- Life and Medical Sciences Institute, University of Bonn, Bonn, Germany
Antigen cross-presentation enables dendritic cells (DCs) to present extracellular antigens on major histocompatibility complex (MHC) I molecules, a process that plays an important role in the induction of immune responses against viruses and tumors and in the induction of peripheral tolerance. In order to allow intracellular processing for cross-presentation, internalized antigens are targeted by distinct endocytic receptors toward specific endosomal compartments, where they are protected from rapid lysosomal degradation. From these compartments, antigens are processed for loading onto MHC I molecules. Such processing generally includes antigen transport into the cytoplasm, a process that is regulated by members of the ER-associated degradation (ERAD) machinery. After proteasomal degradation in the cytoplasm, antigen-derived peptides have been shown to be re-imported into the same endosomal compartment by endosomal transporter associated with antigen processing, another ER protein, which is recruited toward the endosomes after DC maturation. In our review, we highlight the recent advances on the molecular mechanisms of cross-presentation. We focus on the necessity of such antigen storage compartments and point out important parallels to MHC I-restricted presentation of endogenous antigens. We discuss the composition of such endosomes and the targeting of extracellular antigens into this compartment by specific endocytic receptors. Finally, we highlight recent advances on the recruitment of the cross-presentation machinery, like the members of the MHC I loading complex and the ERAD machinery, from the ER toward these storage compartments, a process that can be induced by antigen encounter or by activation of the dendritic cell after contact with endotoxins.
Introduction
Adaptive immune responses are induced when dendritic cells (DCs) encounter antigens in the peripheral tissue. Upon antigen recognition, the DC migrates toward the draining lymph node, where it can activate antigen-specific T cells (Mellman and Steinman, 2001). Therefore, the corresponding antigen is internalized by the DC and processed in specialized intracellular compartments. The resulting antigen-derived peptides are subsequently loaded on major histocompatibility complex (MHC) molecules. Whereas antigen loading onto MHC II molecules can lead to the activation of antigen-specific CD4+ T helper cells, peptide loading onto MHC I can activate antigen-specific cytotoxic CD8+ T cells.
In classical antigen presentation, intracellular antigens are degraded by the cytosolic proteasome. The resulting peptides are subsequently transported through the transporter associated with antigen processing (TAP) complex into the ER, where they can be loaded onto MHC I molecules (Purcell and Elliott, 2008). Exogenous proteins are internalized into the dendritic cell by endocytosis and end up in a lysosomal compartment, where they are degraded by lysosomal proteases to be loaded onto MHC II molecules (Trombetta et al., 2003). Apart from these classical presentation pathways, a process termed cross-presentation allows the presentation of extracellular antigens also on MHC I molecules (Bevan, 1976; Kurts et al., 1996).
Cross-presentation has been demonstrated to play an important role in a variety of processes, including the induction of an immune response against viruses that do not infect antigen-presenting cells directly or against tumors of non-hematopoietic origin (Huang et al., 1994; Sigal et al., 1999; den Haan and Bevan, 2001; Heath and Carbone, 2001).
The molecular mechanisms of antigen cross-presentation, however, remain only partially understood. In this review, we highlight some of the recent advances on these underlying mechanisms. We will focus on antigen targeting into specialized storage compartments, on the composition of these compartments and on the recruitment of members of the MHC I loading machinery toward these compartments.
The Importance of Antigen Storage Compartments for Cross-Presentation
During cross-presentation, antigen-derived peptides are loaded onto MHC I molecules. Subsequently, these peptide–MHC I-complexes are transported toward the cell membrane, where they can be recognized by antigen-specific T cells. Whereas peptide-loaded MHC II molecules are stable at the cell membrane for several days (Cella et al., 1997), differing information on the half-life of peptide-loaded MHC I molecules can be found in literature (Eberl et al., 1996; Rescigno et al., 1998; Cella et al., 1999; Kukutsch et al., 2000). A direct comparison between the stability of loaded MHC I molecules and MHC II molecules, however, showed that the half-life of loaded MHC I molecules is markedly decreased compared to peptide-loaded MHC II molecules (van Montfoort et al., 2009). This shorter half-life of peptide-loaded MHC I molecules has important implications for antigen cross-presentation. After antigen internalization, the cross-presenting DC must migrate toward the draining lymph node to activate antigen-specific T cells. Since this process is estimated to take up to 48 h (Martin-Fontecha et al., 2003), a prolonged MHC I-restricted presentation is required for efficient T cell activation, implying that ongoing antigen processing and loading of antigen-derived peptides onto MHC I molecules is indispensible. To ensure continuous peptide loading, it is essential that internalized antigens are not degraded instantly within the endo/lysosomal compartments of the DC, since this would rapidly eliminate putative epitopes for cross-presentation. For these reasons, prolonged cross-presentation depends on antigen storage in endosomal compartments, where they are protected from lysosomal degradation.
Delamarre et al. (2005) demonstrated that DCs express less lysosomal proteases compared to macrophages, resulting in a limited capacity for lysosomal degradation and a slower degradation rate of internalized antigens in DCs. Additionally, antigen stability in DCs is increased by active inhibition of lysosomal acidification, a process that prevents the activation of lysosomal proteases and therefore increases cross-presentation (Hotta et al., 2006). Endosome acidification is mediated by vacuolar ATPase (V-ATPase), which transports protons from the cytosol into the endosome (Nishi and Forgac, 2002). In DCs, this process is antagonized by NOX2-mediated alkalization of the endosome. The NADPH oxidase NOX2 is recruited by Rab27a toward endosomal membranes (Savina et al., 2006), where it produces reactive oxygen species (ROS). Since the production of such ROS within endosomes consumes large amounts of protons, it causes a strong alkalization of the endosome lumen (Savina et al., 2006), which neutralizes V-ATPase-mediated acidification and a neutral endosomal pH can be maintained. As described above, such neutral pH prevents rapid antigen degradation, resulting in enhanced cross-presentation. NOX2-mediated alkalization has been shown to be involved in cross-presentation of particulate antigens in phagosomes (Savina et al., 2006) and of soluble antigens in endosomes (Mantegazza et al., 2008).
The decreased expression of lysosomal proteases in DCs and endosomal alkalization by NOX2 might also be responsible for the high stability of antigens that are internalized by DCs in form of immune complexes. Ferry Ossendorp and colleagues have demonstrated that OVA-containing immune complexes were cross-presented efficiently over a time period of several days (van Montfoort et al., 2009). Importantly, nearly full-length OVA was present for over 3 days in endosomal storage compartments, from where it was steadily processed for cross-presentation.
Taken together, prolonged MHC I-restricted presentation requires antigen deposition in specialized storage compartments, where they are protected from extensive proteasomal or lysosomal degradation and from where continuous processing for loading onto MHC I molecules can take place.
Antigen Targeting into Storage Compartments for Cross-Presentation by Distinct Endocytic Receptors
In many studies, efficient antigen cross-presentation was shown to be restricted to distinct subsets of DCs. In particular, the CD8α+ splenic DCs were shown to be much better in cross-presentation under steady state conditions compared to their CD8α− counterpart in mice (den Haan et al., 2000; Pooley et al., 2001; Schnorrer et al., 2006). Accordingly, cross-presentation capacities in mice lacking CD8α+ DCs were severely reduced (Hildner et al., 2008) and the human counterpart of murine CD8α+ DCs was also demonstrated to have superior cross-presentation capacities (Bachem et al., 2010; Crozat et al., 2010; Jongbloed et al., 2010; Poulin et al., 2010).
Cross-presentation of antigens targeted toward DEC-205, an endocytic receptor that is predominantly expressed on CD8α+ splenic DCs was demonstrated to be much more efficient than antigens targeted toward DCIR2, which is only expressed on CD8α− DCs (Dudziak et al., 2007). Additionally, specific targeting toward DEC-205 resulted in prolonged cross-presentation for over 2 weeks (Bonifaz et al., 2004). It was postulated that these differences in cross-presentation capacity are due to a reduced overall expression of the cross-presentation machinery in CD8α− DCs (Schnorrer et al., 2006; Dudziak et al., 2007). Indeed, NOX2-mediated alkalization of phagosomes was demonstrated to be more pronounced in CD8α+ DCs (Savina et al., 2009). More recent studies demonstrate that also CD8α− DCs possess intrinsic cross-presentation capacities, but that the mechanism by which the DCs internalize the antigen is crucial for its cross-presentation (Kamphorst et al., 2010). This was demonstrated using transgenic mice expressing the human DEC-205 on both CD8α+ and CD8α− DC subsets. Antigen targeting toward this receptor resulted in similar levels of cross-presentation in both CD8α+ and CD8α− DCs, indicating that also CD8α− DCs are potent cross-presenters if the antigen is internalized via DEC-205 but not via DCIR2, demonstrating an important role for the endocytic receptor itself in cross-presentation.
