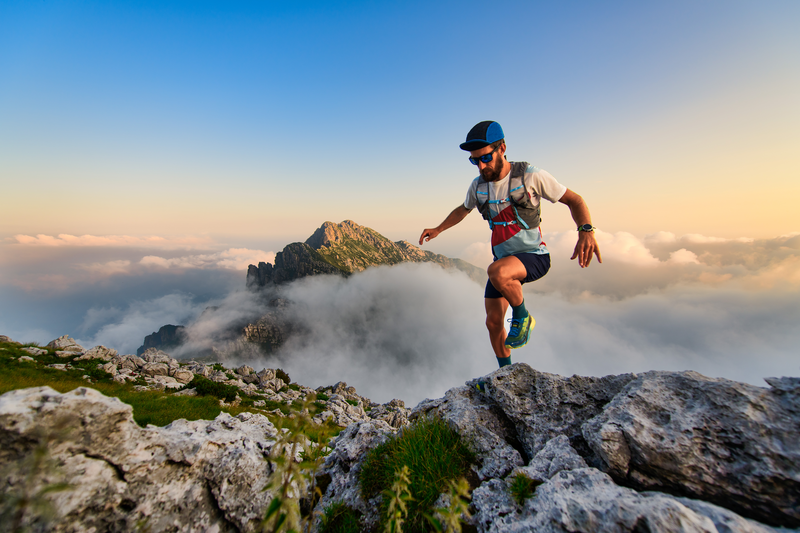
94% of researchers rate our articles as excellent or good
Learn more about the work of our research integrity team to safeguard the quality of each article we publish.
Find out more
MINI REVIEW article
Front. Immunol. , 29 April 2016
Sec. Vaccines and Molecular Therapeutics
Volume 7 - 2016 | https://doi.org/10.3389/fimmu.2016.00171
This article is part of the Research Topic HSPs - ambiguous mediators of immunity View all 10 articles
Intracellular residing heat shock proteins (HSPs) with a molecular weight of approximately 70 and 90 kDa function as molecular chaperones that assist folding/unfolding and transport of proteins across membranes and prevent protein aggregation after environmental stress. In contrast to normal cells, tumor cells have higher cytosolic heat shock protein 70 and Hsp90 levels, which contribute to tumor cell propagation, metastasis, and protection against apoptosis. In addition to their intracellular chaperoning functions, extracellular localized and membrane-bound HSPs have been found to play key roles in eliciting antitumor immune responses by acting as carriers for tumor-derived immunogenic peptides, as adjuvants for antigen presentation, or as targets for the innate immune system. The interaction of HSP–peptide complexes or peptide-free HSPs with receptors on antigen-presenting cells promotes the maturation of dendritic cells, results in an upregulation of major histocompatibility complex class I and class II molecules, induces secretion of pro- and anti-inflammatory cytokines, chemokines, and immune modulatory nitric oxides, and thus integrates adaptive and innate immune phenomena. Herein, we aim to recapitulate the history and current status of HSP-based immunotherapies and vaccination strategies in the treatment of cancer.
Heat shock proteins (HSPs) with the molecular weights of approximately 70 and 90 kDa have the capacity to stimulate antitumor immune responses either as carriers for antigenic peptides, which can be cross-presented by major histocompatibility complex (MHC) class I molecules, or as natural immunogens (1–3). Depending on the availability of ATP and ADP, members of the heat shock protein 70 (HSP70) family have the capacity to release and bind tumor-specific antigens, respectively. Following cross-presentation on MHC class I antigens, a CD8+ cytotoxic T cell response is initiated. Preclinical models revealed that vaccination with HSP–peptide complexes purified from tumor, but not normal cells, are able to mediate specific and protective immunity against autologous tumors. In recent years, a large number of receptors, including the alpha-2 macroglobulin receptor CD91 (4), lectin-like oxidized low-density lipoprotein receptor-1 (LOX-1) (5, 6), scavenger receptor expressed by endothelial cells-1 (SREC-1) (7), toll-like receptors-2/4 (TLRs-2/4) (8–10), their cofactors CD14 (11, 12), fasciclin EGF-like, laminin-type EGF-like and link domain-containing scavenger receptor-1 (FEEL-1), common lymphatic endothelial and vascular endothelial receptor-1 (Clever-1), stabilin-1 (Stab-1), and CD40 (13), have been found to be involved in the uptake of HSPs and HSP-chaperoned exogenous peptides into antigen-presenting cells (APCs).
Despite their high degree of homology certain HSP sequences are not conserved and thus act as immunogenes, which can be recognized as foreign by the host’s innate and adaptive immune system, especially when they are presented in a tissue/tumor-specific manner (14). In combination with pro-inflammatory cytokines, including interleukin 2 (IL-2), IL-12, or IL-15, the major stress-inducible Hsp70 (HSPA1A) or non-conserved sequences derived thereof has been found to activate the cytolytic, proliferative, and migratory capacity of natural killer (NK) cells (15). This activation was accompanied by an upregulated expression density of activatory C-type lectin receptors CD94/NKG2C and NKG2D on NK cells (16). Furthermore, an intra-tumoral infusion of free, recombinant Hsp70 has been shown to increase the infiltration of NK cells and CD8+ cytotoxic T cells into tumors and the secretion of interferon gamma (IFN-γ) (17, 18).
In 1986, the group of Old et al. firstly described a glycoprotein with a molecular weight of 96 kDa (gp96), which was found to act as a tumor rejection antigen. Gp96 was isolated from mouse fibrosarcomas that were chemically induced by the carcinogen methylcholanthrene A (1). Li and Srivastava (19) characterized gp96 as an ER-residing member of the HSP90 family, which contains an ATPase activity. In addition to gp96, members of the HSP70 family that also possess an ATPase domain appeared to be equally immunogenic for the adaptive immune system such as gp96 when tumor-specific antigens were bound to them (20, 21). Since ATP sepharose columns are widely used for the purification of HSP–peptide complexes from tumor cells, there is a risk that the immunogenic peptides are dissolved from the HSP–peptide complexes during purification due to their ATPase activity (22). Therefore, a novel purification method, which was based on ADP-affinity chromatography, has been established for the isolation of intact HSP–peptide complexes (23).
