- 1Stem Cell Laboratory, Medical Faculty Mannheim, Institute of Transfusion Medicine and Immunology, Heidelberg University, Heidelberg, Germany
- 2German Red Cross Blood Service Baden-Württemberg – Hessen, Mannheim, Germany
Mesenchymal stromal cells (MSC) have gained immense attraction in regenerative medicine, tissue engineering, and immunotherapy. This is based on their differentiation potential and the supply of pro-regenerative and immunomodulatory signals. MSC can be isolated from a multitude of tissue sources, but mainly bone marrow, adipose tissue, and birth-associated tissues (e.g., umbilical cord, cord blood, placenta) appear to be relevant for clinical translation in immune-mediated disorders. However, only a few studies directly compared the immunomodulatory potency of MSC from different tissue sources. This review compiles the current literature regarding the similarities and differences between these three sources for MSCs with a special focus on their immunomodulatory effects on T-lymphocyte subsets and monocytes, macrophages, and dendritic cells.
Introduction
Human mesenchymal stromal cells (MSC) have been an attractive target for translational research in a wide range of therapeutic applications due to their paracrine effects, multi-lineage differentiation potential and, most importantly, their immunomodulatory properties (1, 2). Bone marrow was the first established source of these cells (3). Since then it has been the primary and therefore most investigated population. Over the last few years, several other sources for MSC have been identified (4, 5). In our view, after bone marrow-derived MSCs (hereafter referred to as BM-MSC), adipose tissue-derived MSCs (hereafter referred to as AT-MSC), and birth-associated MSCs (from umbilical cord blood, cord tissue, and placenta, referred to as UCB-MSC, UC-MSC, and PL-MSC, respectively) (6) are most commonly used as sources of human MSCs in a clinical setting. These may be favored due to different advantages: greater yields of MSCs than BM, higher proliferative potential, and no ethical restrictions (5–10). It has to be noted that birth-associated tissue is not one single source of MSCs but rather a comprising name for several subpopulations, namely amnion, umbilical cord, cord blood, and placenta. Most of them can be categorized further into even more subpopulations.
Mesenchymal stromal cells from different sources are similar in a range of phenotypic and functional features (5). There are, however, subtle differences, which may result from the microenvironmental niche, the local function (stromal support of hematopoiesis in the BM and immune homeostasis by AT), and the ontogenetic age (birth-associated versus adult) (5, 7, 11) or induced by the isolation and culture procedure. CD106 is one example, as it is significantly reduced on AT-MSCs compared to other MSCs (5, 12, 13). CD34, on the other hand, appears on AT-MSCs in situ and early in culture but on no other MSCs (14, 15). Regarding function, we and others reported for example significantly reduced adipogenic differentiation capacity of UCB-MSC (16). Regarding the stromal supportive capacity, a recent study indicates that only BM-MSC (not MSC from white adipose tissue, umbilical cord, and skin) are capable to form a functional hematopoietic niche (17).
Immunomodulatory functions have been reported for all types of MSC tested. Strikingly, analyses directly comparing these populations with their immunomodulatory effects are limited. As many scientific groups just use one single source for MSCs in their experiments – indeed beneficial for the reproducibility of their own data – it renders it hard to compare the results to those of other scientists and to draw conclusions about their clinical efficacy. To assess immunomodulation, most groups utilize a mixed lymphocyte reaction (MLR) assay or an assay measuring T cell proliferation induced by mitogens or CD3/CD28 stimulation. Fewer groups address distinct effects on T cell subsets (Th1, Th2, Th17, and regulatory T cells) and antigen-presenting cells (APCs) [reviewed in Ref. (2, 18, 19)]. Although the vast majority of studies confirm MSCs to inhibit the immune response, recent data identified allogeneic MSCs to be immunogenic and immune-rejected under appropriate conditions (20–22). There is a large diversity in soluble factors to mediate the effects of MSCs, thus it remains to be clarified whether MSC origin and culture conditions use different molecular mechanisms to exert their effects (2, 23). Some interesting data suggest intrinsic differences in expression of immune-related signature genes, mi- and tRNA species (24, 25). However, a summary of these is beyond the scope of this review. Here, we focused on studies, which directly compared two or more MSC tissue sources addressing MSC effects on T cell subpopulations or APCs, such as monocytes, macrophages, or dendritic cells (DCs) (summarized in Table 1).
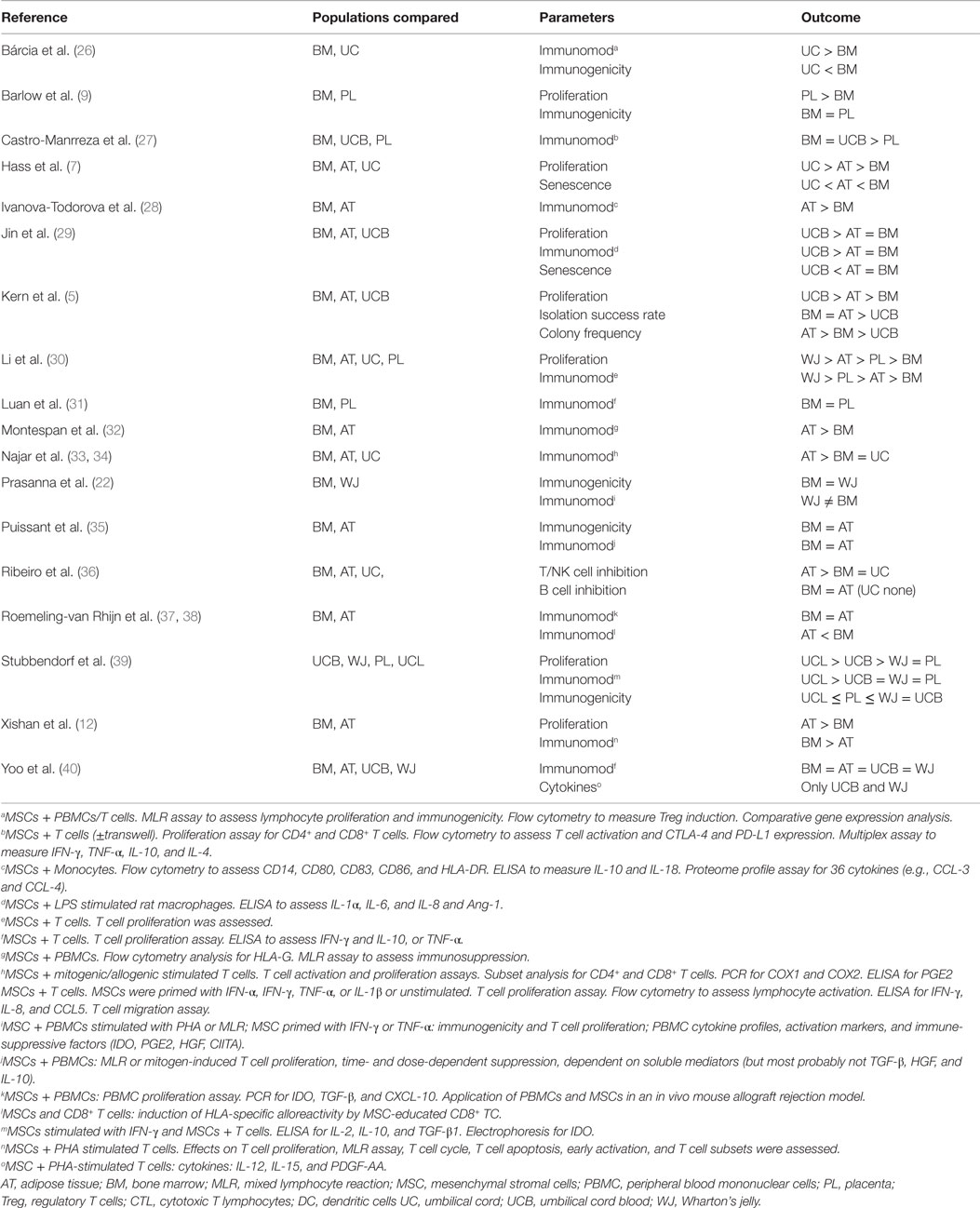
Table 1. Studies directly comparing different sources of MSCs, reporting differences in immunomodulatory capacities.
