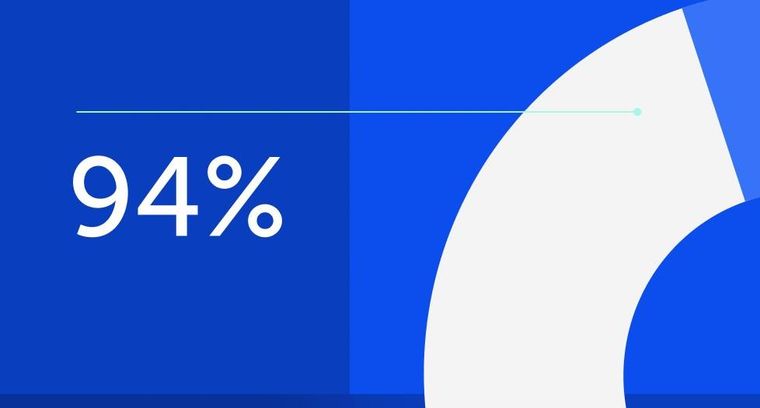
94% of researchers rate our articles as excellent or good
Learn more about the work of our research integrity team to safeguard the quality of each article we publish.
Find out more
MINI REVIEW article
Front. Immunol., 01 July 2011
Sec. Mucosal Immunity
volume 2 - 2011 | https://doi.org/10.3389/fimmu.2011.00025
While dendritic cells (DC) are central to the induction and regulation of adaptive immunity, these cells are very heterogenous and specific subsets can be characterized based on the expression of cell surface markers and functional properties. Intestinal CD103+ DCs are the subject of particular interest due to their role in regulating mucosal immunity. Since the epithelial surfaces are constantly exposed to a high antigenic load, tight regulation of innate and adaptive intestinal immune responses is vital as intestinal inflammation can have detrimental consequences for the host. Strategically positioned within the lamina propria, CD103+ DCs play an important role in maintaining intestinal immune homeostasis. These cells are required for the induction of tolerogenic immune responses and imprinting gut homing phenotypic changes on antigen-specific T cells. Recent insights into their development and regulatory properties have revealed additional immunoregulatory roles and further highlighted their importance for intestinal immunity. In this review we discuss the nature of the intestinal CD103+ DC population and the emerging roles of these cells in the regulation of mucosal immunity.
The epithelial surfaces of the body are constantly exposed to a wide variety of antigenic material, ranging from dietary proteins and commensals to pathogenic bacteria, viruses, and allergens. These antigens are separated from the delicate underlying body surfaces by a thin layer of cells, the epithelium. The cells and tissues comprising the mucosal immune system are located in the gastrointestinal tract (GIT), the upper and lower respiratory tracts and the urogenital tract. It also includes exocrine glands associated with these organs, such as the pancreas, the conjunctive and lacrimal glands of the eye, the salivary glands, and the lactating breast. The GIT and respiratory mucosa are the main portals of pathogen entry and therefore the generation of effective immune responses at these surfaces is vital to prevent infection. However, various regulatory mechanisms must also be in place to prevent damaging inflammatory reactions from occurring against benign antigens. There is increasing evidence that the dendritic cell (DC) populations located at these sites are vital in the maintenance of this immunological balancing act.
The intestinal mucosa is home to many cells of the mononuclear leukocyte family, including macrophages and DCs which are strategically positioned for antigen capture, and it is now well established that they play a vital role in orchestrating intestinal immune responses (Kelsall, 2008). DCs are potent antigen-presenting cells (APC) and are key modulators of the immune response, due to their ability to process and present antigens to T cells. Intestinal DCs are essential for the activation of inflammatory pathways and maintaining local immunological tolerance. Initially described by Steinman and Cohn (1973), an increasing number of DC subtypes have been identified and characterized.
Dendritic cells are enormously plastic in nature, owing to various microenvironmental adaptations and influences. Despite their heterogeneity, these cells share functional properties that distinguish them as a pivotal link between the innate and adaptive immune system (del Rio et al., 2010). DCs are located throughout the intestine, including the lamina propria (LP) of both the small and large intestine, peyers patches (PP), draining mesenteric lymphnodes (MLNs), and at isolated lymphoid follicles (Rescigno and Di Sabatino, 2009). Recent work by Merad and colleagues has revealed the presence of CD11chighMHC class IIhigh DCs within both the intestinal epithelial cell (IEC) fraction and LP, meanwhile these cells were absent from both the serosal and muscularis layers. These cells were further characterized into two distinct populations, CD11chighMHC class IIhighCD103+CD11b+ and CD11chighMHC class IIhighCD103−CD11b+ (Figure 1).