Immunogenic peptides chaperoned by gp96 and HSP70s were not only found to elicit specific immune responses against cancer (24, 25) but also against infectious diseases (26, 27), indicating the broad applicability of HSP-based vaccines. It was also found that following oxidative stress the immunogenicity of HSP-based vaccines was found to be increased (28). This finding might be explained, on the one hand, by the fact that the amount and the repertoire of immunogenic peptides might differ in stressed and non-stressed cells. On the other hand, it is possible that stress-inducible members of HSP families might be better qualified for chaperoning immunogenic peptides than their constitutively expressed correlates. Since HSP-chaperoned peptides only mediate protective immunity against autologous, but not allogeneic tumors (24), and HSP–peptide complexes eluted from healthy tissues were found to be inefficient in stimulating T cell-mediated immunity and was assumed that HSP-chaperoned peptides are tumor cell type specific.
Furthermore, an efficient rejection of tumors in preclinical models requires the presence of CD8+ T cells in the priming phase and that of CD4+ helper, CD8+ cytotoxic T cells, and M1 macrophages in the effector phase (21). Exogenous antigens, which are typically presented by MHC class II antigens, can be channeled by HSPs into the endogenous pathway and thus can be presented on MHC class I molecules (29). This HSP-mediated switch of peptides from the endogenous MHC class II to the MHC class I pathway is also termed as antigen cross-presentation (29–31).
For a while, the mechanism how exogenous HSP–peptide immune complexes are taken up by APCs remained elusive because HSP-specific receptors had not been identified and characterized. The group of Binder et al. classified the interaction of HSP–peptide complexes with APCs as specific and saturable. These attributes are typical for a receptor–ligand interaction (32). The same group was among the first who identified CD91 as a receptor for immunogenic peptides complexed with HSP90 and HSP70 families and for calreticulin (33). CD91, which is also termed low-density lipoprotein-related protein, was initially described as a receptor for alpha-2 macroglobulin (4). Until today, a large variety of different receptors, such as LOX-1 (6), SREC-1, FEEL-1, Clever-1, Stab-1 (5, 7, 34, 35), TLRs-2/4, and their cofactor CD14 (11, 12, 36) and CD40 (13), have been shown to be involved in the uptake and signaling of HSP70 and HSP90 complexes with APCs (37).
It is important to note that the capacity of HSPs or HSP–peptide complexes to elicit antitumor-specific immunity is highly dependent on the dose. Although low doses of HSP–peptide complexes have been found to be efficient in the stimulation of antitumor immune responses, a 5- to 10-fold higher dose than the optimal stimulatory dose turned out to be ineffective or even immunosuppressive (38). High doses of gp96–peptide complexes were found to induce immune tolerance and thus were applied to treat autoimmune diabetes and encephalomyelitis in preclinical models (39, 40). The mechanisms, which are involved in the induction of tolerance by HSP70s, have been found to be associated with TLR2 and TLR4. The TLR2/MyD88 signaling pathway, which is induced after binding of exosomal Hsp70 to TLRs, has been found to mediate protection of the myocardium against ischemic reperfusion injury (10), and the TLR4/ERK1,2/p38/MAPK pathway has been found to initiate pStat3-mediated immunosuppressive activity in myeloid-derived suppressor cells (9).
Based on the knowledge on the molecular characteristics and functions of HSPs and HSP–peptide-based vaccines, the stimulation of antitumor immune responses initiated clinical applications (41). Between 2000 and 2014, gp96 and HSP70–peptide-based vaccines derived from autologous tumor lysates were clinically applied in phase I to phase III clinical trials in different tumor entities including late stage melanoma (42) either alone or in combination with GM-CSF and IFN-γ (43–48), metastatic colon carcinoma (49), renal cell carcinoma (50), gastric carcinoma (51, 52), pancreatic carcinoma (53), chronic myeloid leukemia (54), and glioblastoma (55) (Table 1). The outcome of these trials showed the induction of immunological responses in a large number of patients treated with HSP–peptide complexes; however, clinical responses (CRs) were observed only in certain patient subgroups.
Heat shock protein 70 has been found to be overexpressed in tumor cells. Hsp70 is presented on the cell membrane of a large variety of solid tumors, including lung, colorectal, breast, squamous cell carcinomas of the head and neck, prostate and pancreatic carcinomas, glioblastomas, sarcomas, and hematological malignancies, but not on corresponding normal tissues (56, 57). A membrane Hsp70+ phenotype has been determined either directly on single cell suspensions of freshly isolated tumor biopsies by cell surface iodination/biotinylation (58, 59) and flow cytometry using cmHsp70.1 monoclonal antibody (60) or indirectly in the serum of patients using a novel lipHsp70 ELISA (61). In contrast to commercially available ELISA systems, the lipHsp70 ELISA specifically detects free and lipid-bound, exosomal Hsp70 which is actively released by viable tumor cells. Therefore, it is assumed that the quantification of exosomal Hsp70 in the serum serves as a measure for viable tumor mass in a patient and thus might provide a diagnostic/prognostic biomarker in the future (62). A membrane Hsp70+ tumor phenotype has been found to be associated with highly aggressive tumors, causing invasion and metastases and resistance to cell death (57, 63, 64). However, NK cells, but not T cells, were found to kill membrane Hsp70+ tumor cells after preactivation with naturally occurring Hsp70 or an Hsp70–peptide (TKD) derived thereof in combination with low dose IL-2 (TKD/IL-2) (65). Since the induction of the cytolytic activity of NK cells with Hsp70–peptide is dose dependent and saturable, it was assumed that the interaction of NK cells with the peptide might also be receptor mediated. By antibody and protein/peptide blocking assays, the C-type lectin receptor CD94 was identified as a potential receptor that mediates the interaction of NK cells with Hsp70–peptide. CD94 forms a heterodimer either with the coreceptor NKG2A or NKG2C and thus can act as an inhibitory or activation receptor complex (66–69). Following incubation of NK cells with Hsp70 protein or Hsp70–peptide + IL-2, the density of CD94 was found to be upregulated concomitant with an increased cytolytic and migratory activity against membrane Hsp70+ tumor cells (70). In addition, also other activatory NK cell receptors, such as NKG2D, and natural cytotoxicity receptors (NCRs), but not inhibitory killer-cell immunoglobulin-like receptors (KIRs), were found to be upregulated on NK cells upon stimulation with Hsp70–peptide + IL-2.
