- 1Sotio, Prague, Czech Republic
- 2Department of Immunology, 2nd Faculty of Medicine, University Hospital Motol, Charles University, Prague, Czech Republic
- 3Equipe 11 labellisée par la Ligue Nationale contre le Cancer, Centre de Recherche des Cordeliers, Paris, France
- 4U1138, INSERM, Paris, France
- 5Sorbonne Paris Cité, Université Paris Descartes, Paris, France
- 6Université Pierre et Marie Curie, Paris, France
- 7Metabolomics and Cell Biology Platforms, Gustave Roussy Comprehensive Cancer Institute, Villejuif, France
- 8Equipe 13, Centre de Recherche des Cordeliers, Paris, France
- 9Institute of Hematology and Blood Transfusion, Prague, Czech Republic
- 10Department of Gynecology and Obsterics, 2nd Faculty of Medicine, University Hospital Motol, Charles University, Prague, Czech Republic
- 11Pôle de Biologie, Hopitâl Européen George Pompidou, AP-HP, Paris, France
- 12Gustave Roussy Comprehensive Cancer Institute, Villejuif, France
It is now clear that human neoplasms form, progress, and respond to therapy in the context of an intimate crosstalk with the host immune system. In particular, accumulating evidence demonstrates that the efficacy of most, if not all, chemo- and radiotherapeutic agents commonly employed in the clinic critically depends on the (re)activation of tumor-targeting immune responses. One of the mechanisms whereby conventional chemotherapeutics, targeted anticancer agents, and radiotherapy can provoke a therapeutically relevant, adaptive immune response against malignant cells is commonly known as “immunogenic cell death.” Importantly, dying cancer cells are perceived as immunogenic only when they emit a set of immunostimulatory signals upon the activation of intracellular stress response pathways. The emission of these signals, which are generally referred to as “damage-associated molecular patterns” (DAMPs), may therefore predict whether patients will respond to chemotherapy or not, at least in some settings. Here, we review clinical data indicating that DAMPs and DAMP-associated stress responses might have prognostic or predictive value for cancer patients.
Introduction
For a long time, tumors were considered as highly homogenous entities resulting from the clonal expansion of a single cell with specific genetic or epigenetic defects (1). Now, it is clear that both hematopoietic and solid neoplasms are highly heterogenous, not only because malignant cells with distinct phenotypic and behavioral features generally co-exist, but also because multiple non-transformed cells are co-opted by growing cancers to support their needs. This is especially true for solid tumors, which contain an abundant non-malignant cellular compartment encompassing stromal, endothelial, and immune components (2, 3). The immune compartment of the tumor mass is per se very heterogenous, varying not only with tumor type, stage, and therapeutic regimen, but also on an inter-individual basis (4). Evidence accumulating over the last decade indicates indeed that human tumors form, progress, and respond to therapy in the context of an intimate, bidirectional interaction with the immune system (5, 6). Thus, clinically manifest neoplasms can develop only when they are able to escape immunosurveillance (7, 8), and they do so by evolving under the selective pressure imposed by the immune system (6, 9). Moreover, the composition, density, and intratumoral localization of the immune infiltrate have been ascribed with a robust prognostic or predictive value in several cohorts of cancer patients (10–12). Finally, the efficacy of most, if not all, therapeutic regimens commonly employed in cancer patients has been etiologically linked to the (re)elicitation of an adaptive immune response targeting malignant cells (13, 14).
Conventional chemotherapeutics and targeted anticancer agents can favor the (re)elicitation of anticancer immune responses through several mechanisms (13–15). A precise description of all these immunostimulatory pathways goes largely beyond the scope of this review, and can be found in Ref. (13, 14). However, it is useful to note that anticancer therapy can boost immunosurveillance by either of two mechanisms. First, it can directly modulate the functions of immune cells, including dendritic cells (DCs), myeloid-derived suppressor cells (MDSCs), tumor-associated macrophages (TAMs), CD8+ cytotoxic T lymphocytes (CTLs), and CD4+CD25+FOXP3+ regulatory T (TREG) cells (14). Second, it can promote the immunogenicity or adjuvanticity of cancer cells as it subjects them to a state of stress (which sometimes leads to their death) (14, 16). In particular, some chemotherapeutic agents like anthracyclines, oxaliplatin, and bortezomib, as well as specific forms of radiation therapy and photodynamic therapy, are able to trigger a functionally peculiar variant of caspase-dependent cell death that per se is perceived as immunogenic by the immune system (17–21). This means that, upon inoculation in immunocompetent hosts, cells succumbing to such an immunogenic form of cell death are sufficient to elicit an adaptive immune response against dead cell-associated antigens associated with the establishment of immunological memory (22, 23).
Mechanistically, immunogenic cell death (ICD) relies on the pre-mortem activation of several stress response pathways that are associated with the emission of a well-defined set of danger signals by dying cancer cells (24–26). When delivered in the correct spatiotemporal order, such damage-associated molecular patterns (DAMPs) recruit specific cellular components of the innate and adaptive immune system to the tumor bed and activate them, ultimately resulting in the elicitation of a tumor-targeting immune response (22, 26). Conversely, in physiological conditions DAMPs are generally inaccessible to the immune system, and serve metabolic, structural, or enzymatic functions (26–28). Of note, DAMPs are not only involved in ICD-associated anticancer immunosurveillance, but also play a key role in the etiology of shock conditions triggered by trauma and other non-microbial stimuli (29, 30).
So far, four DAMPs have been ascribed a non-redundant, essential function in the context of anthracycline-induced ICD, namely (1) the pre-apoptotic exposure of the endoplasmic reticulum chaperone calreticulin (CALR) and various heat-shock proteins (HSPs) on the outer leaflet of the plasma membrane, which ensues the activation of an ER stress response orchestrated around the phosphorylation of eukaryotic translation initiation factor 2A, 65 kDa (EIF2A) and the overgeneration of reactive oxygen species (ROS) (31–36); (2) the production of type I interferons (IFNs), which depends on Toll-like receptor 3 (TLR3) signaling (37–40); (3) the secretion of ATP, which relies on the activation of autophagy (41, 42); and (4) the release of the non-histone chromatin-binding protein high mobility group box 1 (HMGB1) into the extracellular space, which correlates with cell death induction (43, 44). The role of other DAMPs such as mitochondrial DNA (mtDNA), N-formylated peptides, cardiolipin, and filamentous (F)-actin in ICD signaling has not yet been investigated in detail (30, 45).
Accumulating preclinical evidence indicates that monitoring DAMPs or DAMP-associated stress responses in cancer patients may have prognostic or predictive value. Here, we review clinical data lending further support to this hypothesis.
Calreticulin, HSPs, and the ER Stress Response
Cancer cells undergoing ICD exhibit several manifestations of the so-called unfolded protein response (UPR) (34, 46), i.e., the ensemble of mechanisms aimed at the re-establishment of intracellular homeostasis following the accumulation of unfolded proteins within the ER lumen (47). In particular, ICD is etiologically associated with the phosphorylation of EIF2A on S51 (48), and this appears to be required for the exposure of CALR and HSPs on the surface of dying cells (34). On the cell surface, CALR, heat shock 70 kDa protein 1A (HSPA1A, best known as HSP70) and heat shock protein 90 kDa alpha (cytosolic), class A member 1 (HSP90AA1, best known as HSP90) play partially overlapping (but not identical) immunostimulatory functions. Indeed, CALR, HSP70 and HSP90 all bind to low density lipoprotein receptor-related protein 1 (LRP1, best known as CD91) on antigen-presenting cells (APCs), hence stimulating the uptake of dead cell-associated antigens in the form of apoptotic bodies (32, 33). HSP70 and HSP90 favor CTL cross-priming by APCs upon interaction with Toll-like receptor 4 (TLR4) and CD14 (33, 49–51). In some settings, soluble HSPs and CALR operate as cytokines, stimulating the NF-κB-dependent secretion of pro-inflammatory mediators like interleukin-6 (IL-6) and tumor necrosis factor α (TNFα) (52, 53). HSP70 boosts the cytotoxic functions of natural killer (NK) cells by binding to killer cell lectin-like receptor subfamily D, member 1 (KLRD1, best known as CD94) (54, 55). Moreover, ecto-HSP70 binds to phosphatidylserine (PS), a phospholipid that is exposed in the course of regulated cell death owing to the caspase-dependent activation of phospholipid scramblase 1 (PLSCR1) (56). The actual relevance of this interaction for ICD, however, has not been determined yet. Along similar lines, it remains obscure whether additional CALR receptors such as CD69; thrombospondin 1 (THBS1); complement component 1, q subcomponent (C1q); lectin, mannose-binding, 1 (LMAN1); and various integrins of the CD49 family are etiologically implicated in the perception of ICD (57). Of note, ecto-CALR has been suggested to act as a DC receptor for the tumor-associated antigen (TAA) NY-ESO-1, hence facilitating the interaction between DCs and malignant cells (58). To the best of our knowledge, however, this finding has not been confirmed by independent investigators.
Accumulating clinical evidence indicates that various parameters linked to ICD-associated CALR and HSP signaling may have prognostic or predictive value for cancer patients (Table 1). In addition, the results of multiple clinical trials suggest that HSPs can be harnessed as a means to boost the efficacy of anticancer vaccines. High CALR levels in malignant cells have been shown to correlate with favorable disease outcome in a cohort of 68 neuroblastoma patients (irrespective of treatment) (59), and in a cohort of 23 lung cancer patients and 220 ovarian cancer patients treated with ICD inducers (i.e., radiotherapy and paclitaxel, respectively) (60). Moreover, increased CALR expression by cancer cells has been associated with tumor infiltration by CD45RO+ memory T cells and improved 5-year overall survival amongst 68 subjects with Stage IIIB colorectal carcinoma (CRC) (61). Elevated levels of HSP90 and CALR on the surface of neoplastic cells have been associated with clinical responses amongst 18 patients with relapsed indolent non-Hodgkin’s lymphoma treated with an autologous cancer cell-based vaccine (62). Moreover, CALR exposure by malignant blasts has been linked to prolonged relapse-free (but not overall) survival in a cohort of 20 individuals with acute myeloid leukemia (AML) (63). Of note, the blasts of some of these patients exposed CALR spontaneously, and this correlated not only with the degree of EIF2A phosphorylation in malignant cells, but also with the ability of autologous T cells to secrete IFNγ on stimulation (63). Along similar lines, healthy individuals have been shown to differ from lung carcinoma patients with respect to the circulating levels of soluble CALR, as well as to the amount of CALR expressed on the surface of pulmonary (normal versus malignant) cells (64). Moreover, increased concentrations of soluble HSP90 have been detected in the serum of CRC patients (n = 172) as compared to healthy individuals (n = 10) (65). Interestingly, soluble HSP90 appears to activate cancer cell-intrinsic signaling pathways that promote disease progression (65, 66). These data indicate that cancer cells expose and/or shed CALR as well as HSPs even in the absence of chemotherapy (at least to some degree), possibly as a result of oncogenic stress and/or adverse microenvironmental conditions. Moreover, they suggest that membrane-bound CALR and HSPs have a different biological activity than their soluble counterparts.
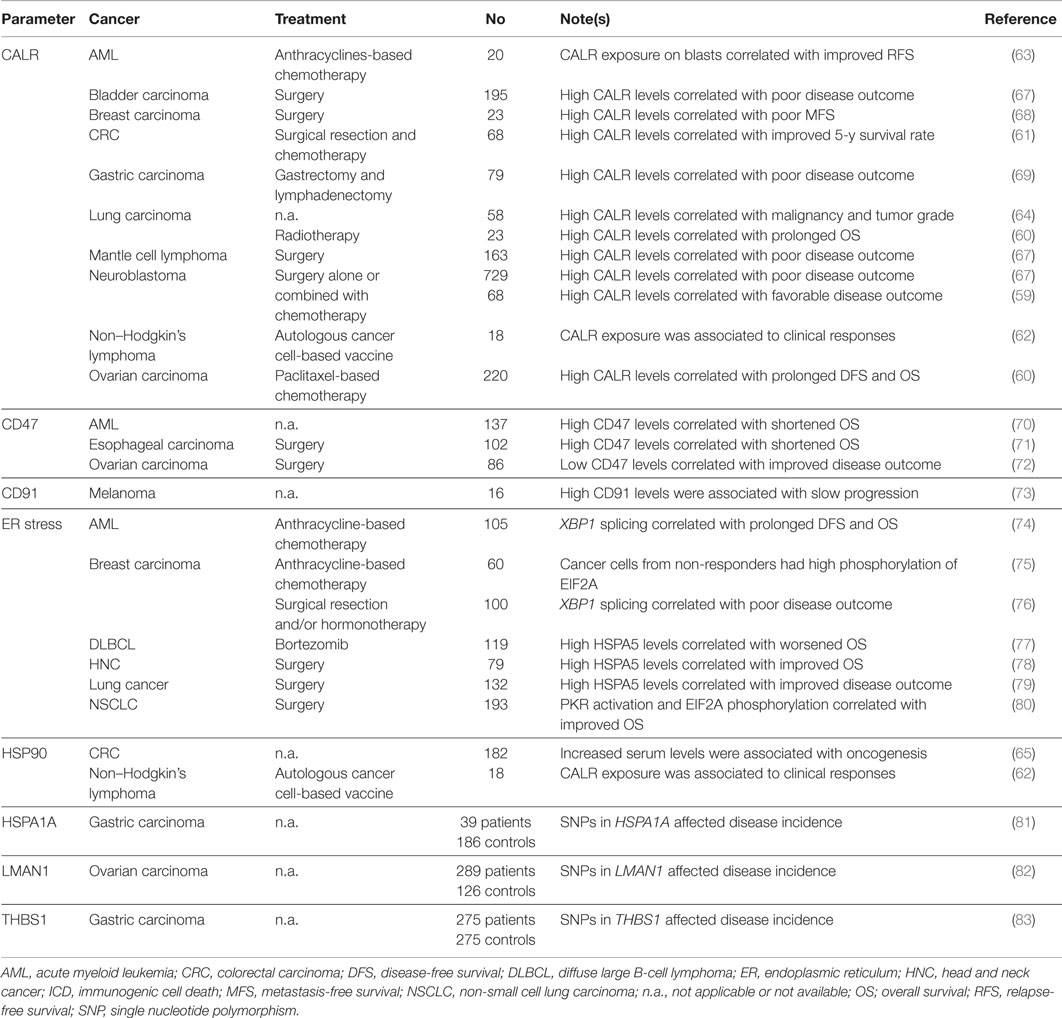
Table 1. Clinical studies assessing the prognostic and predictive value of ICD-associated CALR and HSP signaling in cancer patients.