Figure 1. Characterization of CD103+ DC subsets in the small bowel. With permission (Bogunovic et al., 2009). Single cell suspensions were prepared from various anatomical locations found throughout the small bowel, these included the IEC compartment, lamina propria (LP), serosa with the intestinal muscularis, and PP. The populations were gated on DAPI negative, CD45 positive, MHC Class IIhigh and CD11chigh. The CD11chigh population was further characterized on the basis of CD103 and CD11b expression.
The majority of CD11chighMHCclassIIhigh DCs in the small intestinal LP (SI-LP) express the CD103 integrin and have distinct functional properties (del Rio et al., 2008). These cells are also found within the PPs (Jaensson et al., 2008), colonic LP and (MLN; Johansson-Lindbom et al., 2005). Immunofluorescence studies have revealed the presence of CD103+CD11c+ DCs in the LP and within the intraepithelial space of the apical villi, in contrast CD103−CD11b+ DCs accumulated mainly in the LP (Bogunovic et al., 2009).
The T-epithelial cell associated integrin CD103, also known as the αEβ7 integrin was discovered in the late 1980s as human mucosal lymphocyte antigen HML-1 (Cerf-Bensussan et al., 1987) and its functional role was described in 1994 (Cepek et al., 1994). Integrins are a large group of transmembrane αβ heterodimers that mediate cell to cell binding and cell to extracellular matrix interactions and are vital for T cell homing, cell signaling, adhesion and migration (Dustin et al., 1989; Springer, 1994, 1995). The CD103 integrin is expressed as a heterodimer with its β7 chain partner (Kilshaw and Murant, 1990).
In addition to its expression on mucosal CD11chighMHCIIhigh DCs, CD103 is also expressed on a subset of effector memory CD8+T cells in addition to subsets of CD4+ and CD8+ regulatory (Treg) T cells (Lehmann et al., 2002; Uss et al., 2006). The principal ligand for CD103 is E-cadherin, expression of which is confined to the basolateral surface of epithelial cells and is not detected on endothelial cells. CD103 has also been shown to be responsible for influencing cellular shape and motility in a ligand-dependent fashion (Schlickum et al., 2008).
Dendritic cells within the intestinal compartments have been extensively studied and much is now known regarding their phenotype. CD11c+ MHC class II+ DCs have been shown to be present in the LP and IEC fraction and were absent from the muscularis and serosa. The LP is home to two distinct DC subsets; CD11chighMHC class IIhighCD103+CD11b+CX3CR1−M-CSFlow (CD103+CD11b+) and CD11chighMHC class IIhighCD103− CD11b+CX3CR1+M-CSFhigh (CD103−CD11b+) DCs. Meanwhile the PPs are home to CD11chighMHC class IIhighCD103+ CD11b−CX3CR1− (CD103+CD11b−) DCs. CD11clowMHC class IIhighCD103−CD11b+ DCs are found throughout the muscular and serosal layers of the gut, with equivalent populations found in the large bowel (Bogunovic et al., 2009).
Granulocyte-macrophage–colony-stimulating factor (GM–CSF) and Fms-like tyrosine kinase 3 (Flt3) ligand are two important cytokines involved in DC development (Merad and Manz, 2009). In contrast, the development of macrophage populations in addition to epidermal Langerhans cells requires ligands for the macrophage colony stimulating factor receptor (M-CSFR; Ginhoux et al., 2006). Given the key role of M-CSFR ligands in the development of these populations, there may also be a role for these factors in the development of intestinal DCs. In fact, the development of CD103−CD11b+ LP DCs was dramatically affected following the selective deletion of M-CSFR (Csf1r−/−), whereas the CD103+CD11b+ population was unaffected. Flt3 is required for the development of both populations of LP–DCs, with a stronger requirement in the case of the CD103+CD11b+ cells. CD103+CD11b+ DCs also require GM–CSF for their development unlike the CD103−CD11b+ DC population.
Common DC precursor (CDP) and pre-DC do not contribute substantially to the CD103−CD11b+ LP–DC population under steady state conditions while monocytes actively differentiate into CD103−CD11b+ LP–DCs. However, Bogunovic et al. (2009) revealed that the GM–CSFR is dispensable for CD103− DC differentiation but is required for the differentiation of CD103+CD11b+ and CD103+CD11b− DCs (Bogunovic et al., 2009). Research also suggests that although the CD103+ population expresses CCR7 and actively migrate during steady state conditions, the CD103− DCs appear to be a resident non-migrating population in the MLN. In fact, only approximately 20% of the MLN CD103− DCs express CX3CR1, suggesting a different developmental origin than LP CD103− DCs, which express CX3CR1.
