- Division of Clinical Medicine, Brighton and Sussex Medical School, Brighton, UK
A number of factors are recognized to influence immune responses to vaccinations including age, gender, the dose, and quality of the antigen used, the number of doses given, the route of administration, and the nutritional status of the recipient. Additionally, several immunogenetic studies have identified associations between polymorphisms in genes encoding immune response proteins, both innate and adaptive, and variation in responses to vaccines. Variants in the genes encoding Toll-like receptors, HLA molecules, cytokines, and cytokine receptors have associated with heterogeneity of responses to a wide range of vaccines including measles, hepatitis B, influenza A, BCG, Haemophilus influenzae type b, and certain Neisseria meningitidis serotypes, amongst others. However, the vast majority of these studies have been conducted in older children and adults and there are very few data available from studies conducted in infants. This paper reviews the evidence to date that host genes influencing vaccines responses in these older population and identifies a large gap in our understanding of the genetic regulation of responses in early life. Given the high mortality from infection in early life and the challenges of developing vaccines that generate effective immune responses in the context of the developing immune system further research on infant populations is required.
Introduction
Although infant and under-5 mortality rates are reducing, nonetheless 6.6 million children died in 2012 (1). Infectious diseases were responsible for approximately half of these deaths and the majority occurred in low or middle income countries (LMICs). There are no reliable data on morbidity for children at the global level so the burden of disability such as deafness and epilepsy following meningitis, for example, is unknown but likely to be high. Whilst vaccines are now available for many infections and increasingly administered in developing countries, there remains an urgent need to develop or improve vaccines for this age group. The reasons for this are multiple: there are no vaccines yet for some important infections such as malaria and respiratory syncytial virus; vaccines for other diseases need improving or replacing (e.g., BCG); global differences in microbial epidemiology may render effective vaccines developed in one region ineffective in another [e.g., the human papillomavirus (2)]; finally vaccines that do not cover all strains of a pathogen may drive changes in the microbiological epidemiology (or resistance to the strains that are included) that may render the vaccine ineffective. This has been widely discussed in the context of the pneumococcal vaccines (3).
However, despite these challenges, alongside the biological challenges of developing vaccines for infants with immature immune systems that contribute to their increased susceptibility to infection in the first place, there are strong reasons to investigate immune responses in the age group. In order to inform the development of novel or improved vaccines better understanding of the essential pathways involved in immunity to various pathogens is key. Genetics has over the years proved to be a useful tool with which to dissect out immune responses. Genetic studies of the monogenic primary immunodeficiencies revealed critical roles for a number of genes in protective immunity (e.g., mutations in genes encoding components of the interferon-gamma/interleukin-12/23 pathways predispose to disseminated non-tuberculous mycobacterial infections, highlight the important of this pathway in mycobacterial immunity more broadly (4, 5).
Role of Host Genetics in the Regulation of Vaccine Immune Responses
Most immune responses, like many biological responses, show wide-ranging inter-individual variation within in a population, whether in response to vaccination or natural infection. For example, interferon-gamma (IFN-g) responses following neonatal BGC in the Gambia follow a normal distribution (6) and 68% of variation in in vitro tumor necrosis factor (TNF) responses to microbial components measured in whole blood samples collected from a Ugandan population was genetic (7). Such patterns indicate a multifactorial etiology where both genes and environmental factors interact. Heritability studies can then be used to estimate the magnitude of genetic contribution to this phenotypic variation. It should be remembered however, that heritability estimates are specific to the population that was studied and they do not indicate how many genes may be involved or what the underlying genetic model is, i.e., whether there one major gene whose effects are modified by a few minor genes, or many genes each with a minor impact. Studies have shown that responses to a number of vaccines are heritable. These include BCG (8), hepatitis B virus (8–10), Haemophilus influenzae type b (11), tetanus toxoid (8), pneumococcal polysaccharide vaccine (12, 13), varicella vaccine (14), measles, mumps, and rubella (15, 16). Selected data from these studies are presented in more detail in Table 1.