In accordance to these findings, we previously demonstrated a clear correlation between the mechanism of antigen internalization and its presentation (Burgdorf et al., 2007). We could show that antigens internalized by DCs via fluid phase pinocytosis or scavenger receptor-mediated endocytosis were rapidly targeted toward lysosomal structures, where they were degraded instantly and processed for presentation on MHC II molecules. If, however, antigens were internalized via mannose receptor (MR)-mediated endocytosis, they were not targeted toward lysosomes but rather routed into a distinct endosomal subset, which maintained all characteristics of early endosomes for a prolonged time. Importantly, from these endosomes, MR-internalized antigens were processed exclusively for cross-presentation.
These observations emphasize the importance of the endocytic receptor for cross-presentation and point out that the endocytic receptor on the DC that makes contact to an antigen already determines its fate in terms of presentation. Additionally, targeting antigens intended for cross-presentation into a separate pool of endosomes might enable enhanced endosomal stability, which is essential for prolonged cross-presentation, without affecting overall lysosomal activity, which is essential for simultaneous MHC II-restricted presentation.
Interestingly, the MR has also been proposed to directly inhibit lysosomal maturation (Shimada et al., 2006; Sweet et al., 2010), because phagosomes containing MR-internalized glycopeptidolipids displayed impaired phagosome–lysosome fusion. Such alterations were only observed if the glycopeptidolipids were endocytosed by the MR, which then was present in the glycopeptidolipid-containing phagosomes. In both publications, it was postulated that these effects might be due to MR-dependent signaling inside the DC, resulting in an overall impairment of phagosome–lysosome fusion. Another possibility would be that, similar to the observations on the role of the MR in cross-presentation, the MR targets the glycopeptidolipids into a separate endosomal compartment, which does not undergo normal lysosomal maturation. Further studies will reveal whether signaling via the MR additionally alters endosomal trafficking within DCs.
As described above, the different cross-presentation capacities of CD8α+ and CD8α− splenic DCs might to a large extend be due to the expression of different endocytic receptors. This notion might also explain observations demonstrating that certain yeast antigens and antigens targeted to the neonatal Fc receptor are cross-presented to a higher extend by the CD8α− subset (Backer et al., 2008; Baker et al., 2011). Future experiments will show whether CD8α− DCs bear specific receptors for these antigens, enabling their cross-presentation.
The importance of distinct endocytic receptors for cross-presentation is further supported by experiments of the group of Peter Cresswell. They demonstrated that expression of Fc receptors, whose engagement has been shown to lead to potent cross-presentation (van Montfoort et al., 2009), in the human 293 T embryonic kidney cell line enables this cell line to cross-present extracellular antigens (Giodini et al., 2009), pointing out the possibility that nearly every cell possesses intrinsic cross-presentation capacities if the cell expresses a suited receptor.
Although the decisive role of the endocytic receptor for cross-presentation is indubitable, this might only be one half of the story. Increasing evidence points out that also the nature of interaction between the endocytic receptor and the antigen has an important impact on antigen routing and presentation. First, it has been demonstrated that receptor cross-linking by multivalent antigens alters antigen targeting within the DC. It has been shown that dectin-1, an endocytic receptor associated with cross-presentation (Weck et al., 2008), targets monovalent β-glucans into non-lysosomal compartments. If, however, dectin-1 is cross-linked by the multivalent β-glucan zymosan, these antigens are targeted toward lysosomal structures (Herre et al., 2004), demonstrating that antigen valence can regulate antigen trafficking and degradation. Second, it has been shown that the region of the endocytic receptor that recognizes the antigen is of crucial importance. Antigen targeting using antibodies specific for the carbohydrate recognition domain of DC-SIGN has been shown to efficiently deliver such antigens to lysosomal compartments for MHC II presentation (Tacken et al., 2005). A recent study by the same group demonstrated however, that antigen targeting toward the neck region of DC-SIGN results in prolonged antigen retention in early endosomal compartments and in reduced lysosomal trafficking (Tacken et al., 2011). Importantly, these antigens were efficiently cross-presented, demonstrating that different regions of a single endocytic receptor can target antigens to different processing and presentation pathways.
These findings might also provide an explanation for the observation that antigen targeting toward the MR, which targets OVA specifically toward cross-presentation (Burgdorf et al., 2007) as described above, can induce antigen-specific CD4+ T cell responses (Dasgupta et al., 2007; He et al., 2007; McKenzie et al., 2007). In these studies, antigens were targeted toward the MR by conjugation to a MR-specific antibody, which might alter MR-mediated antigen targeting by receptor cross-linking. Alternatively, these antibodies might target other regions of the MR, resulting in different antigen processing and presentation.
In summary, efficient cross-presentation requires antigen recognition by distinct regions of specific endocytic receptors, which target the internalized antigens toward antigen storage compartments, from where they can be processed for loading onto MHC I molecules.
Molecular Mechanisms of Cross-Presentation
The Vacuolar versus the Phagosome-to-Cytosol Pathway
Despite intensive investigations, the molecular mechanisms governing antigen processing and loading onto MHC I molecules for cross-presentation are not fully resolved yet. Importantly, the diversity of experimental evidence obtained by different research groups indicates that multiple pathways can lead to MHC I-restricted presentation of exogenous antigens, depending on the nature of the antigen, the nature of the antigen-presenting cell, and the immunological context of the cross-presentation process (Figure 1).
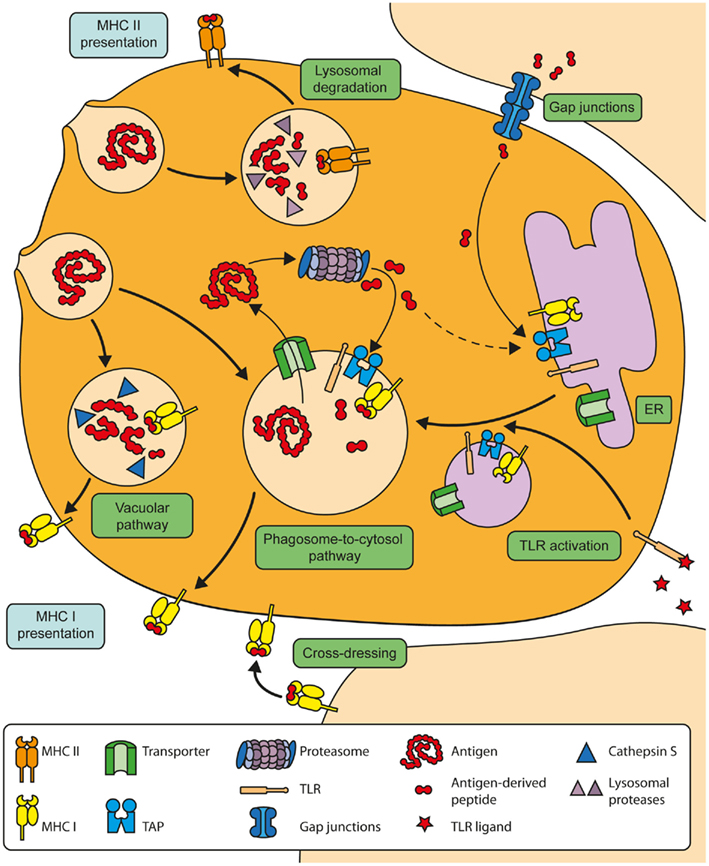
Figure 1. Overview of the molecular mechanisms of cross-presentation. In the vacuolar cross-presentation pathway, extracellular antigens are internalized and degraded in endosomal compartments by Cathepsin S. The resulting peptides are subsequently loaded onto MHC I molecules within the endosomal compartment. In the phagosome-to-cytosol pathway, internalized antigens are transported out of the endosomes into the cytosol for proteasomal degradation. The resulting peptides can be re-imported into the same endosomal compartment by endosomal TAP to be loaded onto MHC I molecules there. The transport of the cross-presentation machinery toward antigen-containing endosomes is induced after stimulation of TLRs. Alternatively, DCs can obtain peptides from neighboring cells via gap junctions. These peptides are thought to subsequently enter the endogenous MHC I-restricted presentation pathway in the ER.
