- Department of Dermatology, Eberhard Karls University Tübingen, Tübingen, Germany
Mast cells (MC) are key mediators of the immune system, most prominently known for their role in eliciting harmful allergic reactions. Mast cell mediator release (e.g. by degranulation) is triggered by FcεRI recognition of antigen – IgE complexes. Until today no therapeutic targeting of this and other mast cell activation pathways is established. Among possible new candidates there are tetraspanins that have been described on MC already several years ago. Tetraspanins are transmembrane proteins acting as scaffolds, mediating local clustering of their interaction partners, and thus amplify their activities. More recently, tetraspanins were also found to exert intrinsic receptor functions. Tetraspanins have been found to be crucial components of fundamental biological processes like cell motility and adhesion. In immune cells, they not only boost the effectiveness of antigen presentation by clustering MHC molecules, they are also key players in all kinds of degranulation events and immune receptor clustering. This review focuses on the contribution of tetraspanins clustered with FcεRI or residing in granule membranes to classical MC functions but also undertakes an outlook on the possible contribution of tetraspanins to newly described mast cell functions and discusses possible targets for drug development.
Introduction
Tetraspanins are evolutionary conserved and are expressed ubiquitously (Levy and Shoham, 2005). They are composed of the four eponymous transmembrane (TM) domains, connected by a small and a large extracellular as well as an intracellular loop (Figure 1). The large extracellular loop consists of two subdomains, a conserved three-helix structure that contains a canonical CCG motif and a variable region that is inserted within the conserved subdomain and is responsible for some of the specific protein-protein interactions of individual tetraspanins. A stretch of residues located in the TM2 – intracellular loop – TM3 region is also highly conserved and referred to as the tetraspanin signature. In the conserved regions, there are a number of canonical cysteine residues that ether serve as palmitoylation sites or form disulfide bonds that establish the topology of the large extracellular region (Seigneuret et al., 2001). Tetraspanins have a different primary sequence and do not share any homologies with the multigene four-transmembrane family proteins (MS4A) that comprise, e.g., CD20 and the high-affinity IgE receptor β chain (FcεRIβ), although the secondary structure of these proteins also forms four transmembrane domains (Ishibashi et al., 2001). Tetraspanins are able to form homodimers and to cluster with a large number of transmembrane proteins like integrins, major histocompatibility complex proteins and Fc receptors, but also with intracellular signaling molecules like phosphatidylinositol-4-kinase or RhoA. The clustering of tetraspanins leads to the formation of distinct membrane domains called tetraspanin enriched microdomains (TEM; Figure 2). By spatial enrichment of their binding partners, they bring together interacting elements of signaling pathways and also cluster homotypic signaling, thus amplifying signaling amplitudes (Hemler, 2005; Yanez-Mo et al., 2009).
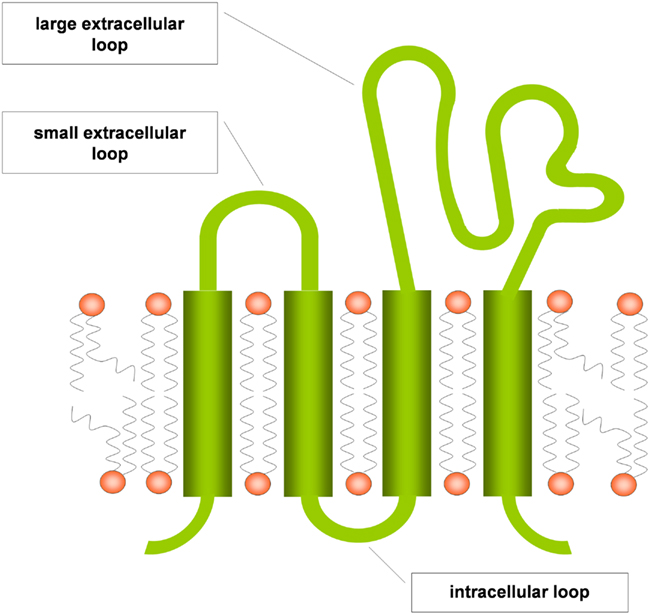
Figure 1. Tetraspanin structure scheme (modified from Levy and Shoham, 2005).
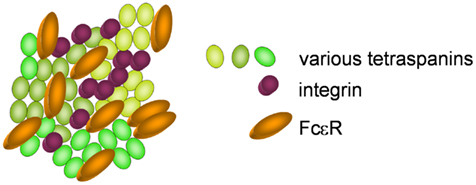
Figure 2. Clustering of various tetraspanins, with integrins and Fcε receptors, forming a tetraspanin enriched microdomain.
Tetraspanin research started in the 1980s. While some were soon established as important cell surface markers, others were long neglected. Major problems were that no mouse antibodies could be raised against some human tetraspanins or that no biological functions could be elucidated because initially no classical receptor/ligand functions were observed (Hemler, 2005; Yanez-Mo et al., 2009).
In the last years, exciting new functions have been elucidated for tetraspanins. Not only their role as scaffold proteins is increasingly appreciated, e.g., in antigen presentation by dendritic cells (Unternaehrer et al., 2007), but also direct action of tetraspanins as receptors has been described lately, e.g., for IL-16 (Qi et al., 2006) or hepatitis C virus entry (Pileri et al., 1998).
Mast cells (MC) are tissue-homing immune cells that differentiate from blood borne committed progenitor cells and have been identified in all vertebrate classes (Crivellato and Ribatti, 2010). Preferentially populating surface organ tissues such as the skin or mucosal surfaces in the gut, MC contribute to the first line defense system against pathogens (Echtenacher et al., 1996). The discovery that MC are much more than an effector cell of type I allergic reactions challenged a paradigm, but was soon confirmed by other studies (for review see (Sayed et al., 2008; Abraham and St John, 2010; Beghdadi et al., 2011). In addition, MC were shown to be crucial mediators of delayed type hypersensitivity reactions (DTHR) of the skin (Biedermann et al., 2000), joints (Lee et al., 2002; Kneilling et al., 2007) and brain (Secor et al., 2000).
While some of these MC functions were questioned recently based on the model systems used (Zhou et al., 2007; Dudeck et al., 2011; Feyerabend et al., 2011), the critical role of MC for cutaneous DTHR was confirmed in different models (Biedermann et al., 2000; Dudeck et al., 2011; Otsuka et al., 2011). Moreover, it was discovered that MC functions are determined by the surrounding microenviroment of their destination and different situations of, e.g., inflammation or regeneration allow MC to adapt “as needed” (Pennock and Grencis, 2004, for review see Hallgren and Gurish, 2007; Collington et al., 2011). Signals and pathways involved in these adaptive processes have not been well characterized. In contrast, molecular pathways triggered by IgE-mediated MC activation have been studied in detail.