CD103+ DCs in the MLN have the unique ability to induce gut homing phenotypic changes (Annacker et al., 2005; Johansson-Lindbom et al., 2005). These cells are responsible for the upregulation of C-C chemokine receptor type 9 (CCR9) and α4β7 expression on CD8+T cells. These cell surface markers are essential for the active migration of cells to peripheral non-lymphoid tissues. Induction of CCR9 and α4β7 expression was initially observed following the co-culture of MLN CD103+ DCs from the MLN with T cells after oral antigen administration. In contrast, these cells failed to induce CCR9 and α4β7 expression on T cells following intraperitoneal (IP) antigen injection. Agace and colleagues transferred TCR transgenic ovalbumin (OVA) specific OT-I cells into recipient mice and the expression of CCR9 and α4β7 in the MLNs was examined 3 days following IP or oral antigen administration. CCR9 and α4β7 endowed the newly activated T cell with the capacity to migrate to the small intestine and exert effector functions (Annacker et al., 2005; Johansson-Lindbom et al., 2005).
The vitamin A metabolite, retinoic acid (RA), has been shown to be responsible for the induction of CCR9 and α4β7 expression on T cells (Iwata et al., 2004). RA conversion depends on retinaldehyde dehydrogenases (RALDH), which are expressed on MLN DCs as well as stromal cells. Despite expression of RALDH2 by both the CD103+ and CD103− MLN DCs, MLN and LP CD103+ DCs express much higher levels of the enzyme than their CD103− counterparts (Coombes et al., 2007). While CD103− DC in the MLN retain the capacity to prime CD4 and CD8 responses, these cells lacked the ability to induce gut homing signatures on naïve T cells (Annacker et al., 2005; Johansson-Lindbom et al., 2005) highlighting the unique role of the CD103+ DC subset.
A recent exciting development has linked bile retinoids to the imprinting of LP CD103+ DCs in the small intestine, which have the ability to generate gut homing T cells. Agace and colleagues demonstrated that the imprinting of CD103+ DCs is itself dependent on vitamin A and occurs locally within the small intestine, with high levels of retinol being detected within the bile (Jaensson-Gyllenback et al., 2011). The authors found that RA induced retinol-metabolizing activity in DCs both in vivo and in vitro. CD103+ DCs in the small intestinal LP constantly receive RA signaling in vivo at much higher levels than colonic CD103+ DCs. In a series of elegant experiments it was revealed that small intestinal CD103+ DCs remained imprinted in mice following the depletion of dietary but not systemic retinol (Jaensson-Gyllenback et al., 2011).
A number of regulatory mechanisms are employed by the immune system to maintain local immune homeostasis, prevent autoimmunity and control inflammation (Mills, 2004). Treg cells are regarded as the primary mediators of peripheral tolerance and are vital in the prevention of chronic inflammatory conditions.
It is now firmly established that there are two groups of Treg cells. These natural (or constitutive) and inducible (or adaptive) Treg cells are believed to have overlapping and complementary functions in the control of the host immune response. Natural Treg (nTreg) cells, which are characterized by the expression of CD4 and CD25, are functionally mature upon leaving the thymus. Initially described by Sakaguchi et al. (1995), these cells leave the thymus and mediate peripheral tolerance by suppressing self-reactive T cells (Bluestone and Abbas, 2003; Cozzo et al., 2003). The transcription factor FoxP3 is the most definitive marker for Treg cells in both humans and mice (Powrie et al., 1996; Shimizu et al., 2002; Fontenot et al., 2003).
In contrast to nTregs, induced Tregs (iTreg) cells are derived from mature CD4+T cells found in the periphery which develop into iTregs following antigen stimulation and/or co-stimulation from APCs. Furthermore, the development of these cells may also be influenced by the presence of exogenous cytokines. iTregs can be categorized into subsets including Tr1, Th3 and CD8+ subsets, which can be defined based on cytokine expression (Haynes et al., 2000; Garba et al., 2002). Unlike nTregs, iTregs are specific for antigens, which are not encountered within the thymus during the process of central tolerance. Due to their importance in maintaining immune homeostasis, inducible antigen-specific Tregs are now being targeted in clinical trials in order to treat a number of autoimmune diseases. In addition to their ability to induce gut homing, CD103+ DCs can induce the development of iTreg cells thereby mediating tolerance (Coombes et al., 2007).
The GIT is constantly exposed to a wide variety of antigenic material and maintenance of the local homeostasis in the gut stretches the discriminatory powers of the immune system to its limits (Wright, 2005). Although the vast majority of incoming antigens are harmless, some are highly immunogenic and have a propensity to induce inflammation, highlighting the importance of regulatory mechanisms in the gut. The GI mucosal immune system must carry out the difficult task of discriminating between the background antigenic noise and the much rarer signals transmitted by pathogens and their associated antigens. One strategy to prevent inflammation is the positioning of DCs in these compartments to capture and process antigens, and induce tolerance. Iliev et al. (2009) revealed that CD103+ DCs induce tolerance and gut homing, protecting against experimentally induced colitis in mice. CD103+ DCs exerting tolerogenic effects has been further suggested by the recent finding the CD11c+CD103+ DCs are decreased in the celiac lesion during celiac disease (Beitnes et al., 2011).