In general, two major pathways are considered to be most relevant for antigen cross-presentation: the vacuolar pathway and the phagosome-to-cytosol pathway (Rock and Shen, 2005).
In the vacuolar pathway, which is also termed TAP-independent cross-presentation, internalized antigens are degraded in endosomal compartments by intra-endosomal proteases such as cathepsin S (Shen et al., 2004). After such degradation, antigenic peptides are loaded within the endosomes onto MHC I molecules, which reach the endosomes from the cell surface during endocytosis. The acid environment in these endosomes might allow already bound peptides to dissociate from the MHC I molecules, enabling the peptides generated within the endosomes to bind MHC I molecules.
Although several studies reported of cross-presentation via the vacuolar pathway (Shen et al., 2004; Bertholet et al., 2006), its physiological significance remains unclear. Therefore, the phagosome-to-cytosol pathway is considered to be the most relevant cross-presentation pathway in vivo (Rock and Shen, 2005).
In the phagosome-to-cytosol pathway, internalized antigens need to be transported from the endosomal lumen into the cytoplasm. Such antigen transport is required for consecutive degradation by the cytosolic proteasome, which is essential for cross-presentation by the phagosome-to-cytosol pathway (Kovacsovics-Bankowski and Rock, 1995; Ackerman et al., 2003; Palmowski et al., 2006).
Antigen Transport from Endosomal Compartments into the Cytosol and Proteasomal Degradation
One of the most intriguing questions concerning the molecular mechanisms of antigen cross-presentation is without any doubt how antigens pass the endosomal membrane to reach the cytosol. Although it has been demonstrated that phagosomes containing Cryptococcus neoformans lose membrane integrity (Tucker and Casadevall, 2002) and the presence of sphingosine within the phagosome might influence membrane stability and permeability (Werneburg et al., 2002), it is assumed that antigen transport into the cytoplasm is not due to disruption of the endosomal membrane. Increasing evidence points out that a pore complex spanning the endosomal membrane might rather mediate this process. Antigen translocation has been demonstrated to be size-selective. Although dextranes of 500 kDa and even 2,000 kDa can still be translocated into the cytosol, the efficiency is clearly lower than the transport of 40 kDa-sized dextranes (Rodriguez et al., 1999), which supports the notion that such antigen transport is not simply due to a simple disrupture of the endosomal membrane.
Increasing evidence points out that the ER-associated degradation (ERAD) machinery plays a very central role in this antigen transport into the cytoplasm (Imai et al., 2005; Ackerman et al., 2006; Giodini and Cresswell, 2008). The ERAD machinery has been studied extensively in the context of protein dislocation at the ER membrane. During dislocation, the ERAD machinery mediates the transport of misfolded proteins from the ER into the cytoplasm for proteasomal degradation, depicting an important function in preventing misfolded proteins from reaching the cell surface. One important member of the ERAD machinery in respect to cross-presentation is Sec61, which is thought to build the pore complex for dislocation of proteins trough the ER membrane. Similar to its role in dislocation, Sec61 has been postulated to build the pore complex through the endosomal membrane for cross-presentation (Ackerman et al., 2006). This hypothesis was based on observations, revealing that DC treatment with exotoxin A, which is assumed to be a specific inhibitor of Sec61, prevented antigen translocation into the cytoplasm and hence cross-presentation (Koopmann et al., 2000). Since such evidence for an involvement of Sec61 in antigen translocation was based on the effect of an inhibitor and therefore indirect, the role of Sec61 in cross-presentation has been questioned (Lin et al., 2008; Segura and Villadangos, 2011). In these studies, it was argued that the size of Sec61, which has been estimated to be about 5–8 Å (Van den Berg et al., 2004), might not be sufficient for antigen translocation. However, this size was calculated for closed or empty Sec61 and it has been postulated that the Sec61 pore complex during protein transport might encompass up to 40–60 Å (Hamman et al., 1997). Additionally, it has been demonstrated that proteins are unfolded during antigen translocation into the cytosol (Giodini and Cresswell, 2008), which might also enable them to transit also through a narrow pore complex.
More direct evidence for an involvement of Sec61 in antigen translocation was provided by experiments, in which Sec61 expression was down-regulated by siRNA (Imai et al., 2005). Such down-regulation prevented cytosolic translocation of the antigen and hence its degradation by the cytosolic proteasome, further supporting an important role of Sec61. However, since Sec61 also interferes with dislocation of MHC I molecules (Wiertz et al., 1996), it cannot be fully excluded that reduced cross-presentation observed in this study was due to an altered expression of MHC I molecules. Therefore, the exact role of Sec61 in antigen translocation for cross-presentation could not be unequivocally determined yet. Additionally, other members of the ERAD machinery, like derlin-1, have also been proposed as candidates to build the pore complex for antigen translocation across the endosomal membrane during cross-presentation (Lilley and Ploegh, 2004; Ye et al., 2004). But as for Sec61, future experiments are needed to reveal a decisive role of these proteins in intracellular antigen transport.
Another member of the ERAD machinery, which has been shown to play an important role in cross-presentation, is the soluble AAA ATPase p97 (Ackerman et al., 2006). P97, which is associated with both Sec61 and derlin-1, is recruited toward the endosomal membrane, where its ATPase activity provides the energy for antigen translocation. Expression of a dominant negative mutant of p97 has been demonstrated to abolish antigen translocation into the cytoplasm and hence cross-presentation (Ackerman et al., 2006; Zehner et al., 2011).
Recent evidence indicates that also Igtp, a protein involved in the generation of lipid bodies, influences antigen cross-presentation (Bougneres et al., 2009). Since Igtp deficiency abolishes cross-presentation but not MHC I-restricted presentation of antigens that were introduced directly into the cytoplasm, Igtp has been postulated as a putative regulator of antigen transport into the cytoplasm (Desjardins, 2009). Whether Igtp and/or lipid bodies indeed play a role in intracellular antigen translocation, however, remains to be elucidated.
After antigen translocation into the cytoplasm, it becomes ubiquitinated and processed by the cytoplasmic proteasome (Kovacsovics-Bankowski and Rock, 1995; Ackerman et al., 2003; Palmowski et al., 2006; Burgdorf et al., 2007, 2008). Importantly, the proteasome constitution in DCs differs from most other cell types. Within DCs, the standard catalytic subunits β1, β2, and β5 are replaced by β1i/LMP2, β2i/MECL-1, and β5i/LMP7 to build the immunoproteasome (Macagno et al., 1999). Such immunoproteasomes display an altered protease activity and cleavage site preference, resulting in the more efficient generation of MHC I epitopes (Kloetzel and Ossendorp, 2004) and the more efficient degradation of poly-ubiquitinated proteins (Seifert et al., 2010). Recent studies additionally reported of proteasomes intermediate between constitutive proteasomes and immunoproteasomes, in which only one or two catalytic subunits were replaced and which displayed an additional cleavage specificity (Guillaume et al., 2010), even enlarging the repertoire of antigens presented on MHC I molecules. The constitutive expression of such immunoproteasomes provides DCs with a unique capacity to generate a broad spectrum of peptides for loading on MHC I molecules.
Since antigens intended for cross-presentation generally enter the DC as proteasome substrates (Norbury et al., 2004), the half-life of the antigen is of crucial importance and epitopes that are degraded shortly after their synthesis are cross-presented very poorly (Wolkers et al., 2004).
Loading of Antigen-Derived Peptides on MHC I Molecules
Subsequent to proteasomal degradation, cross-presentation requires functional TAP activity (Kovacsovics-Bankowski and Rock, 1995; Huang et al., 1996; Song and Harding, 1996; Norbury et al., 1997; Ackerman et al., 2003, 2006), which led to the hypothesis that proteasome-derived peptides might enter the classical MHC I loading pathway in the ER (Kovacsovics-Bankowski and Rock, 1995). Although direct evidence supporting this hypothesis is missing, it was broadly accepted for years. First evidence that peptide loading for cross-presentation might occur in cellular compartments distinct from the ER was based on the observation that antigen-containing phagosomes contain members of the MHC I loading machinery such as calreticulin, ERp57, tapasin, β2-microglobulin, Sec61, MHC I itself, and functional TAP (Ackerman et al., 2003; Guermonprez et al., 2003; Houde et al., 2003). These observations lead to the assumption that proteasome-derived peptides might be re-imported into the same phagosomal compartment for loading onto MHC I molecules there. Indeed, after TAP-mediated peptide transport into these phagosomes, the generation of peptide-loaded MHC I molecules within these phagosomes could be detected (Guermonprez et al., 2003). Such intra-phagosomal peptide loading was further accomplished by the recruitment of proteasomes toward the phagosomal membrane (Houde et al., 2003), providing a spatial proximity of all components of the cross-presentation machinery, which might be essential to minimize rapid degradation of proteasome-derived peptides with very limited half-life (Reits et al., 2003) by cytosolic peptidases.
