- 1 Institutes of Molecular Medicine and Experimental Immunology, Rheinische Friedrich-Wilhelms-University, Bonn, Germany
- 2 Institute for Medical Microbiology, Immunology and Hygiene, Technical University of Munich, Munich, Germany
Dendritic cells (DCs) are the main inducers and regulators of cytotoxic T lymphocyte (CTL) responses against viruses and tumors. One checkpoint to avoid misguided CTL activation, which might damage healthy cells of the body, is the necessity for multiple activation signals, involving both antigenic as well as additional signals that reflect the presence of pathogens. DCs provide both signals when activated by ligands of pattern recognition receptors and “licensed” by helper lymphocytes. Recently, it has been established that such T cell licensing can be facilitated by CD4+ T helper cells (“classical licensing”) or by natural killer T cells (“alternative licensing”). Licensing regulates the DC/CTL cross-talk at multiple layers. Direct recruitment of CTLs through chemokines released by licensed DCs has recently emerged as a common theme and has a crucial impact on the efficiency of CTL responses. Here, we discuss recent advances in our understanding of DC licensing for cross-priming and implications for the temporal and spatial regulation underlying this process. Future vaccination strategies will benefit from a deeper insight into the mechanisms that govern CTL activation.
Introduction
Dendritic cells (DCs) are responsible for activating T cells. CD8+ T cells, also referred to as cytotoxic T lymphocytes (CTLs), are pivotal for host defense against infection with viruses, intracellular bacteria, and parasites, and for immune surveillance of tumor cells, by scanning the surfaces of body cells for alterations. They detect and destroy infected or transformed cells after T cell receptor (TCR)-mediated recognition of, e.g., endogenous viral peptide antigens, which are presented on major histocompatibility complex (MHC) class I molecules on the cell surface. However, the risk of misguided destruction of healthy cells by autoreactive CTLs is diminished by the need for additional activation signals that are upregulated under inflammatory conditions. These signals can only be provided by professional antigen-presenting cells (APCs). However, if an intracellular pathogen does not infect APCs, or if a tumor is not derived from an APC, there must be mechanisms that allow for presentation of exogenous antigen to naïve CTLs. Indeed, APCs possess the ability to acquire and process antigens derived from virus-infected or tumor cells in a process called cross-priming. The cell type best characterized to be capable of this task are murine CD8+ DCs (Shortman and Heath, 2010), but also B cells (Hon et al., 2005), certain macrophages (Ramirez and Sigal, 2002), neutrophilic granulocytes (Tvinnereim et al., 2004), mast cells, and liver sinusoidal endothelial cells (Limmer et al., 2000) have been reported to be capable of cross-presentation.
Since its first description in 1976 (Bevan, 1976), cross-priming has been found to be implicated in a broad variety of physiological and pathophysiological processes, ranging from host defense against viruses and vaccinations to CTL-mediated autoimmune disease (Kurts et al., 2010). Under normal conditions, cross-presentation of self-antigens leads to deletion of autoreactive CTLs rather than to an autoimmune response, a phenomenon termed cross-tolerance (Kurts et al., 1997).
Conferring cytotoxic effector functions to CTLs by cross-priming requires more than mere antigen cross-presentation. This realization caused Cohn and Bretscher in 1970 to formulate their two-signal hypothesis (Bretscher and Cohn, 1970), in which the first signal defines the specificity of the T cell response, and the presence of the second signal caused this response to be immunogenic. B7 costimulatory molecules were found to be required for immunity, and were proposed to be the molecular equivalent of the enigmatic signal 2 (Allison, 1994). Later it became clear that B7 molecules are important, but neither essential nor sufficient for all immune responses. Furthermore, a third signal was postulated that governs the functional differentiation of the T cell to be activated (Curtsinger et al., 1999). The molecular correlates of these three signals are:
(i) peptide antigen presented on MHC class I molecules,
(ii) costimulatory molecules of the immunoglobulin superfamily (particularly B7, i.e., CD80 and CD86, which bind to CD28 on T cells) and the tumor necrosis factor (TNF) superfamily (such as the ligand/receptor pairs CD40L/CD40, 4-1BBL/4-1BB, CD27/CD70, CD30L/CD30, and HVEM/LIGHT),
(iii) cytokines that stimulate CD8+ T cell expansion and differentiation [IL-12, type I interferon (IFN), IL-2].
Originally, CD8α+ DCs in lymphoid tissue had been described as the main cell type capable of cross-presentation (Den Haan et al., 2000). However, CD103+ DCs in peripheral tissues have been identified to share this specialization (Del Rio et al., 2007; Bedoui et al., 2009). Cross-presenting DCs express endocytosis receptors, including DC-SIGN, CD205, CD206, CD36, or Clec9A (DNGR-1; Dudziak et al., 2007; Burgdorf and Kurts, 2008; Caminschi et al., 2008; Sancho et al., 2009), which enable them to direct exogenous antigens toward special endosomes where cross-presentation is believed to occur (Burgdorf et al., 2008).
Until recently, the human correlates of murine CD8α+ DCs were unknown, as human DCs do not express CD8. In 2010, several groups identified the human CD141+ (=BDCA3) DCs as a CD8α+-like DC subset (Bachem et al., 2010; Crozat et al., 2010; Jongbloed et al., 2010; Poulin et al., 2010). These cells share expression of Clec9A, XCR1, and Necl2, as well as developmental dependence on Batf3, IRF8, and Id2 with their murine equivalents. In addition, these cells express TLR3, suggesting that similar to what has been reported for mice, cross-priming by human DCs might be enhanced by endosomal recognition of double-stranded RNA (Schulz et al., 2005). Among the functions in common between human and mouse CD8α+-like DCs are IL-12 production, IFN-λ expression, and cross-presentation of antigens derived from dead cell uptake (Jongbloed et al., 2010; Lauterbach et al., 2010; Poulin et al., 2010).
The Requirement for a “License”
It is essential that CD8+ T cell activation be tightly regulated in order to minimize destruction of uninfected or non-malignant host tissue. Therefore, the initiation of T cell responses is controlled by a series of checkpoints that allow APCs to selectively deliver all three signals for CTL activation. A DC that has been properly activated for this purpose is referred to as a DC “licensed” for cross-priming (Belz et al., 2004).
One such requirement for efficient cross-priming is the stimulation through innate pattern recognition receptors (PRR) that recognize pathogen-associated molecular patterns (PAMPs) on foreign invaders (Maurer et al., 2002). The PRR-induced maturation of DCs and their subsequent migration to secondary lymphoid organs (SLOs) confirm the “relevance” of the cross-presented antigen and its “eligibility” to mount an immunogenic response.
The phenotypic changes induced by PRR signaling in DCs have been extensively studied. For instance, the activation of TLRs upregulates costimulatory molecules on DCs and thus increases signal 2 for efficient cross-priming (Schulz et al., 2005). A recent study indicated that antigen cross-presentation occurred despite the lack of a PRR signal, but failed to result in T cell cross-priming (Keller et al., 2010). Thus, innate stimuli were suggested to enhance cross-priming without regulating antigen presentation, consistent with the classical two-signal hypothesis (Bretscher and Cohn, 1970). However, a previous study has reported enhanced peptide-loading on MHC class I molecules after TLR triggering, facilitated by intracellular relocation of the peptide-loading complex toward antigen-containing endosomes (Burgdorf et al., 2008).
