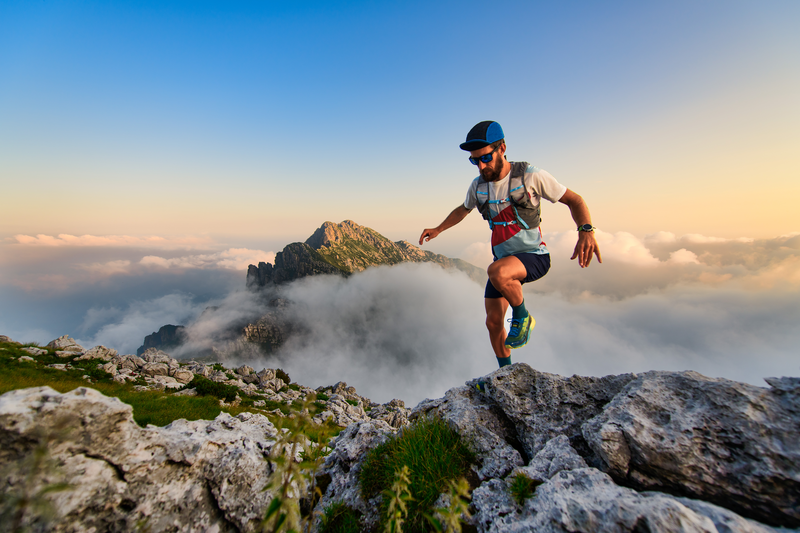
94% of researchers rate our articles as excellent or good
Learn more about the work of our research integrity team to safeguard the quality of each article we publish.
Find out more
REVIEW article
Front. Immunol. , 13 January 2014
Sec. Vaccines and Molecular Therapeutics
Volume 4 - 2013 | https://doi.org/10.3389/fimmu.2013.00498
This article is part of the Research Topic Gamma interferon: From antimicrobial activity to immune regulation View all 10 articles
Interferon-γ (IFNγ) plays various roles in the pathogenesis of HIV/AIDS. In an HIV-1 infected individual, the production of IFNγ is detected as early as the acute phase and continually detected throughout the course of infection. Initially produced to clear the primary infection, IFNγ together with other inflammatory cytokines are involved in establishing a chronic immune activation that exacerbates clinical diseases associated with AIDS. Unlike Type 1 IFNs, IFNγ has no direct antiviral activity against HIV-1 in primary cultures, as supported by the in vivo findings of IFNγ therapy in infected subjects. Results from both in vitro and ex vivo studies show that IFNγ can instead enhance HIV-1 replication and its associated diseases, and therapies aimed at decreasing its production are under consideration. On the other hand, IFNγ has been shown to enhance cytotoxic T lymphocytes and NK cell activities against HIV-1 infected cells. These activities are important in controlling HIV-1 replication in an individual and will most likely play a role in the prophylaxis of an effective vaccine against HIV-1. Additionally, IFNγ has been used in combination with HIV-1 vaccine to augment antiviral immunity. Technological advancements have focused on using IFNγ as a biological marker to analyze the type(s) of immunity generated by candidate HIV vaccines and the levels of immunity restored by anti-retroviral drug therapies or novel immunotherapies. Hence, in addition to its valuable ancillary role as a biological marker for the development of effective HIV-1 prophylactic and therapeutic strategies, IFNγ has a vital role in promoting the pathogenesis of HIV.
Interferon-γ (IFNγ) is a Type II interferon that is pivotal in the regulation of the host immune response against viral and intracellular bacterial pathogens. The effects of IFNγ are broad and far-reaching, exhibiting polyfunctional effects on immune activation, proinflammatory responses, and immune modulation. High levels of IFNγ are secreted by Type 1 T helper cells (Th1 cells), CD8+ cytotoxic T lymphocytes (CD8+ CTLs), and NK cells during active infection (1). IFNγ has a major effect on the regulation of antigen presentation by macrophages and dendritic cells, and in induction of class switching of B cells (2, 3). As a proinflammatory cytokine IFNγ directly activates phagocytic cells and stimulates oxidative burst and the release of degradative enzymes, thereby supporting the host defense responses against intracellular pathogens (4). IFNγ also induces the production of proinflammatory cytokines and chemokines on endothelial cells, epithelial cells, and fibroblasts. Focal release of IFNγ results in vasodilation and upregulation of adhesion molecules, promoting diapedesis of neutrophils, and macrophages to the site of inflammation. In addition to upregulation of innate defense mechanisms, IFNγ is also pivotal in immune modulation. Moreover, IFNγ upregulates the expression of MHC-I and -II molecules, activates antigen presenting cells and induces macrophage maturation toward a proinflammatory phenotype (4, 5). IFNγ also works synergistically with other cytokines such as IL-2 and -4 to balance the T helper subsets Th1/Th2, regulating the cytotoxic versus humoral T cell immune response (6). It is this integral part in the immune regulation and proinflammatory antiviral response that has made IFNγ an attractive biomarker to evaluate the immune competence and antiviral response in HIV-1 patients.
It is estimated that 20–45% of infants born to HIV positive mothers become HIV positive in the perinatal period (7, 8). Of these infants, 25–35% infected within the first year of life acquire the disease through breast feeding (7). Although this patient subset represents individuals with naïve and underdeveloped immune systems, multiple studies have demonstrated competent HIV-1 specific IFNγ responses in infants <1 year of age (7–11). Legrand et al. detected HIV-1 specific IFNγ responses generated specifically from CD8+ T cell subsets, demonstrating the ability of this naïve immune system to develop an antigen-specific T cell response (11). In a Nairobi trial of more than 200 breast fed infants born to HIV-1 positive (HIV+) mothers, more than half of exposed infants remained seronegative for the first year of life (8). The IFNγ response in these exposed but uninfected infants was significantly increased compared with infected cohorts, revealing a positive correlation of increased IFNγ response with those infants that remained HIV-1 seronegative up to 1 year of age (Table 1) (8). Although there was a significant increase in IFNγ expression in the exposed/uninfected infants, IFNγ levels were moderate and often cyclical to transient with only 12–22% of them having detectable IFNγ at any given time point (8). This suggests that prolonged, repeated exposure to HIV-1 through breast feeding was a significant factor in inducing and maintaining an IFNγ response (8, 9). Highly exposed, persistently seronegative sex workers demonstrate high levels of HIV-1 specific IFNγ responses in CD8+ CTLs (Table 1) (12). Individuals within this cohort who had a 2-month or greater break in sex work were 6.5 times more likely to seroconvert, suggesting a significant decrease in HIV-1 antigen-specific response resulting from a gap in prolonged and repeated antigen exposure (12).
Although the naïve state of the neonatal and infant immune system is a concern in HIV-1 exposure, HIV-1 specific CTL responses have been reported in both exposed and infected infants (7, 8, 10, 11). HIV-1 infected infants <1 year of age with a detectable circulating viral load mount a substantial and sustained antigen-specific immune response. However, the magnitude of their response is attenuated as compared with adults (8). It is theorized that this attenuated response in infected infants may be attributed to an overall decrease in IFNγ producing cells, a suppression of the Th1 response, underdevelopment of the CD4+ T cell repertoire, or immaturity of antigen processing related to age (10). Although a reduction of the number of IFNγ producing cells correlates with a decrease in the overall CD4+ counts in infected infants a twofold increase in HIV-1 specific IFNγ response was detected in infected infants in the first year of life (10). This increase not only demonstrates a continued expression of IFNγ in infected infants, but also showed a significant trend toward an increased immunologic response when limited to those breastfed infants surviving 1 year or more (10). Even though an increased IFNγ response and HIV-1 specific CTL can be detected in HIV-1 infected infants, there is no correlation between the presence of antigen-specific CTL or IFNγ response to reduction in peak viral load, viral steady state, or incidence of mortality in infected infants (Table 1) (10). Therefore, this positive trend likely reflects the immunologic stimulation attributed to continued and prolonged exposure to HIV-1 rather than a sustained response to initial infection.
Throughout the acute stage of HIV-1 infection, IFNγ levels in infected adults steadily increase, with a peak approximately 20–24 days post-infection (Table 1) (13). In chronic, stable disease, IFNγ levels decline to a steady state that is often equivalent to healthy controls (17). Although there is a predictable elevated trend in overall IFNγ expression during HIV-1 clinical disease, no significant difference has been reported in HIV-1 specific IFNγ response of the CTLs in both progressor and long-term non-progressor patients with chronic disease (17). Although overall expression of IFNγ by CD8+ CTLs does not correlate to stage or chronicity of clinical disease, significantly larger numbers of HIV-1 specific CTLs are maintained in long-term non-progressors (18, 19). There is a significant trend of steady increase of IFNγ levels in chronic, progressive disease however, there is marked patient to patient variability in overall expression of IFNγ with no demonstrable correlation between IFNγ expression and viral load, viral set point, viral clearance, or chronicity (14–16) (Table 1). In a cross-sectional study performed by Wantanabe et al. (20), proinflammatory cytokines TNF-alpha, IL-6, IL-10, IL-18, and IL-7 levels had a significant correlation with CD4 count in HIV+ patients, but IFNγ levels were often continuously elevated and variable between patients with no significant correlation to progressors and long-term non-progressors in chronically infected patients (20). Several theories exist as to why IFNγ response does not correlate with disease progression and largely center on the polyfunctional and proinflammatory effects of IFNγ (16). It has been suggested that cytokine expression and immunologic profiles in HIV+ patients are more proinflammatory than immunoregulatory when compared to uninfected but exposed controls (21). It is also likely that HIV-1 infection results in modification of antigen presentation in macrophages and dendritic cell lines, resulting in anergy of HIV-1 specific CD4+ and CD8+ T cells (22). Another theory suggests diminished response to IFNγ in target populations may alter immunomodulation of Th1/Th2 response through the production of synergistic (IL-2, TNFα) or inhibitory (TGFβ, IL-10, IL-13) cytokines (16). A recent study evaluated a combined proinflammatory and immunomodulatory cytokine panel including IFNγ to predict viral load set point 12 months after infection (23). In the combined panel, IFNγ, IL-12p40/70, IL-7, and IL-15 levels predicted 66% of viral set point variation in acute phase patients (23). Further studies are required to evaluate the predictive value of this panel on morbidity and progression of patients with chronic disease. In addition to the polyfunctional effects of IFNγ, and due to the marked complexity of the antiviral response, it is likely that monitoring of HIV-1 patients by a proinflammatory cytokine panel rather than relying on a single cytokine will better predict viral load set point and progression of clinical disease.
