatology, Department of Medicine at Penn State Hershey College of Medicine, Hershey, PA, USA
Pro-inflammatory cytokines that are generated by immune system cells and mediate many kinds of immune responses are kinds of endogenous polypeptides. They are also the effectors of the autoimmune system. It is generally accepted that interleukin (IL)-4, IL-6, IL-9, IL-17, and tumor necrosis factor-α are pro-inflammatory cytokines; however, IL-6 becomes a protagonist among them since it predominately induces pro-inflammatory signaling and regulates massive cellular processes. It has been ascertained that IL-6 is associated with a large number of diseases with inflammatory background, such as anemia of chronic diseases, angiogenesis acute-phase response, bone metabolism, cartilage metabolism, and multiple cancers. Despite great progress in the relative field, the targeted regulation of IL-6 response for therapeutic benefits remains incompletely to be understood. Therefore, it is conceivable that understanding mechanisms of IL-6 from the perspective of gene regulation can better facilitate to determine the pathogenesis of the disease, providing more solid scientific basis for clinical treatment translation. In this review, we summarize the candidate genes that have been implicated in clinical target therapy from the perspective of gene transcription regulation.
Introduction
Inflammation is beneficial for pathogen clearance and protection against infection; therefore, pro-inflammatory cytokines are regulators of host responses to infection, inflammation, and trauma, which can also make disease worse in pathological conditions (1, 2). These cytokines at least include interleukin (IL)-1, tumor necrosis factor (TNF), interferon (IFN)-γ, and the IL-6, which is the focus of this review (3). Albeit their biological activities widely overlap, each of them has its own biological properties (4–6). IL-6, which was first identified as an antigen non-specific B-cell differentiation factor, was then named as B-cell stimulatory factor 2. It is a glycoprotein with a molecular weight of 26 kDa (7, 8). Human IL-6 consists of 184 amino acids with 2 potential N-glycosylation sites and four cysteine residues (9).
IL-6 Receptor and Its Signaling Pathway
Interleukin-6 exerts its activity mainly through binding to the cell membrane IL-6 receptor (IL-6R). Cell membrane IL-6R consists of two subunits, IL-6Rα (gp80 or CD126), a 80-kDa type I transmembrane protein, and IL-6Rβ (gp130 or CD130), a 130-kDa second signal transmembrane protein. The soluble IL-6R (sIL-6R), which is cleaved from the cell membrane, can still bind its ligand IL-6 (10–12). The paradigm of IL-6 signal transduction via the membrane bound IL-6R is called “classic signaling.” Conversely, when it signal goes through sIL-6R, it is called “trans-signaling” (13–15). Generally, IL-6 binding with gp80 and gp130 carries on the conduction of biological signal through three pathways (Figure 1).
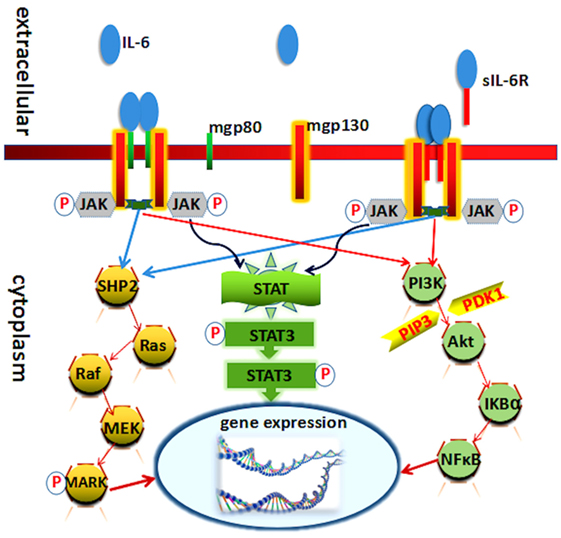
Figure 1. Known molecules involve in interleukin (IL)-6 signal pathway cascades. Schematic representation of the functional organization of IL-6 receptor and its three downstream transduction. IL-6 cytokine yields its biological effects via two receptors: mgp130 (membrane-bound gp130) and mgp80 (membrane-bound gp80). Each receptor can interact with Janus kinase (JAK) directly. The three pathways all needed JAK and its phosphorylation.
Interleukin-6 receptor α (gp80) is mainly expressed on immune cells and therefore immune responses. Recent studies have demonstrated that IL-6 affects the development and balance of Th17 and regulatory T cells, being responsible for the consequence of inflammatory diseases (16). IL-6Rβ is expressed by various cells types, such as lymphocyte, neutrophils, monocytes, macrophages, hepatocytes, affecting immune systems, and others (17, 18).
JAK/STAT Pathway
Interleukin-6 and IL-6R binding initiate the activation of Janus kinase (JAK), one of the tyrosine kinase family members. The activation of these kinases in turn leads to tyrosine phosphorylation and activation of signal transducer and activator of transcription (STAT3) (19, 20). Phosphorylation and activation of these kinases induced by heterodimer/homodimer gp130:gp130 or gp130:leukemia inhibitory factor receptor (ILFR) result in the phosphorylation of six tyrosine residues on the gp130 and ILFR. Following phosphorylation, a variety of molecules at the SH-2 domain were upregulated, such as SHP-2, Shc, and STATs. STAT3 then forms a dimer to transmit signals from the cell membrane to the nucleus (19, 21). The IL-6/JAK/STAT3 canonical pathway regulates the expression of several genes leading to the induction of cell growth differentiation and survival (22).
Ras/Mitogen-Activated Protein Kinases (MAPK) Pathway
Ras protein is also activated in response to IL-6 that involves in the formation and the activation of complex compounds:Grb2 (growth factor receptor-binding protein) and Shc (SH2 and collagen homology domain containing protein) (23). Subsequently, it activates downstream signaling of MAPK and leads to an increase in its serine/threonine kinase activity. Several substrates involve in the MAPK phosphorylation actions, like c-Myc, c-Jun, and c-Fos. It mediates diverse effects including cell growth stimulation acute-phase protein synthesis and immunoglobulin synthesis (24, 25).
