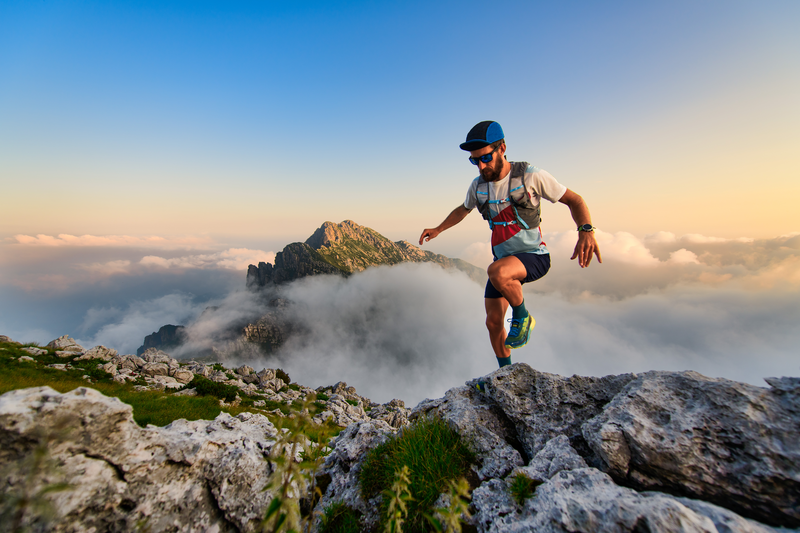
94% of researchers rate our articles as excellent or good
Learn more about the work of our research integrity team to safeguard the quality of each article we publish.
Find out more
REVIEW article
Front. Immunol. , 24 November 2016
Sec. T Cell Biology
Volume 7 - 2016 | https://doi.org/10.3389/fimmu.2016.00530
This article is part of the Research Topic On the Origin and Function of Human NK-like CD8+ T Cells: Charting New Territories View all 14 articles
The fundamental challenge of aging and long-term survivorship is maintenance of functional independence and compression of morbidity despite a life history of disease. Inasmuch as immunity is a determinant of individual health and fitness, unraveling novel mechanisms of immune homeostasis in late life is of paramount interest. Comparative studies of young and old persons have documented age-related atrophy of the thymus, the contraction of diversity of the T cell receptor (TCR) repertoire, and the intrinsic inefficiency of classical TCR signaling in aged T cells. However, the elderly have highly heterogeneous health phenotypes. Studies of defined populations of persons aged 75 and older have led to the recognition of successful aging, a distinct physiologic construct characterized by high physical and cognitive functioning without measurable disability. Significantly, successful agers have a unique T cell repertoire; namely, the dominance of highly oligoclonal αβT cells expressing a diverse array of receptors normally expressed by NK cells. Despite their properties of cell senescence, these unusual NK-like T cells are functionally active effectors that do not require engagement of their clonotypic TCR. Thus, NK-like T cells represent a beneficial remodeling of the immune repertoire with advancing age, consistent with the concept of immune plasticity. Significantly, certain subsets are predictors of physical/cognitive performance among older adults. Further understanding of the roles of these NK-like T cells to host defense, and how they integrate with other physiologic domains of function are new frontiers for investigation in Aging Biology. Such pursuits will require a research paradigm shift from the usual young-versus-old comparison to the analysis of defined elderly populations. These endeavors may also pave way to age-appropriate, group-targeted immune interventions for the growing elderly population.
Studies comparing young and old humans and mice have led to a voluminous body of literature showing a general age-related decline in various physiologic functions. In the immune system, among the most notable age-dependent physiologic retrogressions in the T cell compartment are inefficiencies in classical T cell receptor (TCR) signaling, thymic involution, contraction of the naïve T compartment, expansion of the memory T cell compartment, and overall shortening of telomeres (1–8). At the cellular level, aged CD4+ and CD8+ T cells have a deficiency in the expression of CD28 that coincides with highly shortened telomeres, high levels of expression of mitotic inhibitors, such as p16 and p53, and a severe limitation or complete lack of mitotic activity (9–13). All of these alterations have been argued to underlie the relative poorer antigen-specific T cell-dependent immunity among older adults compared to younger persons.
Older adults (generally defined as those aged ≥65 years), however, have highly heterogeneous health and immune phenotypes. They range from the frail and chronically ill residents of long-term care facilities to the community dwellers that are living independently (14–17). Many of them retain their ability to mount vaccine responses, including to the pandemic and seasonal influenza vaccines, and to the zoster vaccine (18–22). There are evidences of functionally active virus-specific T cells during new and reactivated latent infections (23–25). Old age has also become less of hurdle in the setting of organ transplantation for either organ donors or recipients (26–29). Thus, aging is not synonymous with poor health, or that the elderly are not mere defective versions of the young.
Heterogeneity of older adults provides a compelling rationale for a re-appraisal of “immunosenescence.” In its current usage, the term refers to the poorer degree of immune responsiveness of older adults relative to that seen in the young, a generalized and vague definition that has not substantially differed from the original concept proposed by Walford in the 1950s (30). Learning from epidemiological and geriatric studies (14–17, 31), we have articulated the paramount importance for the analysis of defined populations of the elderly, instead of continuing with the usual young-versus-old comparative approach. Such research paradigm shift is a key toward unraveling immunopathways that underlie discrete physiologic constructs of aging, such as frailty and successful aging (32, 33).
CD28 is the major co-stimulatory molecule that is required to sustain normal T cell activation (34) and for the elaboration of antigen-specific effector function in both naïve and memory compartments (35–37). In cohort studies, we provided the definitive proof for progressive loss of CD28 with chronologic aging (12). Such loss or absence of CD28 has long been thought to lead to deficiency or inefficiency of TCR signaling in aged T cells (10, 38). Indeed, mice with homozygous deletion of CD28 results in an immunosuppressed phenotype, since mouse CD28−/− T cells are anergic and prone to activation-induced cell death (35, 39).
The loss of CD28 on human T cells with aging (10, 12, 40) may not be surprising since CD28 expression is subject to transient downregulation during a normal immune response (41). In fact, deficiency of its expression is characteristic of continuous passages of T cell cultures (40, 42). These unusual CD28null CD8+ T cells have shortened telomeres (13), consistent with telomere-dependent senescence (sometimes referred to as “replicative senescence”) akin to those reported for other human somatic cells (43–47).
Due to more rapid turnover, CD8+ T cells have higher rate of CD28 loss than CD4+ T cells (48, 49). CD28null CD4+ and CD8+ T cells are highly oligoclonal and have highly shortened telomeres, indicating their long replicative history (12, 13). They also have high expression levels of p16 and 53, and they have limited, if not complete lack of, proliferative capacity even under conditions of optimal stimulation via TCR/CD3 in the presence of interleukin (IL)-2 in vitro (11, 12, 50, 51). All these properties are consistent with replicative senescence.
CD28 loss and telomere shortening are properties of primates, being typical of elderly humans as described above, as well as for older macaques and other anthropoids (52–55). In contrast, mouse T cells maintain long telomeres, and neither CD4+ nor CD8+ T cells show perceptible telomere shortening with multiple cell divisions in vitro (56). Indeed, it takes at least four generations for the telomerase-deficient mouse to show quantitative shortening of telomeres (57), indicating mice clearly do not undergo telomere-dependent replicative senescence.
Clonal expansions of T cells are characteristic of old mice similar to old humans (58). However, mouse T cells do not lose CD28 expression with chronologic aging. In fact, CD28 expression level may actually increase with age (59). Such species-specific difference in CD28 expression pattern between humans and mice is attributable to entirely non-homologous DNA sequences in the promoter regions of the CD28 gene (60) (Homo sapiens CD28, NCBI Gene 940, HGNC 1653; Mus musculus CD28, MGI 88327, NCBI Gene 12487). These age-related loss/maintenance of telomeres and loss of CD28 underscore that transposition of data obtained from mouse studies to human biology is unsound. We have articulated that while aging mouse models are instructive about the general biology of aging, they do not substitute for analytical studies of human elderly subjects (61).
The loss of CD28 is generally irreversible, due to the direct inactivation of the gene promoter (42, 62). The transcriptional initiator, a DNA sequence module in the 5′ cis-acting CD28 regulatory region where the activator complex, including nucleolin and heterogeneous ribonucleoprotein-DOA, is unoccupied in senescent CD28null T cells (63). Nucleolin and heterogeneous ribonucleoprotein-DOA are found in senescent T cells, but they do not form a functional initiator complex. While mechanism(s) underlying the failure of the assembly of this transcriptional complex remains to be investigated, it is clear that non-occupancy of the CD28 initiator results in a transcriptional block, leading to the absence of all splice forms of CD28 mRNA and the lack of expression of CD28 on the T cell surface (42, 64, 65).
CD28null T cells are resistant to apoptosis (66), which explains their persistence in circulation for years and their pervasive accumulation in vivo with advancing age. This is attributed to constitutively high levels of expression of Bcl2 and Bcl-xL, with corresponding downregulation of Bax (12, 67). Bcl-independent pathways for the lifelong persistence of these cells have also been reported (68).
Whether they are naturally derived in vivo during aging, or in an in vitro senescence system, oligoclonal senescent CD28null T cells have a unique phenotype for their de novo acquisition of a diverse array of receptors normally expressed on NK cells (12, 50, 69, 70). The repertoire of NK-related receptors they express does not reflect the full complement of the many NK receptor genes normally expressed on NK cells (50). However, the NK-related receptors on aged CD28null T cells are expressed co-dominantly in varying combinations along clonal lineages. CD28null T cells with identical TCR CDR3 sequences, indicating their common origin from a single mother CD28+ T cell, may express different types of NK-related receptors (71, 72).
Whether the loss of CD28 is required for, or is an event independent from, the expression of NK-related receptors remains to be examined. However, it is clear that differences in the patterns of expression of these receptors between NK cells and CD28null T cells are related to cell-specific differences in the regulatory modules of each NK-related receptor. For example, we have shown that differential expression of CD158b1 (KIR 2DL2) between T and NK cells are controlled by two distinct transcriptional regulatory motifs on the upstream cis-acting promoter region of the gene; namely, a proximal element at −51 and an AML site at position −98 for T and NK cells, respectively (73). Other investigators have reported the role of age-related epigenetic alterations. Differential induction of CD158d (KIR 2DL4) and CD158b2 (KIR 2DL3) on T cells is related to methylation/demethylation on promoter regions of these two genes, in contrast to their classical promoter-driven expression as seen in NK cells (74–76). These studies suggest that there may be diverse regulatory machineries involved in the induction of NK-related receptors on T cells with aging. Given the diversity of these receptors and their apparent co-dominant expression, it will be of interest to examine whether and how expression of one NK-receptor affects the expression of another NK-receptor during the aging process. A particular interest is the regulation of expression of the prototypic receptors CD56, CD16, and NKG2D on aged T cells. But regardless of whether such regulation occurs at the level of unique promoter motifs, or through structural alterations of chromatin that favor accessibility of the particular NK-receptor gene, or perhaps through posttranscription controls, it is clear that the acquisition of NK receptors by T cells corresponds with the elaboration of new effector function (77).