Many studies have been performed to determine the roles that IFNγ plays in anti-HIV therapy. Initially, clinical studies determined that IFNγ can either hinder or augment the pathogenesis of HIV-1. The latter observations raised a major concern about the use of IFNγ in HIV-1 therapy. Concomitantly, a number of in vitro studies tested the anti-HIV activity of IFNγ on HIV-1 infection but with conflicting results. Subsequently, a small number of clinical trials investigated whether IFNγ has therapeutic effects against HIV-1 in HIV+ subjects. With the growing understanding of the roles that cytokines play in infection and disease progression, cytokines including IFNγ have been measured to assess the efficacy of anti-retroviral therapy (ART). ART has greatly improved the quality of life and the lifespan of the HIV-infected subjects but does not substantially restore the immune system destroyed by HIV-1. Consequently, IFNγ and cytokines which induce or enhance IFNγ activity have been considered for therapy to restore the immune system in particular T cell number and function. The opposing or conflicting effects of IFNγ on HIV-1 pathogenesis and immune function have complicated the role that IFNγ plays on anti-HIV therapy.
Interferons were originally discovered, named, and characterized based on their ability to inhibit viral replication (24). These soluble factors are classified as Type I and II IFNs with IFNγ being the sole representative of the Type II IFN family (25, 26). Although both Type I and II IFNs can induce an antiviral host response, they differ by both antigenic induction, receptor specificity, and cell expression. While Type I IFNs are largely induced by viral infection of host cells, IFNγ is induced by more generalized antigenic and mitogenic stimulation (25). Type I IFNs are secreted at low levels by almost all cell types, however are primarily secreted by hematopoietic cells (IFNα, IFNω) and fibroblasts (IFNβ) (26). IFNγ is primarily produced by CD4+ and CD8+ T cells as well as NK cells with more recent reports of low level expression in NKT cell and professional antigen presenting cells (26). Both Type I and II IFNs induce a wide range of proteins with activity targeting different stages of viral replication. However, IFNγ upregulates MHC-I on the cell surface, which increases antigenic recognition of intracellular pathogens by CTLs. In addition, only IFNγ can upregulate the MHC-II pathway, supporting antigen-specific activation of CD4+ T cells (25, 26).
There are a number of IFN-induced proteins and gene products that confer antiviral activity. The first of these is dsRNA-regulated protein kinase (PKR) which is a serine/threonine kinase found predominantly in the cytoplasm and associated with ribosomes. PKR is activated by dsRNA and inhibits the synthesis of viral proteins through phosphorylation of eukaryotic translation initiation factor-2 (eIF-2). In addition to antiviral activity, PKR also plays a role in modulation of cell proliferation and induction of apoptosis (25, 26). The dsRNA-specific adenosine deaminase (ADAR), catalyzes the deamination of adenosine to inosine, resulting “editing” or mistranslation of the viral sequence. Mistranslation of gene products can lead to the production of non-functional viral proteins. The 2′,5′-oligoadenylate synthetase (OAS) in combination with RNase L is activated by dsRNA during viral infection and induces degradation of RNA. The protein Mx GTPases, a superfamily of dyamin-like GTPases, associate with viral protein complexes to impair transport of viral nucleocapsids into the nucleus of the host cell, preventing transcription. Type 1 IFN-regulated gene expression of Mx1 and CD317 may be involved in control of HIV neurovirulence (27). Although Mx GTPases are induced by Type I IFNs but not by Type II IFNγ (25), other classes of GTPases are induced by IFNγ allowing antiviral targeting of GTP by other mechanisms (26). More recent findings on IFN-induced tetherin/BST-2, an antagonist of HIV Vpr, may be important in prevention/control of HIV infection via innate immunity (28).
Several IFN-inducible mechanisms are involved in host response and immune evasion of HIV. The expression of HIV Trans-activator of transcription (Tat) can either negatively or positively affect IFN-induced PKR in regulating HIV-1 infection. Tat can prevent autophosphorylation of PKR and competes with eIF-2 while upregulating NF-κB to promote transcription (25). IFN response/regulatory factors (IRFs) compete with the binding site of HIV’s LTR promoter and suppress viral transcription (29). Both Type I and II IFNs induce PKR, OAS, and ADAR, however there is a significant difference in the sensitivity to Type I and II IFNs to HIV in PBMC, T cells, and macrophages. Although the specific cause has not been elucidated, it is likely that the constant presence of IFNγ will most likely promote negative regulation of IFNγ signaling through the SOCS pathway (particularly SOCS1) and PIAS, a Stat inhibitor. Induction of these proteins target at various stages of viral replication and can induce an “antiviral state” within the host. However, adaptation and host evasion mechanisms allow replication of species adapted lentiviruses [HIV in humans, simian immunodeficiency virus (SIV) in macaques] despite the induction of IFN-induced anti-retroviral states (30).
The direct effect of IFNγ on HIV-1 infection was first evaluated in in vitro studies followed by small scale clinical trials. In vitro studies have shown IFNγ treatment to either enhance or have no effect on HIV-1 infection of PBMC (31). These observations with PBMC were strikingly different from what was expected in 1986 since all interferons (α, β, γ) were thought to have direct antiviral activities to all types of viruses (32). Subsequent, in vitro studies demonstrated that IFNγ treatment can enhance HIV-1 infection in both primary macrophages and CD4+ T cells (33–35), suggesting that these immune cell subsets were responsible for the original observation of HIV-1 infection of PBMC.
Given that IFNγ is also produced early during cytokine storms in the acute stage of HIV-1 infection, IFNγ was thought to affect the subsequent development of CTL activities to control HIV-1 load (36–38). There is a conflicting view regarding the role of cytokines such as IFNγ in modulating cellular immunity which can in turn determine the viral set point (38, 39), as a high viral set point is positively associated with progression to AIDS (40, 41). The well documented role of IFNγ in enhancing CTL activities against viral infection has supported the concept that IFNγ therapy can augment anti-HIV CTL activities in HIV+ subjects (42, 43). This is further supported by the finding that early control of HIV-1 load correlates with production of anti-HIV CD4+ and CD8+ CTLs (36, 37, 44), while similar control of virus load has been described for HIV+ long-term survivors (LTSs) (45), elite controllers (ECs) (46, 47), and highly exposed persistently seronegative women (48). Based on these observations, clinical trials in adult patients have evaluated the toxicity, pharmacokinetics, and therapeutic effect of IFNγ on HIV-1 p24 load, AIDS-associated complex (ARC), and AIDS-associated Kaposi’s sarcoma (KS) (49–52). The majority of the clinical trials showed no significant improvement in ARC or KS as well as no significant decrease in HIV-1 p24 load (Table 2) (49–52). IFNγ had no effect at doses that conferred therapeutic efficacy for IFNα or even at higher doses that resulted in mild toxicity (53–57). Notably, in comparison to the potent anti-HIV and -KS activities of IFNα and β, the lack of anti-HIV/AIDS activities of IFNγ greatly reduced the enthusiasm toward IFNγ and therapeutic focus shifted to IFNα as therapy against HIV/AIDS (57). Although clinical trials have also been conducted with pegylated IFNα to modulate its activity, the general consensus is that IFNα therapy is too toxic and significantly less effective than ART in decreasing HIV-1 load but is effective for treatment of KS (58–60).
Clinical assessment of IFNγ levels in the serum of HIV+ adult subjects at different clinical stages has been used to determine the importance of IFNγ in the pathogenesis of HIV-1. Clinical studies have focused on the changes in cytokine levels upon introduction of highly active anti-retroviral therapy (HAART), or ART. The elevation of multifunctional cytokines such as IFNγ and TNFα, can either enhance or control HIV-1 infection depending on the clinical stage of HIV-1 infection. A cross-sectional study showed significantly elevated serum levels of certain cytokines (TNFα, IL-6, IL-7, IL-10, IL-18) and an increasing trend for serum IFNγ levels in symptomatic subjects when compared to asymptomatic subjects prior to treatment with ART (20). Cross sectional and longitudinal clinical studies, comparing pre-ART to post-ART subjects demonstrated high serum levels of many cytokines (IL-6, IL-10, IL-18) in pre-ART subjects which significantly decreased when ART was initiated. Concomitant with ART, the serum HIV-1 load decreased to low or undetectable levels while the CD4+ counts and serum IL-21 often increased (20, 72–77). In the case of IFNγ, cross-sectional study of pre- and post-ART showed a decreasing trend in serum IFNγ levels with the initiation of ART (Table 2) (20). In comparison, a longitudinal study showed a statistically significant decrease in IFNγ after 60 days or longer on ART (20). Although the majority of the subjects had a major decrease in IFNγ, 33% of the subjects maintained high serum IFNγ levels. In this study, all HIV+ subjects were treated for secondary clinical diseases before enrollment to ensure cytokine changes during the study were predominantly attributed to HIV-1 infection. Thus, the authors of this work speculated that sustained high IFNγ levels in this group were due to individual differences in immune responses against HIV-1, the genetic characteristics of HIV-1, or both, and not due to other potentially confounding clinical events (20).