In addition to TLRs, other PRRs have been implicated in the regulation of cross-priming efficiency. For instance, C-type lectin receptors have been found to induce DC maturation and upregulation of costimulatory molecules (Rogers et al., 2005). Moreover, intracellular PRRs such as RIG-I have also been suggested to enhance CTL responses (Loo and Gale, 2011). Their role in the regulation of cross-priming, however, remains to be clarified. Furthermore, the cytokine GM-CSF has been shown to preferentially stimulate cross-presenting DCs (Campbell et al., 2011; Zhan et al., 2011). The source of such GM-CSF can be cross-primed CTL themselves (Min et al., 2010).
At present, it is unclear how the PRR signal reaches the DC that takes up extracellular antigen for cross-presentation (Iwasaki and Medzhitov, 2010). Nonetheless, it is plausible that, similar to the antigen, the PRR ligand might be taken up by endocytosis, which makes endosomal TLRs likely PRR candidates for DC activation for cross-priming. Indeed, the enhancement of cross-priming is especially effective after TLR3 and TLR9 stimulation (Maurer et al., 2002; Schulz et al., 2005).
Dendritic cells may acquire antigen also by transfer of peptides (Neijssen et al., 2005), or as preformed MHC-peptide complexes, the latter of which has been termed cross-dressing (Dolan et al., 2006). Recently, a role for cross-dressing has been described in memory CTL restimulation, where infected APCs transferred peptide-MHC complexes to the surface of uninfected DCs (Wakim and Bevan, 2011), thereby providing them with signal 1 without the need for further antigen processing. Whether the recipient DCs need an additional license to activate T cells remains unclear. However, this need should not be critical in the reactivation of memory T cells, because these have already undergone a rigorous activation control during their initial priming.
Classical Licensing via CD4+ T Helper Cells
Apart from signals delivered via innate immune receptors, efficient cross-priming requires the interaction of DCs with antigen-specific lymphocytes. Bennett et al. (1998) described that the induction of cytotoxic T cell responses depends on the previous activation of a DC by a CD4+ T helper (Th) cell (Bennett et al., 1998). This concept was termed “cognate licensing,” indicating that the DC presents the same antigen on MHC class II to the CD4+ Th cell and on MHC class I to the CD8+ T cell. By functioning as a “second opinion” prior to the elicitation of an efficient CTL response, the need for this additional interaction reduces the risk of inappropriate responses against innocuous antigens or self-peptides. CTLs that did not receive T cell help during priming (called “helpless” CTLs) show altered effector functions and lack the capacity to maintain a sustained or memory CD8+ T cell response (Qiu and Cui, 2007).
It has been shown that the upregulation of costimulatory molecules on DCs is insufficient for breaking cross-tolerance to autoantigens. In contrast, Th cells specific for self-antigens did provide signals to elicit an immunogenic T cells response (Hamilton-Williams et al., 2005). Although autoreactive T cells are present in the periphery despite negative selection in the thymus, an encounter of the antigen-presenting DC with a CD4+ Th cell and a CTL specific for the same self-antigen seems very unlikely. Thus, the need for antigen-specific Th cells to license DCs for cross-priming serves as an additional checkpoint that contributes to the maintenance of peripheral tolerance to self.
The mechanism of T cell help for cross-priming includes a plethora of interactions between cell membrane-bound molecules (Figure 1). The signal that has been repeatedly reported to be essential for this process derives from the interaction of CD40 on the DC with its ligand CD40L (CD154) on Th cells (Bennett et al., 1998). One main mechanism of CD40–CD40L interaction is the induction of IL-12 production as a signal 3 for CTL activation (Schulz et al., 2000). CD40 upregulation requires innate signals through TLRs (Gallucci et al., 1999) or C-type lectins (Geijtenbeek et al., 2004). In turn, the consecutive signal provided by Th cells through CD40L is important for further activation of the DC, including the upregulation of costimulatory molecules and the secretion of cytokines necessary for CTL activation (Grewal and Flavell, 1998). Of note, one study reported that CD40 stimulation could replace Th cell-mediated licensing in vitro (Ridge et al., 1998), raising the question of whether CD40 ligation alone is sufficient as a final step in DC activation. A contrasting view supports the opinion that different receptor–ligand combinations might have overlapping functions and can all contribute to reaching a threshold level of DC activation that renders the DC capable of cross-priming (Castellino and Germain, 2006), assuming that a certain number of signals must exist that confirm the “immunogenic relevance” of the cross-presented antigen before a CTL response is elicited.
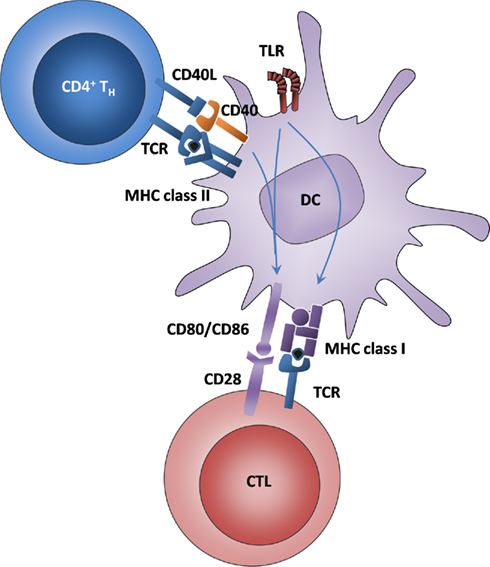
Figure 1. Classical DC licensing for cross-priming is mediated by Th cells and enhanced by TLR ligands. DCs interact with Th cells via antigen that is presented on MHC class II and recognized by the TCR and via CD40–CD40L interactions. The signals downstream of CD40 and TLRs lead to the upregulation of MHC class I and CD80/86, thus allowing the DC to interact with CTL.
Alternative Licensing via NKT Cells
Recently, natural killer T (NKT) cells were identified as a second cell type capable of cognate DC licensing (Semmling et al., 2010). NKT cells expressing invariant TCRs recognize glycolipid antigens presented by DCs on the MHC-like molecule CD1d. The synthetic NKT cell ligand α-galactosylceramide (αGalCer) has been widely used as a model antigen in many studies, however pathogen-associated antigens and the mode of NKT cell activation in different disease models have to be further clarified. A recent study showed that house dust extracts contain NKT cell-activating antigens which function as adjuvants in NKT cell-dependent immunization models, suggesting a role for NKT cells in the response to environmental antigens (Wingender et al., 2011). Upon recognition of their antigen, NKT cells can induce the upregulation of costimulatory molecules on DCs (Fujii et al., 2003). This interaction also activates NKT cells to upregulate CD40L and to rapidly produce cytokines. CD40 signaling plays a crucial role in the activation process, and CD40-deficient mice are unable to develop enhanced CD8+ T cell responses despite the upregulation of DC maturation markers (Fujii et al., 2004).