Effects on T Cells
Effects on Naïve CD4+ T Cells
The exerted effects on naïve CD4+ T cells are of a suppressing and polarizing nature, meaning MSCs inhibit the proliferation and activation of naïve CD4+ T helper cells (Th cells). They are able to influence the differentiation of Th0 cells into Th1, Th2, Th17, or regulatory T cells (Tregs) (36, 41, 42). MSCs seem to hamper T cell proliferation by arresting T cells in the G0/G1 phase of the cell cycle (12, 43), thus reducing the total number of T cells undergoing activation. MSCs exert their immunomodulatory functions through numerous molecules. Although trans-well experiments show an inhibiting function of MSCs, most studies confirm a more pronounced effect without trans-wells, highlighting the importance of cell-cell contact in mediating immunomodulatory functions. Prostaglandin E2 (PGE2) seems to play an important role in suppressing the immune response (33). Just recently, evidence arose that MSC-derived microvesicles contain a variety of immunomodulatory factors, including miRNA and tRNA species (25, 44). Di Nicola et al. proposed transforming growth factor (TGF)-β and hepatocyte growth factor (HGF) as important mediators, as blockage of both significantly reduced the suppressive effect of MSCs (45). Another group identified indoleamine 2,3-dioxygenase (IDO) to be involved (46). IDO catalyzes the conversion of tryptophan, an essential molecule in the activation of T cells, to kynurenine and has been identified as a key pathway for inhibiting T cell response. Additionally, Human Leukocyte Antigen-G5 (HLA-G5) was found to be required to suppress T cell function and to induce Tregs (32, 47).
Comparison
Comparative studies have produced conflicting results. Puissant et al. report similar inhibition of T cell proliferation, both induced in MLR or mitogens, in presence of BM- or AT-MSCs (35). In both settings suppression was induced by soluble mediators. In contrast, whereas Ribeiro et al. (36) found AT-MSCs (compared to BM-MSCs and UC-MSCs) to have the strongest suppressive effect on the activation and acquisition of lymphoblast characteristics on T cells, Xishan et al. (12) determined BM-MSCs to have a superior immunosuppressive effect over AT-MSCs. In a study comparing MSCs from bone marrow, adipose tissue and Wharton’s jelly, AT-MSCs showed the strongest effect on downregulating the activation marker CD38 on T cells, followed by UC-MSCs, whereas BM-MSCs had the weakest effect (33). The authors showed AT-MSCs to be the most potent population in inhibiting allogeneic-induced T cell proliferation (33). Interestingly, different levels of the enzyme cyclooxygenase-1 (COX1), which is essential in PGE2 production, were observed, with highest levels of COX1 in AT-MSC (33). Conflicting data, however, was presented by Li et al., who determined MSCs from Wharton’s jelly to possess the strongest inhibitory effect on T cell proliferation compared to AT-MSCs, BM-MSCs, and PL-MSCs (30). Similar inhibitory effects on T cell proliferation, activation, and cytokine secretion are reported by Luan et al. comparing PL- and BM-MSC (31). They identified programed death-ligand 1 (PD-L1, CD274, or B7-H1) and B7H4 as negative regulators.
Effects on CD4+ Th1 Cells
A large number of studies have been performed to explore the effects of MSCs on Th1 cells, considered to be the main effector cells of proinflammatory cell-mediated immunity and organ-specific autoimmune disorders (23, 48, 49). The results obtained from these studies usually imply an inhibiting effect on Th1 cells (12, 41, 48–50). However, there are conditions in which MSCs seem to promote Th1 cells and inhibit the differentiation of Th2 cells (49). Cho et al. described AT-MSCs to reduce Th2-associated cytokines (interleukin IL-4, IL-5) and increase Th1-derived interferon (IFN)-γ and IL-2 in a model of eosinophilic nasal polyps (51). These data are corroborated by other studies, confirming the Th2-inhibiting function of MSCs in Th2-dominated inflammatory conditions, such as allergic airway inflammation (52). In an inflammatory environment, high levels of IFN-γ and/or tumor necrosis factor (TNF)-α increase the expression of TGF-β by MSCs (53, 54), which in turn prompts Th1 cells to express immunosuppressive IL-10 and ultimately reduces their IFN-γ production. Furthermore, MSC mediate a downregulation of the Th1 cells IFN-γ receptor, which renders them less susceptible to IFN-γ (55). In a recent study, MSCs-induced and expanded a subpopulation of T-bet+ Th1 cells co-expressing IFN-γ and IL-10 (55). T-bet is a Th1 cell-specific transcription factor (56). This suggests that the influence of MSCs on IFN-γ expression is dependent on several factors, such as the cytokine milieu, the stimulation methods or the types of cells present, showing that we are far from having a full grasp of the effects of MSCs on immune cells.