Phosphoinositol-3 Kinase (PI3K)/Akt Pathway
The PI3K–protein kinase B (PkB)/Akt pathway also plays an indispensable role in the transduction of IL-6 signal, especially in the antiapoptotic effect of IL-6 in prostate cancer cells. PI3K protein modifies certain phosphatidylinositides in phosphorylate phosphatidylinositol-4,5-bisphosphate to phosphatidylinositol-3,4,5-trisphosphate (PIP3). PIP3 in turn phosphorylates and activates serine/threonine kinase PkB/Akt which is recruited to the plasma membrane. Akt can be activated by phosphoinositide-dependent kinase-1 through phosphorylation and then phosphorylates several downstream targets to upregulate cellular survival signaling pathways (24, 26).
Cis-Acting Element
The corresponding human gene of IL-6 including three transcription start sites and three TATA like sequences (TATA boxes) is localized on chromosome 7p21 and consists of five exons and four introns (27). Several of its cis-acting elements are located in a 1.2-kb fragment of the 5′-flanking region (28, 29) (Figure 2).
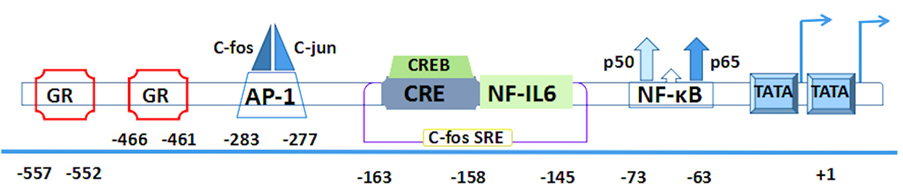
Figure 2. The human interleukin-6 gene prompter with putative cis-regulatory elements and approximate binding site relative to trans-regulatory factors.
First two pairs of glucocorticoid response elements (GREs) are located at the positions –557 to –552 and –466 to –461 in the human IL-6 gene (28). Then comes the activator protein 1 (AP-1)-binding site. It is a consensus sequence (TGAGTCA) found at the position –283 to –277 (30), which is present in a number of phorbol-12-myristate-13-acetate (PMA)-inducible promoters and confers inducibility to heterologous promoters (28–31). Interestingly, a similar structure is also found at the –55 to the –61 region (TGAGTCT) and continues to extend a sequence with high similarity to the human c-fos SRE that is present at the position –169 to –124 (32). Within the c-fos SRE homology, it contains an essential sequence (ACGTCA) of the cyclic AMP (cAMP)-response element (CRE). This CRE is also called multiple response element due to the characteristic that can be induced by serum, TPA, and Forskolin (33). Meanwhile, it is able to combine with cAMP-TPA-induced binding protein, namely, CRE-binding protein (CREB) (34, 35). Furthermore, this region contains not only a CRE motif but also the upper half of the 14bp NF-IL6-binding sequence that is located at the -163 to -145 (36, 37). All of these elements are conserved between human and mouse genes (38).
Both of the AP-1 and CRE sites have a high degree of sequence similarity because they have only a difference on two nucleotides (39). Downstream of the c-fos SRE, the presence of a putative binding site is located on the nuclear factor (NF)-κB transcription factor between -75 and -64 (30), which plays a crucial role in immunoglobulin κ gene expression (40). In addition to the aforementioned important regulatory sites, RBP (also designated CBF1) is a negative regulator that can downregulate expression of the NF-κB responsive IL-6 gene. It binds within the interleukin response element and hinders NF-κB coactivation with C/EBP-β. Its repression depends on the presence and position of the RBP target site within the IL-6 promoter (41). The CpG oligonucleotides on human IL-6 gene transcription reveal another negative regulator, retinoblastoma control element (RCE), which can be dissociated from the 3′-RCE binding site to enable the engagement of the C/EBP-β enhancer (42).
Trans-Acting Factors
There are many trans-regulators that have been specifically identified and incorporated into the corresponding cis-regulatory elements so far. This incorporation plays a vital role on controlling the sequence of inflammatory response.
Nuclear Factor-κB
Nuclear factor-κB is a pleiotropic transcription factor, whose main function is to direct transcriptional activity of various promoters of pro-inflammatory cytokines, especially for triggering IL-6 gene (43, 44). By using electrophoretic mobility shift assays, one can clearly observe that NF-κB binds specifically to the wild-type IL-6 promoter but not to the mutants. Libermann and Baltimore reported that the NF-κB-binding site is essential for the response of the IL-6 promoter to IL-1 and to TNF-α (45). It is a heterodimer consisting of two subunits: p50 and p65. p65 is the most frequent component of activated NF-κB and combines with high affinity to the consensus DNA sequences 5-GGG PuN NPy PyC C-3′(p65/p50) or 5′-GGG PuN PyP yCC-3′(p65/c-Rel) leading to the activation of transcription (46). NF-κB complexes were found inactive in the cells without stimulation and inhibited by a class of proteins called IκBa. Phosphorylation of the IκB is extremely responsible for dissociation of the inactive NF-κB dimers, which makes the latter translocate into the nucleus and binds to the IL-6 DNA (47). Furthermore, Palaga et al. confirmed that NF-κB directly involves in the positive regulation IL-6 gene by Notch1 signal in activated macrophages (48).
There are two major NF-κB activating signal transduction pathways. The classical pathway, which is initiated by pro-inflammatory cytokines, such as TNF-α binds to specific receptor causing the sequential recruitment of various stimulation factors, which makes the rapid phosphorylation of IκBa and dissociation from the NF-κB (49, 50). Another signaling pathway is a much slower process mediated by the NF-κB-inducible kinase. It leads to the phosphorylation of IKKα and the dimerization of p52 subunit and then follows through the classical pathway (49–51). NF-κB significantly mediates the activation of the IL-6 gene by a variety of IL-6 inducers such as PMA, LPS, TNF-α, poly(IC), and human T lymphotropic virus type I (52, 53). Nonetheless, the function is cell specific including U-937 monocytic cells, HeLa cells, and Jurkat T cells (49, 54–57). Meanwhile these inducible enhancer elements probably contribute to IL-6 gene induction in a cell-specific manner (45, 58).
