- 1UMR 1064, INSERM, Nantes, France
- 2Medicine Department, Nantes University, Nantes, France
- 3ITUN, Nantes Hospital, Nantes, France
- 4Department of Neurology, Nantes Hospital, Nantes, France
- 5Centre d’Investigation Clinique, INSERM 004, Nantes, France
Multiple sclerosis (MS) is a chronic autoimmune disease of the central nervous system characterized by focal demyelination patches associated with inflammatory infiltrates containing T lymphocytes. For decades, CD4+ T cells have been recognized as playing a major role in the disease, especially in animal models, which has led to the development of several therapies. However, interest has recently developed in the involvement of CD8+ T cells in MS following the analysis of infiltrating T cells in human brain lesions. A broad range of evidence now suggests that the pathological role of this T cell subset in MS may have been underestimated. In this review, we summarize the literature implicating CD8+ T cells in the pathophysiology of MS. We present data from studies in the fields of genetics, anatomopathology and immunology, mainly in humans but also in animal models of MS. Altogether, this strongly suggests that CD8+ T cells may be major effectors in the disease process, and that the development of treatments specifically targeting this subset would be germane.
Introduction
Multiple sclerosis (MS) is a chronic inflammatory disease of the central nervous system (CNS), resulting in disability. The clinical manifestations are very variable, and include motor, sensory, visual, and cognitive symptoms, none of them being disease specific (1). The disease is thought to be a result of both genetic and environmental factors, including infectious agents, vitamin D deficiency, obesity, and smoking (2). Neuropathological studies show disseminated patches of demyelination among the brain and the spinal cord, resulting in altered nerve conduction. Axonal loss can also be observed. Demyelinated patches are characterized by immune cell infiltration, which is absent in normal brain tissue. The infiltrate is mainly composed of macrophages, and, to a lesser extent, T and B lymphocytes (1, 3, 4).
In addition to the immune cell infiltrates, a wide range of evidence points to the pivotal role of the immune system in the development of the disease. Indeed, the genetic variants conferring a higher susceptibility to MS are associated to immune mechanisms, the animal models used for disease characterization and comprehension are autoimmune ones, and current treatments regulate or modulate the immune system (1). For decades, MS has been considered as being driven by CD4+ T cells, especially in animal models of the disease (1). However, treatment with monoclonal anti-CD4 antibody in phase II trials failed to reduce MS activity [measured by magnetic resonance imaging (MRI)] (5). In recent years, numerous other immune populations have been shown to be important in MS development. Among these, CD8+ T cells have appeared as potential major effectors within the CNS, especially in studies using human samples. In this review, we will focus on the various results that seem pivotal in understanding the involvement of CD8+ T cells in the pathophysiology of MS. The potential regulatory role of CD8+ T cells in the disease will be described elsewhere in this issue [for other reviews, see Ref. (6, 7)].
MHC Class I Genes’ Impact on MS Risk
Numerous alleles associated with immune response have been found to be linked with increased MS risk (8–12). The strongest association, with an odds ratio of 3, was seen for human leukocyte antigen (HLA)-DRB1*15:01 in European and United States populations, which identifies CD4+ T cells as potent effectors in the disease (13). Major histocompatibility complex (MHC) class I alleles are also associated with MS, though to a lesser extent. HLA-A3 and B7 alleles were the first to be described as associated with a higher risk of developing the disease (14, 15). More recently, HLA A*0301 was associated with a higher risk (2×) and HLA A*0201 with a protective effect (50%) (16, 17). These associations were confirmed by Friese and colleagues in “humanized” transgenic mice for HLA*0301 and/or HLA-A*0201. These mice either developed (HLA*0301) or were protected from (HLA*0201) the disease after proteolipid protein (PLP) injection (18). In addition, these alleles are known to work in synergy with MHC class-II alleles, such as DRB1*1501, resulting in an increased risk when both are present (16). These data strongly suggest that some CD8+ T cells may have a beneficial or pathogenic effect, depending on the genetic background (Table 1). On top of this genetic evidence, the presence of CD8+ T cells in MS lesions, as well as their cytotoxic profile, evinces their involvement in the disease.
CD8+ T Cells are Present and have a Pathogenic Profile in the MS CNS
CD8+ T Cell Infiltration in CNS Lesions
One of the major indicators pointing toward an implication of CD8+ T cells in the pathophysiology of MS is the presence of these cells, in a greater number than CD4+ T cells, in the brain lesions of MS patients. The fact that CD8+ T cells outnumber CD4+ T cells in MS lesions was first observed in the 80s, in particular in the parenchyma, and was regardless of differing clinical parameters, such as disease duration, disease evolution, and therapy (19). In 1986, Hauser et al. studied 16 cases of progressive MS, and observed up to 50 times more CD8+ T cells in both the parenchyma and in perivascular cuffs of active lesions, with no case of more CD4+ than CD8+ T cells. CD8+ T cells also predominated in normal-appearing white matter (NAWM) (20). Recently, our study of 22 lesions in three MS patients found the same, CD8+ T cells being predominant regardless of the lesion type studied (21). Other studies have reached the same conclusion by single cell analysis in different MS patients (22–24). Although not all the studies concur with the above (25), others put forward the hypothesis that CD8+ T cells might be more prevalent in the parenchyma while CD4+ T cells would stay in the perivascular areas (26). Recently, in tissue block section of MS patients, CD8+ T cells were described as being often present in cortical plaques (54 of 70 cortical plaques analyzed). This type of plaque has been found to be associated with disease progression and cognitive impairment in the early stages of MS (27).
MHC-I Expression and CNS Damage
MHC-I expression and presentation is necessary for CD8+ T cells to carry out their cytotoxic functions. In 2004, a study including 30 MS patients and 21 controls quantified the expression of MHC-I on various cell subtypes within the CNS by immunohistochemistry and fluorescence methods (28). While constitutive expression of MHC-I on macrophages/microglia and endothelial cells was observed, MHC-I expression was gradually upregulated on astrocytes, oligodendrocytes, neurons, and axons depending on the disease type (inactive, chronic active, and active MS) and lesion activity (inactive, periplaque white matter, and active), making these cells potential targets for CD8+ T cells in the context of the disease. Consistent with this, CD8+ T cells have been shown to be able to mediate axonal transection in vitro (29). In this study, murine neurons induced to express MHC-I were pulsed with a dominant peptide of the lymphochoriomeningitis virus envelope glycoprotein (GP33). Five to 30 min after culture with antigen-specific cytotoxic CD8+ T cells, neurite breakage appeared in contact zones between CD8+ T cells and neurites. Confocal live imaging gave a clear image of this process. Axonal transection has also been suggested in MS (30). Indeed, axonal injury, in 88 brain biopsy samples from 42 patients, correlated with the number of CD8+ T cells, but not CD3+ T cells, found in the lesions (31). Variable proportions of lesion-infiltrating CD8+ T cells express granzyme B [Figure 1, personal results from Ref. (21)] and interferon γ (IFNγ), further evincing the ability of these cells to damage the CNS (21, 25, 32). In conclusion, CD8+ T cells seem more likely than CD4+ T cells to mediate CNS damage, in particular through their cytotoxic and proinflammatory properties.