A summary of major activities of Hsp70 in inducing adaptive and innate antitumor immune responses is illustrated in Figure 1. On the one hand, Hsp70 either alone or in combination with immunogenic peptides is able to induce the maturation of dendritic cells (DCs), activate the cytolytic, proliferative, and migratory capacity of NK cells, stimulate the antigen-dependent T cell activation and IFN-γ secretion, induce the release of pro- and anti-inflammatory cytokines, on the other hand, membrane-bound Hsp70 acts as a tumor-specific antigen, which is recognized by preactivated NK cells. As a carrier for HSP-chaperoned tumor-specific antigens members of the HSP70 and HSP90 family have been found to support antigen uptake, processing, and presentation on MHC class I to CD8+ cytotoxic T lymphocytes and on MHC class II molecules to CD4+ helper T cells.
Figure 1. Major immune modulatory functions of heat shock protein 70 (Hsp70) either alone bound to exosomes or in combination with tumor-derived peptides. Abbreviations: IFN-γ, interferon gamma; IL, interleukin; Mφ, macrophages; MHC, major histocompatibility complex; NK cells, natural killer cell; NO, nitric oxide.
The mechanism how Hsp70 preactivated NK cells lyse membrane Hsp70+ tumor cells could be identified as granzyme B-mediated apoptosis. The cell death-inducing serine protease granzyme B has been found to directly interact with membrane Hsp70 on tumor cells, as determined by different methods including matrix-laser desorption ionization time to flight mass peptide finger printing (MALDI-TOF), Western blot, and flow cytometry (71). NK cells that have been prestimulated with Hsp70–peptide + IL-2 showed a significantly upregulated production of granzyme B, whereas the intracellular levels of perforin were found to be upregulated only moderately (16, 70). Since tumor cells that lack an Hsp70 membrane expression are not lysed by granzyme B, as demonstrated in isogenic tumor cell systems that differ in their membrane Hsp70 expression levels, it was concluded that Hsp70–peptide + IL-2 preactivated NK cells predominantly kill their target cells via granzyme B-mediated apoptosis (71).
Safety and tolerability of ex vivo TKD/IL-2 stimulated, autologous NK cells have been demonstrated in patients with metastasized colorectal and NSCLC in a Phase I clinical trial (72). Based on promising clinical results of this Phase I trial, a Phase II randomized clinical study was initiated in 2015 (73). The primary objective of this multicenter proof-of-concept trial is to examine whether an adjuvant treatment of NSCLC patients after platinum-based radiochemotherapy (RCT) with Hsp70–peptide TKD + IL-2-activated, autologous NK cells is clinically effective. Only membrane Hsp70+ tumor patients will be recruited into the trial since membrane Hsp70 was identified as the tumor-specific target for Hsp70–peptide + IL-2 preactivated NK cells. The primary endpoint of this study is the progression-free survival that will be compared between patients who received RCT or RCT+ an NK cell-based immunotherapy. As secondary endpoints overall survival, toxicity, quality-of-life, and biological responses will be determined in both study groups.
Heat shock proteins, especially the major stress-inducible Hsp70, can provide cytokine function, which initiate both, innate and adaptive immunity (74–77). In parallel, these HSPs can act as classical chaperones that facilitate uptake, processing, and presentation of tumor antigens into APCs. Moreover, exogenously delivered, purified Hsp70 was shown to sensitize cancer cells to lymphocyte-mediated cytotoxicity due to triggering the translocation of its intracellular analog to the tumor cell surface and due to an increased release of Hsp70 into the extracellular milieu (18). For these reasons, the aforementioned immunomodulatory activities of Hsp70 have been widely exploited for therapeutic approaches in recent years either as single treatment or in combination with other treatment modalities to generate an effective antitumor immunity. The intra-tumoral injection of Hsp70 protein or an upregulation of Hsp70 within the tumor by an hsp70.1 gene transfer was shown to have a significant therapeutic potential in preclinical studies (18, 78–81). Thus, prolonged intra-tumoral delivery of exogenous Hsp70 in a rat glioblastoma model caused a significant inhibition of tumor progression, which as accompanied by an increased cytotoxic activity of NK cells and CD8+ T lymphocytes (82). A comparable therapeutic efficacy was previously reported by Rafiee et al. (79) who showed a complete tumor eradication following transfection of the hsp70.1 gene sequence into mouse tumor cells. The systemic antitumor immune response was found to be mediated by CD4+ and CD8+ T cells (79). Presumably, a combination of Hsp70-based therapies with other immunological approaches, such as immune- and T cell check-point inhibitors, might further increase the therapeutic efficacy. In another approach, the intra-tumoral injection of Hsp70 was combined with mild local hyperthermia and magnetite cationic liposomes (MCLs). This strategy demonstrated great potential in the treatment of mouse melanoma (81). With regard to these results, our group coupled Hsp70 to nanocarriers such as superparamagnetic iron oxide nanoparticles (SPIONs) (83). Hsp70-SPIONs were shown to effectively deliver immunogenic peptides from tumor lysates to DCs and thus stimulated a tumor-specific, CD8+ cytotoxic T cell response in experimental glioma models (83). Up-to-date several clinical trials clearly demonstrate that the application of Hsp70 either as a single treatment regimen or in combination with other therapies is feasible and can stimulate antitumor immunity in patients (18, 84, 85). Clinical efficacy could be demonstrated in patients with brain tumors who received surgery and intra-tumoral delivery of recombinant Hsp70. Out of 12 patients with late stage brain tumors, one patient showed a complete CR and another patient showed a partial response (PR) (18). The CRs were accompanied by an enhanced Th1-cell-mediated immune response and a reduction of immunosuppressive Treg cells. In the Phase I clinical trial reported by Maeda et al., DCs transfected with Hsp70 mRNA (HSP70-DCs) were applied in 12 patients with non-resectable or recurrent HCV-related hepatocellular carcinoma (HCC) (85). The authors demonstrated that 7 out of 12 patients had either a CR or stable disease (SD), suggesting the efficacy of the proposed therapy. In another study, an intra-tumoral vaccination with recombinant oncolytic type-2 adenovirus that overexpresses Hsp70 was found to inhibit primary and metastatic tumors via an enhanced oncolytic activity and Hsp70-mediated immune responses (84). Presumably, multimodality tumor-directed therapy based on HSPs in combination with radio, chemo, and/or hyperthermia (86) therapy can be a treatment option for further clinical trials.