Gene Identification
There have been many scientific advances in genetics, genomics, and the accompanying technology to allow high throughput data generation that are being widely applied in the hunt for genes that regulate vaccine responses. Historically, before the human genome sequence was available – which in turn led to genome-wide association studies (GWAS), whole genome sequencing (WGS), whole exome sequencing (WES), and gene expression profiling to mention a few – most investigators used a candidate gene approach to detect genetic variations associated with immune responses. The frequencies of allele variants within a gene hypothesized to be involved in response regulation were correlated with the magnitude of the immune response following vaccination within populations. Many associations have been reported and a selection summarized in Table 2, which is by no means comprehensive but intended to give a range of the studies that have been done, the vaccines studied and the number of putative genes identified. It can be seen that more studies have been published for some vaccines such as measles [reviewed by Haralambieva et al. (18)] and hepatitis B, but it is important to realize that there is publication bias for genetic association studies and negative findings are less frequently reported. With the exception of the BCG study, which used T cell cytokine responses as the phenotype, all the studies included in Table 2 use antibody responses as the phenotype with the goals of trying to understand why vaccines are poorly immunogenic in some but not all individuals and to identify genetic factors associated with persistence often immune response. Of note, all except two the studies included in Table 2 were conducted in older children and adults. The BCG study was conducted in South African neonates (19) and one of the studies showing an association between Class II HLA and failure to respond to hepatitis B vaccination was conducted in neonates in Italy (20). A more comprehensive systematic review of the link between genetic variation and variability in vaccine responses identified over 2500 potentially relevant studies in the initial search (in July 2013) of which 70 were considered in more detail and 34 fully analyzed (21). However, it should be noted that only one of the studies reviewed was conducted in infants.
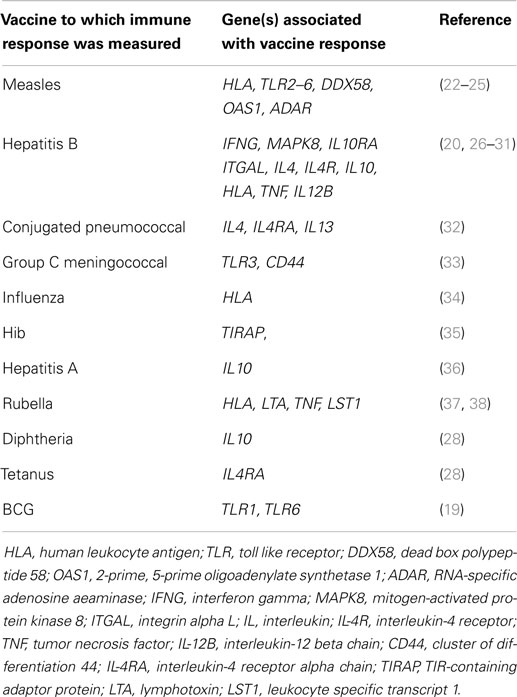
Table 2. Some reported associations between variants in candidate genes and immune responses to vaccines.
One of the limitations of the candidate gene population association approach in the past has been that often studies were small and therefore underpowered, especially if numerous genetic variants were being tested in the same small cohort. Furthermore, results were rarely reproducible between groups and populations. There are a few exceptions: for example meta-analyses for hepatitis B vaccine responses found evidence that variants in class II HLA and interleukin-4 (IL-4) were significantly associated with antibody responses (31, 39). As technology advanced it became possible to test multiple candidate gene variants in much larger sample sizes and large scale experiments such as that by Davila et al. became possible (29). In this study, 6091 single-nucleotide polymorphisms (SNPs) in 914 immune response genes were typed in 918 Indonesian people in a search for variants associated with hepatitis B vaccination responses. Previous associations with class II HLA were confirmed and a new association was identified with a SNP in the FOXP1 gene, a transcription factor involved in B-cell development.
