- 1The American Alpine Club, Golden, CO, United States
- 2Department of Geography, The University of Calgary, Calgary, AB, Canada
- 3Institute for the Study of Earth, Oceans, and Space, The University of New Hampshire, Durham, NH, United States
Ice climbing is important to the culture and economies of mountain communities worldwide. However, warming winters call into question the future of livelihoods associated with ice climbing. In response, this case study presents observed and simulated ice climbing conditions in the Mount Washington Valley, New Hampshire, USA, as well as local climbing guide's experiences of and responses to these changes. First, variability in ice conditions were evaluated by classifying and summarizing ice characteristics depicted in a 20-year collection of conditions reports (n = 372) including photos and written observations for a benchmark ice climb (Standard Route). Next, climate model ensembles were used to simulate probable changes in future ice season lengths according to intermediate and high climate forcing scenarios (i.e., RCP 4.5 & RCP 8.5). Finally, a survey and focus group were conducted with Mount Washington Valley ice climbing guides to examine observations and lived experiences of warming winters. This study, which is the first formal assessment of the implications of warming winters for ice climbing, reveals significant effects of climate change for current and projected ice climbing conditions as well as marked, and often differentiated, vulnerability and adaptability to these changes amongst climbing guides. The unique mixed-methods approach used is applicable in other locales where climate change is impacting ice climbing activities and associated livelihoods.
1. Introduction
Globally, climate change is leading to fewer days below 0°C, reduced snow and ice cover, and winter precipitation shifting from snow to rain (IPCC, 2021), changes that are already impacting winter sports activities (Hayhoe et al., 2007; Ning and Bradley, 2015; Rawlins et al., 2016; Burakowski et al., 2022). For example, in the United States, ski areas are already experiencing fewer snowmaking days and 10–15% of ski resorts are expected to become operationally unviable by 2050 (Scott et al., 2020). Likewise, shrinking snow and ice cover and associated terrain changes including rockfalls (Gruber and Haeberli, 2009; Coe et al., 2018) are impacting mountaineering activities in the Alps by shifting the timing of good conditions and safe passage (Mourey et al., 2020, 2022a,b; Salim et al., 2021). One study on the Mont Blanc documented changes to 93 out of 95 popular climbing routes, with three disappearing altogether (Mourey et al., 2019). Despite the consequential findings of such research, scholarly work examining the impacts of warming winters for ice climbing activities has been absent to date.
Ice climbing is the practice of ascending frozen walls with specialized equipment providing traction. Typically, ice climbing activities take place on “alpine” ice, which forms from cumulative buildup of snow and frozen snowmelt, or on “waterfall” ice (the focus of this paper), which forms and disappears annually in wintertime across regions where sufficient water exists (Chouinard, 1978). The processes dictating waterfall ice formation, evolution, and collapse are primarily controlled by temperature, although local hydrology, the morphology of underlying terrain features, and existing ice structure are also important (Montagnat et al., 2010; Weiss et al., 2011; Gauthier et al., 2013, 2015). This temperature-dependent behavior suggests warming winters may significantly affect ice climbing activities into the foreseeable future, with potentially negative impacts for up to 160,000 individuals who participate in ice climbing activities in the United States annually (Outdoor Industry Association, 2022; Unreported survey data from American Alpine Club, 2022).
This study assesses the impacts of climate change on ice climbing routes and guides in the Mount Washington Valley, New Hampshire, USA, an area of notable historical and contemporary importance for ice climbing (Waterman et al., 2018). In this region, winters have warmed faster than summers (IPCC, 2014; USGCRP, 2017) and are projected to warm by 3°C (RCP 4.5) to 7°C (RCP 8.5) by 2100 (Campbell et al., 2010; Ning and Bradley, 2015; Karmalkar and Bradley, 2017; Burakowski et al., 2022). The summit of Mount Washington, the valley's namesake, has recorded high quality alpine weather observations since 1932, where monthly temperature trends point to warming consistent with the surrounding region (Murray et al., 2021).
This study represents the first formal assessment of the implications of warming winters for ice climbing activities and guides. We assess historical climbing and weather conditions, simulate future climbing season length using a suite of climate models, and assess the implications of different simulations for ice climbing guides based on results of a survey and focus group. The results of this mixed-methods approach are useful for adaptation planning in the Mount Washington Valley and other areas where ice climbing activities play an important role in local recreation cultures and economies.
