- 1Division of Urology, Department of Surgery, The University of Arizona, Tucson, AZ, USA
- 2Department of Urology, Feinberg School of Medicine, Northwestern University, Chicago, IL, USA
- 3Jesse Brown Veterans Affairs Medical Center, Chicago, IL, USA
- 4Department of Pathology, University of Illinois at Chicago, Chicago, IL, USA
Prostate cancer (PCa) is the most common cancer among men in the U.S. African American (AA) men have a higher incidence and mortality rate compared to European American (EA) men, but the cause of PCa disparities is still unclear. Epidemiologic studies have shown that vitamin D deficiency is associated with advanced stage and higher tumor grade and mortality, while its association with overall PCa risk is inconsistent. Vitamin D deficiency is also more common in AAs than EAs, and the difference in serum vitamin D levels may help explain the PCa disparities. However, the role of vitamin D in aggressive PCa in AAs is not well explored. Studies demonstrated that the active form of vitamin D, 1,25-dihydroxyvitamin D, has anti-inflammatory effects by mediating immune-related gene expression in prostate tissue. Inflammation also plays an important role in PCa pathogenesis and progression, and expression of immune-related genes in PCa tissues differs significantly between AAs and EAs. Unfortunately, the evidence linking vitamin D and immune response in relation to PCa is still scarce. This relationship should be further explored at a genomic level in AA populations that are at high risk for vitamin D deficiency and fatal PCa.
Introduction
Prostate cancer (PCa) is the most common cancer among men in the U.S., and African American (AA) men have higher PCa incidence and mortality than other racial/ethnic groups. Social and behavioral factors affect stage, grade, treatment choice, and mortality (1, 2), but the etiologies for the PCa disparities are still being elucidated. Epidemiologic studies have showed that vitamin D deficiency is associated with advanced stage, higher tumor grade, and mortality (3–5), while its association with overall PCa incidence is inconsistent (6–9). Vitamin D deficiency is also common in AAs, even AAs living in southern U.S. (10–12), and differences in serum vitamin D levels may help explain PCa disparities (13, 14). However, epidemiologic studies have mainly been conducted in men of European descent, and the role of vitamin D in AAs, who are more likely to have aggressive PCa, has not been well explored.
Several pathways for how vitamin D affects PCa pathogenesis and progression have been explored. The active form of vitamin D, 1,25-dihydroxyvitamin D [1,25(OH)2D], inhibits tumor cell proliferation and induces apoptosis (15, 16) 1,25(OH)2D also modulates expression of immune-related genes in prostate tissue (17). 1,25(OH)2D binds to vitamin D receptor (VDR) and regulates expression of hundreds of genes that have vitamin D response elements (VDREs), a segment of DNA found in the promoter region of vitamin D target genes. This transcriptional regulation by 1,25(OH)2D and VDR can affect the production of immune-related biomarkers (18, 19). Inflammation also plays an important role in PCa pathogenesis (20), and expression of immune-related genes in PCa tissues differs significantly between AAs and European Americans (EAs) (21–23).
In this review, we discuss the relationships and interactions between vitamin D and immune response. Many recent studies have shown the role of vitamin D on immune response, but the evidence linking vitamin D and immune response in the context of PCa is still scarce. We argue that this relationship should be investigated at genomic level, especially in AA populations that are at high risk for both PCa and vitamin D deficiency.
Prostate Cancer Disparities and African Americans
African American men have a 59% higher incidence and more than a twofold higher mortality rate compared to EA men (24). AAs are often diagnosed with PCa at younger ages and have PCa with a higher Gleason score, clinical stage, and prostate-specific antigen (PSA) level (25–28). AA patients with low risk PCa tend to have worse clinical features after undergoing prostatectomy (29, 30). Molecular differences in tumors from AAs and EAs exist and may result in a faster growth or earlier transformation to aggressive PCa in AAs compared to EAs (31–33). The cause of tumor biological differences between AAs and EAs is still unknown, but ancestry-related factors, such as genetic variation, vitamin D deficiency, and immune functions, may contribute.
Vitamin D and Prostate Cancer
Vitamin D is believed to have protective effects on PCa, especially for aggressive PCa (34, 35). Epidemiologic studies have shown that PCa patients with low serum 25-hydroxyvitamin D [25(OH)D] levels are likely to have higher PCa stage, grade, and mortality (3, 4, 36–38). On the other hand, association with overall PCa risk is inconsistent, and many studies have shown no association (4, 6, 7, 9, 39–42). Interestingly, the Selenium and Vitamin E Cancer Prevention Trial revealed that both low and high vitamin D levels increased PCa risk (43). Because vitamin D deficiency is very common among AAs (44–49), the higher prevalence of vitamin D deficiency may account for a proportion of PCa disparities (13, 14, 34). However, only a few studies demonstrated the association of vitamin D and PCa in AAs (5, 50).
25(OH)D is the main circulating form of vitamin D, and it is metabolized to the biologically more active, but less abundant, 1,25(OH)2D in the kidney by 1α-hydroxylase (Figure 1) (51). Both forms of vitamin D are transported to the prostate and other organs, and 1α-hydroxylase present in the prostate converts 25(OH)D to 1,25(OH)2D (52). Because 25(OH)D is more abundant than 1,25(OH)2D, both metabolites may have important roles in the prostate. Results of various experiments suggest that 1,25(OH)2D inhibits growth of prostate epithelial cells and PCa cells by inducing cell cycle arrest and apoptosis (15, 53–55). Vitamin D inhibits the tumor cell proliferation and induces apoptosis through activities of the VDR (56). 1,25(OH)2D attaches to the VDR, a transcription factor that binds to VDREs usually in the promoter region of vitamin D-responsive genes. Subsequently, activated VDR interacts with coactivators or corepressors to activate or repress these vitamin D-responsive genes. The VDR is expressed in prostate epithelium (57). Expression of VDR decreases after age 60 (58), and PCa patients with low VDR expression are more likely to have advanced and lethal PCa (59).
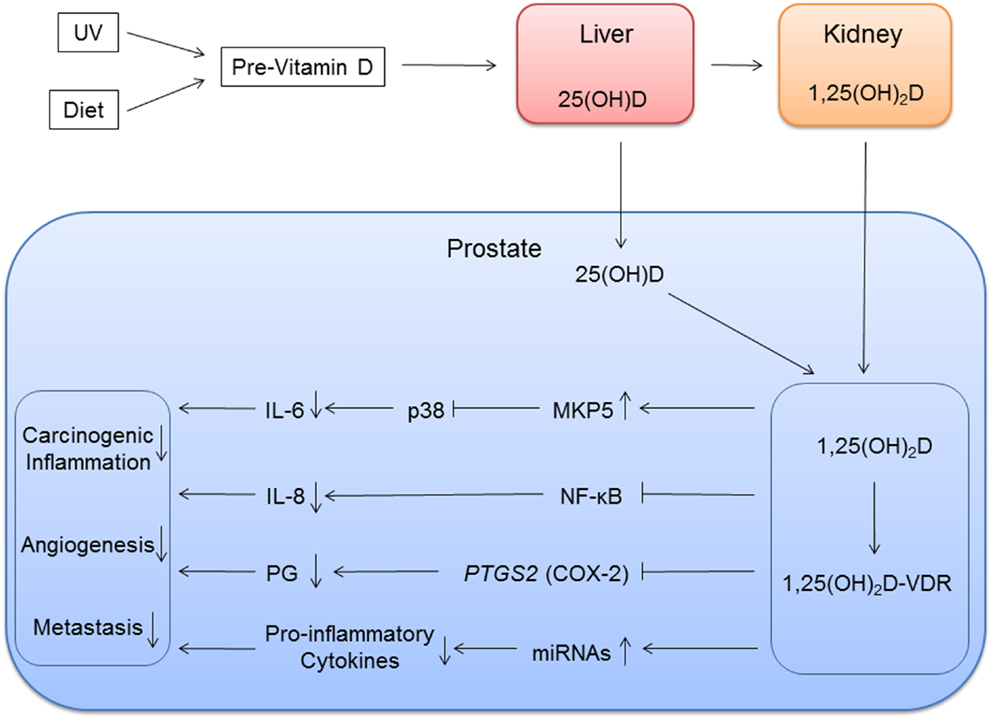
Figure 1. Vitamin D inflammation pathways of prostate cancer. Vitamin D metabolites are transported to the prostate. 25(OH)D is locally metabolized to 1,25(OH)2D, and 1,25(OH)2D binds to vitamin D receptor (VDR) with coactivators. This vitamin D and VDR complex modulates immune response by regulating expression of vitamin D target genes. The genes involved in immune response can be direct targets with vitamin D response element or in downstream in the pathways.