As the field further developed, and the human genome sequence was first published (40), then the extent of variation within it was captured (41), it became possible to systematically interrogate genetic variants (mainly SNPs) across the genome and results could then be analyzed against phenotype data – this was the introduction of the GWAS. There was also a need to develop capacity to store and statistically analyze such large datasets. Over 500,000 SNPs could be typed in several thousand individuals (42) to identify novel diseases susceptibility loci.
A number of GWAS studies have been undertaken or are underway for childhood vaccines including MMR (43). In this study, SNPs in two genes were associated with the magnitude of IFN-g responses to rubella vaccination in school age children. Although not part of Expanded Program on Immunization schedules for children, GWAS have been conducted to detect associations with immune responses to smallpox vaccination, where significant associations were identified within a number of cytokine gene variants (44, 45), and anthrax vaccination where suggestive rather than significant associations was found with SNPs located with the HLA class II loci, the mex-3 homolog C (MEX3C) gene and the splA/ryanodine receptor domain and suppressor of cytokine signaling (SOCS) box containing 1 (SPSB1) gene (46). Genome-wide linkage studies identified three loci on three different chromosomes found to be linked to BCG responses in a Gambian twin cohort described elsewhere (8) and a GWAS has been undertaken in this cohort as well.
Looking forward, technology has developed in other parallel disciplines (or other “omics” as they are increasingly referred to) allowing a much more integrated and holistic approach toward the goal of developing new and more effective vaccines that work in everyone. Indeed, the term vaccinomics was coined by Poland and colleagues to capture the range of technologies now available to investigate the complex biological system responsible for providing protective immunity (47).
Genome-wide expression profiling took studies to the next level, investigating the activity of the genome rather than the inherent genome variation, i.e., which genes are “switched on” in any given situation such as stimulation post vaccination. In one study, microarray transcriptional profiling was used to assess responses to yellow fever vaccine (48). Sixty five of the 97 differentially regulated genes that were studied were shown to correlate with antibody and CD8 T cell specific responses and a subset of these could predict the response to vaccination. Lu et al. used a combination of transcriptional and epigenetic profiling to study both gene expression and methylation patterns in 25 infants who had received hepatitis B vaccine and were know to be either high or low responders according to their antibody responses (49). This study showed that modifications through hypo/hypermethylation, down regulation, and post transcriptional control were associated with low response to hepatitis B vaccine. There is also scope to harness in silico advances in bioinformatics, computational modeling and pathway analysis to enable the prediction of signature immune responses reviewed in more detail by Poland et al. (50).
Cutting edge technology is also being applied to enable more detailed and sophisticated phenotyping of the immune response to vaccination, which is currently relatively crude, involving the measurement of antibody levels and for some vaccines cellular responses through measuring cytokine levels. A recent study used high throughput sequencing technologies to characterize in detail the B-cell receptor repertoires in adults who had received a conjugated Hib/MenC/tetanus vaccine (51). This approach allowed the identification of antigen specific sequences that could be represent a welcome improvement in the ability to measure vaccine immunogenicity in a meaningful way.
Conclusion
There is good evidence that host genetic factors are important, but not sole, determinants of responses to vaccination. Initial genetic epidemiology studies demonstrated many responses were heritable, and using the genetic tools available at the time a number of groups went on to show associations between candidate genes and specific responses. Momentum in the field increased exponentially in the last decade or so due to advances in scientific methodology and supporting technology, which has allowed large scale interrogative studies. The studies referred to above by Querec et al., Lu et al., and Truck et al. illustrate the potential new technologies bring toward unraveling the complexities of immune responses to vaccines and it is likely that the field will advance rapidly. This has obvious implications for the development of better vaccines, and equivalent studies in infectious diseases for which there are no vaccines could help identify pathways critical to the immune responses that could be targeted in vaccine development.
Conflict of Interest Statement
The author declares that the research was conducted in the absence of any commercial or financial relationships that could be construed as a potential conflict of interest.
Acknowledgments
The author would like to acknowledge the support she has received for her work in this area from the Wellcome Trust, The MRC (UK), the British Lung Foundation, and the NC3Rs.