Apparently at odds with the abovementioned clinical findings, total CALR levels have been positively associated with accelerated disease progression and poor outcome in a cohort of 79 gastric cancer patients (69), in 23 women with breast carcinoma upon surgery (68), as well in large cohorts of neuroblastoma (n = 729), bladder carcinoma (n = 195) and mantle cell lymphoma (n = 163) patients, irrespective of treatment type (67). Moreover, CALR expression by malignant cells failed to affect overall survival in 88 patients with esophageal squamous cell carcinoma treated with neo-adjuvant chemoradiotherapy and surgical resection (84). These results may reflect the intracellular functions of CALR in the preservation of reticular homeostasis, which is particularly important for malignant cells owing to their highly accelerated anabolic metabolism (85), or the fact that CALR exposure is generally associated with an increased expression of CD47, a very potent anti-phagocytic signal (67).
The phosphorylation of EIF2A as well as the activation of eukaryotic translation initiation factor 2-alpha kinase 2 (EIF2AK2, best known as PKR) have been associated with favorable disease outcome in a cohort of 193 non-small cell lung carcinoma (NSCLC) patients (80). On the contrary, elevated degrees of EIF2A phosphorylation in neoplastic cells have been correlated with nuclear size (a surrogate marker of DNA content), preferential tumor infiltration by TREG cells, and poor disease outcome in a cohort of 60 breast carcinoma patients treated with anthracycline-based chemotherapy and tested longitudinally (75). Other manifestations on an ongoing UPR have been ascribed with prognostic or predictive value, including (but not limited to): (1) the expression levels of the ER chaperone heat shock 70 kDa protein 5 (HSPA5, best known as GRP78), as demonstrated in cohorts of 132 lung carcinoma patients (79), 79 individuals with head and neck cancer (78) and 119 patients with diffuse large B-cell lymphoma treated with the proteasome inhibitor bortezomib (which is a bona fide ICD inducer) (77); and (2) the splicing of X-box binding protein 1 (XBP1) (48), as demonstrated in a cohort of 105 AML patients tested at diagnosis (74). Of note, both CALR and GRP78 expression levels are also indirect manifestations of the activation of another branch of the ER stress response, i.e., the derepression of activating transcription factor 6 (ATF6) (74, 86). Finally, some studies have associated markers of an ongoing UPR with dismal disease outcome. For instance, Davies and colleagues have linked low levels of unspliced XBP1 as well as a high spliced/unspliced XBP1 ratio with poor disease outcome in 100 primary breast carcinoma patients treated with adjuvant hormonal therapy (76). The apparent discrepancy in these observations may reflect the differential reliance of distinct tumor types (or similar tumors at distinct stages of progression) on the ER stress response for survival in adverse microenvironment conditions (87).
Other processes and parameters linked to CALR and/or HSP exposure and their immunostimulatory effects have been shown to influence disease outcome in cancer patients. For instance, high CD47 levels have been reported to constitute an independent negative prognostic factor in cohorts of 86 patients with ovarian clear cell carcinoma (72), 102 individuals with esophageal squamous cell carcinoma (71), and 137 subjects with karyotypically normal AML (70). Along similar lines, the monocytes of 8 advanced melanoma patients progressing in an unusually slow fashion have been found to express increased amounts of CD91 as compared to those of 8 patients progressing normally (73). Moreover, single nucleotide polymorphisms (SNPs) affecting HSPA1A have been linked to an increased incidence of gastric carcinoma (as determined in a cohort of 39 patients and 186 controls) (81), a SNP affecting THBS1 has been correlated with gastric cancer occurrence and progression in a cohort of 275 patients and 275 healthy individuals (83), while a SNP in LMAN1 as well as the consequent decrease in LMAN1 levels appear to be associated with an increased risk for ovarian carcinoma (as determined in a cohort of 289 women seen in gynecologic oncology practice and 126 healthy volunteers) (82).
The robust immunostimulatory activity of HSPs has been harnessed to develop various anticancer vaccines that are nowadays in clinical development. These preparations generally consist in HSP-enriched (autologous or heterologous) cancer cell lysates that are administered directly to patients, in the presence of adequate immunological adjuvants (88, 89). The most common of these approaches relies on heat shock protein 90 kDa beta (Grp94), member 1 (HSP90B1, best known as GP96) and is often referred to as HSPPC-96 (Oncophage® or Vitespen®) (90). So far, the safety and clinical profile of HSPPC-96 have been tested in cohorts of patients with metastatic melanoma (n = 36–322) (91–94), CRC (n = 29) (95), non-Hodgkin’s lymphoma (n = 20) (96); pancreatic adenocarcinoma (n = 10) (97), metastatic renal cell carcinoma (n = 84–409) (98, 99), glioma (n = 12) (100), recurrent glioblastoma (n = 41) (101), and assorted advanced malignancies (n = 16) (102). These studies demonstrate that the administration of HSPPC-96 to cancer patients is safe and is generally associated with markers of immunostimulation. However, most often such effects are weak and unable to mediate long-term therapeutic activity (99). Thus, further studies are required for translating the well-established ability of HSPs to stimulate the priming of TAA-specific immune responses into a therapeutic reality.
Taken together, these clinical observations suggest that CALR, HSPs and various processes associated with their exposure, secretion and signaling functions may have prognostic, predictive and therapeutic value.
Type I IFN and TLR3 Signaling
Cancer cells responding to anthracyclines secrete type I IFNs as a consequence of TLR3 activation (39), and this is required for cell death to initiate adaptive immunity (39). By binding to homodimeric or heterodimeric receptors expressed on several immune effector cells, type I IFNs mediate multipronged immunostimulatory effects (40). In particular, type I IFNs promote cross-priming (103), boost the cytotoxic functions of CTLs and NK cells (104), and increase the survival of memory CTLs (105). Moreover, type I IFNs can protect antigen-activated CD8+ CTLs from elimination by NK cells (106, 107), trigger the secretion of pro-inflammatory mediators by macrophages (108), and counteract the immunosuppressive functions of TREG cells (109). Besides such immunostimulatory effects, type I IFNs can ignite a cancer cell-intrinsic signal transduction pathway leading, amongst various effects, to the synthesis of the chemotactic factor chemokine (C–X–C motif) ligand 10 (CXCL10) (39). Indeed, at odds with their wild-type counterparts, Ifnar1−/− cancer cells succumbing to anthracyclines are unable to prime adaptive immune responses, even upon inoculation in wild-type hosts (39). Thus, type I IFN signaling in cancer cells appears to be critical for anthracycline-induced cell death to be perceived as immunogenic (39). Conversely, the efficacy of other immunotherapeutic agents such as the TLR7 agonist imiquimod requires type I IFN signaling in the host (110).
So far, only a few studies addressed the prognostic or predictive value of parameters reflecting the proficiency or activation status of TLR3 or type I IFN signaling (Table 2). High expression levels of TLR3 and/or toll-like receptor adaptor molecule 1 (TICAM1, a component of the TLR3 signaling apparatus best known as TRIF) have been associated with improved disease outcome in two cohorts of 85 and 172 subjects with hepatocellular carcinoma (HCC) (111, 112), as well as amongst 99 patients with neuroblastoma (113). Along similar lines, TLR3 expression levels have been shown to predict the response of 194 breast carcinoma patients treated with adjuvant radiotherapy plus a TLR3 agonist (114). SNPs affecting TLR3 have been shown to influence prognosis in cohorts of 582 patients with CRC, especially among untreated individuals with Stage II disease (115) and 568 NSCLC patients (116). Along similar lines, TLR3 SNPs have been associated with an altered risk for cervical cancer amongst 330 Tunisian women (117), breast carcinoma amongst 174 African-American women (118), oral squamous cell carcinoma amongst 197 individuals (119) HCC amongst 948 subjects (120), and CRC amongst more than 5,000 individuals (121). A type I IFN-related transcription signature centered around the expression of MX dynamin-like GTPase 1 (MX1) has been shown to predict the likelihood of 50 breast carcinoma patients to respond to neo-adjuvant anthracycline-based chemotherapy (39). Moreover, SNPs affecting interferon (alpha, beta and omega) receptor 1 (IFNAR1) have been associated with an increased risk for the development of CRC amongst 2085 individuals (122), as well as with significantly reduced overall survival in a cohort of 304 glioma patients (123). Similar results have been obtained for SNPs affecting the genes coding for two variants of IFNα (i.e., IFNA7 and IFNA8) (122, 123).
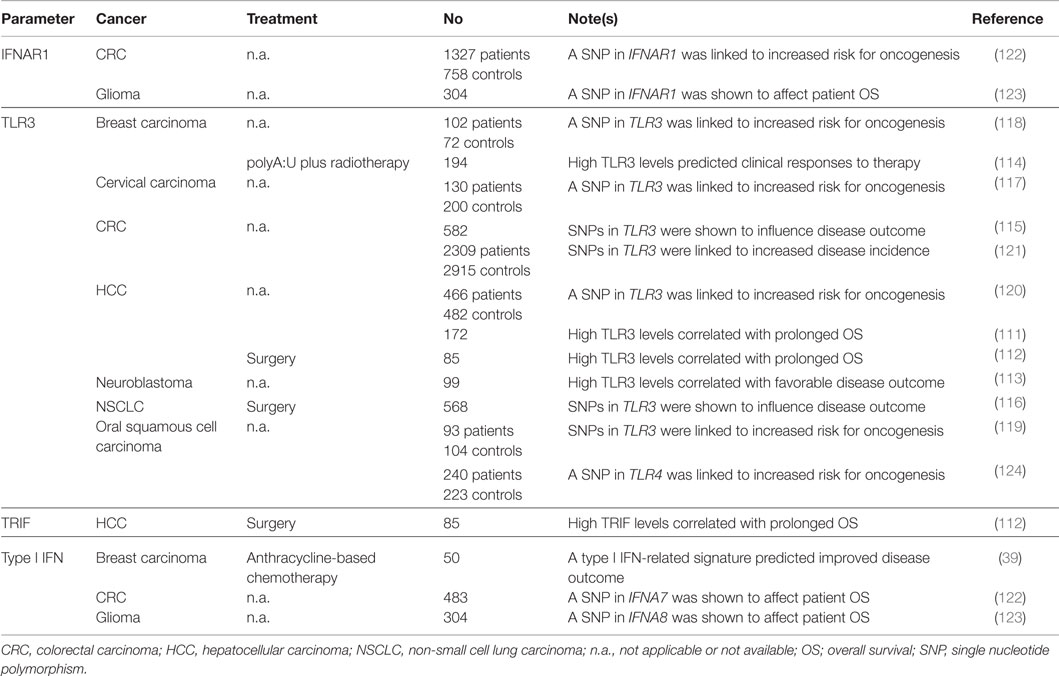
Table 2. Clinical studies assessing the prognostic and predictive value of TLR3 status and type I IFN signaling in cancer patients.
The results of these studies suggest that monitoring biomarkers of TLR3 and type I IFN signaling may not only have prognostic/predictive relevance for cancer patients, but also inform on the risk for cancer development in healthy subjects. Of note, recombinant IFN-α2a (Roferon-A®) is approved by the US Food and Drug Administration and other regulatory agencies worldwide for use in subjects with hairy cell leukemia and Philadelphia chromosome-positive chronic myelogenous leukemia upon minimal pretreatment, while recombinant IFN-α2b (Intron A®) is currently employed for the treatment of hairy cell leukemia, AIDS-related Kaposi’s sarcoma, follicular lymphoma, multiple myeloma, melanoma, condyloma acuminata and cervical intraepithelial neoplasms.(125, 126) It remains to be determined to which extent, if any, the therapeutic efficacy of type I IFNs reflects their ability to promote the initiation of adaptive immune responses against dying cancer cells.
Extracellular ATP and Autophagy
ATP is secreted during ICD through a mechanism that involves pannexin 1 (PANX1) channels and lysosomal exocytosis (127, 128). Importantly, autophagy is required for cancer cells succumbing to anthracyclines to release ATP in immunostimulatory amounts (42, 129, 130). Thus, the ability of anthracyclines to cause bona fide ICD is lost when cancer cells are rendered autophagy-deficient by genetic manipulations or engineered to overexpress ectonucleoside triphosphate diphosphohydrolase 1 (ENTPD1, best known as CD39), an enzyme that degrades extracellular ATP (42, 129). In line with this notion, the administration of CD39 inhibitors or CD39-neutralizing monoclonal antibodies reportedly relieves tumor-mediated immunosuppression (131), and (at least in some models) allows autophagy-deficient cells treated with anthracyclines to elicit normal immune responses upon inoculation in immunocompetent mice (42, 129). Extracellular ATP exerts immunostimulatory functions via at least three mechanistically distinct pathways: (1) by promoting the recruitment of APCs or APC precursors to sites of cell death, upon binding to purinergic receptor P2Y, G-protein coupled, 2 (P2RY2) (132–134); (2) by activating the so-called NLRP3 inflammasome and hence triggering the secretion of pro-inflammatory IL-1β (135, 136), an effect that relies on purinergic receptor P2X, ligand gated ion channel, 7 (41); and (3) by boosting the proliferation and cytotoxic activity of NK cells (26). Notably, extracellular ATP is sequentially metabolized by CD39 and 5′-nucleotidase, ecto (NT5E, best known as CD73) into ADP, AMP and adenosine, the latter of which has robust immunosuppressive effects (137).
Accumulating clinical evidence ascribes to parameters linked to the capacity of cancer cells to recruit and activate immune effectors (through extracellular ATP) a prognostic or predictive value for cancer patients (Table 3). A SNP compromising the function of P2RX7 has been associated with decreased time-to-metastasis in a cohort of 225 breast carcinoma patients treated with adjuvant anthracycline-based chemotherapy (41), with worsened clinicopathological parameters amongst 121 subjects with papillary thyroid cancer (138), and with an increased risk for the development of chronic lymphocytic leukemia (CLL), as determined in a cohort of 40 patients and 46 age-matched healthy individuals (139). Contrasting with these latter findings, however, the same SNP has been associated with increased overall survival in a cohort of 170 subjects with CLL (140), or found to have no correlation with disease incidence and/or outcome in independent cohorts of 144 CLL patients and 348 healthy controls (141), 121 individuals with CLL (142) 111 CLL patients and 97 controls (143), and 136 subjects with multiple myeloma (144). These apparently discrepant observations may reflect the cancer cell-intrinsic functions of P2RX7, which is known to control proliferation and regulated cell death (145). Of note, increased P2RY2 mRNA levels have also been detected in gastric cancer biopsies from 14 patients (as compared to the adjacent healthy mucosa) (146), but these findings do not allow to determine whether gastric neoplasms were infiltrated by P2RY2+ immune cells or whether they overexpressed P2RY2.