Mesenteric lymphnode CD103+ DCs mediate the conversion of naïve T cells to FoxP3-expressing T cells by producing TGF-β and RA (Coombes et al., 2007). When MLN DCs loaded with OVA were cultured in the absence of TGF-β, CD103+ DCs induced the development of antigen-specific iTreg cells. Furthermore, CD103− DCs failed to induce Treg development (del Rio et al., 2010), and it was suggested that “the special capacity of MLN-derived CD103+ DCs to drive iTreg cell differentiation may relate to the abundant expression of tissue plasminogen activator, TGFβ2, and latent TGF-β binding protein 3.” Both TGFβ2 and latent TGF-β binding protein 3 are required for the activation and active secretion of TGF-β. Upon addition of TGF-β, much higher levels of iTregs were induced by the CD103+ DC than the CD103- population.
In addition to TGF-β, RA produced by CD103+ DCs is an essential cofactor for increasing the rate of iTreg cell induction. In fact, preincubation of DCs with a synthetic RA inhibitor prevented the induction of iTreg cells (Coombes et al., 2007). iTreg cells induced via the co-culture of CD103+ and CD4+T cells were capable of directly suppressing the proliferation of CD4+T cells under in vitro culture conditions (Annacker et al., 2005).
Recent reports have also suggested a role for indoleamine 2,3-dioxygenase (IDO) in CD103+ DC-mediated iTreg cell induction. It was shown that CD103+ DCs but not CD103− DCs expressed IDO whose inhibition resulted in reduced CD4+FoxP3+ Treg cell conversion and enhanced T cell proliferation (Matteoli et al., 2010). Genetic deletion of IDO resulted in an increase in proinflammatory Th1 and Th17 cells and moreover IDO depletion results in the blockage of Treg cell induction and exacerbation of colitis in mice (Matteoli et al., 2010).
In contrast to the suppressive role of CD103+ DCs, CD103− DCs isolated from the MLN are associated with the production of proinflammatory cytokines. Upon stimulation with LPS or R848, CD103− DCs produced significant concentrations of TNF-α and IL-6 (Coombes et al., 2007). The CD103− population may be derived from a recruited blood–borne precursor and as a result do not undergo conditioning by the immune suppressive gut microenvironment. In fact it has been shown that IEC induce the expression of CD103 on the surface of DCs (Iliev et al., 2009) and thereby promote their development.
New evidence has also revealed that inflammation directly dampens the tolergenic ability of the MLN CD103+ DC population. This effect on the MLN CD103+ DC population was mediated by a down-regulation of the tgf β2 and aldh1a2 genes in the DC. MLN CD103+ DCs isolated from colitic mice had an impaired ability to induce FoxP3+ Treg cells; in contrast these cells had an enhanced ability to prime CD4+T IFN-γ producing T cells (Laffont et al., 2010). Laffont et al. (2010) also revealed that CD103+ cells from naïve mice and mice with induced colitis share the same developmental pathway. Furthermore, it has also been revealed that CD103 expression is lost from the surface of gut resident DCs during colitis (Strauch et al., 2010). These findings highlight the role of environmental influences in directing the tolerogenic properties of these cells.
Under normal physiological conditions, the intestinal immune system is tolerant to food antigens as well as to local commensal bacteria; break down of this tolerance can result in severe inflammatory reactions. It is therefore assumed that CD103− DCs are poised to respond to pathogenic insult, while CD103+ DCs are responsible for the induction of Treg cells and hence the maintenance of intestinal homeostasis.
It has been suggested that perhaps the resident gut-conditioned DCs favor the development of tolerogenic immune responses, whereas newly recruited inflammatory CD103− DCs may drive protective immunity.
The M cell provides a major route of antigen entry across the intestinal epithelium. M cells are specialized epithelial cells that commonly reside in the follicle-associated epithelium overlying the PPs (Azizi et al., 2010). M cells take up antigens by endo-, phago-, or pinocytosis and transcytosis (Gebert et al., 2000), before delivering them to the underlying immune cells.
In 2001, Rescigno et al. (2001) proposed an M cell-independent pathway for antigen uptake (Rescigno et al., 2001). Previous studies had revealed that Salmonella typhimurium, lacking genes encoded on the pathogenicity island 1 (SPI1) that are necessary for invasion, were observed within the spleen following oral administration (Galan and Curtiss, 1989). Rescigno et al. (2001) revealed the ability of resident intestinal DCs to open tight junctions between epithelial cells and extend their dendrites into the epithelium and directly sampling bacteria (Figure 2). Interestingly, because DCs express the tight junction proteins zonula, occludin and claudin I, the integrity of the epithelial barrier is preserved, preventing the development of overt inflammatory reactions to intestinal bacteria (Rescigno et al., 2001). Further work utilizing transgenic mice which express GFP under the control of CX3CR1, demonstrated that this chemokine receptor was vital for the development of transepithelial dendrites, enabling these cells to directly sample antigens from the lumen (Niess et al., 2005).