Heat shock proteins and especially members of the HSP70 and HSP90 families have been found to elicit protective antitumor immunity in preclinical models and in tumor patients either alone or in complex with tumor-derived peptides. HSPs and HSP–peptide complexes can act as typical tumor-specific foreign antigens, chaperokines, and adjuvants that facilitate uptake, processing, and presentation for tumor-specific antigens which are cross-presented by APCs to CD8+ cytotoxic T lymphocytes. Uptake of HSPs and HSP–peptide complexes is mediated by a large variety of different receptors. Depending on the dose of the HSP-based vaccine either immunosuppressive or immunostimulatory activities can be elicited.
MS wrote the paragraph about HSPs as an adjuvant and produced the figure; GM wrote the MS.
The authors report no conflicts of interest. The authors alone are responsible for the content and preparation of this paper.
GM’s laboratory is supported, in part, by grants from the Deutsche Forschungsgemeinschaft (DFG SFB824/2, INST 411/37-1 FUGG, INST 95/980-1 FUGG), Munich Advanced Photonics (MAP), the Bundesministerium für Forschung und Technologie (BMBF 02NUK038A, 01GU0823, DKTK-ROG), EU CELLEUROPE (EU 315963), Helmholtz Zentrum München, German Research Center for Environmental Health (G-501390-001), and by multimmune GmbH, Munich.
1. Srivastava PK, DeLeo AB, Old LJ. Tumor rejection antigens of chemically induced sarcomas of inbred mice. Proc Natl Acad Sci U S A (1986) 83:3407–11. doi: 10.1073/pnas.83.10.3407
2. Ullrich SJ, Robinson EA, Law LW, Willingham M, Appella E. A mouse tumor-specific transplantation antigen is a heat shock-related protein. Proc Natl Acad Sci U S A (1986) 83:3121–5. doi:10.1073/pnas.83.10.3121
3. Srivastava P. Interaction of heat shock proteins with peptides and antigen presenting cells: chaperoning of the innate and adaptive immune responses. Annu Rev Immunol (2002) 20:395–425. doi:10.1146/annurev.immunol.20.100301.064801
4. Binder RJ, Han DK, Srivastava PK. CD91: a receptor for heat shock protein gp96. Nat Immunol (2000) 1:151–5. doi:10.1038/77835
5. Gong J, Zhu B, Murshid A, Adachi H, Song B, Lee A, et al. T cell activation by heat shock protein 70 vaccine requires TLR signaling and scavenger receptor expressed by endothelial cells-1. J Immunol (2009) 183:3092–8. doi:10.4049/jimmunol.0901235
6. Tosti G, Cocorocchio E, Pennacchioli E, Ferrucci PF, Testori A, Martinoli C. Heat-shock proteins-based immunotherapy for advanced melanoma in the era of target therapies and immunomodulating agents. Expert Opin Biol Ther (2014) 14:955–67. doi:10.1517/14712598.2014.902928
7. Berwin B, Hart JP, Rice S, Gass C, Pizzo SV, Post SR, et al. Scavenger receptor-A mediates gp96/GRP94 and calreticulin internalization by antigen-presenting cells. EMBO J (2003) 22:6127–36. doi:10.1093/emboj/cdg572
8. Yang Y, Liu B, Dai J, Srivastava PK, Zammit DJ, Lefrancois L, et al. Heat shock protein gp96 is a master chaperone for toll-like receptors and is important in the innate function of macrophages. Immunity (2007) 26:215–26. doi:10.1016/j.immuni.2006.12.005
9. Chalmin F, Ladoire S, Mignot G, Vincent J, Bruchard M, Remy-Martin JP, et al. Membrane-associated Hsp72 from tumor-derived exosomes mediates STAT3-dependent immunosuppressive function of mouse and human myeloid-derived suppressor cells. J Clin Invest (2010) 120:457–71. doi:10.1172/JCI40483
10. Vicencio JM, Yellon DM, Sivaraman V, Das D, Boi-Doku C, Arjun S, et al. Plasma exosomes protect the myocardium from ischemia-reperfusion injury. J Am Coll Cardiol (2015) 65:1525–36. doi:10.1016/j.jacc.2015.02.026
11. Asea A, Kraeft SK, Kurt-Jones EA, Stevenson MA, Chen LB, Finberg RW, et al. HSP70 stimulates cytokine production through a CD14-dependant pathway, demonstrating its dual role as a chaperone and cytokine. Nat Med (2000) 6:435–42. doi:10.1038/74697
12. Vabulas RM, Ahmad-Nejad P, Ghose S, Kirschning CJ, Issels RD, Wagner H. HSP70 as endogenous stimulus of the toll/interleukin-1 receptor signal pathway. J Biol Chem (2002) 277:15107–12. doi:10.1074/jbc.M111204200
13. Becker T, Hartl FU, Wieland F. CD40, an extracellular receptor for binding and uptake of Hsp70-peptide complexes. J Cell Biol (2002) 158:1277–85. doi:10.1083/jcb.200208083
14. Botzler C, Li G, Issels RD, Multhoff G. Definition of extracellular localized epitopes of Hsp70 involved in an NK immune response. Cell Stress Chaperones (1998) 3:6–11. doi:10.1379/1466-1268(1998)003<0006:DOELEO>2.3.CO;2
15. Multhoff G, Mizzen L, Winchester CC, Milner CM, Wenk S, Eissner G, et al. Heat shock protein 70 (Hsp70) stimulates proliferation and cytolytic activity of natural killer cells. Exp Hematol (1999) 27:1627–36. doi:10.1016/S0301-472X(99)00104-6