Inflammation and Prostate Cancer
Inflammation may play a role in PCa pathogenesis and progression (20, 60–62), but epidemiologic studies have shown conflicting evidence. In the placebo arm of the Prostate Cancer Prevention Trial, men who had at least one biopsy core with inflammation in benign prostate tissue had increased odds of overall PCa and high grade PCa (63). In the REduction by DUtasteride of Prostate Cancer Events (REDUCE), use of aspirin and/or non-steroid anti-inflammatory drugs (NSAIDs) reduced odds of overall PCa and high-grade PCa (64), but baseline acute and chronic inflammation was associated with reduced PCa risk at follow-up (65).
Although evidence linking inflammation and PCa is limited in EA populations, and prostate biopsy specimens from AA patients revealed infiltration of immune cells more frequently than specimens from EA patients (66). The difference in the inflammatory prostatic microenvironment between AAs and EAs may explain some of the PCa disparities. Microarray gene expression studies revealed different gene expression patterns between AA and EA prostate tumors for genes in immune-related pathways, including cytokines (e.g., IL1B, IL6, and IL8), which were over-expressed in PCa tissues from AAs compared to tissues from EAs (21–23, 67). Many genes were also differentially expressed in the stromal compartment, and approximately 20% of the identified pathways are immune-related, especially cytokine-mediated pathways (23). Giangreco et al. (57) also found that expression of IL6 is more than 18-fold higher in PCa-associated stroma than in PCa or benign epithelium. These studies suggested that both stromal and epithelial cells differentially express immune-related genes and contribute to the inflammatory environment. The stroma surrounding epithelium is known to play an important role in prostate development as well as PCa progression. The stroma microenvironment is complex and consists of monocytes, macrophages, T cells, and neutrophils alongside smooth muscle cells, myofibroblasts, fibroblasts, and collagen fibers (68). Stromal components regulate epithelial cell differentiation and proliferation and also mediate immune response of epithelial cells. The prostatic stroma becomes reactive early in PCa development and coevolves with epithelial cells during progression (68, 69).
Genetic variants may affect expression of genes involved in immune response and also angiogenesis. Genetic studies conducted mainly in EAs showed associations of inflammation pathway gene single nucleotide polymorphisms (SNPs) with PCa, and evidence of associations with aggressive PCa was stronger (70–74). Recent studies in African descent populations also showed that SNPs in many inflammatory genes were associated with PCa (74–79). Allele frequencies of cytokine gene SNPs differ significantly between AAs and EAs, and the frequencies of alleles that upregulate proinflammatory cytokines are higher in AAs than in EAs (80, 81). Cytokines modulate immune response involved in angiogenesis, and proinflammatory cytokine levels are elevated in advanced PCa patients (82–84). The levels of inflammatory markers in serum also vary between racial/ethnic groups, and AAs have higher levels of circulating proinflammatory markers than EAs (85, 86).
However, the effects of genomic variants on the expression of inflammatory genes and the production of cytokines have not been well explored. Moreover, chronic inflammation may cause epigenetic changes and genomic instability, which may promote aggressive PCa in AAs. Additionally, more research is needed to uncover biologically significant environmental exposures that contribute to the differential immune response between AAs and EAs.
Vitamin D and Inflammation
In addition to calcium homeostasis, vitamin D also functions as a modulator of innate and adaptive immune response. Population-based studies, as well as molecular studies, have demonstrated that vitamin D is implicated in many immune-related diseases, such as asthma, atherosclerosis, type 2 diabetes, and autoimmune diseases (87, 88). Serum 25(OH)D levels are also inversely associated with circulating proinflammatory cytokine levels (89–96). Vitamin D supplementation and fortification likely reduce serum proinflammatory markers’ levels (97–101), but other supplementation trials have shown no significant effects of vitamin D supplementation on proinflammatory markers levels (102–105). One study explored the relationship between circulating 25(OH)D and proinflammatory markers in AAs (105). In that study, baseline 25(OH)D levels was significantly associated with C-reactive protein levels, but 3 months of supplementation did not affect inflammatory markers’ levels. These studies varied in the participants’ baseline 25(OH)D levels, length of the trials, and supplementation dosage. In addition, individual genetic variation in vitamin D metabolism and signaling may impact response to vitamin D supplementation and effectiveness of supplementation to regulate inflammatory response (106).
Vitamin D modulates immune response by regulating expression of immune-related genes, such as cytokines, in very complex ways through the VDR activities. VDR is a nuclear transcription factor that interacts with a multitude of signaling pathways and thereby regulates the inflammatory response through transcription (88). Genome-wide screening using non-prostate cell lines (immune cells and colorectal cancer cells) recently identified over 10,000 new VDR-binding sites (107), and many cytokines, cytokine receptors, and other immune-related genes were identified as VDR targets (108–110). Vitamin D supplementation can also alter the expression of genes involved in immune response, including CD14, the gene that encodes surface antigen expressed on monocytes and macrophages, and NFKBIA, a gene for a protein that inhibits NF-κB, which plays a key role in regulating the immune response to infection (106, 111). Other studies found that polymorphisms in the VDR affect cytokine expression and protein production in peripheral blood mononuclear cells (112–114).
1,25(OH)2D binds to the VDR present on B cells, T cells, and antigen-presenting cells and affects the local immunologic milieu (115). The VDR gene expression and VDR signaling affect T cell development, differentiation, and function (116). In vitro studies have also shown that 1,25(OH)2D reduces production of proinflammatory cytokines, including IL-6, IL-8, and tumor necrosis factor α (TNF-α), in monocytes, macrophages, and preadipocytes (117–119). The prostate, like many other organs, harbors immune cells, and the cytokines produced by immune cells in the prostate may promote PCa pathogenesis, proliferation, and metastasis.
Implications for Prostate Cancer
In the prostate, 1,25(OH)2D inhibits production of proinflammatory molecules that contribute to PCa initiation and growth (16, 17), but the molecular pathways involving vitamin D and inflammation in the context of PCa is not well explored. We know that for prostate epithelial cells treated with 1,25(OH)2D, TNFα, IL6, and IL8 expression is suppressed, while TNFα and PTGS2 expression is suppressed in stromal cells (57). PTGS2 encodes COX-2, cyclooxygenase 2, an enzyme that converts arachidonic acid to proinflammatory prostaglandins. PTGS2 levels are higher in PCa (120, 121) and 1,25(OH)2D suppressed PTGS2 expression in PCa cells (122). 1,25(OH)2D also inhibits NF-κB signaling reducing IL-8 production (123). NF-κB is a protein complex involved in the regulation of transcription of numerous genes involved in inflammatory and immune response. Specifically, 1,25(OH)D reduces downstream production of IL-8 production by inhibiting the binding of NF-κB to DNA response elements (Figure 1).