Comparison
AT-MSCs and BM-MSCs showed similar results in inhibiting Th1 differentiation, as both significantly reduced the levels of IL-2 and IFN-γ (12). Another study compared several MSC populations from birth-associated tissue (umbilical cord lining, cord blood, placenta, and Wharton’s jelly), resulting in cord lining MSCs to emerge as the most potent in dampening Th1 and Th2 responses and reducing release of IFN-γ by lymphocytes (39). Castro-Manrreza et al. compared BM-MSCs, UC-MSCs, and PL-MSCs and identified similar proliferation suppression capacities for BM-MSCs and UC-MSCs, but PL-MSCs showed significantly weaker CD4+/CD8+ T lymphocyte suppression (27). AT-MSCs exerted the strongest inhibition on IFN-γ secretion and T cell proliferation compared to BM-MSCs and WJ-MSCs (34).
Effects on CD4+ Th2 Cells
Th2 cells have several functions in the humoral-mediated immune response, as they host the defense against extracellular parasites, inhibit Th1 cells and DCs via IL-10, stimulate B cells via IL-4 and can induce isotype-switches in B cells (57). MSCs have been shown to enhance anti-inflammatory IL-4 production by Th2 cells, supposedly via a PGE2 (48). In inflammatory diseases that are associated with high amounts of Th2 cells (e.g., allergies, asthma, Crohn’s disease), MSCs were able to ameliorate disease activity by inhibiting the cytokine production of Th2 cells (IL-4 and IL-5) and increase Th1-derived cytokines (IFN-γ and IL-2) (51, 58).
Comparison
There are a small number of studies concentrating on the comparison of various MSC sources on T cell subsets. Xishan et al. compared AT-MSCs and BM-MSCs on their ability to induce Th0 differentiation into Th1 and Th2 cells and could show that both populations had no significant effect on the levels of the Th2-associated cytokines IL-4 and IL-10 (12). Concerning Th2 cells, data is especially scarce.
Effects on CD4+ Th17 Cells
Th17 cells play an important role in the human immune system as effectors against extracellular bacterial and fungal infections, but have also been associated with autoimmune diseases, such as multiple sclerosis, psoriasis, rheumatoid arthritis, inflammatory bowel disease, systemic lupus erythematosus, and asthma (59). Although studies about the effects of MSCs on Th17 cells seem to yield rather consistent results, presenting MSCs as potent inhibitors of Th17-mediated immune responses (60–62), data exists where Th17 cells appear to be stimulated by MSCs in vitro (63). The time at which MSCs are added could be important, as Carrión et al. demonstrated opposing effects of MSCs on Th1 and Th17 cells relative to the state of CD4+ T cell activation (49).
Comparison
AT-MSCs, UC-MSCs, and BM-MSCs have all proven to be effective in suppressing the Th17 immune response (41, 60, 64), but studies directly comparing them are rare. In a mouse model of experimental colitis, UC-MSCs and BM-MSCs demonstrated a similar inhibition of Th17 cells, shifting the Th17/Treg ratio toward a more immunosuppressive balance (64).
Effects on CD4+ FoxP3+ Regulatory T Cells (Tregs)
Regulatory T cells are either derived in the thymus as mature Tregs, or from CD4+CD25− naïve T cells as peripherally derived Tregs under the influence of TGF-β and IL-2 (65). Tregs target effector T cells and DCs (65, 66) by inhibiting their differentiation, function, and maturation to prevent autoimmunity and establish a peripheral tolerance (67). MSCs have been shown to induce Tregs via a multitude of factors. HLA-G5, a non-classical HLA class I molecule, plays an important role in the induction of Tregs (68). Another factor of MSCs involved in the activation of Tregs is TGF-β, which seems to be constitutively expressed by MSCs (69). Additionally, MSCs were reported to elevate IL-10 production by Tregs and DCs (70, 71), whereby DC-derived IL-10 in turn promotes the expansion of Tregs (72). Tregs can also be indirectly activated by MSCs through an upregulation of Fas ligand (FasL)/Fas-mediated death pathway, which targets T cells via cell-cell contact and leads to increased apoptosis and Treg induction (73). In several in vivo settings, MSCs increased Tregs, thereby ameliorating disease states as well as promoting graft survival in transplant experiments (41, 50, 74–76).
Comparison
In an in vitro study that compared BM-MSCs and UC-MSCs on their ability to induce Tregs, UC-MSCs had a significantly greater potential to induce Tregs than BM-MSCs (26). Chao et al., on the other hand, did not report a difference in Treg induction of BM-MSCs and UC-MSCs in an in vivo experiment (77).
Effects on CD8+ T Cells (CTL)
Cytotoxic T lymphocytes (CTLs) are major effectors in the immune system through targeting virus-infected cells as well as tumor cells. CTLs have a crucial role in autoimmunity and transplant rejection. CTL activation is triggered following the interaction of the T cell receptor (TCR) with the specific allogeneic peptide–HLA-I complex. The activation of lymphocytes can be divided into several steps, which all have a corresponding phenotype: CD69−CD25−HLA-DR− (non-activated), CD69+CD25−HLA-DR− (earlier activated), CD69+CD25+HLA-DR− (intermediate activated) and CD69+CD25+HLA-DR+ (later activated). It was reported that MSCs are able to dampen the immune response of CTLs as well as inhibiting their proliferation and maturation (36, 37, 51). MSC downregulate the CD8 surface marker on CTLs via an indirect pathway involving CD14+ monocytes, requiring cell-cell contact between the monocytes and the CTLs (78). In this process, CD28 is downregulated on CTLs indicating loss of effector-type and gain of regulatory functions (78).
Comparison
Ribeiro et al. investigated AT-MSCs, BM-MSCs, and UC-MSCs as to their effect of inhibiting CD4+/CD8+ lymphocyte activation (36). Co-culture with BM-MSCs and UC-MSCs similarly inhibited lymphocyte activation, whereas the majority of the CD8+ cells were of the earlier activated phenotype. AT-MSCs here emerged as the most immunosuppressive population, as the majority of the T Cells were found to be in the non-activated compartment (36). Different effects on CD8+ mediated alloreactivity are reported by Roemeling-van Rhijn et al., addressing the capacity of AT- versus BM-MSC to induce HLA-specific alloreactivity (38). CD8+ T cells educated with IFN-γ-treated AT-MSC evoked 31% specific lysis of AT-MSCs with identical HLA. IFN-γ-treated BM-MSC, however, resulted in 76% HLA-specific killing of HLA-identical BM-MSC.