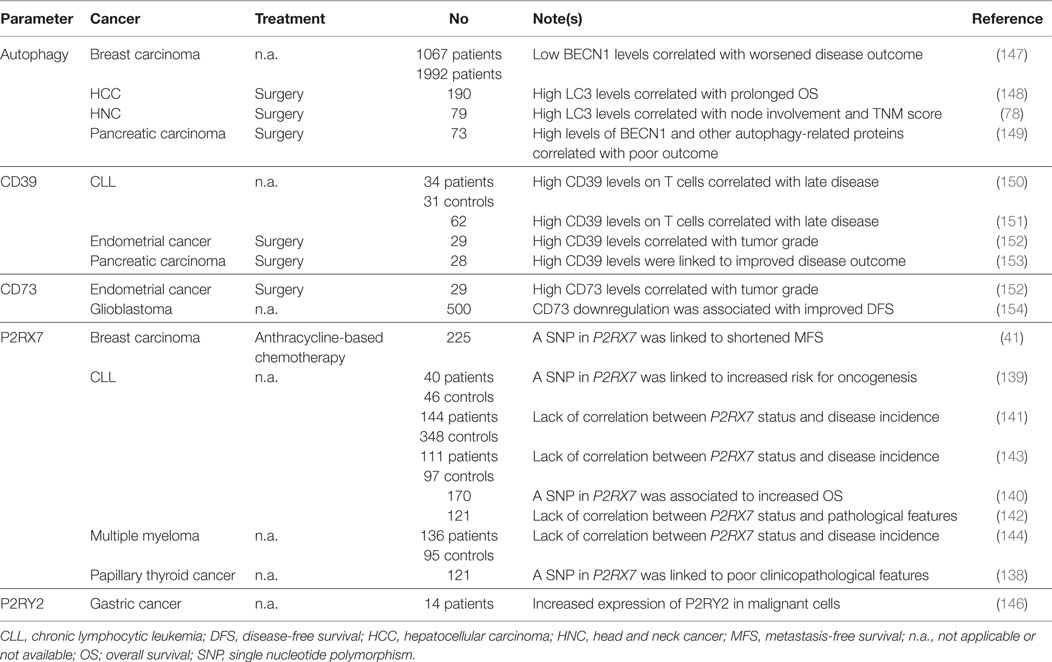
Table 3. Clinical studies assessing the prognostic and predictive value of ATP release and extracellular ATP signaling in cancer patients.
Further corroborating the advantage conferred to malignant cells by an increased ability to convert immunostimulatory extracellular ATP into immunosuppressive AMP and adenosine, several studies ascribed a negative prognostic or predictive value to increased CD39 or CD73 levels. For instance, elevated amounts of CD39 and CD73 have been detected in 29 endometrial tumor samples as compared to the adjacent non-malignant tissues, and expression levels correlated with tumor grade (152). Along similar lines, CD39 (but not CD73) levels on the surface of CD4+ and CD8+ T cells have been shown to positively correlate with disease stage in two independent cohorts of 34 and 62 patients with CLL (150, 151), while CD73 downregulation has been associated with prolonged disease-free survival amongst 500 individuals with glioblastoma (154). At stark contrast with these findings, high levels of CD39 mRNA have been linked to improved disease outcome in a cohort of 28 pancreatic cancer patients treated with surgery (153). The reasons underlying this discrepancy have not yet been clarified.
Of note, quantifying functional autophagy in tissue biopsies is rather complex, because most autophagic markers accumulate both when the autophagic flux is increased and when lysosomal degradation is blocked (155). Moreover, autophagy often serves a dual role in the course of tumor progression: (1) on the one hand it favors the survival of cancer cells exposed to adverse microenvironmental conditions (including nutritional, metabolic and therapeutic cues); (2) on the other hand, it is required for ICD-associated ATP secretion and for the elicitation of robust TAA-targeting immune responses (130, 156, 157). Notwithstanding these caveats, immunohistochemistry has been employed to study the prognostic or predictive value of autophagic markers such as the expression and lipidation of microtubule-associated protein 1 light chain 3 (MAP1LC3, best known as LC3) (158), with mixed results. For instance, LC3 expression has been associated with prolonged overall survival in a cohort of 190 HCC patients (148), but with lymph node involvement and high TNM score amongst 79 individuals with head and neck cancer (78). Along similar lines, reduced expression of beclin 1 (BECN1), a key component of the molecular machinery for autophagy, has been associated with poor prognosis in two independent cohorts of 1067 and 1992 breast carcinoma patients (147), but with improved disease outcome in a cohort of 73 patients with pancreatic cancer (149). These are only two examples of an abundant scientific literature correlating the expression of autophagy proteins in biopsies from patients affected with virtually all types of malignancies to clinicopathological features and/or markers of disease progression. The development of assays to monitor the functionality of the autophagic apparatus in clinical samples is urgently awaited to properly assess the prognostic and predictive value of autophagy for cancer patients.
HMGB1 and Cell Death
According to current models, HMGB1 gets released in the course of cell death passively, upon the breakdown of the nuclear and plasma membrane (145, 159). Thus, besides differences in expression level, the extent of HMGB1 release generally correlates with the degree of cell death (160). However, changes in the oxidation status of extracellular HMGB1 have been suggested to dramatically alter its biological activity (161–163). Indeed, while reduced HMGB1 efficiently dimerizes with CXCL12 and mediate potent chemotactic functions upon binding to chemokine (C–X–C motif) receptor 4 (CXCR4) (164, 165), its oxidized counterpart fails to do so (162). Rather, oxidized HMGB1 signal via TLR2, TLR4 and advanced glycosylation end product-specific receptor (AGER, best known as RAGE) to stimulate the production of pro-inflammatory cytokines (162, 166–168). In addition, TLR4 signaling promotes cross-priming by inhibiting the fusion of antigen-containing endosomes with lysosomes (169). Interestingly, HMGB1 also binds to TLR9 (170) and hepatitis A virus cellular receptor 2 (HAVCR2, best known as TIM-3) (171), in particular when complexed with DNA. However, while TLR9 promotes cytokine secretion by plasmacytoid DCs and B cells (170), TIM-3 signaling blunts the ability of DCs to respond efficiently to inflammatory stimuli (171). Thus, extracellular HMGB1 mediates multipronged and context-dependent immunomodulatory functions.
Various clinical studies indicate that monitoring parameters linked to HMGB1 release and signaling may convey prognostic or predictive information for cancer patients (Table 4). High expression levels of HMGB1 in malignant cells have been shown to correlate with improved overall survival in 88 patients with esophageal squamous cell carcinoma subjected to neo-adjuvant chemoradiotherapy and surgical resection (84), as well as in 76 subjects with reseactable gastric adenocarcinoma (172). In a cohort of 232 breast carcinoma patients treated with anthracycline-based adjuvant chemotherapy, loss of nuclear HMGB1 has been positively associated with tumor size (173). Along similar lines, the co-expression of HMGB1 in the nucleus and in the cytoplasm of malignant cells has been shown to inversely correlate with tumor infiltration by CD45RO+ memory T cells and 5-year survival rate in 72 individuals with Stage IIIB CRC (174). Finally, HMGB1 overexpression has been shown to correlate with advanced clinical stage or decreased disease-free and/or overall survival amongst 164 patients with bladder carcinoma (175), 166 individuals with nasopharyngeal carcinoma (176), 192 CRC patients (177), 208 and 161 individuals with HCC (178, 179), 103 subjets with head and neck squamous cell carcinoma (180), as well as 85 patients with prostate cancer (181).
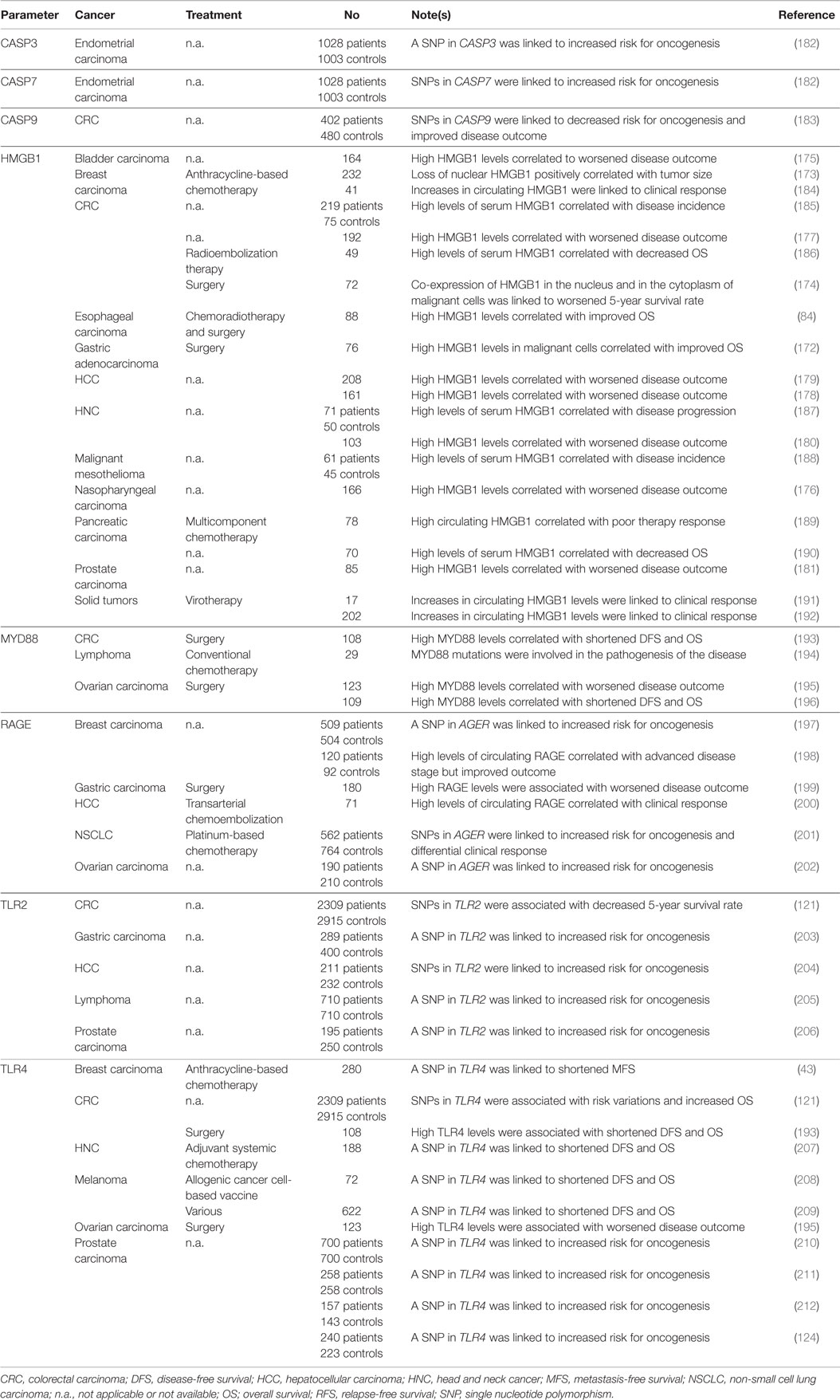
Table 4. Clinical studies assessing the prognostic and predictive value of HMGB1 release and extracellular HMGB1 signaling in cancer patients.
Notably, circulating HMGB1 and RAGE levels have been intensively investigated for their predictive or prognostic value. Elevations of HMGB1 in the serum have been correlated with incidence, progression or unfavorable disease outcome in cohorts of 49 individuals with CRC, or 219 CRC patients and 75 healthy controls (185, 186), 70 individuals with pancreatic adenocarcinoma (190), 71 laryngeal squamous cell carcinoma patients and 50 healthy controls (187), 61 subjects with malignant pleural mesothelioma (188), and 78 pancreatic carcinoma patients (189). Conversely, a treatment-related increase in the circulating levels of HGMB1 has been associated with pathological complete response or partial remission amongst 41 breast carcinoma patients treated with neo-adjuvant chemotherapy based on epirubicin (an ICD inducer) (184), as well as amongst 17 and 202 subjects with chemotherapy-refractory tumors treated with oncolytic virotherapy (191, 192). High levels of RAGE in the serum have been linked to advanced tumor stage but improved clinical outcome amongst 120 patients with breast carcinoma (198). Along similar lines, serum RAGE concentrations were significantly higher in 32 individuals with HCC who favorably responded to transarterial chemoembolization therapy than in 39 patients who progressed upon treatment (200).
Thus, in many (but not all) clinical settings high intratumoral and circulating levels of HMGB1 have a negative prognostic or predictive value. These findings may reflect the ability of some tumors to retain HMGB1 in the course of stress response, the intrinsic resistance of such tumors to the induction of cell death, or the cancer cell-intrinsic functions of HMGB1 (213). In other settings, however, circulating HMGB1 and RAGE levels appear to reflect well the death of cancer cells exposed to immunogenic treatment modalities (184, 191, 192). Possibly, the timing of detection plays a critical role in this setting, calling for the development of optimized monitoring procedures.
SNPs in TLR2, TLR4 and AGER, as well as the circulating levels of a soluble RAGE variant have been shown to affect cancer susceptibility as well as disease outcome in several studies. In particular, TLR2 polymorphisms have been linked to an increased risk for lymphoma (as determined in 710 patients and as many healthy subjects) (205), gastric carcinoma (as assessed in 289 patients and more than 400 controls) (203), prostate carcinoma (as investigated in 195 patients and 250 healthy individuals) (206), HCC (as tested in 211 patients and 232 controls) (204), and CRC (as assessed in 2,309 patients and 2,915 healthy individuals) (121). Loss-of-function variants of TLR4 have been associated with decreased time-to-metastasis amongst 280 women with non-metastatic breast carcinoma treated with surgery followed by anthracycline-based chemotherapy and local irradiation (43), with reduced disease-free and overall survival amongst 188 head and neck cancer patients receiving adjuvant systemic therapy (207), amongst 72 melanoma patients vaccinated with a heat-shocked allogeneic melanoma cell line (208), and amongst 622 melanoma patients subjected to various treatment modalities (209). Along similar lines, SNPs affecting TLR4 or AGER have been linked to an increased risk for prostate cancer (as determined in multiple studies collectively testing more than 1,000 patients and as many age-matched controls) (124, 210–212), ovarian cancer (as assessed in a study testing 190 patients and 210 controls) (202), breast carcinoma (as investigated in 509 patients and 504 healthy women) (197), CRC (as determined in a large cohort encompassing 2,309 patients and 2,915 healthy individuals) (121), and NSCLC (as tested in 562 patients and 764 controls) (201). Notably, this latter study also identified a specific AGER SNP associated with a differential response of NSCLC patients to chemotherapy (201).
Conversely, elevated expression levels of RAGE, TLR4 and/or components of the TLR signaling machinery like myeloid differentiation primary response gene 88 (MYD88) by malignant tissues have been correlated with shortened disease-free and overall survival in 2 cohorts of 109 and 123 ovarian carcinoma patients subjected to surgery (195, 196), in a cohort 108 subjects with CRC (193), and amongst 180 individuals with gastric carcinoma (199). Along similar lines, activating mutations in MYD88 have been linked to the pathogenesis of primary central nervous system lymphomas (194). Most likely, these findings reflect the advantage conferred to malignant cells by the expression of RAGE and TLR4, which can activate robust pro-survival pathways via NF-κB (214).