The phenomenal age-related expression of NK-related receptors on T cells has been associated with seropositivity to cytomegalovirus (CMV) (78). This is in line with reports about similar association of CMV serology with frequency of CD28null T cells, and such serological-cellular association has been argued to be a predictor of poor health outcomes of aging (79, 80). It has also been suggested that CMV infection may lead to the emergence of these senescent T cells that are considered dysfunctional or non-functional (81–83). However, such studies are purely associational rather than causal. Further, the association is not universal. The cited studies are mostly from those on elderly populations in Northern Europe where CMV exposure appears to occur gradually over the life span, which might explain the high CMV seropositivity in old age (80). In the United States, CMV exposure is already widespread at early adolescence (84). Yet, we have shown that senescent NK-like T cells are rarely found among young Americans (12, 32). Importantly, we found very high titers of anti-CMV antibody among older adults and found no clinical evidence of CMV disease. Indeed, another cohort study showed CMV seropositivity alone is an insufficient measure of health risk among older Americans (85). In addition, populations of CMV-specific T cells have been found to be functional with clear beneficial antiviral effects (68, 86, 87). A recent experimental study has shown further that CMV by itself does not induce replicative senescence for T cells (25). CMV disease is undoubtedly serious whether it happens at an early or old age. However, the causative role of CMV in human T cell senescence is yet to be proven. Broader experimental studies are needed to determine what particular environmental and/or endogenous factors trigger, drive, and maintain populations of senescent NK-like T cells in vivo during the aging process.
The array of NK-related receptors expressed on aged CD28null T cells is summarized in Figure 1. They include the prototypic stimulatory NK receptors, CD16, CD56, and NKG2D. They may also express CD161, and various inhibitory NK receptors such as CD94 and NKG2A, and members of the CD158 killer cell immunoglobulin-like receptor family (12, 50, 69, 72, 77, 88–90). Unlike the selective single allelic expression for TCR, NK-related receptors are expressed co-dominantly on aged T cells.
Figure 1. Diagrammatic comparison between conventional CD28+ and senescent CD28null NK-like T cells. This illustration summarizes findings from various investigators as described in the text. The diagram shown was modified from (32) with permission from Aging and Disease journal under the terms of Creative Commons Attribution License (CC BY). This license allows the unrestricted use, distribution, modification, and reproduction in any medium by the author with credited citation of the original publication.
In addition to shortened telomeres, high p16/53 expression levels, and irreversible loss of CD28, aged NK-like T cells express two other markers of senescence, namely, KLRG1 and CD57 (81, 91, 92). KLRG1 is an inhibitory NK-related receptor that has been shown to actively suppress classical TCR signaling (93). CD57 is an adhesion molecule that is typically expressed on terminally differentiated T cells. Although it is still unclear if CD57 itself is a signaling receptor that dictates or alters T cell effector function, its expression on T cells is biomarker for cell cycle arrest in aged T cells (91, 94). It might be noted that CD57 is also expressed on highly differentiated NK cells (95, 96). However, whether such CD57+ NK cells are senescent, and that CD57 directly controls NK cell function are also not yet known.
Despite their senescent properties, CD28null NK-like T cells are highly functional and versatile. While there is general trend for the varying inefficiencies of classical TCR signaling during aging (1, 2, 97–99), there could still be residual TCR signaling as exemplified by long-lived memory T cells in the context small pox and polio vaccination (100, 101). Indeed, experimental studies showing unusually high constitutive level of expression of interferon (IFN) γ in CD28null T cells can further increase following ligation of TCR/CD3 (38, 94, 102). Such residual TCR-driven response may be attributed to other co-stimulatory molecules, such as 41BB ligand, OX40, CD70, and CD58, which substitutes for the defunct CD28 (103–107).
More significantly, we have reported that effector activities of CD28null NK-like CD4+ and CD8+ T cells are directly attributable to signaling of the NK-related receptors they express in a totally TCR-independent manner (12, 70). We have shown that CD56-driven and NKG2D-driven expression of the early activation cell surface antigen CD69; the intracellular expression of IL-4, IFNγ, CD107b/LAMP2, perforin, and granzyme; and the late cell surface expression of exocytosis protein CD107a are to be as effective as, if not better than, classical TCR stimulation. In fact, the CD56-/NKG2D-driven TCR-independent expression of perforin, granzyme, and CD107a occur in both CD4+ and CD8+ NK-like T cells. This indicates that the conventional “helper” and “cytotoxic” designations for CD4+ and CD8+ T cells, respectively, are not instructive about of the biology of T cells in old age. Similarly, the expression of CD154 (CD40 ligand) on aged T cells does not follow the usual CD4 helper paradigm. CD154 is lost on senescent CD28null CD4+ NK-like T cells but is gained by senescent CD28null CD8+ NK-like T cells (38, 108, 109). This suggests that the latter cell subset is a potential target to boost humoral immunity in the elderly.
Physiologic systems are optimized toward reproduction, after which the goal is individual survival (110). There is evolutionary conservation of biological pathways that ensure individual survival beyond reproductive maturity (111, 112), including a variety of genes referred to as “longevity assurance” genes that promote long-term survival (113–116). Older organisms are essential in maintaining population structures particularly among social animals and are therefore involved ultimately in the perpetuation of the species (112, 117–119).
Immunity is an evolutionary determinant of individual fitness and survival (120–122). The accumulation of NK-like CD28null T cells with advancing age represents a remodeling of the immune repertoire as a compensatory mechanism for the general age-related losses in conventional T cell-dependent immunity (123). As described previously, there is thymic atrophy with age leading to impaired production of new naïve T cells, making older adults unable to respond to new and emerging pathogens in an antigen-specific manner (3, 124). With antigenic exposure through life, there is progressive contraction of the naïve T cell compartment, with corresponding expansion of memory and senescent T cell compartment. These events over the lifespan result in the contraction of diversity of the clonotypic TCR repertoire (5, 49). With cycles of expansion and death of T cells during antigenic challenges, the phenomenal accumulation of apoptosis-resistant CD28null NK-like T cells is likely a protection against clinical lymphopenia, which is very rare among older adults (125, 126).
The acquisition of a diverse array of NK-related receptors on CD28null T cells maintains immunologic diversity in old age. As discussed previously, there is co-dominant expression of diverse NK-related receptors along clonal lineages of CD28null T cells in late life. This is in stark contrast to the conventional clonotypic TCR diversity that is characteristic of the young. Signaling of these NK-related receptors effectively imparts an innate function to aged T cells (12, 70); hence, we had originally introduced the term “NK-like T cells” to emphasize their NK-related receptor-driven, TCR-independent effector function (50). The term underlines the diverse array of NK-related receptors expressed along oligoclonal TCRαβ lineages, in contrast to convention αβTCR repertoire diversity in the young (12). NK-like T cells are distinct from conventional NKT cells (or invariant iNKT cells), which are identified a single invariant TCR AV24BV11 that recognizes glycolipid antigens presented in the context of CD1d instead of conventional HLA antigen-presenting molecules (127, 128).
NK-like T cells compensate for the corresponding age-related functional loses in the NK cell compartment (32). NK cell numbers are largely maintained through life, but skewing of certain NK cell subsets with aging have been reported (129). We have shown that octo-/nona-genarians have contracted pools of CD56+ and CD16+ NK cells, which are accompanied by corresponding age-dependent gains of CD56 and CD16 expression on both CD4+ and CD8+ T cells (32, 70). As already described previously, CD56 ligation alone can drive T cell effector activities. The function of CD16 on NK-like T cells remains to be examined.
Induction of NK-related receptors on T cells may not be surprising since T cells and NK cells originate from a common lymphoid progenitor. We have shown that NK cells have an abundance of untranslated, but re-arranged, TCRαβ mRNA with sequences identical to those seen in T cells (130). Thus, inducibility of NK-related receptors in senescent CD28null NK-like T cells is consistent with functional plasticity of T cells (131–133). Although the intricacies of T cell plasticity are still being investigated, such plasticity re-directs the elaboration of effector activities to ensure a vigorous immunity. In old age, signaling of effector activities of NK-like T cells through NK-related receptors is an adaptation of the aging immune system. Such adaptation is a way to maintain immune homeostasis despite the inefficiency of classical TCR signaling and the contraction of diversity of the repertoire of clonotypic TCRs. NK-like T cells are highly resistant to cell death (12) and may represent Darwin’s “fittest” lymphocytes that contribute to immune function into old age.
Cell senescence is undoubtedly a characteristic of old organisms, and it contributes to age-related malfunction in various tissues/organs (44, 134, 135). However, cell senescence also has physiologic benefits. Among these is its role in tumor suppression (134, 136, 137). Cell senescence also plays a role in tissue repair (138), such as in the prevention of fibrosis in liver, skin, kidney, and heart, and in the prevention of atherosclerosis and pulmonary hypertension (139). In addition, there is also programed cell senescence, which is an essential component of embryogenesis (140–142). Along these lines, the age-dependent emergence of functionally competent senescent NK-like CD28null T cells represents a significant and beneficial remodeling of the immune repertoire (123).
T cell repertoire remodeling through the de novo expression of NK-related receptors along clonal lineages of senescent CD28null T cells is also consistent with age-related functional plasticity in certain organ systems. For example, there is age-related structural and functional decline in the central nervous system that leads to varying degrees of cognitive impairment, such as dementia and Alzheimer’s disease. There is heritability of high cognitive function into old age (143, 144). The roles of specific genes or gene polymorphisms, and epigenetic programs have been reported (114, 115, 119, 145–150). But the apparent “default” trajectory of age-related cognitive decline may be altered by physical activity, inclusive of regimented exercise, strength training, or usual activity such as walking. This has been best illustrated by improvement of various aspects of cognitive function, including memory and learning, among older adults engaged in regular physical activity (151–158). Functional brain imaging show extraordinary brain networks of neurocognitive performance following physical activity (159–161). In experimental animals, physical activity elicits an array of genes, along with epigenetic changes, associated with improvement in neurobehavioral performance (162–165). While the mechanisms underlying the improvement of brain/cognitive function with physical activity need to be examined further, aging of the brain is undoubtedly amenable to modulation.