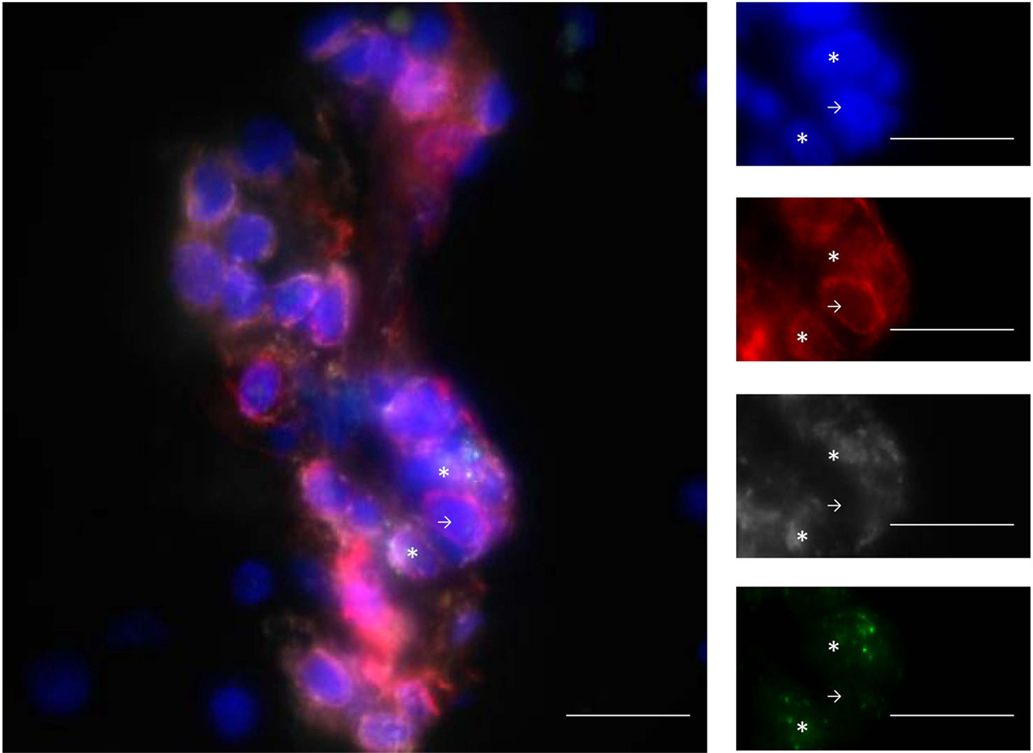
Figure 1. Infiltrating T cells are mainly CD8+ T cells and express GZM-B. Example of staining with DAPI (blue), CD3 (red), CD8 (gray), and GZM-B (green). The line in the pictures indicates 20 μm. Stars show CD3+CD8+GZM-B+ and arrows show CD3+CD8−GZM-B− cells. GZM-B: granzyme-B. From personal data.
Pathogenic CD8+ T Cells in the CSF
Deciphering the mechanisms involved in MS development is made difficult by the limited access to the CNS compartment. As such, a lot of studies focus on the cerebrospinal fluid (CSF) as a surrogate compartment for understanding the T cell processes occurring in situ. In 2007, an enrichment of effector memory CD8+ T cells in the CSF of 52 relapsing–remitting MS patients was observed at the beginning of the disease (33). This, together with an enrichment of granzyme B-expressing CD8+ T cells, has been confirmed in 17 other relapsing-remitting patients (25). Interestingly, the effector memory CD8+ T cells had increased in vitro migration through a model of the blood–brain barrier, especially those producing granzyme B, perforin, IFNγ, and interleukin 17 (IL-17). This was further confirmed in a mouse experimental autoimmune encephalomyelitis (EAE) model (25). Another study found that granzyme A and B levels were higher in the CSF of patients in flare up, compared to those in clinical remission and control patients (34). Altogether, these results suggest a specific enrichment of effector memory CD8+ T cells in the CNS compartment in MS and place them as disease effectors.
CD8+ T Cell Migration into the Brain
Studying the mechanisms leading to CD8+ T cell transmigration into the CNS further highlights their involvement in the disease process. Blockade of α4 integrin in EAE mice immunized with myelin oligodendrocyte glycoprotein (MOG)35–55 yields a decreased number of infiltrating CD8+ T cells, together with a reduced EAE score. However, a similar effect has been described for CD4+ T cells (25). More recently, melanoma cell adhesion molecule (MCAM), expressed by a subset of human effector CD8+ T cells, was reported to be upregulated during MS relapse compared to controls (35). Interestingly, MCAM blockade prevents the transmigration of human CD8+ T cells across a blood–brain barrier (BBB) model and decreases the EAE score in active, transfer and spontaneous models (36–38). As MCAM binds itself and laminin 411 (37), which are both expressed by endothelial cells, the mode of action of MCAM blockade is not yet known (35). P-glycoprotein (also known as multidrug resistance protein 1), a transporter involved in drug efflux (39) and in cytokine/chemokine secretion (40), has also been shown to be important for the trafficking of CD8+ T cells into the brain during the disease. Indeed, Mdr1a/b KO mice show significantly reduced EAE (40). In another study, P-glycoprotein silencing led to decreased CD8 infiltration into the brain, with no effect on CD4+ T cells (41). P-glycoprotein control of endothelial C–C chemokine ligand 2 (CCL2) secretion was responsible for this result. Indeed, EAE mice lacking this protein or CCL2 show significantly reduced CD8+ migration into the brain. More significantly, CCL2 transcript has also been found to be elevated in six MS lesions compared to six controls (41).
In conclusion, various studies on brain, spinal cord, and CSF, as well as on the mechanisms allowing T cell entry into the brain highlight CD8+ T cells’ potential role in the development of MS.
CD8+ T Cell Repertoire and Reactivity in MS
A number of studies have focused on the T cell pools that arise with MS in order to understand their role in its pathophysiology. Different CNS and non-CNS antigens have been used to search for autoreactive T cells (3, 42), but to date the triggering antigen(s) is unknown. The search for a specific antigen is made more difficult because of the mechanisms of molecular mimicry, epitope spreading, bystander activation, and/or dual T cell receptor (TCR) (43–48). Numerous studies have analyzed the T cell repertoire, allowing the identification of reactive T cells without the need to know the recognized antigen. Indeed, after antigen recognition, T cells undergo clonal proliferation, and this expanded T cell population can be identified within the total T cell pool.
CD8+ T Cell Repertoire in Lesions and in CSF
Two different groups, including our own, have studied the TCR repertoire of lesion-infiltrating T cells, either by single cell analysis (22, 23) or by whole TCR analysis using high-throughput sequencing, in comparison with sorted populations from the blood (21). These studies show that infiltrating CD8+ T cells are oligoclonal, i.e., only clones bearing particular TCRs specific to each patient are represented within the lesion, which is less the case for CD4+ T cells. These oligoclonal CD8+ T cells are also found in different locations in the brains of the same patients. In addition, some of these clones harbored silent mutations (23). These data strongly suggest antigen-driven selection or activation processes, and identify these infiltrating CD8+ T cells as disease effectors.
CD8+ T Cell Repertoire Alteration in CSF and Blood
Different studies have observed that the blood of MS patients exhibits more clonal expansions than that of controls (49–53). In 35 patients, our group showed that the repertoire was more skewed in the CD8 than in the CD4 compartment, further suggesting the involvement of CD8+ T cells (50). The repertoire was even more skewed in the CSF than the blood of MS patients, especially in CD8+ T cells which interestingly expressed memory T cell markers (54–56). Of note, clonal expansions were correlated to the clinical activity of the disease. We observed more blood expansions in MS patients with an active form of the disease, assessed by MRI (57). Muraro et al. published a case study that extensively studied the TCR Vβ repertoire in the CSF of one MS patient, and described more expansions in that compartment during relapse than during remission (58). Altogether, these data strongly suggest an implication of oligoclonal T cells, especially CD8+ T cells, in the pathophysiology of MS.