NF-IL6 and Its C/EBP Familty
NF-IL6, also known as C/EBP-β, was identified in 1990 as it can bind to a 14-bp palindromic sequence (ACATTGCACAATCT) within an IL-1 responsive element in the human IL-6 gene (59, 60). NF-IL6 expresses at an undetectable level in normal tissues but is obviously induced following the stimulation with LPS, IL-1, TNF-α, or IL-6 (52). It regulates a variety of genes involved in inflammatory cytokine (36). NF-IL6 contains a potential leucine zipper structure, which is highly homologous to a liver-specific transcriptional factor (30, 61).
The activity of NF-IL6 is regulated by several ways through its phosphorylation. First is the Thr-235 phosphorylated residue, second is within the leucine zipper, and third is the cAMP-mediated phosphorylation that can be associated with nuclear translocation and gene transcription (62, 63). NF-IL6 also cooperates with other transcriptional factors, playing a synergistic role in regulating the IL-6 gene expression. For example, p65, a subunit of NF-κB, results in the synergistic effect although this synergistic function comes to work only when NF-IL6 binds to its binding site of IL-6 promoter region (64, 65). In human intestinal epithelial cells, C/EBP-β (NF-IL6) may regulate IL-6 production through MAPK pathway. For example, when MAPK inhibitor (PD-98059) was used, it markedly attenuated the IL-6 mRNA and protein level, and this suppression is paralleled with the concentrations of PD-98059. In addition, this inhibition could involve in other transcription factors that can be influenced by MAPK signal pathways including AP-1 and NF-κB (65, 66). Although C/EBP interacts with NF-κB synergistically, the function of C/EBP on the IL-6 gene expression is diminutive without the NF-κB (67). Furthermore, C/EBP homologous protein (CHOP) upregulates IL-6 promoter activity at the transcriptional level with a manner dependent on the leucine zipper domain (68). Moreover, in the human melanoma cell line A375, CHOP raises the IL-6 production without binding to its promoter but trapping protein(s) such as liver-enriched inhibitory protein, an isoform of NF-IL6, which would otherwise inhibit IL-6 transcription (69). Within C/EBP family members, C/EBPζ (C/EBP heterodimers) acts as a negative regulator of IL-6 expression in B cells. Ectopic expression of C/EBPζ inhibited C/EBPζ-dependent IL-6 expression from both the endogenous IL-6 gene and the IL-6 promoter-reporter, while others such as C/EBPα, β, δ, and ϵ reveal the capacity of conferring LPS-inducible IL-6 transcription to P388 B lymphoblast, which is murine B lymphoblastic cell line (70, 71).
Activator Protein 1
The AP-1, which belongs to the class of basic leucine zipper transcription factors, has homodimeric and heterodimeric complexes. AP-1-binding sequence (5′TGAG/CTCA3′), also known as the TPA response element, mediates the regulatory transcription of target genes (72, 73). AP-1 can bind to the specific binding site of the IL-6 promoter, exerting a significant effect on the regulation of IL-6 gene expression (74, 75). Combination of AP-1 with other transcriptional factors such as NF-κB and cAMP may have a synergistic role in transcriptional regulation of IL-6 gene in thyroid FRTL-5 cells, and the synergistic effects of TSH and cAMP on IL-6 secretion stimulated by IL-1 mainly involves the AP-1-binding site and an increase in the expression of its subunits: c-Fos and Fra-2 transcription factors (76). In IM9 cells, IL6-AP-1 is the most important cis-regulatory combination compared to other IL-6 promoter region: IL6–NF-κB, IL6-C/EBP, and IL6-CREB (77). However, there is a controversial observation on regulatory sites using IL-6 autocrine human prostate cancer cells. Xiao et al. showed that the cooperation between NF-κB and C/EBP-b is important for constitutively activated IL-6 promoter activity, while another study implicated the cooperation between NF-κB and AP-1 to be crucial (77, 78). Thus, the regulation of IL-6 gene expression is complex, and the AP-1 activation also works at a multilevel.
Sp-1
Sp-1 is a ubiquitous transcription factor, which belongs to the Sp/XKLF family (79). Sp-1 binding site lies between the NF-IL6 and NF-κB enhancer, which is a G/C-rich sequence containing three repeats of the element CCACC in IL-6 gene (80). It is considered as an important bridge in binding between NF-κB and C/EBP isoforms in the IL-6 promoter (80). Sp-1 and NF-κB were demonstrated to have a positive cooperation in regulation of the human immunodeficiency virus promoter (81). In addition, Sp-1 may facilitate these interactions in IL-6 promoter for rapid response to inflammatory stimuli (82–84).
Interferon Regulatory Factor (IRF)
Interferon regulatory factor could be one of other regulatory factors. By using the transient transfection of the chloramphenicol acetyltransferase (CAT) reporter gene linked to the IL-6 promoter to analyze the function of the 5′-flanking region of the IL-6 gene, it has demonstrated that IRF-binding site at position -267 to -254 is essential for induction of IL-6 gene expression following stimulation by IFN-γ. Transient transfection assays in HeLa cells demonstrated that the co-operation between IRF-1 and NF-κB at a low constitutive level is required for the comprehensive transcriptional activation of the IL-6 promoter directing CAT expression (85). IRF7 positively regulates IL-6 gene expression via enhancing IL-6 mRNA stability (86).
Estrogen Receptor, Androgen Receptor, and Glucocorticoid
Apart from the positive transcriptional factors, some are also potent repressors involving in IL-6 gene expression. Steroid hormones, important regulators of physiological homeostasis, play a role on endogenous IL-6 expression.
Glucocorticoid classical function is mostly based on binding of a glucocorticoid receptor (GR) dimer to GREs in the regulatory regions of target genes including IL-6 (87). GRα, the pivotal subunit of GR, can repress pro-inflammatory gene by directly binding to a negative GRE, which involves the interactions between GRα and other transcription factors, particularly AP-1 and NF-κB (88, 89). P65 interacts with the GR and leads to mutual transcriptional antagonism in various studies in vitro. The interaction involves the DNA-binding domain of GR and the Rel homology domain of p65 (90). However, the exact mechanism is still controversial. It has been evident that p65 subunit of NF-κB and GR are physically interact. Physiological antagonism between two cytokines is based on a mutual transcriptional antagonism. On the other hand, others considered that NF-κB dissociation from DNA is not a requirement (87). Glucocorticoids may also repress NF-κB activity through induction of the NF-κB inhibitor IκB. Moreover, GR-mediated trans-repression is also through the direct protein–protein interactions between GRα and the c-Jun subunits (91).