Effects on Monocytes, Macrophages, and Dendritic Cells
Monocytes are a subpopulation of leukocytes able to differentiate into macrophages and DCs. Macrophages and DCs are antigen-presenting cells that can initiate an immune response and act as a mediator between the innate and the adaptive immune system. MSCs were reported to strongly induce the secretion of IL-10 on CD14+ monocytes via HGF, thereby suppressing T cell proliferation (79). Melief et al. could show that MSCs promote the survival of monocytes and induce the differentiation into CD163+ CD206+ type 2 macrophages, which secrete IL-10 and CCL18 (69). CCL18 in turn has a crucial role in inducing Tregs (69). MSCs were frequently shown to be able to inhibit the proinflammatory functions of DCs and macrophages and skew the cells toward a more immunosuppressive response (70, 71, 80). The proinflammatory molecules TNF-α and macrophage inflammatory protein (MIP)-1β, produced by macrophages and mature DCs, were suppressed under the influence of MSCs (80). Concerning maturation markers such as CD1a, CD14, CD83 and HLA-DR, MSCs inhibited the maturation of DCs and furthermore downregulated the costimulatory molecules CD86/CD80 (81, 82). Conflicting data exist, as Laranjera et al. could not detect any influence of MSCs on maturation markers CD83, CCR7, and HLA-DR (80) on DCs, thus leading the group to suppose that MSCs exhibit their anti-inflammatory functions on macrophages and DCs mainly by inhibiting the secretion of proinflammatory cytokines. MSCs are also able to inhibit the differentiation at a more upstream step by interfering with monocyte maturation (83).
Comparison
AT-MSCs seem to have a more pronounced effect on DC differentiation than BM-MSCs (28). Saeidi et al. examined UC-MSCs on their potential to interfere with maturation and endocytotic capability of DCs comparing them with BM-MSCs (81). While being equally effective in hampering the maturation of DCs, UC-MSCs had a stronger effect on reducing the endocytotic ability of DCs (81). Jin et al. compared anti-inflammatory activity of BM-, AT-, and UCB-MSCs (29). UCB-MSCs were most potent in suppressing cytokine release from LPS-challenged alveolar macrophages. Angiopoietin-1 was at least partly responsible for this effect.
Conclusion
The increasing numbers of studies conducted on comparing MSC sources in vitro and in vivo yield largely congruent results, presenting MSCs as promising cells for a multitude of immunological applications (Table 1). Nevertheless, the heterogeneity in MSC populations and experimental protocols still poses a major obstacle when trying to compare and merge different results and to translate them into clinical practice (84). Our survey reflects that the vast majority of data showed no significant deficiencies in the immunomodulatory potential of MSCs from alternative sources but often even stronger immunosuppressive capabilities than BM-MSCs (26, 28, 30, 33, 34, 36, 81). This was especially the case for MSCs from adipose tissue (28, 32–34, 36). This claim, however, is based on the few studies directly comparing MSCs from different tissue sources. Future studies should elucidate whether the similarities of tissue MSCs in vitro relate to similar functions in situ or are artificially gained by ex vivo isolation and culture adaptation (85, 86) and whether the subtle differences in function relate to the role of MSCs in situ. What is certain is that MSCs expanded in vitro are highly sensitive to their microenvironment; they may alternate their function based on the surrounding conditions. Important parameters are the culture conditions (e.g., choice of serum supplement), types of immune cells present, cell activation status, ratio of MSC to immune cells and, of course, the cytokine levels in the milieu (87–90). Additionally, variations in isolation methods, culture media, cell counts, and different stimulation protocols can further blur the potential differences among distinct MSC sources. A standardization of assays to assess the effects of MSCs is essential to guarantee trustworthy and reproducible results (18). Ideally, these assays are capable of predicting efficacy of MSCs in vivo, to serve as potency assay. We would therefore appreciate more comparative studies to give us a better understanding of the immunomodulatory mechanisms of MSCs, facilitating the choice between different sources for defined clinical settings to improve safety and efficacy of MSC-based therapies.
Author Contributions
PM, KB: conception, acquisition, analysis, or interpretation of data, drafting and critical revision, final approval before submission, and agreement to be accountable for all aspects of the work in ensuring that questions related to the accuracy or integrity of any part of the work are appropriately investigated and resolved.
Conflict of Interest Statement
The authors declare that the work was conducted in the absence of any commercial or financial relationships that could be construed as a potential conflict of interest.
Acknowledgments
We would like to thank Stefanie Uhlig, Susanne Elvers-Hornung and Andrea Hecker for excellent technical and scientific support and Eva Ossenbühn for critical proofreading.
Funding
This work was supported by research funds of the German Federal Ministry of Education and Research (START-MSC: 01GN0531 and 01GN0939).
Abbreviations
AT, adipose tissue; BM, bone marrow; CTL, cytotoxic T lymphocytes; DC, dendritic cells; MLR, mixed lymphocyte reaction; MSC, mesenchymal stromal cells; PBMC, peripheral blood mononuclear cells; PL, placenta; Treg, regulatory T cells; UC, umbilical cord; UCB, umbilical cord blood; WJ, Wharton’s jelly.
References
1. Sharma RR, Pollock K, Hubel A, McKenna D. Mesenchymal stem or stromal cells: a review of clinical applications and manufacturing practices. Transfusion (2014) 54(5):1418–37. doi:10.1111/trf.12421
2. Wang Y, Chen X, Cao W, Shi Y. Plasticity of mesenchymal stem cells in immunomodulation: pathological and therapeutic implications. Nat Immunol (2014) 15(11):1009–16. doi:10.1038/ni.3002
3. Friedenstein AJ, Gorskaja JF, Kulagina NN. Fibroblast precursors in normal and irradiated mouse hematopoietic organs. Exp Hematol (1976) 4(5):267–74.