Similarly, aging leads to a decline skeletal muscle function, including an age-related inefficiency of muscle mitochondria. Yet, the aging skeletal muscle is functionally plastic. Whereas certain gene polymorphisms have been implicated to maintain muscle function with age (166), physical activity has been shown to improve muscle and mitochondrial function among older adults (167–170). An important component of physical activity-induced improvement of function of the aging muscle is the equally plastic satellite cells that maintain muscle organization (171, 172). Clearly, certain physiologic systems including immune cells are functionally plastic, a property that may be exploited to maintain, if not improve, functional performance in old age.
As described previously, older adults are highly heterogeneous, with varying health phenotypes and life expectancy. An improved understanding of this heterogeneity has been facilitated by objective measurements of physical and cognitive function. Such measurements have led to better stratification of elders; from frail residents of long-term care facilities, to successfully aging community dwellers (16, 31, 153, 173–177). Thus, we have been proponents for the integration of immunity with other domains of function (32).
Integration of immunity to other physiologic systems may be best illustrated by our cross sectional study of the All Stars cohort (70) of the survivors from the Cardiovascular Health Study, a multicenter long-term study of aging (16, 178, 179). Categorization of elders was based on cognition scores (3MS), measured by the average of three tests using the modified minimental examination (180), and self report of difficulty in performing activities of daily living (ADL), namely, dressing, toileting, transferring, eating, and bathing (181). High functioning (or “unimpaired”) was defined as 3MS score >80 and ADL = 0. The data showed that the stimulatory NK-related receptors CD16, CD56, and NKG2D in all T cell subsets were the most prominent cellular components of the immune signature of the high functioning group as determined by factor analysis. In contrast, the inhibitory NK-related receptors NKG2A, CD158a, and CD158e comprised the cell signature of the functionally impaired. In line with these fingerprints, logistic regression analysis of the same dataset showed CD56 and CD16 expression was significant predictors of high functional performance. In contrast, NKG2A and CD158a were negative predictors. More importantly, CD28null T cells in the CD4 but not in the CD8, compartment expressing these four NK-related receptors were the cell subset predictor of high cognitive/physical functioning.
Another way to illustrate the relationship between NK-like CD28null T cells and physical/cognitive functioning is shown in Figure 2 with a three dimensional plot for CD16 or CD56 expression levels (measured as GMFI, geometric mean fluorescence intensity), 3MS cognition score, and gait speed. The latter measure of physical function was determined by a 4-m walk test that has been standardized/validated from various cohort studies (16, 176). The data show a clear segregation between the high functioning and functionally impaired elders. This is surprising given that “impaired” and “unimpaired” categories in this graphical illustration are very loosely defined by ADL ≥ 1 and ADL = 0, respectively. Therefore, it will be of significant interest to determine if this three-way relationship between subsets of NK-like T cells, physical function, and cognitive ability translates into vigorous immune defense. In addition, the underlying mechanistic link(s) between these three physiologic systems will be instructive about integrative physiology of successful aging.
Figure 2. NK-like T cells are linked to high cognitive and physical function. Data shown are 3D scatter plot summaries from the re-analyses of our data from the All Stars cohort of the Cardiovascular Health Study (70). CD16 and CD56 expression on CD4+ CD28null and CD8+ CD28null T cells are expressed as GMFI, which was determined by multicolor flow cytometry. Older adults were grouped as unimpaired (solid triangles) or impaired (open circles) based on a simple criterion of ADL = 0 and ADL > 1, respectively. Measurements of 3MS cognition score and gait speed and ADL scoring are as described in the text.
NK-like CD28null T cells represent a beneficial remodeling of the T cell repertoire with aging. Paradoxically, similar cells have also been found among young patients with chronic immune-mediated diseases in an age-disproportionate manner. We have shown the infiltration of CD56+ CD28null CD4+ T cells in extra-articular lesions in rheumatoid arthritis (182). Inflammatory CD56+ T cells have been reported in coronary artery disease, asthma, ulcerative colitis, and chronic hepatitis C disease (183–186). NKG2D+ CD28null T cells have some tumor-promoting activity in experimental settings (187, 188) and as inflammatory mediators in Wegener’s granulomatosis, rheumatoid arthritis, juvenile-onset systemic lupus erythematosus, and celiac disease (189–192).
Many of these diseases have characteristic systemic upregulation of TNFα (193). We have shown that TNFα can directly block the CD28 transcriptional initiator (65, 194). In a TNFα-rich environment, such as in the case of rheumatoid arthritis, we found that anti-TNF therapy prevents the TNFα-induced loss of CD28 on the residual CD28+ CD8+ and CD4+ T cells, but the numbers of CD28null T cells remain the same (194). Whether or not TNFα induces the gain NK-related receptors has not yet been examined.
Interestingly, CD56+/NKG2D+ T cells also have beneficial effects in disease settings. Regulatory CD56+ CD28null CD8+ T cells and NKG2D+ T cells have been reported in rheumatoid arthritis and in juvenile-onset systemic lupus erythematosus, respectively (195, 196). Similar NK-like T cell subsets appear to be normal components of regional host defense in the gut. They may have auxiliary antitumor effect and have been associated with antiviral immunity in the setting of allergies and chronic hepatitis B disease (197–200).
Such age-disproportionate emergence of senescent CD28null NK-like T cells supports the provocative idea that premature senescence of T cells is a critical factor in the pathogenesis and clinical prognosis of chronic diseases of the young (201). These apparent beneficial and detrimental effects of certain NK-like T cell subsets among young patients, and the beneficial effects of similar cells during aging as described above, are consistent with the evolutionary concept of antagonistic pleiotropy (202). This concept posits that genes and biological pathways that are beneficial in the young may be detrimental in the old, and vice-versa. Therefore, a scientific challenge is to determine conditions in disease states of the young where CD28null NK-like T cells might exert a pathogenic effect. It will be of similar interest to determine what drives the accumulation of beneficial senescent CD28null NK-like T cells during the aging process.
The expression of NK-related receptors along clonal lineages of CD28null T cells with aging clearly represents a reshaping or remodeling of the immune repertoire. T cell signaling through these receptors independent of the TCR also illustrates the emerging theme that cell senescence may not necessarily be synonymous with dysfunction. One scientific challenge is to determine what drives the induction of diversity of expression of NK-related receptors on T cells with advancing age. Another is to determine whether the TCR-independent effector function of NK-like T cells translates into vigorous immune defense and/or immune surveillance in late life. A corollary interest is a possible dual functionality of these T cells, namely, their ability to trigger a classic TCR-driven response, while triggering a complementary innate TCR-independent response mediated through the particular NK-receptor(s) they express. Plausibility of this dual function has been shown experimentally for the interaction between tumor cells and particular NK-like CD8+ T cell lines in vitro (203). An equal challenge is to elucidate the paradoxical age-disproportionate accumulation of NK-like T cells in disease states. Whether they represent cells involved in tissue repair or if they are true pathogenic effectors will be instructive into harnessing or dampening their effector function in disease settings. During the aging process, the most significant challenge is to determine how and why particular subsets of NK-like CD28null T cells are closely linked to physical performance and cognitive ability. Dissecting these mechanisms will depend on the analyses of defined populations of the elderly, rather than continuing with the usual young-versus-old comparisons.
JM, PG, and AV drafted and edited the manuscript. JM and PG generated the figures. AV secured funding.
The authors declare that the research was conducted in the absence of any commercial or financial relationships that could be construed as a potential conflict of interest.
The authors thank Dr. Roger S. Crowther for editing this manuscript.
Research is supported by grants from the American Federation for Aging Research (AFAR) and Ellison Medical Foundation (M12589), and the National Institutes of Health (R01 AG030734). Supplemental support is provided by the Pittsburgh Claude Pepper Older Americans Independence Center, an NIH-funded center of excellence (P30 AG024827). AV is recipient of the AFAR Julie Martin Career Award on Aging.
3MS, average of three tests of the modified minimental examination; ADL, activities of daily living; GMFI, geometric mean fluorescence intensity; IFN, interferon; IL, interleukin; TCR, T cell receptor; TNF, tumor necrosis factor.
1. Larbi A, Pawelec G, Wong SC, Goldeck D, Tai JJ, Fulop T. Impact of age on T cell signaling: a general defect or specific alterations? Ageing Res Rev (2011) 10(3):370–8. doi: 10.1016/j.arr.2010.09.008
2. Fulop T, Le Page A, Fortin C, Witkowski JM, Dupuis G, Larbi A. Cellular signaling in the aging immune system. Curr Opin Immunol (2014) 29:105–11. doi:10.1016/j.coi.2014.05.007
3. Schulz AR, Malzer JN, Domingo C, Jurchott K, Grutzkau A, Babel N, et al. Low thymic activity and dendritic cell numbers are associated with the immune response to primary viral infection in elderly humans. J Immunol (2015) 195(10):4699–711. doi:10.4049/jimmunol.1500598
4. Ventevogel MS, Sempowski GD. Thymic rejuvenation and aging. Curr Opin Immunol (2013) 25(4):516–22. doi:10.1016/j.coi.2013.06.002
5. Naylor K, Li G, Vallejo AN, Lee WW, Koetz K, Bryl E, et al. The influence of age on T cell generation and TCR diversity. J Immunol (2005) 174(11):7446–52. doi:10.4049/jimmunol.174.11.7446
6. Sfikakis PP, Gourgoulis GM, Moulopoulos LA, Kouvatseas G, Theofilopoulos AN, Dimopoulos MA. Age-related thymic activity in adults following chemotherapy-induced lymphopenia. Eur J Clin Invest. (2005) 35(6):380–7. doi:10.1111/j.1365-2362.2005.01499.x
7. Fagnoni FF, Vescovini R, Passeri G, Bologna G, Pedrazzoni M, Lavagetto G, et al. Shortage of circulating naive CD8(+) T cells provides new insights on immunodeficiency in aging. Blood (2000) 95(9):2860–8.