Formal evidence that peptide loading for cross-presentation indeed takes places in antigen-containing endosomes came from experiments that were aimed at inhibiting TAP activity in an endosome-specific fashion (Burgdorf et al., 2008). In this study, the soluble TAP inhibitor US6 (Ackerman et al., 2003), which inhibits TAP activity from its luminal side, was covalently linked to transferrin, resulting in its specific targeting to antigen-containing endosomes. Such endosome-specific targeting abolished TAP activity in endosomes without affecting TAP activity in the ER. By this approach, it was demonstrated that endosomal TAP was absolutely required for cross-presentation and that peptide loading for cross-presentation of MR-internalized antigens does not take place in the ER but occurs in antigen-containing endosomes. Such a spatial separation of endogenous MHC I-restricted antigen presentation and cross-presentation further supports the notion of a strong compartmentalization of MHC I-restricted antigen presentation (Lev et al., 2010), although peptide loading in the ER under certain circumstances cannot be excluded.
After proteasomal degradation and TAP-mediated transport into endosomal compartments, antigen-derived peptides must be trimmed into the suitable size for optimal binding to MHC I molecules, a function that is exerted in the endogenous MHC I presentation pathway by the ER-resident peptidase ERAP. Recent work by the group of Peter Van Endert identified IRAP as an endosome-specific peptidase required for such peptide-trimming in cross-presentation (Saveanu et al., 2009). IRAP, which is specifically targeted toward endosomes by its amino-terminal cytoplasmic tail (Hou et al., 2006), displays a broader pH optimum compared to ERAP, allowing IRAP activity at a slightly acidic endosomal pH (Georgiadou et al., 2010). IRAP activity might ensure that antigen-derived peptides, which were generated by proteasomal degradation in the cytoplasm and re-imported into the endosomes by endosomal TAP, are trimmed to their optimal size for loading on MHC I molecules, providing potent cross-presentation by the phagosome-to-cytosol pathway.
Cross-Presentation via Gap Junctions-Mediated Peptide Transfer
In addition to the vacuolar and the phagosome-to-cytosol cross-presentation pathway, it has been demonstrated that DCs can obtain peptides from other cells by gap junctions-mediated cell–cell contact (Neijssen et al., 2005; Mendoza-Naranjo et al., 2007). Such peptides are though to directly enter the endogenous MHC I-restricted presentation pathway via TAP-mediated transport into the ER. Saccheri et al. (2010) demonstrated that infection of melanoma cells with salmonella induced an upregulation of Cx43, which increased the formation of gap junctions with DCs. These gap junctions enabled peptide transfer from the melanoma cell toward the DC, which resulted in the induction of an anti-melanoma immune response (Neijssen et al., 2005; Mendoza-Naranjo et al., 2007; Saccheri et al., 2010). Whether cross-presentation via gap junctions-mediated antigen transfer has a broad physiological relevance, however, remains unclear, especially because cytoplasmic peptides are rapidly degraded by cytosolic peptidases and display a half-life of only a few seconds (Reits et al., 2003).
Cross-Dressing of DCs with Peptide–MHC I-Complexes
Independent of cross-presentation of internalized and processed antigens, DCs can also acquire MHC I molecules that are already loaded with antigen-derived peptides from a donor cell, a process that has been termed cross-dressing (Dolan et al., 2006; Smyth et al., 2008). Within this process, the antigen-presenting cell can obtain peptide–MHC I-complexes from a large variety of living or apoptotic donor cells. Presentation of such complexes to antigen-specific T cells does not require further processing by the DC. Transfer of the loaded MHC I molecules has been shown to be mediated by direct cell contact between the DC and the donor cell rather than by transfer of secreted vesicles like exosomes (Dolan et al., 2006; Wakim and Bevan, 2011). Such transfer occurred even at limited antigen concentrations (Smyth et al., 2008) and allows a direct antigen transfer from infected cells to DCs also in vivo (Dolan et al., 2006; Wakim and Bevan, 2011). The relevance of cross-dressing compared to direct or cross-presentation by DCs in the control of an infection remains to be analyzed further and will be the topic of intensive future investigations.
Influence of DC Maturation on Cross-Presentation and on the Recruitment of MHC Loading Machinery from the ER Toward Endosomes
In the absence of inflammatory stimuli, cross-presentation of internalized antigens, which occurs at moderate efficiency in immature DCs (Burgdorf et al., 2008), leads to T cell tolerance. Once the DC becomes activated by the recognition of microbial substances, its cross-presentation capacities are enhanced. First, in maturing DCs, total antigen uptake is increased (Gil-Torregrosa et al., 2004). Additionally, the composition of the ubiquitin–proteasome system is altered during DC maturation (Ebstein et al., 2009) and overall proteasomal activity is increased (Gil-Torregrosa et al., 2004). Finally, also antigen translocation into the cytoplasm is increased in maturing DCs (Gil-Torregrosa et al., 2004). Since such antigen translocation is mediated by proteins derived from the ER, the transport of these proteins toward the endosomes is an important prerequisite for cross-presentation.
A process termed ER-mediated phagocytosis has been postulated to mediate the transport of ER membrane components to endosomal compartments (Gagnon et al., 2002). In this process, the ER serves as a membrane donor for the developing phagosome, which then contains fragments of both the ER and the plasma membrane. This model, however, has been discussed controversially (Touret et al., 2005) and its physiological significance remains unclear. The same is true for transient fusions between the ER and endosomes after internalization, which has also been proposed to be a putative mechanism for delivery of ER components to endosomal membranes. The existence of such fusion events, however, has never been clearly demonstrated.
Increasing evidence points out that the transport of ER components to endosomes is a process that is controlled very tightly and that is increased during DC maturation. Goldszmid et al. (2009) demonstrated that the transport of ER components to Toxoplasma gondii-containing phagosomes only occurred if living protozoa were present in the phagosome. Additionally, our group demonstrated that TAP is only transported toward the endosomal membrane upon DC stimulation with LPS and due to the activation of the TLR4–MyD88 signaling pathway (Burgdorf et al., 2008). Likewise, the group of Peter Van Endert demonstrated that in unstimulated DCs, TAP is not present in IRAP-containing early endosomes (Saveanu et al., 2009). After phagocytosis of yeast cells, however, a clear TAP translocation from the ER toward the IRAP-containing endosomes was observed. Importantly, ERAP was not recruited to the antigen-containing endosomes, but maintained in the ER. This demonstrates that not all ER components are transported toward the endosome, but a process regulated by microbial substances rather induces the transport of only selected ER proteins toward the endosomal membrane. The selectivity of this transport also implicates that these ER components might not be recruited by ER-mediated endocytosis or by transient fusion events between the ER and the endosomes, since such events would result in an equal transport of all ER components toward the antigen-containing endosomes. These results might rather imply that upon stimulation with microbial substances, selective members of the ER undergo a directed ER-to-endosome transport.
Such TLR ligand-mediated transport from the ER toward endosomes reminds very much of the transport of several TLRs themselves. It has been demonstrated that TLR3, TLR7, and TLR9 in unstimulated DCs are localized in the ER (Latz et al., 2004). Upon DC stimulation with TLR ligands, however, these receptors are rapidly translocated toward early endosomes, from where their signaling takes place (Kagan et al., 2008). It is thinkable that, upon DC stimulation with endotoxin, some ER components involved in antigen presentation are translocated along with the TLRs toward early endosomes, where they can exert their function in cross-presentation. This transport has been shown to occur without passing the golgi and is regulated by the polytopic membrane protein UNC93B1 (Kim et al., 2008). Interestingly, a loss of function mutation of UNC93B1 has a severe influence on antigen presentation and in particular cross-presentation (Tabeta et al., 2006). Whether this loss of cross-presentation capacity indeed is due to an impaired transport of the cross-presentation machinery toward endosomes upon TLR signaling, however, still needs to be investigated.