4. de Girolamo L, Lucarelli E, Alessandri G, Avanzini MA, Bernardo ME, Biagi E, et al. Mesenchymal stem/stromal cells: a new “cells as drugs” paradigm. Efficacy and critical aspects in cell therapy. Curr Pharm Des (2013) 19(13):2459–73. doi:10.2174/1381612811319130015
5. Kern S, Eichler H, Stoeve J, Kluter H, Bieback K. Comparative analysis of mesenchymal stem cells from bone marrow, umbilical cord blood, or adipose tissue. Stem Cells (2006) 24(5):1294–301. doi:10.1634/stemcells.2005-0342
6. Bieback K, Brinkmann I. Mesenchymal stromal cells from human perinatal tissues: from biology to cell therapy. World J Stem Cells (2010) 2(4):81–92. doi:10.4252/wjsc.v2.i4.81
7. Hass R, Kasper C, Bohm S, Jacobs R. Different populations and sources of human mesenchymal stem cells (MSC): a comparison of adult and neonatal tissue-derived MSC. Cell Commun Signal (2011) 9:12. doi:10.1186/1478-811X-9-12
8. Bieback K, Kern S, Kluter H, Eichler H. Critical parameters for the isolation of mesenchymal stem cells from umbilical cord blood. Stem Cells (2004) 22(4):625–34. doi:10.1634/stemcells.22-4-625
9. Barlow S, Brooke G, Chatterjee K, Price G, Pelekanos R, Rossetti T, et al. Comparison of human placenta- and bone marrow-derived multipotent mesenchymal stem cells. Stem Cells Dev (2008) 17(6):1095–107. doi:10.1089/scd.2007.0154
10. In ‘t Anker PS, Scherjon SA, Kleijburg-van der Keur C, de Groot-Swings GM, Claas FH, Fibbe WE, et al. Isolation of mesenchymal stem cells of fetal or maternal origin from human placenta. Stem Cells (2004) 22(7):1338–45. doi:10.1634/stemcells.2004-0058
11. Hoogduijn MJ, Betjes MG, Baan CC. Mesenchymal stromal cells for organ transplantation: different sources and unique characteristics? Curr Opin Organ Transplant (2014) 19(1):41–6. doi:10.1097/MOT.0000000000000036
12. Xishan Z, Baoxin H, Xinna Z, Jun R. Comparison of the effects of human adipose and bone marrow mesenchymal stem cells on T lymphocytes. Cell Biol Int (2013) 37(1):11–8. doi:10.1002/cbin.10002
13. Yang ZX, Han ZB, Ji YR, Wang YW, Liang L, Chi Y, et al. CD106 identifies a subpopulation of mesenchymal stem cells with unique immunomodulatory properties. PLoS One (2013) 8(3):e59354. doi:10.1371/journal.pone.0059354
14. Maumus M, Peyrafitte JA, D’Angelo R, Fournier-Wirth C, Bouloumie A, Casteilla L, et al. Native human adipose stromal cells: localization, morphology and phenotype. Int J Obes (2011) 35(9):1141–53. doi:10.1038/ijo.2010.269
15. Bourin P, Bunnell BA, Casteilla L, Dominici M, Katz AJ, March KL, et al. Stromal cells from the adipose tissue-derived stromal vascular fraction and culture expanded adipose tissue-derived stromal/stem cells: a joint statement of the International Federation for Adipose Therapeutics and Science (IFATS) and the International Society for Cellular Therapy (ISCT). Cytotherapy (2013) 15(6):641–8. doi:10.1016/j.jcyt.2013.02.006
16. Karagianni M, Brinkmann I, Kinzebach S, Grassl M, Weiss C, Bugert P, et al. A comparative analysis of the adipogenic potential in human mesenchymal stromal cells from cord blood and other sources. Cytotherapy (2013) 15(1):76–88. doi:10.1016/j.jcyt.2012.11.001
17. Reinisch A, Etchart N, Thomas D, Hofmann NA, Fruehwirth M, Sinha S, et al. Epigenetic and in vivo comparison of diverse MSC sources reveals an endochondral signature for human hematopoietic niche formation. Blood (2015) 125(2):249–60. doi:10.1182/blood-2014-04-572255
18. Krampera M, Galipeau J, Shi Y, Tarte K, Sensebe L; MSC Committee of the International Society for Cellular Therapy (ISCT). Immunological characterization of multipotent mesenchymal stromal cells – the International Society for Cellular Therapy (ISCT) working proposal. Cytotherapy (2013) 15(9):1054–61. doi:10.1016/j.jcyt.2013.02.010
19. Rasmusson I, Ringden O, Sundberg B, Le Blanc K. Mesenchymal stem cells inhibit lymphocyte proliferation by mitogens and alloantigens by different mechanisms. Exp Cell Res (2005) 305(1):33–41. doi:10.1016/j.yexcr.2004.12.013
20. Nauta AJ, Westerhuis G, Kruisselbrink AB, Lurvink EG, Willemze R, Fibbe WE. Donor-derived mesenchymal stem cells are immunogenic in an allogeneic host and stimulate donor graft rejection in a nonmyeloablative setting. Blood (2006) 108(6):2114–20. doi:10.1182/blood-2005-11-011650
21. Ankrum JA, Ong JF, Karp JM. Mesenchymal stem cells: immune evasive, not immune privileged. Nat Biotechnol (2014) 32(3):252–60. doi:10.1038/nbt.2816
22. Prasanna SJ, Gopalakrishnan D, Shankar SR, Vasandan AB. Pro-inflammatory cytokines, IFNgamma and TNFalpha, influence immune properties of human bone marrow- and Wharton jelly mesenchymal stem cells differentially. PLoS One (2010) 5(2):e9016. doi:10.1371/journal.pone.0009016
23. Duffy MM, Ritter T, Ceredig R, Griffin MD. Mesenchymal stem cell effects on T-cell effector pathways. Stem Cell Res Ther (2011) 2(4):34. doi:10.1186/scrt75
24. Tsai MS, Hwang SM, Chen KD, Lee YS, Hsu LW, Chang YJ, et al. Functional network analysis of the transcriptomes of mesenchymal stem cells derived from amniotic fluid, amniotic membrane, cord blood, and bone marrow. Stem Cells (2007) 25(10):2511–23. doi:10.1634/stemcells.2007-0023
25. Baglio SR, Rooijers K, Koppers-Lalic D, Verweij FJ, Perez Lanzon M, Zini N, et al. Human bone marrow- and adipose-mesenchymal stem cells secrete exosomes enriched in distinctive miRNA and tRNA species. Stem Cell Res Ther (2015) 6(1):127. doi:10.1186/s13287-015-0116-z
26. Barcia RN, Santos JM, Filipe M, Teixeira M, Martins JP, Almeida J, et al. What makes umbilical cord tissue-derived mesenchymal Stromal cells superior immunomodulators when compared to bone marrow derived mesenchymal stromal cells? Stem Cells Int (2015) 2015:583984. doi:10.1155/2015/583984
27. Castro-Manrreza ME, Mayani H, Monroy-Garcia A, Flores-Figueroa E, Chavez-Rueda K, Legorreta-Haquet V, et al. Human mesenchymal stromal cells from adult and neonatal sources: a comparative in vitro analysis of their immunosuppressive properties against T cells. Stem Cells Dev (2014) 23(11):1217–32. doi:10.1089/scd.2013.0363