8. Weng NP, Levine BL, June CH, Hodes RJ. Human naive and memory T lymphocytes differ in telomeric length and replicative potential. Proc Natl Acad Sci U S A (1995) 92(24):11091–4. doi:10.1073/pnas.92.24.11091
9. Effros RB. Loss of CD28 expression on T lymphocytes: a marker of replicative senescence. Dev Comp Immunol (1997) 21(6):471–8. doi:10.1016/S0145-305X(97)00027-X
10. Posnett DN, Sinha R, Kabak S, Russo C. Clonal populations of T cells in normal elderly humans: the T cell equivalent to “benign monoclonal gammapathy”. J Exp Med (1994) 179(2):609–18. doi:10.1084/jem.179.2.609
11. Scheuring UJ, Sabzevari H, Theofilopoulos AN. Proliferative arrest and cell cycle regulation in CD8(+)CD28(-) versus CD8(+)CD28(+) T cells. Hum Immunol (2002) 63(11):1000–9. doi:10.1016/S0198-8859(02)00683-3
12. Lemster BH, Michel JJ, Montag DT, Paat JJ, Studenski SA, Newman AB, et al. Induction of CD56 and TCR-independent activation of T cells with aging. J Immunol (2008) 180(3):1979–90. doi:10.4049/jimmunol.180.3.1979
13. Batliwalla FM, Rufer N, Lansdorp PM, Gregersen PK. Oligoclonal expansions in the CD8(+)CD28(-) T cells largely explain the shorter telomeres detected in this subset: analysis by flow FISH. Hum Immunol (2000) 61(10):951–8. doi:10.1016/S0198-8859(00)00157-9
14. Fried LP, Tangen CM, Walston J, Newman AB, Hirsch C, Gottdiener J, et al. Frailty in older adults: evidence for a phenotype. J Gerontol A Biol Sci Med Sci (2001) 56(3):M146–56. doi:10.1093/gerona/56.3.M146
15. Bandeen-Roche K, Xue QL, Ferrucci L, Walston J, Guralnik JM, Chaves P, et al. Phenotype of frailty: characterization in the women’s health and aging studies. J Gerontol A Biol Sci Med Sci (2006) 61(3):262–6. doi:10.1093/gerona/61.3.262
16. Newman AB, Arnold AM, Sachs MC, Ives DG, Cushman M, Strotmeyer ES, et al. Long-term function in an older cohort – the cardiovascular health study all stars study. J Am Geriatr Soc (2009) 57(3):432–40. doi:10.1111/j.1532-5415.2008.02152.x
17. Strotmeyer ES, Arnold AM, Boudreau RM, Ives DG, Cushman M, Robbins JA, et al. Long-term retention of older adults in the Cardiovascular Health Study: implications for studies of the oldest old. J Am Geriatr Soc (2010) 58(4):696–701. doi:10.1111/j.1532-5415.2010.02770.x
18. Fisman DN, Savage R, Gubbay J, Achonu C, Akwar H, Farrell DJ, et al. Older age and a reduced likelihood of 2009 H1N1 virus infection. N Engl J Med (2009) 361(20):2000–1. doi:10.1056/NEJMc0907256
19. McElhaney JE, Xie D, Hager WD, Barry MB, Wang Y, Kleppinger A, et al. T cell responses are better correlates of vaccine protection in the elderly. J Immunol (2006) 176(10):6333–9. doi:10.4049/jimmunol.176.10.6333
20. Shahid Z, Kleppinger A, Gentleman B, Falsey AR, McElhaney JE. Clinical and immunologic predictors of influenza illness among vaccinated older adults. Vaccine (2010) 28(38):6145–51. doi:10.1016/j.vaccine.2010.07.036
21. Levin MJ, Oxman MN, Zhang JH, Johnson GR, Stanley H, Hayward AR, et al. Varicella-zoster virus-specific immune responses in elderly recipients of a herpes zoster vaccine. J Infect Dis. (2008) 197(6):825–35. doi:10.1086/528696
22. Weinberg A, Zhang JH, Oxman MN, Johnson GR, Hayward AR, Caulfield MJ, et al. Varicella-zoster virus-specific immune responses to herpes zoster in elderly participants in a trial of a clinically effective zoster vaccine. J Infect Dis. (2009) 200(7):1068–77. doi:10.1086/605611
23. Zhou X, McElhaney JE. Age-related changes in memory and effector T cells responding to influenza A/H3N2 and pandemic A/H1N1 strains in humans. Vaccine (2011) 29(11):2169–77. doi:10.1016/j.vaccine.2010.12.029
24. Weekes MP, Carmichael AJ, Wills MR, Mynard K, Sissons JG. Human CD28-CD8+ T cells contain greatly expanded functional virus-specific memory CTL clones. J Immunol (1999) 162(12):7569–77.
25. Riddell NE, Griffiths SJ, Rivino L, King DC, Teo GH, Henson SM, et al. Multifunctional cytomegalovirus (CMV)-specific CD8(+) T cells are not restricted by telomere-related senescence in young or old adults. Immunology (2015) 144(4):549–60. doi:10.1111/imm.12409
26. Karatzas T, Bokos J, Katsargyris A, Diles K, Sotirchos G, Barlas A, et al. Advanced donor age alone is not a risk factor for graft survival in kidney transplantation. Transplant Proc. (2011) 43(5):1537–43. doi:10.1016/j.transproceed.2011.03.014
27. Taner CB, Ung RL, Rosser BG, Aranda-Michel J. Age is not a contraindication for orthotopic liver transplantation: a single institution experience with recipients older than 75 years. Hepatol Int (2011) 6(1):403–7. doi:10.1007/s12072-011-9286-7
28. Alamo JM, Olivares C, Jimenez G, Bernal C, Marin LM, Tinoco J, et al. Donor characteristics that are associated with survival in liver transplant recipients older than 70 years with grafts. Transplant Proc (2013) 45(10):3633–6. doi:10.1016/j.transproceed.2013.10.031
29. Paterno F, Wima K, Hoehn RS, Cuffy MC, Diwan TS, Woodle SE, et al. Use of elderly allografts in liver transplantation. Transplantation (2016) 100(1):153–8. doi:10.1097/TP.0000000000000806
30. Walford RL. The immunologic theory of aging. Gerontologist (1964) 4:195–7. doi:10.1093/geront/4.4.195
31. Christensen K, McGue M, Petersen I, Jeune B, Vaupel JW. Exceptional longevity does not result in excessive levels of disability. Proc Natl Acad Sci U S A (2008) 105(36):13274–9. doi:10.1073/pnas.0804931105
32. Griffin P, Michel JJ, Huysman K, Logar AJ, Vallejo AN. Integration of immunity with physical and cognitive function in definitions of successful aging. Aging Dis (2012) 3(1):34–50.
33. Lowry KA, Vallejo AN, Studenski SA. Successful aging as a continuum of functional independence: lessons from physical disability models of aging. Aging Dis. (2012) 3(1):5–15.
34. Esensten JH, Helou YA, Chopra G, Weiss A, Bluestone JA. CD28 costimulation: from mechanism to therapy. Immunity (2016) 44(5):973–88. doi:10.1016/j.immuni.2016.04.020
35. Noel PJ, Boise LH, Green JM, Thompson CB. CD28 costimulation prevents cell death during primary T cell activation. J Immunol (1996) 157(2):636–42.
36. Boesteanu AC, Katsikis PD. Memory T cells need CD28 costimulation to remember. Semin Immunol (2009) 21(2):69–77. doi:10.1016/j.smim.2009.02.005
37. Welten SP, Melief CJ, Arens R. The distinct role of T cell costimulation in antiviral immunity. Curr Opin Virol (2013) 3(4):475–82. doi:10.1016/j.coviro.2013.06.012
38. Weyand CM, Brandes JC, Schmidt D, Fulbright JW, Goronzy JJ. Functional properties of CD4+ CD28- T cells in the aging immune system. Mech Ageing Dev (1998) 102(2–3):131–47. doi:10.1016/S0047-6374(97)00161-9
39. Shahinian A, Pfeffer K, Lee KP, Kundig TM, Kishihara K, Wakeham A, et al. Differential T cell costimulatory requirements in CD28-deficient mice. Science (1993) 261(5121):609–12. doi:10.1126/science.7688139
40. Effros RB, Boucher N, Porter V, Zhu X, Spaulding C, Walford RL, et al. Decline in CD28+ T cells in centenarians and in long-term T cell cultures: a possible cause for both in vivo and in vitro immunosenescence. Exp Gerontol (1994) 29(6):601–9. doi:10.1016/0531-5565(94)90073-6
41. Eck SC, Chang D, Wells AD, Turka LA. Differential down-regulation of CD28 by B7-1 and B7-2 engagement. Transplantation (1997) 64(10):1497–9. doi:10.1097/00007890-199711270-00025
42. Vallejo AN, Brandes JC, Weyand CM, Goronzy JJ. Modulation of CD28 expression: distinct regulatory pathways during activation and replicative senescence. J Immunol (1999) 162(11):6572–9.
43. Sharpless NE, DePinho RA. Telomeres, stem cells, senescence, and cancer. J Clin Invest (2004) 113(2):160–8. doi:10.1172/JCI20761
44. Minamino T, Miyauchi H, Yoshida T, Tateno K, Kunieda T, Komuro I. Vascular cell senescence and vascular aging. J Mol Cell Cardiol (2004) 36(2):175–83. doi:10.1016/j.yjmcc.2003.11.010
45. Wege H, Chui MS, Le HT, Strom SC, Zern MA. In vitro expansion of human hepatocytes is restricted by telomere-dependent replicative aging. Cell Transplant (2003) 12(8):897–906. doi:10.3727/000000003771000138
46. Ramirez RD, Herbert BS, Vaughan MB, Zou Y, Gandia K, Morales CP, et al. Bypass of telomere-dependent replicative senescence (M1) upon overexpression of Cdk4 in normal human epithelial cells. Oncogene (2003) 22(3):433–44. doi:10.1038/sj.onc.1206046
47. Voghel G, Thorin-Trescases N, Farhat N, Nguyen A, Villeneuve L, Mamarbachi AM, et al. Cellular senescence in endothelial cells from atherosclerotic patients is accelerated by oxidative stress associated with cardiovascular risk factors. Mech Ageing Dev (2007) 128(11–12):662–71. doi:10.1016/j.mad.2007.09.006
48. Naumov YN, Naumova EN, Yassai MB, Gorski J. Selective T cell expansion during aging of CD8 memory repertoires to influenza revealed by modeling. J Immunol (2011) 186(11):6617–24. doi:10.4049/jimmunol.1100091
49. Wack A, Cossarizza A, Heltai S, Barbieri D, D’Addato S, Fransceschi C, et al. Age-related modifications of the human alphabeta T cell repertoire due to different clonal expansions in the CD4+ and CD8+ subsets. Int Immunol (1998) 10(9):1281–8. doi:10.1093/intimm/10.9.1281
50. Abedin S, Michel JJ, Lemster B, Vallejo AN. Diversity of NKR expression in aging T cells and in T cells of the aged: the new frontier into the exploration of protective immunity in the elderly. Exp Gerontol (2005) 40(7):537–48. doi:10.1016/j.exger.2005.04.012
51. Perillo NL, Naeim F, Walford RL, Effros RB. The in vitro senescence of human T lymphocytes: failure to divide is not associated with a loss of cytolytic activity or memory T cell phenotype. Mech Ageing Dev (1993) 67(1–2):173–85. doi:10.1016/0047-6374(93)90121-7
52. Jankovic V, Messaoudi I, Nikolich-Zugich J. Phenotypic and functional T-cell aging in rhesus macaques (Macaca mulatta): differential behavior of CD4 and CD8 subsets. Blood (2003) 102(9):3244–51. doi:10.1182/blood-2003-03-0927
53. Pitcher CJ, Hagen SI, Walker JM, Lum R, Mitchell BL, Maino VC, et al. Development and homeostasis of T cell memory in rhesus macaque. J Immunol (2002) 168(1):29–43. doi:10.4049/jimmunol.168.1.29
54. Steinert S, White DM, Zou Y, Shay JW, Wright WE. Telomere biology and cellular aging in nonhuman primate cells. Exp Cell Res (2002) 272(2):146–52. doi:10.1006/excr.2001.5409
55. Trochet D, Mergui X, Ivkovic I, Porreca RM, Gerbault-Seureau M, Sidibe A, et al. Telomere regulation during ageing and tumorigenesis of the grey mouse lemur. Biochimie (2015) 113:100–10. doi:10.1016/j.biochi.2015.04.002
56. Kipling D, Cooke HJ. Hypervariable ultra-long telomeres in mice. Nature (1990) 347(6291):400–2. doi:10.1038/347400a0
57. Blasco MA, Lee HW, Hande MP, Samper E, Lansdorp PM, DePinho RA, et al. Telomere shortening and tumor formation by mouse cells lacking telomerase RNA. Cell (1997) 91(1):25–34. doi:10.1016/S0092-8674(01)80006-4
58. Callahan JE, Kappler JW, Marrack P. Unexpected expansions of CD8-bearing cells in old mice. J Immunol (1993) 151(12):6657–69.