References
1. UNICEF, WHO, World Bank, Division U-DP. Levels and Trends in Child Mortality 2013. New York: United Nations Children’s Fund (2013).
2. Allan B, Marais DJ, Hoffman M, Shapiro S, Williamson AL. Cervical human papillomavirus (HPV) infection in South African women: implications for HPV screening and vaccine strategies. J Clin Microbiol (2008) 46:740–2. doi: 10.1128/JCM.01981-07
Pubmed Abstract | Pubmed Full Text | CrossRef Full Text | Google Scholar
3. Weinberger DM, Malley R, Lipsitch M. Serotype replacement in disease after pneumococcal vaccination. Lancet (2011) 378:1962–73. doi:10.1016/S0140-6736(10)62225-8
Pubmed Abstract | Pubmed Full Text | CrossRef Full Text | Google Scholar
4. Newport MJ, Huxley CM, Huston S, Hawrylowicz CM, Oostra BA, Williamson R, et al. A mutation in the interferon-gamma receptor gene and susceptibility to mycobacterial infections in man. N Engl J Med (1996) 335:1941–9. doi:10.1056/NEJM199612263352602
Pubmed Abstract | Pubmed Full Text | CrossRef Full Text | Google Scholar
5. Bustamante J, Boisson-Dupuis S, Abel L, Casanova JL. Mendelian susceptibility to mycobacterial disease: genetic, immunological, and clinical features of inborn errors of IFN-gamma immunity. Semin Immunol (2014) 26:454–70. doi:10.1016/j.smim.2014.09.008
Pubmed Abstract | Pubmed Full Text | CrossRef Full Text | Google Scholar
6. Finan C, Ota MO, Marchant A, Newport MJ. Natural variation in immune responses to neonatal Mycobacterium Bacillus calmette-guerin (BCG) vaccination in a cohort of Gambian infants. PLoS One (2008) 3:e485. doi:10.1371/journal.pone.0003485
Pubmed Abstract | Pubmed Full Text | CrossRef Full Text | Google Scholar
7. Stein CM, Guwatudde D, Nakakeeto M, Peters P, Elston RC, Tiwari HK, et al. Heritability analysis of cytokines as intermediate phenotypes of tuberculosis. J Infect Dis (2003) 187:1679–85. doi:10.1086/375249
Pubmed Abstract | Pubmed Full Text | CrossRef Full Text | Google Scholar
8. Newport MJ, Goetghebuer T, Weiss HA; The Mrc Gambia Twin Study Group, Whittle H, Siegrist CA, et al. Genetic regulation of immune responses to vaccines in early life. Genes Immun (2004) 5:122–9. doi:10.1038/sj.gene.6364051
Pubmed Abstract | Pubmed Full Text | CrossRef Full Text | Google Scholar
9. Hohler T, Reuss E, Evers N, Dietrich E, Rittner C, Freitag CM, et al. Differential genetic determination of immune responsiveness to hepatitis B surface antigen and to hepatitis A virus: a vaccination study in twins. Lancet (2002) 360:991–5. doi:10.1016/S0140-6736(02)11083-X
Pubmed Abstract | Pubmed Full Text | CrossRef Full Text | Google Scholar
10. Yan K, Cai W, Cao F, Sun H, Chen S, Xu R, et al. Genetic effects have a dominant role on poor responses to infant vaccination to hepatitis B virus. J Hum Genet (2013) 58:293–7. doi:10.1038/jhg.2013.18
Pubmed Abstract | Pubmed Full Text | CrossRef Full Text | Google Scholar
11. Lee YC, Newport MJ, Goetghebuer T, Siegrist C-A, Weiss HA, Pollard AJ, et al. Influence of genetic and environment factors on the immunogenicity of Hib vaccine in Gambian twins. Vaccine (2006) 24:5335–40. doi:10.1016/j.vaccine.2006.04.021
Pubmed Abstract | Pubmed Full Text | CrossRef Full Text | Google Scholar