Another approach for evaluating the immune status at pre- and post-ART is to measure the level of IFNγ responses to HIV-1 proteins or peptides by the PBMC or T cells from HIV+ subjects (78, 79). The hallmark of HIV-1 infection is the loss of CD3+CD4+ T cell counts correlating with increases in both virus load and disease progression (80). As a result, measuring T cell immunity, specifically CD3+CD4+ T cell activities, was thought to be useful at assessing the immune status of the HIV+ subject when analyzed in combination with CD3+CD4+ T cell count and virus load (81, 82). HIV-specific CD3+CD8+ T cell activities develop shortly after the cytokine storm and work to control HIV-1 load during acute infection (37–39). Remarkably, IFNγ responses were consistently detected in both CD3+CD4+ and CD3+CD8+ T cells of the HIV+ subjects at various clinical stages, but IFNγ responses alone had no direct correlation to delay in progression to AIDS (78, 82–84). The presence of polyfunctional T cells, which expressed IFNγ in combination with other cytokines (IL-2 and TNFα) and/or cytotoxins (perforin or granzyme), was associated with HIV-1 non-progression (81–84). CD3+CD4+ and CD3+CD8+ T cells induced with viral epitopes are important effector cells against HIV-1 infection. The IFNγ analysis of T cells from chronically HIV-1 infected patients during ART demonstrated that HIV-specific IFNγ responses varied within the T cell subsets evaluated (68–71, 85). Moreover, during ART, IFNγ responses of HIV-specific CD4+ T cells expanded and contracted (68), decreased (69), or increased (70); while those of CD8+ T cells generally decreased (70, 71) (Table 2). Interestingly, HIV-specific IFNγ responses of polyfunctional CD8+ T cells increased (85).
The highly effective ART was released in developed countries in late 1990s (86) and in developing/underdeveloped countries in mid 2000 (87). ART is a combination of two or more anti-retroviral drugs that inhibits viral reverse transcriptase (RT) (nucleoside and non-nucleoside RT inhibitors), protease, integrase, viral co-receptor attachment (CCR5 inhibitor), and/or virus penetration (fusion inhibitor) (88). ART will decrease the circulating HIV-1 load to low or undetectable levels in plasma within weeks to months (89). It has dramatically reduced the HIV-associated morbidity and mortality but the opportunistic infections and AIDS-associated cancers still persist despite ART (88). Moreover, even after 7–10 years of ART and viral control, a complete reconstitution of immune responses to HIV-1 has not been achieved while only a modest improvement in HIV-specific T cell responses was observed (90–92). Consequently, a rapid means to restore anti-HIV T cell immunity is still required.
Many therapy using cytokines (IL-2, IL-12, G-CSF, GM-CSF) including IFNγ have been evaluated in combination with ART as immune reconstitution therapy (93–95). These therapies are needed due to the new-onset opportunistic infections resulting from failed ART combinations or simply due to the inability of ART to completely eliminate the opportunistic infection (96–98). In one study in South Africa, tuberculosis (TB) incidence rates during 8 years of follow-up showed substantially higher rates in HIV+ subjects on long-term ART than in HIV uninfected individuals living in the same community (92). IFNγ has been used as adjunctive immunotherapy with or without ART for the treatment of HIV-associated opportunistic infections such as cryptococcal meningitis (61–63), Pneumocystis carinii (64, 65), Toxoplasma gondii (65), Candida albicans (64, 65), Mycobacterium avium (65, 66), and visceral leishmaniasis (65, 67). In a majority of the cases, adjunctive IFNγ therapy with or without other cytokines did not adversely affect the ART therapy for those on ART (i.e., maintained low to undetectable virus load) and did not increase CD4+ T cell counts in most HIV+ patients except for those ART (61–66). These therapies had either a decreasing trend or a significant decrease in various HIV-associated opportunistic infections which were often resistant to conventional therapy against the organism (Table 2) (61–67).
Ten to 32% of AIDS patients starting ART develop an unusual disease condition called immune restoration disease (IRD) or immune reconstitution inflammatory syndrome (IRIS) (99–103). IRIS is a disease condition where the opportunistic infections or other diseases (e.g., Graves’ disease, neoplasm, or virus-associated diseases) of the AIDS patients worsen shortly after the initiation of ART (99–101). The neoplasm and/or virus-associated diseases observed in IRIS included KS with human herpesvirus-8, non-Hodgkin’s lymphoma with Epstein–Barr virus, and progressive multifocal leukoencephalopathy with JC virus (99, 101). IFNγ together with TNFα, C-reactive protein, and IL-7 are the inflammatory cytokines all contribute to the development of IRIS (101–103). As a result, anti-inflammatory therapy in addition to the anti-microbial therapy, is commonly used to treat IRIS associated with opportunistic infections (99).
The development of an effective HIV-1 vaccine for humans requires the identification of protective HIV-1 vaccine epitopes conserved among most HIV-1 subtypes, the construction of protective epitopes into a vaccine immunogen, and determining the best vaccine delivery system for induction of both mucosal and systemic immunity against HIV-1. As the cytokines expressed by many T cell subsets, IFNγ and IL-2 have been used as the biomarkers for CD4+ and CD8+ T cell activities induced by candidate HIV-1 vaccine antigens. Both of these cytokines are important in enhancing HIV-specific CTL activities and antibody synthesis essential for generating vaccine immunity. Ideally, cytokines produced by CD3+CD4+ Th cells should augment effector functions of both T and B cells upon vaccination. Initial HIV-1 vaccine studies searched for B-cell epitopes on HIV-1 envelopes (transmembrane and surface envelopes) that induced broadly reactive virus neutralizing antibodies, while subsequent vaccine studies focused on developing an HIV-1 vaccine that induced potent anti-HIV CTL activities. IFNγ has had a major impact on determining the presence of CD8+ CTL epitopes on HIV-1 proteins, with the highest levels detected from HIV-1 Gag, Pol, and Nef proteins. In the most successful HIV-1 vaccine trial, both polyfunctional CD4+ T cells and CD4+ CTLs that expressed IFNγ and other cytokines or cytotoxins were detected in the vaccinees. Thus far, IFNγ has played a major role as a biomarker of T cell activation in the development of an HIV-1 vaccine.
The IFNγ responses to HIV-1 peptides by the PBMC or T cells from HIV+ subjects have been used to identify the regions on the virus that induced CD3+CD8+ CTL and CD3+CD4+ T cell activity (78). Three of the most commonly used assays for such analysis are tetramer staining, IFNγ ELISpot analysis, and FACS-based intracellular staining (ICS) for IFNγ in combination with T cell phenotypic markers (16, 78, 104, 105). Since the reagents for IFNγ became available before other cytokines and cytotoxins, the latter two analyses frequently utilize IFNγ. Furthermore, IFNγ responses of T cells are detected throughout the duration of HIV-1 infection (78, 106). IFNγ ELISpot is a more rapid and cost efficient assay than ICS or tetramer analyses. The IFNγ ELISpot analysis using purified CD3+CD8+ and CD3+CD4+ T cells was initially used to map the CD8+ CTL and CD4+ Th epitopes on HIV-1 proteins. Some of the HIV-1 epitopes defined by IFNγ ELISpot analysis has been confirmed by IFNγ-specific ICS (104, 105). The CTL and TH epitopes on all HIV-1 proteins have been listed in Los Alamos National Laboratory (LANL) database (http://www.hiv.lanl.gov/content/immunology/maps/maps.html). Such database is useful in identifying the HIV-1 epitopes needed for developing prophylactic vaccines as well as immune-based therapy against HIV-1.
Perhaps the most problematic issue with the use of IFNγ-based analysis is that the IFNγ levels from CD4+ and CD8+ T cells alone do not correlate with HIV-1 load or disease progression (Table 3) (78, 79, 83, 105, 107). For this reason, the HIV-specific IFNγ levels only indicate the ability of these T cell subsets to produce IFNγ responses to HIV-1 peptides. Polyfunctional T cells, which are involved in controlling HIV-1 load, express IFNγ in addition to other cytokines and cytotoxins (48, 49, 81–84) particularly in a combination of IFNγ with perforin, IL-2, TNFα, or granzyme B. These T cells are thought to be important in the control of HIV-1 infection in HIV+ LTSs and ECs (46, 81, 82). In addition, proliferative T cells expressing IFNγ have also been observed at higher levels in LTS and EC, but at lower levels in HIV+ progressors (46, 82). These studies suggest that vaccines that only induce IFNγ in HIV-specific CD4+ T cells and/or CD8+ T cells are unlikely to protect humans against HIV-1. Instead, those that induce polyfunctional T cell activities against HIV-1 are more likely to be useful as vaccine immunogens.