59. Ortiz-Suarez A, Miller RA. A subset of CD8 memory T cells from old mice have high levels of CD28 and produce IFN-gamma. Clin Immunol (2002) 104(3):282–92. doi:10.1006/clim.2002.5221
60. Vallejo AN. CD28 extinction in human T cells: altered functions and the program of T-cell senescence. Immunol Rev (2005) 205:158–69. doi:10.1111/j.0105-2896.2005.00256.x
61. Vallejo AN. Immunological hurdles of ageing: indispensable research of the human model. Ageing Res Rev (2011) 10(3):315–8. doi:10.1016/j.arr.2011.01.005
62. Vallejo AN, Nestel AR, Schirmer M, Weyand CM, Goronzy JJ. Aging-related deficiency of CD28 expression in CD4+ T cells is associated with the loss of gene-specific nuclear factor binding activity. J Biol Chem (1998) 273(14):8119–29. doi:10.1074/jbc.273.14.8119
63. Vallejo AN, Bryl E, Klarskov K, Naylor S, Weyand CM, Goronzy JJ. Molecular basis for the loss of CD28 expression in senescent T cells. J Biol Chem (2002) 277(49):46940–9. doi:10.1074/jbc.M207352200
64. Bryl E, Vallejo AN, Weyand CM, Goronzy JJ. Down-regulation of CD28 expression by TNF-alpha. J Immunol (2001) 167(6):3231–8. doi:10.4049/jimmunol.167.6.3231
65. Lewis DE, Merched-Sauvage M, Goronzy JJ, Weyand CM, Vallejo AN. Tumor necrosis factor-alpha and CD80 modulate CD28 expression through a similar mechanism of T-cell receptor-independent inhibition of transcription. J Biol Chem (2004) 279(28):29130–8. doi:10.1074/jbc.M402194200
66. Spaulding C, Guo W, Effros RB. Resistance to apoptosis in human CD8+ T cells that reach replicative senescence after multiple rounds of antigen-specific proliferation. Exp Gerontol (1999) 34(5):633–44. doi:10.1016/S0531-5565(99)00033-9
67. Vallejo AN, Schirmer M, Weyand CM, Goronzy JJ. Clonality and longevity of CD4+CD28null T cells are associated with defects in apoptotic pathways. J Immunol (2000) 165(11):6301–7. doi:10.4049/jimmunol.165.11.6301
68. Wallace DL, Masters JE, De Lara CM, Henson SM, Worth A, Zhang Y, et al. Human cytomegalovirus-specific CD8(+) T-cell expansions contain long-lived cells that retain functional capacity in both young and elderly subjects. Immunology (2011) 132(1):27–38. doi:10.1111/j.1365-2567.2010.03334.x
69. Tarazona R, DelaRosa O, Alonso C, Ostos B, Espejo J, Pena J, et al. Increased expression of NK cell markers on T lymphocytes in aging and chronic activation of the immune system reflects the accumulation of effector/senescent T cells. Mech Ageing Dev (2000) 121(1–3):77–88. doi:10.1016/S0047-6374(00)00199-8
70. Vallejo AN, Hamel DL Jr, Mueller RG, Ives DG, Michel JJ, Boudreau RM, et al. NK-like T cells and plasma cytokines, but not anti-viral serology, define immune fingerprints of resilience and mild disability in exceptional aging. PLoS One (2011) 6(10):e26558. doi:10.1371/journal.pone.0026558
71. Snyder MR, Muegge LO, Offord C, O’Fallon WM, Bajzer Z, Weyand CM, et al. Formation of the killer Ig-like receptor repertoire on CD4+CD28null T cells. J Immunol (2002) 168(8):3839–46. doi:10.4049/jimmunol.168.8.3839
72. Uhrberg M, Valiante NM, Young NT, Lanier LL, Phillips JH, Parham P. The repertoire of killer cell Ig-like receptor and CD94:NKG2A receptors in T cells: clones sharing identical alpha beta TCR rearrangement express highly diverse killer cell Ig-like receptor patterns. J Immunol (2001) 166(6):3923–32. doi:10.4049/jimmunol.166.6.3923
73. Xu J, Vallejo AN, Jiang Y, Weyand CM, Goronzy JJ. Distinct transcriptional control mechanisms of killer immunoglobulin-like receptors in natural killer (NK) and in T cells. J Biol Chem (2005) 280(25):24277–85. doi:10.1074/jbc.M500727200
74. Li G, Weyand CM, Goronzy JJ. Epigenetic mechanisms of age-dependent KIR2DL4 expression in T cells. J Leukoc Biol (2008) 84(3):824–34. doi:10.1189/jlb.0807583
75. Li G, Yu M, Weyand CM, Goronzy JJ. Epigenetic regulation of killer immunoglobulin-like receptor expression in T cells. Blood (2009) 114(16):3422–30. doi:10.1182/blood-2009-01-200170
76. Strickland FM, Patel D, Khanna D, Somers E, Robida AM, Pihalja M, et al. Characterisation of an epigenetically altered CD4(+) CD28(+) Kir(+) T cell subset in autoimmune rheumatic diseases by multiparameter flow cytometry. Lupus Sci Med (2016) 3(1):e000147. doi:10.1136/lupus-2016-000147
77. Young NT, Uhrberg M, Phillips JH, Lanier LL, Parham P. Differential expression of leukocyte receptor complex-encoded Ig-like receptors correlates with the transition from effector to memory CTL. J Immunol (2001) 166(6):3933–41. doi:10.4049/jimmunol.166.6.3933
78. Almehmadi M, Flanagan BF, Khan N, Alomar S, Christmas SE. Increased numbers and functional activity of CD56(+) T cells in healthy cytomegalovirus positive subjects. Immunology (2014) 142(2):258–68. doi:10.1111/imm.12250
79. Hooper M, Kallas EG, Coffin D, Campbell D, Evans TG, Looney RJ. Cytomegalovirus seropositivity is associated with the expansion of CD4+CD28- and CD8+CD28- T cells in rheumatoid arthritis. J Rheumatol (1999) 26(7):1452–7.
80. Wikby A, Ferguson F, Forsey R, Thompson J, Strindhall J, Lofgren S, et al. An immune risk phenotype, cognitive impairment, and survival in very late life: impact of allostatic load in Swedish octogenarian and nonagenarian humans. J Gerontol A Biol Sci Med Sci (2005) 60(5):556–65. doi:10.1093/gerona/60.5.556
81. Ouyang Q, Wagner WM, Voehringer D, Wikby A, Klatt T, Walter S, et al. Age-associated accumulation of CMV-specific CD8+ T cells expressing the inhibitory killer cell lectin-like receptor G1 (KLRG1). Exp Gerontol (2003) 38(8):911–20. doi:10.1016/S0531-5565(03)00134-7
82. Ouyang Q, Wagner WM, Wikby A, Walter S, Aubert G, Dodi AI, et al. Large numbers of dysfunctional CD8+ T lymphocytes bearing receptors for a single dominant CMV epitope in the very old. J Clin Immunol (2003) 23(4):247–57. doi:10.1023/A:1024580531705
83. Ouyang Q, Wagner WM, Zheng W, Wikby A, Remarque EJ, Pawelec G. Dysfunctional CMV-specific CD8(+) T cells accumulate in the elderly. Exp Gerontol (2004) 39(4):607–13. doi:10.1016/j.exger.2003.11.016
84. Bate SL, Dollard SC, Cannon MJ. Cytomegalovirus seroprevalence in the United States: the national health and nutrition examination surveys, 1988-2004. Clin Infect Dis (2010) 50(11):1439–47. doi:10.1086/652438
85. Leng SX, Li H, Xue QL, Tian J, Yang X, Ferrucci L, et al. Association of detectable cytomegalovirus (CMV) DNA in monocytes rather than positive CMV IgG serology with elevated neopterin levels in community-dwelling older adults. Exp Gerontol (2011) 46(8):679–84. doi:10.1016/j.exger.2011.04.002
86. Lachmann R, Bajwa M, Vita S, Smith H, Cheek E, Akbar A, et al. Polyfunctional T cells accumulate in large human cytomegalovirus-specific T cell responses. J Virol (2012) 86(2):1001–9. doi:10.1128/JVI.00873-11
87. Gehrz RC, Rutzick SR. Cytomegalovirus (CMV)-specific lysis of CMV-infected target cells can be mediated by both NK-like and virus-specific cytotoxic T lymphocytes. Clin Exp Immunol (1985) 61(1):80–9.