Additional to the recruitment of ER components by TLR ligands, the endocytic receptor seems to play an important role for the recruitment of soluble ERAD components. In a recent study, we demonstrated that the MR, which targets its antigens specifically toward cross-presentation as described above, plays an important role in the recruitment of p97 (Zehner et al., 2011). During the ERAD process, p97 is recruited toward the ER by binding to poly-ubiquitinated proteins at the ER membrane (Ye et al., 2003). The recruitment of p97 toward the endosomal membrane for cross-presentation seems to be regulated in a very similar way. P97 recruitment for cross-presentation of MR-internalized antigens was regulated by ubiquitination of the MR (Zehner et al., 2011). Ligand binding to the MR induced poly-ubiquitination of its cytoplasmic tail. Without receptor poly-ubiquitination, no p97 recruitment toward the endosomal membrane took place and antigen transport into the cytoplasm and cross-presentation were impaired. These data demonstrate that the endocytic receptor is not only required for antigen targeting into a suited endosomal compartment for cross-presentation as described above, but also is of decisive importance for the antigen to get out of the endosomal compartment to reach the cytosol for proteasomal degradation.
Conclusion
In conclusion, it is important to emphasize that there are many different roads to cross-presentation. Whether extracellular antigens are cross-presented via the cytosolic or via the phagosome-to-cytosol pathway might be determined by the physiological conditions of both the antigen-presenting cell and the antigen itself or might even vary for different epitopes of the same antigen.
Furthermore, future experiments are needed to fully understand the molecular mechanisms underlying cross-presentation. Of special interest will be the identification of the pore complex that mediated antigen translocation into the cytoplasm, which without any doubt is one of the most important remaining open questions regarding cross-presentation. In this context, it will be important to unequivocally determine the role of Sec61 and the other postulated candidates in building the transmembrane pore complex.
Another question that will be subject of intense research is the transport of members of the MHC I loading machinery from the ER toward antigen-containing endosomes. Future experiments will show whether such components might be transported along with the different TLRs as postulated in this review, providing an explanation for the dependency of efficient cross-presentation on DC activation by TLR ligands.
Conflict of Interest Statement
The authors declare that the research was conducted in the absence of any commercial or financial relationships that could be construed as a potential conflict of interest.
Acknowledgments
This work is supported by the German Research Foundation grant BU2441/1-1 and consortium SFB645 project C1.
References
Ackerman, A. L., Giodini, A., and Cresswell, P. (2006). A role for the endoplasmic reticulum protein retrotranslocation machinery during cross-presentation by dendritic cells. Immunity 25, 607–617.
Ackerman, A. L., Kyritsis, C., Tampe, R., and Cresswell, P. (2003). Early phagosomes in dendritic cells form a cellular compartment sufficient for cross presentation of exogenous antigens. Proc. Natl. Acad. Sci. U.S.A. 100, 12889–12894.
Bachem, A., Guttler, S., Hartung, E., Ebstein, F., Schaefer, M., Tannert, A., Salama, A., Movassaghi, K., Opitz, C., Mages, H. W., Henn, V., Kloetzel, P. M., Gurka, S., and Kroczek, R. A. (2010). Superior antigen cross-presentation and XCR1 expression define human CD11c+CD141+ cells as homologues of mouse CD8+ dendritic cells. J. Exp. Med. 207, 1273–1281.
Backer, R., van Leeuwen, F., Kraal, G., and den Haan, J. M. (2008). CD8- dendritic cells preferentially cross-present Saccharomyces cerevisiae antigens. Eur. J. Immunol. 38, 370–380.
Baker, K., Qiao, S. W., Kuo, T. T., Aveson, V. G., Platzer, B., Andersen, J. T., Sandlie, I., Chen, Z., de Haar, C., Lencer, W. I., Fiebiger, E., and Blumberg, R. S. (2011). Neonatal Fc receptor for IgG (FcRn) regulates cross-presentation of IgG immune complexes by CD8-CD11b+ dendritic cells. Proc. Natl. Acad. Sci. U.S.A. 108, 9927–9932.
Bertholet, S., Goldszmid, R., Morrot, A., Debrabant, A., Afrin, F., Collazo-Custodio, C., Houde, M., Desjardins, M., Sher, A., and Sacks, D. (2006). Leishmania antigens are presented to CD8+T cells by a transporter associated with antigen processing-independent pathway in vitro and in vivo. J. Immunol. 177, 3525–3533.
Bevan, M. J. (1976). Cross-priming for a secondary cytotoxic response to minor H antigens with H-2 congenic cells which do not cross-react in the cytotoxic assay. J. Exp. Med. 143, 1283–1288.
Bonifaz, L. C., Bonnyay, D. P., Charalambous, A., Darguste, D. I., Fujii, S., Soares, H., Brimnes, M. K., Moltedo, B., Moran, T. M., and Steinman, R. M. (2004). In vivo targeting of antigens to maturing dendritic cells via the DEC-205 receptor improves T cell vaccination. J. Exp. Med. 199, 815–824.
Bougneres, L., Helft, J., Tiwari, S., Vargas, P., Chang, B. H., Chan, L., Campisi, L., Lauvau, G., Hugues, S., Kumar, P., Kamphorst, A. O., Dumenil, A. M., Nussenzweig, M., MacMicking, J. D., Amigorena, S., and Guermonprez, P. (2009). A role for lipid bodies in the cross-presentation of phagocytosed antigens by MHC class I in dendritic cells. Immunity 31, 232–244.
Burgdorf, S., Kautz, A., Bohnert, V., Knolle, P. A., and Kurts, C. (2007). Distinct pathways of antigen uptake and intracellular routing in CD4 and CD8 T cell activation. Science 316, 612–616.
Burgdorf, S., Scholz, C., Kautz, A., Tampe, R., and Kurts, C. (2008). Spatial and mechanistic separation of cross-presentation and endogenous antigen presentation. Nat. Immunol. 9, 558–566.
Cella, M., Engering, A., Pinet, V., Pieters, J., and Lanzavecchia, A. (1997). Inflammatory stimuli induce accumulation of MHC class II complexes on dendritic cells. Nature 388, 782–787.
Cella, M., Salio, M., Sakakibara, Y., Langen, H., Julkunen, I., and Lanzavecchia, A. (1999). Maturation, activation, and protection of dendritic cells induced by double-stranded RNA. J. Exp. Med. 189, 821–829.
Crozat, K., Guiton, R., Contreras, V., Feuillet, V., Dutertre, C. A., Ventre, E., Vu Manh, T. P., Baranek, T., Storset, A. K., Marvel, J., Boudinot, P., Hosmalin, A., Schwartz-Cornil, I., and Dalod, M. (2010). The XC chemokine receptor 1 is a conserved selective marker of mammalian cells homologous to mouse CD8alpha+ dendritic cells. J. Exp. Med. 207, 1283–1292.
Dasgupta, S., Navarrete, A. M., Bayry, J., Delignat, S., Wootla, B., Andre, S., Christophe, O., Nascimbeni, M., Jacquemin, M., Martinez-Pomares, L., Geijtenbeek, T. B., Moris, A., Saint-Remy, J. M., Kazatchkine, M. D., Kaveri, S. V., and Lacroix-Desmazes, S. (2007). A role for exposed mannosylations in presentation of human therapeutic self-proteins to CD4+ T lymphocytes. Proc. Natl. Acad. Sci. U.S.A. 104, 8965–8970.
Delamarre, L., Pack, M., Chang, H., Mellman, I., and Trombetta, E. S. (2005). Differential lysosomal proteolysis in antigen-presenting cells determines antigen fate. Science 307, 1630–1634.
den Haan, J. M., and Bevan, M. J. (2001). Antigen presentation to CD8+ T cells: cross-priming in infectious diseases. Curr. Opin. Immunol. 13, 437–441.
den Haan, J. M., Lehar, S. M., and Bevan, M. J. (2000). CD8(+) but not CD8(-) dendritic cells cross-prime cytotoxic T cells in vivo. J. Exp. Med. 192, 1685–1696.
Desjardins, M. (2009). The good fat: a link between lipid bodies and antigen cross-presentation. Immunity 31, 176–178.