28. Ivanova-Todorova E, Bochev I, Mourdjeva M, Dimitrov R, Bukarev D, Kyurkchiev S, et al. Adipose tissue-derived mesenchymal stem cells are more potent suppressors of dendritic cells differentiation compared to bone marrow-derived mesenchymal stem cells. Immunol Lett (2009) 126(1–2):37–42. doi:10.1016/j.imlet.2009.07.010
29. Jin HJ, Bae YK, Kim M, Kwon SJ, Jeon HB, Choi SJ, et al. Comparative analysis of human mesenchymal stem cells from bone marrow, adipose tissue, and umbilical cord blood as sources of cell therapy. Int J Mol Sci (2013) 14(9):17986–8001. doi:10.3390/ijms140917986
30. Li X, Bai J, Ji X, Li R, Xuan Y, Wang Y. Comprehensive characterization of four different populations of human mesenchymal stem cells as regards their immune properties, proliferation and differentiation. Int J Mol Med (2014) 34(3):695–704. doi:10.3892/ijmm.2014.1821
31. Luan X, Li G, Wang G, Wang F, Lin Y. Human placenta-derived mesenchymal stem cells suppress T cell proliferation and support the culture expansion of cord blood CD34(+) cells: a comparison with human bone marrow-derived mesenchymal stem cells. Tissue Cell (2013) 45(1):32–8. doi:10.1016/j.tice.2012.09.002
32. Montespan F, Deschaseaux F, Sensebe L, Carosella ED, Rouas-Freiss N. Osteodifferentiated mesenchymal stem cells from bone marrow and adipose tissue express HLA-G and display immunomodulatory properties in HLA-mismatched settings: implications in bone repair therapy. J Immunol Res (2014) 2014:230346. doi:10.1155/2014/230346
33. Najar M, Raicevic G, Boufker HI, Fayyad Kazan H, De Bruyn C, Meuleman N, et al. Mesenchymal stromal cells use PGE2 to modulate activation and proliferation of lymphocyte subsets: combined comparison of adipose tissue, Wharton’s jelly and bone marrow sources. Cell Immunol (2010) 264(2):171–9. doi:10.1016/j.cellimm.2010.06.006
34. Najar M, Raicevic G, Fayyad-Kazan H, De Bruyn C, Bron D, Toungouz M, et al. Impact of different mesenchymal stromal cell types on T-cell activation, proliferation and migration. Int Immunopharmacol (2013) 15(4):693–702. doi:10.1016/j.intimp.2013.02.020
35. Puissant B, Barreau C, Bourin P, Clavel C, Corre J, Bousquet C, et al. Immunomodulatory effect of human adipose tissue-derived adult stem cells: comparison with bone marrow mesenchymal stem cells. Br J Haematol (2005) 129(1):118–29. doi:10.1111/j.1365-2141.2005.05409.x
36. Ribeiro A, Laranjeira P, Mendes S, Velada I, Leite C, Andrade P, et al. Mesenchymal stem cells from umbilical cord matrix, adipose tissue and bone marrow exhibit different capability to suppress peripheral blood B, natural killer and T cells. Stem Cell Res Ther (2013) 4(5):125. doi:10.1186/scrt336
37. Roemeling-van Rhijn M, Khairoun M, Korevaar SS, Lievers E, Leuning DG, Ijzermans JN, et al. Human bone marrow and adipose tissue-derived mesenchymal stromal cells are immunosuppressive and in a humanized allograft rejection model. J Stem Cell Res Ther (2013) 6(Suppl 1):20780. doi:10.4172/2157-7633.S6-001
38. Roemeling-van Rhijn M, Reinders ME, Franquesa M, Engela AU, Korevaar SS, Roelofs H, et al. Human allogeneic bone marrow and adipose tissue derived mesenchymal stromal cells induce CD8+ cytotoxic T cell reactivity. J Stem Cell Res Ther (2013) 3(Suppl 6):004. doi:10.4172/2157-7633.S6-004
39. Stubbendorff M, Deuse T, Hua X, Phan TT, Bieback K, Atkinson K, et al. Immunological properties of extraembryonic human mesenchymal stromal cells derived from gestational tissue. Stem Cells Dev (2013) 22(19):2619–29. doi:10.1089/scd.2013.0043
40. Yoo KH, Jang IK, Lee MW, Kim HE, Yang MS, Eom Y, et al. Comparison of immunomodulatory properties of mesenchymal stem cells derived from adult human tissues. Cell Immunol (2009) 259(2):150–6. doi:10.1016/j.cellimm.2009.06.010
41. Larocca RA, Moraes-Vieira PM, Bassi EJ, Semedo P, de Almeida DC, da Silva MB, et al. Adipose tissue-derived mesenchymal stem cells increase skin allograft survival and inhibit Th-17 immune response. PLoS One (2013) 8(10):e76396. doi:10.1371/journal.pone.0076396
42. Jacobs SA, Roobrouck VD, Verfaillie CM, Van Gool SW. Immunological characteristics of human mesenchymal stem cells and multipotent adult progenitor cells. Immunol Cell Biol (2013) 91(1):32–9. doi:10.1038/icb.2012.64
43. Glennie S, Soeiro I, Dyson PJ, Lam EW, Dazzi F. Bone marrow mesenchymal stem cells induce division arrest anergy of activated T cells. Blood (2005) 105(7):2821–7. doi:10.1182/blood-2004-09-3696
44. Fierabracci A, Del Fattore A, Luciano R, Muraca M, Teti A, Muraca M. Recent advances in mesenchymal stem cell immunomodulation: the role of microvesicles. Cell Transplant (2015) 24(2):133–49. doi:10.3727/096368913X675728
45. Di Nicola M, Carlo-Stella C, Magni M, Milanesi M, Longoni PD, Matteucci P, et al. Human bone marrow stromal cells suppress T-lymphocyte proliferation induced by cellular or nonspecific mitogenic stimuli. Blood (2002) 99(10):3838–43. doi:10.1182/blood.V99.10.3838
46. Meisel R, Zibert A, Laryea M, Gobel U, Daubener W, Dilloo D. Human bone marrow stromal cells inhibit allogeneic T-cell responses by indoleamine 2,3-dioxygenase-mediated tryptophan degradation. Blood (2004) 103(12):4619–21. doi:10.1182/blood-2003-11-3909
47. Wang Q, Yang Q, Wang Z, Tong H, Ma L, Zhang Y, et al. Comparative analysis of human mesenchymal stem cells from fetal-bone marrow, adipose tissue, and Warton’s jelly as sources of cell immunomodulatory therapy. Hum Vaccin Immunother (2015). doi:10.1080/21645515.2015.1030549
48. Aggarwal S, Pittenger MF. Human mesenchymal stem cells modulate allogeneic immune cell responses. Blood (2005) 105(4):1815–22. doi:10.1182/blood-2004-04-1559