The NKT cell-DC interaction was recently defined as a bona fide cognate licensing process, since the very same DC had to present the glycolipid antigen to NKT cells and the peptide antigen to CTLs in order to prime an efficient cytotoxic response (Figure 2). Furthermore, this “alternative” DC licensing occurred completely independent from “classical” Th cell-mediated licensing, since it was unimpaired in the absence of CD4+ Th cells (Semmling et al., 2010). Although the role of alternative DC licensing in the host defense against infections and in immune-mediated diseases still remains to be demonstrated, it appears that at least two analogous systems have evolved to synergistically license DCs for CTL cross-priming after presentation of peptide and lipid antigens, respectively.
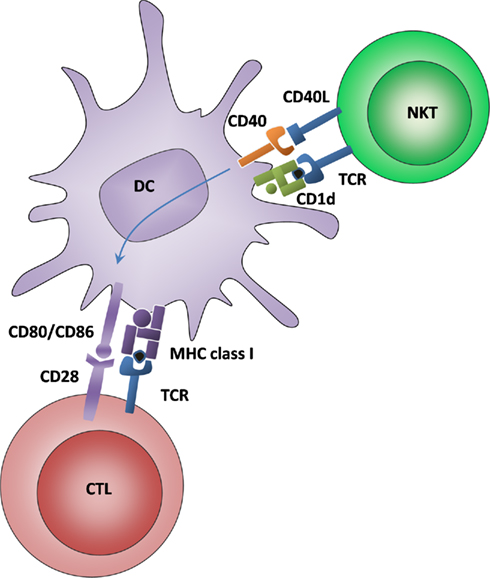
Figure 2. Alternative DC licensing for cross-priming is mediated by NKT cells recognizing glycolipid antigens. DCs interact with NKT cells via glycolipid antigen that is presented on CD1d and recognized by an invariant TCR. Moreover, CD40–CD40L interactions lead to an upregulation of costimulatory molecules for CTL cross-priming.
Chemokines as Regulators of Cross-Priming
The concept of DC licensing implies that at least three rare immune cells – a DC capable of antigen cross-presentation, an antigen-specific CTL, and an antigen-specific Th or NKT cell – have to physically interact. Chemokines and their receptors are potent agents regulating such multi-cellular interactions. The best-described example are CCR7 and its ligands CCL19 and CCL21, which coordinate attraction of both antigen-presenting DCs and naïve T cells into the T cell zone of SLOs. By recruiting both cell types to the same compartment inside of SLOs, CCR7 increases the likeliness of an encounter between a naïve T cell and its cognate antigen on a DC (Forster et al., 2008). Moreover, these chemokines increase the basal motility of lymphocytes in SLOs and thus provide an additional means to facilitate DC/T cell encounters (Worbs et al., 2007). However, the regulation of cognate DC licensing necessitates an even more precise positioning involving more than two cells. To date, several chemokine/chemokine receptor pairs have been shown to facilitate this task.
CCR5 and Its Ligands
Castellino et al. (2006) first described the involvement of a chemokine/chemokine receptor pair in the recruitment of CD8 T+ cells for cross-priming. They showed that CTL were preferentially attracted toward mature DCs that had previously been licensed by a Th cell. This attraction depended on CCR5 expression on CTLs and happened in an antigen-independent way. CCR5 is upregulated on naïve CTLs in the inflammatory milieu of a “challenged” SLO. The CCR5 ligands CCL3 and CCL4 are produced by Th cells and DCs after their antigen-specific interaction, thereby creating a chemokine micromilieu which recruits naïve CCR5-expressing CTLs. Subsequent studies showed that these chemokines surround a network of fibroblastic reticular cells (FRCs) which serve as roadways for naïve CTLs (Germain et al., 2008). Together, the chemokine trace and the FRC network facilitate efficient CD8+ T cell migration toward DCs licensed for cross-priming.
A subsequent report by Hugues et al. (2007) confirmed the involvement of CCR5 in T cell help and extended it by demonstrating that CTLs themselves can contribute to CCR5-dependend attraction of further naïve CTLs toward DCs that evidently have cross-primed successfully.
The CCR4 – CCL17 Axis
The production of CCR5 ligands does not play a role in NKT cell-mediated DC licensing. Instead, alternatively licensed splenic CD8α+ DCs produced CCL17 (Semmling et al., 2010). After systemic administration of αGalCer, these DCs specifically attracted naïve CTLs expressing the CCL17 receptor CCR4, thereby greatly enhancing the efficiency of the cross-priming. When DCs could not produce CCL17, or if CTLs did not express CCR4, the enhancement of cross-priming by NKT cells was mostly abrogated. Furthermore, the very same DC that produced CCL17 also cross-presented antigen to CTLs, demonstrating that chemokine production is a specific feature of alternatively licensed DC.
The identification of distinct chemokine/chemokine receptor pairs for classical and alternative licensing support their independent roles in the initiation and enhancement of CTL cross-priming. In addition, these findings suggest that the principle of chemokine-mediated CTL recruitment toward antigen-presenting DCs is a common component of “help” delivered to DCs through cognate licensing.
CXCR3 and Its Ligands
Until recently, it was thought that once primed in SLOs by a licensed DC, CTLs can freely enter inflamed tissues and sites of infection in the periphery, depending of the inflammatory chemokine receptors they express. Interestingly, however, a study by Nakanishi et al. (2009) suggested that at least for some tissues, T cell help by CD4+ Th cells in the periphery is required for efficient CTL recruitment because when transferred to a mouse lacking CD4+ Th cells, CTL accumulation at the site of viral infection was severely impaired. Here again, chemokines were found to be the central mediators of CTL recruitment. CTLs had to express CXCR3, the receptor for CXCL9 and CXCL10, to gain access to virally infected vaginal tissue. Chemokine-mediated CTL recruitment, in turn, was dependent on IFN-γ production by Th cells. Since Th cell activation and IFN-γ production in the periphery is known to be mediated by infiltrating DCs (Wakim et al., 2008; Mclachlan et al., 2009), it is possible that, similar to CTL attraction in SLOs, DCs are the central hubs that initiate CTL recruitment to sites of infection.
XCR1 and XCL1
A further chemokine/chemokine receptor pair critically involved in cross-priming is XCR1–XCL1 (Dorner et al., 2009). However, in contrast to the CCR4 and CCR5 systems, this interaction does not appear to serve the attraction of naïve CTLs toward cross-priming DCs. Instead, in this interaction the cross-presenting DC expresses the receptor, not the ligand, suggesting that the information flow occurs in the opposite direction. Notably, XCR1 is exclusively expressed on cross-presenting CD8+ DCs in the spleen and lymph nodes (LNs), indicating a dedicated role in cross-priming. Its ligand was secreted mainly by cross-primed CTLs 12–48 h after initial antigen contact. How exactly XCR1 and XCL1 interact to enhance cross-priming is unclear. Under immunogenic conditions, XCL1-deficiency in T cells results in a lower CTL number and impaired in vivo cytotoxicity 12 days after the initial antigen encounter. Without an immunogenic stimulus, the XCL1–XCR1 axis does not seem to play a role, as XCL1-deficient CTLs behaved similar to the wildtype control, suggesting that XCR1 might not be critical for cross-tolerance.