In mammals, MC express tetrameric high-affinity receptors for IgE (FcεRI) as well as stem cell factor receptors (c-kit, CD117) on the cell surface. Signaling events downstream of FcεRI are mediated by a large multi-protein complex, sometimes called the FcεRI signalosome mainly consisting of kinases and adaptor proteins (Draber et al., 2012). Upon FcεRI crosslinking by antigen – IgE complexes large intracellular granules are released that store a variety of mediators, among them histamine and proteases (tryptases, chymases; Amin, 2012). Additionally, upon activation they synthesize from arachidonic acid leukotrienes (e.g. leukotriene C4) that add to the recruitment of leukocytes (Mandal et al., 2008). These features highlight MC as key players in allergic and inflammatory reactions. In addition, subsequently to degranulation after FcεRI crosslinking mediator release from MC is continued, contributing to delayed immune responses following MC activation. Some of the very early events following FcεRI crosslinking even distinguish between mediator release from granules and cytokine production including downstream immune consequences (Shumilina et al., 2008; Sobiesiak et al., 2009). In the last decade, MC and MC mediators have been increasingly appreciated as instructors of other cells in immune reactions by the release of cytokines or cell contact dependent signaling (Bradding et al., 1994; Sayed et al., 2008). For example, MC derived TNF has been shown to be crucial for neutrophil recruitment and local endothelial inflammation (Biedermann et al., 2000; Kneilling et al., 2009). While most prominently associated with allergic and autoimmune diseases as well as infections, MC and MC derived mediators also play an important role in the detoxification of insect or snake venoms (Metz et al., 2006; Akahoshi et al., 2011). This may be especially interesting, as it indicates also a physiologic relevance of venom specific IgE and IgE-dependent MC activation: low level sensitization and IgE-mediated MC activation may have contributed to survival following intoxications and lead to a positive selection of this activation pathway during evolution.
By and large, tetraspanin expression and function in MC and basophils and in the variety of responses in which MC and basophils contribute have not been very well characterized. However, it is clear that tetraspanins play an important role in MC functions, because they are closely linked to FcεRI signaling and degranulation. Furthermore, they may also play an important role in some of the rather newly described MC functions, but this remains to be determined.
Distribution and Function of Tetraspanins in MC and Basophils
CD9
On their search for an antibody specific to lymphohematopoietic progenitors, Kersey et al. (1981) identified CD9 (TSPAN29, Leukemia-Associated Cell Surface Antigen p24, Motility-Related Protein-1) by a monoclonal antibody (mAb) that bound to acute lymphoblastic leukemia cells.
CD9 is expressed on a wide variety of cells, including B cells, T cells, dendritic cells, platelets, and endothelial cells. By immunophenotyping human MC, CD9 has been detected on subtypes such as bone marrow MC, skin MC, renal MC, tonsillar MC, uterine MC, cardiac MC, lung MC as well as on basophils (Guo et al., 1992; Sperr et al., 1994; Fureder et al., 1997; Beil et al., 1998; Escribano et al., 1998; Ghannadan et al., 1998). Malignant MC clones express CD9 as well, though in contrast to CD63 no upregulation compared to healthy MC has been described (Baghestanian et al., 1996; Jost et al., 2001).
Data gained from experiments with platelets show co-localization and most likely functional interaction of CD9 and FcγRII, which is also the predominant FcγR in MC (Huang et al., 1992; Moseley, 2005). Degranulation of rat basophil leukemia (RBL) cells transfected with CD9 could be triggered by mAb directed against CD9 but not with F(ab′)2 fragments of these mAb. Therefore, these data argue that the artificial mAb treatment acts as a surrogate for IgE coated antigen leading to FcεRI clustering as well. While co-localization of CD9 and FcεRI was also proven by co-precipitation, no functional consequences were documented (Higginbottom et al., 2000).
IL-16 has been described as CD4 ligand inducing T helper cell chemotaxis and IL-2 receptor expression (Parada et al., 1998). However, MC migrate and maturate in response to IL-16 as well and while some MC lines and human cord blood derived MC do express CD4, human bone marrow and peripheral MC are CD4 negative. Even in the case of CD4 expression, IL-16 dependent migration is abrogated only partially by a CD4 blocking antibody treatment of MC and other monocytes (Sperr et al., 1994; Kirshenbaum et al., 2003; Qi et al., 2006). In the quest for an additional receptor, unexpectedly CD9 was identified as a receptor conferring IL-16 responsiveness to MC by induction of PI3K signaling and Ca2+ influx (Qi et al., 2006).
CD9, CD81 (see below), and FcεRI have been shown to be co-expressed and co-localized in human dendritic cells. In these cells, co-activation of FcεRI (by crosslinking) and CD9 (by mAb) resulted in increased IL-10 production (Peng et al., 2011).
A CD9 blocking antibody exists (Chen et al., 1999), and was shown to block MC migration in vitro (Qi et al., 2006). However, and in contrast to some other MC tetraspanins, it is unknown whether it affects degranulation. If so, it could be used in combination with other tetraspanin blocking antibodies and thus might synergistically increase the effectiveness of a tetraspanin targeting treatment option intended to ameliorate the symptoms of erratic MC activation in allergic disease. If it furthermore blocks chemotaxis also in vivo, it might also prevent MC accumulation at sites affected. CD9 has been described to expose a conformation dependent epitope when clustered with activated β1-integrins. A mAb directed against this epitope could mimic the PI3K activation (Gutierrez-Lopez et al., 2003). Hence it would be interesting to know if this epitope is also involved in IL-16 induced PI3K signaling by CD9.
CD63
CD63 (TSPAN30, Granulophysin, Melanoma Antigen ME491, Platelet Glycoprotein 40 kDa, sometimes ambiguously lamp-3) was the first tetraspanin that has been cloned in 1988 from human cancer cells (Hotta et al., 1988). Three years later it was already demonstrated to be located in the vicinity of the FcεRI on RBL-2H3 basophils (Kitani et al., 1991). If used in high concentrations, the antibody directed against CD63 moderately inhibited degranulation. In experiments with RBL cells transfected with human CD63, two out of five mAb clones specific for hCD63 stimulated degranulation in the absence of any further stimuli. The addition of a secondary antibody did not further increase degranulation, arguing against a mechanism of mere crosslinking (Smith et al., 1995). The authors of these studies thus speculated that CD63 might be a mediator or promoter of FcεRI signaling, but at that time, no experimental evidence supported this hypothesis. Instead it had been shown intracellularly to co-localize with lamp-1 and lamp-2 on lysosomes (Metzelaar et al., 1991) and on endosomes. Importantly, CD63 is also present on various kinds of secretory granules. It has been detected on basophil granules (Knol et al., 1991), but also on platelet granules (Nishibori et al., 1993), the granzyme/perforin containing granules of cytotoxic T cells (Peters et al., 1991) and the von Willebrand Factor/P-selectin containing Weibel Palade bodies of endothelial cells (Vischer and Wagner, 1993). CD63 is targeted to lysosomes by C-terminal GYEVM motif that is recognized by the adaptor protein AP-3 subunit μ3 (Rous et al., 2002) and has been found to be cycling between endocytic vesicles and secretory granules (Kobayashi et al., 2000).
In the event of granule exocytosis, CD63 containing granule membrane fuses with the plasma membrane. Therefore, CD63 soon was used extensively as activation marker of basophils (Knol et al., 1991) and MC (reviewed in Valent et al., 2001). Close spatial examination of the site of CD63+ vesicle exocytosis showed that it is roughly targeted into the direction of the stimulus, but spares the site of FcεRI crosslinking. This is in contrast to CD63− recycling endosomes, which are also exocytosed in response to FcεRI activation and are targeted directly to the site of the stimulus (Wu et al., 2007). When additional activation markers were uncovered, we defined a group of activation markers that behaved like CD63, consisting of CD63 and CD107a and indicating anaphylactic exocytosis of large granules. The surface exposure time course of these “slow” activation markers parallels that of histamine release, in contrast to the “fast” CD203c group (CD203c, CD13, CD64) that rather indicates piecemeal degranulation (Hennersdorf et al., 2005).