Genome-wide screening using non-prostate cell lines found little overlap in VDR-binding sites, suggesting VDR binding is cell-specific (107). Thus, a study using prostate tissue is necessary in order to identify prostate-specific VDR-binding sites and to further understand the role of vitamin D in PCa. However, microarray studies using PCa cell lines have identified some VDR targets, and some of the targets are genes mediating downstream productions of cytokines (18, 19). One of the VDREs that were identified is mitogen-activated protein kinase phosphates 5 (MKP5). MKP5 was upregulated in response to 1,25(OH)2D treatment (18). Upregulation of MKP5 inactivated p38 resulting in reduced IL-6 production (124). 1,25(OH)2D also attenuated TNF-α-stimulated p38 activity to reduce IL-6 production.
1,25(OH)2D can also impact inflammation and PCa through its regulation of microRNAs (miRNAs) expression. miRNA is a small non-coding RNA molecule of about 22 nucleotides that has post-transcription gene regulatory functions. Studies have identified miRNAs that are regulated by 1,25(OH)2D (125–128), and eight miRNAs, including miR-100 and mi125b, were positively correlated to prostatic 1,25(OH)D levels from PCa tissues from the vitamin D supplementation clinical trials (129, 130). Some of these miRNAs are also involved in the regulation of cancer-associated inflammatory response (16, 131).
The aforementioned studies provided some mechanistic insights into vitamin D regulation of prostatic inflammation. PCa develops and grows slowly over decades, indicating that the protective effects of vitamin D must include regulatory processes other than cell proliferation. Given the well-characterized actions of vitamin D on immune cells, vitamin D’s anti-inflammatory actions are likely to influence PCa initiation and progression. However, previous studies have only identified a few vitamin D inflammatory pathways, which could putatively lead to PCa, and there are other relationships between vitamin D and immune-related genes for PCa initiation and progression remain unexplored. Further investigation is necessary to elucidate the mechanisms, by which vitamin D regulates immune gene expression in indolent and aggressive prostate tumors.
Clinical Significance and Future Directions
Understanding how vitamin D affects PCa initiation and progression may contribute to the development of better primary prevention and therapeutic strategies using vitamin D supplementation, especially AAs who are at high risk for both vitamin D deficiency and aggressive PCa. In a vitamin D supplementation trial among healthy AA men from Boston, 3 months of supplementation use did not lower PSA levels (132), but there are several clinical trials that have demonstrated benefits of vitamin D supplementation in PCa patients. PSA velocity and PSA doubling time are strong predictors of PCa mortality, and persistently rising PSA levels after radical prostatectomy or radiation therapy indicates biochemical recurrence (133). In vitamin D supplementation trials among PCa patients, supplementation reduced PSA levels and rate of PSA rise, and increased PSA doubling time (130, 134, 135). In a pilot clinical trial of low risk PCa patients who were on active surveillance and had a repeat biopsy at 1 year (27% AAs), supplementation did not lower their PSA levels, but men on supplements had a decreased number of positive cores and no increase in Gleason Score (136). In another vitamin D supplementation trial in Canada that included four (6%) black Canadians, prostatic 25(OH)D and 1,25(OH)2D were significantly higher in supplement groups (130). In laser-capture microdissected PCa epithelium from the study, PTGS2 expression was lower in the highest prostatic 1,25(OH)2D tertile compared to the lowest tertile (57).
In these vitamin D supplementation trials, supplementation improved clinical characteristics of many PCa patients but not all. Future studies need to investigate dosage necessary for the non-responders and genetic variations, epigenomic changes, and biological and behavioral factors that modify the efficacy of vitamin D supplementation in non-responders. For example, VDR and inflammatory gene variants may alter the effectiveness of vitamin D supplementation. It is also possible that combined use of vitamin D supplement and NSAIDs that inhibit COX-2 enzymatic activities is more effective for primary prevention and clinical management (17, 122).
Despite the high PCa incidence and mortality in AAs and tumor biological differences between AAs and EAs, AAs are still underrepresented in PCa epidemiologic studies, clinical trials, and molecular mechanistic studies. Differences in genetic variation partly account for the PCa disparities between AAs and other racial/ethnic groups (137–141). However, genome-wide association study in AAs did not identify immune and vitamin D-related gene variant as a risk locus for PCa, and it is likely that behavioral and biological factors, such as serum vitamin D levels, modify the associations between immune and vitamin D-related gene variants and PCa. Future studies need to explore these behavioral and biological factors that modify the relationship between PCa and immune response. Vitamin D may modify associations between sequence variants in immune-related genes and PCa. Sequence variants, especially in and around VDRE, may have heterogeneous effects on PCa between vitamin D-deficient and -sufficient individuals.
Future studies also need to explore the epigenomic effects of vitamin D. Vitamin D may regulate immune response through epigenetic mechanisms. Vitamin D supplementation may induce epigenetic changes to VDR and VDR targets (142, 143). Diverse methylation patterns were observed between a 1,25(OH)2D-responsive non-malignant prostate cell line and a non-responsive PCa cell line after treatment with vitamin D (144). It is clear though those actions of the VDR are very complex. Epigenetic regulations by VDR involve interactions with corepressors, such as NCOR1, histone deacetylases (HDACs), and miRNA, to repress transcription and with coactivators and histone acetyltransferases (HATs) for gene transcription (142, 145). However, the epigenetic regulatory role of vitamin D in inflammatory response in PCa cells has not been explored.
In conclusion, chronic vitamin D deficiency may create a tumor microenvironment with increased inflammation. This type of tumor microenvironment could be more common in PCa from AA patients than EA patients or could cause tumors to become more aggressive. However, the vitamin D inflammation pathways have not been a well-explored mechanism in PCa pathogenesis and progression. Future studies need to explore this relationship in order to improve our understanding of the biologic basis of PCa health disparities.
Author Contributions
KB, ABM, LN, and RAK each contributed to the literature review and drafting of this manuscript.
Conflict of Interest Statement
The authors declare that the research was conducted in the absence of any commercial or financial relationships that could be construed as a potential conflict of interest.
Funding
The authors would like to acknowledge the support from the National Institutes of Health (1R01MD007105-01 and P50CA090386), the U.S. Department of Defense (W81XWH-10-1-0532), and the Veterans Health Administration (1IK2 CX000926-01).
References
1. Chornokur G, Dalton K, Borysova ME, Kumar NB. Disparities at presentation, diagnosis, treatment, and survival in African American men, affected by prostate cancer. Prostate (2011) 71:985–97. doi: 10.1002/pros.21314
2. Major J, Norman Oliver M, Doubeni C, Hollenbeck A, Graubard B, Sinha R. Socioeconomic status, healthcare density, and risk of prostate cancer among African American and Caucasian men in a large prospective study. Cancer Causes Control (2012) 23:1185–91. doi:10.1007/s10552-012-9988-8
3. Gilbert R, Metcalfe C, Fraser WD, Donovan J, Hamdy F, Neal DE, et al. Associations of circulating 25-hydroxyvitamin D with prostate cancer diagnosis, stage and grade. Int J Cancer (2012) 131:1187–96. doi:10.1002/ijc.27327
4. Shui IM, Mucci LA, Kraft P, Tamimi RM, Lindstrom S, Penney KL, et al. Vitamin D-related genetic variation, plasma vitamin D, and risk of lethal prostate cancer: a prospective nested case-control study. J Natl Cancer Inst (2012) 104:690–9. doi:10.1093/jnci/djs189
5. Murphy AB, Nyame Y, Martin IK, Catalona WJ, Hollowell CMP, Nadler RB, et al. Vitamin D deficiency predicts prostate biopsy outcomes. Clin Cancer Res (2014) 20:2289–99. doi:10.1158/1078-0432.ccr-13-3085
6. Ahn J, Peters U, Albanes D, Purdue MP, Abnet CC, Chatterjee N, et al. Serum vitamin D concentration and prostate cancer risk: a nested case-control study. J Natl Cancer Inst (2008) 100:796–804. doi:10.1093/jnci/djn152
7. Travis RC, Crowe FL, Allen NE, Appleby PN, Roddam AW, Tjønneland A, et al. Serum vitamin D and risk of prostate cancer in a case-control analysis nested within the European prospective investigation into cancer and nutrition (EPIC). Am J Epidemiol (2009) 169:1223–32. doi:10.1093/aje/kwp022
8. Albanes D, Mondul AM, Yu K, Parisi D, Horst RL, Virtamo J, et al. Serum 25-hydroxy vitamin D and prostate cancer risk in a large nested case-control study. Cancer Epidemiol Biomarkers Prev (2011) 20:1850–60. doi:10.1158/1055-9965.epi-11-0403
9. Park S-Y, Cooney RV, Wilkens LR, Murphy SP, Henderson BE, Kolonel LN. Plasma 25-hydroxyvitamin D and prostate cancer risk: the multiethnic cohort. Eur J Cancer (2010) 46:932–6. doi:10.1016/j.ejca.2009.12.030
10. Egan K, Signorello L, Munro H, Hargreaves M, Hollis B, Blot W. Vitamin D insufficiency among African-Americans in the southeastern United States: implications for cancer disparities (United States). Cancer Causes Control (2008) 19:527–35. doi:10.1007/s10552-008-9115-z
11. Jacobs ET, Alberts DS, Foote JA, Green SB, Hollis BW, Yu Z, et al. Vitamin D insufficiency in southern Arizona. Am J Clin Nutr (2008) 87:608–13.