Preclinical trials in the SIV/macaques model demonstrate the induction of SIV-specific polyfunctional CD4+ and CD8+ T cell activity resulting in marked decreases in viral load using DNA or viral vector vaccine strategy as either post-infection therapy or prophylaxis against SIV (117–120). Epidermal co-delivery of SIV Gag, RT, Nef, and envelope (Env) DNA vaccine formulated with a mucosal genetic adjuvant induced a substantial decrease in peripheral and mucosal viral burden in chronically infected macaques (117). Durable and sustained suppression of viral load and non-progression was positively associated with significant increases in SIV-specific IFNγ responses in T cells from peripheral blood (117, 118) and gut mucosa (117). In addition, vaccination induced SIV-specific CD8+ T cells with dual TNF-α and cytolytic effector functions in peripheral blood (117). In vectored vaccine studies, rhesus cytomegalovirus vector expressing HIV-1 Gag, Pol, Nef, Env, RT, and integrase sequences demonstrated a high frequency of CD69+IFNγ+ and/or CD69+TNFα+ CD4+ and CD8+ effector memory T cell response that correlated to control of highly pathogenic SIVmac239 infection after mucosal challenge (119). The suppression of peak viral load and protection from challenge correlated with the magnitude of the peak SIV-specific CD8+ T cell responses in the acute phase post-vaccination (described as the vaccine phase). Thirteen of the 24 vaccinated macaques had undetectable plasma viral loads that persisted up to 1 year (119). These responses have been demonstrated in distinct MHC-I and -II restricted CD8+ T cells, suggesting distinct patterns of epitope recognition in these T cell subsets (120). These studies outline the importance of the polyfunctional T cell responses in suppression of viral load in chronic infection and prevention of virus rebound after cessation of ART, and in the prophylaxis against AIDS viruses.
The use of IFNγ as cytokine adjuvant for HIV-1 vaccines has been studied more extensively in animal models of AIDS than in humans. The rationale for using IFNγ is based on its ability to promote CTL and NK cell activities as well as antibody production including induction of isotype switching (6, 42, 43, 121–124). Many cytokines, including those of IFNγ and IFNγ-inducing cytokine (IL-12), have been used as a genetic-based cytokine adjuvant to enhance lentiviral DNA vaccines (108, 125). SIV/macaque and feline immunodeficiency virus (FIV)/cat AIDS models have been used extensively to determine the effect of cytokine as an adjuvant (Table 3) (108–111, 125). In one study, a vaccine consisting of SIV and IFNγ DNA constructs was more effective against challenge with heterologous SIV than SIV DNA vaccine alone (109). However, a later SIV/macaque study was unable to confirm the results from the initial study (108). Furthermore, in FIV/cat model, laboratory cats vaccinated with FIV proviral deletion mutant (Δvif or ΔRT) and IFNγ DNA construct conferred either no protection (FIVΔvif/IFNγ) or marginal protection (FIVΔRT/IFNγ) against heterologous FIV challenge when compared to cats vaccinated with FIV DNA construct alone (110, 111).
Cytokine adjuvant is more commonly used in DNA vaccines to enhance the low immune responses generated by the low viral antigen expression of the viral DNA (126, 127). Nevertheless, cytokine-adjuvant studies have been performed with protein-based FIV vaccines containing conventional adjuvant. These studies show no (IFNγ and IL-18) to moderate (IL-12 and -15) enhancement of protective activity of the viral immunogen or inactive whole-virus FIV when compared to those without cytokine (Table 3) (128, 129). In one study, laboratory cats immunized with inactivated FIV vaccine supplemented with IFNγ in conventional adjuvant did not augment the protection observed with the vaccine without IFNγ (Pu and Yamamoto, unpublished observation). The inability of IFNγ to enhance the vaccine efficacy may be attributed to the fact that AIDS lentiviral proteins themselves can induce IFNγ production in T cells as observed in animals vaccinated with viral protein or inactivated virus in conventional adjuvant (128, 130–133). Similarly, many of the HIV-1 proteins and peptides (core p24, enzyme RT, accessory Nef, and envelope gp120) can directly stimulate T cells from HIV+ subjects or vaccinated HIV-negative subjects to produce IFNγ (78, 79, 133–135). For these reasons, the use of IFNγ as a cytokine adjuvant is unlikely to enhance the activities of an HIV-1 protein vaccine.
The last four major HIV-1 vaccine trials in humans consisted of two phase III vaccine trials using recombinant HIV-1 envelope gp120 protein of subtype B (VaxGen 004 trial) and subtypes B and E combined (VacGen 003) (135, 136); phase IIb STEP trial using adenovirus-5 vector (Ad5) expressing subtype-B HIV-1 gag/pol/nef (137); and phase III RV144 prime-boost trial consisting of canarypox vectored HIV-1 gag/pr/gp41–120 priming and boosting with subtypes B and E recombinant gp120 proteins (114, 115). More importantly, among the four trials only the prime-boost RV144 trial had some efficacy. The RV144 trial had an efficacy of 31.2% in a general population but the efficacy of only 3.7% in the high risk group. In contrast, VaxGen gp120 trials had neither efficacy nor adverse effects (135, 136), while the STEP Ad5-vectored gag/pol/nef trial showed more HIV-1 infection in vaccinated subjects than placebo immunized subjects (137). All of the above vaccines induced HIV-specific IFNγ responses from either CD4+ or CD8+ T cells and the duration of IFNγ expression varied between the trials (Table 3) (112, 113, 116, 138).
In the initial report on RV144 trial, the CD4+ and CD8+ T cells of the vaccinees were evaluated for IFNγ ELISpot and IFNγ/IL-2-specific ICS responses to HIV-1 Gag and Env (115). Only IFNγ/IL-2-specific ICS to Env in the CD4+ T cells were significantly higher (p < 0.001) in the vaccinated group than in the placebo group (Table 3) (115). A more extensive immune-correlated analysis of RV144 trial demonstrated that the binding of IgG antibodies to variable regions 1 and 2 (V1, V2) of HIV-1 Env inversely correlated with the rate of HIV-1 infection (p = 0.02) (114). In contrast, the binding of plasma IgA antibodies to Env correlated positively with the rate of HIV-1 infection. Moreover, HIV-1 neutralizing antibodies, T cell responses, and specifically T cell produced IFNγ responses that were detected in the vaccinees did not significantly affect the HIV-1 infection rate (114). A more extensive analysis of T cell activity indicated that the prime-boost vaccination induced polyfunctional (IFNγ+, IL-2+, and/or TNFα+) and potentially cytolytic (cytolytic marker CD107a+) CD4+ T cell responses to HIV-1 Env peptides, including the V2 peptides, 6 months after the last immunization (116). Thus, the most promising RV144 trial demonstrated the importance of IFNγ in detecting polyfunctional T cells.
The difficulty in correlating serum IFNγ levels with HIV/AIDS clinical status has been attributed to the role that IFNγ plays as an inflammatory cytokine as well as a cytokine that enhances antiviral immunity. During the acute stage of HIV-1 infection, the host immune system mounts an inflammatory response resulting in a cytokine storm. In the cytokine storm, a number of inflammatory cytokines including IFNγ are produced which decrease as the adaptive immune responses against HIV-1 develop. If not appropriately controlled, such inflammatory activities can enhance HIV-1 infection and may cause a higher viral set point before T cell immunity can control the HIV-1 load. Remarkably, low levels of IFNγ are detected throughout the course of HIV-1 infection correlating with persistently increasing HIV-1 load. Furthermore, many of the HIV-1 proteins can directly stimulate T cells from HIV+ subjects to produce IFNγ, leading to chronic immune activation and ultimately the exhaustion of the immune system and resulting in the loss of IFNγ production.
Interferon-γ therapy had no effect on HIV-1 load or AIDS progression whereas ART had a dramatic effect on both. However, long-term ART did not completely restore the immune responses in HIV-1 or completely eradicate the opportunistic infections. As a result, IFNγ alone or in combination with other cytokines has successfully been used together with ART against HIV-associated opportunistic infections. Lastly, polyfunctional CD4+ T cells that expressed IFNγ were observed in the vaccines of the most effective HIV-1 vaccine (RV144) trial to date. Hence, IFNγ may still play an important role as a product of HIV-specific polyfunctional CD4+ T cells which may serve to enhance the anti-HIV antibody production as well as CTLs against HIV-1.
The authors declare that the research was conducted in the absence of any commercial or financial relationships that could be construed as a potential conflict of interest.
This work was supported by R01-AI30904 (Janet K. Yamamoto) and Janet K. Yamamoto Miscellaneous Donors Fund.
1. Spellberg B, Edwards JE Jr. Type 1/type 2 immunity in infectious diseases. Clin Infect Dis (2001) 32(1):76–102. doi: 10.1086/317537
2. Frucht DM, Fukao T, Bogdan C, Schindler H, O’Shea JJ, Koyasu S. IFN-gamma production by antigen-presenting cells: mechanisms emerge. Trends Immunol (2001) 22(10):556–60. doi:10.1016/S1471-4906(01)02005-1
3. Harris DP, Goodrich S, Gerth AJ, Peng SL, Lund FE. Regulation of IFN-gamma production by B effector 1 cells: essential roles for T-bet and the IFN-gamma receptor. J Immunol (2005) 174(11):6781–90.