12. Konradsen H, Henrichsen J, Wachmann H, Holm N. The influence on genetic factors on the immune response as judged by pneumococcal vaccination of mono and dizygotic Caucasian twins. Clin Exp Immunol (1993) 92:532–6. doi:10.1111/j.1365-2249.1993.tb03433.x
Pubmed Abstract | Pubmed Full Text | CrossRef Full Text | Google Scholar
13. Konradsen H, Oxelius V, Hahn-Zoric M, Hanson L. The importance of G1m and 2 allotypes for the IgG2 antibody levels and avidity against pneumococcal polysaccharide type 1 within mono- and dizygotic twin-pairs. Scand J Immunol (1994) 40:251–6. doi:10.1111/j.1365-3083.1994.tb03484.x
Pubmed Abstract | Pubmed Full Text | CrossRef Full Text | Google Scholar
14. Klein NP, Fireman B, Enright A, Ray P, Black S, Dekker CL. A role for genetics in the immune response to the varicella vaccine. Pediatr Infect Dis J (2007) 26:300–5. doi:10.1097/01.inf.0000257454.74513.07
15. Tan PL, Jacobson RM, Poland GA, Jacobsen SJ, Pankrantz VS. Twin studies of immunogenicity – determining the genetic contribution to vaccine failure. Vaccine (2001) 19:2434–9. doi:10.1016/S0264-410X(00)00468-0
Pubmed Abstract | Pubmed Full Text | CrossRef Full Text | Google Scholar
16. Poland GA, Ovsyannikova IG, Jacobson RM. Vaccine immunogenetics: bedside to bench to population. Vaccine (2008) 26:6183–8. doi:10.1016/j.vaccine.2008.06.057
Pubmed Abstract | Pubmed Full Text | CrossRef Full Text | Google Scholar
17. Tan PL, Jacobson RM, Poland GA, Jacobsen SJ, Pankratz VS. Twin studies of immunogenicity – determining the genetic contribution to vaccine failure. Vaccine (2001) 19:2434–9. doi:10.1016/S0264-410X(00)00468-0
Pubmed Abstract | Pubmed Full Text | CrossRef Full Text | Google Scholar
18. Haralambieva IH, Ovsyannikova IG, Pankratz VS, Kennedy RB, Jacobson RM, Poland GA. The genetic basis for interindividual immune response variation to measles vaccine: new understanding and new vaccine approaches. Expert Rev Vaccines (2013) 12:57–70. doi:10.1586/erv.12.134
Pubmed Abstract | Pubmed Full Text | CrossRef Full Text | Google Scholar
19. Randhawa AK, Shey MS, Keyser A, Peixoto B, Wells RD, De Kock M, et al. Association of human TLR1 and TLR6 deficiency with altered immune responses to BCG vaccination in South African infants. PLoS Pathog (2011) 7:e1002174. doi:10.1371/journal.ppat.1002174
Pubmed Abstract | Pubmed Full Text | CrossRef Full Text | Google Scholar
20. Martinetti M, De Silvestri A, Belloni C, Pasi A, Tinelli C, Pistorio A, et al. Humoral response to recombinant hepatitis B virus vaccine at birth: role of HLA and beyond. Clin Immunol (2000) 97:234–40. doi:10.1006/clim.2000.4933
Pubmed Abstract | Pubmed Full Text | CrossRef Full Text | Google Scholar
21. Posteraro B, Pastorino R, Di Giannantonio P, Ianuale C, Amore R, Ricciardi W, et al. The link between genetic variation and variability in vaccine responses: systematic review and meta-analyses. Vaccine (2014) 32:1661–9. doi:10.1016/j.vaccine.2014.01.057
Pubmed Abstract | Pubmed Full Text | CrossRef Full Text | Google Scholar
22. Poland GA, Ovsyannikova IG, Jacobson RM, Vierkant RA, Jacobsen SJ, Pankratz VS, et al. Identification of an association between HLA class II alleles and low antibody levels after measles immunization. Vaccine (2001) 20:430–8. doi:10.1016/S0264-410X(01)00346-2
Pubmed Abstract | Pubmed Full Text | CrossRef Full Text | Google Scholar