The peak of XCL1-expression occurred when the interaction between CTL and DC was most stable, suggesting that XCL1 stabilizes the contact between CTL and DC (Dorner et al., 2009). It needs however to be formerly demonstrated whether XCL1-deficiency indeed shortens CTL–DC interactions and/or reduces cross-priming. Another possible mechanism is that XCL1 attracts additional CD8+ DCs to the site where the initial cross-presenting DC acquired antigen. These additional DCs might then take up and process the antigen as well, thereby increasing the overall antigen-presenting capacity.
The importance of the XCR1–XCL1 axis is underlined by its conservation between species. Like murine CD8+ DCs, human CD141+ XCR1+ DCs are able to cross-present antigen to CD8+ T cells and migrate in response to a XCL1 gradient (Bachem et al., 2010). Together with BDCA3, a recently identified marker for the human equivalent of CD8+ murine DCs (Poulin et al., 2010), XCR1 has been proposed as a marker to identify cross-presenting DCs.
The Signal 0 Hypothesis
From these studies, chemokine-mediated attraction of naïve CTLs has emerged as a novel mechanism that facilitates cross-priming, extending the list of signal 1 (antigen on MHC class I), 2 (costimulatory molecules), and 3 (cytokines) to a fourth signal. Since chemokines mediate CTL recruitment and consequently act before the other signals come into play, it has been proposed to refer to the chemokine trace as “signal 0” (Bousso and Albert, 2010).
Thus, chemokines do not only locate DCs and CTLs to the same compartment inside of SLOs, they also actively recruit CTLs to sites of DC licensing where mature, antigen-presenting DCs can readily prime naïve CTLs. After T cell entry into SLOs, CCR7 ligands enhance motility, and positioning in the T cell zone to restrict the area of potential DC/T cell encounters. Subsequently, ligands for CCR5 and CCR4 bias the migration of CTL toward Th cell- and NKT cell-licensed DCs, respectively. This directed migration highly enhances the efficiency of CTL responses, due to a more rapid activation and the attraction of higher numbers of CTLs.
Remarkably, when classical and alternative DC licensing acted simultaneously in a non-infectious immunization model, both chemokine systems synergistically enhanced CTL recruitment (Figure 3). Thus, CTL priming was most efficient when DCs received both Th cell- and NKT cell-mediated licensing (Semmling et al., 2010). Although it is unclear whether similar synergistic effects also exist at the level of signals 1–3, the concept that DC licensing might not be an “on”-or-“off”-phenomenon, but instead exists at several intermediate levels of licensing capacity, has important implications for vaccination strategies. The strength of cross-priming capability might hence be determined by the integration of all licensing signals that a particular DC has received.
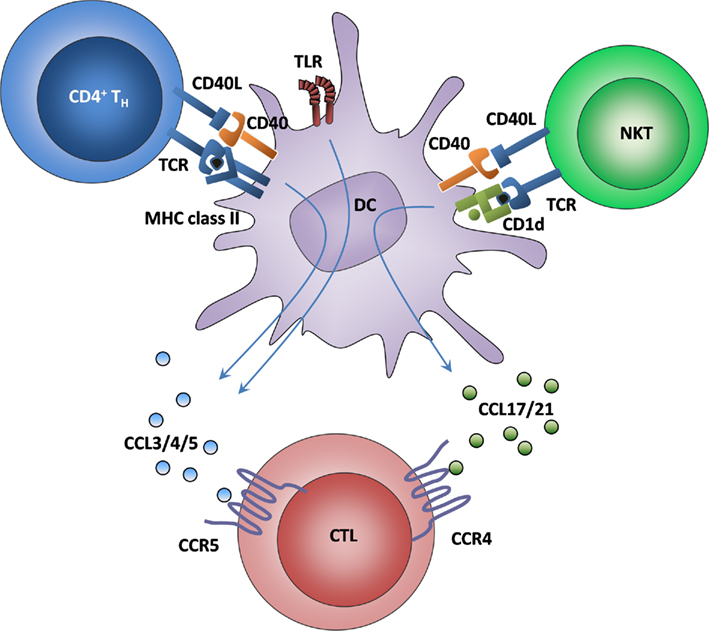
Figure 3. Synergy of classical and alternative licensing. It is known that classical licensing leads to CTL attraction via the secretion of ligands for CCR5. In contrast, CTL recruitment toward alternatively licensed DCs acts via ligands for CCR4. By taking advantage of both systems, DCs are rendered even more attractive to CTLs, which leads to an increased exploitation of the few antigen-specific CTLs from the precursor pool. How CCR4 and CCR5 are upregulated on naïve CTL is currently unknown.
Open Questions Regarding the Signal 0 Hypothesis
There are many unanswered questions on the regulation of signal 0 chemokines in CTL cross-priming. First, it is currently unknown which molecular signals induce chemokine expression in DCs. Both soluble factors and membrane-bound molecules are involved in the interactions between DCs and helper cells, but their precise role in signal 0 induction remains to be addressed. Second, the signals which render naïve CTL response to different chemokines remain elusive. It is well known that primed effector CTL upregulate the chemokine receptors CCR2, CCR5, and CXCR3, which allow them to enter infected tissues expressing several chemokines, including CCL2, CCL3, CCL4, CCL5, and CXCL10 (Bromley et al., 2008). However, since neither CCR5 nor CCR4 have been associated with circulating naïve CTL, it is conceivable that these receptors are upregulated around the time of entry into SLOs, depending on the inflammatory milieu. Then again, however, it is unclear which cells produce the factors that lead to the upregulation of chemokine receptors on CTL prior to antigen recognition. A recent study indicated that DC/Th cell interactions strongly enhance naïve CD8+ T cell input into LNs through vascular remodeling of the LN feed arteriole (Kumamoto et al., 2011). This effect required DC licensing though CD40 signaling. This finding suggests that signals derived from DC/Th cell interactions may act on endothelial cells of SLOs, thereby facilitating CTL entry and chemokine responsiveness.
Both CCR5 and CCR4 were found to be expressed only by subsets of naïve CTL (Castellino et al., 2006; Semmling et al., 2010). It is not yet clear whether this was simply due to limiting amounts or availability of the elusive factor(s) that lead to the upregulation of chemokine receptors on naïve CTL, but it is equally possible that several subsets of naïve CTL precursors exist which are capable of upregulating distinct chemokine receptors – potentially in response to early mediators that are released into the circulation and inform circulating CTL about the pathogenic profile of a local infection. It will be interesting to see whether further chemokine/chemokine receptor pairs are involved in the guidance of naïve CTL through their search for cognate antigen presented by an activated DC.