However, even under steady state conditions many types of human MC showed constitutive CD63 surface expression. This included skin MC, renal MC, tonsillar MC, lung MC, uterus MC, and gastrointestinal MC as well as cultured MC progenitors from cord blood and basophils (Fureder et al., 1997; Beil et al., 1998; Ghannadan et al., 1998; Krauth et al., 2005; Schernthaner et al., 2005). Furthermore, patients with indolent systemic mastocytosis showed upregulated CD63 on MC (Escribano et al., 1998).
Interactions of various integrins and tetraspanins have been observed in many cell types. Tetraspanins have been shown to be important for integrin mediated intercellular adhesion, regulate integrin mediated cell motility and are involved in integrin signaling (Berditchevski, 2001). CD63 is no exception from the rule that integrins are classical interaction partners of tetraspanins. For example, CD63 has been found associated with LFA-1 (CD11a/CD18) and some mAb against CD63 triggered increased cell adhesiveness probably resulting from Src kinase activation (Skubitz et al., 1996). Recently, in a very similar finding interaction of CD63 and β1-Integrin triggered by their natural ligand ameloblastin resulted in Src activation as well (Iizuka et al., 2011). In a major advance in the quest for the role of CD63 in MC with a blocking mAb directed against CD63 not only MC adhesion to fibronectin and vitronectin could be inhibited but also FcεRI mediated degranulation of adherent MC. Furthermore, the Ab was sufficient to inhibit FcεRI mediated degranulation in rats. While no effect on tyrosine phosphorylation was observed, the Gab2 PI3K pathway known to affect adhesion as well as degranulation was shown to be suppressed (Kraft et al., 2005).
Work focusing on intracellular CD63 showed co-localization of CD63 with the SNARE proteins VAMP-7 and syntaxin 3 together with N-ethylmaleimide-sensitive factor (NSF; Puri et al., 2003). SNARES are proteins mediating the fusion of granule membrane with the plasma membrane and NSF is an ATPase initiating SNARE disassembly (reviewed by Benhamou and Blank, 2010). SNARE disassembly “primes” MC, enabling further degranulation events. Recently, a mAb was developed that specifically recognizes CD63 of granular origin. Specificity was found to depend on a glucosylation pattern only present on the intracellular isoform. Recognizing CD63 that had reached the cell membrane during a previous degranulation event, this Ab proved that MC can degranulate at least a second time in response to a different IgE-antigen stimulus (Schafer et al., 2010). These findings are complemented by a report that investigated the superior stimulus provided by particulate antigens (pAg). The authors show long lasting interaction of pAg/IgE/FcεRI in CD63+ granule compartments that did not occur when soluble antigen was administered instead (Jin et al., 2011).
New findings show involvement of the autophagy pathway in degranulation and co-localization of CD63 with the autophagy marker LCR-II (Ushio et al., 2011).
The new mAb recognizing granular CD63 will be an important tool in MC research and might also be useful as a diagnostic tool. The capacity of the CD63 blocking antibody to block MC activation in vivo might make it a lead for a novel therapy targeting MC granule release. However, as observed with the Hermansky Pudlak syndrome, a rare hereditary disease shown to be the result of CD63 expression deficiency (Nishibori et al., 1993) high dose application of anti-CD63 might negatively affect blood coagulation. The interesting finding that CD63 promotes FcεRI signaling only of adherent MC prompts the question whether integrin binding might effect an activating conformation change in associated CD63 as has been shown for CD9 (Gutierrez-Lopez et al., 2003), thereby amplifying intracellular FcεRI signaling.
CD81
CD81 (TSPAN28, TAPA-1) was identified as the target of an anti-proliferative antibody with strong cDNA homology to CD63 (Oren et al., 1990). It is very broadly expressed on hematolymphoid, neuroectodermal, and mesenchymal cells and has been shown to amplify B-cell- and T-cell-receptor signaling (Levy et al., 1998). Similarly a mAb directed against CD81 was found to down-regulate FcεRI mediated degranulation of RBL cells (Fleming et al., 1997). As was later shown in a similar manner for an CD63 specific mAb (Kraft et al., 2005, see above), this treatment failed to inhibit FcεRI-induced tyrosine phosphorylation. Also, calcium mobilization or leukotriene synthesis was not affected (Fleming et al., 1997). While no signaling pathway mediating the anti-CD81 effect could be elucidated, effectiveness of this Ab in curing passive cutaneous anaphylaxis in rats could be demonstrated. CD81 has been shown to associate with VLA-4 and LFA-1 and to activate their adhesiveness in lymphocytes (Levy et al., 1998). Furthermore it was demonstrated to regulate Rac1 activation in cell migration (Quast et al., 2011). Maybe, reduction of allergen induced airway hyperreactivity observed in CD81 deficient mice (Deng et al., 2000) might in part result of a disrupted FcεRI–CD81 interaction that probably regulates FcεRI signaling in wildtype mice. Compared to CD9 and CD63 the knowledge and understanding of the role of CD81 in MC is quite limited. It would be interesting to investigate whether it promotes adhesion and migration also in MC. The potential application of blocking anti-CD81 as an anti-allergic treatment however, might most prominently inhibit B cell antibody production by inhibiting signaling of the CD19 CD81 B-cell-receptor complex (van Zelm et al., 2010).
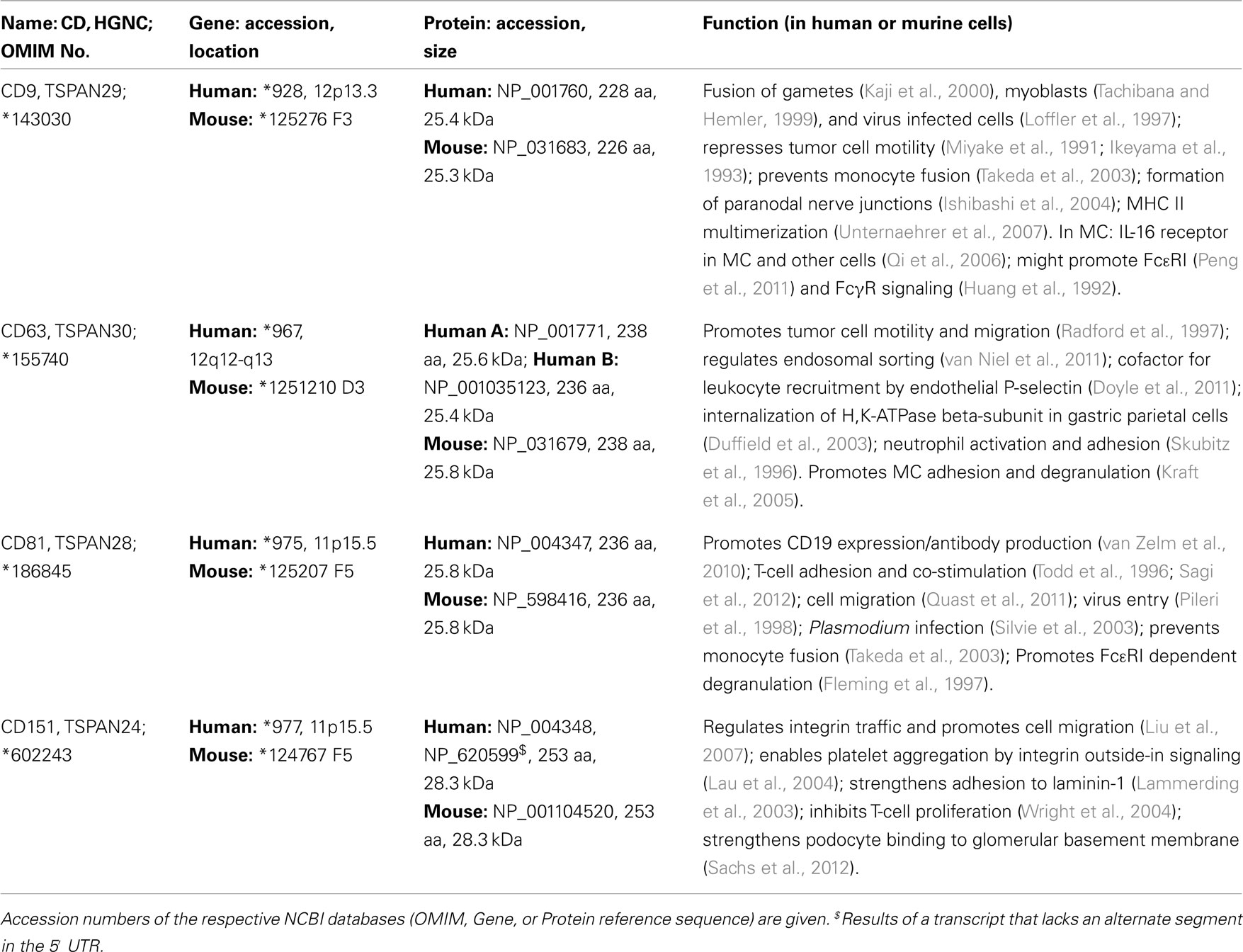
Table 1. General molecular and prominent functional properties of tetraspanins also expressed in MC.