23. Dhiman N, Ovsyannikova IG, Vierkant RA, Ryan JE, Pankratz VS, Jacobson RM, et al. Associations between SNPs in toll-like receptors and related intracellular signaling molecules and immune responses to measles vaccine: preliminary results. Vaccine (2008) 26:1731–6. doi:10.1016/j.vaccine.2008.01.017
Pubmed Abstract | Pubmed Full Text | CrossRef Full Text | Google Scholar
24. Haralambieva IH, Ovsyannikova IG, Umlauf BJ, Vierkant RA, Shane Pankratz V, Jacobson RM, et al. Genetic polymorphisms in host antiviral genes: associations with humoral and cellular immunity to measles vaccine. Vaccine (2011) 29:8988–97. doi:10.1016/j.vaccine.2011.09.043
Pubmed Abstract | Pubmed Full Text | CrossRef Full Text | Google Scholar
25. Clifford HD, Yerkovich ST, Khoo SK, Zhang G, Upham J, Le Souef PN, et al. TLR3 and RIG-I gene variants: associations with functional effects on receptor expression and responses to measles virus and vaccine in vaccinated infants. Hum Immunol (2012) 73:677–85. doi:10.1016/j.humimm.2012.03.004
Pubmed Abstract | Pubmed Full Text | CrossRef Full Text | Google Scholar
26. Hohler T, Reuss E, Freitag CM, Schneider PM. A functional polymorphism in the IL-10 promoter influences the response after vaccination with HBsAg and hepatitis A. Hepatology (2005) 42:72–6. doi:10.1002/hep.20740
Pubmed Abstract | Pubmed Full Text | CrossRef Full Text | Google Scholar
27. Hennig BJ, Fielding K, Broxholme J, Diatta M, Mendy M, Moore C, et al. Host genetic factors and vaccine-induced immunity to hepatitis B virus infection. PLoS One (2008) 3:e1898. doi:10.1371/journal.pone.0001898
Pubmed Abstract | Pubmed Full Text | CrossRef Full Text | Google Scholar
28. Yucesoy B, Johnson VJ, Fluharty K, Kashon ML, Slaven JE, Wilson NW, et al. Influence of cytokine gene variations on immunization to childhood vaccines. Vaccine (2009) 27:6991–7. doi:10.1016/j.vaccine.2009.09.076
Pubmed Abstract | Pubmed Full Text | CrossRef Full Text | Google Scholar
29. Davila S, Froeling FE, Tan A, Bonnard C, Boland GJ, Snippe H, et al. New genetic associations detected in a host response study to hepatitis B vaccine. Genes Immun (2010) 11:232–8. doi:10.1038/gene.2010.1
Pubmed Abstract | Pubmed Full Text | CrossRef Full Text | Google Scholar
30. Lin YJ, Lan YC, Huang YC, Lin TH, Huang SM, Lai CC, et al. Effects of cytokine and cytokine receptor gene variation on high anti-HB titers: following up on Taiwan’s neonatal hepatitis B immunization program. Clin Chim Acta (2012) 413:1194–8. doi:10.1016/j.cca.2012.03.004
Pubmed Abstract | Pubmed Full Text | CrossRef Full Text | Google Scholar
31. Li ZK, Nie JJ, Li J, Zhuang H. The effect of HLA on immunological response to hepatitis B vaccine in healthy people: a meta-analysis. Vaccine (2013) 31:4355–61. doi:10.1016/j.vaccine.2013.06.108
Pubmed Abstract | Pubmed Full Text | CrossRef Full Text | Google Scholar
32. Wiertsema SP, Baynam G, Khoo SK, Veenhoven RH, Van Heerbeek N, Zhang G, et al. Impact of genetic variants in IL-4, IL-4 RA and IL-13 on the anti-pneumococcal antibody response. Vaccine (2007) 25:306–13. doi:10.1016/j.vaccine.2006.07.024
Pubmed Abstract | Pubmed Full Text | CrossRef Full Text | Google Scholar
33. Moore CE, Hennig BJ, Perrett KP, Hoe JC, Lee SJ, Fletcher H, et al. Single nucleotide polymorphisms in the toll-like receptor 3 and CD44 genes are associated with persistence of vaccine-induced immunity to the serogroup C meningococcal conjugate vaccine. Clin Vaccine Immunol (2012) 19:295–303. doi:10.1128/CVI.05379-11