16. Gross C, Hansch D, Gastpar R, Multhoff G. Interaction of heat shock protein 70 peptide with NK cells involves the NK receptor CD94. Biol Chem (2003) 384:267–79. doi:10.1515/BC.2003.030
17. Shevtsov MA, Pozdnyakov AV, Mikhrina AL, Yakovleva LY, Nikolaev BP, Dobrodumov AV, et al. Effective immunotherapy of rat glioblastoma with prolonged intra-tumoral delivery of exogenous heat shock protein Hsp70. Int J Cancer (2014) 135:2118–28. doi:10.1002/ijc.28858
18. Shevtsov MA, Kim AV, Samochernych KA, Romanova IV, Margulis BA, Guzhova IV, et al. Pilot study of intra-tumoral injection of recombinant heat shock protein 70 in the treatment of malignant brain tumors in children. Onco Targets Ther (2014) 7:1071–81. doi:10.2147/OTT.S62764
19. Li Z, Srivastava PK. Tumor rejection antigen gp96/grp94 is an ATPase: implications for protein folding and antigen presentation. EMBO J (1993) 12:3143–51.
20. Udono H, Srivastava PK. Heat shock protein 70-associated peptides elicit specific cancer immunity. J Exp Med (1993) 178:1391–6. doi:10.1084/jem.178.4.1391
21. Udono H, Levey DL, Srivastava PK. Cellular requirements for tumor-specific immunity elicited by heat shock proteins: tumor rejection antigen gp96 primes CD8+ T cells in vivo. Proc Natl Acad Sci U S A (1994) 91:3077–81. doi:10.1073/pnas.91.8.3077
22. Srivastava PK. Purification of heat shock protein-peptide complexes for use in vaccination against cancers and intracellular pathogens. Methods (1997) 12:165–71. doi:10.1006/meth.1997.0464
23. Peng P, Menoret A, Srivastava PK. Purification of immunogenic heat shock protein 70-peptide complexes by ADP-affinity chromatography. J Immunol Methods (1997) 204:13–21. doi:10.1016/S0022-1759(97)00017-3
24. Srivastava PK. Heat shock proteins in immune response to cancer: the fourth paradigm. Experientia (1994) 50:1054–60. doi:10.1007/BF01923461
25. Srivastava IK, Liu MA. Gene vaccines. Ann Intern Med (2003) 138:550–9. doi:10.7326/0003-4819-138-7-200304010-00011
26. SenGupta D, Norris PJ, Suscovich TJ, Hassan-Zahraee M, Moffett HF, Trocha A, et al. Heat shock protein-mediated cross-presentation of exogenous HIV antigen on HLA class I and class II. J Immunol (2004) 173:1987–93. doi:10.4049/jimmunol.173.3.1987
27. Blachere NE, Li Z, Chandawarkar RY, Suto R, Jaikaria NS, Basu S, et al. Heat shock protein-peptide complexes, reconstituted in vitro, elicit peptide-specific cytotoxic T lymphocyte response and tumor immunity. J Exp Med (1997) 186:1315–22. doi:10.1084/jem.186.8.1315
28. Belli F, Testori A, Rivoltini L, Maio M, Andreola G, Sertoli MR, et al. Vaccination of metastatic melanoma patients with autologous tumor-derived heat shock protein gp96-peptide complexes: clinical and immunologic findings. J Clin Oncol (2002) 20:4169–80. doi:10.1200/JCO.2002.09.134
29. Suto R, Srivastava PK. A mechanism for the specific immunogenicity of heat shock protein-chaperoned peptides. Science (1995) 269:1585–8. doi:10.1126/science.7545313
30. Noessner E, Gastpar R, Milani V, Brandl A, Hutzler PJ, Kuppner MC, et al. Tumor-derived heat shock protein 70 peptide complexes are cross-presented by human dendritic cells. J Immunol (2002) 169:5424–32. doi:10.4049/jimmunol.169.10.5424
31. Murshid A, Gong J, Calderwood SK. The role of heat shock proteins in antigen cross presentation. Front Immunol (2012) 3:63. doi:10.3389/fimmu.2012.00063
32. Binder RJ, Harris ML, Menoret A, Srivastava PK. Saturation, competition, and specificity in interaction of heat shock proteins (hsp) gp96, hsp90, and hsp70 with CD11b+ cells. J Immunol (2000) 165:2582–7. doi:10.4049/jimmunol.165.5.2582
33. Basu S, Binder RJ, Ramalingam T, Srivastava PK. CD91 is a common receptor for heat shock proteins gp96, hsp90, hsp70, and calreticulin. Immunity (2001) 14:303–13. doi:10.1016/S1074-7613(01)00111-X
34. Murshid A, Theriault J, Gong J, Calderwood SK. Investigating receptors for extracellular heat shock proteins. Methods Mol Biol (2011) 787:289–302. doi:10.1007/978-1-61779-295-3_22
35. Murshid A, Borges TJ, Calderwood SK. Emerging roles for scavenger receptor SREC-I in immunity. Cytokine (2015) 75:256–60. doi:10.1016/j.cyto.2015.02.009
36. Asea A, Rehli M, Kabingu E, Boch JA, Bare O, Auron PE, et al. Novel signal transduction pathway utilized by extracellular HSP70: role of TLR2 and TLR4. J Biol Chem (2002) 277:15028–34. doi:10.1074/jbc.M200497200
37. Arnold-Schild D, Hanau D, Spehner D, Schmid C, Rammensee HG, de la SH, et al. Cutting edge: receptor-mediated endocytosis of heat shock proteins by professional antigen-presenting cells. J Immunol (1999) 162:3757–60.