Figure 2. Mechanisms of antigen sampling by lamina propria DC populations under steady state conditions. DCs can capture antigenic material (including bacteria and other antigens) from the luminal compartment via direct transport through the M cell. CX3CR1+ cells can samples antigens directly by extending their dendrites into the luminal compartment. This is achieved due to the ability of the CX3CR1+ cell population to open the tight junctions between epithelial cells. CD103+ CX3CR1− DCs actively migrate to the mesenteric lymph nodes under steady state conditions; these cells are responsible for the induction of antigen-specific Treg cells. Moreover these cells are also responsible for the induction of gut homing phenotypic changes, such as up regulation of CCR9 and α4β7 on T cells. Antigens can also be captured by the underlying immune cells via breaches in epithelial integrity (not shown) and by direct transport via the fetal Fc receptor (not shown).
Regarding the CD103+ DC population, it now appears that these cells are distinct from the CX3CR1-expressing population within the LP. Despite the fact the CX3CR1 DC population outnumbers the CD103+ DCs, CD103+ DCs selectively localize close to the epithelium (Schulz et al., 2009). These cells also differ in their ability to respond to growth factors and have different turnover rates (Schulz et al., 2009), suggesting that they are derived from very distinct precursors. Schulz et al. (2009) elegantly revealed that in mice lacking CX3CR1, the LP CD103+ DC population are the main migratory population moving to the MLNs. Meanwhile the CX3CR1high LP cells may represent a non-migratory tissue resident population (Schulz et al., 2009). Evidence also suggests that the CD103+ DCs are much more potent at inducing intestinal T cell responses compared to the CX3CR1int and CX3CR1high populations, highlighting their importance. These populations also differed in their ability to generate RA. CX3CR1+ DCs expressed much lower levels of aldha1a2 and as a result were inefficient at inducing the upregulation of CCR9 on T cells. It has been postulated that perhaps CX3CR1+ LP DCs engulf luminal bacteria (Niess et al., 2005) and take up orally administered antigens (Schulz et al., 2009) before directly passing these antigens to CD103+ LP DCs, which migrate to the MLNs where they mediate tolergenic immune responses. However this has yet to be observed experimentally. Impairment of DC trafficking to the MLNs in mice lacking the CCR7 receptor results in defective induction of tolerance to oral antigens (Worbs et al., 2006), therefore establishing the importance of continual antigen transport to the MLNs in maintaining normal mucosal integrity. This was further highlighted by Varol et al. (2009) they determined that when mice display only CD103−CX3CR1+ DCs, they are more susceptible to dextran sodium sulfhate (DSS)-induced colitis (Varol et al., 2009).
Dendritic cells are essential modulators of the immune system as they maintain the balance between immunogenic and tolerogenic immune responses in the intestine. Dysregulation of DC function as a result of environmental cues or genetic mutation can result in severe intestinal inflammation. Despite extensive recent research into intestinal DCs, many questions remain such as: “are the progenitor cells intrinsically committed to becoming CD103+ or CD103− DCs?” and are these linage commitments dependent on local microenvironmental influences? The answer to the latter question appears to be yes. Bogunovic et al. (2009) have demonstrated the association of CD103+ DCs with epithelial cells and luminal bacteria may play a role in CX3CR1 phenotypic differentiation, as shown by the fact that bacteria-derived ATP is involved in the development of Th17-cell-inducing DCs (Atarashi et al., 2008). Therefore environmental conditioning may be essential for CD103+ and CD103− DC differentiation. The so called “specialized division of labor” (Helft et al., 2010) between the CD103+ and CD103− DC populations in the intestine has yet to be fully substantiated with many fascinating new aspects of biology of CD103+/CD103− DCs emerging. With the discovery that CD103+ DCs mediate gut homing and induce Treg cell development. CD103+ DCs may provide a novel therapeutic target to induce the expansion of Treg cells in the intestine. Targeted delivery of antigens responsible for celiac disease, IBD and other autoimmune diseases to intestinal CD103+ DCs may result in the expansion of antigen-specific Tregs that mediate tolerance. Despite many advances in DC biology, more in-depth insights into the intestinal CD103+ DC subpopulation are required before their therapeutic potential can be optimally determined.
The authors declare that the research was conducted in the absence of any commercial or financial relationships that could be construed as a potential conflict of interest.
We thank Saurabh Mehandru, Michelle L. Seth-Smith, Karen Misstear, and Lucas Brane for critical reading of the manuscript.