4. Koirala J, Adamski A, Koch L, Stueber D, El-Azizi M, Khardori NM, et al. Interferon-gamma receptors in HIV-1 infection. AIDS Res Hum Retroviruses (2008) 24(8):1097–102. doi:10.1089/aid.2007.0261
5. Cobos Jiménez V, Booiman T, de Taeye SW, van Dort KA, Rits MA, Hamann J, et al. Differential expression of HIV-1 interfering factors in monocyte-derived macrophages stimulated with polarizing cytokines or interferons. Sci Rep (2012) 2:763. doi:10.1038/srep00763
6. Boehm U, Klamp T, Groot M, Howard JC. Cellular responses to interferon-gamma. Annu Rev Immunol (1997) 15:749–95. doi:10.1146/annurev.immunol.15.1.749
7. Kumar SB, Rice CE, Milner DA Jr, Ramirez NC, Ackerman WE IV, Mwapasa V, et al. Elevated cytokine and chemokine levels in the placenta are associated with in-utero HIV-1 mother-to-child transmission. AIDS (2012) 26:685–94. doi:10.1097/QAD.0b013e3283519b00
8. John-Stewart GC, Mbori-Ngacha D, Payne BL, Farquhar C, Richardson BA, Emery S, et al. HIV-1-specific cytotoxic T lymphocytes and breast milk HIV-1 transmission. J Infect Dis (2009) 199:889–98. doi:10.1086/597120
9. Lohman-Payne B, Slyker JA, Moore S, Maleche-Obimbo E, Wamalwa DC, Richardson BA, et al. Breast milk cellular HIV-specific interferon gamma responses are associated with peripartum HIV transmission. AIDS (2012) 26:2007–16. doi:10.1097/QAD.0b013e328359b7e0
10. Lohman BL, Slyker JA, Richardson BA, Farquhar C, Mabuka JM, Crudder C, et al. Longitudinal assessment of human immunodeficiency virus type 1 (HIV-1)-specific gamma interferon responses during the first year of life in HIV-1-infected infants. J Virol (2005) 79(13):8121–30. doi:10.1128/JVI.79.13.8121-8130.2005
11. Legrand FA, Nixon DF, Loo CP, Ono E, Chapman JM, Miyamoto M, et al. Strong HIV-1 specific T cell responses in HIV-1 exposed uninfected infants and neonates revealed after regulatory T cell removal. PLoS One (2006) 20(1):e102. doi:10.1371/journal.pone.0000102
12. Kaul R, Rowland-Jones SL, Kimani J, Dong R, Yang H-B, Kiama P, et al. Late seroconversion in HIV-resistant Nairobi prostitutes despite pre-existing HIV-specific CD8+ responses. J Clin Invest (2001) 107(3):341–9. doi:10.1172/JCI10714
13. Stacey AR, Norris PJ, Qin L, Haygreen EA, Taylor E, Heitman J, et al. Induction of a striking systemic cytokine cascade prior to peak viremia in acute human immunodeficiency virus type 1 infection, in contrast to more modest and delated responses in acute hepatitis B and C virus infections. J Virol (2009) 83:3719–33. doi:10.1128/JVI.01844-08
14. Cao J, McNevin J, Holte S, Fink L, Corey L, McElrath MJ. Comprehensive analysis of human immunodeficiency virus type 1 (HIV-1)-specific gamma interferon-secreting CD8+ T-cells in primary HIV-1 infection. J Virol (2003) 77:6867–78. doi:10.1128/JVI.77.12.6867-6878.2003
15. Dalod M, Dupuis M, Deschemin JC, Goujard C, Deveau C, Meyer L, et al. Weak anti-HIV CD8+ T-cell effector activity in HIV primary infection. J Clin Invest (1999) 104:1431–9. doi:10.1172/JCI7162
16. Lieberman J. Tracking the killers: how should we measure CD8 T cells in HIV infection? AIDS (2004) 18:1489–93. doi:10.1097/01.aids.0000131320.75396.4d
17. Zanussi S, D’Andrea MD, Simonelli C, Tirelli U, DePaoli P. Serum levels of RANTES and MIP-1α in HIV-positive long-term survivors and progressor patients. AIDS (1996) 10:1431–2. doi:10.1097/00002030-199610000-00018
18. Harrer T, Harrer E, Kalams SA, Barbosa P, Trocha A, Johnson RP, et al. Cytotoxic T lymphocytes in asymptomatic long-term nonprogressing HIV-1 infection. Breadth and specificity of the response and relation to in vivo viral quasispecies in a person with prolonged infection and low viral load. J Immunol (1996) 156:2616–23.
19. Rinaldo C, Huang XL, Fan ZF, Ding M, Beltz L, Logar A, et al. High levels of anti-human immunodeficiency virus type-1 (HIV-1) memory cytotoxic T-lymphocyte activity and low viral load are associated with lack of disease in HIV-1 infected long-term nonprogressors. J Virol (1995) 69:5838–42.
20. Watanabe D, Uehira T, Yonemoto H, Bando H, Ogawa Y, Yajima K, et al. Sustained high levels of serum interferon-gamma during HIV-1 infection: a specific trend different from other cytokines. Viral Immunol (2010) 23(6):619–25. doi:10.1089/vim.2010.0065
21. Naranbhai V, Abdool Karim SS, Altfeld M, Samsunder N, Durgiah R, Sibeko S, et al. Innate immune activation enhances HIV acquisition in women, diminishing the effectiveness of tenofovir microbicide gel. J Infect Dis (2012) 206(7):993–1001. doi:10.1093/infdis/jis465
22. Lieberman J, Shankar P, Manjunath N, Andersson J. Dressed to kill? A review of why antiviral CD8 T lymphocytes fail to prevent progressive immunodeficiency in HIV-1 infection. Blood (2001) 98:1667–77. doi:10.1182/blood.V98.6.1667
23. Roberts L, Passmore JA, Williamson C, Little F, Bebell LM, Mlisana K, et al. Plasma cytokine levels during acute HIV-1 infection predict HIV disease progression. AIDS (2010) 24:819–31. doi:10.1097/QAD.0b013e3283367836
24. Isaacs A, Lindermann J. Virus interference. I. The interferon. Proc R Soc Lond B Biol Sci (1957) 147:258–67. doi:10.1098/rspb.1957.0048
25. Samuel CE. Antiviral actions of interferons. Clin Microbiol Rev (2001) 14(4):778–809. doi:10.1128/CMR.14.4.778-809.2001
26. Schroeder J, Hertzog PJ, Ravasi T, Hume DA. Interferon-γ: an overview of signals, mechanisms and functions. J Leukoc Biol (2004) 75:163–89. doi:10.1189/jlb.0603252
27. Polyak MJ, Vivithanaporn P, Maingat FG, Walsh JG, Branton W, Cohen EA, et al. Differential type 1 interferon-regulated gene expression in the brain during AIDS: interactions with viral diversity and neurovirulence. FASEB J (2013) 27(7):2829–44. doi:10.1096/fj.13-227868
28. Tokarev A, Skasko M, Fitzpatrick K, Guatelli J. Antiviral activity of the interferon-induced cellular protein BST-2/tetherin. AIDS Res Hum Retroviruses (2009) 25(12):1197–210. doi:10.1089/aid.2009.0253
29. Simm M. The innate cellular responses to HIV-1 invasion: emerging molecules of ancient-defense mechanisms. Arch Immunol Ther Exp (Warsz) (2007) 55(3):131–8. doi:10.1007/s00005-007-0023-9
30. Bitzegeio J, Sampias M, Bieniasz PD, Hatziioannou T. Adaptation to the interferon-induced antiviral state by human and simian immunodeficiency viruses. J Virol (2013) 87(6):3549–60. doi:10.1128/JVI.03219-12
31. Yamamoto JK, Barré-Sinoussi F, Bolton V, Pedersen NC, Gardner MB. Human alpha- and beta-interferon but not gamma-suppress the in vitro replication of LAV, HTLV-III, and ARV-2. J Interferon Res (1986) 6(2):143–52. doi:10.1089/jir.1986.6.143
32. Kirchner H. The interferon system as an integral part of the defense system against infections. Antiviral Res (1986) 6(1):1–17. doi:10.1016/0166-3542(86)90035-5
33. Mackewicz CE, Ortega H, Levy JA. Effect of cytokines on HIV replication in CD4+ lymphocytes: lack of identity with the CD8+ cell antiviral factor. Cell Immunol (1994) 153(2):329–43. doi:10.1006/cimm.1994.1032
34. Matsuyama T, Kobayashi N, Yamamoto N. Cytokines and HIV infection: is AIDS a tumor necrosis factor disease? AIDS (1991) 5(12):1405–17. doi:10.1097/00002030-199112000-00001
35. Levy JA, editor. Effect of HIV on various tissues and organ systems in the host. 3rd ed. Chap. 5. HIV and the Pathogenesis of AIDS. Washington DC: ASM Press (2007). p. 165–207.
36. Borrow P, Lewicki H, Hahn BH, Shaw GM, Oldstone MB. Virus-specific CD8+ cytotoxic T-lymphocyte activity associated with control of viremia in primary human immunodeficiency virus type 1 infection. J Virol (1994) 68(9):6103–10.
37. Yang OO, Daar ES, Ng HL, Shih R, Jamieson BD. Increasing CTL targeting of conserved sequences during early HIV-1 infection is correlated to decreasing viremia. AIDS Res Hum Retroviruses (2011) 27(4):391–8. doi:10.1089/aid.2010.0183
38. Leeansyah E, Malone DF, Anthony DD, Sandberg JK. Soluble biomarkers of HIV transmission, disease progression and comorbidities. Curr Opin HIV AIDS (2013) 8(2):117–24. doi:10.1097/COH.0b013e32835c7134
39. Katsikis PD, Mueller YM, Villinger F. The cytokine network of acute HIV infection: a promising target for vaccines and therapy to reduce viral set-point? PLoS Pathog (2011) 7(8):e1002055. doi:10.1371/journal.ppat.1002055
40. Sterling TR, Vlahov D, Astemborski J, Hoover DR, Margolick JB, Quinn TC. Initial plasma HIV-1 RNA levels and progression to AIDS in women and men. N Engl J Med (2001) 344(10):720–5. doi:10.1056/NEJM200103083441003
41. Lavreys L, Baeten JM, Chohan V, McClelland RS, Hassan WM, Richardson BA, et al. Higher set point plasma viral load and more-severe acute HIV type 1 (HIV-1) illness predict mortality among high-risk HIV-1-infected African women. Clin Infect Dis (2006) 42(9):1333–9. doi:10.1086/503258
42. Gromo G, Geller RL, Inverardi L, Bach FH. Signal requirements in the step-wise functional maturation of cytotoxic T lymphocytes. Nature (1987) 327(6121):424–6. doi:10.1038/327424a0
43. Maraskovsky E, Chen WF, Shortman K. IL-2 and IFN-gamma are two necessary lymphokines in the development of cytolytic T cells. J Immunol (1989) 143(4):1210–4.