However, whether expanded T cells in blood and CSF correlates with T cell expansion in the CNS, which is likely to participate in disease development, was unknown until recently. Indeed, only one study had suggested that some expanded CNS CD8+ T cell clones could persist in the blood and in the CSF for several years, seen in two MS patients (24). Very recently, we confirmed and extended this result by comparing the TCR repertoires in the blood, CSF, and CNS (22 lesions with various locations and activities) of three MS patients (21). Using CDR3 spectratyping and high-throughput sequencing, we showed for the first time that the CSF repertoire mirrors that of the CNS, which is, to a lesser extent, also similar to that of blood CD8+ T cells. This further evinces the involvement of CSF and blood expanded CD8+ T cells in MS and further identifies CSF expanded T cells as good surrogates for infiltrating T cells.
CD8+ T Cell Autoreactivity
A number of studies have been looking at autoreactive T cells in MS, using different methods and antigens, but the results are hardly comparable between the studies and no consensus has been found as to the presence of CNS-specific CD4+ or CD8+ T cells in MS patients [reviewed in Ref. (42)]. In 2004, Crawford et al. adapted a flow cytometry approach to analyze antigen-specific CD4+ and CD8+ T cell proliferative response in MS patients. They showed that relapsing–remitting MS patients have a higher proportion of CD8+ T cell responses against CNS peptides compared to healthy volunteers (HV) or primary progressive MS patients and that this is not the case for CD4+ T cells (59). In another study, CD8+ T cells specific to apoptotic epitopes have been shown to be overrepresented in MS patients (in a cohort of 26 compared to 27 HV), and to be able to produce IFNγ and/or IL-17 when stimulated with apoptotic epitopes (60). Interestingly, these cells have been found also in the CSF and correlate with the level of disease disability, which strongly suggests their involvement in the immunopathology (60). Finally, Zang et al. tested the proliferation of CD8+ T cells in contact with irradiated PBMC incubated with degenerated myelin basic proteins (MBP), showing that autoreactive CD8+ T cells recognizing MBP proteins were increased in the blood (15 MS patients compared to 15 HV) (61). Recently, high-throughput sequencing on paired blood and CSF samples of MS patients and control gave further evidence of the implication of a particular subset of CD8+ T cells in MS (62), a specific enrichment of Epstein–Barr virus (EBV)-specific CD8+ T cells being observed in the CSF of MS patients.
These results evince the existence of a pool of CD8+ T cells able to damage the CNS, however the triggering events as well as the antigens recognized remain unclear, necessitating the development of other methods in order to isolate and study the relevant T cells.
Animal Models Identify CD8+ T Cells as Potent Effectors in the Disease
Prior to 2001, most models focused on the involvement of CD4+ T cells in the development of EAE. Two groups, developed, in parallel, EAE models based on specific CD8+ T cell adoptive transfer. First, in mice C57BL/6, Sun et al. observed that the adoptive transfer of pMOG35–55 CD8+ T cells led to the development of an EAE that was both longer and more severe than the disease actively induced by the injection of the MOG35–55 peptide (63). EAE did not develop in mice lacking β2 microglobulin, further supporting the involvement of CD8+ T cells in the development of the disease (63). These data were confirmed in 2005, and the authors further identified the pMOG37–46 peptide as the minimal peptide recognized by the pMOG35–55-specific CD8+ T cells that both led to IFNγ production by these cells and to the development of severe EAE (64). In another 2001 study, Huseby et al. showed that a rapid and severe CNS-autoimmune disease could be induced in C3H mice after adoptive transfer of CD8+ T cells lines reacting against the MBP79–87 peptide (65). The severity of the disease was reduced when coinjecting anti-IFNγ with the CD8 MBP-specific cell lines, supporting a pivotal role for this cytokine in the development of the disease. Interestingly, the clinical symptoms, as well as the CNS lesion types and distributions observed, were different from those induced after CD4+ T cell transfer, better reflecting the clinical features of the human disease (65). The authors then proposed that the different MS clinical manifestations could be linked to the activation of either CD4+ or CD8+ T cells. Since then, CD8+ specific models have mainly been developed by transfer of T cells bearing TCR recognizing transgenic epitopes expressed on oligodendrocytes (66). In 2008, Saxena et al. developed a double knock-in transgenic mouse in which the influenza hemagglutinin (HA) expression was restricted to oligodendrocytes (67). The injection of in vitro activated CD8+ T cells bearing a TCR specific for HA led to CNS inflammation and demyelination. Of note, the activated CD8+ T cells produced granzyme B and IFNγ and exhibited cytotoxicity against cells loaded with HA in vitro. The authors were able to track these cells in the lesions, in close proximity to oligodendrocytes and in association with microglia activation. In another study, Na et al. showed that double transgenic mice with ovalbumin (OVA) expression in oligodendrocytes and OVA-specific TCR CD8+ T cells (OT-I) – but not OVA-specific TCR CD4+ T cells (OT-II) – develop a spontaneous EAE with demylinated and infiltrated lesions (68). The disease developed during the first 10–12 days of life, when CD8+ T cells still have access to the CNS, and was amplified by IFNγ. Of note, blocking the recognition of the OT-I CD8+ T cells with an antibody specific for the OVA-peptide/MHC-I complex prevents disease development (69). Interestingly, most of these models develop either clinical manifestations and/or infiltration characteristics that have a greater resemblance to MS symptoms and infiltrates than CD4-mediated EAE models (65, 67, 68).
Another model has been used to study the mechanisms by which CD8+ T cell infiltrate and damage the CNS. In Theiler’s murine encephalomyelitis virus (TMEV)-infected mice, the viral model of MS, CD8+ T cells secreting perforin were shown to be involved in BBB disruption and astrocyte activation (70). CD8+ T cells have also been shown to be able to enter the CNS in a naïve CL4 mice model without peripheral activation (CD8+ T cells specific for HA). Even if they remained inactivated in situ, they were able to proliferate when HA was injected intracerebrally. Interestingly, blocking MHC-I led to the reduction by 76% of the trafficking (71). Recently, Sobottka et al. further described mechanisms of presentation into the CNS. Using living brain slices preincubated with IFNγ (to mimic CNS inflammation) and OVA, they showed that OT-I CD8+ T cells (OVA-specific) were able to mediate axonal damage. Moreover, they obtained the same results using transgenic oligodendrocytes-expressing OVA in their living brain slices, making them potential target cells for CD8+ T cell pathogenicity (72).
Animal models depending on CD8+ T cells are, thus, relatively recent, and their study may shed new light on the mechanisms involving these cells in disease development (Table 2).
IL-17-Producing CD8+ T Cells as Potent Effectors in MS
Similarly to CD4+ T cells, the implication of IL-17-producing CD8+ T cells in MS has been recently suggested. The first study suggesting this was performed on 18 frozen CNS samples from 14 MS patients (26). Seventy to 80% of the infiltrating T cells, both CD4+ and CD8+ T cells, expressed IL-17 in active and chronic active lesions, shown through double immunofluorescent staining. This percentage was dramatically lower, at 20%, in chronic inactive lesions and NAWM, suggesting significant involvement of these IL-17-producing CD4 and CD8+ T cells in MS pathogenesis. After in vitro stimulation of blood samples, another study observed more IL-17 producing CD8+ T cells in the 20 MS patients than in the 16 controls (74). CD8+ T cells secreting IL-17 after in vitro stimulation were present in greater frequency in the CSF than in the blood of 17 MS patients in the early stages of the disease (75). Finally, in EAE, IL-17-producing CD8+ T cells were found to be necessary for IL-17-producing CD4+ T cell accumulation in the CNS and for disease development (75).