Dolan, B. P., Gibbs, K. D. Jr., and Ostrand-Rosenberg, S. (2006). Dendritic cells cross-dressed with peptide MHC class I complexes prime CD8+ T cells. J. Immunol. 177, 6018–6024.
Dudziak, D., Kamphorst, A. O., Heidkamp, G. F., Buchholz, V. R., Trumpfheller, C., Yamazaki, S., Cheong, C., Liu, K., Lee, H. W., Park, C. G., Steinman, R. M., and Nussenzweig, M. C. (2007). Differential antigen processing by dendritic cell subsets in vivo. Science 315, 107–111.
Eberl, G., Widmann, C., and Corradin, G. (1996). The functional half-life of H-2Kd-restricted T cell epitopes on living cells. Eur. J. Immunol. 26, 1993–1999.
Ebstein, F., Lange, N., Urban, S., Seifert, U., Kruger, E., and Kloetzel, P. M. (2009). Maturation of human dendritic cells is accompanied by functional remodelling of the ubiquitin-proteasome system. Int. J. Biochem. Cell Biol. 41, 1205–1215.
Gagnon, E., Duclos, S., Rondeau, C., Chevet, E., Cameron, P. H., Steele-Mortimer, O., Paiement, J., Bergeron, J. J., and Desjardins, M. (2002). Endoplasmic reticulum-mediated phagocytosis is a mechanism of entry into macrophages. Cell 110, 119–131.
Georgiadou, D., Hearn, A., Evnouchidou, I., Chroni, A., Leondiadis, L., York, I. A., Rock, K. L., and Stratikos, E. (2010). Placental leucine aminopeptidase efficiently generates mature antigenic peptides in vitro but in patterns distinct from endoplasmic reticulum aminopeptidase 1. J. Immunol. 185, 1584–1592.
Gil-Torregrosa, B. C., Lennon-Dumenil, A. M., Kessler, B., Guermonprez, P., Ploegh, H. L., Fruci, D., van Endert, P., and Amigorena, S. (2004). Control of cross-presentation during dendritic cell maturation. Eur. J. Immunol. 34, 398–407.
Giodini, A., and Cresswell, P. (2008). Hsp90-mediated cytosolic refolding of exogenous proteins internalized by dendritic cells. EMBO J. 27, 201–211.
Giodini, A., Rahner, C., and Cresswell, P. (2009). Receptor-mediated phagocytosis elicits cross-presentation in nonprofessional antigen-presenting cells. Proc. Natl. Acad. Sci. U.S.A. 106, 3324–3329.
Goldszmid, R. S., Coppens, I., Lev, A., Caspar, P., Mellman, I., and Sher, A. (2009). Host ER-parasitophorous vacuole interaction provides a route of entry for antigen cross-presentation in Toxoplasma gondii-infected dendritic cells. J. Exp. Med. 206, 399–410.
Guermonprez, P., Saveanu, L., Kleijmeer, M., Davoust, J., Van Endert, P., and Amigorena, S. (2003). ER-phagosome fusion defines an MHC class I cross-presentation compartment in dendritic cells. Nature 425, 397–402.
Guillaume, B., Chapiro, J., Stroobant, V., Colau, D., Van Holle, B., Parvizi, G., Bousquet-Dubouch, M. P., Theate, I., Parmentier, N., and Van den Eynde, B. J. (2010). Two abundant proteasome subtypes that uniquely process some antigens presented by HLA class I molecules. Proc. Natl. Acad. Sci. U.S.A. 107, 18599–18604.
Hamman, B. D., Chen, J. C., Johnson, E. E., and Johnson, A. E. (1997). The aqueous pore through the translocon has a diameter of 40-60 A during cotranslational protein translocation at the ER membrane. Cell 89, 535–544.
He, L. Z., Crocker, A., Lee, J., Mendoza-Ramirez, J., Wang, X. T., Vitale, L. A., O’Neill, T., Petromilli, C., Zhang, H. F., Lopez, J., Rohrer, D., Keler, T., and Clynes, R. (2007). Antigenic targeting of the human mannose receptor induces tumor immunity. J. Immunol. 178, 6259–6267.
Heath, W. R., and Carbone, F. R. (2001). Cross-presentation in viral immunity and self-tolerance. Nat. Rev. Immunol. 1, 126–134.
Herre, J., Marshall, A. S., Caron, E., Edwards, A. D., Williams, D. L., Schweighoffer, E., Tybulewicz, V., Reis e Sousa, C., Gordon, S., and Brown, G. D. (2004). Dectin-1 uses novel mechanisms for yeast phagocytosis in macrophages. Blood 104, 4038–4045.
Hildner, K., Edelson, B. T., Purtha, W. E., Diamond, M., Matsushita, H., Kohyama, M., Calderon, B., Schraml, B. U., Unanue, E. R., Diamond, M. S., Schreiber, R. D., Murphy, T. L., and Murphy, K. M. (2008). Batf3 deficiency reveals a critical role for CD8alpha+ dendritic cells in cytotoxic T cell immunity. Science 322, 1097–1100.
Hotta, C., Fujimaki, H., Yoshinari, M., Nakazawa, M., and Minami, M. (2006). The delivery of an antigen from the endocytic compartment into the cytosol for cross-presentation is restricted to early immature dendritic cells. Immunology 117, 97–107.
Hou, J. C., Suzuki, N., Pessin, J. E., and Watson, R. T. (2006). A specific dileucine motif is required for the GGA-dependent entry of newly synthesized insulin-responsive aminopeptidase into the insulin-responsive compartment. J. Biol. Chem. 281, 33457–33466.
Houde, M., Bertholet, S., Gagnon, E., Brunet, S., Goyette, G., Laplante, A., Princiotta, M. F., Thibault, P., Sacks, D., and Desjardins, M. (2003). Phagosomes are competent organelles for antigen cross-presentation. Nature 425, 402–406.
Huang, A. Y., Bruce, A. T., Pardoll, D. M., and Levitsky, H. I. (1996). In vivo cross-priming of MHC class I-restricted antigens requires the TAP transporter. Immunity 4, 349–355.
Huang, A. Y., Golumbek, P., Ahmadzadeh, M., Jaffee, E., Pardoll, D., and Levitsky, H. (1994). Role of bone marrow-derived cells in presenting MHC class I-restricted tumor antigens. Science 264, 961–965.
Imai, J., Hasegawa, H., Maruya, M., Koyasu, S., and Yahara, I. (2005). Exogenous antigens are processed through the endoplasmic reticulum-associated degradation (ERAD) in cross-presentation by dendritic cells. Int. Immunol. 17, 45–53.
Jongbloed, S. L., Kassianos, A. J., McDonald, K. J., Clark, G. J., Ju, X., Angel, C. E., Chen, C. J., Dunbar, P. R., Wadley, R. B., Jeet, V., Vulink, A. J., Hart, D. N., and Radford, K. J. (2010). Human CD141+ (BDCA-3)+ dendritic cells (DCs) represent a unique myeloid DC subset that cross-presents necrotic cell antigens. J. Exp. Med. 207, 1247–1260.
Kagan, J. C., Su, T., Horng, T., Chow, A., Akira, S., and Medzhitov, R. (2008). TRAM couples endocytosis of Toll-like receptor 4 to the induction of interferon-beta. Nat. Immunol. 9, 361–368.
Kamphorst, A. O., Guermonprez, P., Dudziak, D., and Nussenzweig, M. C. (2010). Route of antigen uptake differentially impacts presentation by dendritic cells and activated monocytes. J. Immunol. 185, 3426–3435.
Kim, Y. M., Brinkmann, M. M., Paquet, M. E., and Ploegh, H. L. (2008). UNC93B1 delivers nucleotide-sensing toll-like receptors to endolysosomes. Nature 452, 234–238.
Kloetzel, P. M., and Ossendorp, F. (2004). Proteasome and peptidase function in MHC-class-I-mediated antigen presentation. Curr. Opin. Immunol. 16, 76–81.
Koopmann, J. O., Albring, J., Huter, E., Bulbuc, N., Spee, P., Neefjes, J., Hammerling, G. J., and Momburg, F. (2000). Export of antigenic peptides from the endoplasmic reticulum intersects with retrograde protein translocation through the Sec61p channel. Immunity 13, 117–127.