Estrogens employ estrogen receptor to negatively regulate IL-6 gene expression via inhibition of the DNA-binding activities of the transcription factors NF-IL6 and NF-κB, as well as disruption of NF-κB transactivation (92, 93). Androgens also inhibit IL-6 gene expression via NF-κB. Using a prostate cancer cell line, dihydrotestosterone confirmed that it requires the androgen receptor to inhibit IL-6 gene promoter (94).
Single-Nucleotide Polymorphism (SNP)
Polymorphisms in the promoter of IL-6 gene can result in interindividual variation in transcription and expression influencing an individual’s susceptibility to a diverse range of diseases (95). SNPs also play an important role in IL-6 gene expression that is related to common DNA sequence variations among individuals and associated to several human diseases. Up-to-date, four major polymorphisms of IL-6 have been identified where its polymorphism site is located at positions -572 G/C, -597 G/A, -1363 G/T, and -2954 G/C. These sites have a cooperative impact on the IL-6 gene transcription (96–98). A single nucleotide change from G to C at position -174 in the IL-6 promoter influences its transcription rate and is related to several diseases such as polycystic ovary syndrome (98–100). The – 174G/C SNP maps to a negative regulatory domain (−225 to −164), which approaches the CRE. Moreover, it is contained within a sequence bearing partial nucleotide homology with the Smad4-binding element and the “C” allele may bind Smad4 more effectively and thereby inhibit IL-6 transcription (100).
Conclusion
Interleukin-6 acts as either a pro-inflammatory cytokine or an anti-inflammatory cytokine. Its role ranges from stimulating immune response, fighting infection, and responding to specific microbial molecules to changing the body’s temperature set-point, stimulating osteoclast formation, assisting hybridoma growth, as well as affecting muscle contraction. Both its upstream and downstream signaling pathways differ obviously between myocytes and macrophages, which involve in several pivotal protein molecules. IL-6 triggers its receptors CD130 and CD126 proteins to form a complex, thus initiating a signal transduction cascade through certain transcription factors like JAKs and STATs. Although IL-6 and its receptor are well acknowledged as a potential and vital target for the treatment of many diseases, there is still a large gap between cognization and utilization. Targeting the specific downstream molecular pathway may have the better therapeutic efficacy on inflammatory and autoimmune diseases.
Author Contributions
YL wrote draft of MS and SZ edited and revised MS.
Conflict of Interest Statement
The authors declare that the research was conducted in the absence of any commercial or financial relationships that could be construed as a potential conflict of interest.
Funding
This work was partially supported by NIH R01 AR059103, NIH Star Award and NSFC grant (81671611), Natural Science Foundation of Guangdong Province (Grant No. 2014A030308005), Science and Technology Program of Guangzhou, China (201508020060).
References
2. Gao Y, Tang J, Chen W, Li Q, Nie J, Lin F, et al. Inflammation negatively regulates FOXP3 and regulatory T-cell function via DBC1. Proc Natl Acad Sci U S A (2015) 112:E3246–54. doi:10.1073/pnas.1421463112
3. Morita H, Moro K, Koyasu S. Innate lymphoid cells in allergic and nonallergic inflammation. J Allergy Clin Immunol (2016) 138:1253–64. doi:10.1016/j.jaci.2016.09.011
4. Akira S, Hirano T, Taga T, Kishimoto T. Biology of multifunctional cytokines: IL 6 and related molecules (IL 1 and TNF). FASEB J (1990) 4:2860–7.
5. Akira S, Taga T, Kishimoto T. Interleukin-6 in biology and medicine. Adv Immunol (1993) 54:1–78. doi:10.1016/S0065-2776(08)60532-5
6. Goujon E, Parnet P, Laye S, Combe C, Dantzer R. Adrenalectomy enhances pro-inflammatory cytokines gene expression, in the spleen, pituitary and brain of mice in response to lipopolysaccharide. Brain Res Mol Brain Res (1996) 36:53–62. doi:10.1016/0169-328X(95)00242-K
7. Muraguchi A, Kishimoto T, Miki Y, Kuritani T, Kaieda T, Yoshizaki K, et al. T cell-replacing factor- (TRF) induced IgG secretion in a human B blastoid cell line and demonstration of acceptors for TRF. J Immunol (1981) 127:412–6.
8. Yoshizaki K, Nakagawa T, Kaieda T, Muraguchi A, Yamamura Y, Kishimoto T. Induction of proliferation and Ig production in human B leukemic cells by anti-immunoglobulins and T cell factors. J Immunol (1982) 128:1296–301.