In human, the in vitro production of IL-17 is restricted to CD161-expressing cells (76). These cells have been shown to be present in CNS lesions of MS patients and the majority of them produce IFNγ (IL-17 staining was not performed on these samples) (32). In addition, an enrichment of CD8+CD161hi in the blood of MS patients has also been evidenced, suggesting a specific involvement in the disease (32).
Recently, it has been shown that more than 80% of these cells are mucosal-associated invariant T (MAIT) cells. MAIT cells are a subset of innate effector memory T cells bearing a semi-invariant TCR (Vα7.2-Jα33/12/20) in humans (77–82). They are restricted to the MHC class-I related protein I (MR1) and have antimicrobial properties both in vitro and in vivo (83–86). Although they have been correlated with various autoimmune diseases (87–90), their implication, especially in MS (32, 91, 92), remain elusive. MAIT cells are present in the CNS of MS patients, but at very low frequencies compared to in the blood (92–94). This argues against a particular implication of this IL-17-producing CD8+ T cell subset in the pathophysiology of MS (92, 95), similar to what has been described for psoriasis, where conventional IL-17-producing CD8+ T cells might be more pathogenic than MAIT cells (87).
Other IL-17-producing CD8+ T cell subsets have been described (96), with different markers, such as MCAM (97), but further research is necessary to decipher their involvement in MS pathogenesis.
Multiple Sclerosis: A Close Collaboration Between CD4+ and CD8+ T Cells
Even though we are convinced that CD8+ T cells play a pivotal role in MS pathophysiology, it seems obvious that they interact with other subsets – especially CD4+ T cells – in mediating MS development. Indeed, CD8+ T cells may migrate first into the CNS, further allowing the infiltration of CD4+ T cells. This proposition is supported by the fact that a small infiltrate of CD4+ T cells was found in the CNS of a CD8-specific mouse model of EAE (67). Moreover, that fact that both CD8+ and CD4+ T cells produce IL-17 at the same frequency in brain lesions gives evidence for the involvement of both cell types. However, whether the damage caused by these cells is direct or bystander is still unclear. Indeed, the initiating event, which is still unknown, leads to an in situ inflammatory context. Amplifying inflammatory loops are likely to develop, leading to BBB disruption and to the infiltration of other immune cell subsets. The inflammatory climate and the subsequent destruction of CNS cell types result in the release of self-antigens. These antigens, usually sequestered in a compartment that is poorly accessible for immune cells, are then available for recognition and further activation of the immune compartment in situ. This phenomenon is well described in the EAE model. Ji et al. showed that in their EAE model, induced by the transfer of MOG-specific CD4+ T cells, particular dentritic cells (DC) derived from inflammatory monocytes (Tip-DC) lead to epitope spreading to MBP-specific CD8+ T cells in situ (98).
To conclude, it appears clear to us that CD8+ T cells are involved in the pathophysiology of MS, in particular as potent effectors for CNS damage. However, other cell subsets, including CD4+ T cells, are likely to act in synergy to trigger the disease, probably by giving aid to pathogenic CD8+ T cells. More studies are needed to decipher the exact steps involving CD8+ T cells in the disease. Indeed, numerous questions remain unanswered: how and why CD8+ T cells get activated/reactivated in MS patients; how they cross the BBB; what the target antigen(s) is (are); how they mediate damage in situ. Focusing on these different questions and mechanisms is essential in order to develop effective therapeutic approaches (Figure 2).
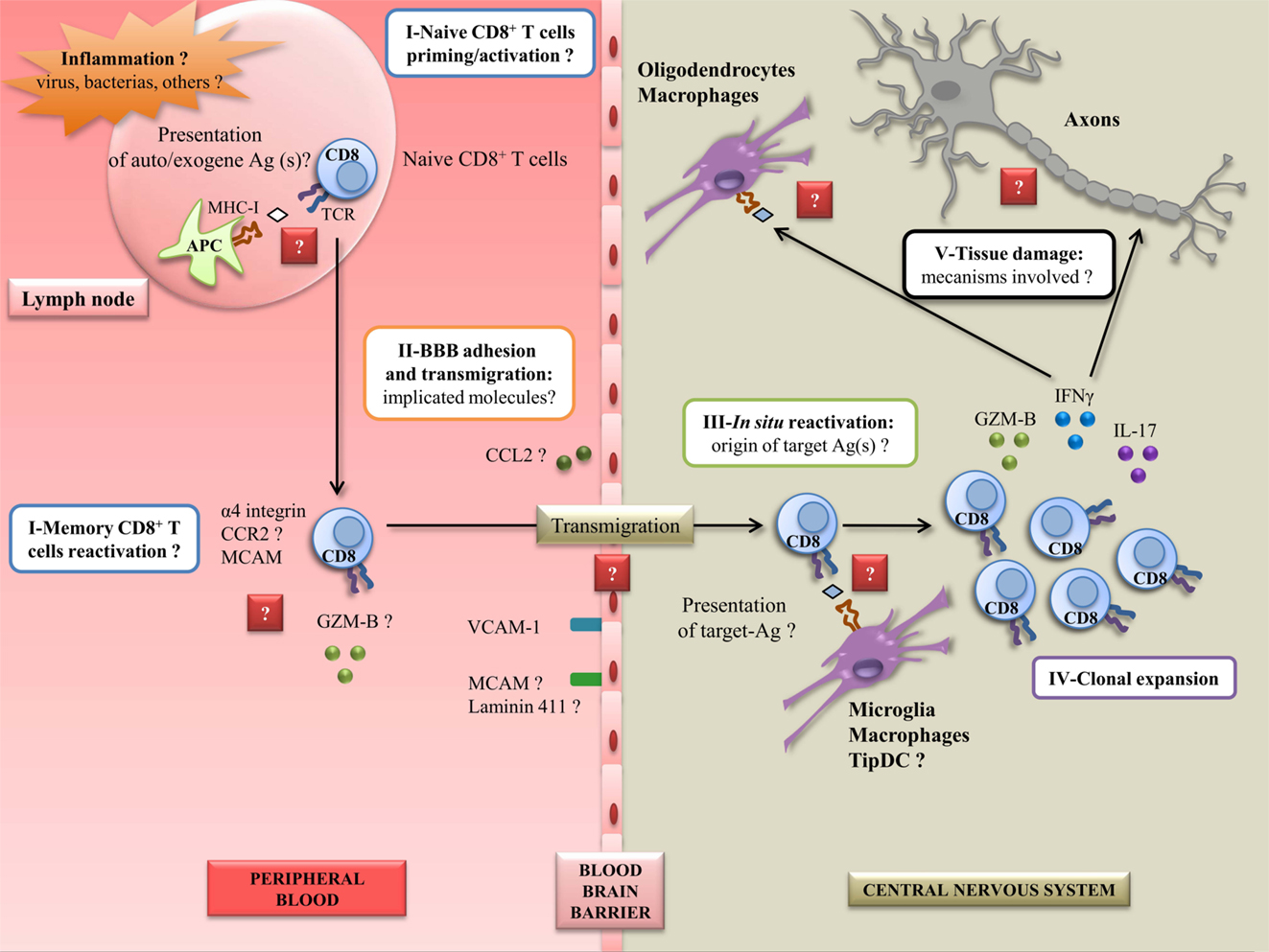
Figure 2. Steps to elucidate to better understand CD8+ autoreactivity mechanisms in MS disease. A peripheral inflammation induced by pathogens (such as EBV) could occur in case of uncontrolled infection. This can lead to the activation or reactivation of CD8+ T cells, and the expression of several molecules implicated in adhesion, migration, and cytotoxicity, currently not well characterized. In this inflamed state, the BBB could overexpress adhesion and chimoattractant molecules, leading to the entry of CD8+ T cells into the CNS. In situ, CD8+ T cells could be reactivated by resident APC presenting target Ag(s), unknown for now. This could lead to the clonal expansion of CD8+ T cells along with the secretion of proinflammatory molecules. Finally, in this step, CD8+ T cells could be able to mediate damage to resident cells and axons potentially by the recognition of CNS derived peptides. Ag: antigen; GZM-B: granzyme-B; BBB: blood–brain barrier; CNS: central nervous system; MS: multiple sclerosis; EBV: Epstein–Barr virus; APC: antigen-presenting cell; TCR: T-cell receptor; MCAM: melanoma cell adhesion molecule; VCAM-1: vascular cell adhesion molecule 1; DC: dendritic cell; MHC-I: major histocompatibility complex I; CCR2: C–C chemokine receptor type 2; CCL2: C–C chemokine ligand 2; IFNγ: interferon γ; IL-17: interleukin-17.