Finally, distinct SNPs affecting caspase-7 (CASP7) and one affecting caspase-3 (CASP3) have been associated with an altered risk for endometrial carcinoma (as investigated in a cohort of 1,028 patients and 1,003 healthy women) (182), whereas SNPs affecting caspase-9 (CASP9) have been linked to reduced CRC incidence or improved disease outcome (as determined in a cohort of 402 patients and 480 healthy controls) (183). It remains to be determined whether these SNPs truly compromise the ability of cancer cells to emit DAMPs (and hence trigger immunosurveillance mechanisms).
Other DAMPs
The abovementioned molecules and processes may constitute only the tip of an iceberg, meaning that several other DAMPs may contribute to the immunogenicity of cell death, at least in some circumstances. These DAMPs include (but are not limited to) various mitochondrial products like mtDNA, cardiolipin and N-formylated peptides (30) as well as cytosolic proteins like filamentous F-actin (45). Robust preclinical evidence implicates mtDNA in the etiology of septic and non-septic shock as well as in heart failure (29, 215). Cytosolic, extra-cytosolic and extracellular mtDNA molecules have indeed robust pro-inflammatory effects as they trigger type I IFN synthesis via transmembrane protein 173 (TM173, best known as STING) (216) or TLR9 activation (215). In line with this notion, circulating mtDNA levels have been shown to reflect the degree of inflammation and the extent of tissue damage in patients under maintenance hemodialysis (217). Moreover, mtDNA concentrations in the plasma of severe sepsis patients admitted to the emergency room have been ascribed robust predictive value on disease outcome (218). Upon binding to formyl peptide receptor 1 (FPR1), N-formylated peptides reportedly attract neutrophils, stimulate their degranulation, activate monocytes and favor the production of pro-inflammatory cytokines (219–223). Cardiolipin, a lipid that is specifically contained in the inner mitochondrial membrane, binds CD1D on the surface of APC, thus endowing them with the ability of priming CD1D-restricted γδ T cells (224). Finally, F-actin becomes accessible upon disruption of the plasma membrane and promotes the elicitation of adaptive immune responses against dead cell-associated antigens by binding to C-type lectin domain family 9, member A (CLEC9A, best known as DNGR1) on the surface of DCs (45). Studies elucidating the actual contribution of these DAMPs to ICD are urgently awaited.
Concluding Remarks
It is now clear that the emission of DAMPs according to a specific spatiotemporal pattern is an absolute requirement for the elicitation of immune responses against malignant cells succumbing to treatment, and that such responses are necessary for the full-blown efficacy of most (if not all) anticancer therapeutic regimens. In many settings, however, neoplastic cells exposed to conventional chemotherapeutics, radiotherapy or targeted anticancer agents fail to emit DAMPs in a manner compatible with the activation of the immune system, calling for the development of complementation strategies (16). Several approaches are being conceived to address this issue, including the implementation of combinatorial therapeutic regimens including (1) ER stressors, recombinant CALR or recombinant HSPs, to complement for defects in the CALR or HSP exposure pathway; (2) TLR3 agonists or recombinant type I IFNs, to correct problems in the secretion of type I IFN; (3) autophagy inducers or inhibitors of extracellular ATP-degrading enzymes, to maximize the amount of ATP secreted in the course of cell death; and (4) recombinant HMGB1, TLR4 agonists or cytotoxic agents, to restore HMGB1-dependent immunostimulation (225). Besides, consistent efforts are being devoted to the identification of additional strategies that per se induce ICD, in vivo (with direct therapeutic purposes), and in vitro (for instance, for the development of anticancer vaccines) (20). Monitoring DAMPs and DAMP-associated processes may therefore have a dual clinical relevance (Figure 1). First, it may improve patient stratification by allowing for the identification of individuals with different prognosis and/or subjects who are likely to respond (or are responding) to a particular therapeutic regimen. Second, it may instruct therapeutic choices by spotting specific molecular or cellular defects that may be corrected pharmacologically. We surmise that the prognostic and/or predictive value of DAMPs and DAMP-associated processes will have a significant impact on the clinical management of cancer patients.
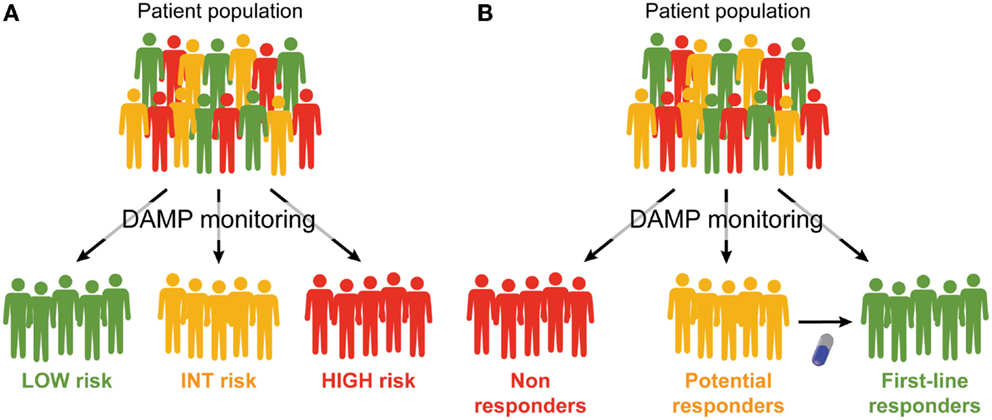
Figure 1. Prognostic and predictive value of DAMPs and DAMP-associated processes. (A,B). Monitoring the emission of damage-associated molecular patterns (DAMPs) or DAMP-associated processes may have a multifaceted impact on the clinical management of cancer patients. First, it may allow for a prognostic assessment and permit the stratification of patients in different risk groups (A). Second, it may allow for the identification of patients who are intrinsically capable or uncapable to respond to a specific treatment, and amongst the latter, those who may benefit from combinatorial therapeutic approaches aimed at restoring normal DAMP signaling (B).
Conflict of Interest Statement
Jitka Fucikova, Irena Moserova, Linda Urbanova, and Radek Spisek are employees of Sotio (Prague, Czech Republic). The other co-authors declare that the research was conducted in the absence of any commercial or financial relationships that could be construed as a potential conflict of interest. The handling editor, Fabrizio Mattei declares that, despite having co-authored a paper with the manuscript’s authors within the past two years, the review process was handled objectively.
Acknowledgments
GK is supported by the Ligue contre le Cancer (équipe labelisée); Agence National de la Recherche (ANR) – Projets blancs; ANR under the frame of E-Rare-2, the ERA-Net for Research on Rare Diseases; Association pour la recherche sur le cancer (ARC); Cancéropôle Ile-de-France; Institut National du Cancer (INCa); Fondation Bettencourt-Schueller; Fondation de France; Fondation pour la Recherche Médicale (FRM); the European Commission (ArtForce); the European Research Council (ERC); the LabEx Immuno-Oncology; the SIRIC Stratified Oncology Cell DNA Repair and Tumor Immune Elimination (SOCRATE); the SIRIC Cancer Research and Personalized Medicine (CARPEM); and the Paris Alliance of Cancer Research Institutes (PACRI). RS is supported by the Ministry of Health of Czech Republic, grant numbers: IGA NT 14533-3 and IGA NT 11 404-5.
Abbreviations
AGER, advanced glycosylation end product-specific receptor; AML, acute myeloid leukemia; APC, antigen-presenting cell; ATF6, activating transcription factor 6; BECN1, beclin 1; C1q, complement component 1, q subcomponent; CALR, calreticulin; CLEC9A, C-type lectin domain family 9, member A; CLL, chronic lymphocytic leukemia; CRC, colorectal carcinoma; CXCL10, chemokine (C-X-C motif) ligand 10; CXCR4, chemokine (C-X-C motif) receptor 4; DAMP, damage-associated molecular pattern; EIF2A, eukaryotic translation initiation factor 2A; EIF2AK2, eukaryotic translation initiation factor 2-alpha kinase 2; ENTPD1, ectonucleoside triphosphate diphosphohydrolase 1; FPR1, formyl peptide receptor 1; HCC, hepatocellular carcinoma; HMGB1, high mobility group box 1; HSP, heat-shock protein; HSP90AA1, heat shock protein 90 kDa alpha (cytosolic), class A member 1; HSPA1A, heat shock 70 kDa protein 1A; HSPA5, heat shock 70 kDa protein 5; HSP90B1, heat shock protein 90 kDa beta (Grp94), member 1; ICD, immunogenic cell death; IFN, interferon; IFNA8, interferon, alpha 8; IFNAR1, interferon (alpha, beta and omega) receptor 1; IL-6, interleukin-6; KLRD1, killer cell lectin-like receptor subfamily D; LMAN1, lectin, mannose-binding, 1; MAP1LC3, microtubule-associated protein 1 light chain 3; MX1, MX dynamin-like GTPase 1; MYD88, myeloid differentiation primary response gene 88; NSCLC, non-small cell lung carcinoma; NK, natural killer; NT5E, ecto 5′-nucleotidase; P2RY2, purinergic receptor P2Y, G-protein coupled, 2; PLSCR1, phospholipid scramblase 1; PS, phosphatidylserine; TAA, tumor-associated antigen; THBS1, thrombospondin 1; TICAM1, Toll-like receptor adaptor molecule 1; TLR, Toll-like receptor; TM173, transmembrane protein 173; TNFα, tumor necrosis factor α; UPR, unfolded protein response; XBP1, X-box binding protein 1.
References
1. Hanahan D, Weinberg RA. The hallmarks of cancer. Cell (2000) 100:57–70. doi:10.1016/S0092-8674(00)81683-9
2. Whiteside TL. The tumor microenvironment and its role in promoting tumor growth. Oncogene (2008) 27:5904–12. doi:10.1038/onc.2008.271
3. Meacham CE, Morrison SJ. Tumour heterogeneity and cancer cell plasticity. Nature (2013) 501:328–37. doi:10.1038/nature12624
4. Sautes-Fridman C, Cherfils-Vicini J, Damotte D, Fisson S, Fridman WH, Cremer I, et al. Tumor microenvironment is multifaceted. Cancer Metastasis Rev (2011) 30:13–25. doi:10.1007/s10555-011-9279-y
5. Schreiber RD, Old LJ, Smyth MJ. Cancer immunoediting: integrating immunity’s roles in cancer suppression and promotion. Science (2011) 331:1565–70. doi:10.1126/science.1203486
6. Mittal D, Gubin MM, Schreiber RD, Smyth MJ. New insights into cancer immunoediting and its three component phases – elimination, equilibrium and escape. Curr Opin Immunol (2014) 27:16–25. doi:10.1016/j.coi.2014.01.004
7. Zitvogel L, Tesniere A, Kroemer G. Cancer despite immunosurveillance: immunoselection and immunosubversion. Nat Rev Immunol (2006) 6:715–27. doi:10.1038/nri1936
8. Senovilla L, Galluzzi L, Zitvogel L, Kroemer G. Immunosurveillance as a regulator of tissue homeostasis. Trends Immunol (2013) 34:471–81. doi:10.1016/j.it.2013.06.005
9. Becht E, Goc J, Germain C, Giraldo NA, Dieu-Nosjean MC, Sautes-Fridman C, et al. Shaping of an effective immune microenvironment to and by cancer cells. Cancer Immunol Immunother (2014) 63:991–7. doi:10.1007/s00262-014-1590-3
10. Fridman WH, Pages F, Sautes-Fridman C, Galon J. The immune contexture in human tumours: impact on clinical outcome. Nat Rev Cancer (2012) 12:298–306. doi:10.1038/nrc3245
11. Senovilla L, Vacchelli E, Galon J, Adjemian S, Eggermont A, Fridman WH, et al. Trial watch: prognostic and predictive value of the immune infiltrate in cancer. Oncoimmunology (2012) 1:1323–43. doi:10.4161/onci.22009
12. Senovilla L, Aranda F, Galluzzi L, Kroemer G. Impact of myeloid cells on the efficacy of anticancer chemotherapy. Curr Opin Immunol (2014) 30:24–31. doi:10.1016/j.coi.2014.05.009
13. Galluzzi L, Senovilla L, Zitvogel L, Kroemer G. The secret ally: immunostimulation by anticancer drugs. Nat Rev Drug Discov (2012) 11:215–33. doi:10.1038/nrd3626
14. Zitvogel L, Galluzzi L, Smyth MJ, Kroemer G. Mechanism of action of conventional and targeted anticancer therapies: reinstating immunosurveillance. Immunity (2013) 39:74–88. doi:10.1016/j.immuni.2013.06.014
15. Galluzzi L, Vacchelli E, Bravo-San Pedro JM, Buque A, Senovilla L, Baracco EE, et al. Classification of current anticancer immunotherapies. Oncotarget (2014) 5:12472–508.
16. Vacchelli E, Prada N, Kepp O, Galluzzi L. Current trends of anticancer immunochemotherapy. Oncoimmunology (2013) 2:e25396. doi:10.4161/onci.25396
17. Fucikova J, Kralikova P, Fialova A, Brtnicky T, Rob L, Bartunkova J, et al. Human tumor cells killed by anthracyclines induce a tumor-specific immune response. Cancer Res (2011) 71:4821–33. doi:10.1158/0008-5472.CAN-11-0950
18. Dudek AM, Garg AD, Krysko DV, De Ruysscher D, Agostinis P. Inducers of immunogenic cancer cell death. Cytokine Growth Factor Rev (2013) 24:319–33. doi:10.1016/j.cytogfr.2013.01.005
19. Vacchelli E, Senovilla L, Eggermont A, Fridman WH, Galon J, Zitvogel L, et al. Trial watch: chemotherapy with immunogenic cell death inducers. Oncoimmunology (2013) 2:e23510. doi:10.4161/onci.23510
20. Fucikova J, Moserova I, Truxova I, Hermanova I, Vancurova I, Partlova S, et al. High hydrostatic pressure induces immunogenic cell death in human tumor cells. Int J Cancer (2014) 135:1165–77. doi:10.1002/ijc.28766
21. Vacchelli E, Aranda F, Eggermont A, Galon J, Sautes-Fridman C, Cremer I, et al. Trial watch: chemotherapy with immunogenic cell death inducers. Oncoimmunology (2014) 3:e27878. doi:10.4161/onci.27878
22. Kroemer G, Galluzzi L, Kepp O, Zitvogel L. Immunogenic cell death in cancer therapy. Annu Rev Immunol (2013) 31:51–72. doi:10.1146/annurev-immunol-032712-100008
23. Kepp O, Senovilla L, Vitale I, Vacchelli E, Adjemian S, Agostinis P, et al. Consensus guidelines for the detection of immunogenic cell death. Oncoimmunology (2014) 3:e955691. doi:10.4161/21624011.2014.955691
24. Matzinger P. Tolerance, danger, and the extended family. Annu Rev Immunol (1994) 12:991–1045. doi:10.1146/annurev.iy.12.040194.005015
25. Kepp O, Galluzzi L, Martins I, Schlemmer F, Adjemian S, Michaud M, et al. Molecular determinants of immunogenic cell death elicited by anticancer chemotherapy. Cancer Metastasis Rev (2011) 30:61–9. doi:10.1007/s10555-011-9273-4
26. Krysko DV, Garg AD, Kaczmarek A, Krysko O, Agostinis P, Vandenabeele P. Immunogenic cell death and DAMPs in cancer therapy. Nat Rev Cancer (2012) 12:860–75. doi:10.1038/nrc3380
27. Garg AD, Dudek AM, Agostinis P. Cancer immunogenicity, danger signals, and DAMPs: what, when, and how? Biofactors (2013) 39:355–67. doi:10.1002/biof.1125
28. Garg AD, Martin S, Golab J, Agostinis P. Danger signalling during cancer cell death: origins, plasticity and regulation. Cell Death Differ (2014) 21:26–38. doi:10.1038/cdd.2013.48
29. Zhang Q, Raoof M, Chen Y, Sumi Y, Sursal T, Junger W, et al. Circulating mitochondrial DAMPs cause inflammatory responses to injury. Nature (2010) 464:104–7. doi:10.1038/nature08780
30. Galluzzi L, Kepp O, Kroemer G. Mitochondria: master regulators of danger signalling. Nat Rev Mol Cell Biol (2012) 13:780–8. doi:10.1038/nrm3479
31. Korbelik M, Sun J, Cecic I. Photodynamic therapy-induced cell surface expression and release of heat shock proteins: relevance for tumor response. Cancer Res (2005) 65:1018–26.