CD151
Immunophenotyping with a mAb raised against myeloid leukemia cells identified CD151 (TSPAN24, gp27, PETA-3, Raph blood group) on platelets and megakaryocytes as well as on various types of myeloid, endothelial and epithelial cells (Ashman et al., 1991; Sincock et al., 1997). The examination of CD151 on MC was not as comprehensive as with other tetraspanins, however it has been found on human foreskin, gastrointestinal, uterine, lung, and cord blood derived MC as well as on basophils (Ghannadan et al., 1998; Wimazal et al., 1999; Krauth et al., 2005; Schernthaner et al., 2005). It is well established that CD151 is co-expressed and co-localized with laminin binding integrins, facilitating adhesion, and motility processes, the latter possibly by mediating TEM endocytosis and recycling (Liu et al., 2007). However, there are no functional data regarding the role of CD151 in MC yet. The data from other cell types, however, suggest it to be an integral TEM component. Thus it is unlikely, that CD151 present on MC is devoid of functions regarding intercellular binding, migration, and recycling of TEM membrane patches.
Concluding Remarks
Tetraspanins are closely linked to MC biology. While CD63 is commonly used as an activation marker for human cells and co-localization of tetraspanins with FcεRI has been known for a long time, it has been much more difficult to gain functional insights. While knowledge about the modes of MC tetraspanin action is increasing recently, there are very few results generated with primary cells and little attention has been paid to the fact that the tetraspanins expressed in MC might be redundant in function. Anyway, it is becoming increasingly clear that tetraspanins form functional clusters with Fcε receptors and integrins on MC surfaces and connect them with downstream signaling components like Src or Gab2.
The development of novel mAb that specifically recognize CD63 of intracellular origin (Schafer et al., 2010) or that are able to attenuate MC degranulation by targeting CD63 or CD81 (Fleming et al., 1997; Kraft et al., 2005) might improve diagnostics and provide the basis to develop powerful tools for the treatment of acute allergic reactions. Furthermore, tetraspanins might play a key role in emerging topics of MC research: (1) MC are regarded as important sources of TNF under pro-inflammatory conditions (Biedermann et al., 2000; Kneilling et al., 2009) and tetraspanins might contribute to metallo-protease cleavage of pro-TNF as has been described for other cells (Arduise et al., 2008). (2) Tetraspanins might be involved in establishing mast cell adhesion and cell–cell contacts to a much larger extent than anticipated today (Kraft et al., 2005). (3) MC are increasingly perceived as antigen presenting cells (Kambayashi et al., 2009; Stelekati et al., 2009), thus it is of interest if the effects of tetraspanins on antigen presentation reported from dendritic cells and B cells might also be observed in MC. (4) Recent research demonstrated MC as a source of exosomes (Skokos et al., 2002) of which tetraspanins are major membrane components. As has been described for exosomes of many other cells types, MC exosomes stained positive for CD63 (Valadi et al., 2007). Thus, adding to tetraspanin research on MC plasma membrane or MC granules, MC exosomes open an additional new field for the examination of MC tetraspanins.
All together, accumulating evidence suggests that tetraspanins are hitherto underrated contributors in the regulation of effector functions also in MC. Although we are still missing some important data on tetraspanin functions in MC, already our present understanding highlights that tetraspanins may be relevant druggable targets in future therapeutic strategies to treat allergic and possibly also other MC-dependent diseases.
Conflict of Interest Statement
The authors declare that the research was conducted in the absence of any commercial or financial relationships that could be construed as a potential conflict of interest.
Acknowledgments
This work was supported by the COST Action BM1007 (Mast cells and basophils – targets for innovative therapies) of the European Community, and the Deutsche Forschungsgemeinschaft (MC SPP Bi 696/5-1, SFB 685).
References
Abraham, S. N., and St John, A. L. (2010). Mast cell-orchestrated immunity to pathogens. Nat. Rev. 10, 440–452.
Akahoshi, M., Song, C. H., Piliponsky, A. M., Metz, M., Guzzetta, A., Abrink, M., Schlenner, S. M., Feyerabend, T. B., Rodewald, H. R., Pejler, G., Tsai, M., and Galli, S. J. (2011). Mast cell chymase reduces the toxicity of Gila monster venom, scorpion venom, and vasoactive intestinal polypeptide in mice. J. Clin. Invest. 121, 4180–4191.
Arduise, C., Abache, T., Li, L., Billard, M., Chabanon, A., Ludwig, A., Mauduit, P., Boucheix, C., Rubinstein, E., and Le Naour, F. (2008). Tetraspanins regulate ADAM10-mediated cleavage of TNF-alpha and epidermal growth factor. J. Immunol. 181, 7002–7013.
Ashman, L. K., Aylett, G. W., Mehrabani, P. A., Bendall, L. J., Niutta, S., Cambareri, A. C., Cole, S. R., and Berndt, M. C. (1991). The murine monoclonal antibody, 14A2.H1, identifies a novel platelet surface antigen. Br. J. Haematol. 79, 263–270.
Baghestanian, M., Bankl, H., Sillaber, C., Beil, W. J., Radaszkiewicz, T., Fureder, W., Preiser, J., Vesely, M., Schernthaner, G., Lechner, K., and Valent, P. (1996). A case of malignant mastocytosis with circulating mast cell precursors: biologic and phenotypic characterization of the malignant clone. Leukemia 10, 159–166.
Beghdadi, W., Madjene, L. C., Benhamou, M., Charles, N., Gautier, G., Launay, P., and Blank, U. (2011). Mast cells as cellular sensors in inflammation and immunity. Front. Immun. 2:37. doi: 10.3389/fimmu.2011.00037
Beil, W. J., Fureder, W., Wiener, H., Grossschmidt, K., Maier, U., Schedle, A., Bankl, H. C., Lechner, K., and Valent, P. (1998). Phenotypic and functional characterization of mast cells derived from renal tumor tissues. Exp. Hematol. 26, 158–169.
Benhamou, M., and Blank, U. (2010). Stimulus-secretion coupling by high-affinity IgE receptor: new developments. FEBS Lett. 584, 4941–4948.
Berditchevski, F. (2001). Complexes of tetraspanins with integrins: more than meets the eye. J. Cell. Sci. 114, 4143–4151.