Kovacsovics-Bankowski, M., and Rock, K. L. (1995). A phagosome-to-cytosol pathway for exogenous antigens presented on MHC class I molecules. Science 267, 243–246.
Kukutsch, N. A., Rossner, S., Austyn, J. M., Schuler, G., and Ltz, M. B. (2000). Formation and kinetics of MHC class I-ovalbumin peptide complexes on immature and mature dendritic cells. J. Invest. Dermatol. 115, 449–453.
Kurts, C., Heath, W. R., Carbone, F. R., Allison, J., Miller, J. F., and Kosaka, H. (1996). Constitutive class I-restricted exogenous presentation of self antigens in vivo. J. Exp. Med. 184, 923–930.
Latz, E., Schoenemeyer, A., Visintin, A., Fitzgerald, K. A., Monks, B. G., Knetter, C. F., Lien, E., Nilsen, N. J., Espevik, T., and Golenbock, D. T. (2004). TLR9 signals after translocating from the ER to CpG DNA in the lysosome. Nat. Immunol. 5, 190–198.
Lev, A., Princiotta, M. F., Zanker, D., Takeda, K., Gibbs, J. S., Kumagai, C., Waffarn, E., Dolan, B. P., Burgevin, A., Van Endert, P., Chen, W., Bennink, J. R., and Yewdell, J. W. (2010). Compartmentalized MHC class I antigen processing enhances immunosurveillance by circumventing the law of mass action. Proc. Natl. Acad. Sci. U.S.A. 107, 6964–6969.
Lilley, B. N., and Ploegh, H. L. (2004). A membrane protein required for dislocation of misfolded proteins from the ER. Nature 429, 834–840.
Lin, M. L., Zhan, Y., Villadangos, J. A., and Lew, A. M. (2008). The cell biology of cross-presentation and the role of dendritic cell subsets. Immunol. Cell Biol. 86, 353–362.
Macagno, A., Gilliet, M., Sallusto, F., Lanzavecchia, A., Nestle, F. O., and Groettrup, M. (1999). Dendritic cells up-regulate immunoproteasomes and the proteasome regulator PA28 during maturation. Eur. J. Immunol. 29, 4037–4042.
Mantegazza, A. R., Savina, A., Vermeulen, M., Perez, L., Geffner, J., Hermine, O., Rosenzweig, S. D., Faure, F., and Amigorena, S. (2008). NADPH oxidase controls phagosomal pH and antigen cross-presentation in human dendritic cells. Blood 112, 4712–4722.
Martin-Fontecha, A., Sebastiani, S., Hopken, U. E., Uguccioni, M., Lipp, M., Lanzavecchia, A., and Sallusto, F. (2003). Regulation of dendritic cell migration to the draining lymph node: impact on T lymphocyte traffic and priming. J. Exp. Med. 198, 615–621.
McKenzie, E. J., Taylor, P. R., Stillion, R. J., Lucas, A. D., Harris, J., Gordon, S., and Martinez-Pomares, L. (2007). Mannose receptor expression and function define a new population of murine dendritic cells. J. Immunol. 178, 4975–4983.
Mellman, I., and Steinman, R. M. (2001). Dendritic cells: specialized and regulated antigen processing machines. Cell 106, 255–258.
Mendoza-Naranjo, A., Saez, P. J., Johansson, C. C., Ramirez, M., Mandakovic, D., Pereda, C., Lopez, M. N., Kiessling, R., Saez, J. C., and Salazar-Onfray, F. (2007). Functional gap junctions facilitate melanoma antigen transfer and cross-presentation between human dendritic cells. J. Immunol. 178, 6949–6957.
Neijssen, J., Herberts, C., Drijfhout, J. W., Reits, E., Janssen, L., and Neefjes, J. (2005). Cross-presentation by intercellular peptide transfer through gap junctions. Nature 434, 83–88.
Nishi, T., and Forgac, M. (2002). The vacuolar (H+)-ATPases – nature’s most versatile proton pumps. Nat. Rev. Mol. Cell Biol. 3, 94–103.
Norbury, C. C., Basta, S., Donohue, K. B., Tscharke, D. C., Princiotta, M. F., Berglund, P., Gibbs, J., Bennink, J. R., and Yewdell, J. W. (2004). CD8+ T cell cross-priming via transfer of proteasome substrates. Science 304, 1318–1321.
Norbury, C. C., Chambers, B. J., Prescott, A. R., Ljunggren, H. G., and Watts, C. (1997). Constitutive macropinocytosis allows TAP-dependent major histocompatibility complex class I presentation of exogenous soluble antigen by bone marrow-derived dendritic cells. Eur. J. Immunol. 27, 280–288.
Palmowski, M. J., Gileadi, U., Salio, M., Gallimore, A., Millrain, M., James, E., Addey, C., Scott, D., Dyson, J., Simpson, E., and Cerundolo, V. (2006). Role of immunoproteasomes in cross-presentation. J. Immunol. 177, 983–990.
Pooley, J. L., Heath, W. R., and Shortman, K. (2001). Cutting edge: intravenous soluble antigen is presented to CD4 T cells by CD8- dendritic cells, but cross-presented to CD8 T cells by CD8+ dendritic cells. J. Immunol. 166, 5327–5330.
Poulin, L. F., Salio, M., Griessinger, E., Anjos-Afonso, F., Craciun, L., Chen, J. L., Keller, A. M., Joffre, O., Zelenay, S., Nye, E., Le Moine, A., Faure, F., Donckier, V., Sancho, D., Cerundolo, V., Bonnet, D., and Reis e Sousa, C. (2010). Characterization of human DNGR-1+ BDCA3+ leukocytes as putative equivalents of mouse CD8alpha+ dendritic cells. J. Exp. Med. 207, 1261–1271.
Purcell, A. W., and Elliott, T. (2008). Molecular machinations of the MHC-I peptide loading complex. Curr. Opin. Immunol. 20, 75–81.
Reits, E., Griekspoor, A., Neijssen, J., Groothuis, T., Jalink, K., van Veelen, P., Janssen, H., Calafat, J., Drijfhout, J. W., and Neefjes, J. (2003). Peptide diffusion, protection, and degradation in nuclear and cytoplasmic compartments before antigen presentation by MHC class I. Immunity 18, 97–108.
Rescigno, M., Citterio, S., Thery, C., Rittig, M., Medaglini, D., Pozzi, G., Amigorena, S., and Ricciardi-Castagnoli, P. (1998). Bacteria-induced neo-biosynthesis, stabilization, and surface expression of functional class I molecules in mouse dendritic cells. Proc. Natl. Acad. Sci. U.S.A. 95, 5229–5234.
Rock, K. L., and Shen, L. (2005). Cross-presentation: underlying mechanisms and role in immune surveillance. Immunol. Rev. 207, 166–183.
Rodriguez, A., Regnault, A., Kleijmeer, M., Ricciardi-Castagnoli, P., and Amigorena, S. (1999). Selective transport of internalized antigens to the cytosol for MHC class I presentation in dendritic cells. Nat. Cell Biol. 1, 362–368.
Saccheri, F., Pozzi, C., Avogadri, F., Barozzi, S., Faretta, M., Fusi, P., and Rescigno, M. (2010). Bacteria-induced gap junctions in tumors favor antigen cross-presentation and antitumor immunity. Sci. Transl. Med. 2, 44–57.
Saveanu, L., Carroll, O., Weimershaus, M., Guermonprez, P., Firat, E., Lindo, V., Greer, F., Davoust, J., Kratzer, R., Keller, S. R., Niedermann, G., and van Endert, P. (2009). IRAP identifies an endosomal compartment required for MHC class I cross-presentation. Science 325, 213–217.
Savina, A., Jancic, C., Hugues, S., Guermonprez, P., Vargas, P., Moura, I. C., Lennon-Dumenil, A. M., Seabra, M. C., Raposo, G., and Amigorena, S. (2006). NOX2 controls phagosomal pH to regulate antigen processing during crosspresentation by dendritic cells. Cell 126, 205–218.
Savina, A., Peres, A., Cebrian, I., Carmo, N., Moita, C., Hacohen, N., Moita, L. F., and Amigorena, S. (2009). The small GTPase Rac2 controls phagosomal alkalinization and antigen crosspresentation selectively in CD8(+) dendritic cells. Immunity 30, 544–555.