Implications of the Signal 0 Hypothesis on the Spatial and Temporal Orchestration of DC Licensing and Cross-Priming
The concept of chemokine-mediated CD8+ T cell attraction toward DCs for cross-priming opens a new view on T cell priming in SLOs. First, early imaging studies suggested that T cell migration in SLOs follows the pattern of random walk kinetics at a speed of about 10 μm/min (Miller et al., 2002). This picture was later refined by the discovery that T cells migrate along defined roadways consisting of a sophisticated FRC network and that also DCs are associated with this network (Bajenoff et al., 2006). Chemokines now appear to function as the road signs which lead naïve CTL in search for cognate peptide through the maze of FRCs toward activated DCs (Germain et al., 2008). Whether chemokines produced by DCs and helper lymphocytes are also physically attached to the FRC network is not entirely clear, but one can envision a scenario in which chemokines decorate the FRC network only in close proximity to a cross-priming “hot spot” consisting of a licensed DC and one or more helping lymphocytes. Once a naïve CTL reaches the chemokine trace on its random walk along the FRCs, its path may now deviate from the random movement, and the T cell is preferentially recruited to the site of antigen presentation.
Taken together, the involvement of chemokines in the regulation of cross-priming supports the idea that CTL movement in SLOs might not be random, but rather directed toward DCs that are cross-presenting antigen. Consequently, DCs that are presenting multiple relevant microbial antigens will receive multiple licensing signals, and might potentially co-produce several sets of chemokines. Guided by signal 0, naïve CTLs hence migrate preferentially toward areas where the likelihood of finding the remaining three signals for full activation and expansion is the highest.
Second, the notion that three cell types have to engage for effective CTL cross-priming bears the question how such an interaction might be spatially orchestrated. In the early 1980s, investigators first noticed the requirement for T cell help for the generation of an efficient CTL response (Keene and Forman, 1982). Considering the low frequency of T lymphocytes specific for antigens from a single infectious microorganism, they concluded that a mechanism was needed to bring these cells together both temporally and spatially. The most convincing idea was that this platform is provided by the DC, because this cell has to engage in physical contact with both lymphocytes. This notion was supported by the finding that indeed the same DC has to present antigen on MHC class II molecules to Th cells (or on CD1d to NKT cells, respectively) and on MHC class I molecules to CTLs (Ridge et al., 1998; Semmling et al., 2010). However, the temporal detail of this interaction, i.e., the question whether both lymphocytes have to engage the DC at the same time, has remained unclear.
The first model suggested simultaneous interaction of the DC with both helper cell and CTL (“three cell cluster” model) and was consistent with the hypothesis that optimal cross-priming requires a specific micromilieu of paracrine factors, such as IL-2, which are produced by APC-bound Th cells or NKT cells. The opposing model is based on the conception that an encounter of three rare immune cells (with the precursor frequency of naïve T cells specific for a particular antigen being as low as 1:1,000,000) is highly improbable. This gave rise to the idea that DCs become fully licensed by CD4+ Th cells through CD40 and its ligand, and that the licensing process is sufficient to confer to the DC a cell-intrinsic cross-priming ability without the need for further help (“temporal bridge” model). Thus, two serial cell encounters would suffice because DCs retain the information that they have received through cognate licensing (Smith et al., 2004). It was deduced from these results that in vivo, the helper cell might move randomly from DC to DC, scanning for cognate antigen and leaving behind each “qualified” DC in a licensed state. Thereby, the capacity of T cell help is amplified and produces many licensed DCs from a single Th cell.
Although no study has directly addressed whether CTLs are recruited to DCs while the helper lymphocyte is still engaged, the model that chemokines actively recruit naïve CTLs for cross-priming sheds new light on the discussion about which model best describes the cellular interactions involved in this process. If DCs that are still in contact with the helper lymphocyte (or even several helper lymphocytes) were more effective chemokine producers, then a tripartite encounter might be possible, although the low precursor frequency argues against this. Investigating the kinetics of chemokine expression by licensed DCs, as well as the role of helper lymphocytes for sustained chemokine secretion, will be critical to further elucidate the orchestration of cellular contacts involved in CTL cross-priming.
Third, it is known that chemokines influence CD8+ T cell priming not only by guiding CTLs to the site of antigen presentation, but also by modulating the interaction between T cells and DCs. Friedman et al. (2006) demonstrated that surface-bound CCL21 prolonged cell contacts during which T cells can scan the DC surface. This long-lasting contact was necessary to ensure the sustained signaling that maintains gene transcription and promotes T cell-cycle progression. The mechanistic events underlying the chemokine-dependent increase in contact duration have been partially characterized. It was suggested that chemokine contacts transiently tether CTLs to chemokine-presenting DCs in an LFA-1 dependent fashion (Friedman et al., 2006). It is well established that chemokine receptor activation can increase the affinity of LFA1 for ICAM1 (Campbell et al., 1998; Iezzi et al., 1998), which would augment the stability of cell–cell contacts. Besides integrin-mediated adhesiveness, Friedman et al. (2006) observed that CTLs acquired a polarized morphology and the TCR localized toward the contact site, whereby the hyperpolarized T cell gained enhanced sensitivity to antigen at the leading edge. This might be a mechanism that has a costimulatory effect on the induction of CTL responses and may also play a role in CCR5- and CCR4-mediated contact duration. This study did not find any influence of CCL3 and CCL17 on tethering of CTL, but this could be due to the fact that untreated CTL were used which express neither CCR5 nor CCR4. An additional mechanism that could increase contact duration is the recruitment of certain chemokine receptors to the immunological synapse (IS), a supramolecular structure that forms at the site of membrane contact between DCs and T cells. CCR4 and CCR5 belong to the so-called subordinate receptors that are recruited to the IS and subsequently become unresponsive to chemokine gradients (Bromley et al., 2000; Molon et al., 2005). Through this mechanism, more stable contacts between DC and CTL might be formed, thus permitting more extensive scanning of the DC surface.
Outlook
The recognition of virtually any antigenic structure through a vast TCR repertoire is one of the most important features of the adaptive immune system. However, this advantage comes at a cost, namely the challenge to quickly recruit rare antigen-specific precursors for priming and expansion. Chemokine-mediated attraction toward antigen-presenting DCs, albeit independent of antigen-specificity, might play an important role in facilitating this recruitment and can be exploited for the generation of effective vaccines.
The discovery of chemokine-mediated recruitment of naïve CTLs toward classically or alternatively licensed DCs has uncovered an additional functional role of chemokines in the orchestration of adaptive immune responses. We are just beginning to understand the many roles that chemokines play in CTL priming, and many questions remain. For example, it will be interesting to see whether and which chemokine signals act as signal 0 in CD4+ Th cell activation, and whether microbes have developed ways to evade the signal 0 pathway. Defining the signals that cause chemokine production by licensed DCs and render naïve CTLs responsive to these chemokines may reveal interesting insights into the complex regulation of cross-priming and open new avenues for optimizing vaccine strategies.
Conflict of Interest Statement
The authors declare that the research was conducted in the absence of any commercial or financial relationships that could be construed as a potential conflict of interest.