Biedermann, T., Kneilling, M., Mailhammer, R., Maier, K., Sander, C. A., Kollias, G., Kunkel, S. L., Hultner, L., and Rocken, M. (2000). Mast cells control neutrophil recruitment during T cell-mediated delayed-type hypersensitivity reactions through tumor necrosis factor and macrophage inflammatory protein 2. J. Exp. Med. 192, 1441–1452.
Bradding, P., Roberts, J. A., Britten, K. M., Montefort, S., Djukanovic, R., Mueller, R., Heusser, C. H., Howarth, P. H., and Holgate, S. T. (1994). Interleukin-4, -5, and -6 and tumor necrosis factor-alpha in normal and asthmatic airways: evidence for the human mast cell as a source of these cytokines. Am. J. Respir. Cell Mol. Biol. 10, 471–480.
Chen, M. S., Tung, K. S., Coonrod, S. A., Takahashi, Y., Bigler, D., Chang, A., Yamashita, Y., Kincade, P. W., Herr, J. C., and White, J. M. (1999). Role of the integrin-associated protein CD9 in binding between sperm ADAM 2 and the egg integrin alpha6beta1: implications for murine fertilization. Proc. Natl. Acad. Sci. U.S.A. 96, 11830–11835.
Collington, S. J., Williams, T. J., and Weller, C. L. (2011). Mechanisms underlying the localisation of mast cells in tissues. Trends Immunol. 32, 478–485.
Crivellato, E., and Ribatti, D. (2010). The mast cell: an evolutionary perspective. Biol. Rev. Camb. Philos. Soc. 85, 347–360.
Deng, J., Yeung, V. P., Tsitoura, D., Dekruyff, R. H., Umetsu, D. T., and Levy, S. (2000). Allergen-induced airway hyperreactivity is diminished in CD81-deficient mice. J. Immunol. 165, 5054–5061.
Doyle, E. L., Ridger, V., Ferraro, F., Turmaine, M., Saftig, P., and Cutler, D. F. (2011). CD63 is an essential cofactor to leukocyte recruitment by endothelial P-selectin. Blood 118, 4265–4273.
Draber, P., Halova, I., Levi-Schaffer, F., and Draberova, L. (2012). Transmembrane adaptor proteins in the high-affinity IgE receptor signaling. Front. Immun. 2:95. doi: 10.3389/fimmu.2011.00095
Dudeck, A., Dudeck, J., Scholten, J., Petzold, A., Surianarayanan, S., Kohler, A., Peschke, K., Vohringer, D., Waskow, C., Krieg, T., Muller, W., Waisman, A., Hartmann, K., Gunzer, M., and Roers, A. (2011). Mast cells are key promoters of contact allergy that mediate the adjuvant effects of haptens. Immunity 34, 973–984.
Duffield, A., Kamsteeg, E. J., Brown, A. N., Pagel, P., and Caplan, M. J. (2003). The tetraspanin CD63 enhances the internalization of the H,K-ATPase beta-subunit. Proc. Natl. Acad. Sci. U.S.A. 100, 15560–15565.
Echtenacher, B., Mannel, D. N., and Hultner, L. (1996). Critical protective role of mast cells in a model of acute septic peritonitis. Nature 381, 75–77.
Escribano, L., Orfao, A., Diaz Agustin, B., Cervero, C., Herrero, S., Villarrubia, J., Bravo, P., Torrelo, A., Montero, T., Valdemoro, M., Velasco, J. L., Navarro, J. L., and San Miguel, J. F. (1998). Human bone marrow mast cells from indolent systemic mast cell disease constitutively express increased amounts of the CD63 protein on their surface. Cytometry 34, 223–228.
Feyerabend, T. B., Weiser, A., Tietz, A., Stassen, M., Harris, N., Kopf, M., Radermacher, P., Moller, P., Benoist, C., Mathis, D., Fehling, H. J., and Rodewald, H. R. (2011). Cre-mediated cell ablation contests mast cell contribution in models of antibody- and T cell-mediated autoimmunity. Immunity 35, 832–844.
Fleming, T. J., Donnadieu, E., Song, C. H., Laethem, F. V., Galli, S. J., and Kinet, J. P. (1997). Negative regulation of Fc epsilon RI-mediated degranulation by CD81. J. Exp. Med. 186, 1307–1314.
Fureder, W., Bankl, H. C., Toth, J., Walchshofer, S., Sperr, W., Agis, H., Semper, H., Sillaber, C., Lechner, K., and Valent, P. (1997). Immunophenotypic and functional characterization of human tonsillar mast cells. J. Leukoc. Biol. 61, 592–599.
Ghannadan, M., Baghestanian, M., Wimazal, F., Eisenmenger, M., Latal, D., Kargul, G., Walchshofer, S., Sillaber, C., Lechner, K., and Valent, P. (1998). Phenotypic characterization of human skin mast cells by combined staining with toluidine blue and CD antibodies. J. Invest. Dermatol. 111, 689–695.
Guo, C. B., Kagey-Sobotka, A., Lichtenstein, L. M., and Bochner, B. S. (1992). Immunophenotyping and functional analysis of purified human uterine mast cells. Blood 79, 708–712.
Gutierrez-Lopez, M. D., Ovalle, S., Yanez-Mo, M., Sanchez-Sanchez, N., Rubinstein, E., Olmo, N., Lizarbe, M. A., Sanchez-Madrid, F., and Cabanas, C. (2003). A functionally relevant conformational epitope on the CD9 tetraspanin depends on the association with activated beta1 integrin. J. Biol. Chem. 278, 208–218.
Hallgren, J., and Gurish, M. F. (2007). Pathways of murine mast cell development and trafficking: tracking the roots and routes of the mast cell. Immunol. Rev. 217, 8–18.
Hemler, M. E. (2005). Tetraspanin functions and associated microdomains. Nat. Rev. Mol. Cell Biol. 6, 801–811.
Hennersdorf, F., Florian, S., Jakob, A., Baumgartner, K., Sonneck, K., Nordheim, A., Biedermann, T., Valent, P., and Buhring, H. J. (2005). Identification of CD13, CD107a, and CD164 as novel basophil-activation markers and dissection of two response patterns in time kinetics of IgE-dependent upregulation. Cell Res. 15, 325–335.
Higginbottom, A., Wilkinson, I., Mccullough, B., Lanza, F., Azorsa, D. O., Partridge, L. J., and Monk, P. N. (2000). Antibody cross-linking of human CD9 and the high-affinity immunoglobulin E receptor stimulates secretion from transfected rat basophilic leukaemia cells. Immunology 99, 546–552.
Hotta, H., Ross, A. H., Huebner, K., Isobe, M., Wendeborn, S., Chao, M. V., Ricciardi, R. P., Tsujimoto, Y., Croce, C. M., and Koprowski, H. (1988). Molecular cloning and characterization of an antigen associated with early stages of melanoma tumor progression. Cancer Res. 48, 2955–2962.
Huang, M. M., Indik, Z., Brass, L. F., Hoxie, J. A., Schreiber, A. D., and Brugge, J. S. (1992). Activation of Fc gamma RII induces tyrosine phosphorylation of multiple proteins including Fc gamma RII. J. Biol. Chem. 267, 5467–5473.
Iizuka, S., Kudo, Y., Yoshida, M., Tsunematsu, T., Yoshiko, Y., Uchida, T., Ogawa, I., Miyauchi, M., and Takata, T. (2011). Ameloblastin regulates osteogenic differentiation by inhibiting Src kinase via cross talk between integrin beta1 and CD63. Mol. Cell. Biol. 31, 783–792.