Schnorrer, P., Behrens, G. M., Wilson, N. S., Pooley, J. L., Smith, C. M., El-Sukkari, D., Davey, G., Kupresanin, F., Li, M., Maraskovsky, E., Belz, G. T., Carbone, F. R., Shortman, K., Heath, W. R., and Villadangos, J. A. (2006). The dominant role of CD8+ dendritic cells in cross-presentation is not dictated by antigen capture. Proc. Natl. Acad. Sci. U.S.A. 103, 10729–10734.
Segura, E., and Villadangos, J. A. (2011). A modular and combinatorial view of the antigen cross-presentation pathway in dendritic cells. Traffic 12, 1677–1685.
Seifert, U., Bialy, L. P., Ebstein, F., Bech-Otschir, D., Voigt, A., Schroter, F., Prozorovski, T., Lange, N., Steffen, J., Rieger, M., Kuckelkorn, U., Aktas, O., Kloetzel, P. M., and Krüger, E. (2010). Immunoproteasomes preserve protein homeostasis upon interferon-induced oxidative stress. Cell 142, 613–624.
Shen, L., Sigal, L. J., Boes, M., and Rock, K. L. (2004). Important role of cathepsin S in generating peptides for TAP-independent MHC class I crosspresentation in vivo. Immunity 21, 155–165.
Shimada, K., Takimoto, H., Yano, I., and Kumazawa, Y. (2006). Involvement of mannose receptor in glycopeptidolipid-mediated inhibition of phagosome-lysosome fusion. Microbiol. Immunol. 50, 243–251.
Sigal, L. J., Crotty, S., Andino, R., and Rock, K. L. (1999). Cytotoxic T-cell immunity to virus-infected non-haematopoietic cells requires presentation of exogenous antigen. Nature 398, 77–80.
Smyth, L. A., Harker, N., Turnbull, W., El-Doueik, H., Klavinskis, L., Kioussis, D., Lombardi, G., and Lechler, R. (2008). The relative efficiency of acquisition of MHC: peptide complexes and cross-presentation depends on dendritic cell type. J. Immunol. 181, 3212–3220.
Song, R., and Harding, C. V. (1996). Roles of proteasomes, transporter for antigen presentation (TAP), and beta 2-microglobulin in the processing of bacterial or particulate antigens via an alternate class I MHC processing pathway. J. Immunol. 156, 4182–4190.
Sweet, L., Singh, P. P., Azad, A. K., Rajaram, M. V., Schlesinger, L. S., and Schorey, J. S. (2010). Mannose receptor-dependent delay in phagosome maturation by Mycobacterium avium glycopeptidolipids. Infect. Immun. 78, 518–526.
Tabeta, K., Hoebe, K., Janssen, E. M., Du, X., Georgel, P., Crozat, K., Mudd, S., Mann, N., Sovath, S., Goode, J., Shamel, L., Herskovits, A. A., Portnoy, D. A., Cooke, M., Tarantino, L. M., Wiltshire, T., Steinberg, B. E., Grinstein, S., and Beutler, B. (2006). The Unc93b1 mutation 3d disrupts exogenous antigen presentation and signaling via Toll-like receptors 3, 7 and 9. Nat. Immunol. 7, 156–164.
Tacken, P. J., de Vries, I. J., Gijzen, K., Joosten, B., Wu, D., Rother, R. P., Faas, S. J., Punt, C. J., Torensma, R., Adema, G. J., and Figdor, C. G. (2005). Effective induction of naive and recall T-cell responses by targeting antigen to human dendritic cells via a humanized anti-DC-SIGN antibody. Blood 106, 1278–1285.
Tacken, P. J., Ginter, W., Berod, L., Cruz, L. J., Joosten, B., Sparwasser, T., Figdor, C. G., and Cambi, A. (2011). Targeting DC-SIGN via its neck region leads to prolonged antigen residence in early endosomes, delayed lysosomal degradation, and cross-presentation. Blood 118, 4111–4119.
Touret, N., Paroutis, P., Terebiznik, M., Harrison, R. E., Trombetta, S., Pypaert, M., Chow, A., Jiang, A., Shaw, J., Yip, C., Moore, H. P., van der Wel, N., Houben, D., Peters, P. J., de Chastellier, C., Mellman, I., and Grinstein, S. (2005). Quantitative and dynamic assessment of the contribution of the ER to phagosome formation. Cell 123, 157–170.
Trombetta, E. S., Ebersold, M., Garrett, W., Pypaert, M., and Mellman, I. (2003). Activation of lysosomal function during dendritic cell maturation. Science 299, 1400–1403.
Tucker, S. C., and Casadevall, A. (2002). Replication of Cryptococcus neoformans in macrophages is accompanied by phagosomal permeabilization and accumulation of vesicles containing polysaccharide in the cytoplasm. Proc. Natl. Acad. Sci. U.S.A. 99, 3165–3170.
Van den Berg, B., Clemons, W. M. Jr., Collinson, I., Modis, Y., Hartmann, E., Harrison, S. C., and Rapoport, T. A. (2004). X-ray structure of a protein-conducting channel. Nature 427, 36–44.
van Montfoort, N., Camps, M. G., Khan, S., Filippov, D. V., Weterings, J. J., Griffith, J. M., Geuze, H. J., van Hall, T., Verbeek, J. S., Melief, C. J., and Ossendorp, F. (2009). Antigen storage compartments in mature dendritic cells facilitate prolonged cytotoxic T lymphocyte cross-priming capacity. Proc. Natl. Acad. Sci. U.S.A. 106, 6730–6735.
Wakim, L. M., and Bevan, M. J. (2011). Cross-dressed dendritic cells drive memory CD8+ T-cell activation after viral infection. Nature 471, 629–632.
Weck, M. M., Appel, S., Werth, D., Sinzger, C., Bringmann, A., Grunebach, F., and Brossart, P. (2008). hDectin-1 is involved in uptake and cross-presentation of cellular antigens. Blood 111, 4264–4272.
Werneburg, N. W., Guicciardi, M. E., Bronk, S. F., and Gores, G. J. (2002). Tumor necrosis factor-alpha-associated lysosomal permeabilization is cathepsin B dependent. Am. J. Physiol. Gastrointest. Liver Physiol. 283, G947–G956.
Wiertz, E. J., Tortorella, D., Bogyo, M., Yu, J., Mothes, W., Jones, T. R., Rapoport, T. A., and Ploegh, H. L. (1996). Sec61-mediated transfer of a membrane protein from the endoplasmic reticulum to the proteasome for destruction. Nature 384, 432–438.
Wolkers, M. C., Brouwenstijn, N., Bakker, A. H., Toebes, M., and Schumacher, T. N. (2004). Antigen bias in T cell cross-priming. Science 304, 1314–1317.
Ye, Y., Meyer, H. H., and Rapoport, T. A. (2003). Function of the p97-Ufd1-Npl4 complex in retrotranslocation from the ER to the cytosol: dual recognition of nonubiquitinated polypeptide segments and polyubiquitin chains. J. Cell Biol. 162, 71–84.
Ye, Y., Shibata, Y., Yun, C., Ron, D., and Rapoport, T. A. (2004). A membrane protein complex mediates retro-translocation from the ER lumen into the cytosol. Nature 429, 841–847.
Keywords: dendritic cells, cross-presentation, antigen storage compartments, endosomes
Citation: Kreer C, Rauen J, Zehner M and Burgdorf S (2012) Cross-presentation: how to get there – or how to get the ER. Front. Immun. 2:87. doi: 10.3389/fimmu.2011.00087
Received: 04 November 2011; Accepted: 16 December 2011;
Published online: 03 January 2012.
Edited by:
Christian Kurts, Friedrich Wilhelms-Universität Bonn, GermanyReviewed by:
Peter Michael Kloetzel, Charite-Universitatsmedizin Berlin, GermanyJoke M. M. Den Haan, VU University Medical Center, Netherlands
Copyright: © 2012 Kreer, Rauen, Zehner and Burgdorf. This is an open-access article distributed under the terms of the Creative Commons Attribution Non Commercial License, which permits non-commercial use, distribution, and reproduction in other forums, provided the original authors and source are credited.
*Correspondence: Sven Burgdorf, Laboratory of Cellular Immunology, Life and Medical Sciences Institute, Carl-Troll-Str. 31, 53105 Bonn, Germany. e-mail:YnVyZ2RvcmZAdW5pLWJvbm4uZGU=
† Christoph Kreer, Judith Rauen and Matthias Zehner have contributed equally to this work.