Pubmed Abstract | Pubmed Full Text | CrossRef Full Text | Google Scholar
34. Gelder CM, Lambkin R, Hart KW, Fleming D, Williams OM, Bunce M, et al. Associations between human leukocyte antigens and nonresponsiveness to influenza vaccine. J Infect Dis (2002) 185:114–7. doi:10.1086/338014
Pubmed Abstract | Pubmed Full Text | CrossRef Full Text | Google Scholar
35. Ladhani SN, Davila S, Hibberd ML, Heath PT, Ramsay ME, Slack MP, et al. Association between single-nucleotide polymorphisms in Mal/TIRAP and interleukin-10 genes and susceptibility to invasive haemophilus influenzae serotype b infection in immunized children. Clin Infect Dis (2010) 51:761–7. doi:10.1086/656236
Pubmed Abstract | Pubmed Full Text | CrossRef Full Text | Google Scholar
36. Höhler T, Reuss E, Adams P, Bartsch B, Weigmann B, Wörns M, et al. A genetic basis for IFN-gamma production and T-bet expression in humans. J Immunol (2005) 175:5457–62. doi:10.4049/jimmunol.175.8.5457
Pubmed Abstract | Pubmed Full Text | CrossRef Full Text | Google Scholar
37. Ovsyannikova IG, Vierkant RA, Pankratz VS, O’byrne MM, Jacobson RM, Poland GA. HLA haplotype and supertype associations with cellular immune responses and cytokine production in healthy children after rubella vaccine. Vaccine (2009) 27:3349–58. doi:10.1016/j.vaccine.2009.01.080
Pubmed Abstract | Pubmed Full Text | CrossRef Full Text | Google Scholar
38. Ovsyannikova IG, Vierkant RA, Pankratz VS, Jacobson RM, Poland GA. Extended LTA, TNF, LST1 and HLA gene haplotypes and their association with rubella vaccine-induced immunity. PLoS One (2010) 5:e11806. doi:10.1371/journal.pone.0011806
Pubmed Abstract | Pubmed Full Text | CrossRef Full Text | Google Scholar
39. Cui W, Sun CM, Deng BC, Liu P. Association of polymorphisms in the interleukin-4 gene with response to hepatitis B vaccine and susceptibility to hepatitis B virus infection: a meta-analysis. Gene (2013) 525:35–40. doi:10.1016/j.gene.2013.04.065
Pubmed Abstract | Pubmed Full Text | CrossRef Full Text | Google Scholar
40. International Human Genome Sequencing Consortium. Initial sequence and analysis of the human genome. Nature (2001) 409:861–921. doi:10.1038/35057062
41. The International Hapmap Consortium. The international hapmap project. Nature (2003) 426:789–96. doi:10.1038/nature02168
42. Wellcome Trust Case Control Consortium. Genome-wide association study of 14,000 cases of seven common diseases and 3,000 shared controls. Nature (2007) 447:661–78. doi:10.1038/nature05911
Pubmed Abstract | Pubmed Full Text | CrossRef Full Text | Google Scholar
43. Kennedy RB, Ovsyannikova IG, Haralambieva IH, Lambert ND, Pankratz VS, Poland GA. Genome-wide SNP associations with rubella-specific cytokine responses in measles-mumps-rubella vaccine recipients. Immunogenetics (2014) 66:493–9. doi:10.1007/s00251-014-0776-3
Pubmed Abstract | Pubmed Full Text | CrossRef Full Text | Google Scholar
44. Kennedy RB, Ovsyannikova IG, Pankratz VS, Haralambieva IH, Vierkant RA, Jacobson RM, et al. Genome-wide genetic associations with IFNgamma response to smallpox vaccine. Hum Genet (2012) 131:1433–51. doi:10.1007/s00439-012-1179-x
Pubmed Abstract | Pubmed Full Text | CrossRef Full Text | Google Scholar