Ikeyama, S., Koyama, M., Yamaoko, M., Sasada, R., and Miyake, M. (1993). Suppression of cell motility and metastasis by transfection with human motility-related protein (MRP-1/CD9) DNA. J. Exp. Med. 177, 1231–1237.
Ishibashi, T., Ding, L., Ikenaka, K., Inoue, Y., Miyado, K., Mekada, E., and Baba, H. (2004). Tetraspanin protein CD9 is a novel paranodal component regulating paranodal junctional formation. J. Neurosci. 24, 96–102.
Ishibashi, K., Suzuki, M., Sasaki, S., and Imai, M. (2001). Identification of a new multigene four-transmembrane family (MS4A) related to CD20, HTm4 and beta subunit of the high-affinity IgE receptor. Gene 264, 87–93.
Jin, C., Shelburne, C. P., Li, G., Potts, E. N., Riebe, K. J., Sempowski, G. D., Foster, W. M., and Abraham, S. N. (2011). Particulate allergens potentiate allergic asthma in mice through sustained IgE-mediated mast cell activation. J. Clin. Invest. 121, 941–955.
Jost, E., Michaux, L., Vanden Abeele, M., Boland, B., Latinne, D., Godfraind, C., Scheiff, J. M., Vaerman, J. L., Lecouvet, F., and Ferrant, A. (2001). Complex karyotype and absence of mutation in the c-kit receptor in aggressive mastocytosis presenting with pelvic osteolysis, eosinophilia and brain damage. Ann. Hematol. 80, 302–307.
Kaji, K., Oda, S., Shikano, T., Ohnuki, T., Uematsu, Y., Sakagami, J., Tada, N., Miyazaki, S., and Kudo, A. (2000). The gamete fusion process is defective in eggs of Cd9-deficient mice. Nat. Genet. 24, 279–282.
Kambayashi, T., Allenspach, E. J., Chang, J. T., Zou, T., Shoag, J. E., Reiner, S. L., Caton, A. J., and Koretzky, G. A. (2009). Inducible MHC class II expression by mast cells supports effector and regulatory T cell activation. J. Immunol. 182, 4686–4695.
Kersey, J. H., Lebien, T. W., Abramson, C. S., Newman, R., Sutherland, R., and Greaves, M. (1981). P-24: a human leukemia-associated and lymphohemopoietic progenitor cell surface structure identified with monoclonal antibody. J. Exp. Med. 153, 726–731.
Kirshenbaum, A. S., Akin, C., Wu, Y., Rottem, M., Goff, J. P., Beaven, M. A., Rao, V. K., and Metcalfe, D. D. (2003). Characterization of novel stem cell factor responsive human mast cell lines LAD 1 and 2 established from a patient with mast cell sarcoma/leukemia; activation following aggregation of FcepsilonRI or FcgammaRI. Leuk. Res. 27, 677–682.
Kitani, S., Berenstein, E., Mergenhagen, S., Tempst, P., and Siraganian, R. P. (1991). A cell surface glycoprotein of rat basophilic leukemia cells close to the high affinity IgE receptor (Fc epsilon RI). Similarity to human melanoma differentiation antigen ME491. J. Biol. Chem. 266, 1903–1909.
Kneilling, M., Hultner, L., Pichler, B. J., Mailhammer, R., Morawietz, L., Solomon, S., Eichner, M., Sabatino, J., Biedermann, T., Krenn, V., Weber, W. A., Illges, H., Haubner, R., and Rocken, M. (2007). Targeted mast cell silencing protects against joint destruction and angiogenesis in experimental arthritis in mice. Arthritis Rheum. 56, 1806–1816.
Kneilling, M., Mailhammer, R., Hultner, L., Schonberger, T., Fuchs, K., Schaller, M., Bukala, D., Massberg, S., Sander, C. A., Braumuller, H., Eichner, M., Maier, K. L., Hallmann, R., Pichler, B. J., Haubner, R., Gawaz, M., Pfeffer, K., Biedermann, T., and Rocken, M. (2009). Direct crosstalk between mast cell-TNF and TNFR1-expressing endothelia mediates local tissue inflammation. Blood 114, 1696–1706.
Knol, E. F., Mul, F. P., Jansen, H., Calafat, J., and Roos, D. (1991). Monitoring human basophil activation via CD63 monoclonal antibody 435. J. Allergy Clin. Immunol. 88, 328–338.
Kobayashi, T., Vischer, U. M., Rosnoblet, C., Lebrand, C., Lindsay, M., Parton, R. G., Kruithof, E. K., and Gruenberg, J. (2000). The tetraspanin CD63/lamp3 cycles between endocytic and secretory compartments in human endothelial cells. Mol. Biol. Cell 11, 1829–1843.
Kraft, S., Fleming, T., Billingsley, J. M., Lin, S. Y., Jouvin, M. H., Storz, P., and Kinet, J. P. (2005). Anti-CD63 antibodies suppress IgE-dependent allergic reactions in vitro and in vivo. J. Exp. Med. 201, 385–396.
Krauth, M. T., Majlesi, Y., Florian, S., Bohm, A., Hauswirth, A. W., Ghannadan, M., Wimazal, F., Raderer, M., Wrba, F., and Valent, P. (2005). Cell surface membrane antigen phenotype of human gastrointestinal mast cells. Int. Arch. Allergy Immunol. 138, 111–120.
Lammerding, J., Kazarov, A. R., Huang, H., Lee, R. T., and Hemler, M. E. (2003). Tetraspanin CD151 regulates alpha6beta1 integrin adhesion strengthening. Proc. Natl. Acad. Sci. U.S.A. 100, 7616–7621.
Lau, L. M., Wee, J. L., Wright, M. D., Moseley, G. W., Hogarth, P. M., Ashman, L. K., and Jackson, D. E. (2004). The tetraspanin superfamily member CD151 regulates outside-in integrin alphaIIbbeta3 signaling and platelet function. Blood 104, 2368–2375.
Lee, D. M., Friend, D. S., Gurish, M. F., Benoist, C., Mathis, D., and Brenner, M. B. (2002). Mast cells: a cellular link between autoantibodies and inflammatory arthritis. Science 297, 1689–1692.
Levy, S., and Shoham, T. (2005). Protein-protein interactions in the tetraspanin web. Physiology (Bethesda) 20, 218–224.
Levy, S., Todd, S. C., and Maecker, H. T. (1998). CD81 (TAPA-1): a molecule involved in signal transduction and cell adhesion in the immune system. Annu. Rev. Immunol. 16, 89–109.
Liu, L., He, B., Liu, W. M., Zhou, D., Cox, J. V., and Zhang, X. A. (2007). Tetraspanin CD151 promotes cell migration by regulating integrin trafficking. J. Biol. Chem. 282, 31631–31642.
Loffler, S., Lottspeich, F., Lanza, F., Azorsa, D. O., Ter Meulen, V., and Schneider-Schaulies, J. (1997). CD9, a tetraspan transmembrane protein, renders cells susceptible to canine distemper virus. J. Virol. 71, 42–49.
Mandal, A. K., Jones, P. B., Bair, A. M., Christmas, P., Miller, D., Yamin, T. T., Wisniewski, D., Menke, J., Evans, J. F., Hyman, B. T., Bacskai, B., Chen, M., Lee, D. M., Nikolic, B., and Soberman, R. J. (2008). The nuclear membrane organization of leukotriene synthesis. Proc. Natl. Acad. Sci. U.S.A. 105, 20434–20439.