88. van Bergen J, Thompson A, van der Slik A, Ottenhoff TH, Gussekloo J, Koning F. Phenotypic and functional characterization of CD4 T cells expressing killer Ig-like receptors. J Immunol (2004) 173(11):6719–26. doi:10.4049/jimmunol.173.11.6719
89. Fann M, Chiu WK, Wood WH III, Levine BL, Becker KG, Weng NP. Gene expression characteristics of CD28null memory phenotype CD8+ T cells and its implication in T-cell aging. Immunol Rev (2005) 205:190–206. doi:10.1111/j.0105-2896.2005.00262.x
90. Peralbo E, Alonso C, Solana R. Invariant NKT and NKT-like lymphocytes: two different T cell subsets that are differentially affected by ageing. Exp Gerontol (2007) 42(8):703–8. doi:10.1016/j.exger.2007.05.002
91. Brenchley JM, Karandikar NJ, Betts MR, Ambrozak DR, Hill BJ, Crotty LE, et al. Expression of CD57 defines replicative senescence and antigen-induced apoptotic death of CD8+ T cells. Blood (2003) 101(7):2711–20. doi:10.1182/blood-2002-07-2103
92. Lee SA, Sinclair E, Hatano H, Hsue PY, Epling L, Hecht FM, et al. Impact of HIV on CD8+ T cell CD57 expression is distinct from that of CMV and aging. PLoS One (2014) 9(2):e89444. doi:10.1371/journal.pone.0089444
93. Henson SM, Franzese O, Macaulay R, Libri V, Azevedo RI, Kiani-Alikhan S, et al. KLRG1 signaling induces defective Akt (ser473) phosphorylation and proliferative dysfunction of highly differentiated CD8+ T cells. Blood (2009) 113(26):6619–28. doi:10.1182/blood-2009-01-199588
94. Bandres E, Merino J, Vazquez B, Inoges S, Moreno C, Subira ML, et al. The increase of IFN-gamma production through aging correlates with the expanded CD8(+high)CD28(-)CD57(+) subpopulation. Clin Immunol (2000) 96(3):230–5. doi:10.1006/clim.2000.4894
95. Campos C, Pera A, Sanchez-Correa B, Alonso C, Lopez-Fernandez I, Morgado S, et al. Effect of age and CMV on NK cell subpopulations. Exp Gerontol (2014) 54:130–7. doi:10.1016/j.exger.2014.01.008
96. Lopez-Verges S, Milush JM, Pandey S, York VA, Arakawa-Hoyt J, Pircher H, et al. CD57 defines a functionally distinct population of mature NK cells in the human CD56dimCD16+ NK-cell subset. Blood (2010) 116(19):3865–74. doi:10.1182/blood-2010-04-282301
97. Fulop T, Le Page A, Garneau H, Azimi N, Baehl S, Dupuis G, et al. Aging, immunosenescence and membrane rafts: the lipid connection. Longev Healthspan (2012) 1:6. doi:10.1186/2046-2395-1-6
98. Goronzy JJ, Li G, Yu M, Weyand CM. Signaling pathways in aged T cells – a reflection of T cell differentiation, cell senescence and host environment. Semin Immunol (2012) 24(5):365–72. doi:10.1016/j.smim.2012.04.003
99. Chen Y, Gorelik GJ, Strickland FM, Richardson BC. Decreased ERK and JNK signaling contribute to gene overexpression in “senescent” CD4+CD28- T cells through epigenetic mechanisms. J Leukoc Biol (2010) 87(1):137–45. doi:10.1189/jlb.0809562
100. Esser MT, Marchese RD, Kierstead LS, Tussey LG, Wang F, Chirmule N, et al. Memory T cells and vaccines. Vaccine (2003) 21(5–6):419–30. doi:10.1016/S0264-410X(02)00407-3
101. Wahid R, Cannon MJ, Chow M. Virus-specific CD4+ and CD8+ cytotoxic T-cell responses and long-term T-cell memory in individuals vaccinated against polio. J Virol (2005) 79(10):5988–95. doi:10.1128/JVI.79.10.5988-5995.2005
102. Kared H, Camous X, Larbi A. T cells and their cytokines in persistent stimulation of the immune system. Curr Opin Immunol (2014) 29:79–85. doi:10.1016/j.coi.2014.05.003
103. Bukczynski J, Wen T, Watts TH. Costimulation of human CD28- T cells by 4-1BB ligand. Eur J Immunol (2003) 33(2):446–54. doi:10.1002/immu.200310020
104. Dumitriu IE, Baruah P, Finlayson CJ, Loftus IM, Antunes RF, Lim P, et al. High levels of costimulatory receptors OX40 and 4-1BB characterize CD4+CD28null T cells in patients with acute coronary syndrome. Circ Res (2012) 110(6):857–69. doi:10.1161/CIRCRESAHA.111.261933
105. Hodge G, Mukaro V, Reynolds PN, Hodge S. Role of increased CD8/CD28(null) T cells and alternative co-stimulatory molecules in chronic obstructive pulmonary disease. Clin Exp Immunol (2011) 166(1):94–102. doi:10.1111/j.1365-2249.2011.04455.x
106. Lee WW, Yang ZZ, Li G, Weyand CM, Goronzy JJ. Unchecked CD70 expression on T cells lowers threshold for T cell activation in rheumatoid arthritis. J Immunol (2007) 179(4):2609–15. doi:10.4049/jimmunol.179.4.2609
107. Leitner J, Herndler-Brandstetter D, Zlabinger GJ, Grubeck-Loebenstein B, Steinberger P. CD58/CD2 is the primary costimulatory pathway in human CD28-CD8+ T cells. J Immunol (2015) 195(2):477–87. doi:10.4049/jimmunol.1401917
108. Namekawa T, Wagner UG, Goronzy JJ, Weyand CM. Functional subsets of CD4 T cells in rheumatoid synovitis. Arthritis Rheum (1998) 41(12):2108–16. doi:10.1002/1529-0131(199812)41:12<2108::AID-ART5>3.3.CO;2-H
109. Wagner UG, Kurtin PJ, Wahner A, Brackertz M, Berry DJ, Goronzy JJ, et al. The role of CD8+ CD40L+ T cells in the formation of germinal centers in rheumatoid synovitis. J Immunol (1998) 161(11):6390–7.
110. de Magalhaes JP, Church GM. Genomes optimize reproduction: aging as a consequence of the developmental program. Physiology (2005) 20:252–9. doi:10.1152/physiol.00010.2005
111. McElwee JJ, Schuster E, Blanc E, Piper MD, Thomas JH, Patel DS, et al. Evolutionary conservation of regulated longevity assurance mechanisms. Genome Biol (2007) 8(7):R132. doi:10.1186/gb-2007-8-7-r132
112. Zwaan BJ. Linking development and aging. Sci Aging Knowledge Environ (2003) 2003(47):e32. doi:10.1126/sageke.2003.47.pe32
113. Kuningas M, Mooijaart SP, van Heemst D, Zwaan BJ, Slagboom PE, Westendorp RG. Genes encoding longevity: from model organisms to humans. Aging Cell (2008) 7(2):270–80. doi:10.1111/j.1474-9726.2008.00366.x
114. Sanders AE, Wang C, Katz M, Derby CA, Barzilai N, Ozelius L, et al. Association of a functional polymorphism in the cholesteryl ester transfer protein (CETP) gene with memory decline and incidence of dementia. JAMA (2010) 303(2):150–8. doi:10.1001/jama.2009.1988
115. Barzilai N, Atzmon G, Derby CA, Bauman JM, Lipton RB. A genotype of exceptional longevity is associated with preservation of cognitive function. Neurology (2006) 67(12):2170–5. doi:10.1212/01.wnl.0000249116.50854.65
116. Pawlikowska L, Hu D, Huntsman S, Sung A, Chu C, Chen J, et al. Association of common genetic variation in the insulin/IGF1 signaling pathway with human longevity. Aging Cell (2009) 8(4):460–72. doi:10.1111/j.1474-9726.2009.00493.x
117. Hawkes K. Grandmothers and the evolution of human longevity. Am J Hum Biol (2003) 15(3):380–400. doi:10.1002/ajhb.10156
118. Hawkes K. Genomic evidence for the evolution of human postmenopausal longevity. Proc Natl Acad Sci U S A (2016) 113(1):17–8. doi:10.1073/pnas.1522936113
119. Schwarz F, Springer SA, Altheide TK, Varki NM, Gagneux P, Varki A. Human-specific derived alleles of CD33 and other genes protect against postreproductive cognitive decline. Proc Natl Acad Sci U S A (2016) 113(1):74–9. doi:10.1073/pnas.1517951112
120. Turner AK, Begon M, Jackson JA, Bradley JE, Paterson S. Genetic diversity in cytokines associated with immune variation and resistance to multiple pathogens in a natural rodent population. PLoS Genet (2011) 7(10):e1002343. doi:10.1371/journal.pgen.1002343
121. Kubinak JL, Ruff JS, Hyzer CW, Slev PR, Potts WK. Experimental viral evolution to specific host MHC genotypes reveals fitness and virulence trade-offs in alternative MHC types. Proc Natl Acad Sci U S A (2012) 109(9):3422–7. doi:10.1073/pnas.1112633109
122. Siddle KJ, Quintana-Murci L. The Red Queen’s long race: human adaptation to pathogen pressure. Curr Opin Genet Dev (2014) 29:31–8. doi:10.1016/j.gde.2014.07.004
123. Vallejo AN. Immune remodeling: lessons from repertoire alterations during chronological aging and in immune-mediated disease. Trends Mol Med (2007) 13(3):94–102. doi:10.1016/j.molmed.2007.01.005
124. Haynes BF, Sempowski GD, Wells AF, Hale LP. The human thymus during aging. Immunol Res (2000) 22(2–3):253–61. doi:10.1385/IR:22:2-3:253
125. Zonios DI, Falloon J, Bennett JE, Shaw PA, Chaitt D, Baseler MW, et al. Idiopathic CD4+ lymphocytopenia: natural history and prognostic factors. Blood (2008) 112(2):287–94. doi:10.1182/blood-2007-12-127878
126. Scott-Algara D, Balabanian K, Chakrabarti LA, Mouthon L, Dromer F, Didier C, et al. Idiopathic CD4+ T-cell lymphocytopenia is associated with impaired membrane expression of the chemokine receptor CXCR4. Blood (2010) 115(18):3708–17. doi:10.1182/blood-2009-02-202796
127. Chandra S, Kronenberg M. Activation and function of iNKT and MAIT cells. Adv Immunol (2015) 127:145–201. doi:10.1016/bs.ai.2015.03.003
128. Pei B, Vela JL, Zajonc D, Kronenberg M. Interplay between carbohydrate and lipid in recognition of glycolipid antigens by natural killer T cells. Ann N Y Acad Sci (2012) 1253:68–79. doi:10.1111/j.1749-6632.2011.06435.x
129. Le Garff-Tavernier M, Beziat V, Decocq J, Siguret V, Gandjbakhch F, Pautas E, et al. Human NK cells display major phenotypic and functional changes over the life span. Aging Cell (2010) 9(4):527–35. doi:10.1111/j.1474-9726.2010.00584.x
130. Pilbeam K, Basse P, Brossay L, Vujanovic N, Gerstein R, Vallejo AN, et al. The ontogeny and fate of NK cells marked by permanent DNA rearrangements. J Immunol (2008) 180(3):1432–41. doi:10.4049/jimmunol.180.3.1432
131. Miskov-Zivanov N, Turner MS, Kane LP, Morel PA, Faeder JR. The duration of T cell stimulation is a critical determinant of cell fate and plasticity. Sci Signal (2013) 6(300):ra97. doi:10.1126/scisignal.2004217
132. Slack M, Wang T, Wang R. T cell metabolic reprogramming and plasticity. Mol Immunol (2015) 68(2 Pt C):507–12. doi:10.1016/j.molimm.2015.07.036
133. DuPage M, Bluestone JA. Harnessing the plasticity of CD4(+) T cells to treat immune-mediated disease. Nat Rev Immunol (2016) 16(3):149–63. doi:10.1038/nri.2015.18
134. Campisi J. Aging, cellular senescence, and cancer. Annu Rev Physiol (2013) 75:685–705. doi:10.1146/annurev-physiol-030212-183653