38. Chandawarkar RY, Wagh MS, Srivastava PK. The dual nature of specific immunological activity of tumor-derived gp96 preparations. J Exp Med (1999) 189:1437–42. doi:10.1084/jem.189.9.1437
39. Chandawarkar RY, Wagh MS, Kovalchin JT, Srivastava P. Immune modulation with high-dose heat-shock protein gp96: therapy of murine autoimmune diabetes and encephalomyelitis. Int Immunol (2004) 16:615–24. doi:10.1093/intimm/dxh063
40. Binder RJ, Zhou YJ, Messmer MN, Pawaria S. CD91-dependent modulation of immune responses by heat shock proteins: a role in autoimmunity. Autoimmune Dis (2012) 2012:863041. doi:10.1155/2012/863041
41. Oki Y, Younes A. Heat shock protein-based cancer vaccines. Expert Rev Vaccines (2004) 3:403–11. doi:10.1586/14760584.3.4.403
42. Janetzki S, Palla D, Rosenhauer V, Lochs H, Lewis JJ, Srivastava PK. Immunization of cancer patients with autologous cancer-derived heat shock protein gp96 preparations: a pilot study. Int J Cancer (2000) 88:232–8. doi:10.1002/1097-0215(20001015)88:2<232::AID-IJC14>3.0.CO;2-8
43. Srivastava PK. Therapeutic cancer vaccines. Curr Opin Immunol (2006) 18:201–5. doi:10.1016/j.coi.2006.01.009
44. Castelli C, Rivoltini L, Rini F, Belli F, Testori A, Maio M, et al. Heat shock proteins: biological functions and clinical application as personalized vaccines for human cancer. Cancer Immunol Immunother (2004) 53:227–33. doi:10.1007/s00262-003-0481-9
45. Pilla L, Patuzzo R, Rivoltini L, Maio M, Pennacchioli E, Lamaj E, et al. A Phase II trial of vaccination with autologous, tumor-derived heat-shock protein peptide complexes Gp96, in combination with GM-CSF and interferon-alpha in metastatic melanoma patients. Cancer Immunol Immunother (2006) 55:958–68. doi:10.1007/s00262-005-0084-8
46. Testori A, Richards J, Whitman E, Mann GB, Lutzky J, Camacho L, et al. Phase III comparison of vitespen, an autologous tumor-derived heat shock protein gp96 peptide complex vaccine, with physician’s choice of treatment for stage IV melanoma: the C-100-21 Study Group. J Clin Oncol (2008) 26:955–62. doi:10.1200/JCO.2007.11.9941
47. Schadendorf D, Ugurel S, Schuler-Thurner B, Nestle FO, Enk A, Brocker EB, et al. Dacarbazine (DTIC) versus vaccination with autologous peptide-pulsed dendritic cells (DC) in first-line treatment of patients with metastatic melanoma: a randomized Phase III trial of the DC study group of the DeCOG. Ann Oncol (2006) 17:563–70. doi:10.1093/annonc/mdj138
48. Nestle FO, Alijagic S, Gilliet M, Sun Y, Grabbe S, Dummer R, et al. Vaccination of melanoma patients with peptide- or tumor lysate-pulsed dendritic cells. Nat Med (1998) 4:328–32. doi:10.1038/nm0398-328
49. Mazzaferro V, Coppa J, Carrabba MG, Rivoltini L, Schiavo M, Regalia E, et al. Vaccination with autologous tumor-derived heat-shock protein gp96 after liver resection for metastatic colorectal cancer. Clin Cancer Res (2003) 9:3235–45.
51. Hertkorn C, Lehr A, Wölfel T, Junginger T, Dippold WG, Galle PR, et al. Phase I trial of vaccination with autologous tumor-derived gp96 in patients after surgery for gastric cancer. Proc Am Soc Clin Oncol (2002) 21:30a.
52. Heike M, Hertkorn C, Reitsma DJ, Kommoss F, Simiantonaki N, Woelfel T, et al. Pilot trial of vaccination with autologous tumor-derived GP96 heat shock protein-peptide complex (HSPPC-96) in patients after surgery for gastric carcinoma. Proc Am Soc Clin Oncol (2000) 19:1825.