32. Obeid M, Tesniere A, Ghiringhelli F, Fimia GM, Apetoh L, Perfettini JL, et al. Calreticulin exposure dictates the immunogenicity of cancer cell death. Nat Med (2007) 13:54–61. doi:10.1038/nm1523
33. Spisek R, Charalambous A, Mazumder A, Vesole DH, Jagannath S, Dhodapkar MV. Bortezomib enhances dendritic cell (DC)-mediated induction of immunity to human myeloma via exposure of cell surface heat shock protein 90 on dying tumor cells: therapeutic implications. Blood (2007) 109:4839–45. doi:10.1182/blood-2006-10-054221
34. Panaretakis T, Kepp O, Brockmeier U, Tesniere A, Bjorklund AC, Chapman DC, et al. Mechanisms of pre-apoptotic calreticulin exposure in immunogenic cell death. EMBO J (2009) 28:578–90. doi:10.1038/emboj.2009.1
35. Krysko DV, Agostinis P, Krysko O, Garg AD, Bachert C, Lambrecht BN, et al. Emerging role of damage-associated molecular patterns derived from mitochondria in inflammation. Trends Immunol (2011) 32:157–64. doi:10.1016/j.it.2011.01.005
36. Garg AD, Krysko DV, Verfaillie T, Kaczmarek A, Ferreira GB, Marysael T, et al. A novel pathway combining calreticulin exposure and ATP secretion in immunogenic cancer cell death. EMBO J (2012) 31:1062–79. doi:10.1038/emboj.2011.497
37. Galluzzi L, Vacchelli E, Eggermont A, Fridman WH, Galon J, Sautes-Fridman C, et al. Trial watch: experimental toll-like receptor agonists for cancer therapy. Oncoimmunology (2012) 1:699–716. doi:10.4161/onci.20696
38. Aranda F, Vacchelli E, Obrist F, Eggermont A, Galon J, Sautes-Fridman C, et al. Trial watch: toll-like receptor agonists in oncological indications. Oncoimmunology (2014) 3:e29179. doi:10.4161/onci.29179
39. Sistigu A, Yamazaki T, Vacchelli E, Chaba K, Enot DP, Adam J, et al. Cancer cell-autonomous contribution of type I interferon signaling to the efficacy of chemotherapy. Nat Med (2014) 20:1301–9. doi:10.1038/nm.3708
40. Zitvogel L, Galluzzi L, Kepp O, Smyth MJ, Kroemer G. Type I interferons in anticancer immunity. Nat Rev Immunol (2015) 15(7):405–14. doi:10.1038/nri3845
41. Ghiringhelli F, Apetoh L, Tesniere A, Aymeric L, Ma Y, Ortiz C, et al. Activation of the NLRP3 inflammasome in dendritic cells induces IL-1beta-dependent adaptive immunity against tumors. Nat Med (2009) 15:1170–8. doi:10.1038/nm.2028
42. Michaud M, Martins I, Sukkurwala AQ, Adjemian S, Ma Y, Pellegatti P, et al. Autophagy-dependent anticancer immune responses induced by chemotherapeutic agents in mice. Science (2011) 334:1573–7. doi:10.1126/science.1208347
43. Apetoh L, Ghiringhelli F, Tesniere A, Obeid M, Ortiz C, Criollo A, et al. Toll-like receptor 4-dependent contribution of the immune system to anticancer chemotherapy and radiotherapy. Nat Med (2007) 13:1050–9. doi:10.1038/nm1622
44. Brenner C, Galluzzi L, Kepp O, Kroemer G. Decoding cell death signals in liver inflammation. J Hepatol (2013) 59:583–94. doi:10.1016/j.jhep.2013.03.033
45. Ahrens S, Zelenay S, Sancho D, Hanc P, Kjaer S, Feest C, et al. F-actin is an evolutionarily conserved damage-associated molecular pattern recognized by DNGR-1, a receptor for dead cells. Immunity (2012) 36:635–45. doi:10.1016/j.immuni.2012.03.008
46. Tufi R, Panaretakis T, Bianchi K, Criollo A, Fazi B, Di Sano F, et al. Reduction of endoplasmic reticulum Ca2+ levels favors plasma membrane surface exposure of calreticulin. Cell Death Differ (2008) 15:274–82. doi:10.1038/sj.cdd.4402275
47. Hetz C. The unfolded protein response: controlling cell fate decisions under ER stress and beyond. Nat Rev Mol Cell Biol (2012) 13:89–102. doi:10.1038/nrm3270
48. Kepp O, Semeraro M, Bravo-San Pedro JM, Bloy N, Buque A, Huang X, et al. eIF2alpha phosphorylation as a biomarker of immunogenic cell death. Semin Cancer Biol (2015). doi:10.1016/j.semcancer.2015.02.004
49. Binder RJ, Blachere NE, Srivastava PK. Heat shock protein-chaperoned peptides but not free peptides introduced into the cytosol are presented efficiently by major histocompatibility complex I molecules. J Biol Chem (2001) 276:17163–71. doi:10.1074/jbc.M011547200
50. Doody AD, Kovalchin JT, Mihalyo MA, Hagymasi AT, Drake CG, Adler AJ. Glycoprotein 96 can chaperone both MHC class I- and class II-restricted epitopes for in vivo presentation, but selectively primes CD8+ T cell effector function. J Immunol (2004) 172:6087–92. doi:10.4049/jimmunol.172.10.6087
51. Spisek R, Dhodapkar MV. Towards a better way to die with chemotherapy: role of heat shock protein exposure on dying tumor cells. Cell Cycle (2007) 6:1962–5. doi:10.4161/cc.6.16.4601
52. Basu S, Binder RJ, Suto R, Anderson KM, Srivastava PK. Necrotic but not apoptotic cell death releases heat shock proteins, which deliver a partial maturation signal to dendritic cells and activate the NF-kappa B pathway. Int Immunol (2000) 12:1539–46. doi:10.1093/intimm/12.11.1539
53. Duo CC, Gong FY, He XY, Li YM, Wang J, Zhang JP, et al. Soluble calreticulin induces tumor necrosis factor-alpha (TNF-alpha) and interleukin (IL)-6 production by macrophages through mitogen-activated protein kinase (MAPK) and NFkappaB signaling pathways. Int J Mol Sci (2014) 15:2916–28. doi:10.3390/ijms15022916
54. Multhoff G, Botzler C, Wiesnet M, Eissner G, Issels R. CD3- large granular lymphocytes recognize a heat-inducible immunogenic determinant associated with the 72-kD heat shock protein on human sarcoma cells. Blood (1995) 86:1374–82.
55. Multhoff G, Botzler C, Jennen L, Schmidt J, Ellwart J, Issels R. Heat shock protein 72 on tumor cells: a recognition structure for natural killer cells. J Immunol (1997) 158:4341–50.
56. Segawa K, Kurata S, Yanagihashi Y, Brummelkamp TR, Matsuda F, Nagata S. Caspase-mediated cleavage of phospholipid flippase for apoptotic phosphatidylserine exposure. Science (2014) 344:1164–8. doi:10.1126/science.1252809
57. Gold LI, Eggleton P, Sweetwyne MT, Van Duyn LB, Greives MR, Naylor SM, et al. Calreticulin: non-endoplasmic reticulum functions in physiology and disease. FASEB J (2010) 24:665–83. doi:10.1096/fj.09-145482
58. Zeng G, Aldridge ME, Tian X, Seiler D, Zhang X, Jin Y, et al. Dendritic cell surface calreticulin is a receptor for NY-ESO-1: direct interactions between tumor-associated antigen and the innate immune system. J Immunol (2006) 177:3582–9. doi:10.4049/jimmunol.177.6.3582
59. Hsu WM, Hsieh FJ, Jeng YM, Kuo ML, Chen CN, Lai DM, et al. Calreticulin expression in neuroblastoma – a novel independent prognostic factor. Ann Oncol (2005) 16:314–21. doi:10.1093/annonc/mdi062
60. Garg AD, Elsen S, Krysko DV, Vandenabeele P, De Witte P, Agostinis P. Resistance to anticancer vaccination effect is controlled by a cancer cell-autonomous phenotype that disrupts immunogenic phagocytic removal. Oncotarget (2015).
61. Peng RQ, Chen YB, Ding Y, Zhang R, Zhang X, Yu XJ, et al. Expression of calreticulin is associated with infiltration of T-cells in stage IIIB colon cancer. World J Gastroenterol (2010) 16:2428–34. doi:10.3748/wjg.v16.i19.2428
62. Zappasodi R, Pupa SM, Ghedini GC, Bongarzone I, Magni M, Cabras AD, et al. Improved clinical outcome in indolent B-cell lymphoma patients vaccinated with autologous tumor cells experiencing immunogenic death. Cancer Res (2010) 70:9062–72. doi:10.1158/0008-5472.CAN-10-1825
63. Wemeau M, Kepp O, Tesniere A, Panaretakis T, Flament C, De Botton S, et al. Calreticulin exposure on malignant blasts predicts a cellular anticancer immune response in patients with acute myeloid leukemia. Cell Death Dis (2010) 1:e104. doi:10.1038/cddis.2010.82
64. Liu R, Gong J, Chen J, Li Q, Song C, Zhang J, et al. Calreticulin as a potential diagnostic biomarker for lung cancer. Cancer Immunol Immunother (2012) 61:855–64. doi:10.1007/s00262-011-1146-8
65. Chen JS, Hsu YM, Chen CC, Chen LL, Lee CC, Huang TS. Secreted heat shock protein 90alpha induces colorectal cancer cell invasion through CD91/LRP-1 and NF-kappaB-mediated integrin alphaV expression. J Biol Chem (2010) 285:25458–66. doi:10.1074/jbc.M110.139345
66. Chen WS, Chen CC, Chen LL, Lee CC, Huang TS. Secreted heat shock protein 90alpha (HSP90alpha) induces nuclear factor-kappaB-mediated TCF12 protein expression to down-regulate E-cadherin and to enhance colorectal cancer cell migration and invasion. J Biol Chem (2013) 288:9001–10. doi:10.1074/jbc.M112.437897
67. Chao MP, Jaiswal S, Weissman-Tsukamoto R, Alizadeh AA, Gentles AJ, Volkmer J, et al. Calreticulin is the dominant pro-phagocytic signal on multiple human cancers and is counterbalanced by CD47. Sci Transl Med (2010) 2:63ra94. doi:10.1126/scitranslmed.3001375
68. Eric A, Juranic Z, Milovanovic Z, Markovic I, Inic M, Stanojevic-Bakic N, et al. Effects of humoral immunity and calreticulin overexpression on postoperative course in breast cancer. Pathol Oncol Res (2009) 15:89–90. doi:10.1007/s12253-008-9112-2
69. Chen CN, Chang CC, Su TE, Hsu WM, Jeng YM, Ho MC, et al. Identification of calreticulin as a prognosis marker and angiogenic regulator in human gastric cancer. Ann Surg Oncol (2009) 16:524–33. doi:10.1245/s10434-008-0243-1
70. Majeti R, Chao MP, Alizadeh AA, Pang WW, Jaiswal S, Gibbs KD Jr, et al. CD47 is an adverse prognostic factor and therapeutic antibody target on human acute myeloid leukemia stem cells. Cell (2009) 138:286–99. doi:10.1016/j.cell.2009.05.045
71. Suzuki S, Yokobori T, Tanaka N, Sakai M, Sano A, Inose T, et al. CD47 expression regulated by the miR-133a tumor suppressor is a novel prognostic marker in esophageal squamous cell carcinoma. Oncol Rep (2012) 28:465–72. doi:10.3892/or.2012.1831
72. Wang H, Tan M, Zhang S, Li X, Gao J, Zhang D, et al. Expression and significance of CD44, CD47 and c-met in ovarian clear cell carcinoma. Int J Mol Sci (2015) 16:3391–404. doi:10.3390/ijms16023391
73. Stebbing J, Bower M, Gazzard B, Wildfire A, Pandha H, Dalgleish A, et al. The common heat shock protein receptor CD91 is up-regulated on monocytes of advanced melanoma slow progressors. Clin Exp Immunol (2004) 138:312–6. doi:10.1111/j.1365-2249.2004.02619.x
74. Schardt JA, Weber D, Eyholzer M, Mueller BU, Pabst T. Activation of the unfolded protein response is associated with favorable prognosis in acute myeloid leukemia. Clin Cancer Res (2009) 15:3834–41. doi:10.1158/1078-0432.CCR-08-2870
75. Senovilla L, Vitale I, Martins I, Tailler M, Pailleret C, Michaud M, et al. An immunosurveillance mechanism controls cancer cell ploidy. Science (2012) 337:1678–84. doi:10.1126/science.1224922
76. Davies MP, Barraclough DL, Stewart C, Joyce KA, Eccles RM, Barraclough R, et al. Expression and splicing of the unfolded protein response gene XBP-1 are significantly associated with clinical outcome of endocrine-treated breast cancer. Int J Cancer (2008) 123:85–8. doi:10.1002/ijc.23479
77. Mozos A, Roue G, Lopez-Guillermo A, Jares P, Campo E, Colomer D, et al. The expression of the endoplasmic reticulum stress sensor BiP/GRP78 predicts response to chemotherapy and determines the efficacy of proteasome inhibitors in diffuse large b-cell lymphoma. Am J Pathol (2011) 179:2601–10. doi:10.1016/j.ajpath.2011.07.031
78. Jiang L, Huang S, Li W, Zhang D, Zhang S, Zhang W, et al. Expression of autophagy and ER stress-related proteins in primary salivary adenoid cystic carcinoma. Pathol Res Pract (2012) 208:635–41. doi:10.1016/j.prp.2012.07.008
79. Uramoto H, Sugio K, Oyama T, Nakata S, Ono K, Yoshimastu T, et al. Expression of endoplasmic reticulum molecular chaperone Grp78 in human lung cancer and its clinical significance. Lung Cancer (2005) 49:55–62. doi:10.1016/j.lungcan.2004.12.011
80. He Y, Correa AM, Raso MG, Hofstetter WL, Fang B, Behrens C, et al. The role of PKR/eIF2alpha signaling pathway in prognosis of non-small cell lung cancer. PLoS One (2011) 6:e24855. doi:10.1371/journal.pone.0024855
81. Ferrer-Ferrer M, Malespin-Bendana W, Ramirez V, Gonzalez MI, Carvajal A, Une C. Polymorphisms in genes coding for HSP-70 are associated with gastric cancer and duodenal ulcer in a population at high risk of gastric cancer in Costa Rica. Arch Med Res (2013) 44:467–74. doi:10.1016/j.arcmed.2013.08.008