Metz, M., Piliponsky, A. M., Chen, C. C., Lammel, V., Abrink, M., Pejler, G., Tsai, M., and Galli, S. J. (2006). Mast cells can enhance resistance to snake and honeybee venoms. Science 313, 526–530.
Metzelaar, M. J., Wijngaard, P. L., Peters, P. J., Sixma, J. J., Nieuwenhuis, H. K., and Clevers, H. C. (1991). CD63 antigen. A novel lysosomal membrane glycoprotein, cloned by a screening procedure for intracellular antigens in eukaryotic cells. J. Biol. Chem. 266, 3239–3245.
Miyake, M., Koyama, M., Seno, M., and Ikeyama, S. (1991). Identification of the motility-related protein (MRP-1), recognized by monoclonal antibody M31-15, which inhibits cell motility. J. Exp. Med. 174, 1347–1354.
Nishibori, M., Cham, B., Mcnicol, A., Shalev, A., Jain, N., and Gerrard, J. M. (1993). The protein CD63 is in platelet dense granules, is deficient in a patient with Hermansky-Pudlak syndrome, and appears identical to granulophysin. J. Clin. Invest. 91, 1775–1782.
Oren, R., Takahashi, S., Doss, C., Levy, R., and Levy, S. (1990). TAPA-1, the target of an antiproliferative antibody, defines a new family of transmembrane proteins. Mol. Cell. Biol. 10, 4007–4015.
Otsuka, A., Kubo, M., Honda, T., Egawa, G., Nakajima, S., Tanizaki, H., Kim, B., Matsuoka, S., Watanabe, T., Nakae, S., Miyachi, Y., and Kabashima, K. (2011). Requirement of interaction between mast cells and skin dendritic cells to establish contact hypersensitivity. PLoS ONE 6, e25538. doi: 10.1371/journal.pone.0025538
Parada, N. A., Center, D. M., Kornfeld, H., Rodriguez, W. L., Cook, J., Vallen, M., and Cruikshank, W. W. (1998). Synergistic activation of CD4 + T cells by IL-16 and IL-2. J. Immunol. 160, 2115–2120.
Peng, W. M., Yu, C. F., Kolanus, W., Mazzocca, A., Bieber, T., Kraft, S., and Novak, N. (2011). Tetraspanins CD9 and CD81 are molecular partners of trimeric FcvarepsilonRI on human antigen-presenting cells. Allergy 66, 605–611.
Pennock, J. L., and Grencis, R. K. (2004). In vivo exit of c-kit+/CD49d(hi)/beta7+ mucosal mast cell precursors from the bone marrow following infection with the intestinal nematode Trichinella spiralis. Blood 103, 2655–2660.
Peters, P. J., Borst, J., Oorschot, V., Fukuda, M., Krahenbuhl, O., Tschopp, J., Slot, J. W., and Geuze, H. J. (1991). Cytotoxic T lymphocyte granules are secretory lysosomes, containing both perforin and granzymes. J. Exp. Med. 173, 1099–1109.
Pileri, P., Uematsu, Y., Campagnoli, S., Galli, G., Falugi, F., Petracca, R., Weiner, A. J., Houghton, M., Rosa, D., Grandi, G., and Abrignani, S. (1998). Binding of hepatitis C virus to CD81. Science 282, 938–941.
Puri, N., Kruhlak, M. J., Whiteheart, S. W., and Roche, P. A. (2003). Mast cell degranulation requires N-ethylmaleimide-sensitive factor-mediated SNARE disassembly. J. Immunol. 171, 5345–5352.
Qi, J. C., Wang, J., Mandadi, S., Tanaka, K., Roufogalis, B. D., Madigan, M. C., Lai, K., Yan, F., Chong, B. H., Stevens, R. L., and Krilis, S. A. (2006). Human and mouse mast cells use the tetraspanin CD9 as an alternate interleukin-16 receptor. Blood 107, 135–142.
Quast, T., Eppler, F., Semmling, V., Schild, C., Homsi, Y., Levy, S., Lang, T., Kurts, C., and Kolanus, W. (2011). CD81 is essential for the formation of membrane protrusions and regulates Rac1-activation in adhesion-dependent immune cell migration. Blood 118, 1818–1827.
Radford, K. J., Thorne, R. F., and Hersey, P. (1997). Regulation of tumor cell motility and migration by CD63 in a human melanoma cell line. J. Immunol. 158, 3353–3358.
Rous, B. A., Reaves, B. J., Ihrke, G., Briggs, J. A., Gray, S. R., Stephens, D. J., Banting, G., and Luzio, J. P. (2002). Role of adaptor complex AP-3 in targeting wild-type and mutated CD63 to lysosomes. Mol. Biol. Cell 13, 1071–1082.
Sachs, N., Claessen, N., Aten, J., Kreft, M., Teske, G. J., Koeman, A., Zuurbier, C. J., Janssen, H., and Sonnenberg, A. (2012). Blood pressure influences end-stage renal disease of Cd151 knockout mice. J. Clin. Invest. 122, 348–358.
Sagi, Y., Landrigan, A., Levy, R., and Levy, S. (2012). Complementary costimulation of human T-cell subpopulations by cluster of differentiation 28 (CD28) and CD81. Proc. Natl. Acad. Sci. U.S.A. 109, 1613–1618.
Sayed, B. A., Christy, A., Quirion, M. R., and Brown, M. A. (2008). The master switch: the role of mast cells in autoimmunity and tolerance. Annu. Rev. Immunol. 26, 705–739.
Schafer, T., Starkl, P., Allard, C., Wolf, R. M., and Schweighoffer, T. (2010). A granular variant of CD63 is a regulator of repeated human mast cell degranulation. Allergy 65, 1242–1255.
Schernthaner, G. H., Hauswirth, A. W., Baghestanian, M., Agis, H., Ghannadan, M., Worda, C., Krauth, M. T., Printz, D., Fritsch, G., Sperr, W. R., and Valent, P. (2005). Detection of differentiation- and activation-linked cell surface antigens on cultured mast cell progenitors. Allergy 60, 1248–1255.
Secor, V. H., Secor, W. E., Gutekunst, C. A., and Brown, M. A. (2000). Mast cells are essential for early onset and severe disease in a murine model of multiple sclerosis. J. Exp. Med. 191, 813–822.
Seigneuret, M., Delaguillaumie, A., Lagaudriere-Gesbert, C., and Conjeaud, H. (2001). Structure of the tetraspanin main extracellular domain. A partially conserved fold with a structurally variable domain insertion. J. Biol. Chem. 276, 40055–40064.
Shumilina, E., Lam, R. S., Wolbing, F., Matzner, N., Zemtsova, I. M., Sobiesiak, M., Mahmud, H., Sausbier, U., Biedermann, T., Ruth, P., Sausbier, M., and Lang, F. (2008). Blunted IgE-mediated activation of mast cells in mice lacking the Ca2+-activated K+ channel KCa3.1 . J. Immunol. 180, 8040–8047.
Silvie, O., Rubinstein, E., Franetich, J. F., Prenant, M., Belnoue, E., Renia, L., Hannoun, L., Eling, W., Levy, S., Boucheix, C., and Mazier, D. (2003). Hepatocyte CD81 is required for Plasmodium falciparum and Plasmodium yoelii sporozoite infectivity. Nat. Med. 9, 93–96.
Sincock, P. M., Mayrhofer, G., and Ashman, L. K. (1997). Localization of the transmembrane 4 superfamily (TM4SF) member PETA-3 (CD151) in normal human tissues: comparison with CD9, CD63, and alpha5beta1 integrin. J. Histochem. Cytochem. 45, 515–525.