49. Carrion F, Nova E, Luz P, Apablaza F, Figueroa F. Opposing effect of mesenchymal stem cells on Th1 and Th17 cell polarization according to the state of CD4+ T cell activation. Immunol Lett (2011) 135(1–2):10–6. doi:10.1016/j.imlet.2010.09.006
50. Sun L, Akiyama K, Zhang H, Yamaza T, Hou Y, Zhao S, et al. Mesenchymal stem cell transplantation reverses multiorgan dysfunction in systemic lupus erythematosus mice and humans. Stem Cells (2009) 27(6):1421–32. doi:10.1002/stem.68
51. Cho KS, Kim YW, Kang MJ, Park HY, Hong SL, Roh HJ. Immunomodulatory effect of mesenchymal stem cells on T lymphocyte and cytokine expression in nasal polyps. Otolaryngol Head Neck Surg (2014) 150(6):1062–70. doi:10.1177/0194599814525751
52. Kang B-J, Ryu H-H, Park SS, Koyama Y, Kikuchi M, Woo H-M, et al. Comparing the osteogenic potential of canine mesenchymal stem cells derived from adipose tissues, bone marrow, umbilical cord blood, and Wharton’s jelly for treating bone defects. J Vet Sci (2012) 13(3):299. doi:10.4142/jvs.2012.13.3.299
53. English K, Ryan JM, Tobin L, Murphy MJ, Barry FP, Mahon BP. Cell contact, prostaglandin E(2) and transforming growth factor beta 1 play non-redundant roles in human mesenchymal stem cell induction of CD4+CD25(High) forkhead box P3+ regulatory T cells. Clin Exp Immunol (2009) 156(1):149–60. doi:10.1111/j.1365-2249.2009.03874.x
54. Engela AU, Baan CC, Dor FJ, Weimar W, Hoogduijn MJ. On the interactions between mesenchymal stem cells and regulatory T cells for immunomodulation in transplantation. Front Immunol (2012) 3:126. doi:10.3389/fimmu.2012.00126
55. Selleri S, Dieng MM, Nicoletti S, Louis I, Beausejour C, Le Deist F, et al. Cord-blood-derived mesenchymal stromal cells downmodulate CD4+ T-cell activation by inducing IL-10-producing Th1 cells. Stem Cells Dev (2013) 22(7):1063–75. doi:10.1089/scd.2012.0315
56. Szabo SJ, Kim ST, Costa GL, Zhang X, Fathman CG, Glimcher LH. A novel transcription factor, T-bet, directs Th1 lineage commitment. Cell (2000) 100(6):655–69. doi:10.1016/S0092-8674(00)80702-3
57. Batten P, Sarathchandra P, Antoniw JW, Tay SS, Lowdell MW, Taylor PM, et al. Human mesenchymal stem cells induce T cell anergy and downregulate T cell allo-responses via the TH2 pathway: relevance to tissue engineering human heart valves. Tissue Eng (2006) 12(8):2263–73. doi:10.1089/ten.2006.12.2263
58. Algeri M, Conforti A, Pitisci A, Starc N, Tomao L, Bernardo ME, et al. Mesenchymal stromal cells and chronic inflammatory bowel disease. Immunol Lett (2015). doi:10.1016/j.imlet.2015.06.018
59. Singh RP, Hasan S, Sharma S, Nagra S, Yamaguchi DT, Wong DT, et al. Th17 cells in inflammation and autoimmunity. Autoimmun Rev (2014) 13(12):1174–81. doi:10.1016/j.autrev.2014.08.019
60. Lee JJ, Jeong HJ, Kim MK, Wee WR, Lee WW, Kim SU, et al. CD39-mediated effect of human bone marrow-derived mesenchymal stem cells on the human Th17 cell function. Purinergic Signal (2014) 10(2):357–65. doi:10.1007/s11302-013-9385-0
61. Tatara R, Ozaki K, Kikuchi Y, Hatanaka K, Oh I, Meguro A, et al. Mesenchymal stromal cells inhibit Th17 but not regulatory T-cell differentiation. Cytotherapy (2011) 13(6):686–94. doi:10.3109/14653249.2010.542456
62. Qu X, Liu X, Cheng K, Yang R, Zhao RC. Mesenchymal stem cells inhibit Th17 cell differentiation by IL-10 secretion. Exp Hematol (2012) 40(9):761–70. doi:10.1016/j.exphem.2012.05.006
63. Eljaafari A, Tartelin ML, Aissaoui H, Chevrel G, Osta B, Lavocat F, et al. Bone marrow-derived and synovium-derived mesenchymal cells promote Th17 cell expansion and activation through caspase 1 activation: contribution to the chronicity of rheumatoid arthritis. Arthritis Rheum (2012) 64(7):2147–57. doi:10.1002/art.34391
64. Li L, Liu S, Xu Y, Zhang A, Jiang J, Tan W, et al. Human umbilical cord-derived mesenchymal stem cells downregulate inflammatory responses by shifting the Treg/Th17 profile in experimental colitis. Pharmacology (2013) 92(5–6):257–64. doi:10.1159/000354883
65. Josefowicz SZ, Lu LF, Rudensky AY. Regulatory T cells: mechanisms of differentiation and function. Annu Rev Immunol (2012) 30:531–64. doi:10.1146/annurev.immunol.25.022106.141623
66. Chattopadhyay G, Shevach EM. Antigen-specific induced T regulatory cells impair dendritic cell function via an IL-10/MARCH1-dependent mechanism. J Immunol (2013) 191(12):5875–84. doi:10.4049/jimmunol.1301693
67. Wing K, Sakaguchi S. Regulatory T cells exert checks and balances on self tolerance and autoimmunity. Nat Immunol (2010) 11(1):7–13. doi:10.1038/ni.1818
68. Selmani Z, Naji A, Zidi I, Favier B, Gaiffe E, Obert L, et al. Human leukocyte antigen-G5 secretion by human mesenchymal stem cells is required to suppress T lymphocyte and natural killer function and to induce CD4+CD25highFOXP3+ regulatory T cells. Stem Cells (2008) 26(1):212–22. doi:10.1634/stemcells.2007-0554
69. Melief SM, Schrama E, Brugman MH, Tiemessen MM, Hoogduijn MJ, Fibbe WE, et al. Multipotent stromal cells induce human regulatory T cells through a novel pathway involving skewing of monocytes toward anti-inflammatory macrophages. Stem Cells (2013) 31(9):1980–91. doi:10.1002/stem.1432
70. Nemeth K, Leelahavanichkul A, Yuen PS, Mayer B, Parmelee A, Doi K, et al. Bone marrow stromal cells attenuate sepsis via prostaglandin E(2)-dependent reprogramming of host macrophages to increase their interleukin-10 production. Nat Med (2009) 15(1):42–9. doi:10.1038/nm.1905
71. Zhang W, Ge W, Li C, You S, Liao L, Han Q, et al. Effects of mesenchymal stem cells on differentiation, maturation, and function of human monocyte-derived dendritic cells. Stem Cells Dev (2004) 13(3):263–71. doi:10.1089/154732804323099190
72. Maccario R, Podesta M, Moretta A, Cometa A, Comoli P, Montagna D, et al. Interaction of human mesenchymal stem cells with cells involved in alloantigen-specific immune response favors the differentiation of CD4+ T-cell subsets expressing a regulatory/suppressive phenotype. Haematologica (2005) 90(4):516–25.