9. Tanaka T, Kishimoto T. The biology and medical implications of interleukin-6. Cancer Immunol Res (2014) 2:288–94. doi:10.1158/2326-6066.CIR-14-0022
10. Mullberg J, Schooltink H, Stoyan T, Gunther M, Graeve L, Buse G, et al. The soluble interleukin-6 receptor is generated by shedding. Eur J Immunol (1993) 23:473–80. doi:10.1002/eji.1830230226
11. Taga T, Hibi M, Hirata Y, Yamasaki K, Yasukawa K, Matsuda T, et al. Interleukin-6 triggers the association of its receptor with a possible signal transducer, gp130. Cell (1989) 58:573–81. doi:10.1016/0092-8674(89)90438-8
12. Yamasaki K, Taga T, Hirata Y, Yawata H, Kawanishi Y, Seed B, et al. Cloning and expression of the human interleukin-6 (BSF-2/IFN beta 2) receptor. Science (1988) 241:825–8. doi:10.1126/science.3136546
13. Garbers C, Hermanns HM, Schaper F, Muller-Newen G, Grotzinger J, Rose-John S, et al. Plasticity and cross-talk of interleukin 6-type cytokines. Cytokine Growth Factor Rev (2012) 23:85–97. doi:10.1016/j.cytogfr.2012.04.001
14. Kallinich T. Regulating against the dysregulation: new treatment options in autoinflammation. Semin Immunopathol (2015) 37:429–37. doi:10.1007/s00281-015-0501-9
15. Rose-John S, Heinrich PC. Soluble receptors for cytokines and growth factors: generation and biological function. Biochem J (1994) 300(Pt 2):281–90. doi:10.1042/bj3000281
16. Zheng SG, Wang J, Horwitz DA. Cutting edge: Foxp3+CD4+CD25+ regulatory T cells induced by IL-2 and TGF-beta are resistant to Th17 conversion by IL-6. J Immunol (2008) 180:7112–6. doi:10.4049/jimmunol.180.11.7112
17. Rincon M. Interleukin-6: from an inflammatory marker to a target for inflammatory diseases. Trends Immunol (2012) 33:571–7. doi:10.1016/j.it.2012.07.003
18. Zhang Q, Zhao K, Shen Q, Han Y, Gu Y, Li X, et al. Tet2 is required to resolve inflammation by recruiting Hdac2 to specifically repress IL-6. Nature (2015) 525:389–93. doi:10.1038/nature15252
19. Guschin D, Rogers N, Briscoe J, Witthuhn B, Watling D, Horn F, et al. A major role for the protein tyrosine kinase JAK1 in the JAK/STAT signal transduction pathway in response to interleukin-6. EMBO J (1995) 14:1421–9.
20. Wang S, Zhang W. Genetic variants in IL-6/JAK/STAT3 pathway and the risk of CRC. Tumour Biol (2016) 37:6561–9. doi:10.1007/s13277-015-4529-1
21. Heinrich PC, Behrmann I, Haan S, Hermanns HM, Muller-Newen G, Schaper F. Principles of interleukin (IL)-6-type cytokine signalling and its regulation. Biochem J (2003) 374:1–20. doi:10.1042/bj20030407
22. Garbers C, Aparicio-Siegmund S, Rose-John S. The IL-6/gp130/STAT3 signaling axis: recent advances towards specific inhibition. Curr Opin Immunol (2015) 34:75–82. doi:10.1016/j.coi.2015.02.008
23. Ancrile BB, O’Hayer KM, Counter CM. Oncogenic ras-induced expression of cytokines: a new target of anti-cancer therapeutics. Mol Interv (2008) 8:22–7. doi:10.1124/mi.8.1.6
24. Ataie-Kachoie P, Pourgholami MH, Morris DL. Inhibition of the IL-6 signaling pathway: a strategy to combat chronic inflammatory diseases and cancer. Cytokine Growth Factor Rev (2013) 24:163–73. doi:10.1016/j.cytogfr.2012.09.001
25. Nakajima T, Kinoshita S, Sasagawa T, Sasaki K, Naruto M, Kishimoto T, et al. Phosphorylation at threonine-235 by a ras-dependent mitogen-activated protein kinase cascade is essential for transcription factor NF-IL6. Proc Natl Acad Sci U S A (1993) 90:2207–11. doi:10.1073/pnas.90.6.2207
26. Hennessy BT, Smith DL, Ram PT, Lu Y, Mills GB. Exploiting the PI3K/AKT pathway for cancer drug discovery. Nat Rev Drug Discov (2005) 4:988–1004. doi:10.1038/nrd1902
27. Sehgal PB, Zilberstein A, Ruggieri RM, May LT, Ferguson-Smith A, Slate DL, et al. Human chromosome 7 carries the beta 2 interferon gene. Proc Natl Acad Sci U S A (1986) 83:5219–22. doi:10.1073/pnas.83.14.5219
28. Isshiki H, Akira S, Tanabe O, Nakajima T, Shimamoto T, Hirano T, et al. Constitutive and interleukin-1 (IL-1)-inducible factors interact with the IL-1-responsive element in the IL-6 gene. Mol Cell Biol (1990) 10:2757–64. doi:10.1128/MCB.10.6.2757
29. Zhang Y, Lin JX, Vilcek J. Synthesis of interleukin 6 (interferon-beta 2/B cell stimulatory factor 2) in human fibroblasts is triggered by an increase in intracellular cyclic AMP. J Biol Chem (1988) 263:6177–82.
30. Edbrooke MR, Burt DW, Cheshire JK, Woo P. Identification of cis-acting sequences responsible for phorbol ester induction of human serum amyloid A gene expression via a nuclear factor kappaB-like transcription factor. Mol Cell Biol (1989) 9:1908–16. doi:10.1128/MCB.9.5.1908
31. Angel P, Imagawa M, Chiu R, Stein B, Imbra RJ, Rahmsdorf HJ, et al. Phorbol ester-inducible genes contain a common cis element recognized by a TPA-modulated trans-acting factor. Cell (1987) 49:729–39. doi:10.1016/0092-8674(87)90611-8
32. Walther Z, May LT, Sehgal PB. Transcriptional regulation of the interferon-beta 2/B cell differentiation factor BSF-2/hepatocyte-stimulating factor gene in human fibroblasts by other cytokines. J Immunol (1988) 140:974–7.
33. Montminy MR, Sevarino KA, Wagner JA, Mandel G, Goodman RH. Identification of a cyclic-AMP-responsive element within the rat somatostatin gene. Proc Natl Acad Sci U S A (1986) 83:6682–6. doi:10.1073/pnas.83.18.6682
34. Dendorfer U, Oettgen P, Libermann TA. Multiple regulatory elements in the interleukin-6 gene mediate induction by prostaglandins, cyclic AMP, and lipopolysaccharide. Mol Cell Biol (1994) 14:4443–54. doi:10.1128/MCB.14.7.4443
35. Sehgal PB. Regulation of IL6 gene expression. Res Immunol (1992) 143:724–34. doi:10.1016/0923-2494(92)80011-9
36. Akira S, Isshiki H, Nakajima T, Kinoshita S, Nishio Y, Natsuka S, et al. Regulation of expression of the interleukin 6 gene: structure and function of the transcription factor NF-IL6. Ciba Found Symp (1992) 167:47–62; discussion 62–47.