Skokos, D., Goubran-Botros, H., Roa, M., and Mecheri, S. (2002). Immunoregulatory properties of mast cell-derived exosomes. Mol. Immunol. 38, 1359–1362.
Skubitz, K. M., Campbell, K. D., Iida, J., and Skubitz, A. P. (1996). CD63 associates with tyrosine kinase activity and CD11/CD18, and transmits an activation signal in neutrophils. J. Immunol. 157, 3617–3626.
Smith, D. A., Monk, P. N., and Partridge, L. J. (1995). Antibodies against human CD63 activate transfected rat basophilic leukemia (RBL-2H3) cells. Mol. Immunol. 32, 1339–1344.
Sobiesiak, M., Shumilina, E., Lam, R. S., Wolbing, F., Matzner, N., Kaesler, S., Zemtsova, I. M., Lupescu, A., Zahir, N., Kuhl, D., Schaller, M., Biedermann, T., and Lang, F. (2009). Impaired mast cell activation in gene-targeted mice lacking the serum- and glucocorticoid-inducible kinase SGK1. J. Immunol. 183, 4395–4402.
Sperr, W. R., Bankl, H. C., Mundigler, G., Klappacher, G., Grossschmidt, K., Agis, H., Simon, P., Laufer, P., Imhof, M., Radaszkiewicz, T., Glogar, D., Lechner, K., and Valent, P. (1994). The human cardiac mast cell: localization, isolation, phenotype, and functional characterization. Blood 84, 3876–3884.
Stelekati, E., Bahri, R., D’Orlando, O., Orinska, Z., Mittrucker, H. W., Langenhaun, R., Glatzel, M., Bollinger, A., Paus, R., and Bulfone-Paus, S. (2009). Mast cell-mediated antigen presentation regulates CD8+ T cell effector functions. Immunity 31, 665–676.
Tachibana, I., and Hemler, M. E. (1999). Role of transmembrane 4 superfamily (TM4SF) proteins CD9 and CD81 in muscle cell fusion and myotube maintenance. J. Cell Biol. 146, 893–904.
Takeda, Y., Tachibana, I., Miyado, K., Kobayashi, M., Miyazaki, T., Funakoshi, T., Kimura, H., Yamane, H., Saito, Y., Goto, H., Yoneda, T., Yoshida, M., Kumagai, T., Osaki, T., Hayashi, S., Kawase, I., and Mekada, E. (2003). Tetraspanins CD9 and CD81 function to prevent the fusion of mononuclear phagocytes. J. Cell Biol. 161, 945–956.
Todd, S. C., Lipps, S. G., Crisa, L., Salomon, D. R., and Tsoukas, C. D. (1996). CD81 expressed on human thymocytes mediates integrin activation and interleukin 2-dependent proliferation. J. Exp. Med. 184, 2055–2060.
Unternaehrer, J. J., Chow, A., Pypaert, M., Inaba, K., and Mellman, I. (2007). The tetraspanin CD9 mediates lateral association of MHC class II molecules on the dendritic cell surface. Proc. Natl. Acad. Sci. U.S.A. 104, 234–239.
Ushio, H., Ueno, T., Kojima, Y., Komatsu, M., Tanaka, S., Yamamoto, A., Ichimura, Y., Ezaki, J., Nishida, K., Komazawa-Sakon, S., Niyonsaba, F., Ishii, T., Yanagawa, T., Kominami, E., Ogawa, H., Okumura, K., and Nakano, H. (2011). Crucial role for autophagy in degranulation of mast cells. J. Allergy Clin. Immunol. 127, 1267–1276 e1266.
Valadi, H., Ekstrom, K., Bossios, A., Sjostrand, M., Lee, J. J., and Lotvall, J. O. (2007). Exosome-mediated transfer of mRNAs and microRNAs is a novel mechanism of genetic exchange between cells. Nat. Cell Biol. 9, 654–659.
Valent, P., Schernthaner, G. H., Sperr, W. R., Fritsch, G., Agis, H., Willheim, M., Buhring, H. J., Orfao, A., and Escribano, L. (2001). Variable expression of activation-linked surface antigens on human mast cells in health and disease. Immunol. Rev. 179, 74–81.
van Niel, G., Charrin, S., Simoes, S., Romao, M., Rochin, L., Saftig, P., Marks, M. S., Rubinstein, E., and Raposo, G. (2011). The tetraspanin CD63 regulates ESCRT-independent and -dependent endosomal sorting during melanogenesis. Dev. Cell 21, 708–721.
van Zelm, M. C., Smet, J., Adams, B., Mascart, F., Schandene, L., Janssen, F., Ferster, A., Kuo, C. C., Levy, S., Van Dongen, J. J., and Van Der Burg, M. (2010). CD81 gene defect in humans disrupts CD19 complex formation and leads to antibody deficiency. J. Clin. Invest. 120, 1265–1274.
Vischer, U. M., and Wagner, D. D. (1993). CD63 is a component of Weibel-Palade bodies of human endothelial cells. Blood 82, 1184–1191.
Wimazal, F., Ghannadan, M., Muller, M. R., End, A., Willheim, M., Meidlinger, P., Schernthaner, G. H., Jordan, J. H., Hagen, W., Agis, H., Sperr, W. R., Czerwenka, K., Lechner, K., and Valent, P. (1999). Expression of homing receptors and related molecules on human mast cells and basophils: a comparative analysis using multi-color flow cytometry and toluidine blue/immunofluorescence staining techniques. Tissue Antigens 54, 499–507.
Wright, M. D., Geary, S. M., Fitter, S., Moseley, G. W., Lau, L. M., Sheng, K. C., Apostolopoulos, V., Stanley, E. G., Jackson, D. E., and Ashman, L. K. (2004). Characterization of mice lacking the tetraspanin superfamily member CD151. Mol. Cell. Biol. 24, 5978–5988.
Wu, M., Baumgart, T., Hammond, S., Holowka, D., and Baird, B. (2007). Differential targeting of secretory lysosomes and recycling endosomes in mast cells revealed by patterned antigen arrays. J. Cell. Sci. 120, 3147–3154.
Yanez-Mo, M., Barreiro, O., Gordon-Alonso, M., Sala-Valdes, M., and Sanchez-Madrid, F. (2009). Tetraspanin-enriched microdomains: a functional unit in cell plasma membranes. Trends Cell Biol. 19, 434–446.
Keywords: mast cell, tetraspanin, CD9, CD63, CD81, CD151
Citation: Köberle M, Kaesler S, Kempf W, Wölbing F and Biedermann T (2012) Tetraspanins in mast cells. Front. Immun. 3:106. doi: 10.3389/fimmu.2012.00106
Received: 14 March 2012; Accepted: 17 April 2012;
Published online: 07 May 2012.
Edited by:
Ulrich Blank, Institut National de la Santé et de la Recherche Médicale, FranceReviewed by:
Marc Benhamou, Institut National de la Santé et de la Recherche Médicale, FranceFrank A. Redegeld, Utrecht University, Netherlands
Copyright: © 2012 Köberle, Kaesler, Kempf, Wölbing and Biedermann. This is an open-access article distributed under the terms of the Creative Commons Attribution Non Commercial License, which permits non-commercial use, distribution, and reproduction in other forums, provided the original authors and source are credited.
*Correspondence: Tilo Biedermann, Department of Dermatology, Eberhard Karls University, Liebermeisterstrasse 25, 72076 Tübingen, Germany. e-mail: tilo.biedermann@med.uni-tuebingen.de