44. Soghoian DZ, Jessen H, Flanders M, Sierra-Davidson K, Cutler S, Pertel T, et al. HIV-specific cytolytic CD4 T cell responses during acute HIV infection predict disease outcome. Sci Transl Med (2012) 4(123):123ra25. doi:10.1126/scitranslmed.3003165
45. Betts MR, Krowka JF, Kepler TB, Davidian M, Christopherson C, Kwok S, et al. Human immunodeficiency virus type 1-specific cytotoxic T lymphocyte activity is inversely correlated with HIV type 1 viral load in HIV type 1-infected long-term survivors. AIDS Res Hum Retroviruses (1999) 15(13):1219–28. doi:10.1089/088922299310313
46. Blankson JN. Effector mechanisms in HIV-1 infected elite controllers: highly active immune responses? Antiviral Res (2010) 85(1):295–302. doi:10.1016/j.antiviral.2009.08.007
47. Deeks SG, Walker BD. Human immunodeficiency virus controllers: mechanisms of durable virus control in the absence of antiretroviral therapy. Immunity (2007) 27(3):406–16. doi:10.1016/j.immuni.2007.08.010
48. McNicholl JM, Promadej N. Insights into the role of host genetic and T-cell factors in resistance to HIV transmission from studies of highly HIV-exposed Thais. Immunol Res (2004) 29(1–3):161–74. doi:10.1385/IR:29:1-3:161
49. Agosti JM, Coombs RW, Collier AC, Paradise MA, Benedetti JK, Jaffe HS, et al. A randomized, double-blind, phase I/II trial of tumor necrosis factor and interferon-gamma for treatment of AIDS-related complex (Protocol 025 from the AIDS Clinical Trials Group). AIDS Res Hum Retroviruses (1992) 8(5):581–7. doi:10.1089/aid.1992.8.581
50. Kaplan LD, Abrams DI, Sherwin SA, Kahn J, Volberding PA. A phase I/II study of recombinant tumor necrosis factor and recombinant interferon gamma in patients with AIDS-related complex. Biotechnol Ther (1989–1990) 1(3):229–36.
51. Lane HC, Davey RT Jr, Sherwin SA, Masur H, Rook AH, Manischewitz JF, et al. A phase I trial of recombinant human interferon-gamma in patients with Kaposi’s sarcoma and the acquired immunodeficiency syndrome (AIDS). J Clin Immunol (1989) 9(4):351–61. doi:10.1007/BF00918667
52. Heagy W, Groopman J, Schindler J, Finberg R. Use of IFN-gamma in patients with AIDS. J Acquir Immune Defic Syndr (1990) 3(6):584–90.
53. Krown SE. Interferon-alpha: evolving therapy for AIDS-associated Kaposi’s sarcoma. J Interferon Cytokine Res (1998) 18(4):209–14. doi:10.1089/jir.1998.18.209
54. Krown SE. Interferon and other biologic agents for the treatment of Kaposi’s sarcoma. Hematol Oncol Clin North Am (1991) 5(2):311–22.
55. Shearer WT, Kline MW, Abramson SL, Fenton T, Starr SE, Douglas SD. Recombinant human gamma interferon in human immunodeficiency virus-infected children: safety, CD4(+)-lymphocyte count, viral load, and neutrophil function (AIDS Clinical Trials Group Protocol 211). Clin Diagn Lab Immunol (1999) 6(3):311–5.
56. Valdez H, Lederman MM. Cytokines and cytokine therapies in HIV infection. AIDS Clin Rev (1997)1997-1998:187–228.
57. Poli G, Biswas P, Fauci AS. Interferons in the pathogenesis and treatment of human immunodeficiency virus infection. Antiviral Res (1994) 24(2–3):221–33. doi:10.1016/0166-3542(94)90069-8
58. Asmuth DM, Murphy RL, Rosenkranz SL, Lertora JJ, Kottilil S, Cramer Y, et al. AIDS Clinical Trials Group A5192 Team. Safety, tolerability, and mechanisms of antiretroviral activity of pegylated interferon Alfa-2a in HIV-1 monoinfected participants: a phase II clinical trial. J Infect Dis (2010) 201(11):1686–96. doi:10.1086/652420
59. Aldenhoven M, Barlo NP, Sanders CJ. Therapeutic strategies for epidemic Kaposi’s sarcoma. Int J STD AIDS (2006) 17(9):571–8. doi:10.1258/095646206778113131
60. Aversa SM, Cattelan AM, Salvagno L, Crivellari G, Banna G, Trevenzoli M, et al. Treatments of AIDS-related Kaposi’s sarcoma. Crit Rev Oncol Hematol (2005) 53(3):253–65. doi:10.1016/j.critrevonc.2004.10.009
61. Pappas PG, Bustamante B, Ticona E, Hamill RJ, Johnson PC, Reboli A, et al. Recombinant interferon-gamma 1b as adjunctive therapy for AIDS-related acute cryptococcal meningitis. J Infect Dis (2004) 189(12):2185–91. doi:10.1086/420829
62. Jarvis JN, Meintjes G, Rebe K, Williams GN, Bicanic T, Williams A, et al. Adjunctive interferon-γ immunotherapy for the treatment of HIV-associated cryptococcal meningitis: a randomized controlled trial. AIDS (2012) 26(9):1105–13. doi:10.1097/QAD.0b013e3283536a93
63. Gamaletsou MN, Sipsas NV, Kontoyiannis DP, Tsiakalos A, Kontos AN, Stefanou I, et al. Successful salvage therapy of refractory HIV-related cryptococcal meningitis with the combination of liposomal amphotericin B, voriconazole, and recombinant interferon-γ. Diagn Microbiol Infect Dis (2012) 74(4):409–11. doi:10.1016/j.diagmicrobio.2012.08.009
64. Riddell LA, Pinching AJ, Hill S, Ng TT, Arbe E, Lapham GP, et al. A phase III study of recombinant human interferon gamma to prevent opportunistic infections in advanced HIV disease. AIDS Res Hum Retroviruses (2001) 17(9):789–97. doi:10.1089/088922201750251981
65. Kedzierska K, Azzam R, Ellery P, Mak J, Jaworowski A, Crowe SM. Defective phagocytosis by human monocyte/macrophages following HIV-1 infection: underlying mechanisms and modulation by adjunctive cytokine therapy. J Clin Virol (2003) 26(2):247–63. doi:10.1016/S1386-6532(02)00123-3
66. Squires KE, Brown ST, Armstrong D, Murphy WF, Murray HW. Interferon-gamma treatment for Mycobacterium avium-intracellular complex bacillemia in patients with AIDS. J Infect Dis (1992) 166(3):686–7. doi:10.1093/infdis/166.3.686
67. Murray HW. Current and future clinical applications of interferon-gamma in host antimicrobial defense. Intensive Care Med (1996) 22(Suppl 4):S456–61. doi:10.1007/BF01743724
68. Gasser O, Brander C, Wolbers M, Brown NV, Rauch A, Günthard HF, et al. Cohort Study. Expansion of interferon-γ-secreting HIV-specific T cells during successful antiretroviral therapy. HIV Med (2013) 14(4):241–6. doi:10.1111/j.1468-1293.2012.01040.x
69. Harari A, Petitpierre S, Vallelian F, Pantaleo G. Skewed representation of functionally distinct populations of virus-specific CD4 T cells in HIV-1-infected subjects with progressive disease: changes after antiretroviral therapy. Blood (2004) 103(3):966–72. doi:10.1182/blood-2003-04-1203
70. Trabattoni D, Piconi S, Biasin M, Rizzardini G, Migliorino M, Seminari E, et al. Granule-dependent mechanisms of lysis are defective in CD8 T cells of HIV-infected, antiretroviral therapy-treated individuals. AIDS (2004) 18(6):859–69. doi:10.1097/00002030-200404090-00003
71. Casazza JP, Betts MR, Picker LJ, Koup RA. Decay kinetics of human immunodeficiency virus-specific CD8+ T cells in peripheral blood after initiation of highly active antiretroviral therapy. J Virol (2001) 75(14):6508–16. doi:10.1128/JVI.75.14.6508-6516.2001
72. Orsilles MA, Pieri E, Cooke P, Caula C. IL-2 and IL-10 serum levels in HIV-1-infected patients with or without active antiretroviral therapy. APMIS (2006) 114(1):55–60. doi:10.1111/j.1600-0463.2006.apm_108.x
73. Stylianou E, Aukrust P, Kvale D, Müller F, Frøland SS. IL-10 in HIV infection: increasing serum IL-10 levels with disease progression – down-regulatory effect of potent anti-retroviral therapy. Clin Exp Immunol (1999) 116(1):115–20.