Conflict of Interest Statement
The authors declare that the research was conducted in the absence of any commercial or financial relationships that could be construed as a potential conflict of interest.
Abbreviations
BBB, blood–brain barrier; CNS, central nervous system; CSF, cerebrospinal fluid; EAE, experimental autoimmune encephalomyelitis; EBV, Epstein–Barr virus; HLA, human leukocyte antigen; HV, healthy volunteers; MAIT, mucosal-associated invariant T; MBP, myelin basic protein; MHC, major histocompatibility complex; MRI, magnetic resonance imaging; MS, multiple sclerosis; NAWM, normal-appearing white matter; TCR, T-cell receptor.
References
1. Compston A, Coles A. Multiple sclerosis. Lancet (2008) 372:1502–17. doi:10.1016/S0140-6736(08)61620-7
2. Pierrot-Deseilligny C, Souberbielle J-C. Is hypovitaminosis D one of the environmental risk factors for multiple sclerosis? Brain (2010) 133:1869–88. doi:10.1093/brain/awq147
3. Sospedra M, Martin R. Immunology of multiple sclerosis. Annu Rev Immunol (2005) 23:683–747. doi:10.1146/annurev.immunol.23.021704.115707
4. Noseworthy JH, Lucchinetti C, Rodriguez M, Weinshenker BG. Multiple sclerosis. N Engl J Med (2000) 343:938–52. doi:10.1056/NEJM200009283431307
5. Van Oosten BW, Lai M, Hodgkinson S, Barkhof F, Miller DH, Moseley IF, et al. Treatment of multiple sclerosis with the monoclonal anti-CD4 antibody cM-T412: results of a randomized, double-blind, placebo-controlled, MR-monitored phase II trial. Neurology (1997) 49:351–7. doi:10.1212/WNL.49.2.351
6. Sinha S, Itani FR, Karandikar NJ. Immune regulation of multiple sclerosis by CD8+ T cells. Immunol Res (2014) 59:254–65. doi:10.1007/s12026-014-8529-9
7. Denic A, Wootla B, Rodriguez M. CD8(+) T cells in multiple sclerosis. Expert Opin Ther Targets (2013) 17:1053–66. doi:10.1517/14728222.2013.815726
8. Brown MA, Rubio JP, Bahlo M, Stankovich J, Danoy P, Hickey PF, et al. Saliva-derived DNA performs well in large-scale, high-density single-nucleotide polymorphism microarray studies. Cancer Epidemiol Biomarkers Prev (2010) 19:794–8. doi:10.1158/1055-9965.EPI-09-0812
9. Baranzini SE, Wang J, Gibson RA, Galwey N, Naegelin Y, Barkhof F, et al. Genome-wide association analysis of susceptibility and clinical phenotype in multiple sclerosis. Hum Mol Genet (2009) 18:767–78. doi:10.1093/hmg/ddn388
10. Sawcer S, Hellenthal G, Pirinen M, Spencer CCA, Patsopoulos NA, Moutsianas L, et al. Genetic risk and a primary role for cell-mediated immune mechanisms in multiple sclerosis. Nature (2011) 476:214–9. doi:10.1038/nature10251
11. Hafler DA, Compston A, Sawcer S, Lander ES, Daly MJ, De Jager PL, et al. Risk alleles for multiple sclerosis identified by a genomewide study. N Engl J Med (2007) 357:851–62. doi:10.1056/NEJMoa073493
12. Esposito F, Reischl J, Lehr S, Bauer D, Heubach J, Sandbrink R, et al. Genome-wide meta-analysis identifies novel multiple sclerosis susceptibility loci. Ann Neurol (2011) 70:897–912. doi:10.1002/ana.22609
13. Sawcer S, Franklin RJM, Ban M. Multiple sclerosis genetics. Lancet Neurol (2014) 13:700–9. doi:10.1016/S1474-4422(14)70041-9
14. Jersild C, Svejgaard A, Fog T. HL-A antigens and multiple sclerosis. Lancet (1972) 1:1240–1. doi:10.1016/S0140-6736(72)90962-2
15. Naito S, Namerow N, Mickey MR, Terasaki PI. Multiple sclerosis: association with HL-A3. Tissue Antigens (1972) 2:1–4. doi:10.1111/j.1399-0039.1972.tb00111.x
16. Fogdell-Hahn A, Ligers A, Grønning M, Hillert J, Olerup O. Multiple sclerosis: a modifying influence of HLA class I genes in an HLA class II associated autoimmune disease. Tissue Antigens (2000) 55:140–8. doi:10.1034/j.1399-0039.2000.550205.x
17. Harbo HF, Lie BA, Sawcer S, Celius EG, Dai K-Z, Oturai A, et al. Genes in the HLA class I region may contribute to the HLA class II-associated genetic susceptibility to multiple sclerosis. Tissue Antigens (2004) 63:237–47. doi:10.1111/j.0001-2815.2004.00173.x
18. Friese MA, Jakobsen KB, Friis L, Etzensperger R, Craner MJ, McMahon RM, et al. Opposing effects of HLA class I molecules in tuning autoreactive CD8+ T cells in multiple sclerosis. Nat Med (2008) 14:1227–35. doi:10.1038/nm.1881
19. Booss J, Esiri MM, Tourtellotte WW, Mason DY. Immunohistological analysis of T lymphocyte subsets in the central nervous system in chronic progressive multiple sclerosis. J Neurol Sci (1983) 62:219–32. doi:10.1016/0022-510X(83)90201-0
20. Hauser SL, Bhan AK, Gilles F, Kemp M, Kerr C, Weiner HL. Immunohistochemical analysis of the cellular infiltrate in multiple sclerosis lesions. Ann Neurol (1986) 19:578–87. doi:10.1002/ana.410190610
21. Salou M, Garcia A, Michel L, Gainche-Salmon A, Loussouarn D, Nicol B, et al. Expanded CD8 T-cell sharing between periphery and CNS in multiple sclerosis. Ann Clin Transl Neurol (2015) 2:609–22. doi:10.1002/acn3.199
22. Babbe H, Roers A, Waisman A, Lassmann H, Goebels N, Hohlfeld R, et al. Clonal expansions of CD8(+) T cells dominate the T cell infiltrate in active multiple sclerosis lesions as shown by micromanipulation and single cell polymerase chain reaction. J Exp Med (2000) 192:393–404. doi:10.1084/jem.192.3.393
23. Junker A, Ivanidze J, Malotka J, Eiglmeier I, Lassmann H, Wekerle H, et al. Multiple sclerosis: T-cell receptor expression in distinct brain regions. Brain (2007) 130:2789–99. doi:10.1093/brain/awm214
24. Skulina C, Schmidt S, Dornmair K, Babbe H, Roers A, Rajewsky K, et al. Multiple sclerosis: brain-infiltrating CD8+ T cells persist as clonal expansions in the cerebrospinal fluid and blood. Proc Natl Acad Sci U S A (2004) 101:2428–33. doi:10.1073/pnas.0308689100
25. Ifergan I, Kebir H, Alvarez JI, Marceau G, Bernard M, Bourbonnière L, et al. Central nervous system recruitment of effector memory CD8+ T lymphocytes during neuroinflammation is dependent on α4 integrin. Brain (2011) 134:3557–74. doi:10.1093/brain/awr268