12. Levis S, Gomez A, Jimenez C, Veras L, Ma F, Lai S, et al. Vitamin D deficiency and seasonal variation in an adult South Florida population. J Clin Endocrinol Metab (2005) 90:1557–62. doi:10.1210/jc.2004-0746
13. Grant WB, Peiris AN. Possible role of serum 25-hydroxyvitamin D in Black-White health disparities in the United States. J Am Med Dir Assoc (2010) 11:617–28. doi:10.1016/j.jamda.2010.03.013
14. Hollis BW, Marshall DT, Savage SJ, Garrett-Mayer E, Kindy MS, Gattoni-Celli S. Vitamin D3 supplementation, low-risk prostate cancer, and health disparities. J Steroid Biochem Mol Biol (2013) 136:233–7. doi:10.1016/j.jsbmb.2012.11.012
15. Deeb KK, Trump DL, Johnson CS. Vitamin D signalling pathways in cancer: potential for anticancer therapeutics. Nat Rev Cancer (2007) 7:684–700. doi:10.1038/nrc2196
16. Feldman D, Krishnan AV, Swami S, Giovannucci E, Feldman BJ. The role of vitamin D in reducing cancer risk and progression. Nat Rev Cancer (2014) 14:342–57. doi:10.1038/nrc3691
17. Krishnan AV, Feldman D. Molecular pathways mediating the anti-inflammatory effects of calcitriol: implications for prostate cancer chemoprevention and treatment. Endocr Relat Cancer (2010) 17:R19–38. doi:10.1677/erc-09-0139
18. Peehl DM, Shinghal R, Nonn L, Seto E, Krishnan AV, Brooks JD, et al. Molecular activity of 1,25-dihydroxyvitamin D3 in primary cultures of human prostatic epithelial cells revealed by cDNA microarray analysis. J Steroid Biochem Mol Biol (2004) 92:131–41. doi:10.1016/j.jsbmb.2004.07.003
19. Krishnan AV, Shinghal R, Raghavachari N, Brooks JD, Peehl DM, Feldman D. Analysis of vitamin D-regulated gene expression in LNCaP human prostate cancer cells using cDNA microarrays. Prostate (2004) 59:243–51. doi:10.1002/pros.20006
20. De Marzo AM, Platz EA, Sutcliffe S, Xu J, Gronberg H, Drake CG, et al. Inflammation in prostate carcinogenesis. Nat Rev Cancer (2007) 7:256–69. doi:10.1038/nrc2090
21. Powell IJ, Dyson G, Land S, Ruterbusch J, Bock CH, Lenk S, et al. Genes associated with prostate cancer are differentially expressed in African American and European American men. Cancer Epidemiol Biomarkers Prev (2013) 22:891–7. doi:10.1158/1055-9965.epi-12-1238
22. Wallace TA, Prueitt RL, Yi M, Howe TM, Gillespie JW, Yfantis HG, et al. Tumor immunobiological differences in prostate cancer between African-American and European-American men. Cancer Res (2008) 68:927–36. doi:10.1158/0008-5472.can-07-2608
23. Kinseth MA, Jia Z, Rahmatpanah F, Sawyers A, Sutton M, Wang-Rodriguez J, et al. Expression differences between African American and Caucasian prostate cancer tissue reveals that stroma is the site of aggressive changes. Int J Cancer (2014) 134:81–91. doi:10.1002/ijc.28326
24. Siegel R, Ma J, Zou Z, Jemal A. Cancer statistics, 2014. CA Cancer J Clin (2014) 64:9–29. doi:10.3322/caac.21208
25. Pettaway CA, Troncoso P, Ramirez EI, Johnston DA, Steelhammer L, Babaian RJ. Prostate specific antigen and pathological features of prostate cancer in black and white patients: a comparative study based on radical prostatectomy specimens. J Urol (1998) 160:437–42. doi:10.1016/S0022-5347(01)62919-7
26. Berger AD, Satagopan J, Lee P, Taneja SS, Osman I. Differences in clinicopathologic features of prostate cancer between black and white patients treated in the 1990s and 2000s. Urology (2006) 67:120–4. doi:10.1016/j.urology.2005.08.005
27. Bigler SA, Pound CR, Zhou X. A retrospective study on pathologic features and racial disparities in prostate cancer. Prostate Cancer (2011) 2011:239460. doi:10.1155/2011/239460
28. Cullen J, Brassell SA, Chen Y, Porter C, L’esperance J, Brand T, et al. Racial/ethnic patterns in prostate cancer outcomes in an active surveillance cohort. Prostate Cancer (2011) 2011:234519. doi:10.1155/2011/234519
29. Ha Y-S, Salmasi A, Karellas M, Singer EA, Kim JH, Han M, et al. Increased incidence of pathologically nonorgan confined prostate cancer in African-American men eligible for active surveillance. Urology (2013) 81:831–6. doi:10.1016/j.urology.2012.12.046
30. Sundi D, Ross AE, Humphreys EB, Han M, Partin AW, Carter HB, et al. African American men with very low-risk prostate cancer exhibit adverse oncologic outcomes after radical prostatectomy: should active surveillance still be an option for them? J Clin Oncol (2013) 31:2991–7. doi:10.1200/JCO.2012.47.0302
31. Farrell J, Petrovics G, McLeod D, Srivastava S. Genetic and molecular differences in prostate carcinogenesis between African American and Caucasian American men. Int J Mol Sci (2013) 14:15510–31. doi:10.3390/ijms140815510
32. Martin DN, Starks AM, Ambs S. Biological determinants of health disparities in prostate cancer. Curr Opin Oncol (2013) 25:235–41. doi:10.1097/CCO.0b013e32835eb5d1
33. Powell IJ, Bock CH, Ruterbusch JJ, Sakr W. Evidence supports a faster growth rate and/or earlier transformation to clinically significant prostate cancer in Black than in White American men, and influences racial progression and mortality disparity. J Urol (2010) 183:1792–7. doi:10.1016/j.juro.2010.01.015
34. Garland CF, Garland FC, Gorham ED, Lipkin M, Newmark H, Mohr SB, et al. The role of vitamin D in cancer prevention. Am J Public Health (2006) 96:252–61. doi:10.2105/ajph.2004.045260
35. Giovannucci E. The epidemiology of vitamin D and cancer incidence and mortality: a review (United States). Cancer Causes Control (2005) 16:83–95. doi:10.1007/s10552-004-1661-4
36. Fang F, Kasperzyk JL, Shui I, Hendrickson W, Hollis BW, Fall K, et al. Prediagnostic plasma vitamin D metabolites and mortality among patients with prostate cancer. PLoS One (2011) 6:e18625. doi:10.1371/journal.pone.0018625
37. Li H, Stampfer MJ, Hollis JBW, Mucci LA, Gaziano JM, Hunter D, et al. A prospective study of plasma vitamin D metabolites, vitamin D receptor polymorphisms, and prostate cancer. PLoS Med (2007) 4:e103. doi:10.1371/journal.pmed.0040103
38. Tretli S, Hernes E, Berg JP, Hestvik UE, Robsahm TE. Association between serum 25(OH)D and death from prostate cancer. Br J Cancer (2009) 100:450–4. doi:10.1038/sj.bjc.6604865
39. Gann PH, Ma J, Hennekens CH, Hollis BW, Haddad JG, Stampfer MJ. Circulating vitamin D metabolites in relation to subsequent development of prostate cancer. Cancer Epidemiol Biomarkers Prev (1996) 5:121–6.