Acknowledgments
The authors apologize to all colleagues whose work could not be cited due to space restrictions. This work is supported by the Deutsche Forschungsgemeinschaft (consortia SFB704, SFB645, SFB TR57, KFO115, KFO228, and individual grants) and by the German National Academic Foundation (Studienstiftung des Deutschen Volkes).
References
Bachem, A., Guttler, S., Hartung, E., Ebstein, F., Schaefer, M., Tannert, A., Salama, A., Movassaghi, K., Opitz, C., Mages, H. W., Henn, V., Kloetzel, P. M., Gurka, S., and Kroczek, R. A. (2010). Superior antigen cross-presentation and XCR1 expression define human CD11c+CD141+ cells as homologues of mouse CD8+ dendritic cells. J. Exp. Med. 207, 1273–1281.
Bajenoff, M., Egen, J. G., Koo, L. Y., Laugier, J. P., Brau, F., Glaichenhaus, N., and Germain, R. N. (2006). Stromal cell networks regulate lymphocyte entry, migration, and territoriality in lymph nodes. Immunity 25, 989–1001.
Bedoui, S., Whitney, P. G., Waithman, J., Eidsmo, L., Wakim, L., Caminschi, I., Allan, R. S., Wojtasiak, M., Shortman, K., Carbone, F. R., Brooks, A., and Heath, W. R. (2009). Cross-presentation of viral and self antigens by skin-derived CD103+ dendritic cells. Nat. Immunol. 10, 488–495.
Belz, G. T., Smith, C. M., Kleinert, L., Reading, P., Brooks, A., Shortman, K., Carbone, F. R., and Heath, W. R. (2004). Distinct migrating and nonmigrating dendritic cell populations are involved in MHC class I-restricted antigen presentation after lung infection with virus. Proc. Natl. Acad. Sci. U.S.A. 101, 8670–8675.
Bennett, S. M. R., Carbone, F. R., Karamalis, F., Flavell, R. A., Miller, J. F., and Heath, W. R. (1998). Help for inducing cytotoxic T cell responses by cross-priming is mediated via CD40 signalling. Nature 393, 478–480.
Bevan, M. J. (1976). Cross-priming for a secondary cytotoxic response to minor H antigens with H-2 congenic cells which do not cross-react in the cytotoxic assay. J. Exp. Med. 143, 1283–1288.
Bousso, P., and Albert, M. L. (2010). Signal 0 for guided priming of CTLs: NKT cells do it too. Nat. Immunol. 11, 284–286.
Bretscher, P., and Cohn, M. (1970). A theory of self-nonself discrimination. Science 169, 1042–1049.
Bromley, S. K., Mempel, T. R., and Luster, A. D. (2008). Orchestrating the orchestrators: chemokines in control of T cell traffic. Nat. Immunol. 9, 970–980.
Bromley, S. K., Peterson, D. A., Gunn, M. D., and Dustin, M. L. (2000). Cutting edge: hierarchy of chemokine receptor and TCR signals regulating T cell migration and proliferation. J. Immunol. 165, 15–19.
Burgdorf, S., and Kurts, C. (2008). Endocytosis mechanisms and the cell biology of antigen presentation. Curr. Opin. Immunol. 20, 89–95.
Burgdorf, S., Scholz, C., Kautz, A., Tampe, R., and Kurts, C. (2008). Spatial and mechanistic separation of cross-presentation and endogenous antigen presentation. Nat. Immunol. 9, 558–566.
Caminschi, I., Proietto, A. I., Ahmet, F., Kitsoulis, S., Shin Teh, J., Lo, J. C., Rizzitelli, A., Wu, L., Vremec, D., Van Dommelen, S. L., Campbell, I. K., Maraskovsky, E., Braley, H., Davey, G. M., Mottram, P., Van De Velde, N., Jensen, K., Lew, A. M., Wright, M. D., Heath, W. R., Shortman, K., and Lahoud, M. H. (2008). The dendritic cell subtype-restricted C-type lectin Clec9A is a target for vaccine enhancement. Blood 112, 3264–3273.
Campbell, I. K., Van Nieuwenhuijze, A., Segura, E., O’donnell, K., Coghill, E., Hommel, M., Gerondakis, S., Villadangos, J. A., and Wicks, I. P. (2011). Differentiation of inflammatory dendritic cells is mediated by NF-kappaB1-dependent GM-CSF production in CD4 T cells. J. Immunol. 186, 5468–5477.
Campbell, J. J., Bowman, E. P., Murphy, K., Youngman, K. R., Siani, M. A., Thompson, D. A., Wu, L., Zlotnik, A., and Butcher, E. C. (1998). 6-C-kine (SLC), a lymphocyte adhesion-triggering chemokine expressed by high endothelium, is an agonist for the MIP-3beta receptor CCR7. J. Cell Biol. 141, 1053–1059.
Castellino, F., and Germain, R. N. (2006). Cooperation between CD4+ and CD8+ T cells: when, where, and how. Annu. Rev. Immunol. 24, 519–540.
Castellino, F., Huang, A. Y., Altan-Bonnet, G., Stoll, S., Scheinecker, C., and Germain, R. N. (2006). Chemokines enhance immunity by guiding naive CD8+ T cells to sites of CD4+ T cell-dendritic cell interaction. Nature 440, 890–895.
Crozat, K., Guiton, R., Contreras, V., Feuillet, V., Dutertre, C. A., Ventre, E., Vu Manh, T. P., Baranek, T., Storset, A. K., Marvel, J., Boudinot, P., Hosmalin, A., Schwartz-Cornil, I., and Dalod, M. (2010). The XC chemokine receptor 1 is a conserved selective marker of mammalian cells homologous to mouse CD8alpha+ dendritic cells. J. Exp. Med. 207, 1283–1292.
Curtsinger, J. M., Schmidt, C. S., Mondino, A., Lins, D. C., Kedl, R. M., Jenkins, M. K., and Mescher, M. F. (1999). Inflammatory cytokines provide a third signal for activation of naive CD4+ and CD8+ T cells. J. Immunol. 162, 3256–3262.
Del Rio, M. L., Rodriguez-Barbosa, J. I., Kremmer, E., and Forster, R. (2007). CD103- and CD103+ bronchial lymph node dendritic cells are specialized in presenting and cross-presenting innocuous antigen to CD4+ and CD8+ T cells. J. Immunol. 178, 6861–6866.
Den Haan, J. M., Lehar, S. M., and Bevan, M. J. (2000). CD8(+) but not CD8(-) dendritic cells cross-prime cytotoxic T cells in vivo. J. Exp. Med. 192, 1685–1696.
Dolan, B. P., Gibbs, K. D. Jr., and Ostrand-Rosenberg, S. (2006). Dendritic cells cross-dressed with peptide MHC class I complexes prime CD8+ T cells. J. Immunol. 177, 6018–6024.
Dorner, B. G., Dorner, M. B., Zhou, X., Opitz, C., Mora, A., Guttler, S., Hutloff, A., Mages, H. W., Ranke, K., Schaefer, M., Jack, R. S., Henn, V., and Kroczek, R. A. (2009). Selective expression of the chemokine receptor XCR1 on cross-presenting dendritic cells determines cooperation with CD8+ T cells. Immunity 31, 823–833.