135. Campisi J, Robert L. Cell senescence: role in aging and age-related diseases. Interdiscip Top Gerontol (2014) 39:45–61. doi:10.1159/000358899
136. Collado M, Blasco MA, Serrano M. Cellular senescence in cancer and aging. Cell (2007) 130(2):223–33. doi:10.1016/j.cell.2007.07.003
137. Rodier F, Campisi J. Four faces of cellular senescence. J Cell Biol (2011) 192(4):547–56. doi:10.1083/jcb.201009094
138. Adams PD. Healing and hurting: molecular mechanisms, functions, and pathologies of cellular senescence. Mol Cell (2009) 36(1):2–14. doi:10.1016/j.molcel.2009.09.021
139. Munoz-Espin D, Serrano M. Cellular senescence: from physiology to pathology. Nat Rev Mol Cell Biol (2014) 15(7):482–96. doi:10.1038/nrm3823
140. Triana-Martinez F, Pedraza-Vazquez G, Maciel-Baron LA, Konigsberg M. Reflections on the role of senescence during development and aging. Arch Biochem Biophys (2016) 598:40–9. doi:10.1016/j.abb.2016.04.004
141. Munoz-Espin D, Canamero M, Maraver A, Gomez-Lopez G, Contreras J, Murillo-Cuesta S, et al. Programmed cell senescence during mammalian embryonic development. Cell (2013) 155(5):1104–18. doi:10.1016/j.cell.2013.10.019
142. Storer M, Mas A, Robert-Moreno A, Pecoraro M, Ortells MC, Di Giacomo V, et al. Senescence is a developmental mechanism that contributes to embryonic growth and patterning. Cell (2013) 155(5):1119–30. doi:10.1016/j.cell.2013.10.041
143. Barral S, Cosentino S, Costa R, Andersen SL, Christensen K, Eckfeldt JH, et al. Exceptional memory performance in the Long Life Family Study. Neurobiol Aging (2013) 34(11):2445–8. doi:10.1016/j.neurobiolaging.2013.05.002
144. Miller LS, Mitchell MB, Woodard JL, Davey A, Martin P, Poon LW, et al. Cognitive performance in centenarians and the oldest old: norms from the Georgia Centenarian Study. Neuropsychol Dev Cogn B Aging Neuropsychol Cogn (2010) 17(5):575–90. doi:10.1080/13825585.2010.481355
145. Kaup AR, Nettiksimmons J, Harris TB, Sink KM, Satterfield S, Metti AL, et al. Cognitive resilience to apolipoprotein E epsilon4: contributing factors in Black and White older adults. JAMA Neurol (2015) 72(3):340–8. doi:10.1001/jamaneurol.2014.3978
146. Barral S, Cosentino S, Christensen K, Newman AB, Perls TT, Province MA, et al. Common genetic variants on 6q24 associated with exceptional episodic memory performance in the elderly. JAMA Neurol (2014) 71(12):1514–9. doi:10.1001/jamaneurol.2014.1663
147. Bonafe M, Olivieri F. Genetic polymorphism in long-lived people: cues for the presence of an insulin/IGF-pathway-dependent network affecting human longevity. Mol Cell Endocrinol (2009) 299(1):118–23. doi:10.1016/j.mce.2008.10.038
148. Carru C, Pes GM, Deiana L, Baggio G, Franceschi C, Lio D, et al. Association between the HFE mutations and longevity: a study in Sardinian population. Mech Ageing Dev (2003) 124(4):529–32. doi:10.1016/S0047-6374(03)00032-0
149. Cupples LA, Arruda HT, Benjamin EJ, D’Agostino RB Sr, Demissie S, DeStefano AL, et al. The Framingham Heart Study 100K SNP genome-wide association study resource: overview of 17 phenotype working group reports. BMC Med Genet (2007) 8(Suppl 1):S1. doi:10.1186/1471-2350-8-S1-S1
150. Lotrich FE, Albusaysi S, Ferrell RE. Brain-derived neurotrophic factor serum levels and genotype: association with depression during interferon-alpha treatment. Neuropsychopharmacology (2013) 38(6):985–95. doi:10.1038/npp.2012.263
151. Carlson MC, Erickson KI, Kramer AF, Voss MW, Bolea N, Mielke M, et al. Evidence for neurocognitive plasticity in at-risk older adults: the experience corps program. J Gerontol A Biol Sci Med Sci (2009) 64(12):1275–82. doi:10.1093/gerona/glp117
152. Erickson KI, Gildengers AG, Butters MA. Physical activity and brain plasticity in late adulthood. Dialogues Clin Neurosci (2013) 15(1):99–108.
153. Frandin K, Gronstedt H, Helbostad JL, Bergland A, Andresen M, Puggaard L, et al. Long-term effects of individually tailored physical training and activity on physical function, well-being and cognition in Scandinavian nursing home residents: a randomized controlled trial. Gerontology (2016) 62(6):571–80. doi:10.1159/000443611
154. Rejeski WJ, Axtell R, Fielding R, Katula J, King AC, Manini TM, et al. Promoting physical activity for elders with compromised function: the lifestyle interventions and independence for elders (LIFE) study physical activity intervention. Clin Interv Aging (2013) 8:1119–31. doi:10.2147/CIA.S49737
155. Berchicci M, Lucci G, Di Russo F. Benefits of physical exercise on the aging brain: the role of the prefrontal cortex. J Gerontol A Biol Sci Med Sci (2013) 68(11):1337–41. doi:10.1093/gerona/glt094
156. Burzynska AZ, Wong CN, Voss MW, Cooke GE, Gothe NP, Fanning J, et al. Physical activity is linked to greater moment-to-moment variability in spontaneous brain activity in older adults. PLoS One (2015) 10(8):e0134819. doi:10.1371/journal.pone.0134819
157. Campos C, Rocha NB, Lattari E, Paes F, Nardi AE, Machado S. Exercise-induced neuroprotective effects on neurodegenerative diseases: the key role of trophic factors. Expert Rev Neurother (2016) 16(6):723–34. doi:10.1080/14737175.2016.1179582
158. Eggenberger P, Wolf M, Schumann M, de Bruin ED. Exergame and balance training modulate prefrontal brain activity during walking and enhance executive function in older adults. Front Aging Neurosci (2016) 8:66. doi:10.3389/fnagi.2016.00066
159. Erickson KI, Raji CA, Lopez OL, Becker JT, Rosano C, Newman AB, et al. Physical activity predicts gray matter volume in late adulthood: the Cardiovascular Health Study. Neurology (2010) 75(16):1415–22. doi:10.1212/WNL.0b013e3181f88359
160. Voss MW, Prakash RS, Erickson KI, Basak C, Chaddock L, Kim JS, et al. Plasticity of brain networks in a randomized intervention trial of exercise training in older adults. Front Aging Neurosci (2010) 2:32. doi:10.3389/fnagi.2010.00032
161. Venkatraman VK, Aizenstein H, Guralnik J, Newman AB, Glynn NW, Taylor C, et al. Executive control function, brain activation and white matter hyperintensities in older adults. Neuroimage (2009) 49(4):3436–42. doi:10.1016/j.neuroimage.2009.11.019
162. Gomez-Pinilla F, Zhuang Y, Feng J, Ying Z, Fan G. Exercise impacts brain-derived neurotrophic factor plasticity by engaging mechanisms of epigenetic regulation. Eur J Neurosci (2011) 33(3):383–90. doi:10.1111/j.1460-9568.2010.07508.x
163. Kobilo T, Guerrieri D, Zhang Y, Collica SC, Becker KG, van Praag H. AMPK agonist AICAR improves cognition and motor coordination in young and aged mice. Learn Mem (2014) 21(2):119–26. doi:10.1101/lm.033332.113
164. Kohman RA, Rodriguez-Zas SL, Southey BR, Kelley KW, Dantzer R, Rhodes JS. Voluntary wheel running reverses age-induced changes in hippocampal gene expression. PLoS One (2011) 6(8):e22654. doi:10.1371/journal.pone.0022654
165. Neidl R, Schneider A, Bousiges O, Majchrzak M, Barbelivien A, de Vasconcelos AP, et al. Late-life environmental enrichment induces acetylation events and nuclear factor kappaB-dependent regulations in the hippocampus of aged rats showing improved plasticity and learning. J Neurosci (2016) 36(15):4351–61. doi:10.1523/JNEUROSCI.3239-15.2016
166. Schrager MA, Roth SM, Ferrell RE, Metter EJ, Russek-Cohen E, Lynch NA, et al. Insulin-like growth factor-2 genotype, fat-free mass, and muscle performance across the adult life span. J Appl Physiol (2004) 97(6):2176–83. doi:10.1152/japplphysiol.00985.2003
167. Hortobagyi T, Rider P, Gruber AH, DeVita P. Age and muscle strength mediate the age-related biomechanical plasticity of gait. Eur J Appl Physiol (2016) 116(4):805–14. doi:10.1007/s00421-015-3312-8
168. Konopka AR, Trappe TA, Jemiolo B, Trappe SW, Harber MP. Myosin heavy chain plasticity in aging skeletal muscle with aerobic exercise training. J Gerontol A Biol Sci Med Sci (2011) 66(8):835–41. doi:10.1093/gerona/glr088
169. Reeves ND, Maganaris CN, Narici MV. Plasticity of dynamic muscle performance with strength training in elderly humans. Muscle Nerve (2005) 31(3):355–64. doi:10.1002/mus.20275
170. Urso ML, Fiatarone Singh MA, Ding W, Evans WJ, Cosmas AC, Manfredi TG. Exercise training effects on skeletal muscle plasticity and IGF-1 receptors in frail elders. Age (2005) 27(2):117–25. doi:10.1007/s11357-005-1629-7
171. Snijders T, Nederveen JP, McKay BR, Joanisse S, Verdijk LB, van Loon LJ, et al. Satellite cells in human skeletal muscle plasticity. Front Physiol (2015) 6:283. doi:10.3389/fphys.2015.00283
172. Anderson JE. The satellite cell as a companion in skeletal muscle plasticity: currency, conveyance, clue, connector and colander. J Exp Biol (2006) 209(Pt 12):2276–92. doi:10.1242/jeb.02088
173. Newman AB, Murabito JM. The epidemiology of longevity and exceptional survival. Epidemiol Rev (2013) 35:181–97. doi:10.1093/epirev/mxs013
174. Newman AB, Boudreau RM, Naydeck BL, Fried LF, Harris TB. A physiologic index of comorbidity: relationship to mortality and disability. J Gerontol A Biol Sci Med Sci (2008) 63(6):603–9. doi:10.1093/gerona/63.6.603
175. Rosano C, Venkatraman VK, Guralnik J, Newman AB, Glynn NW, Launer L, et al. Psychomotor speed and functional brain MRI 2 years after completing a physical activity treatment. J Gerontol A Biol Sci Med Sci (2010) 65(6):639–47. doi:10.1093/gerona/glq038
176. Studenski S, Perera S, Patel K, Rosano C, Faulkner K, Inzitari M, et al. Gait speed and survival in older adults. JAMA (2011) 305(1):50–8. doi:10.1001/jama.2010.1923
177. Robertson DA, Savva GM, Coen RF, Kenny RA. Cognitive function in the prefrailty and frailty syndrome. J Am Geriatr Soc (2014) 62(11):2118–24. doi:10.1111/jgs.13111
178. Burke GL, Arnold AM, Bild DE, Cushman M, Fried LP, Newman A, et al. Factors associated with healthy aging: the cardiovascular health study. J Am Geriatr Soc (2001) 49(3):254–62. doi:10.1046/j.1532-5415.2001.4930254.x
179. Tell GS, Fried LP, Hermanson B, Manolio TA, Newman AB, Borhani NO. Recruitment of adults 65 years and older as participants in the Cardiovascular Health Study. Ann Epidemiol (1993) 3(4):358–66. doi:10.1016/1047-2797(93)90062-9