40. Jacobs ET, Giuliano AR, Martínez ME, Hollis BW, Reid ME, Marshall JR. Plasma levels of 25-hydroxyvitamin D, 1,25-dihydroxyvitamin D and the risk of prostate cancer. J Steroid Biochem Mol Biol (2004) 8(9–90):533–7. doi:10.1016/j.jsbmb.2004.03.063
41. Platz EA, Leitzmann MF, Hollis BW, Willett WC, Giovannucci E. Plasma 1,25-dihydroxy- and 25-hydroxyvitamin D and subsequent risk of prostate cancer. Cancer Causes Control (2004) 15:255–65. doi:10.1023/B:CACO.0000024245.24880.8a
42. Deschasaux M, Souberbielle J-C, Latino-Martel P, Sutton A, Charnaux N, Druesne-Pecollo N, et al. A prospective study of plasma 25-hydroxyvitamin D concentration and prostate cancer risk. Br J Nutr (2016) 115:305–14. doi:10.1017/S0007114515004353
43. Kristal AR, Till C, Song X, Tangen CM, Goodman PJ, Neuhauser ML, et al. Plasma vitamin D and prostate cancer risk: results from the Selenium and Vitamin E Cancer Prevention Trial. Cancer Epidemiol Biomarkers Prev (2014) 23:1494–504. doi:10.1158/1055-9965.epi-14-0115
44. Chan J, Jaceldo-Siegl K, Fraser G. Determinants of serum 25 hydroxyvitamin D levels in a nationwide cohort of blacks and non-Hispanic whites. Cancer Causes Control (2010) 21:501–11. doi:10.1007/s10552-009-9481-1
45. Looker AC, Pfeiffer CM, Lacher DA, Schleicher RL, Picciano MF, Yetley EA. Serum 25-hydroxyvitamin D status of the US population: 1988-1994 compared with 2000-2004. Am J Clin Nutr (2008) 88:1519–27. doi:10.3945/ajcn.2008.26182
46. Batai K, Murphy A, Shah E, Ruden M, Newsome J, Agate S, et al. Common vitamin D pathway gene variants reveal contrasting effects on serum vitamin D levels in African Americans and European Americans. Hum Genet (2014) 133:1395–405. doi:10.1007/s00439-014-1472-y
47. Murphy AB, Kelley B, Nyame YA, Martin IK, Smith DJ, Castaneda L, et al. Predictors of serum vitamin D levels in African American and European American men in Chicago. Am J Mens Health (2012) 6:420–6. doi:10.1177/1557988312437240
48. Tseng M, Giri V, Bruner D, Giovannucci E. Prevalence and correlates of vitamin D status in African American men. BMC Public Health (2009) 9:191. doi:10.1186/1471-2458-9-191
49. Shea MK, Houston DK, Tooze JA, Davis CC, Johnson MA, Hausman DB, et al. Correlates and prevalence of insufficient 25-hydroxyvitamin D status in Black and White older adults: the Health, Aging and Body Composition Study. J Am Geriatr Soc (2011) 59:1165–74. doi:10.1111/j.1532-5415.2011.03476.x
50. Giovannucci E, Liu Y, Willett WC. Cancer incidence and mortality and vitamin D in black and white male health professionals. Cancer Epidemiol Biomarkers Prev (2006) 15:2467–72. doi:10.1158/1055-9965.epi-06-0357
52. Schwartz GG, Whitlatch LW, Chen TC, Lokeshwar BL, Holick MF. Human prostate cells synthesize 1,25-dihydroxyvitamin D3 from 25-hydroxyvitamin D3. Cancer Epidemiol Biomarkers Prev (1998) 7:391–5.
53. Barreto AM, Schwartz GG, Woodruff R, Cramer SD. 25-Hydroxyvitamin D3, the prohormone of 1,25-dihydroxyvitamin D3, inhibits the proliferation of primary prostatic epithelial cells. Cancer Epidemiol Biomarkers Prev (2000) 9:265–70.
54. Banks M, Holick MF. Molecular mechanism(s) involved in 25-hydroxyvitamin D’s antiproliferative effects in CYP27B1-transfected LNCaP cells. Anticancer Res (2015) 35:3773–9.
55. Li H-X, Gao J-M, Liang J-Q, Xi J-M, Fu M, Wu Y-J. Vitamin D3 potentiates the growth inhibitory effects of metformin in DU145 human prostate cancer cells mediated by AMPK/mTOR signalling pathway. Clin Exp Pharmacol Physiol (2015) 42:711–7. doi:10.1111/1440-1681.12409
56. Trump DL, Deeb KK, Johnson CS. Vitamin D: considerations in the continued development as an agent for cancer prevention and therapy. Cancer J (2010) 16:1–9. doi:10.1097/PPO.0b013e3181c51ee6
57. Giangreco AA, Dambal S, Wagner D, Van der Kwast T, Vieth R, Prins GS, et al. Differential expression and regulation of vitamin D hydroxylases and inflammatory genes in prostate stroma and epithelium by 1,25-dihydroxyvitamin D in men with prostate cancer and an in vitro model. J Steroid Biochem Mol Biol (2015) 148:156–65. doi:10.1016/j.jsbmb.2014.10.004
58. Krill D, DeFlavia P, Dhir R, Luo J, Becich MJ, Lehman E, et al. Expression patterns of vitamin D receptor in human prostate. J Cell Biochem (2001) 82:566–72. doi:10.1002/jcb.1185
59. Hendrickson WK, Flavin R, Kasperzyk JL, Fiorentino M, Fang F, Lis R, et al. Vitamin D receptor protein expression in tumor tissue and prostate cancer progression. J Clin Oncol (2011) 29:2378–85. doi:10.1200/jco.2010.30.9880
60. Strasner A, Karin M. Immune infiltration and prostate cancer. Front Oncol (2015) 5:128. doi:10.3389/fonc.2015.00128
61. Nelson WG, De Marzo AM, Isaacs WB. Prostate cancer. N Engl J Med (2003) 349:366–81. doi:10.1056/NEJMra021562
62. Sfanos KS, De Marzo AM. Prostate cancer and inflammation: the evidence. Histopathology (2012) 60:199–215. doi:10.1111/j.1365-2559.2011.04033.x
63. Gurel B, Lucia MS, Thompson IM, Goodman PJ, Tangen CM, Kristal AR, et al. Chronic inflammation in benign prostate tissue is associated with high-grade prostate cancer in the placebo arm of the Prostate Cancer Prevention Trial. Cancer Epidemiol Biomarkers Prev (2014) 23:847–56. doi:10.1158/1055-9965.epi-13-1126
64. Vidal AC, Howard LE, Moreira DM, Castro-Santamaria R, Andriole GL, Freedland SJ. Aspirin, NSAIDs, and risk of prostate cancer: results from the REDUCE study. Clin Cancer Res (2015) 21:756–62. doi:10.1158/1078-0432.CCR-14-2235
65. Moreira DM, Nickel JC, Gerber L, Muller RL, Andriole GL, Castro-Santamaria R, et al. Baseline prostate inflammation is associated with a reduced risk of prostate cancer in men undergoing repeat prostate biopsy: results from the REDUCE study. Cancer (2014) 120:190–6. doi:10.1002/cncr.28349
66. Eastham JA, May RA, Whatley T, Crow A, Venable DD, Sartor O. Clinical characteristics and biopsy specimen features in African-American and white men without prostate cancer. J Natl Cancer Inst (1998) 90:756–60. doi:10.1093/jnci/90.10.756
67. Reams RR, Agrawal D, Davis M, Yoder S, Odedina F, Kumar N, et al. Microarray comparison of prostate tumor gene expression in African-American and Caucasian American males: a pilot project study. Infect Agent Cancer (2009) 4:S3. doi:10.1186/1750-9378-4-S1-S3
68. Barron DA, Rowley DR. The reactive stroma microenvironment and prostate cancer progression. Endocr Relat Cancer (2012) 19:R187–204. doi:10.1530/ERC-12-0085
69. Hägglöf C, Bergh A. The stroma – a key regulator in prostate function and malignancy. Cancers (2012) 4:531–48. doi:10.3390/cancers4020531
70. Dossus L, Kaaks R, Canzian F, Albanes D, Berndt SI, Boeing H, et al. PTGS2 and IL6 genetic variation and risk of breast and prostate cancer: results from the Breast and Prostate Cancer Cohort Consortium (BPC3). Carcinogenesis (2010) 31:455–61. doi:10.1093/carcin/bgp307