82. Nevadunsky NS, Korneeva I, Caputo T, Witkin SS. Mannose-binding lectin codon 54 genetic polymorphism and vaginal protein levels in women with gynecologic malignancies. Eur J Obstet Gynecol Reprod Biol (2012) 163:216–8. doi:10.1016/j.ejogrb.2012.04.020
83. Hong B, Chen S, Qi Y, Zhu J, Lin J. Association of THBS1 rs1478605 T>C in 5’-untranslated regions with the development and progression of gastric cancer. Biomed Rep (2015) 3:207–14. doi:10.3892/br.2015.414
84. Suzuki Y, Mimura K, Yoshimoto Y, Watanabe M, Ohkubo Y, Izawa S, et al. Immunogenic tumor cell death induced by chemoradiotherapy in patients with esophageal squamous cell carcinoma. Cancer Res (2012) 72:3967–76. doi:10.1158/0008-5472.CAN-12-0851
85. Galluzzi L, Kepp O, Vander Heiden MG, Kroemer G. Metabolic targets for cancer therapy. Nat Rev Drug Discov (2013) 12:829–46. doi:10.1038/nrd4145
86. Schardt JA, Mueller BU, Pabst T. Activation of the unfolded protein response in human acute myeloid leukemia. Methods Enzymol (2011) 489:227–43. doi:10.1016/B978-0-12-385116-1.00013-3
87. Chen X, Iliopoulos D, Zhang Q, Tang Q, Greenblatt MB, Hatziapostolou M, et al. XBP1 promotes triple-negative breast cancer by controlling the HIF1alpha pathway. Nature (2014) 508:103–7. doi:10.1038/nature13119
88. Vacchelli E, Martins I, Eggermont A, Fridman WH, Galon J, Sautes-Fridman C, et al. Trial watch: peptide vaccines in cancer therapy. Oncoimmunology (2012) 1:1557–76. doi:10.4161/onci.22428
89. Aranda F, Vacchelli E, Eggermont A, Galon J, Sautes-Fridman C, Tartour E, et al. Trial watch: peptide vaccines in cancer therapy. Oncoimmunology (2013) 2:e26621. doi:10.4161/onci.26621
90. Wood CG, Mulders P. Vitespen: a preclinical and clinical review. Future Oncol (2009) 5:763–74. doi:10.2217/fon.09.46
91. Belli F, Testori A, Rivoltini L, Maio M, Andreola G, Sertoli MR, et al. Vaccination of metastatic melanoma patients with autologous tumor-derived heat shock protein gp96-peptide complexes: clinical and immunologic findings. J Clin Oncol (2002) 20:4169–80. doi:10.1200/JCO.2002.09.134
92. Pilla L, Patuzzo R, Rivoltini L, Maio M, Pennacchioli E, Lamaj E, et al. A phase II trial of vaccination with autologous, tumor-derived heat-shock protein peptide complexes Gp96, in combination with GM-CSF and interferon-alpha in metastatic melanoma patients. Cancer Immunol Immunother (2006) 55:958–68. doi:10.1007/s00262-005-0084-8
93. Testori A, Richards J, Whitman E, Mann GB, Lutzky J, Camacho L, et al. Phase III comparison of vitespen, an autologous tumor-derived heat shock protein gp96 peptide complex vaccine, with physician’s choice of treatment for stage IV melanoma: the C-100-21 study group. J Clin Oncol (2008) 26:955–62. doi:10.1200/JCO.2007.11.9941
94. Eton O, Ross MI, East MJ, Mansfield PF, Papadopoulos N, Ellerhorst JA, et al. Autologous tumor-derived heat-shock protein peptide complex-96 (HSPPC-96) in patients with metastatic melanoma. J Transl Med (2010) 8:9. doi:10.1186/1479-5876-8-9
95. Mazzaferro V, Coppa J, Carrabba MG, Rivoltini L, Schiavo M, Regalia E, et al. Vaccination with autologous tumor-derived heat-shock protein gp96 after liver resection for metastatic colorectal cancer. Clin Cancer Res (2003) 9:3235–45.
96. Oki Y, McLaughlin P, Fayad LE, Pro B, Mansfield PF, Clayman GL, et al. Experience with heat shock protein-peptide complex 96 vaccine therapy in patients with indolent non-Hodgkin lymphoma. Cancer (2007) 109:77–83. doi:10.1002/cncr.22389
97. Maki RG, Livingston PO, Lewis JJ, Janetzki S, Klimstra D, Desantis D, et al. A phase I pilot study of autologous heat shock protein vaccine HSPPC-96 in patients with resected pancreatic adenocarcinoma. Dig Dis Sci (2007) 52:1964–72. doi:10.1007/s10620-006-9205-2
98. Jonasch E, Wood C, Tamboli P, Pagliaro LC, Tu SM, Kim J, et al. Vaccination of metastatic renal cell carcinoma patients with autologous tumour-derived vitespen vaccine: clinical findings. Br J Cancer (2008) 98:1336–41. doi:10.1038/sj.bjc.6604266
99. Wood C, Srivastava P, Bukowski R, Lacombe L, Gorelov AI, Gorelov S, et al. An adjuvant autologous therapeutic vaccine (HSPPC-96; vitespen) versus observation alone for patients at high risk of recurrence after nephrectomy for renal cell carcinoma: a multicentre, open-label, randomised phase III trial. Lancet (2008) 372:145–54. doi:10.1016/S0140-6736(08)60697-2
100. Crane CA, Han SJ, Ahn B, Oehlke J, Kivett V, Fedoroff A, et al. Individual patient-specific immunity against high-grade glioma after vaccination with autologous tumor derived peptides bound to the 96 KD chaperone protein. Clin Cancer Res (2013) 19:205–14. doi:10.1158/1078-0432.CCR-11-3358
101. Bloch O, Crane CA, Fuks Y, Kaur R, Aghi MK, Berger MS, et al. Heat-shock protein peptide complex-96 vaccination for recurrent glioblastoma: a phase II, single-arm trial. Neuro Oncol (2014) 16:274–9. doi:10.1093/neuonc/not203
102. Janetzki S, Palla D, Rosenhauer V, Lochs H, Lewis JJ, Srivastava PK. Immunization of cancer patients with autologous cancer-derived heat shock protein gp96 preparations: a pilot study. Int J Cancer (2000) 88:232–8. doi:10.1002/1097-0215(20001015)88:2<232::AID-IJC14>3.0.CO;2-8
103. Papewalis C, Jacobs B, Wuttke M, Ullrich E, Baehring T, Fenk R, et al. IFN-alpha skews monocytes into CD56+-expressing dendritic cells with potent functional activities in vitro and in vivo. J Immunol (2008) 180:1462–70. doi:10.4049/jimmunol.180.3.1462
104. Guillot B, Portales P, Thanh AD, Merlet S, Dereure O, Clot J, et al. The expression of cytotoxic mediators is altered in mononuclear cells of patients with melanoma and increased by interferon-alpha treatment. Br J Dermatol (2005) 152:690–6. doi:10.1111/j.1365-2133.2005.06512.x
105. Ilander M, Kreutzman A, Rohon P, Melo T, Faber E, Porkka K, et al. Enlarged memory T-cell pool and enhanced Th1-type responses in chronic myeloid leukemia patients who have successfully discontinued IFN-alpha monotherapy. PLoS One (2014) 9:e87794. doi:10.1371/journal.pone.0087794
106. Crouse J, Bedenikovic G, Wiesel M, Ibberson M, Xenarios I, Von Laer D, et al. Type I interferons protect T cells against NK cell attack mediated by the activating receptor NCR1. Immunity (2014) 40:961–73. doi:10.1016/j.immuni.2014.05.003
107. Xu HC, Grusdat M, Pandyra AA, Polz R, Huang J, Sharma P, et al. Type I interferon protects antiviral CD8+ T cells from NK cell cytotoxicity. Immunity (2014) 40:949–60. doi:10.1016/j.immuni.2014.05.004
108. Novikov A, Cardone M, Thompson R, Shenderov K, Kirschman KD, Mayer-Barber KD, et al. Mycobacterium tuberculosis triggers host type I IFN signaling to regulate IL-1beta production in human macrophages. J Immunol (2011) 187:2540–7. doi:10.4049/jimmunol.1100926
109. Bacher N, Raker V, Hofmann C, Graulich E, Schwenk M, Baumgrass R, et al. Interferon-alpha suppresses cAMP to disarm human regulatory T cells. Cancer Res (2013) 73:5647–56. doi:10.1158/0008-5472.CAN-12-3788
110. Drobits B, Holcmann M, Amberg N, Swiecki M, Grundtner R, Hammer M, et al. Imiquimod clears tumors in mice independent of adaptive immunity by converting pDCs into tumor-killing effector cells. J Clin Invest (2012) 122:575–85. doi:10.1172/JCI61034
111. Chew V, Tow C, Huang C, Bard-Chapeau E, Copeland NG, Jenkins NA, et al. Toll-like receptor 3 expressing tumor parenchyma and infiltrating natural killer cells in hepatocellular carcinoma patients. J Natl Cancer Inst (2012) 104:1796–807. doi:10.1093/jnci/djs436
112. Yuan MM, Xu YY, Chen L, Li XY, Qin J, Shen Y. TLR3 expression correlates with apoptosis, proliferation and angiogenesis in hepatocellular carcinoma and predicts prognosis. BMC Cancer (2015) 15:245. doi:10.1186/s12885-015-1262-5
113. Hsu WM, Huang CC, Wu PY, Lee H, Huang MC, Tai MH, et al. Toll-like receptor 3 expression inhibits cell invasion and migration and predicts a favorable prognosis in neuroblastoma. Cancer Lett (2013) 336:338–46. doi:10.1016/j.canlet.2013.03.024
114. Salaun B, Zitvogel L, Asselin-Paturel C, Morel Y, Chemin K, Dubois C, et al. TLR3 as a biomarker for the therapeutic efficacy of double-stranded RNA in breast cancer. Cancer Res (2011) 71:1607–14. doi:10.1158/0008-5472.CAN-10-3490
115. Castro FA, Forsti A, Buch S, Kalthoff H, Krauss C, Bauer M, et al. TLR-3 polymorphism is an independent prognostic marker for stage II colorectal cancer. Eur J Cancer (2011) 47:1203–10. doi:10.1016/j.ejca.2010.12.011
116. Dai J, Hu Z, Dong J, Xu L, Pan S, Jiang Y, et al. Host immune gene polymorphisms were associated with the prognosis of non-small-cell lung cancer in Chinese. Int J Cancer (2012) 130:671–6. doi:10.1002/ijc.26067
117. Zidi S, Verdi H, Yilmaz-Yalcin Y, Yazici AC, Gazouani E, Mezlini A, et al. Impact of toll-like receptors 2/3/4/9, IL-1-alpha/beta and TNF-alpha polymorphisms in cervical cancer susceptibility in Tunisia : genetic polymorphisms implicated in the occurence of cervical cancer. Pathol Oncol Res (2014). doi:10.1007/s12253-014-9793-7
118. Yeyeodu ST, Kidd LR, Oprea-Ilies GM, Burns BG, Vancleave TT, Shim JY, et al. IRAK4 and TLR3 sequence variants may alter breast cancer risk among African-American women. Front Immunol (2013) 4:338. doi:10.3389/fimmu.2013.00338
119. Zeljic K, Supic G, Jovic N, Kozomara R, Brankovic-Magic M, Obrenovic M, et al. Association of TLR2, TLR3, TLR4 and CD14 genes polymorphisms with oral cancer risk and survival. Oral Dis (2014) 20:416–24. doi:10.1111/odi.12144
120. Li G, Zheng Z. Toll-like receptor 3 genetic variants and susceptibility to hepatocellular carcinoma and HBV-related hepatocellular carcinoma. Tumour Biol (2013) 34:1589–94. doi:10.1007/s13277-013-0689-z
121. Slattery ML, Herrick JS, Bondurant KL, Wolff RK. Toll-like receptor genes and their association with colon and rectal cancer development and prognosis. Int J Cancer (2012) 130:2974–80. doi:10.1002/ijc.26314
122. Lu S, Pardini B, Cheng B, Naccarati A, Huhn S, Vymetalkova V, et al. Single nucleotide polymorphisms within interferon signaling pathway genes are associated with colorectal cancer susceptibility and survival. PLoS One (2014) 9:e111061. doi:10.1371/journal.pone.0111061
123. Fujita M, Scheurer ME, Decker SA, McDonald HA, Kohanbash G, Kastenhuber ER, et al. Role of type 1 IFNs in antiglioma immunosurveillance – using mouse studies to guide examination of novel prognostic markers in humans. Clin Cancer Res (2010) 16:3409–19. doi:10.1158/1078-0432.CCR-10-0644
124. Kim HJ, Bae JS, Chang IH, Kim KD, Lee J, Shin HD, et al. Sequence variants of toll-like receptor 4 (TLR4) and the risk of prostate cancer in Korean men. World J Urol (2012) 30:225–32. doi:10.1007/s00345-011-0690-3
125. Vacchelli E, Eggermont A, Fridman WH, Galon J, Zitvogel L, Kroemer G, et al. Trial watch: immunostimulatory cytokines. Oncoimmunology (2013) 2:e24850. doi:10.4161/onci.24850
126. Vacchelli E, Aranda F, Obrist F, Eggermont A, Galon J, Cremer I, et al. Trial watch: immunostimulatory cytokines in cancer therapy. Oncoimmunology (2014) 3:e29030. doi:10.4161/onci.29030
127. Martins I, Tesniere A, Kepp O, Michaud M, Schlemmer F, Senovilla L, et al. Chemotherapy induces ATP release from tumor cells. Cell Cycle (2009) 8:3723–8. doi:10.4161/cc.8.22.10026
128. Martins I, Wang Y, Michaud M, Ma Y, Sukkurwala AQ, Shen S, et al. Molecular mechanisms of ATP secretion during immunogenic cell death. Cell Death Differ (2014) 21:79–91. doi:10.1038/cdd.2013.75
129. Michaud M, Sukkurwala AQ, Martins I, Shen S, Zitvogel L, Kroemer G. Subversion of the chemotherapy-induced anticancer immune response by the ecto-ATPase CD39. Oncoimmunology (2012) 1:393–5. doi:10.4161/onci.19070
130. Galluzzi L, Pietrocola F, Bravo-San Pedro JM, Amaravadi RK, Baehrecke EH, Cecconi F, et al. Autophagy in malignant transformation and cancer progression. EMBO J (2015) 34:856–80. doi:10.15252/embj.201490784