26. Tzartos JS, Friese MA, Craner MJ, Palace J, Newcombe J, Esiri MM, et al. Interleukin-17 production in central nervous system-infiltrating T cells and glial cells is associated with active disease in multiple sclerosis. Am J Pathol (2008) 172:146–55. doi:10.2353/ajpath.2008.070690
27. Lucchinetti CF, Popescu BFG, Bunyan RF, Moll NM, Roemer SF, Lassmann H, et al. Inflammatory cortical demyelination in early multiple sclerosis. N Engl J Med (2011) 365:2188–97. doi:10.1056/NEJMoa1100648
28. Höftberger R, Aboul-Enein F, Brueck W, Lucchinetti C, Rodriguez M, Schmidbauer M, et al. Expression of major histocompatibility complex class I molecules on the different cell types in multiple sclerosis lesions. Brain Pathol (2004) 14:43–50. doi:10.1111/j.1750-3639.2004.tb00496.x
29. Medana I, Martinic MA, Wekerle H, Neumann H. Transection of major histocompatibility complex class I-induced neurites by cytotoxic T lymphocytes. Am J Pathol (2001) 159:809–15. doi:10.1016/S0002-9440(10)61755-5
30. Trapp BD, Peterson J, Ransohoff RM, Rudick R, Mörk S, Bö L. Axonal transection in the lesions of multiple sclerosis. N Engl J Med (1998) 338:278–85. doi:10.1056/NEJM199801293380502
31. Bitsch A, Schuchardt J, Bunkowski S, Kuhlmann T, Brück W. Acute axonal injury in multiple sclerosis. Correlation with demyelination and inflammation. Brain (2000) 123(Pt 6):1174–83. doi:10.1093/brain/123.6.1174
32. Annibali V, Ristori G, Angelini DF, Serafini B, Mechelli R, Cannoni S, et al. CD161(high)CD8+T cells bear pathogenetic potential in multiple sclerosis. Brain (2011) 134:542–54. doi:10.1093/brain/awq354
33. Jilek S, Schluep M, Rossetti AO, Guignard L, Le Goff G, Pantaleo G, et al. CSF enrichment of highly differentiated CD8+ T cells in early multiple sclerosis. Clin Immunol (2007) 123:105–13. doi:10.1016/j.clim.2006.11.004
34. Malmeström C, Lycke J, Haghighi S, Andersen O, Carlsson L, Wadenvik H, et al. Relapses in multiple sclerosis are associated with increased CD8+ T-cell mediated cytotoxicity in CSF. J Neuroimmunol (2008) 196:159–65. doi:10.1016/j.jneuroim.2008.03.001
35. Larochelle C, Lécuyer M-A, Alvarez JI, Charabati M, Saint-Laurent O, Ghannam S, et al. Melanoma cell adhesion molecule–positive CD8 T lymphocytes mediate central nervous system inflammation. Ann Neurol (2015) 78:39–53. doi:10.1002/ana.24415
36. Larochelle C, Cayrol R, Kebir H, Alvarez JI, Lécuyer M-A, Ifergan I, et al. Melanoma cell adhesion molecule identifies encephalitogenic T lymphocytes and promotes their recruitment to the central nervous system. Brain (2012) 135:2906–24. doi:10.1093/brain/aws212
37. Flanagan K, Fitzgerald K, Baker J, Regnstrom K, Gardai S, Bard F, et al. Laminin-411 is a vascular ligand for MCAM and facilitates TH17 cell entry into the CNS. PLoS One (2012) 7:e40443. doi:10.1371/journal.pone.0040443
38. Duan H, Xing S, Luo Y, Feng L, Gramaglia I, Zhang Y, et al. Targeting endothelial CD146 attenuates neuroinflammation by limiting lymphocyte extravasation to the CNS. Sci Rep (2013) 3:1687. doi:10.1038/srep01687
39. Juliano RL, Ling V. A surface glycoprotein modulating drug permeability in Chinese hamster ovary cell mutants. Biochim Biophys Acta (1976) 455:152–62. doi:10.1016/0005-2736(76)90160-7
40. Kooij G, Backer R, Koning JJ, Reijerkerk A, van Horssen J, van der Pol SMA, et al. P-glycoprotein acts as an immunomodulator during neuroinflammation. PLoS One (2009) 4:e8212. doi:10.1371/journal.pone.0008212
41. Kooij G, Kroon J, Paul D, Reijerkerk A, Geerts D, van der Pol SMA, et al. P-glycoprotein regulates trafficking of CD8(+) T cells to the brain parenchyma. Acta Neuropathol (2014) 127:699–711. doi:10.1007/s00401-014-1244-8
42. Elong Ngono A, Pettré S, Salou M, Bahbouhi B, Soulillou J-P, Brouard S, et al. Frequency of circulating autoreactive T cells committed to myelin determinants in relapsing-remitting multiple sclerosis patients. Clin Immunol (2012) 144:117–26. doi:10.1016/j.clim.2012.05.009
43. Sewell AK. Why must T cells be cross-reactive? Nat Rev Immunol (2012) 12:669–77. doi:10.1038/nri3279
44. Zhang X, Tang Y, Sujkowska D, Wang J, Ramgolam V, Sospedra M, et al. Degenerate TCR recognition and dual DR2 restriction of autoreactive T cells: implications for the initiation of the autoimmune response in multiple sclerosis. Eur J Immunol (2008) 38:1297–309. doi:10.1002/eji.200737519
45. Ji Q, Perchellet A, Goverman JM. Viral infection triggers central nervous system autoimmunity via activation of CD8+ T cells expressing dual TCRs. Nat Immunol (2010) 11:628–34. doi:10.1038/ni.1888
46. Kim S-M, Bhonsle L, Besgen P, Nickel J, Backes A, Held K, et al. Analysis of the paired TCR α- and β-chains of single human T cells. PLoS One (2012) 7:e37338. doi:10.1371/journal.pone.0037338
47. Goebels N, Hofstetter H, Schmidt S, Brunner C, Wekerle H, Hohlfeld R. Repertoire dynamics of autoreactive T cells in multiple sclerosis patients and healthy subjects: epitope spreading versus clonal persistence. Brain (2000) 123(Pt 3):508–18. doi:10.1093/brain/123.3.508
48. Croxford JL, Olson JK, Miller SD. Epitope spreading and molecular mimicry as triggers of autoimmunity in the Theiler’s virus-induced demyelinating disease model of multiple sclerosis. Autoimmun Rev (2002) 1:251–60. doi:10.1016/S1568-9972(02)00080-0
49. Gran B, Gestri D, Sottini A, Quiròs Roldàn E, Bettinardi A, Signorini S, et al. Detection of skewed T-cell receptor V-beta gene usage in the peripheral blood of patients with multiple sclerosis. J Neuroimmunol (1998) 85:22–32. doi:10.1016/S0165-5728(97)00250-6
50. Laplaud D-A, Ruiz C, Wiertlewski S, Brouard S, Berthelot L, Guillet M, et al. Blood T-cell receptor beta chain transcriptome in multiple sclerosis. Characterization of the T cells with altered CDR3 length distribution. Brain (2004) 127:981–95. doi:10.1093/brain/awh119
51. Matsumoto Y, Yoon WK, Jee Y, Fujihara K, Misu T, Sato S, et al. Complementarity-determining region 3 spectratyping analysis of the TCR repertoire in multiple sclerosis. J Immunol (2003) 170:4846–53. doi:10.4049/jimmunol.170.9.4846