73. Wang L, Zhao Y, Shi S. Interplay between mesenchymal stem cells and lymphocytes: implications for immunotherapy and tissue regeneration. J Dent Res (2012) 91(11):1003–10. doi:10.1177/0022034512460404
74. Casiraghi F, Azzollini N, Cassis P, Imberti B, Morigi M, Cugini D, et al. Pretransplant infusion of mesenchymal stem cells prolongs the survival of a semiallogeneic heart transplant through the generation of regulatory T cells. J Immunol (2008) 181(6):3933–46. doi:10.4049/jimmunol.181.6.3933
75. Fan XB, Gay FPH, Ong SY, Ang JML, Chu PPY, Bari S, et al. Mesenchymal stromal cell supported umbilical cord blood ex vivo expansion enhances regulatory T cells and reduces graft versus host disease. Cytotherapy (2013) 15(5):610–9. doi:10.1016/j.jcyt.2012.12.007
76. Tasso R, Ilengo C, Quarto R, Cancedda R, Caspi RR, Pennesi G. Mesenchymal stem cells induce functionally active T-regulatory lymphocytes in a paracrine fashion and ameliorate experimental autoimmune uveitis. Invest Ophthalmol Vis Sci (2012) 53(2):786–93. doi:10.1167/iovs.11-8211
77. Chao YH, Wu HP, Wu KH, Tsai YG, Peng CT, Lin KC, et al. An increase in CD3+CD4+CD25+ regulatory T cells after administration of umbilical cord-derived mesenchymal stem cells during sepsis. PLoS One (2014) 9(10):e110338. doi:10.1371/journal.pone.0110338
78. Hof-Nahor I, Leshansky L, Shivtiel S, Eldor L, Aberdam D, Itskovitz-Eldor J, et al. Human mesenchymal stem cells shift CD8+ T cells towards a suppressive phenotype by inducing tolerogenic monocytes. J Cell Sci (2012) 125(Pt 19):4640–50. doi:10.1242/jcs.108860
79. Chen PM, Liu KJ, Hsu PJ, Wei CF, Bai CH, Ho LJ, et al. Induction of immunomodulatory monocytes by human mesenchymal stem cell-derived hepatocyte growth factor through ERK1/2. J Leukoc Biol (2014) 96(2):295–303. doi:10.1189/jlb.3A0513-242R
80. Laranjeira P, Gomes J, Pedreiro S, Pedrosa M, Martinho A, Antunes B, et al. Human bone marrow-derived mesenchymal stromal cells differentially inhibit cytokine production by peripheral blood monocytes subpopulations and myeloid dendritic cells. Stem Cells Int (2015) 2015:819084. doi:10.1155/2015/819084
81. Saeidi M, Masoud A, Shakiba Y, Hadjati J, Mohyeddin Bonab M, Nicknam MH, et al. Immunomodulatory effects of human umbilical cord Wharton’s jelly-derived mesenchymal stem cells on differentiation, maturation and endocytosis of monocyte-derived dendritic cells. Iran J Allergy Asthma Immunol (2013) 12(1):37–49.
82. Jiang XX, Zhang Y, Liu B, Zhang SX, Wu Y, Yu XD, et al. Human mesenchymal stem cells inhibit differentiation and function of monocyte-derived dendritic cells. Blood (2005) 105(10):4120–6. doi:10.1182/blood-2004-02-0586
83. Du Rocher B, Mencalha AL, Gomes BE, Abdelhay E. Mesenchymal stromal cells impair the differentiation of CD14(++) CD16(-) CD64(+) classical monocytes into CD14(++) CD16(+) CD64(++) activate monocytes. Cytotherapy (2012) 14(1):12–25. doi:10.3109/14653249.2011.594792
84. Kim N, Cho SG. New strategies for overcoming limitations of mesenchymal stem cell-based immune modulation. Int J Stem Cells (2015) 8(1):54–68. doi:10.15283/ijsc.2015.8.1.54
85. Galderisi U, Giordano A. The gap between the physiological and therapeutic roles of mesenchymal stem cells. Med Res Rev (2014) 34(5):1100–26. doi:10.1002/med.21322
86. Shi C. Recent progress toward understanding the physiological function of bone marrow mesenchymal stem cells. Immunology (2012) 136(2):133–8. doi:10.1111/j.1365-2567.2012.03567.x
87. Kronsteiner B, Wolbank S, Peterbauer A, Hackl C, Redl H, van Griensven M, et al. Human mesenchymal stem cells from adipose tissue and amnion influence T-cells depending on stimulation method and presence of other immune cells. Stem Cells Dev (2011) 20(12):2115–26. doi:10.1089/scd.2011.0031
88. Najar M, Rouas R, Raicevic G, Boufker HI, Lewalle P, Meuleman N, et al. Mesenchymal stromal cells promote or suppress the proliferation of T lymphocytes from cord blood and peripheral blood: the importance of low cell ratio and role of interleukin-6. Cytotherapy (2009) 11(5):570–83. doi:10.1080/14653240903079377
89. Menard C, Pacelli L, Bassi G, Dulong J, Bifari F, Bezier I, et al. Clinical-grade mesenchymal stromal cells produced under various good manufacturing practice processes differ in their immunomodulatory properties: standardization of immune quality controls. Stem Cells Dev (2013) 22(12):1789–801. doi:10.1089/scd.2012.0594
Keywords: mesenchymal stromal cells, immunomodulation, T cells, regulatory T cells, macrophages, bone marrow, adipose tissue, umbilical cord
Citation: Mattar P and Bieback K (2015) Comparing the immunomodulatory properties of bone marrow, adipose tissue, and birth-associated tissue mesenchymal stromal cells. Front. Immunol. 6:560. doi: 10.3389/fimmu.2015.00560
Received: 11 August 2015; Accepted: 19 October 2015;
Published: 03 November 2015
Edited by:
Luis Graca, University of Lisbon, PortugalReviewed by:
Paulo Vieira, Institut Pasteur de Paris, FranceMartin Johannes Hoogduijn, Erasmus Medical Center, Netherlands
Copyright: © 2015 Mattar and Bieback. This is an open-access article distributed under the terms of the Creative Commons Attribution License (CC BY). The use, distribution or reproduction in other forums is permitted, provided the original author(s) or licensor are credited and that the original publication in this journal is cited, in accordance with accepted academic practice. No use, distribution or reproduction is permitted which does not comply with these terms.
*Correspondence: Karen Bieback, a2FyZW4uYmllYmFjayYjeDAwMDQwO21lZG1hLnVuaS1oZWlkZWxiZXJnLmRl