37. Ray A, Sassone-Corsi P, Sehgal PB. A multiple cytokine- and second messenger-responsive element in the enhancer of the human interleukin-6 gene: similarities with c-fos gene regulation. Mol Cell Biol (1989) 9:5537–47. doi:10.1128/MCB.9.12.5537
38. Tanabe O, Akira S, Kamiya T, Wong GG, Hirano T, Kishimoto T. Genomic structure of the murine IL-6 gene. High degree conservation of potential regulatory sequences between mouse and human. J Immunol (1988) 141:3875–81.
39. Grassl C, Luckow B, Schlondorff D, Dendorfer U. Transcriptional regulation of the interleukin-6 gene in mesangial cells. J Am Soc Nephrol (1999) 10:1466–77.
40. Ray A, Tatter SB, May LT, Sehgal PB. Activation of the human “beta 2-interferon/hepatocyte-stimulating factor/interleukin 6” promoter by cytokines, viruses, and second messenger agonists. Proc Natl Acad Sci U S A (1988) 85:6701–5. doi:10.1073/pnas.85.18.6701
41. Kannabiran C, Zeng X, Vales LD. The mammalian transcriptional repressor RBP (CBF1) regulates interleukin-6 gene expression. Mol Cell Biol (1997) 17:1–9. doi:10.1128/MCB.17.1.1
42. Takeshita F, Ishii KJ, Ueda A, Ishigatsubo Y, Klinman DM. Positive and negative regulatory elements contribute to CpG oligonucleotide-mediated regulation of human IL-6 gene expression. Eur J Immunol (2000) 30:108–16. doi:10.1002/1521-4141(200001)30:1<108::AID-IMMU108>3.3.CO;2-W
43. Ndlovu MN, Van Lint C, Van Wesemael K, Callebert P, Chalbos D, Haegeman G, et al. Hyperactivated NF-{kappa}B and AP-1 transcription factors promote highly accessible chromatin and constitutive transcription across the interleukin-6 gene promoter in metastatic breast cancer cells. Mol Cell Biol (2009) 29:5488–504. doi:10.1128/MCB.01657-08
44. Siebenlist U, Franzoso G, Brown K. Structure, regulation and function of NF-kappa B. Annu Rev Cell Biol (1994) 10:405–55. doi:10.1146/annurev.cb.10.110194.002201
45. Libermann TA, Baltimore D. Activation of interleukin-6 gene expression through the NF-kappa B transcription factor. Mol Cell Biol (1990) 10:2327–34. doi:10.1128/MCB.10.5.2327
46. Barnes PJ. Nuclear factor-kappa B. Int J Biochem Cell Biol (1997) 29:867–70. doi:10.1016/S1357-2725(96)00159-8
47. DiDonato JA, Mercurio F, Karin M. NF-kappaB and the link between inflammation and cancer. Immunol Rev (2012) 246:379–400. doi:10.1111/j.1600-065X.2012.01099.x
48. Palaga T, Ratanabunyong S, Pattarakankul T, Sangphech N, Wongchana W, Hadae Y, et al. Notch signaling regulates expression of Mcl-1 and apoptosis in PPD-treated macrophages. Cell Mol Immunol (2013) 10:444–52. doi:10.1038/cmi.2013.22
49. Sen R, Baltimore D. Inducibility of kappa immunoglobulin enhancer-binding protein Nf-kappa B by a posttranslational mechanism. Cell (1986) 47:921–8. doi:10.1016/0092-8674(86)90807-X
50. Viatour P, Merville MP, Bours V, Chariot A. Phosphorylation of NF-kappaB and IkappaB proteins: implications in cancer and inflammation. Trends Biochem Sci (2005) 30:43–52. doi:10.1016/j.tibs.2004.11.009
51. Tapia MA, Gonzalez-Navarrete I, Dalmases A, Bosch M, Rodriguez-Fanjul V, Rolfe M, et al. Inhibition of the canonical IKK/NF kappa B pathway sensitizes human cancer cells to doxorubicin. Cell Cycle (2007) 6:2284–92. doi:10.4161/cc.6.18.4721
52. Neurath MF, Finotto S. IL-6 signaling in autoimmunity, chronic inflammation and inflammation-associated cancer. Cytokine Growth Factor Rev (2011) 22:83–9. doi:10.1016/j.cytogfr.2011.02.003
53. Zineldeen DH, Uranishi H, Okamoto T. NF-kappa B signature on the aging wall. Curr Drug Metab (2010) 11:266–75. doi:10.2174/138920010791196283
54. Baeuerle PA, Baltimore D. Activation of DNA-binding activity in an apparently cytoplasmic precursor of the NF-kappa B transcription factor. Cell (1988) 53:211–7. doi:10.1016/0092-8674(88)90382-0
55. Baeuerle PA, Lenardo M, Pierce JW, Baltimore D. Phorbol-ester-induced activation of the NF-kappa B transcription factor involves dissociation of an apparently cytoplasmic NF-kappa B/inhibitor complex. Cold Spring Harb Symp Quant Biol (1988) 53(Pt 2):789–98. doi:10.1101/SQB.1988.053.01.089
56. Ballard DW, Bohnlein E, Lowenthal JW, Wano Y, Franza BR, Greene WC. HTLV-I tax induces cellular proteins that activate the kappa B element in the IL-2 receptor alpha gene. Science (1988) 241:1652–5. doi:10.1126/science.2843985
57. Lowenthal JW, Ballard DW, Bohnlein E, Greene WC. Tumor necrosis factor alpha induces proteins that bind specifically to kappa B-like enhancer elements and regulate interleukin 2 receptor alpha-chain gene expression in primary human T lymphocytes. Proc Natl Acad Sci U S A (1989) 86:2331–5. doi:10.1073/pnas.86.7.2331
58. Lenardo M, Pierce JW, Baltimore D. Protein-binding sites in Ig gene enhancers determine transcriptional activity and inducibility. Science (1987) 236:1573–7. doi:10.1126/science.3109035
59. Akira S, Isshiki H, Sugita T, Tanabe O, Kinoshita S, Nishio Y, et al. A nuclear factor for IL-6 expression (NF-IL6) is a member of a C/EBP family. EMBO J (1990) 9:1897–906.