52. Monteiro J, Hingorani R, Peroglizzi R, Apatoff B, Gregersen PK. Oligoclonality of CD8+ T cells in multiple sclerosis. Autoimmunity (1996) 23:127–38. doi:10.3109/08916939608995336
53. Muraro PA, Bonanni L, Mazzanti B, Pantalone A, Traggiai E, Massacesi L, et al. Short-term dynamics of circulating T cell receptor V beta repertoire in relapsing-remitting MS. J Neuroimmunol (2002) 127:149–59. doi:10.1016/S0165-5728(02)00105-4
54. Démoulins T, Mouthon F, Clayette P, Bequet D, Gachelin G, Dormont D. The same TCR (N)Dbeta(N)Jbeta junctional region is associated with several different vbeta13 subtypes in a multiple sclerosis patient at the onset of the disease. Neurobiol Dis (2003) 14:470–82. doi:10.1016/j.nbd.2003.07.001
55. Jacobsen M, Cepok S, Quak E, Happel M, Gaber R, Ziegler A, et al. Oligoclonal expansion of memory CD8+ T cells in cerebrospinal fluid from multiple sclerosis patients. Brain (2002) 125:538–50. doi:10.1093/brain/awf059
56. Lozeron P, Chabas D, Duprey B, Lyon-Caen O, Liblau R. T cell receptor V beta 5 and V beta 17 clonal diversity in cerebrospinal fluid and peripheral blood lymphocytes of multiple sclerosis patients. Mult Scler (1998) 4:154–61. doi:10.1177/135245859800400313
57. Laplaud D-A, Berthelot L, Miqueu P, Bourcier K, Moynard J, Oudinet Y, et al. Serial blood T cell repertoire alterations in multiple sclerosis patients; correlation with clinical and MRI parameters. J Neuroimmunol (2006) 177:151–60. doi:10.1016/j.jneuroim.2006.05.006
58. Muraro PA, Cassiani-Ingoni R, Chung K, Packer AN, Sospedra M, Martin R. Clonotypic analysis of cerebrospinal fluid T cells during disease exacerbation and remission in a patient with multiple sclerosis. J Neuroimmunol (2006) 171:177–83. doi:10.1016/j.jneuroim.2005.10.002
59. Crawford MP, Yan SX, Ortega SB, Mehta RS, Hewitt RE, Price DA, et al. High prevalence of autoreactive, neuroantigen-specific CD8+ T cells in multiple sclerosis revealed by novel flow cytometric assay. Blood (2004) 103:4222–31. doi:10.1182/blood-2003-11-4025
60. Lolli F, Martini H, Citro A, Franceschini D, Portaccio E, Amato MP, et al. Increased CD8+ T cell responses to apoptotic T cell-associated antigens in multiple sclerosis. J Neuroinflammation (2013) 10:94. doi:10.1186/1742-2094-10-94
61. Zang YCQ, Li S, Rivera VM, Hong J, Robinson RR, Breitbach WT, et al. Increased CD8+ cytotoxic T cell responses to myelin basic protein in multiple sclerosis. J Immunol (2004) 172:5120–7. doi:10.4049/jimmunol.172.8.5120
62. Lossius A, Johansen JN, Vartdal F, Robins H, Jūratė Šaltytė B, Holmøy T, et al. High-throughput sequencing of TCR repertoires in multiple sclerosis reveals intrathecal enrichment of EBV-reactive CD8+ T cells. Eur J Immunol (2014) 44:3439–52. doi:10.1002/eji.201444662
63. Sun D, Whitaker JN, Huang Z, Liu D, Coleclough C, Wekerle H, et al. Myelin antigen-specific CD8+ T cells are encephalitogenic and produce severe disease in C57BL/6 mice. J Immunol (2001) 166:7579–87. doi:10.4049/jimmunol.166.12.7579
64. Ford ML, Evavold BD. Specificity, magnitude, and kinetics of MOG-specific CD8+ T cell responses during experimental autoimmune encephalomyelitis. Eur J Immunol (2005) 35:76–85. doi:10.1002/eji.200425660
65. Huseby ES, Liggitt D, Brabb T, Schnabel B, Ohlén C, Goverman J. A pathogenic role for myelin-specific CD8(+) T cells in a model for multiple sclerosis. J Exp Med (2001) 194:669–76. doi:10.1084/jem.194.5.669
66. Lassmann H. Experimental models of multiple sclerosis. Rev Neurol (2007) 163:651–5. doi:10.1016/S0035-3787(07)90474-9
67. Saxena A, Bauer J, Scheikl T, Zappulla J, Audebert M, Desbois S, et al. Cutting edge: multiple sclerosis-like lesions induced by effector CD8 T cells recognizing a sequestered antigen on oligodendrocytes. J Immunol (2008) 181:1617–21. doi:10.4049/jimmunol.181.3.1617
68. Na S-Y, Cao Y, Toben C, Nitschke L, Stadelmann C, Gold R, et al. Naive CD8 T-cells initiate spontaneous autoimmunity to a sequestered model antigen of the central nervous system. Brain (2008) 131:2353–65. doi:10.1093/brain/awn148
69. Na S-Y, Eujen H, Göbel K, Meuth SG, Martens K, Wiendl H, et al. Antigen-specific blockade of lethal CD8 T-cell mediated autoimmunity in a mouse model of multiple sclerosis. J Immunol (2009) 182:6569–75. doi:10.4049/jimmunol.0804200
70. Johnson HL, Willenbring RC, Jin F, Manhart WA, LaFrance SJ, Pirko I, et al. Perforin competent CD8 T cells are sufficient to cause immune-mediated blood-brain barrier disruption. PLoS One (2014) 9:e111401. doi:10.1371/journal.pone.0111401
71. Galea I, Bernardes-Silva M, Forse PA, van Rooijen N, Liblau RS, Perry VH. An antigen-specific pathway for CD8 T cells across the blood-brain barrier. J Exp Med (2007) 204:2023–30. doi:10.1084/jem.20070064
72. Sobottka B, Harrer MD, Ziegler U, Fischer K, Wiendl H, Hünig T, et al. Collateral bystander damage by myelin-directed CD8+ T cells causes axonal loss. Am J Pathol (2009) 175:1160–6. doi:10.2353/ajpath.2009.090340
73. Mars LT, Bauer J, Gross DA, Bucciarelli F, Firat H, Hudrisier D, et al. CD8 T cell responses to myelin oligodendrocyte glycoprotein-derived peptides in humanized HLA-A*0201-transgenic mice. J Immunol (2007) 179:5090–8. doi:10.4049/jimmunol.179.8.5090
74. Wang HH, Dai YQ, Qiu W, Lu ZQ, Peng FH, Wang YG, et al. Interleukin-17-secreting T cells in neuromyelitis optica and multiple sclerosis during relapse. J Clin Neurosci (2011) 18:1313–7. doi:10.1016/j.jocn.2011.01.031
75. Huber M, Heink S, Pagenstecher A, Reinhard K, Ritter J, Visekruna A, et al. IL-17A secretion by CD8+ T cells supports Th17-mediated autoimmune encephalomyelitis. J Clin Invest (2013) 123:247–60. doi:10.1172/JCI63681
76. Billerbeck E, Kang Y-H, Walker L, Lockstone H, Grafmueller S, Fleming V, et al. Analysis of CD161 expression on human CD8+ T cells defines a distinct functional subset with tissue-homing properties. Proc Natl Acad Sci U S A (2010) 107:3006–11. doi:10.1073/pnas.0914839107