45. Kennedy RB, Ovsyannikova IG, Pankratz VS, Haralambieva IH, Vierkant RA, Poland GA. Genome-wide analysis of polymorphisms associated with cytokine responses in smallpox vaccine recipients. Hum Genet (2012) 131:1403–21. doi:10.1007/s00439-012-1174-2
Pubmed Abstract | Pubmed Full Text | CrossRef Full Text | Google Scholar
46. Pajewski NM, Shrestha S, Quinn CP, Parker SD, Wiener H, Aissani B, et al. A genome-wide association study of host genetic determinants of the antibody response to anthrax vaccine adsorbed. Vaccine (2012) 30:4778–84. doi:10.1016/j.vaccine.2012.05.032
Pubmed Abstract | Pubmed Full Text | CrossRef Full Text | Google Scholar
47. Poland GA, Kennedy RB, McKinney BA, Ovsyannikova IG, Lambert ND, Jacobson RM, et al. Vaccinomics, adversomics, and the immune response network theory: individualized vaccinology in the 21st century. Semin Immunol (2013) 25:89–103. doi:10.1016/j.smim.2013.04.007
Pubmed Abstract | Pubmed Full Text | CrossRef Full Text | Google Scholar
48. Querec TD, Akondy RS, Lee EK, Cao W, Nakaya HI, Teuwen D, et al. Systems biology approach predicts immunogenicity of the yellow fever vaccine in humans. Nat Immunol (2009) 10:116–25. doi:10.1038/ni.1688
Pubmed Abstract | Pubmed Full Text | CrossRef Full Text | Google Scholar
49. Lu Y, Cheng Y, Yan W, Nardini C. Exploring the molecular causes of hepatitis B virus vaccination response: an approach with epigenomic and transcriptomic data. BMC Med Genomics (2014) 7:12. doi:10.1186/1755-8794-7-12
Pubmed Abstract | Pubmed Full Text | CrossRef Full Text | Google Scholar
50. Poland GA, Kennedy RB, Ovsyannikova IG. Vaccinomics and personalized vaccinology: is science leading us toward a new path of directed vaccine development and discovery? PLoS Pathog (2011) 7:e1002344. doi:10.1371/journal.ppat.1002344
Pubmed Abstract | Pubmed Full Text | CrossRef Full Text | Google Scholar
51. Truck J, Ramasamy MN, Galson JD, Rance R, Parkhill J, Lunter G, et al. Identification of antigen-specific B cell receptor sequences using public repertoire analysis. J Immunol (2015) 194:252–61. doi:10.4049/jimmunol.1401405
Pubmed Abstract | Pubmed Full Text | CrossRef Full Text | Google Scholar
Keywords: SNPs, transcriptional profiling, candidate gene, GWAS
Citation: Newport MJ (2015) The genetic regulation of infant immune responses to vaccination. Front. Immunol. 6:18. doi: 10.3389/fimmu.2015.00018
Received: 10 September 2014; Accepted: 09 January 2015;
Published online: 02 February 2015.
Edited by:
Tobias R. Kollmann, University of British Columbia, CanadaReviewed by:
Olivia J. White, University of Queensland, AustraliaManish Sadarangani, University of Oxford, UK
Copyright: © 2015 Newport. This is an open-access article distributed under the terms of the Creative Commons Attribution License (CC BY). The use, distribution or reproduction in other forums is permitted, provided the original author(s) or licensor are credited and that the original publication in this journal is cited, in accordance with accepted academic practice. No use, distribution or reproduction is permitted which does not comply with these terms.
*Correspondence: Melanie J. Newport, Division of Clinical Medicine, Brighton and Sussex Medical School, Falmer, Brighton BN1 9PS, UK e-mail: m.j.newport@bsms.ac.uk