60. Rummel C. Inflammatory transcription factors as activation markers and functional readouts in immune-to-brain communication. Brain Behav Immun (2016) 54:1–14. doi:10.1016/j.bbi.2015.09.003
61. Landschulz WH, Johnson PF, McKnight SL. The leucine zipper: a hypothetical structure common to a new class of DNA binding proteins. Science (1988) 240:1759–64. doi:10.1126/science.3289117
62. Metz R, Ziff E. cAMP stimulates the C/EBP-related transcription factor rNFIL-6 to trans-locate to the nucleus and induce c-fos transcription. Genes Dev (1991) 5:1754–66. doi:10.1101/gad.5.10.1754
63. Peant B, Gilbert S, Le Page C, Poisson A, L’Ecuyer E, Boudhraa Z, et al. IkappaB-Kinase-epsilon (IKKepsilon) over-expression promotes the growth of prostate cancer through the C/EBP-beta dependent activation of IL-6 gene expression. Oncotarget (2016). doi:10.18632/oncotarget.11629
64. Akira S, Kishimoto T. NF-IL6 and NF-kappa B in cytokine gene regulation. Adv Immunol (1997) 65:1–46. doi:10.1016/S0065-2776(08)60740-3
65. Kishimoto T. Interleukin-6: from basic science to medicine – 40 years in immunology. Annu Rev Immunol (2005) 23:1–21. doi:10.1146/annurev.immunol.23.021704.115806
66. Hungness ES, Luo GJ, Pritts TA, Sun X, Robb BW, Hershko D, et al. Transcription factors C/EBP-beta and -delta regulate IL-6 production in IL-1beta-stimulated human enterocytes. J Cell Physiol (2002) 192:64–70. doi:10.1002/jcp.10116
67. Matsusaka T, Fujikawa K, Nishio Y, Mukaida N, Matsushima K, Kishimoto T, et al. Transcription factors NF-IL6 and NF-kappa B synergistically activate transcription of the inflammatory cytokines, interleukin 6 and interleukin 8. Proc Natl Acad Sci U S A (1993) 90:10193–7. doi:10.1073/pnas.90.21.10193
68. Hattori T, Itoh S, Hayashi H, Chiba T, Takii T, Yoshizaki K, et al. CHOP, a basic leucine zipper transcriptional factor, contributes to the antiproliferative effect of IL-1 on A375 human melanoma cells through augmenting transcription of IL-6. J Interferon Cytokine Res (2001) 21:323–32. doi:10.1089/107999001300177510
69. Hattori T, Ohoka N, Hayashi H, Onozaki K. C/EBP homologous protein (CHOP) up-regulates IL-6 transcription by trapping negative regulating NF-IL6 isoform. FEBS Lett (2003) 541:33–9. doi:10.1016/S0014-5793(03)00283-7
70. Gao H, Parkin S, Johnson PF, Schwartz RC. C/EBP gamma has a stimulatory role on the IL-6 and IL-8 promoters. J Biol Chem (2002) 277:38827–37. doi:10.1074/jbc.M206224200
71. Tsukada J, Yoshida Y, Kominato Y, Auron PE. The CCAAT/enhancer (C/EBP) family of basic-leucine zipper (bZIP) transcription factors is a multifaceted highly-regulated system for gene regulation. Cytokine (2011) 54:6–19. doi:10.1016/j.cyto.2010.12.019
72. Adcock IM. Transcription factors as activators of gene transcription: AP-1 and NF-κB. Monaldi Arch Chest Dis (1997) 52:178–86.
73. Hsu CK, Lee IT, Lin CC, Hsiao LD, Yang CM. Sphingosine-1-phosphate mediates COX-2 expression and PGE2/IL-6 secretion via c-Src-dependent AP-1 activation. J Cell Physiol (2015) 230:702–15. doi:10.1002/jcp.24795
74. Wagner EF. Bone development and inflammatory disease is regulated by AP-1 (Fos/Jun). Ann Rheum Dis (2010) 69(Suppl 1):i86–8. doi:10.1136/ard.2009.119396
75. Wisdom R. AP-1: one switch for many signals. Exp Cell Res (1999) 253:180–5. doi:10.1006/excr.1999.4685
76. Szabo-Fresnais N, Blondeau JP, Pomerance M. Activation of the cAMP pathway synergistically increases IL-1-induced IL-6 gene expression in FRTL-5 thyroid cells: involvement of AP-1 transcription factors. Mol Cell Endocrinol (2008) 284:28–37. doi:10.1016/j.mce.2007.12.017
77. Xiao W, Hodge DR, Wang L, Yang X, Zhang X, Farrar WL. NF-kappaB activates IL-6 expression through cooperation with c-Jun and IL6-AP1 site, but is independent of its IL6-NFkappaB regulatory site in autocrine human multiple myeloma cells. Cancer Biol Ther (2004) 3:1007–17. doi:10.4161/cbt.3.10.1141
78. Zerbini LF, Wang Y, Cho JY, Libermann TA. Constitutive activation of nuclear factor kappaB p50/p65 and Fra-1 and JunD is essential for deregulated interleukin 6 expression in prostate cancer. Cancer Res (2003) 63:2206–15.
79. Bouwman P, Philipsen S. Regulation of the activity of Sp1-related transcription factors. Mol Cell Endocrinol (2002) 195:27–38. doi:10.1016/S0303-7207(02)00221-6
80. Kang SH, Brown DA, Kitajima I, Xu X, Heidenreich O, Gryaznov S, et al. Binding and functional effects of transcriptional factor Sp1 on the murine interleukin-6 promotor. J Biol Chem (1996) 271:7330–5. doi:10.1074/jbc.271.13.7330
81. Perkins ND, Edwards NL, Duckett CS, Agranoff AB, Schmid RM, Nabel GJ. A cooperative interaction between NF-kappa B and Sp1 is required for HIV-1 enhancer activation. EMBO J (1993) 12:3551–8.