131. Bastid J, Regairaz A, Bonnefoy N, Dejou C, Giustiniani J, Laheurte C, et al. Inhibition of CD39 enzymatic function at the surface of tumor cells alleviates their immunosuppressive activity. Cancer Immunol Res (2015) 3:254–65. doi:10.1158/2326-6066.CIR-14-0018
132. Elliott MR, Chekeni FB, Trampont PC, Lazarowski ER, Kadl A, Walk SF, et al. Nucleotides released by apoptotic cells act as a find-me signal to promote phagocytic clearance. Nature (2009) 461:282–6. doi:10.1038/nature08296
133. Ma Y, Adjemian S, Mattarollo SR, Yamazaki T, Aymeric L, Yang H, et al. Anticancer chemotherapy-induced intratumoral recruitment and differentiation of antigen-presenting cells. Immunity (2013) 38:729–41. doi:10.1016/j.immuni.2013.03.003
134. Ma Y, Adjemian S, Yang H, Catani JP, Hannani D, Martins I, et al. ATP-dependent recruitment, survival and differentiation of dendritic cell precursors in the tumor bed after anticancer chemotherapy. Oncoimmunology (2013) 2:e24568. doi:10.4161/onci.24568
135. Aymeric L, Apetoh L, Ghiringhelli F, Tesniere A, Martins I, Kroemer G, et al. Tumor cell death and ATP release prime dendritic cells and efficient anticancer immunity. Cancer Res (2010) 70:855–8. doi:10.1158/0008-5472.CAN-09-3566
136. Zitvogel L, Kepp O, Galluzzi L, Kroemer G. Inflammasomes in carcinogenesis and anticancer immune responses. Nat Immunol (2012) 13:343–51. doi:10.1038/ni.2224
137. Beavis PA, Stagg J, Darcy PK, Smyth MJ. CD73: a potent suppressor of antitumor immune responses. Trends Immunol (2012) 33:231–7. doi:10.1016/j.it.2012.02.009
138. Dardano A, Falzoni S, Caraccio N, Polini A, Tognini S, Solini A, et al. 1513A>C polymorphism in the P2X7 receptor gene in patients with papillary thyroid cancer: correlation with histological variants and clinical parameters. J Clin Endocrinol Metab (2009) 94:695–8. doi:10.1210/jc.2008-1322
139. Wiley JS, Dao-Ung LP, Gu BJ, Sluyter R, Shemon AN, Li C, et al. A loss-of-function polymorphic mutation in the cytolytic P2X7 receptor gene and chronic lymphocytic leukaemia: a molecular study. Lancet (2002) 359:1114–9. doi:10.1016/S0140-6736(02)08156-4
140. Thunberg U, Tobin G, Johnson A, Soderberg O, Padyukov L, Hultdin M, et al. Polymorphism in the P2X7 receptor gene and survival in chronic lymphocytic leukaemia. Lancet (2002) 360:1935–9. doi:10.1016/S0140-6736(02)11917-9
141. Zhang LY, Ibbotson RE, Orchard JA, Gardiner AC, Seear RV, Chase AJ, et al. P2X7 polymorphism and chronic lymphocytic leukaemia: lack of correlation with incidence, survival and abnormalities of chromosome 12. Leukemia (2003) 17:2097–100. doi:10.1038/sj.leu.2403125
142. Starczynski J, Pepper C, Pratt G, Hooper L, Thomas A, Hoy T, et al. The P2X7 receptor gene polymorphism 1513 A – >C has no effect on clinical prognostic markers, in vitro sensitivity to fludarabine, Bcl-2 family protein expression or survival in B-cell chronic lymphocytic leukaemia. Br J Haematol (2003) 123:66–71. doi:10.1046/j.1365-2141.2003.04563.x
143. Nuckel H, Frey UH, Durig J, Duhrsen U, Siffert W. 1513A/C polymorphism in the P2X7 receptor gene in chronic lymphocytic leukemia: absence of correlation with clinical outcome. Eur J Haematol (2004) 72:259–63. doi:10.1111/j.0902-4441.2003.00210.x
144. Paneesha S, Starczynski J, Pepper C, Delgado J, Hooper L, Fegan C, et al. The P2X7 receptor gene polymorphism 1513 A – >C has no effect on clinical prognostic markers and survival in multiple myeloma. Leuk Lymphoma (2006) 47:281–4. doi:10.1080/10428190500305901
145. Galluzzi L, Bravo-San Pedro JM, Vitale I, Aaronson SA, Abrams JM, Adam D, et al. Essential versus accessory aspects of cell death: recommendations of the NCCD 2015. Cell Death Differ (2015) 22:58–73. doi:10.1038/cdd.2014.137
146. Aquea G, Bresky G, Lancellotti D, Madariaga JA, Zaffiri V, Urzua U, et al. Increased expression of P2RY2, CD248 and EphB1 in gastric cancers from Chilean patients. Asian Pac J Cancer Prev (2014) 15:1931–6. doi:10.7314/APJCP.2014.15.5.1931
147. Tang H, Sebti S, Titone R, Zhou Y, Isidoro C, Ross TS, et al. Decreased mRNA expression in human breast cancer is associated with estrogen receptor-negative subtypes and poor prognosis. EBioMedicine (2015) 2:255–63. doi:10.1016/j.ebiom.2015.01.008
148. Lee YJ, Hah YJ, Kang YN, Kang KJ, Hwang JS, Chung WJ, et al. The autophagy-related marker LC3 can predict prognosis in human hepatocellular carcinoma. PLoS One (2013) 8:e81540. doi:10.1371/journal.pone.0081540
149. Ko YH, Cho YS, Won HS, Jeon EK, An HJ, Hong SU, et al. Prognostic significance of autophagy-related protein expression in resected pancreatic ductal adenocarcinoma. Pancreas (2013) 42:829–35. doi:10.1097/MPA.0b013e318279d0dc
150. Pulte D, Furman RR, Broekman MJ, Drosopoulos JH, Ballard HS, Olson KE, et al. CD39 expression on T lymphocytes correlates with severity of disease in patients with chronic lymphocytic leukemia. Clin Lymphoma Myeloma Leuk (2011) 11:367–72. doi:10.1016/j.clml.2011.06.005
151. Perry C, Hazan-Halevy I, Kay S, Cipok M, Grisaru D, Deutsch V, et al. Increased CD39 expression on CD4(+) T lymphocytes has clinical and prognostic significance in chronic lymphocytic leukemia. Ann Hematol (2012) 91:1271–9. doi:10.1007/s00277-012-1425-2
152. Aliagas E, Vidal A, Texido L, Ponce J, Condom E, Martin-Satue M. High expression of ecto-nucleotidases CD39 and CD73 in human endometrial tumors. Mediators Inflamm (2014) 2014:509027. doi:10.1155/2014/509027
153. Kunzli BM, Berberat PO, Giese T, Csizmadia E, Kaczmarek E, Baker C, et al. Upregulation of CD39/NTPDases and P2 receptors in human pancreatic disease. Am J Physiol Gastrointest Liver Physiol (2007) 292:G223–30. doi:10.1152/ajpgi.00259.2006
154. Xu S, Shao QQ, Sun JT, Yang N, Xie Q, Wang DH, et al. Synergy between the ectoenzymes CD39 and CD73 contributes to adenosinergic immunosuppression in human malignant gliomas. Neuro Oncol (2013) 15:1160–72. doi:10.1093/neuonc/not067
155. Klionsky DJ, Abdalla FC, Abeliovich H, Abraham RT, Acevedo-Arozena A, Adeli K, et al. Guidelines for the use and interpretation of assays for monitoring autophagy. Autophagy (2012) 8:445–544. doi:10.4161/auto.19496
156. Ma Y, Galluzzi L, Zitvogel L, Kroemer G. Autophagy and cellular immune responses. Immunity (2013) 39:211–27. doi:10.1016/j.immuni.2013.07.017
157. Ko A, Kanehisa A, Martins I, Senovilla L, Chargari C, Dugue D, et al. Autophagy inhibition radiosensitizes in vitro, yet reduces radioresponses in vivo due to deficient immunogenic signalling. Cell Death Differ (2014) 21:92–9. doi:10.1038/cdd.2013.124
158. Ladoire S, Chaba K, Martins I, Sukkurwala AQ, Adjemian S, Michaud M, et al. Immunohistochemical detection of cytoplasmic LC3 puncta in human cancer specimens. Autophagy (2012) 8:1175–84. doi:10.4161/auto.20353
159. Scaffidi P, Misteli T, Bianchi ME. Release of chromatin protein HMGB1 by necrotic cells triggers inflammation. Nature (2002) 418:191–5. doi:10.1038/nature00858
160. Martins I, Kepp O, Menger L, Michaud M, Adjemian S, Sukkurwala AQ, et al. Fluorescent biosensors for the detection of HMGB1 release. Methods Mol Biol (2013) 1004:43–56. doi:10.1007/978-1-62703-383-1_4
161. Liu A, Fang H, Dirsch O, Jin H, Dahmen U. Oxidation of HMGB1 causes attenuation of its pro-inflammatory activity and occurs during liver ischemia and reperfusion. PLoS One (2012) 7:e35379. doi:10.1371/journal.pone.0035379
162. Venereau E, Casalgrandi M, Schiraldi M, Antoine DJ, Cattaneo A, De Marchis F, et al. Mutually exclusive redox forms of HMGB1 promote cell recruitment or proinflammatory cytokine release. J Exp Med (2012) 209:1519–28. doi:10.1084/jem.20120189
163. Yu Y, Tang D, Kang R. Oxidative stress-mediated HMGB1 biology. Front Physiol (2015) 6:93. doi:10.3389/fphys.2015.00093
164. Dumitriu IE, Bianchi ME, Bacci M, Manfredi AA, Rovere-Querini P. The secretion of HMGB1 is required for the migration of maturing dendritic cells. J Leukoc Biol (2007) 81:84–91. doi:10.1189/jlb.0306171
165. Schiraldi M, Raucci A, Munoz LM, Livoti E, Celona B, Venereau E, et al. HMGB1 promotes recruitment of inflammatory cells to damaged tissues by forming a complex with CXCL12 and signaling via CXCR4. J Exp Med (2012) 209:551–63. doi:10.1084/jem.20111739
166. Rovere-Querini P, Capobianco A, Scaffidi P, Valentinis B, Catalanotti F, Giazzon M, et al. HMGB1 is an endogenous immune adjuvant released by necrotic cells. EMBO Rep (2004) 5:825–30. doi:10.1038/sj.embor.7400205
167. Apetoh L, Ghiringhelli F, Tesniere A, Criollo A, Ortiz C, Lidereau R, et al. The interaction between HMGB1 and TLR4 dictates the outcome of anticancer chemotherapy and radiotherapy. Immunol Rev (2007) 220:47–59. doi:10.1111/j.1600-065X.2007.00573.x
168. Sims GP, Rowe DC, Rietdijk ST, Herbst R, Coyle AJ. HMGB1 and RAGE in inflammation and cancer. Annu Rev Immunol (2010) 28:367–88. doi:10.1146/annurev.immunol.021908.132603
169. Shiratsuchi A, Watanabe I, Takeuchi O, Akira S, Nakanishi Y. Inhibitory effect of toll-like receptor 4 on fusion between phagosomes and endosomes/lysosomes in macrophages. J Immunol (2004) 172:2039–47. doi:10.4049/jimmunol.172.4.2039
170. Tian J, Avalos AM, Mao SY, Chen B, Senthil K, Wu H, et al. Toll-like receptor 9-dependent activation by DNA-containing immune complexes is mediated by HMGB1 and RAGE. Nat Immunol (2007) 8:487–96. doi:10.1038/ni1457
171. Chiba S, Baghdadi M, Akiba H, Yoshiyama H, Kinoshita I, Dosaka-Akita H, et al. Tumor-infiltrating DCs suppress nucleic acid-mediated innate immune responses through interactions between the receptor TIM-3 and the alarmin HMGB1. Nat Immunol (2012) 13:832–42. doi:10.1038/ni.2376
172. Bao G, Qiao Q, Zhao H, He X. Prognostic value of HMGB1 overexpression in resectable gastric adenocarcinomas. World J Surg Oncol (2010) 8:52. doi:10.1186/1477-7819-8-52
173. Yamazaki T, Hannani D, Poirier-Colame V, Ladoire S, Locher C, Sistigu A, et al. Defective immunogenic cell death of HMGB1-deficient tumors: compensatory therapy with TLR4 agonists. Cell Death Differ (2014) 21:69–78. doi:10.1038/cdd.2013.72
174. Peng RQ, Wu XJ, Ding Y, Li CY, Yu XJ, Zhang X, et al. Co-expression of nuclear and cytoplasmic HMGB1 is inversely associated with infiltration of CD45RO+ T cells and prognosis in patients with stage IIIB colon cancer. BMC Cancer (2010) 10:496. doi:10.1186/1471-2407-10-496
175. Yang GL, Zhang LH, Bo JJ, Huo XJ, Chen HG, Cao M, et al. Increased expression of HMGB1 is associated with poor prognosis in human bladder cancer. J Surg Oncol (2012) 106:57–61. doi:10.1002/jso.23040
176. Wu D, Ding Y, Wang S, Zhang Q, Liu L. Increased expression of high mobility group box 1 (HMGB1) is associated with progression and poor prognosis in human nasopharyngeal carcinoma. J Pathol (2008) 216:167–75. doi:10.1002/path.2391
177. Yao X, Zhao G, Yang H, Hong X, Bie L, Liu G. Overexpression of high-mobility group box 1 correlates with tumor progression and poor prognosis in human colorectal carcinoma. J Cancer Res Clin Oncol (2010) 136:677–84. doi:10.1007/s00432-009-0706-1
178. Liu F, Zhang Y, Peng Z, Gao H, Xu L, Chen M. High expression of high mobility group box 1 (hmgb1) predicts poor prognosis for hepatocellular carcinoma after curative hepatectomy. J Transl Med (2012) 10:135. doi:10.1186/1479-5876-10-135
179. Xiao J, Ding Y, Huang J, Li Q, Liu Y, Ni W, et al. The association of HMGB1 gene with the prognosis of HCC. PLoS One (2014) 9:e89097. doi:10.1371/journal.pone.0089097
180. Liu Y, Xie C, Zhang X, Huang D, Zhou X, Tan P, et al. Elevated expression of HMGB1 in squamous-cell carcinoma of the head and neck and its clinical significance. Eur J Cancer (2010) 46:3007–15. doi:10.1016/j.ejca.2010.07.016
181. Zhao CB, Bao JM, Lu YJ, Zhao T, Zhou XH, Zheng DY, et al. Co-expression of RAGE and HMGB1 is associated with cancer progression and poor patient outcome of prostate cancer. Am J Cancer Res (2014) 4:369–77.