53. Maki RG, Livingston PO, Lewis JJ, Janetzki S, Klimstra D, Desantis D, et al. A Phase I pilot study of autologous heat shock protein vaccine HSPPC-96 in patients with resected pancreatic adenocarcinoma. Dig Dis Sci (2007) 52:1964–72. doi:10.1007/s10620-006-9205-2
54. Hammond DB. What ASCO does for its members. J Clin Oncol (2004) 22:2251–2. doi:10.1200/JCO.2004.99.075
55. Bloch O, Crane CA, Fuks Y, Kaur R, Aghi MK, Berger MS, et al. Heat-shock protein peptide complex-96 vaccination for recurrent glioblastoma: a Phase II, single-arm trial. Neuro Oncol (2014) 16:274–9. doi:10.1093/neuonc/not203
56. Gehrmann M, Schmetzer H, Eissner G, Haferlach T, Hiddemann W, Multhoff G. Membrane-bound heat shock protein 70 (Hsp70) in acute myeloid leukemia: a tumor specific recognition structure for the cytolytic activity of autologous NK cells. Haematologica (2003) 88:474–6.
57. Multhoff G, Botzler C, Jennen L, Schmidt J, Ellwart J, Issels R. Heat shock protein 72 on tumor cells: a recognition structure for natural killer cells. J Immunol (1997) 158:4341–50.
58. Multhoff G, Botzler C, Wiesnet M, Muller E, Meier T, Wilmanns W, et al. A stress-inducible 72-kDa heat-shock protein (HSP72) is expressed on the surface of human tumor cells, but not on normal cells. Int J Cancer (1995) 61:272–9. doi:10.1002/ijc.2910610222
59. Shin BK, Wang H, Yim AM, Le Naour F, Brichory F, Jang JH, et al. Global profiling of the cell surface proteome of cancer cells uncovers an abundance of proteins with chaperone function. J Biol Chem (2003) 278:7607–16. doi:10.1074/jbc.M210455200
60. Stangl S, Gehrmann M, Riegger J, Kuhs K, Riederer I, Sievert W, et al. Targeting membrane heat-shock protein 70 (Hsp70) on tumors by cmHsp70.1 antibody. Proc Natl Acad Sci U S A (2011) 108:733–8. doi:10.1073/pnas.1016065108
61. Breuninger S, Erl J, Knape C, Gunther S, Regel I, Rodel F, et al. Quantitative analysis of liposomal heat shock protein 70 (Hsp70) in the blood of tumor patients using an novel lipHsp70 ELISA. J Clin Cell Immunol (2014) 5:2–10. doi:10.4172/2155-9899.1000264
62. Ciocca DR, Calderwood SK. Heat shock proteins in cancer: diagnostic, prognostic, predictive, and treatment implications. Cell Stress Chaperones (2005) 10:86–103. doi:10.1379/CSC-99r.1
63. Gastpar R, Gehrmann M, Bausero MA, Asea A, Gross C, Schroeder JA, et al. Heat shock protein 70 surface-positive tumor exosomes stimulate migratory and cytolytic activity of natural killer cells. Cancer Res (2005) 65:5238–47. doi:10.1158/0008-5472.CAN-04-3804
64. Murakami N, Kuhnel A, Schmid TE, Ilicic K, Stangl S, Braun IS, et al. Role of membrane Hsp70 in radiation sensitivity of tumor cells. Radiat Oncol (2015) 10:149. doi:10.1186/s13014-015-0461-1
65. Multhoff G, Pfister K, Gehrmann M, Hantschel M, Gross C, Hafner M, et al. A 14-mer Hsp70 peptide stimulates natural killer (NK) cell activity. Cell Stress Chaperones (2001) 6:337–44. doi:10.1379/1466-1268(2001)006<0337:AMHPSN>2.0.CO;2
66. Biassoni R. Human natural killer receptors, co-receptors, and their ligands. Curr Protoc Immunol (2009) Chapter 14:Unit14.10. doi:10.1002/0471142735.im1410s84
67. Pegram HJ, Andrews DM, Smyth MJ, Darcy PK, Kershaw MH. Activating and inhibitory receptors of natural killer cells. Immunol Cell Biol (2011) 89:216–24. doi:10.1038/icb.2010.78
68. Sullivan LC, Clements CS, Beddoe T, Johnson D, Hoare HL, Lin J, et al. The heterodimeric assembly of the CD94-NKG2 receptor family and implications for human leukocyte antigen-E recognition. Immunity (2007) 27:900–11. doi:10.1016/j.immuni.2007.10.013
69. Borrego F, Masilamani M, Marusina AI, Tang X, Coligan JE. The CD94/NKG2 family of receptors: from molecules and cells to clinical relevance. Immunol Res (2006) 35:263–78. doi:10.1385/IR:35:3:263
70. Gross C, Schmidt-Wolf IG, Nagaraj S, Gastpar R, Ellwart J, Kunz-Schughart LA, et al. Heat shock protein 70-reactivity is associated with increased cell surface density of CD94/CD56 on primary natural killer cells. Cell Stress Chaperones (2003) 8:348–60. doi:10.1379/1466-1268(2003)008<0348:HSPRIA>2.0.CO;2
71. Gross C, Koelch W, DeMaio A, Arispe N, Multhoff G. Cell surface-bound heat shock protein 70 (Hsp70) mediates perforin-independent apoptosis by specific binding and uptake of granzyme B. J Biol Chem (2003) 278:41173–81. doi:10.1074/jbc.M302644200
72. Krause SW, Gastpar R, Andreesen R, Gross C, Ullrich H, Thonigs G, et al. Treatment of colon and lung cancer patients with ex vivo heat shock protein 70-peptide-activated, autologous natural killer cells: a clinical Phase I trial. Clin Cancer Res (2004) 10:3699–707. doi:10.1158/1078-0432.CCR-03-0683
73. Specht HM, Ahrens N, Blankenstein C, Duell T, Fietkau R, Gaipl US, et al. Heat shock protein 70 (Hsp70) peptide activated natural killer (NK) cells for the treatment of patients with non-small cell lung cancer (NSCLC) after radiochemotherapy (RCTx) – from preclinical studies to a clinical Phase II trial. Front Immunol (2015) 6:162. doi:10.3389/fimmu.2015.00162