71. Kwon EM, Salinas CA, Kolb S, Fu R, Feng Z, Stanford JL, et al. Genetic polymorphisms in inflammation pathway genes and prostate cancer risk. Cancer Epidemiol Biomarkers Prev (2011) 20:923–33. doi:10.1158/1055-9965.EPI-10-0994
72. Magalhães JF, Cortinhas AJ, Albuquerque CM, Baptista CS, Ribeiro R, Viegas C, et al. Interleukin-6 gene -174G>C and -636G>C promoter polymorphisms and prostate cancer risk. Mol Biol Rep (2013) 40:449–55. doi:10.1007/s11033-012-2079-9
73. Tan D, Wu X, Hou MIN, Lee SOK, Lou WEI, Wang J, et al. Interleukin-6 polymorphism is associated with more aggressive prostete caner. J Urol (2005) 174:753–6. doi:10.1097/01.ju.0000168723.42824.40
74. Zabaleta J, Lin H-Y, Sierra RA, Hall MC, Clark PE, Sartor OA, et al. Interactions of cytokine gene polymorphisms in prostate cancer risk. Carcinogenesis (2008) 29:573–8. doi:10.1093/carcin/bgm277
75. Batai K, Shah E, Murphy AB, Newsome J, Ruden M, Ahaghotu C, et al. Fine-mapping of IL16 gene and prostate cancer risk in African Americans. Cancer Epidemiol Biomarkers Prev (2012) 21:2059–68. doi:10.1158/1055-9965.epi-12-0707
76. Kidd L, Jones D, Rogers E, Kidd N, Beache S, Rudd J, et al. Chemokine ligand 5 (CCL5) and chemokine receptor (CCR5) genetic variants and prostate cancer risk among men of African Descent: a case-control study. Hered Cancer Clin Pract (2012) 10:16. doi:10.1186/1897-4287-10-16
77. Jones D, Ragin C, Kidd N, Flores-Obando R, Jackson M, McFarlane-Anderson N, et al. The impact of genetic variants in inflammatory-related genes on prostate cancer risk among men of African Descent: a case control study. Hered Cancer Clin Pract (2013) 11:19. doi:10.1186/1897-4287-11-19
78. Rogers EN, Jones DZ, Kidd NC, Yeyeodu S, Brock G, Ragin C, et al. Toll-like receptor-associated sequence variants and prostate cancer risk among men of African descent. Genes Immun (2013) 14:347–55. doi:10.1038/gene.2013.22
79. Mason TE, Ricks-Santi L, Chen W, Apprey V, Joykutty J, Ahaghotu C, et al. Association of CD14 variant with prostate cancer in African American men. Prostate (2010) 70:262–9. doi:10.1002/pros.21060
80. Van Dyke AL, Cote ML, Wenzlaff AS, Land S, Schwartz AG. Cytokine SNPs: comparison of allele frequencies by race and implications for future studies. Cytokine (2009) 46:236–44. doi:10.1016/j.cyto.2009.02.003
81. Ness RB, Haggerty CL, Harger G, Ferrell R. Differential distribution of allelic variants in cytokine genes among African Americans and White Americans. Am J Epidemiol (2004) 160:1033–8. doi:10.1093/aje/kwh325
82. Adler HL, McCurdy MA, Kattan MW, Timme TL, Scardino PT, Thompson TC. Elevated levels of circulating interleukinn-6 and transforming growth factor-beta1 in patients with metastatic prostatic carcinoma. J Urol (1999) 161:182–7. doi:10.1016/S0022-5347(01)62092-5
83. Michalaki V, Syrigos K, Charles P, Waxman J. Serum levels of IL-6 and TNF-alpha correlate with clinicopathological features and patient survival in patients with prostate cancer. Br J Cancer (2004) 90:2312–6. doi:10.1038/sj.bjc.6601814
84. Tazaki E, Shimizu N, Tanaka R, Yoshizumi M, Kamma H, Imoto S, et al. Serum cytokine profiles in patients with prostate carcinoma. Exp Ther Med (2011) 2:887–91. doi:10.3892/etm.2011.286
85. Coe CL, Love GD, Karasawa M, Kawakami N, Kitayama S, Markus HR, et al. Population differences in proinflammatory biology: Japanese have healthier profiles than Americans. Brain Behav Immun (2011) 25:494–502. doi:10.1016/j.bbi.2010.11.013
86. Beasley LE, Koster A, Newman AB, Javaid MK, Ferrucci L, Kritchevsky SB, et al. Inflammation and race and gender differences in computerized tomography-measured adipose depots. Obesity (2009) 17:1062–9. doi:10.1038/oby.2008.627
87. Wacker M, Holick M. Vitamin D – effects on skeletal and extraskeletal health and the need for supplementation. Nutrients (2013) 5:111–48. doi:10.3390/nu5010111
88. Wöbke TK, Sorg BL, Steinhilber D. Vitamin D in inflammatory diseases. Front Physiol (2014) 5:244. doi:10.3389/fphys.2014.00244
89. Barker T, Martins T, Hill H, Kjeldsberg C, Dixon B, Schneider E, et al. Circulating pro-inflammatory cytokines are elevated and peak power output correlates with 25-hydroxyvitamin D in vitamin D insufficient adults. Eur J Appl Physiol (2013) 113:1523–34. doi:10.1007/s00421-012-2582-7
90. Bellia A, Garcovich C, D’Adamo M, Lombardo M, Tesauro M, Donadel G, et al. Serum 25-hydroxyvitamin D levels are inversely associated with systemic inflammation in severe obese subjects. Intern Emerg Med (2013) 8:33–40. doi:10.1007/s11739-011-0559-x
91. Peterson C, Heffernan M. Serum tumor necrosis factor-alpha concentrations are negatively correlated with serum 25(OH)D concentrations in healthy women. J Inflamm (2008) 5:10. doi:10.1186/1476-9255-5-10
92. Sun X, Cao Z-B, Zhang Y, Ishimi Y, Tabata I, Higuchi M. Association between serum 25-hydroxyvitamin D and inflammatory cytokines in healthy adults. Nutrients (2014) 6:221–30. doi:10.3390/nu6010221
93. De Vita F, Lauretani F, Bauer J, Bautmans I, Shardell M, Cherubini A, et al. Relationship between vitamin D and inflammatory markers in older individuals. Age (2014) 36:1–13. doi:10.1007/s11357-014-9694-4
94. Amer M, Qayyum R. Relation between serum 25-hydroxyvitamin D and C-reactive protein in asymptomatic adults (from the continuous National Health and Nutrition Examination Survey 2001 to 2006). Am J Cardiol (2012) 109:226–30. doi:10.1016/j.amjcard.2011.08.032
95. Laird E, McNulty H, Ward M, Hoey L, McSorley E, Wallace JMW, et al. Vitamin D deficiency is associated with inflammation in older Irish adults. J Clin Endocrinol Metab (2014) 99:1807–15. doi:10.1210/jc.2013-3507
96. Chen N, Wan Z, Han S-F, Li B-Y, Zhang Z-L, Qin L-Q. Effect of vitamin D supplementation on the level of circulating high-sensitivity C-reactive protein: a meta-analysis of randomized controlled trials. Nutrients (2014) 6:2206–16. doi:10.3390/nu6062206
97. Beilfuss J, Berg V, Sneve M, Jorde R, Kamycheva E. Effects of a 1-year supplementation with cholecalciferol on interleukin-6, tumor necrosis factor-alpha and insulin resistance in overweight and obese subjects. Cytokine (2012) 60:870–4. doi:10.1016/j.cyto.2012.07.032
98. Hopkins MH, Owen J, Ahearn T, Fedirko V, Flanders WD, Jones DP, et al. Effects of supplemental vitamin D and calcium on biomarkers of inflammation in colorectal adenoma patients: a randomized, controlled clinical trial. Cancer Prev Res (2011) 4:1645–54. doi:10.1158/1940-6207.capr-11-0105
99. Schleithoff SS, Zittermann A, Tenderich G, Berthold HK, Stehle P, Koerfer R. Vitamin D supplementation improves cytokine profiles in patients with congestive heart failure: a double-blind, randomized, placebo-controlled trial. Am J Clin Nutr (2006) 83:754–9.