82. Bidwell JP, Van Wijnen AJ, Fey EG, Dworetzky S, Penman S, Stein JL, et al. Osteocalcin gene promoter-binding factors are tissue-specific nuclear matrix components. Proc Natl Acad Sci U S A (1993) 90:3162–6. doi:10.1073/pnas.90.8.3162
83. Gerlo S, Haegeman G, Vanden Berghe W. Transcriptional regulation of autocrine IL-6 expression in multiple myeloma cells. Cell Signal (2008) 20:1489–96. doi:10.1016/j.cellsig.2008.04.004
84. van Wijnen AJ, Bidwell JP, Fey EG, Penman S, Lian JB, Stein JL, et al. Nuclear matrix association of multiple sequence-specific DNA binding activities related to SP-1, ATF, CCAAT, C/EBP, OCT-1, and AP-1. Biochemistry (1993) 32:8397–402.
85. Faggioli L, Merola M, Hiscott J, Furia A, Monese R, Tovey M, et al. Molecular mechanisms regulating induction of interleukin-6 gene transcription by interferon-gamma. Eur J Immunol (1997) 27:3022–30. doi:10.1002/eji.1830271140
86. Sweeney SE. Targeting interferon regulatory factors to inhibit activation of the type I IFN response: implications for treatment of autoimmune disorders. Cell Immunol (2011) 271:342–9. doi:10.1016/j.cellimm.2011.07.014
87. Smoak KA, Cidlowski JA. Mechanisms of glucocorticoid receptor signaling during inflammation. Mech Ageing Dev (2004) 125:697–706. doi:10.1016/j.mad.2004.06.010
88. Almawi WY, Melemedjian OK. Molecular mechanisms of glucocorticoid antiproliferative effects: antagonism of transcription factor activity by glucocorticoid receptor. J Leukoc Biol (2002) 71:9–15.
89. Morrison N, Eisman J. Role of the negative glucocorticoid regulatory element in glucocorticoid repression of the human osteocalcin promoter. J Bone Miner Res (1993) 8:969–75. doi:10.1002/jbmr.5650080810
90. Caldenhoven E, Liden J, Wissink S, Van de Stolpe A, Raaijmakers J, Koenderman L, et al. Negative cross-talk between RelA and the glucocorticoid receptor: a possible mechanism for the antiinflammatory action of glucocorticoids. Mol Endocrinol (1995) 9:401–12. doi:10.1210/mend.9.4.7659084
91. Schule R, Rangarajan P, Kliewer S, Ransone LJ, Bolado J, Yang N, et al. Functional antagonism between oncoprotein c-Jun and the glucocorticoid receptor. Cell (1990) 62:1217–26. doi:10.1016/0092-8674(90)90397-W
92. Liu H, Liu K, Bodenner DL. Estrogen receptor inhibits interleukin-6 gene expression by disruption of nuclear factor kappaB transactivation. Cytokine (2005) 31:251–7. doi:10.1016/j.cyto.2004.12.008
93. Ray A, Prefontaine KE, Ray P. Down-modulation of interleukin-6 gene expression by 17 beta-estradiol in the absence of high affinity DNA binding by the estrogen receptor. J Biol Chem (1994) 269:12940–6.
94. Ershler WB, Keller ET. Age-associated increased interleukin-6 gene expression, late-life diseases, and frailty. Annu Rev Med (2000) 51:245–70. doi:10.1146/annurev.med.51.1.245
95. Terry CF, Loukaci V, Green FR. Cooperative influence of genetic polymorphisms on interleukin 6 transcriptional regulation. J Biol Chem (2000) 275:18138–44. doi:10.1074/jbc.M000379200
96. Demirturk F, Ates O, Gunal O, Bozkurt N, Aysal T, Nacar MC. IL-6 gene promoter polymorphisms: genetic susceptibility to recurrent pregnancy loss. Bratisl Lek Listy (2014) 115:479–82. doi:10.4149/BLL_2014_092
97. Fishman D, Faulds G, Jeffery R, Mohamed-Ali V, Yudkin JS, Humphries S, et al. The effect of novel polymorphisms in the interleukin-6 (IL-6) gene on IL-6 transcription and plasma IL-6 levels, and an association with systemic-onset juvenile chronic arthritis. J Clin Invest (1998) 102:1369–76. doi:10.1172/JCI2629
98. von Linsingen R, Bompeixe EP, Bicalho Mda G. A case-control study in IL6 and TGFB1 gene polymorphisms and recurrent spontaneous abortion in southern Brazilian patients. Am J Reprod Immunol (2005) 53:94–9. doi:10.1111/j.1600-0897.2005.00250.x
99. Bhanoori M, Babu KA, Deenadayal M, Kennedy S, Shivaji S. The interleukin-6 – 174G/C promoter polymorphism is not associated with endometriosis in South Indian women. J Soc Gynecol Investig (2005) 12:365–9. doi:10.1016/j.jsgi.2005.03.005
Keywords: interleukin-6, interleukin-6 gene, pro-inflammatory cytokines, transcriptional regulation, signaling pathway
Citation: Luo Y and Zheng SG (2016) Hall of Fame among Pro-inflammatory Cytokines: Interleukin-6 Gene and Its Transcriptional Regulation Mechanisms. Front. Immunol. 7:604. doi: 10.3389/fimmu.2016.00604
Received: 13 October 2016; Accepted: 01 December 2016;
Published: 19 December 2016
Edited by:
Massimo Gadina, National Institute of Arthritis and Musculoskeletal and Skin Diseases, USAReviewed by:
Jaya Talreja, Wayne State University School of Medicine, USAKiyoshi Hirahara, Chiba University, Japan
Copyright: © 2016 Luo and Zheng. This is an open-access article distributed under the terms of the Creative Commons Attribution License (CC BY). The use, distribution or reproduction in other forums is permitted, provided the original author(s) or licensor are credited and that the original publication in this journal is cited, in accordance with accepted academic practice. No use, distribution or reproduction is permitted which does not comply with these terms.
*Correspondence: Song Guo Zheng, szheng1@hmc.psu.edu