180. Teng EL, Chui HC. The Modified Mini-Mental State (3MS) examination. J Clin Psychiatry (1987) 48(8):314–8.
181. Lawton MP, Brody EM. Assessment of older people: self-maintaining and instrumental activities of daily living. Gerontologist (1969) 9(3):179–86. doi:10.1093/geront/9.3_Part_1.179
182. Michel JJ, Turesson C, Lemster B, Atkins SR, Iclozan C, Bongartz T, et al. CD56-expressing T cells that have features of senescence are expanded in rheumatoid arthritis. Arthritis Rheum (2007) 56(1):43–57. doi:10.1002/art.22310
183. Bergstrom I, Backteman K, Lundberg A, Ernerudh J, Jonasson L. Persistent accumulation of interferon-gamma-producing CD8+CD56+ T cells in blood from patients with coronary artery disease. Atherosclerosis (2012) 224(2):515–20. doi:10.1016/j.atherosclerosis.2012.07.033
184. Hamzaoui A, Chaouch N, Grairi H, Ammar J, Hamzaoui K. Inflammatory process of CD8+ CD28- T cells in induced sputum from asthmatic patients. Mediators Inflamm (2005) 2005(3):160–6. doi:10.1155/MI.2005.160
185. Shimamoto M, Ueno Y, Tanaka S, Onitake T, Hanaoka R, Yoshioka K, et al. Selective decrease in colonic CD56(+) T and CD161(+) T cells in the inflamed mucosa of patients with ulcerative colitis. World J Gastroenterol (2007) 13(45):5995–6002. doi:10.3748/wjg.13.5995
186. Yonekura K, Ichida T, Sato K, Yamagiwa S, Uchida M, Sugahara S, et al. Liver-infiltrating CD56 positive T lymphocytes in hepatitis C virus infection. Liver (2000) 20(5):357–65. doi:10.1034/j.1600-0676.2000.020005357.x
187. El-Gazzar A, Cai X, Reeves RS, Dai Z, Caballero-Benitez A, McDonald DL, et al. Effects on tumor development and metastatic dissemination by the NKG2D lymphocyte receptor expressed on cancer cells. Oncogene (2014) 33(41):4932–40. doi:10.1038/onc.2013.435
188. Cai X, Dai Z, Reeves RS, Caballero-Benitez A, Duran KL, Delrow JJ, et al. Autonomous stimulation of cancer cell plasticity by the human NKG2D lymphocyte receptor coexpressed with its ligands on cancer cells. PLoS One (2014) 9(10):e108942. doi:10.1371/journal.pone.0108942
189. Capraru D, Muller A, Csernok E, Gross WL, Holl-Ulrich K, Northfield J, et al. Expansion of circulating NKG2D+ effector memory T-cells and expression of NKG2D-ligand MIC in granulomaous lesions in Wegener’s granulomatosis. Clin Immunol (2008) 127(2):144–50. doi:10.1016/j.clim.2007.12.004
190. Groh V, Bruhl A, El-Gabalawy H, Nelson JL, Spies T. Stimulation of T cell autoreactivity by anomalous expression of NKG2D and its MIC ligands in rheumatoid arthritis. Proc Natl Acad Sci U S A (2003) 100(16):9452–7. doi:10.1073/pnas.1632807100
191. Hanaoka N, Jabri B, Dai Z, Ciszewski C, Stevens AM, Yee C, et al. NKG2D initiates caspase-mediated CD3zeta degradation and lymphocyte receptor impairments associated with human cancer and autoimmune disease. J Immunol (2010) 185(10):5732–42. doi:10.4049/jimmunol.1002092
192. Tang F, Chen Z, Ciszewski C, Setty M, Solus J, Tretiakova M, et al. Cytosolic PLA2 is required for CTL-mediated immunopathology of celiac disease via NKG2D and IL-15. J Exp Med (2009) 206(3):707–19. doi:10.1084/jem.20071887
193. Kalliolias GD, Ivashkiv LB. TNF biology, pathogenic mechanisms and emerging therapeutic strategies. Nat Rev Rheumatol (2016) 12(1):49–62. doi:10.1038/nrrheum.2015.169
194. Bryl E, Vallejo AN, Matteson EL, Witkowski JM, Weyand CM, Goronzy JJ. Modulation of CD28 expression with anti-tumor necrosis factor alpha therapy in rheumatoid arthritis. Arthritis Rheum (2005) 52(10):2996–3003. doi:10.1002/art.21353
195. Davila E, Kang YM, Park YW, Sawai H, He X, Pryshchep S, et al. Cell-based immunotherapy with suppressor CD8+ T cells in rheumatoid arthritis. J Immunol (2005) 174(11):7292–301. doi:10.4049/jimmunol.174.11.7292
196. Dai Z, Turtle CJ, Booth GC, Riddell SR, Gooley TA, Stevens AM, et al. Normally occurring NKG2D+CD4+ T cells are immunosuppressive and inversely correlated with disease activity in juvenile-onset lupus. J Exp Med (2009) 206(4):793–805. doi:10.1084/jem.20081648
197. Cohavy O, Targan SR. CD56 marks an effector T cell subset in the human intestine. J Immunol (2007) 178(9):5524–32. doi:10.4049/jimmunol.178.9.5524
198. Groh V, Wu J, Yee C, Spies T. Tumour-derived soluble MIC ligands impair expression of NKG2D and T-cell activation. Nature (2002) 419(6908):734–8. doi:10.1038/nature01112
199. McAlpine SM, Issekutz TB, Marshall JS. Virus stimulation of human mast cells results in the recruitment of CD56(+) T cells by a mechanism dependent on CCR5 ligands. FASEB J (2012) 26(3):1280–9. doi:10.1096/fj.11-188979
200. Rong YH, Wan ZH, Song H, Li YL, Zhu B, Zang H, et al. Tim-3 expression on peripheral monocytes and CD3+CD16/CD56+natural killer-like T cells in patients with chronic hepatitis B. Tissue Antigens (2014) 83(2):76–81. doi:10.1111/tan.12278
201. Vallejo AN, Weyand CM, Goronzy JJ. T-cell senescence: a culprit of immune abnormalities in chronic inflammation and persistent infection. Trends Mol Med (2004) 10(3):119–24. doi:10.1016/j.molmed.2004.01.002
202. Hughes KA, Reynolds RM. Evolutionary and mechanistic theories of aging. Annu Rev Entomol (2005) 50:421–45. doi:10.1146/annurev.ento.50.071803.130409
Keywords: CD16, CD56, cell senescence, functional performance, immune remodeling, NKG2D, plasticity, TCR-independent
Citation: Michel JJ, Griffin P and Vallejo Abbe N (2016) Functionally Diverse NK-Like T Cells Are Effectors and Predictors of Successful Aging. Front. Immunol. 7:530. doi: 10.3389/fimmu.2016.00530
Received: 10 October 2016; Accepted: 10 November 2016;
Published: 24 November 2016
Edited by:
Fernando A. Arosa, University of Beira Interior, PortugalReviewed by:
Jacques A Nunes, Centre de Recherche en Cancerologie de Marseille, FranceCopyright: © 2016 Michel, Griffin and Vallejo. This is an open-access article distributed under the terms of the Creative Commons Attribution License (CC BY). The use, distribution or reproduction in other forums is permitted, provided the original author(s) or licensor are credited and that the original publication in this journal is cited, in accordance with accepted academic practice. No use, distribution or reproduction is permitted which does not comply with these terms.
*Correspondence: Abbe N. de Vallejo, andv26@pitt.edu
Disclaimer: All claims expressed in this article are solely those of the authors and do not necessarily represent those of their affiliated organizations, or those of the publisher, the editors and the reviewers. Any product that may be evaluated in this article or claim that may be made by its manufacturer is not guaranteed or endorsed by the publisher.
Research integrity at Frontiers
Learn more about the work of our research integrity team to safeguard the quality of each article we publish.