Dudziak, D., Kamphorst, A. O., Heidkamp, G. F., Buchholz, V. R., Trumpfheller, C., Yamazaki, S., Cheong, C., Liu, K., Lee, H. W., Park, C. G., Steinman, R. M., and Nussenzweig, M. C. (2007). Differential antigen processing by dendritic cell subsets in vivo. Science 315, 107–111.
Forster, R., Davalos-Misslitz, A. C., and Rot, A. (2008). CCR7 and its ligands: balancing immunity and tolerance. Nat. Rev. Immunol. 8, 362–371.
Friedman, R. S., Jacobelli, J., and Krummel, M. F. (2006). Surface-bound chemokines capture and prime T cells for synapse formation. Nat. Immunol. 7, 1101–1108.
Fujii, S., Liu, K., Smith, C., Bonito, A. J., and Steinman, R. M. (2004). The linkage of innate to adaptive immunity via maturing dendritic cells in vivo requires CD40 ligation in addition to antigen presentation and CD80/86 costimulation. J. Exp. Med. 199, 1607–1618.
Fujii, S., Shimizu, K., Smith, C., Bonifaz, L., and Steinman, R. M. (2003). Activation of natural killer T cells by alpha-galactosylceramide rapidly induces the full maturation of dendritic cells in vivo and thereby acts as an adjuvant for combined CD4 and CD8 T cell immunity to a coadministered protein. J. Exp. Med. 198, 267–279.
Gallucci, S., Lolkema, M., and Matzinger, P. (1999). Natural adjuvants: endogenous activators of dendritic cells. Nat. Med. 5, 1249–1255.
Geijtenbeek, T. B., Van Vliet, S. J., Engering, A., T Hart, B. A., and Van Kooyk, Y. (2004). Self- and nonself-recognition by C-type lectins on dendritic cells. Annu. Rev. Immunol. 22, 33–54.
Germain, R. N., Bajenoff, M., Castellino, F., Chieppa, M., Egen, J. G., Huang, A. Y., Ishii, M., Koo, L. Y., and Qi, H. (2008). Making friends in out-of-the-way places: how cells of the immune system get together and how they conduct their business as revealed by intravital imaging. Immunol. Rev. 221, 163–181.
Grewal, I. S., and Flavell, R. A. (1998). CD40 and CD154 in cell-mediated immunity. Annu. Rev. Immunol. 16, 111–135.
Hamilton-Williams, E. E., Lang, A., Benke, D., Davey, G. M., Wiesmuller, K. H., and Kurts, C. (2005). Cutting edge: TLR ligands are not sufficient to break cross-tolerance to self-antigens. J. Immunol. 174, 1159–1163.
Hon, H., Oran, A., Brocker, T., and Jacob, J. (2005). B lymphocytes participate in cross-presentation of antigen following gene gun vaccination. J. Immunol. 174, 5233–5242.
Hugues, S., Scholer, A., Boissonnas, A., Nussbaum, A., Combadiere, C., Amigorena, S., and Fetler, L. (2007). Dynamic imaging of chemokine-dependent CD8+ T cell help for CD8+ T cell responses. Nat. Immunol. 8, 921–930.
Iezzi, G., Karjalainen, K., and Lanzavecchia, A. (1998). The duration of antigenic stimulation determines the fate of naive and effector T cells. Immunity 8, 89–95.
Iwasaki, A., and Medzhitov, R. (2010). Regulation of adaptive immunity by the innate immune system. Science 327, 291–295.
Jongbloed, S. L., Kassianos, A. J., Mcdonald, K. J., Clark, G. J., Ju, X., Angel, C. E., Chen, C. J., Dunbar, P. R., Wadley, R. B., Jeet, V., Vulink, A. J., Hart, D. N., and Radford, K. J. (2010). Human CD141+ (BDCA-3)+ dendritic cells (DCs) represent a unique myeloid DC subset that cross-presents necrotic cell antigens. J. Exp. Med. 207, 1247–1260.
Keene, J. A., and Forman, J. (1982). Helper activity is required for the in vivo generation of cytotoxic T lymphocytes. J. Exp. Med. 155, 768–782.
Keller, S. A., Schwarz, K., Manolova, V., Von Allmen, C. E., Kinzler, M. G., Bauer, M., Muntwiler, S., Saudan, P., and Bachmann, M. F. (2010). Innate signaling regulates cross-priming at the level of DC licensing and not antigen presentation. Eur. J. Immunol. 40, 103–112.
Kumamoto, Y., Mattei, L. M., Sellers, S., Payne, G. W., and Iwasaki, A. (2011). CD4+ T cells support cytotoxic T lymphocyte priming by controlling lymph node input. Proc. Natl. Acad. Sci. U.S.A. 108, 8749–8754.
Kurts, C., Kosaka, H., Carbone, F. R., Miller, J. F., and Heath, W. R. (1997). Class I-restricted cross-presentation of exogenous self-antigens leads to deletion of autoreactive CD8(+) T cells. J. Exp. Med. 186, 239–245.
Kurts, C., Robinson, B. W., and Knolle, P. A. (2010). Cross-priming in health and disease. Nat. Rev. Immunol. 10, 403–414.
Lauterbach, H., Bathke, B., Gilles, S., Traidl-Hoffmann, C., Luber, C. A., Fejer, G., Freudenberg, M. A., Davey, G. M., Vremec, D., Kallies, A., Wu, L., Shortman, K., Chaplin, P., Suter, M., O’keeffe, M., and Hochrein, H. (2010). Mouse CD8alpha+ DCs and human BDCA3+ DCs are major producers of IFN-lambda in response to poly IC. J. Exp. Med. 207, 2703–2717.
Limmer, A., Ohl, J., Kurts, C., Ljunggren, H. G., Reiss, Y., Groettrup, M., Momburg, F., Arnold, B., and Knolle, P. A. (2000). Efficient presentation of exogenous antigen by liver endothelial cells to CD8+ T cells results in antigen-specific T-cell tolerance. Nat. Med. 6, 1348–1354.
Loo, Y. M., and Gale, M. Jr. (2011). Immune signaling by RIG-I-like receptors. Immunity 34, 680–692.
Maurer, T., Heit, A., Hochrein, H., Ampenberger, F., O’keeffe, M., Bauer, S., Lipford, G. B., Vabulas, R. M., and Wagner, H. (2002). CpG-DNA aided cross-presentation of soluble antigens by dendritic cells. Eur. J. Immunol. 32, 2356–2364.
Mclachlan, J. B., Catron, D. M., Moon, J. J., and Jenkins, M. K. (2009). Dendritic cell antigen presentation drives simultaneous cytokine production by effector and regulatory T cells in inflamed skin. Immunity 30, 277–288.
Miller, M. J., Wei, S. H., Parker, I., and Cahalan, M. D. (2002). Two-photon imaging of lymphocyte motility and antigen response in intact lymph node. Science 296, 1869–1873.