77. Martin E, Treiner E, Duban L, Guerri L, Laude H, Toly C, et al. Stepwise development of MAIT cells in mouse and human. PLoS Biol (2009) 7:e1000054. doi:10.1371/journal.pbio.1000054
78. Walker LJ, Kang Y-H, Smith MO, Tharmalingham H, Ramamurthy N, Fleming VM, et al. Human MAIT and CD8 cells develop from a pool of type-17 precommitted CD8+ T cells. Blood (2012) 119:422–33. doi:10.1182/blood-2011-05-353789
79. Dusseaux M, Martin E, Serriari N, Peguillet I, Premel V, Louis D, et al. Human MAIT cells are xenobiotic-resistant, tissue-targeted, CD161hi IL-17-secreting T cells. Blood (2010) 117:1250–9. doi:10.1182/blood-2010-08-303339
80. Patel O, Kjer-Nielsen L, Le Nours J, Eckle SBG, Birkinshaw R, Beddoe T, et al. Recognition of vitamin B metabolites by mucosal-associated invariant T cells. Nat Commun (2013) 4:2142. doi:10.1038/ncomms3142
81. Reantragoon R, Corbett AJ, Sakala IG, Gherardin NA, Furness JB, Chen Z, et al. Antigen-loaded MR1 tetramers define T cell receptor heterogeneity in mucosal-associated invariant T cells. J Exp Med (2013) 210:2305–20. doi:10.1084/jem.20130958
82. Treiner E, Duban L, Bahram S, Radosavljevic M, Wanner V, Tilloy F, et al. Selection of evolutionarily conserved mucosal-associated invariant T cells by MR1. Nature (2003) 422:164–9. doi:10.1038/nature01433
83. Chua W-J, Kim S, Myers N, Huang S, Yu L, Fremont DH, et al. Endogenous MHC-related protein 1 is transiently expressed on the plasma membrane in a conformation that activates mucosal-associated invariant T cells. J Immunol (2011) 186:4744–50. doi:10.4049/jimmunol.1003254
84. Le Bourhis L, Martin E, Péguillet I, Guihot A, Froux N, Coré M, et al. Antimicrobial activity of mucosal-associated invariant T cells. Nat Immunol (2010) 11:701–8. doi:10.1038/ni.1890
85. Gold MC, Cerri S, Smyk-Pearson S, Cansler ME, Vogt TM, Delepine J, et al. Human mucosal associated invariant T cells detect bacterially infected cells. PLoS Biol (2010) 8:e1000407. doi:10.1371/journal.pbio.1000407
86. Meierovics A, Yankelevich W-JC, Cowley SC. MAIT cells are critical for optimal mucosal immune responses during in vivo pulmonary bacterial infection. Proc Natl Acad Sci U S A (2013) 110:E3119–28. doi:10.1073/pnas.1302799110
87. Teunissen MBM, Yeremenko NG, Baeten DLP, Chielie S, Spuls PI, de Rie MA, et al. The IL-17A-producing CD8+ T-cell population in psoriatic lesional skin comprises mucosa-associated invariant T cells and conventional T cells. J Invest Dermatol (2014) 134:2898–907. doi:10.1038/jid.2014.261
88. Serriari N-E, Eoche M, Lamotte L, Lion J, Fumery M, Marcelo P, et al. Innate mucosal-associated invariant T (MAIT) cells are activated in inflammatory bowel diseases: MAIT cells in IBD. Clin Exp Immunol (2014) 176:266–74. doi:10.1111/cei.12277
89. Dunne MR, Elliott L, Hussey S, Mahmud N, Kelly J, Doherty DG, et al. Persistent changes in circulating and intestinal γδ T cell subsets, invariant natural killer T cells and mucosal-associated invariant T cells in children and adults with coeliac disease. PLoS One (2013) 8:e76008. doi:10.1371/journal.pone.0076008
90. Eidson M, Wahlstrom J, Beaulieu AM, Zaidi B, Carsons SE, Crow PK, et al. Altered development of NKT cells, γδ T cells, CD8 T cells and NK cells in a PLZF deficient patient. PLoS One (2011) 6:e24441. doi:10.1371/journal.pone.0024441
91. Miyazaki Y, Miyake S, Chiba A, Lantz O, Yamamura T. Mucosal-associated invariant T cells regulate Th1 response in multiple sclerosis. Int Immunol (2011) 23:529–35. doi:10.1093/intimm/dxr047
92. Abrahamsson SV, Angelini DF, Dubinsky AN, Morel E, Oh U, Jones JL, et al. Non-myeloablative autologous haematopoietic stem cell transplantation expands regulatory cells and depletes IL-17 producing mucosal-associated invariant T cells in multiple sclerosis. Brain (2013) 136:2888–903. doi:10.1093/brain/awt182
93. Illes Z. Accumulation of V 7.2-J 33 invariant T cells in human autoimmune inflammatory lesions in the nervous system. Int Immunol (2004) 16:223–30. doi:10.1093/intimm/dxh018
94. Held K, Bhonsle-Deeng L, Siewert K, Sato W, Beltrán E, Schmidt S, et al. αβ T-cell receptors from multiple sclerosis brain lesions show MAIT cell-related features. Neurol Neuroimmunol Neuroinflamm (2015) 2:e107. doi:10.1212/NXI.0000000000000107
95. Willing A, Leach OA, Ufer F, Attfield KE, Steinbach K, Kursawe N, et al. CD8+ MAIT cells infiltrate into the CNS and alterations in their blood frequencies correlate with IL-18 serum levels in multiple sclerosis. Eur J Immunol (2014) 44:3119–28. doi:10.1002/eji.201344160
96. Fergusson JR, Fleming VM, Klenerman P. CD161-expressing human T cells. Front Immunol (2011) 2:36. doi:10.3389/fimmu.2011.00036
97. Dagur PK, Biancotto A, Stansky E, Sen HN, Nussenblatt RB, McCoy JP. Secretion of interleukin-17 by CD8+ T cells expressing CD146 (MCAM). Clin Immunol (2014) 152:36–47. doi:10.1016/j.clim.2014.01.009
Keywords: multiple sclerosis, autoimmunity, CD8+ T cells
Citation: Salou M, Nicol B, Garcia A and Laplaud D-A (2015) Involvement of CD8+ T Cells in Multiple Sclerosis. Front. Immunol. 6:604. doi: 10.3389/fimmu.2015.00604
Received: 08 July 2015; Accepted: 12 November 2015;
Published: 26 November 2015
Edited by:
Jorge Ivan Alvarez, University of Pennsylvania, USAReviewed by:
Catherine Larochelle, Mainz Universität Medizin, GermanyJohanna Olweus, University of Oslo, Norway
Copyright: © 2015 Salou, Nicol, Garcia and Laplaud. This is an open-access article distributed under the terms of the Creative Commons Attribution License (CC BY). The use, distribution or reproduction in other forums is permitted, provided the original author(s) or licensor are credited and that the original publication in this journal is cited, in accordance with accepted academic practice. No use, distribution or reproduction is permitted which does not comply with these terms.
*Correspondence: David-Axel Laplaud, david.laplaud@univ-nantes.fr
†Marion Salou and Bryan Nicol have contributed equally to this work.