100. Yusupov E, Li-Ng M, Pollack S, Yeh JK, Mikhail M, Aloia JF. Vitamin D and serum cytokines in a randomized clinical trial. Int J Endocrinol (2010) 2010. doi:10.1155/2010/305054
101. Shab-Bidar S, Neyestani TR, Djazayery A, Eshraghian M-R, Houshiarrad A, Kalayi A, et al. Improvement of vitamin D status resulted in amelioration of biomarkers of systemic inflammation in the subjects with type 2 diabetes. Diabetes Metab Res Rev (2012) 28:424–30. doi:10.1002/dmrr.2290
102. Barnes MS, Horigan G, Cashman KD, Hill TR, Forsythe LK, Lucey AJ, et al. Maintenance of wintertime vitamin D status with cholecalciferol supplementation is not associated with alterations in serum cytokine concentrations among apparently healthy younger or older adults. J Nutr (2011) 141:476–81. doi:10.3945/jn.110.131516
103. Jorde R, Sneve M, Torjesen PA, Figenschau Y, Gøransson LG, Omdal R. No effect of supplementation with cholecalciferol on cytokines and markers of inflammation in overweight and obese subjects. Cytokine (2010) 50:175–80. doi:10.1016/j.cyto.2009.12.006
104. Pittas AG, Harris SS, Stark PC, Dawson-Hughes B. The effects of calcium and vitamin D supplementation on blood glucose and markers of inflammation in nondiabetic adults. Diabetes Care (2007) 30:980–6. doi:10.2337/dc06-1994
105. Chandler PD, Scott JB, Drake BF, Ng K, Manson JE, Rifai N, et al. Impact of vitamin D supplementation on inflammatory markers in African Americans: results of a four-arm, randomized, placebo-controlled trial. Cancer Prev Res (2014) 7:218–25. doi:10.1158/1940-6207.capr-13-0338-t
106. Carlberg C, Seuter S, de Mello VDF, Schwab U, Voutilainen S, Pulkki K, et al. Primary vitamin D target genes allow a categorization of possible benefits of vitamin D3 supplementation. PLoS One (2013) 8:e71042. doi:10.1371/journal.pone.0071042
107. Carlberg C. Genome-wide (over)view on the actions of vitamin D. Front Physiol (2014) 5:167. doi:10.3389/fphys.2014.00167
108. Ramagopalan SV, Heger A, Berlanga AJ, Maugeri NJ, Lincoln MR, Burrell A, et al. A ChIP-seq defined genome-wide map of vitamin D receptor binding: associations with disease and evolution. Genome Res (2010) 20:1352–60. doi:10.1101/gr.107920.110
109. Heikkinen S, Väisänen S, Pehkonen P, Seuter S, Benes V, Carlberg C. Nuclear hormone 1α,25-dihydroxyvitamin D3 elicits a genome-wide shift in the locations of VDR chromatin occupancy. Nucleic Acids Res (2011) 39:9181–93. doi:10.1093/nar/gkr654
110. Meyer MB, Goetsch PD, Pike JW. VDR/RXR and TCF4/β-catenin cistromes in colonic cells of colorectal tumor origin: impact on c-FOS and c-MYC gene expression. Mol Endocrinol (2012) 26:37–51. doi:10.1210/me.2011-1109
111. Hossein-nezhad A, Spira A, Holick MF. Influence of vitamin D status and vitamin D3 supplementation on genome wide expression of white blood cells: a randomized double-blind clinical trial. PLoS One (2013) 8:e58725. doi:10.1371/journal.pone.0058725
112. van Etten E, Verlinden L, Giulietti A, Ramos-Lopez E, Branisteanu DD, Ferreira GB, et al. The vitamin D receptor gene FokI polymorphism: functional impact on the immune system. Eur J Immunol (2007) 37:395–405. doi:10.1002/eji.200636043
113. Selvaraj P, Vidyarani M, Alagarasu K, Prabhu Anand S, Narayanan PR. Regulatory role of promoter and 3′ UTR variants of vitamin D receptor gene on cytokine response in pulmonary tuberculosis. J Clin Immunol (2008) 28:306–13. doi:10.1007/s10875-007-9152-5
114. Al-Daghri NM, Guerini FR, Al-Attas OS, Alokail MS, Alkharfy KM, Draz HM, et al. Vitamin D receptor gene polymorphisms are associated with obesity and inflammasome activity. PLoS One (2014) 9:e102141. doi:10.1371/journal.pone.0102141
115. Aranow C. Vitamin D and the immune system. J Investig Med (2011) 59:881–6. doi:10.231/JIM.0b013e31821b8755
116. Kongsbak M, Levring TB, Geisler C, von Essen MR. The vitamin D receptor and T cell function. Front Immunol (2013) 4:148. doi:10.3389/fimmu.2013.00148
117. Di Rosa M, Malaguarnera G, De Gregorio C, Palumbo M, Nunnari G, Malaguarnera L. Immuno-modulatory effects of vitamin D3 in human monocyte and macrophages. Cell Immunol (2012) 280:36–43. doi:10.1016/j.cellimm.2012.10.009
118. Gao D, Trayhurn P, Bing C. 1,25-Dihydroxyvitamin D3 inhibits the cytokine-induced secretion of MCP-1 and reduces monocyte recruitment by human preadipocytes. Int J Obes (2013) 37:357–65. doi:10.1038/ijo.2012.53
119. Zhang Y, Leung DYM, Richers BN, Liu Y, Remigio LK, Riches DW, et al. Vitamin D inhibits monocyte/macrophage proinflammatory cytokine production by targeting MAPK phosphatase-1. J Immunol (2012) 188:2127–35. doi:10.4049/jimmunol.1102412
120. Gupta S, Srivastava M, Ahmad N, Bostwick DG, Mukhtar H. Over-expression of cyclooxygenase-2 in human prostate adenocarcinoma. Prostate (2000) 42:73–8. doi:10.1002/(SICI)1097-0045(20000101)42:1<73::AID-PROS9>3.0.CO;2-G
121. Kirschenbaum A, Klausner AP, Lee R, Unger P, Yao S, Liu X-H, et al. Expression of cyclooxygenase-1 and cyclooxygenase-2 in the human prostate. Urology (2000) 56:671–6. doi:10.1016/S0090-4295(00)00674-9
122. Moreno J, Krishnan AV, Swami S, Nonn L, Peehl DM, Feldman D. Regulation of prostaglandin metabolism by calcitriol attenuates growth stimulation in prostate cancer cells. Cancer Res (2005) 65:7917–25. doi:10.1158/0008-5472.can-05-1435
123. Bao B-Y, Yao J, Lee Y-F. 1α, 25-dihydroxyvitamin D3 suppresses interleukin-8-mediated prostate cancer cell angiogenesis. Carcinogenesis (2006) 27:1883–93. doi:10.1093/carcin/bgl041
124. Nonn L, Peng L, Feldman D, Peehl DM. Inhibition of p38 by vitamin D reduces interleukin-6 production in normal prostate cells via mitogen-activated protein kinase phosphatase 5: implications for prostate cancer prevention by vitamin D. Cancer Res (2006) 66:4516–24. doi:10.1158/0008-5472.CAN-05-3796