Annacker, O., Coombes, J. L., Malmstrom, V., Uhlig, H. H., Bourne, T., Johansson-Lindbom, B., Agace, W. W., Parker, C. M., and Powrie, F. (2005). Essential role for CD103 in the T cell-mediated regulation of experimental colitis. J. Exp. Med. 202, 1051–1061.
Atarashi, K., Nishimura, J., Shima, T., Umesaki, Y., Yamamoto, M., Onoue, M., Yagita, H., Ishii, N., Evans, R., Honda, K., and Takeda, K. (2008). ATP drives lamina propria T(H)17 cell differentiation. Nature 455, 808–812.
Azizi, A., Kumar, A., Diaz-Mitoma, F., and Mestecky, J. (2010). Enhancing oral vaccine potency by targeting intestinal M cells. PLoS Pathog. 6, e1001147. doi: 10.1371/journal.ppat.1001147
Beitnes, A. C., Raki, M., Lundin, K. E., Jahnsen, J., Sollid, L. M., and Jahnsen, F. L. (2011). Density of CD163(+) CD11c(+) dendritic cells increases and CD103(+) dendritic cells decreases in the coeliac lesion. Scand. J. Immunol. doi: 10.1111/j.1365-3083.2011.02549.x
Bluestone, J. A., and Abbas, A. K. (2003). Natural versus adaptive regulatory T cells. Nat. Rev. Immunol. 3, 253–257.
Bogunovic, M., Ginhoux, F., Helft, J., Shang, L., Hashimoto, D., Greter, M., Liu, K., Jakubzick, C., Ingersoll, M. A., Leboeuf, M., Stanley, E. R., Nussenzweig, M., Lira, S. A., Randolph, G. J., and Merad, M. (2009). Origin of the lamina propria dendritic cell network. Immunity 31, 513–525.
Cepek, K. L., Shaw, S. K., Parker, C. M., Russell, G. J., Morrow, J. S., Rimm, D. L., and Brenner, M. B. (1994). Adhesion between epithelial cells and T lymphocytes mediated by E-cadherin and the alpha E beta 7 integrin. Nature 372, 190–193.
Cerf-Bensussan, N., Jarry, A., Brousse, N., Lisowska-Grospierre, B., Guy-Grand, D., and Griscelli, C. (1987). A monoclonal antibody (HML-1) defining a novel membrane molecule present on human intestinal lymphocytes. Eur. J. Immunol. 17, 1279–1285.
Coombes, J. L., Siddiqui, K. R., Arancibia-Cárcamo, C. V., Hall, J., Sun, C. M., Belkaid, Y., and Powrie, F. (2007). A functionally specialized population of mucosal CD103+ DCs induces Foxp3+ regulatory T cells via a TGF-beta and retinoic acid-dependent mechanism. J. Exp. Med. 204, 1757–1764.
Cozzo, C., Larkin, J. III, and Caton, A. J. (2003). Cutting edge: self-peptides drive the peripheral expansion of CD4+CD25+ regulatory T cells. J. Immunol. 171, 5678–5682.
del Rio, M. L., Bernhardt, G., Rodriguez-Barbosa, J. I., and Förster, R. (2010). Development and functional specialization of CD103+ dendritic cells. Immunol. Rev. 234, 268–281.
del Rio, M. L., Rodriguez-Barbosa, J. I., Bölter, J., Ballmaier, M., Dittrich-Breiholz, O., Kracht, M., Jung, S., and Förster, R. (2008). CX3CR1+ c-kit+ bone marrow cells give rise to CD103+ and CD103- dendritic cells with distinct functional properties. J. Immunol. 181, 6178–6188.
Dustin, M. L., Garcia-Aguilar, J., Hibbs, M. L., Larson, R. S., Stacker, S. A., Staunton, D. E., Wardlaw, A. J., and Springer, T. A. (1989). Structure and regulation of the leukocyte adhesion receptor LFA-1 and its counterreceptors, ICAM-1 and ICAM-2. Cold Spring Harb. Symp. Quant. Biol. 54(Pt 2), 753–765.
Fontenot, J. D., Gavin, M. A., and Rudensky, A. Y. (2003). Foxp3 programs the development and function of CD4+CD25+ regulatory T cells. Nat. Immunol. 4, 330–336.
Galan, J. E., and Curtiss, R. III. (1989). Cloning and molecular characterization of genes whose products allow Salmonella typhimurium to penetrate tissue culture cells. Proc. Natl. Acad. Sci. U.S.A. 86, 6383–6387.
Garba, M. L., Pilcher, C. D., Bingham, A. L., Eron, J., and Frelinger, J. A. (2002). HIV antigens can induce TGF-beta(1)-producing immunoregulatory CD8+ T cells. J. Immunol. 168, 2247–2254.