74. Stylianou E, Bjerkeli V, Yndestad A, Heggelund L, Waehre T, Damås JK, et al. Raised serum levels of interleukin-18 is associated with disease progression and may contribute to virological treatment failure in HIV-1-infected patients. Clin Exp Immunol (2003) 132(3):462–6. doi:10.1046/j.1365-2249.2003.02179.x
75. Torre D, Speranza F, Martegani R, Pugliese A, Castelli F, Basilico C, et al. Circulating levels of IL-18 in adult and paediatric patients with HIV-1 infection. AIDS (2000) 14(14):2211–2. doi:10.1097/00002030-200009290-00023
76. Iannello A, Tremblay C, Routy JP, Boulassel MR, Toma E, Ahmad A. Decreased levels of circulating IL-21 in HIV-infected AIDS patients: correlation with CD4+ T-cell counts. Viral Immunol (2008) 21(3):385–8. doi:10.1089/vim.2008.0025
77. Iannello A, Boulassel MR, Samarani S, Debbeche O, Tremblay C, Toma E, et al. Dynamics and consequences of IL-21 production in HIV-infected individuals: a longitudinal and cross-sectional study. J Immunol (2010) 184(1):114–26. doi:10.4049/jimmunol.0901967
78. Streeck H, Frahm N, Walker BD. The role of IFN-gamma Elispot assay in HIV vaccine research. Nat Protoc (2009) 4(4):461–9. doi:10.1038/nprot.2009.7
79. Addo MM, Yu XG, Rathod A, Cohen D, Eldridge RL, Strick D, et al. Comprehensive epitope analysis of human immunodeficiency virus type 1 (HIV-1)-specific T-cell responses directed against the entire expressed HIV-1 genome demonstrate broadly directed responses, but no correlation to viral load. J Virol (2003) 77(3):2081–92. doi:10.1128/JVI.77.3.2081-2092.2003
80. Streeck H, Nixon DF. T cell immunity in acute HIV-1 infection. J Infect Dis (2010) 202(Suppl 2):S302–8. doi:10.1086/655652
81. Boaz MJ, Waters A, Murad S, Easterbrook PJ, Vyakarnam A. Presence of HIV-1 Gag-specific IFN-gamma+IL-2+ and CD28+IL-2+ CD4 T cell responses is associated with nonprogression in HIV-1 infection. J Immunol (2002) 169(11):6376–85.
82. Boaz MJ, Waters A, Murad S, Easterbrook PJ, D’Sousa E, van Wheeley C, et al. CD4 responses to conserved HIV-1 T helper epitopes show both negative and positive associations with virus load in chronically infected subjects. Clin Exp Immunol (2003) 134(3):454–63. doi:10.1111/j.1365-2249.2003.02307.x
83. Richmond M, McKinnon LR, Kiazyk SA, Wachihi C, Kimani M, Kimani J, et al. Epitope mapping of HIV-specific CD8+ T cell responses by multiple immunological readouts reveals distinct specificities defined by function. J Virol (2011) 85(3):1275–86. doi:10.1128/JVI.01707-10
84. Zanussi S, Simonelli C, D’Andrea M, Caffau C, Clerici M, Tirelli U, et al. CD8+ lymphocyte phenotype and cytokine production in long-term non-progressor and in progressor patients with HIV-1 infection. Clin Exp Immunol (1996) 105(2):220–4.
85. Rehr M, Cahenzli J, Haas A, Price DA, Gostick E, Huber M, et al. Emergence of polyfunctional CD8+ T cells after prolonged suppression of human immunodeficiency virus replication by antiretroviral therapy. J Virol (2008) 82(7):3391–404. doi:10.1128/JVI.02383-07
86. McQuillan GM, Kruszon-Moran D, Kottiri BJ, Kamimoto LA, Lam L, Cowart MF, et al. Prevalence of HIV in the US household population: the National Health and Nutrition Examination Surveys, 1988 to 2002. J Acquir Immune Defic Syndr (2006) 41(5):651–6. doi:10.1097/01.qai.0000194235.31078.f6
87. World Health Organization (WHO). Towards Universal Access: Scaling Up Priority HIV/AIDS Interventions in the Health Sector. Progress report (2009). Available from: http://www.who.int/hiv/pub/2009progressreport/en
88. Boyd MA, Hill AM. Clinical management of treatment-experienced, HIV/AIDS patients in the combination antiretroviral therapy era. Pharmacoeconomics (2010) 28(Suppl 1):17–34. doi:10.2165/11587420-000000000-00000
89. Zolopa AR. The evolution of HIV treatment guidelines: current state-of-the-art of ART. Antiviral Res (2010) 85(1):241–4. doi:10.1016/j.antiviral.2009.10.018
90. Guihot A, Tubiana R, Breton G, Marcelin AG, Samri A, Assoumou L, et al. Immune and virological benefits of 10 years of permanent viral control with antiretroviral therapy. AIDS (2010) 24(4):614–7. doi:10.1097/QAD.0b013e32833556f3
91. Lok JJ, Bosch RJ, Benson CA, Collier AC, Robbins GK, Shafer RW, et al. Long-term increase in CD4+ T-cell counts during combination antiretroviral therapy for HIV-1 infection. AIDS (2010) 24(12):1867–76. doi:10.1097/QAD.0b013e32833adbcf
92. Gupta A, Wood R, Kaplan R, Bekker LG, Lawn SD. Tuberculosis incidence rates during 8 years of follow-up of an antiretroviral treatment cohort in South Africa: comparison with rates in the community. PLoS One (2012) 7(3):e34156. doi:10.1371/journal.pone.0034156
93. Markowitz N, Lopardo G, Wentworth D, Gey D, Babiker A, Fox L, et al. Long-term effects of intermittent IL-2 in HIV infection: extended follow-up of the INSIGHT STALWART Study. PLoS One (2012) 7(10):e47506. doi:10.1371/journal.pone.0047506
94. Sternfeld T, Nigg A, Belohradsky BH, Bogner JR. Treatment of relapsing Mycobacterium avium infection with interferon-gamma and interleukin-2 in an HIV-negative patient with low CD4 syndrome. Int J Infect Dis (2010) 14(Suppl 3):198–201. doi:10.1016/j.ijid.2009.08.004
95. Abzug MJ, Walsh TJ. Interferon-gamma and colony-stimulating factors as adjuvant therapy for refractory fungal infections in children. Pediatr Infect Dis J (2004) 23(8):769–73. doi:10.1097/01.inf.0000134314.65398.bf
96. Naidoo K, Yende-Zuma N, Padayatchi N, Naidoo K, Jithoo N, Nair G, et al. The immune reconstitution inflammatory syndrome after antiretroviral therapy initiation in patients with tuberculosis: findings from the SAPiT trial. Ann Intern Med (2012) 157(5):313–24. doi:10.7326/0003-4819-157-5-201209040-00004
97. Corey DM, Kim HW, Salazar R, Illescas R, Villena J, Gutierrez L, et al. Brief report: effectiveness of combination antiretroviral therapy on survival and opportunistic infections in a developing world setting: an observational cohort study. J Acquir Immune Defic Syndr (2007) 44(4):451–5. doi:10.1097/QAI.0b013e31802f8512
98. Koenig SP, Rodriguez LA, Bartholomew C, Edwards A, Carmichael TE, Barrow G, et al. Long-term antiretroviral treatment outcomes in seven countries in the Caribbean. J Acquir Immune Defic Syndr (2012) 59(4):60–71. doi:10.1097/QAI.0b013e318245d3c1
99. Armstrong WS. The immune reconstitution inflammatory syndrome: a clinical update. Curr Infect Dis Rep (2013) 15(1):39–45. doi:10.1007/s11908-012-0308-y
100. Rasul S, Delapenha R, Farhat F, Gajjala J, Zahra SM. Graves’ disease as a manifestation of immune reconstitution in HIV-infected individuals after initiation of highly active antiretroviral therapy. AIDS Res Treat (2011) 2011:743597. doi:10.1155/2011/743597
101. Sharma SK, Soneja M. HIV & immune reconstitution inflammatory syndrome (IRIS). Indian J Med Res (2011) 134(6):866–77. doi:10.4103/0971-5916.92632
102. Wiesner DL, Boulware DR. Cryptococcus-related immune reconstitution inflammatory syndrome (IRIS): pathogenesis and its clinical implications. Curr Fungal Infect Rep (2011) 5(4):252–61. doi:10.1007/s12281-011-0064-8
103. Grant PM, Komarow L, Lederman MM, Pahwa S, Zolopa AR, Andersen J, et al. Elevated interleukin 8 and T-helper 1 and T-helper 17 cytokine levels prior to antiretroviral therapy in participants who developed immune reconstitution inflammatory syndrome during ACTG A5164. J Infect Dis (2012) 206(11):1715–23. doi:10.1093/infdis/jis604
104. Harari A, Bart PA, Stöhr W, Tapia G, Garcia M, Medjitna-Rais E, et al. An HIV-1 clade C DNA prime, NYVAC boost vaccine regimen induces reliable, polyfunctional, and long-lasting T cell responses. J Exp Med (2008) 205(1):63–77. doi:10.1084/jem.20071331
105. Draenert R, Altfeld M, Brander C, Basgoz N, Corcoran C, Wurcel AG, et al. Comparison of overlapping peptide sets for detection of antiviral CD8 and CD4 T cell responses. J Immunol Methods (2003) 275(1–2):19–29. doi:10.1016/S0022-1759(02)00541-0
106. Baier-Bitterlich G, Fuchs D, Wachter H. Chronic immune stimulation, oxidative stress, and apoptosis in HIV infection. Biochem Pharmacol (1997) 53(6):755–63. doi:10.1016/S0006-2952(96)00651-X
107. Yang OO. Will we be able to “spot” an effective HIV-1 vaccine? Trends Immunol (2003) 24(2):67–72. doi:10.1016/S1471-4906(02)00034-0
108. Lena P, Villinger F, Giavedoni L, Miller CJ, Rhodes G, Luciw P. Co-immunization of rhesus macaques with plasmid vectors expressing IFN-gamma, GM-CSF, and SIV antigens enhances anti-viral humoral immunity but does not affect viremia after challenge with highly pathogenic virus. Vaccine (2002) 20(Suppl 4):A69–79. doi:10.1016/S0264-410X(02)00391-2
109. Giavedoni L, Ahmad S, Jones L, Yilma T. Expression of gamma interferon by simian immunodeficiency virus increases attenuation and reduces postchallenge virus load in vaccinated rhesus macaques. J Virol (1997) 71(2):866–72.