Min, L., Mohammad Isa, S. A., Shuai, W., Piang, C. B., Nih, F. W., Kotaka, M., and Ruedl, C. (2010). Cutting edge: granulocyte-macrophage colony-stimulating factor is the major CD8+ T cell-derived licensing factor for dendritic cell activation. J. Immunol. 184, 4625–4629.
Molon, B., Gri, G., Bettella, M., Gomez-Mouton, C., Lanzavecchia, A., Martinez, A. C., Manes, S., and Viola, A. (2005). T cell costimulation by chemokine receptors. Nat. Immunol. 6, 465–471.
Nakanishi, Y., Lu, B., Gerard, C., and Iwasaki, A. (2009). CD8(+) T lymphocyte mobilization to virus-infected tissue requires CD4(+) T-cell help. Nature 462, 510–513.
Neijssen, J., Herberts, C., Drijfhout, J. W., Reits, E., Janssen, L., and Neefjes, J. (2005). Cross-presentation by intercellular peptide transfer through gap junctions. Nature 434, 83–88.
Poulin, L. F., Salio, M., Griessinger, E., Anjos-Afonso, F., Craciun, L., Chen, J. L., Keller, A. M., Joffre, O., Zelenay, S., Nye, E., Le Moine, A., Faure, F., Donckier, V., Sancho, D., Cerundolo, V., Bonnet, D., and Reis E Sousa, C. (2010). Characterization of human DNGR-1+ BDCA3+ leukocytes as putative equivalents of mouse CD8alpha+ dendritic cells. J. Exp. Med. 207, 1261–1271.
Qiu, F., and Cui, Z. (2007). CD4+ T helper cell response is required for memory in CD8+ T lymphocytes induced by a poly(I:C)-adjuvanted MHC I-restricted peptide epitope. J. Immunother. 30, 180–189.
Ramirez, M. C., and Sigal, L. J. (2002). Macrophages and dendritic cells use the cytosolic pathway to rapidly cross-present antigen from live, vaccinia-infected cells. J. Immunol. 169, 6733–6742.
Ridge, J. P., Di Rosa, F., and Matzinger, P. (1998). A conditioned dendritic cell can be a temporal bridge between a CD4+ T-helper and a T-killer cell. Nature 393, 474–478.
Rogers, N. C., Slack, E. C., Edwards, A. D., Nolte, M. A., Schulz, O., Schweighoffer, E., Williams, D. L., Gordon, S., Tybulewicz, V. L., Brown, G. D., and Reis E Sousa, C. (2005). Syk-dependent cytokine induction by Dectin-1 reveals a novel pattern recognition pathway for C type lectins. Immunity 22, 507–517.
Sancho, D., Joffre, O. P., Keller, A. M., Rogers, N. C., Martinez, D., Hernanz-Falcon, P., Rosewell, I., and Reis E Sousa, C. (2009). Identification of a dendritic cell receptor that couples sensing of necrosis to immunity. Nature 458, 899–903.
Schulz, O., Diebold, S. S., Chen, M., Naslund, T. I., Nolte, M. A., Alexopoulou, L., Azuma, Y. T., Flavell, R. A., Liljestrom, P., and Reis E Sousa, C. (2005). Toll-like receptor 3 promotes cross-priming to virus-infected cells. Nature 433, 887–892.
Schulz, O., Edwards, A. D., Schito, M., Aliberti, J., Manickasingham, S., Sher, A., and Reis E Sousa, C. (2000). CD40 triggering of heterodimeric IL-12 p70 production by dendritic cells in vivo requires a microbial priming signal. Immunity 13, 453–462.
Semmling, V., Lukacs-Kornek, V., Thaiss, C. A., Quast, T., Hochheiser, K., Panzer, U., Rossjohn, J., Perlmutter, P., Cao, J., Godfrey, D. I., Savage, P. B., Knolle, P. A., Kolanus, W., Forster, I., and Kurts, C. (2010). Alternative cross-priming through CCL17-CCR4-mediated attraction of CTLs toward NKT cell-licensed DCs. Nat. Immunol. 11, 313–320.
Smith, C. M., Wilson, N. S., Waithman, J., Villadangos, J. A., Carbone, F. R., Heath, W. R., and Belz, G. T. (2004). Cognate CD4(+) T cell licensing of dendritic cells in CD8(+) T cell immunity. Nat. Immunol. 5, 1143–1148.
Tvinnereim, A. R., Hamilton, S. E., and Harty, J. T. (2004). Neutrophil involvement in cross-priming CD8+ T cell responses to bacterial antigens. J. Immunol. 173, 1994–2002.
Wakim, L. M., and Bevan, M. J. (2011). Cross-dressed dendritic cells drive memory CD8+ T-cell activation after viral infection. Nature 471, 629–632.
Wakim, L. M., Waithman, J., Van Rooijen, N., Heath, W. R., and Carbone, F. R. (2008). Dendritic cell-induced memory T cell activation in nonlymphoid tissues. Science 319, 198–202.
Wingender, G., Rogers, P., Batzer, G., Lee, M. S., Bai, D., Pei, B., Khurana, A., Kronenberg, M., and Horner, A. A. (2011). Invariant NKT cells are required for airway inflammation induced by environmental antigens. J. Exp. Med. 208, 1151–1162.
Worbs, T., Mempel, T. R., Bolter, J., Von Andrian, U. H., and Forster, R. (2007). CCR7 ligands stimulate the intranodal motility of T lymphocytes in vivo. J. Exp. Med. 204, 489–495.
Zhan, Y., Carrington, E. M., Van Nieuwenhuijze, A., Bedoui, S., Seah, S., Xu, Y., Wang, N., Mintern, J. D., Villadangos, J. A., Wicks, I. P., and Lew, A. M. (2011). GM-CSF increases cross presentation and CD103 expression by mouse CD8(+) spleen dendritic cells. Eur. J. Immunol. doi: 10.1002/eji.201141540. [Epub ahead of print].
Keywords: chemokines, dendritic cells, T cell activation, NKT cells, costimulation, antigen presentation
Citation: Thaiss CA, Semmling V, Franken L, Wagner H and Kurts C (2011) Chemokines: a new dendritic cell signal for T cell activation. Front. Immun. 2:31. doi: 10.3389/fimmu.2011.00031
Received: 13 June 2011; Paper pending published: 03 July 2011;
Accepted: 20 July 2011; Published online: 01 August 2011.
Edited by:
Matthew Griffin, National University of Ireland, IrelandReviewed by:
Hubertus Hochrein, Bavarian Nordic GmbH, GermanyKen Shortman, The Walter and Eliza Hall Institute, Australia
Copyright: © 2011 Thaiss, Semmling, Franken, Wagner and Kurts. This is an open-access article subject to a non-exclusive license between the authors and Frontiers Media SA, which permits use, distribution and reproduction in other forums, provided the original authors and source are credited and other Frontiers conditions are complied with.
*Correspondence: Christian Kurts, Institutes of Molecular Medicine and Experimental Immunology, Rheinische Friedrich-Wilhelms-University, Sigmund-Freud-Str. 25, D-53105 Bonn, Germany. e-mail: ckurts@web.de