Gebert, A., Goke, M., Rothkötter, H. J., and Dietrich, C. F. (2000). The mechanisms of antigen uptake in the small and large intestines: the roll of the M cells for the initiation of immune responses. Z. Gastroenterol. 38, 855–872.
Ginhoux, F., Tacke, F., Angeli, V., Bogunovic, M., Loubeau, M., Dai, X. M., Stanley, E. R., Randolph, G. J., and Merad, M. (2006). Langerhans cells arise from monocytes in vivo. Nat. Immunol. 7, 265–273.
Haynes, L. M., Vanderlugt, C. L., Dal Canto, M. C., Melvold, R. W., and Miller, S. D. (2000). CD8(+) T cells from Theiler’s virus-resistant BALB/cByJ mice downregulate pathogenic virus-specific CD4(+) T cells. J. Neuroimmunol. 106, 43–52.
Helft, J., Ginhoux, F., Bogunovic, M., and Merad, M. (2010). Origin and functional heterogeneity of non-lymphoid tissue dendritic cells in mice. Immunol. Rev. 234, 55–75.
Iliev, I. D., Mileti, E., Matteoli, G., Chieppa, M., and Rescigno, M. (2009). Intestinal epithelial cells promote colitis-protective regulatory T-cell differentiation through dendritic cell conditioning. Mucosal Immunol. 2, 340–350.
Iwata, M., Hirakiyama, A., Eshima, Y., Kagechika, H., Kato, C., and Song, S. Y. (2004). Retinoic acid imprints gut-homing specificity on T cells. Immunity 21, 527–538.
Jaensson, E., Uronen-Hansson, H., Pabst, O., Eksteen, B., Tian, J., Coombes, J. L., Berg, P. L., Davidsson, T., Powrie, F., Johansson-Lindbom, B., and Agace, W. W. (2008). Small intestinal CD103+ dendritic cells display unique functional properties that are conserved between mice and humans. J. Exp. Med. 205, 2139–2149.
Jaensson-Gyllenback, E., Kotarsky, K., Zapata, F., Persson, E. K., Gundersen, T. E., Blomhoff, R., and Agace, W. W. (2011). Bile retinoids imprint intestinal CD103(+) dendritic cells with the ability to generate gut-tropic T cells. Mucosal Immunol. 4, 438–447.
Johansson-Lindbom, B., Svensson, M., Pabst, O., Palmqvist, C., Marquez, G., Förster, R., and Agace, W. W. (2005). Functional specialization of gut CD103+ dendritic cells in the regulation of tissue-selective T cell homing. J. Exp. Med. 202, 1063–1073.
Kelsall, B. (2008). Recent progress in understanding the phenotype and function of intestinal dendritic cells and macrophages. Mucosal Immunol. 1, 460–469.
Kilshaw, P. J., and Murant, S. J. (1990). A new surface antigen on intraepithelial lymphocytes in the intestine. Eur. J. Immunol. 20, 2201–2207.
Laffont, S., Siddiqui, K. R., and Powrie, F. (2010). Intestinal inflammation abrogates the tolerogenic properties of MLN CD103+ dendritic cells. Eur. J. Immunol. 40, 1877–1883.
Lehmann, J., Huehn, J., de la Rosa, M., Maszyna, F., Kretschmer, U., Krenn, V., Brunner, M., Scheffold, A., and Hamann, A. (2002). Expression of the integrin alpha Ebeta 7 identifies unique subsets of CD25+ as well as CD25- regulatory T cells. Proc. Natl. Acad. Sci. U.S.A. 99, 13031–13036.
Matteoli, G., Mazzini, E., Iliev, I. D., Mileti, E., Fallarino, F., Puccetti, P., Chieppa, M., and Rescigno, M. (2010). Gut CD103+ dendritic cells express indoleamine 2,3-dioxygenase which influences T regulatory/T effector cell balance and oral tolerance induction. Gut 59, 595–604.
Mills, K. H. (2004). Regulatory T cells: friend or foe in immunity to infection? Nat. Rev. Immunol. 4, 841–855.
Niess, J. H., Brand, S., Gu, X., Landsman, L., Jung, S., McCormick, B. A., Vyas, J. M., Boes, M., Ploegh, H. L., Fox, J. G., Littman, D. R., and Reinecker, H. C. (2005). CX3CR1-mediated dendritic cell access to the intestinal lumen and bacterial clearance. Science 307, 254–258.
Powrie, F., Carlino, J., Leach, M. W., Mauze, S., and Coffman, R. L. (1996). A critical role for transforming growth factor-beta but not interleukin 4 in the suppression of T helper type 1-mediated colitis by CD45RB(low) CD4+ T cells. J. Exp. Med. 183, 2669–2674.