182. Xu HL, Xu WH, Cai Q, Feng M, Long J, Zheng W, et al. Polymorphisms and haplotypes in the caspase-3, caspase-7, and caspase-8 genes and risk for endometrial cancer: a population-based, case-control study in a Chinese population. Cancer Epidemiol Biomarkers Prev (2009) 18:2114–22. doi:10.1158/1055-9965.EPI-09-0152
183. Theodoropoulos GE, Gazouli M, Vaiopoulou A, Leandrou M, Nikouli S, Vassou E, et al. Polymorphisms of caspase 8 and caspase 9 gene and colorectal cancer susceptibility and prognosis. Int J Colorectal Dis (2011) 26:1113–8. doi:10.1007/s00384-011-1217-5
184. Arnold T, Michlmayr A, Baumann S, Burghuber C, Pluschnig U, Bartsch R, et al. Plasma HMGB-1 after the initial dose of epirubicin/docetaxel in cancer. Eur J Clin Invest (2013) 43:286–91. doi:10.1111/eci.12043
185. Lee H, Song M, Shin N, Shin CH, Min BS, Kim HS, et al. Diagnostic significance of serum HMGB1 in colorectal carcinomas. PLoS One (2012) 7:e34318. doi:10.1371/journal.pone.0034318
186. Fahmueller YN, Nagel D, Hoffmann RT, Tatsch K, Jakobs T, Stieber P, et al. Immunogenic cell death biomarkers HMGB1, RAGE, and DNAse indicate response to radioembolization therapy and prognosis in colorectal cancer patients. Int J Cancer (2013) 132:2349–58. doi:10.1002/ijc.27894
187. Qiu G, Li Y, Liu Z, Wang M, Ge J, Bai X. Clinical value of serum HMGB1 in diagnosis and prognosis of laryngeal squamous cell carcinoma. Med Oncol (2014) 31:316. doi:10.1007/s12032-014-0316-x
188. Tabata C, Shibata E, Tabata R, Kanemura S, Mikami K, Nogi Y, et al. Serum HMGB1 as a prognostic marker for malignant pleural mesothelioma. BMC Cancer (2013) 13:205. doi:10.1186/1471-2407-13-205
189. Wittwer C, Boeck S, Heinemann V, Haas M, Stieber P, Nagel D, et al. Circulating nucleosomes and immunogenic cell death markers HMGB1, sRAGE and DNAse in patients with advanced pancreatic cancer undergoing chemotherapy. Int J Cancer (2013) 133:2619–30. doi:10.1002/ijc.28294
190. Chung HW, Lim JB, Jang S, Lee KJ, Park KH, Song SY. Serum high mobility group box-1 is a powerful diagnostic and prognostic biomarker for pancreatic ductal adenocarcinoma. Cancer Sci (2012) 103:1714–21. doi:10.1111/j.1349-7006.2012.02358.x
191. Liikanen I, Ahtiainen L, Hirvinen ML, Bramante S, Cerullo V, Nokisalmi P, et al. Oncolytic adenovirus with temozolomide induces autophagy and antitumor immune responses in cancer patients. Mol Ther (2013) 21:1212–23. doi:10.1038/mt.2013.51
192. Liikanen I, Koski A, Merisalo-Soikkeli M, Hemminki O, Oksanen M, Kairemo K, et al. Serum HMGB1 is a predictive and prognostic biomarker for oncolytic immunotherapy. Oncoimmunology (2015) 4:e989771. doi:10.4161/2162402X.2014.989771
193. Wang EL, Qian ZR, Nakasono M, Tanahashi T, Yoshimoto K, Bando Y, et al. High expression of toll-like receptor 4/myeloid differentiation factor 88 signals correlates with poor prognosis in colorectal cancer. Br J Cancer (2010) 102:908–15. doi:10.1038/sj.bjc.6605558
194. Gonzalez-Aguilar A, Idbaih A, Boisselier B, Habbita N, Rossetto M, Laurenge A, et al. Recurrent mutations of MYD88 and TBL1XR1 in primary central nervous system lymphomas. Clin Cancer Res (2012) 18:5203–11. doi:10.1158/1078-0432.CCR-12-0845
195. Kim KH, Jo MS, Suh DS, Yoon MS, Shin DH, Lee JH, et al. Expression and significance of the TLR4/MyD88 signaling pathway in ovarian epithelial cancers. World J Surg Oncol (2012) 10:193. doi:10.1186/1477-7819-10-193
196. Zhu Y, Huang JM, Zhang GN, Zha X, Deng BF. Prognostic significance of MyD88 expression by human epithelial ovarian carcinoma cells. J Transl Med (2012) 10:77. doi:10.1186/1479-5876-10-77
197. Pan H, He L, Wang B, Niu W. The relationship between RAGE gene four common polymorphisms and breast cancer risk in northeastern Han Chinese. Sci Rep (2014) 4:4355. doi:10.1038/srep04355
198. Tesarova P, Kalousova M, Jachymova M, Mestek O, Petruzelka L, Zima T. Receptor for advanced glycation end products (RAGE) – soluble form (sRAGE) and gene polymorphisms in patients with breast cancer. Cancer Invest (2007) 25:720–5. doi:10.1080/07357900701560521
199. Wang D, Li T, Ye G, Shen Z, Hu Y, Mou T, et al. Overexpression of the receptor for advanced glycation endproducts (RAGE) is associated with poor prognosis in gastric cancer. PLoS One (2015) 10:e0122697. doi:10.1371/journal.pone.0122697
200. Kohles N, Nagel D, Jungst D, Stieber P, Holdenrieder S. Predictive value of immunogenic cell death biomarkers HMGB1, sRAGE, and DNase in liver cancer patients receiving transarterial chemoembolization therapy. Tumour Biol (2012) 33:2401–9. doi:10.1007/s13277-012-0504-2
201. Wang X, Cui E, Zeng H, Hua F, Wang B, Mao W, et al. RAGE genetic polymorphisms are associated with risk, chemotherapy response and prognosis in patients with advanced NSCLC. PLoS One (2012) 7:e43734. doi:10.1371/journal.pone.0043734
202. Zhang S, Hou X, Zi S, Wang Y, Chen L, Kong B. Polymorphisms of receptor for advanced glycation end products and risk of epithelial ovarian cancer in Chinese patients. Cell Physiol Biochem (2013) 31:525–31. doi:10.1159/000350073
203. Tahara T, Arisawa T, Wang F, Shibata T, Nakamura M, Sakata M, et al. Toll-like receptor 2 -196 to 174del polymorphism influences the susceptibility of Japanese people to gastric cancer. Cancer Sci (2007) 98:1790–4. doi:10.1111/j.1349-7006.2007.00590.x
204. Junjie X, Songyao J, Minmin S, Yanyan S, Baiyong S, Xiaxing D, et al. The association between toll-like receptor 2 single-nucleotide polymorphisms and hepatocellular carcinoma susceptibility. BMC Cancer (2012) 12:57. doi:10.1186/1471-2407-12-57
205. Nieters A, Beckmann L, Deeg E, Becker N. Gene polymorphisms in toll-like receptors, interleukin-10, and interleukin-10 receptor alpha and lymphoma risk. Genes Immun (2006) 7:615–24. doi:10.1038/sj.gene.6364337
206. Mandal RK, George GP, Mittal RD. Association of toll-like receptor (TLR) 2, 3 and 9 genes polymorphism with prostate cancer risk in North Indian population. Mol Biol Rep (2012) 39:7263–9. doi:10.1007/s11033-012-1556-5
207. Bergmann C, Bachmann HS, Bankfalvi A, Lotfi R, Putter C, Wild CA, et al. Toll-like receptor 4 single-nucleotide polymorphisms Asp299Gly and Thr399Ile in head and neck squamous cell carcinomas. J Transl Med (2011) 9:139. doi:10.1186/1479-5876-9-139
208. Tittarelli A, Gonzalez FE, Pereda C, Mora G, Munoz L, Saffie C, et al. Toll-like receptor 4 gene polymorphism influences dendritic cell in vitro function and clinical outcomes in vaccinated melanoma patients. Cancer Immunol Immunother (2012) 61:2067–77. doi:10.1007/s00262-012-1268-7
209. Gast A, Bermejo JL, Claus R, Brandt A, Weires M, Weber A, et al. Association of inherited variation in toll-like receptor genes with malignant melanoma susceptibility and survival. PLoS One (2011) 6:e24370. doi:10.1371/journal.pone.0024370
210. Chen YC, Giovannucci E, Lazarus R, Kraft P, Ketkar S, Hunter DJ. Sequence variants of toll-like receptor 4 and susceptibility to prostate cancer. Cancer Res (2005) 65:11771–8. doi:10.1158/0008-5472.CAN-05-2078
211. Wang MH, Helzlsouer KJ, Smith MW, Hoffman-Bolton JA, Clipp SL, Grinberg V, et al. Association of IL10 and other immune response- and obesity-related genes with prostate cancer in CLUE II. Prostate (2009) 69:874–85. doi:10.1002/pros.20933
212. Song J, Kim DY, Kim CS, Kim HJ, Lee DH, Lee HM, et al. The association between toll-like receptor 4 (TLR4) polymorphisms and the risk of prostate cancer in Korean men. Cancer Genet Cytogenet (2009) 190:88–92. doi:10.1016/j.cancergencyto.2008.12.011
213. Kang R, Zhang Q, Zeh HJ III, Lotze MT, Tang D. HMGB1 in cancer: good, bad, or both? Clin Cancer Res (2013) 19:4046–57. doi:10.1158/1078-0432.CCR-13-0495
214. Galluzzi L, Bravo-San Pedro JM, Kroemer G. Organelle-specific initiation of cell death. Nat Cell Biol (2014) 16:728–36. doi:10.1038/ncb3005
215. Oka T, Hikoso S, Yamaguchi O, Taneike M, Takeda T, Tamai T, et al. Mitochondrial DNA that escapes from autophagy causes inflammation and heart failure. Nature (2012) 485:251–5. doi:10.1038/nature10992
216. West AP, Khoury-Hanold W, Staron M, Tal MC, Pineda CM, Lang SM, et al. Mitochondrial DNA stress primes the antiviral innate immune response. Nature (2015) 520:553–7. doi:10.1038/nature14156
217. Cao H, Ye H, Sun Z, Shen X, Song Z, Wu X, et al. Circulatory mitochondrial DNA is a pro-inflammatory agent in maintenance hemodialysis patients. PLoS One (2014) 9:e113179. doi:10.1371/journal.pone.0113179
218. Kung CT, Hsiao SY, Tsai TC, Su CM, Chang WN, Huang CR, et al. Plasma nuclear and mitochondrial DNA levels as predictors of outcome in severe sepsis patients in the emergency room. J Transl Med (2012) 10:130. doi:10.1186/1479-5876-10-130
219. Carp H. Mitochondrial N-formylmethionyl proteins as chemoattractants for neutrophils. J Exp Med (1982) 155:264–75. doi:10.1084/jem.155.1.264
220. Rabiet MJ, Huet E, Boulay F. Human mitochondria-derived N-formylated peptides are novel agonists equally active on FPR and FPRL1, while Listeria monocytogenes-derived peptides preferentially activate FPR. Eur J Immunol (2005) 35:2486–95. doi:10.1002/eji.200526338
221. Crouser ED, Shao G, Julian MW, Macre JE, Shadel GS, Tridandapani S, et al. Monocyte activation by necrotic cells is promoted by mitochondrial proteins and formyl peptide receptors. Crit Care Med (2009) 37:2000–9. doi:10.1097/CCM.0b013e3181a001ae
222. McDonald B, Pittman K, Menezes GB, Hirota SA, Slaba I, Waterhouse CC, et al. Intravascular danger signals guide neutrophils to sites of sterile inflammation. Science (2010) 330:362–6. doi:10.1126/science.1195491
223. Raoof M, Zhang Q, Itagaki K, Hauser CJ. Mitochondrial peptides are potent immune activators that activate human neutrophils via FPR-1. J Trauma (2010) 68:1328–32. doi:10.1097/TA.0b013e3181dcd28d
224. Dieude M, Striegl H, Tyznik AJ, Wang J, Behar SM, Piccirillo CA, et al. Cardiolipin binds to CD1d and stimulates CD1d-restricted gammadelta T cells in the normal murine repertoire. J Immunol (2011) 186:4771–81. doi:10.4049/jimmunol.1000921
Keywords: ATP, autophagy, calreticulin, ER stress response, HSPs, type I interferon
Citation: Fucikova J, Moserova I, Urbanova L, Bezu L, Kepp O, Cremer I, Salek C, Strnad P, Kroemer G, Galluzzi L and Spisek R (2015) Prognostic and predictive value of DAMPs and DAMP-associated processes in cancer. Front. Immunol. 6:402. doi: 10.3389/fimmu.2015.00402
Received: 23 June 2015; Accepted: 22 July 2015;
Published: 07 August 2015
Edited by:
Fabrizio Mattei, Istituto Superiore di Sanità, ItalyReviewed by:
Stephan Gasser, National University of Singapore, SingaporeZong Sheng Guo, Harvard University, USA
Copyright: © 2015 Fucikova, Moserova, Urbanova, Bezu, Kepp, Cremer, Salek, Strnad, Kroemer, Galluzzi and Spisek. This is an open-access article distributed under the terms of the Creative Commons Attribution License (CC BY). The use, distribution or reproduction in other forums is permitted, provided the original author(s) or licensor are credited and that the original publication in this journal is cited, in accordance with accepted academic practice. No use, distribution or reproduction is permitted which does not comply with these terms.
*Correspondence: Lorenzo Galluzzi, Equipe 11 - “Apoptose, Cancer et Immunité” Centre de Recherche des Cordeliers de Jussieu15, rue de l’École de Médecine, Paris 75006, France, deadoc@vodafone.it;
Radek Spisek, Sotio, Jankovcova 1518/2, 170 00 Prague 7, Czech Republic, spisek@sotio.com
†Lorenzo Galluzzi and Radek Spisek share senior co-authorship.