125. Giangreco AA, Nonn L. The sum of many small changes: microRNAs are specifically and potentially globally altered by vitamin D3 metabolites. J Steroid Biochem Mol Biol (2013) 136:86–93. doi:10.1016/j.jsbmb.2013.01.001
126. Wang W-LW, Chatterjee N, Chittur SV, Welsh J, Tenniswood MP. Effects of 1α,25 dihydroxyvitamin D3 and testosterone on miRNA and mRNA expression in LNCaP cells. Mol Cancer (2011) 10:1–15. doi:10.1186/1476-4598-10-58
127. Kutmon M, Coort SL, de Nooijer K, Lemmens C, Evelo C. Integrative network-based analysis of mRNA and microRNA expression in 1,25-dihydroxyvitamin D3-treated cancer cells. Genes Nutr (2015) 10:1–12. doi:10.1007/s12263-015-0484-0
128. Singh PK, Long MD, Battaglia S, Hu Q, Liu S, Sucheston-Campbell LE, et al. VDR regulation of microRNA differs across prostate cell models suggesting extremely flexible control of transcription. Epigenetics (2015) 10:40–9. doi:10.4161/15592294.2014.989088
129. Giangreco AA, Vaishnav A, Wagner D, Finelli A, Fleshner N, Van der Kwast T, et al. Tumor suppressor microRNAs, miR-100 and -125b, are regulated by 1,25-dihydroxyvitamin D in primary prostate cells and in patient tissue. Cancer Prev Res (2013) 6:483–94. doi:10.1158/1940-6207.capr-12-0253
130. Wagner D, Trudel D, Van der Kwast T, Nonn L, Giangreco AA, Li D, et al. Randomized clinical trial of vitamin D3 doses on prostatic vitamin D metabolite levels and ki67 labeling in prostate cancer patients. J Clin Endocrinol Metab (2013) 98:1498–507. doi:10.1210/jc.2012-4019
131. Schetter AJ, Heegaard NHH, Harris CC. Inflammation and cancer: interweaving microRNA, free radical, cytokine and p53 pathways. Carcinogenesis (2010) 31:37–49. doi:10.1093/carcin/bgp272
132. Chandler PD, Giovannucci EL, Scott JB, Bennett GG, Ng K, Chan AT, et al. Null association between vitamin D and PSA levels among black men in a vitamin D supplementation trial. Cancer Epidemiol Biomarkers Prev (2014) 23:1944–7. doi:10.1158/1055-9965.epi-14-0522
133. D’Amico AV, Renshaw AA, Sussman B, Chen M. Pretreatment PSA velocity and risk of death from prostate cancer following external beam radiation therapy. JAMA (2005) 294:440–7. doi:10.1001/jama.294.4.440
134. Gross C, Stamey T, Hancock S, Feldman D. Treatment of early recurrent prostate cancer with 1,25-dihydroxyvitamin D3 (calcitriol). J Urol (1998) 159:2035–40. doi:10.1016/S0022-5347(01)63236-1
135. Beer TM, Lemmon D, Lowe BA, Henner WD. High-dose weekly oral calcitriol in patients with a rising PSA after prostatectomy or radiation for prostate carcinoma. Cancer (2003) 97:1217–24. doi:10.1002/cncr.11179
136. Marshall DT, Savage SJ, Garrett-Mayer E, Keane TE, Hollis BW, Horst RL, et al. Vitamin D3 supplementation at 4000 international units per day for one year results in a decrease of positive cores at repeat biopsy in subjects with low-risk prostate cancer under active surveillance. J Clin Endocrinol Metab (2012) 97:2315–24. doi:10.1210/jc.2012-1451
137. Haiman CA, Chen GK, Blot WJ, Strom SS, Berndt SI, Kittles RA, et al. Characterizing genetic risk at known prostate cancer susceptibility loci in African Americans. PLoS Genet (2011) 7:e1001387. doi:10.1371/journal.pgen.1001387
138. Haiman CA, Chen GK, Blot WJ, Strom SS, Berndt SI, Kittles RA, et al. Genome-wide association study of prostate cancer in men of African ancestry identifies a susceptibility locus at 17q21. Nat Genet (2011) 43:570–3. doi:10.1038/ng.839
139. Bock C, Schwartz A, Ruterbusch J, Levin A, Neslund-Dudas C, Land S, et al. Results from a prostate cancer admixture mapping study in African-American men. Hum Genet (2009) 126:637–42. doi:10.1007/s00439-009-0712-z
140. Freedman ML, Haiman CA, Patterson N, McDonald GJ, Tandon A, Waliszewska A, et al. Admixture mapping identifies 8q24 as a prostate cancer risk locus in African-American men. Proc Natl Acad Sci U S A (2006) 103:14068–73. doi:10.1073/pnas.0605832103
141. Han Y, Signorello LB, Strom SS, Kittles RA, Rybicki BA, Stanford JL, et al. Generalizability of established prostate cancer risk variants in men of African ancestry. Int J Cancer (2014) 136:1210–7. doi:10.1002/ijc.29066
142. Fetahu IS, Höbaus J, Kallay E. Vitamin D and the epigenome. Front Physiol (2014) 5:164. doi:10.3389/fphys.2014.00164
143. Rawson JB, Sun Z, Dicks E, Daftary D, Parfrey PS, Green RC, et al. Vitamin D intake is negatively associated with promoter methylation of the Wnt antagonist gene DKK1 in a large group of colorectal cancer patients. Nutr Cancer (2012) 64:919–28. doi:10.1080/01635581.2012.711418
144. Doig CL, Singh PK, Dhiman VK, Thorne JL, Battaglia S, Sobolewski M, et al. Recruitment of NCOR1 to VDR target genes is enhanced in prostate cancer cells and associates with altered DNA methylation patterns. Carcinogenesis (2013) 34:248–56. doi:10.1093/carcin/bgs331
Keywords: vitamin D, prostate cancer, inflammation, African Americans, health disparities
Citation: Batai K, Murphy AB, Nonn L and Kittles RA (2016) Vitamin D and Immune Response: Implications for Prostate Cancer in African Americans. Front. Immunol. 7:53. doi: 10.3389/fimmu.2016.00053
Received: 01 December 2015; Accepted: 04 February 2016;
Published: 22 February 2016
Edited by:
Kevin Sean Kimbro, North Carolina Central University, USAReviewed by:
Taruna Madan, National Institute for Research in Reproductive Health, IndiaPhilippe Georgel, Strasbourg University, France
Copyright: © 2016 Batai, Murphy, Nonn and Kittles. This is an open-access article distributed under the terms of the Creative Commons Attribution License (CC BY). The use, distribution or reproduction in other forums is permitted, provided the original author(s) or licensor are credited and that the original publication in this journal is cited, in accordance with accepted academic practice. No use, distribution or reproduction is permitted which does not comply with these terms.
*Correspondence: Rick A. Kittles, rkittles@email.arizona.edu