Rescigno, M., and Di Sabatino, A. (2009). Dendritic cells in intestinal homeostasis and disease. J. Clin. Invest. 119, 2441–2450.
Rescigno, M., Urbano, M., Valzasina, B., Francolini, M., Rotta, G., Bonasio, R., Granucci, F., Kraehenbuhl, J. P., and Ricciardi-Castagnoli, P. (2001). Dendritic cells express tight junction proteins and penetrate gut epithelial monolayers to sample bacteria. Nat. Immunol. 2, 361–367.
Sakaguchi, S., Sakaguchi, N., Asano, M., Itoh, M., and Toda, M. (1995). Immunologic self-tolerance maintained by activated T cells expressing IL-2 receptor alpha-chains (CD25). Breakdown of a single mechanism of self-tolerance causes various autoimmune diseases. J. Immunol. 155, 1151–1164.
Schlickum, S., Sennefelder, H., Friedrich, M., Harms, G., Lohse, M. J., Kilshaw, P., and Schön, M. P. (2008). Integrin alpha E(CD103)beta 7 influences cellular shape and motility in a ligand-dependent fashion. Blood 112, 619–625.
Schulz, O., Jaensson, E., Persson, E. K., Liu, X., Worbs, T., Agace, W. W., and Pabst, O. (2009). Intestinal CD103+, but not CX3CR1+, antigen sampling cells migrate in lymph and serve classical dendritic cell functions. J. Exp. Med. 206, 3101–3114.
Shimizu, J., Yamazaki, S., Takahashi, T., Ishida, Y., and Sakaguchi, S. (2002). Stimulation of CD25(+)CD4(+) regulatory T cells through GITR breaks immunological self-tolerance. Nat. Immunol. 3, 135–142.
Springer, T. A. (1994). Traffic signals for lymphocyte recirculation and leukocyte emigration: the multistep paradigm. Cell 76, 301–314.
Springer, T. A. (1995). Traffic signals on endothelium for lymphocyte recirculation and leukocyte emigration. Annu. Rev. Physiol. 57, 827–872.
Steinman, R. M., and Cohn, Z. A. (1973). Identification of a novel cell type in peripheral lymphoid organs of mice. I. Morphology, quantitation, tissue distribution. J. Exp. Med. 137, 1142–1162.
Strauch, U. G., Grunwald, N., Obermeier, F., Gürster, S., and Rath, H. C. (2010). Loss of CD103+ intestinal dendritic cells during colonic inflammation. World J. Gastroenterol. 16, 21–29.
Uss, E., Rowshani, A. T., Hooibrink, B., Lardy, N. M., van Lier, R. A., and Ten Berge, I. J. (2006). CD103 is a marker for alloantigen-induced regulatory CD8+ T cells. J. Immunol. 177, 2775–2783.
Varol, C., Vallon-Eberhard, A., Elinav, E., Aychek, T., Shapira, Y., Luche, H., Fehling, H. J., Hardt, W. D., Shakhar, G., and Jung, S. (2009). Intestinal lamina propria dendritic cell subsets have different origin and functions. Immunity 31, 502–512.
Worbs, T., Bode, U., Yan, S., Hoffmann, M. W., Hintzen, G., Bernhardt, G., Förster, R., and Pabst, O. (2006). Oral tolerance originates in the intestinal immune system and relies on antigen carriage by dendritic cells. J. Exp. Med. 203, 519–527.
Keywords: dendritic cells, CD103+ dendritic cells, intestine
Citation: Ruane DT and Lavelle EC (2011) The role of CD103+ dendritic cells in the intestinal mucosal immune system. Front. Immun. 2:25. doi: 10.3389/fimmu.2011.00025
Received: 18 April 2011;
Paper pending published: 17 May 2011;
Accepted: 16 June 2011;
Published online: 01 July 2011.
Edited by:
Yasmin Thanavala, Roswell Park Cancer Institute, USAReviewed by:
Ali M. Harandi, University of Gothenburg, SwedenCopyright: © 2011 Ruane and Lavelle. This is an open-access article subject to a non-exclusive license between the authors and Frontiers Media SA, which permits use, distribution and reproduction in other forums, provided the original authors and source are credited and other Frontiers conditions are complied with.
*Correspondence: Darren Thomas Ruane, Adjuvant Research Group, School of Biochemistry and Immunology, Trinity College Dublin, College Green, Dublin 2, Ireland. e-mail:cnVhbmVkdEB0Y2QuaWU=
Disclaimer: All claims expressed in this article are solely those of the authors and do not necessarily represent those of their affiliated organizations, or those of the publisher, the editors and the reviewers. Any product that may be evaluated in this article or claim that may be made by its manufacturer is not guaranteed or endorsed by the publisher.
Research integrity at Frontiers
Learn more about the work of our research integrity team to safeguard the quality of each article we publish.