110. Hosie MJ, Flynn JN, Rigby MA, Cannon C, Dunsford T, Mackay NA, et al. DNA vaccination affords significant protection against feline immunodeficiency virus infection without inducing detectable antiviral antibodies. J Virol (1998) 72(9):7310–9.
111. Gupta S, Leutenegger CM, Dean GA, Steckbeck JD, Cole KS, Sparger EE. Vaccination of cats with attenuated feline immunodeficiency virus proviral DNA vaccine expressing gamma interferon. J Virol (2007) 81(2):465–73. doi:10.1128/JVI.00815-06
112. Jones NG, DeCamp A, Gilbert P, Peterson ML, Gurwith M, Cao H. AIDSVAX immunization induces HIV-specific CD8+ T-cell responses in high-risk, HIV-negative volunteers who subsequently acquire HIV infection. Vaccine (2009) 27(7):1136–40. doi:10.1016/j.vaccine.2008.11.071
113. Li F, Finnefrock AC, Dubey SA, Korber BT, Szinger J, Cole S, et al. Mapping HIV-1 vaccine induced T-cell responses: bias towards less-conserved regions and potential impact on vaccine efficacy in the Step study. PLoS One (2011) 6:e20479. doi:10.1371/journal.pone.0020479
114. de Souza MS, Ratto-Kim S, Chuenarom W, Schuetz A, Chantakulkij S, Nuntapinit B, et al. The Thai phase III trial (RV144) vaccine regimen induces T cell responses that preferentially target epitopes within the V2 region of HIV-1 envelope. J Immunol (2012) 188(10):5166–76. doi:10.4049/jimmunol.1102756
115. Rerks-Ngarm S, Pitisuttithum P, Nitayaphan S, Kaewkungwal J, Chiu J, Paris R, et al. Vaccination with ALVAC and AIDSVAX to prevent HIV-1 infection in Thailand. N Engl J Med (2009) 361(23):2209–20. doi:10.1056/NEJMoa0908492
116. Haynes BF, Gilbert PB, McElrath MJ, Zolla-Pazner S, Tomaras GD, Alam SM, et al. Immune-correlates analysis of an HIV-1 vaccine efficacy trial. N Engl J Med (2012) 366(14):1275–86. doi:10.1056/NEJMoa1113425
117. Fuller DH, Rajakumar P, Che JW, Narendran A, Nyaundi J, Michael H, et al. Therapeutic DNA vaccine induces broad T cell responses in the gut and sustained protection from viral rebound and AIDS in SIV-infected rhesus macaques. PLoS One (2012) 87(3):e33715. doi:10.1371/journal.pone.0033715
118. von Gegerfelt AS, Rosati M, Alicea C, Valentin A, Roth P, Bear J, et al. Long-lasting decrease in viremia in macaques chronically infected with simian immunodeficiency virus SIVmac251 after therapeutic DNA immunization. J Virol (2007) 81(4):1972–9. doi:10.1128/JVI.01990-06
119. Hansen SG, Ford JC, Lewis MS, Ventura AB, Colette M, Hughes CM, et al. Profound early control of highly pathogenic SIV by an effector-memory T cell vaccine. Nature (2011) 473(7348):523–7. doi:10.1038/nature10003
120. Hansen SG, Sacha JB, Hughes CM, Ford JC, Burwitz B, Scholz I, et al. Cytomegalovirus vectors violate CD8+ T cell epitope recognition paradigms. Science (2013) 340(6135):1237874. doi:10.1126/science.1237874
121. Blackman MJ, Morris AG. The effect of interferon treatment of targets on susceptibility to cytotoxic T-lymphocyte killing: augmentation of allogeneic killing and virus-specific killing relative to viral antigen expression. Immunology (1985) 56(3):451–7.
122. Mills FC, Brooker JS, Camerini-Otero RD. Sequences of human immunoglobulin switch regions: implications for recombination and transcription. Nucleic Acids Res (1990) 18(24):7305–16. doi:10.1093/nar/18.24.7305
124. Senik A, Stefanos S, Kolb JP, Lucero M, Falcoff E. Enhancement of mouse natural killer cell activity by type II interferon. Ann Immunol (Paris) (1980) 131c:349–61.
125. Halwani R, Boyer JD, Yassine-Diab B, Haddad EK, Robinson TM, Kumar S, et al. Therapeutic vaccination with simian immunodeficiency virus (SIV)-DNA + IL-12 or IL-15 induces distinct CD8 memory subsets in SIV-infected macaques. J Immunol (2008) 180(12):7969–79.
126. Muthumani K, Kudchodkar S, Zhang D, Bagarazzi ML, Kim JJ, Boyer JD, et al. Issues for improving multiplasmid DNA vaccines for HIV-1. Vaccine (2002) 20(15):1999–2003. doi:10.1016/S0264-410X(02)00086-5
127. Abdulhaqq SA, Weiner DB. DNA vaccines: developing new strategies to enhance immune responses. Immunol Res (2008) 42(1–3):219–32. doi:10.1007/s12026-008-8076-3
128. Coleman JK, Pu R, Martin M, Sato E, Yamamoto JK. HIV-1 p24 vaccine protects cats against feline immunodeficiency virus infection. AIDS (2005) 19(14):1457–66. doi:10.1097/01.aids.0000183627.81922.be
129. Coleman JK, Pu P, Martin MM, Noon-Song EN, Zwijnenberg R, Yamamoto JK. Feline immunodeficiency virus (FIV) vaccine and neutralizing antibodies against heterologous FIV subtype strains. Vaccine (2013). doi:10.1016/j.vaccine.2013.05.024
130. Abbott JR, Pu R, Coleman JK, Yamamoto JK. Utilization of feline ELISPOT for mapping vaccine epitopes. Methods Mol Biol (2012) 792:47–63. doi:10.1007/978-1-61779-325-7_4
131. Uhl EW, Martin M, Coleman JK, Yamamoto JK. Advances in FIV vaccine technology. Vet Immunol Immunopath (2008) 123:65–80. doi:10.1016/j.vetimm.2008.01.030
132. Van Braeckel E, Bourguignon P, Koutsoukos M, Clement F, Janssens M, Carletti I, et al. An adjuvanted polyprotein HIV-1 vaccine induces polyfunctional cross-reactive CD4+ T cell responses in seronegative volunteers. Clin Infect Dis (2011) 52(4):522–31. doi:10.1093/cid/ciq160
133. Mortara L, Gras-Masse H, Rommens C, Venet A, Guillet JG, Bourgault-Villada I. Type 1 CD4(+) T-cell help is required for induction of antipeptide multispecific cytotoxic T lymphocytes by a lipopeptidic vaccine in rhesus macaques. J Virol (1999) 73(5):4447–51.
134. Evans TG, Fitzgerald T, Gibbons DC, Keefer MC, Soucier H. Th1/Th2 cytokine responses following HIV-1 immunization in seronegative volunteers. The AIDS Vaccine Evaluation Group. Clin Exp Immunol (1998) 111(2):243–50.
135. Flynn NM, Forthal DN, Harro CD, Judson FN, Mayer KH, Para MF, et al. Placebo-controlled phase 3 trial of a recombinant glycoprotein 120 vaccine to prevent HIV-1 infection. J Infect Dis (2005) 191(5):654–65. doi:10.1086/428404
136. Pitisuttithum P, Gilbert P, Gurwith M, Heyward W, Martin M, van Griensven F, et al. Randomized, double-blind, placebo-controlled efficacy trial of a bivalent recombinant glycoprotein 120 HIV-1 vaccine among injection drug users in Bangkok, Thailand. J Infect Dis (2006) 194(12):1661–71. doi:10.1086/508748
137. Buchbinder SP, Mehrotra DV, Duerr A, Fitzgerald DW, Mogg R, Li D, et al. Efficacy assessment of a cell-mediated immunity HIV-1 vaccine (the Step Study): a double-blind, randomised, placebo-controlled, test-of-concept trial. Lancet (2008) 372(9653):1881–93. doi:10.1016/S0140-6736(08)61591-3
Keywords: interferon-γ, HIV-1 pathogenesis, IFNγ therapy, anti-retroviral therapy, HIV-1 vaccine
Citation: Roff SR, Noon-Song EN and Yamamoto JK (2014) The significance of interferon-γ in HIV-1 pathogenesis, therapy, and prophylaxis. Front. Immunol. 4:498. doi: 10.3389/fimmu.2013.00498
Received: 18 October 2013; Accepted: 17 December 2013;
Published online: 13 January 2014.
Edited by:
Howard M. Johnson, University of Florida, USAReviewed by:
So-Yon Lim, Harvard Medical School, USACopyright: © 2014 Roff, Noon-Song and Yamamoto. This is an open-access article distributed under the terms of the Creative Commons Attribution License (CC BY). The use, distribution or reproduction in other forums is permitted, provided the original author(s) or licensor are credited and that the original publication in this journal is cited, in accordance with accepted academic practice. No use, distribution or reproduction is permitted which does not comply with these terms.
*Correspondence: Janet K. Yamamoto, Department of Infectious Diseases and Pathology, College of Veterinary Medicine, University of Florida, P.O. Box 110880, Gainesville, FL 32611, USA e-mail:eWFtYW1vdG9AdWZsLmVkdQ==
Disclaimer: All claims expressed in this article are solely those of the authors and do not necessarily represent those of their affiliated organizations, or those of the publisher, the editors and the reviewers. Any product that may be evaluated in this article or claim that may be made by its manufacturer is not guaranteed or endorsed by the publisher.
Research integrity at Frontiers
Learn more about the work of our research integrity team to safeguard the quality of each article we publish.