74. Elsner L, Muppala V, Gehrmann M, Lozano J, Malzahn D, Bickeboller H, et al. The heat shock protein Hsp70 promotes mouse NK cell activity against tumors that express inducible NKG2D ligands. J Immunol (2007) 179:5523–33. doi:10.4049/jimmunol.179.8.5523
75. Takemoto S, Nishikawa M, Guan X, Ohno Y, Yata T, Takakura Y. Enhanced generation of cytotoxic T lymphocytes by heat shock protein 70 fusion proteins harboring both CD8(+) T cell and CD4(+) T cell epitopes. Mol Pharm (2010) 7:1715–23. doi:10.1021/mp1001069
76. Guzhova IV, Shevtsov MA, Abkin SV, Pankratova KM, Margulis BA. Intracellular and extracellular Hsp70 chaperone as a target for cancer therapy. Int J Hyperthermia (2013) 29:399–408. doi:10.3109/02656736.2013.807439
77. Asea A. Initiation of the immune response by extracellular Hsp72: chaperokine activity of Hsp72. Curr Immunol Rev (2006) 2:209–15. doi:10.2174/157339506778018514
78. Geng H, Zhang GM, Xiao H, Yuan Y, Li D, Zhang H, et al. HSP70 vaccine in combination with gene therapy with plasmid DNA encoding sPD-1 overcomes immune resistance and suppresses the progression of pulmonary metastatic melanoma. Int J Cancer (2006) 118:2657–64. doi:10.1002/ijc.21795
79. Rafiee M, Kanwar JR, Berg RW, Lehnert K, Lisowska K, Krissansen GW. Induction of systemic anti-tumor immunity by gene transfer of mammalian heat shock protein 70.1 into tumors in situ. Cancer Gene Ther (2001) 8:974–81. doi:10.1038/sj.cgt.7700395
80. Nishikawa M, Otsuki T, Ota A, Guan X, Takemoto S, Takahashi Y, et al. Induction of tumor-specific immune response by gene transfer of Hsp70-cell-penetrating peptide fusion protein to tumors in mice. Mol Ther (2010) 18:421–8. doi:10.1038/mt.2009.203
81. Ito A, Matsuoka F, Honda H, Kobayashi T. Anti-tumor effects of combined therapy of recombinant heat shock protein 70 and hyperthermia using magnetic nanoparticles in an experimental subcutaneous murine melanoma. Cancer Immunol Immunother (2004) 53:26–32. doi:10.1007/s00262-003-0416-5
82. Shevtsov MA, Komarova EY, Meshalkina DA, Bychkova NV, Aksenov ND, Abkin SV, et al. Exogenously delivered heat shock protein 70 displaces its endogenous analogue and sensitizes cancer cells to lymphocytes-mediated cytotoxicity. Oncotarget (2014) 5:3101–14. doi:10.18632/oncotarget.1820
83. Shevtsov MA, Nikolaev BP, Yakovleva LY, Parr MA, Marchenko YY, Eliseev I, et al. 70-kDa heat shock protein coated magnetic nanocarriers as a nanovaccine for induction of anti-tumor immune response in experimental glioma. J Control Release (2015) 220:329–40. doi:10.1016/j.jconrel.2015.10.051
84. Li JL, Liu HL, Zhang XR, Xu JP, Hu WK, Liang M, et al. A Phase I trial of intra-tumoral administration of recombinant oncolytic adenovirus overexpressing HSP70 in advanced solid tumor patients. Gene Ther (2009) 16:376–82. doi:10.1038/gt.2008.179
85. Maeda Y, Yoshimura K, Matsui H, Shindo Y, Tamesa T, Tokumitsu Y, et al. Dendritic cells transfected with heat-shock protein 70 messenger RNA for patients with hepatitis C virus-related hepatocellular carcinoma: a Phase I dose escalation clinical trial. Cancer Immunol Immunother (2015) 64:1047–56. doi:10.1007/s00262-015-1709-1
86. Schildkopf P, Frey B, Ott OJ, Rubner Y, Multhoff G, Sauer R, et al. Radiation combined with hyperthermia induces Hsp70-dependent maturation of dendritic cells and release of pro-inflammatory cytokines by dendritic cells and macrophages. Radiother Oncol (2011) 101:109–15. doi:10.1016/j.radonc.2011.05.056
Keywords: HSP70 heat shock proteins, HSP90 heat shock proteins, cancer vaccine, innate immunity, adaptive immunity
Citation: Shevtsov M and Multhoff G (2016) Heat Shock Protein–Peptide and HSP-Based Immunotherapies for the Treatment of Cancer. Front. Immunol. 7:171. doi: 10.3389/fimmu.2016.00171
Received: 30 November 2015; Accepted: 18 April 2016;
Published: 29 April 2016
Edited by:
Ayesha Murshid, Harvard Medical School, USAReviewed by:
Daniel Olive, INSERM UMR 891 Institut Paoli Calmettes, FranceCopyright: © 2016 Shevtsov and Multhoff. This is an open-access article distributed under the terms of the Creative Commons Attribution License (CC BY). The use, distribution or reproduction in other forums is permitted, provided the original author(s) or licensor are credited and that the original publication in this journal is cited, in accordance with accepted academic practice. No use, distribution or reproduction is permitted which does not comply with these terms.
*Correspondence: Gabriele Multhoff, Z2FicmllbGUubXVsdGhvZmZAdHVtLmRl
Disclaimer: All claims expressed in this article are solely those of the authors and do not necessarily represent those of their affiliated organizations, or those of the publisher, the editors and the reviewers. Any product that may be evaluated in this article or claim that may be made by its manufacturer is not guaranteed or endorsed by the publisher.
Research integrity at Frontiers
Learn more about the work of our research integrity team to safeguard the quality of each article we publish.