2. Materials and methods
2.1. Study site
This study focuses on ice climbs and guiding activities in the northern New England region of the United States (Figure 1a; Vermont, New Hampshire, and Maine). Our study site, the Saco River Valley, colloquially known as the Mount Washington Valley, is in the White Mountain region of New Hampshire (Figure 1b), and has a humid continental climate with warm summers and cold winters according to the Köppen-Geiger climate classification (Beck et al., 2018). Mount Washington, which sits at the head of the valley, is the tallest mountain in the greater northeastern United States (1916 m a.s.l.) and is traditionally known as Agiocochook (“Place of the Great Spirit”) by the Abenaki peoples (Piotrowski, 2002). During the last Glacial Maximum and subsequent glacial retreat, the valley was scoured into a U-shaped “notch” with substantial bedrock exposure (Eusden et al., 2013). The combination of plentiful surface and groundwater, craggy landforms, and cold winter temperatures leads to an abundance of high quality and historically reliable seasonal waterfall ice flows. Consequently, the region is known nationally as a significant ice climbing destination. As summarized in an “Ice Climbers Guide to Northern New England” (Lewis and Wilcox, 2002, p. 15):
“Northern New England is one of the best places to ice climb in the world. The region's climate produces vast quantities of groundwater which leaks out each winter, flowing down our hillsides forming frozen fun-parks all over the place. From late November to mid-April, somewhere in Vermont, New Hampshire, or Maine, there is always something really cool frozen and waiting for us… water ice forms in New England each year regardless of snowfall and by mid-winter most areas are in good shape.”
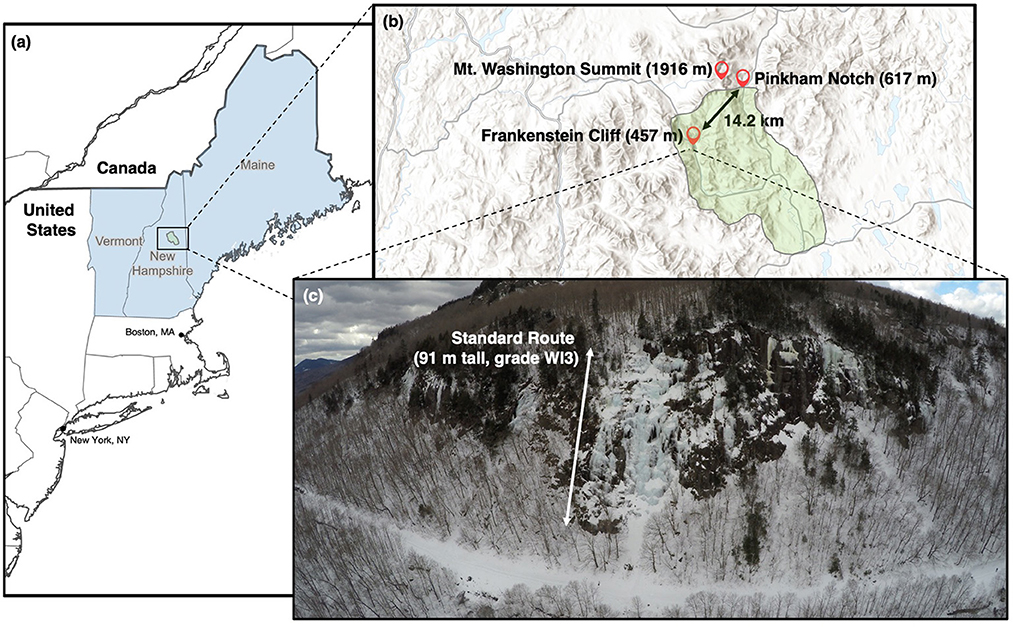
Figure 1. The geographic context of Standard Route within the United States. (a) Northern New England consists of the states Maine, New Hampshire, and Vermont, which border Canada. The approximate outline of the upper Mount Washington Valley is shaded. (b) The Mount Washington Valley hosts the highest mountains in the Eastern United States. Mt. Washington, Pinkham Notch, and Frankenstein cliff are all within 15 km of each other. (c) An aerial view of the ice climb Standard Route, the largest climb at Frankenstein cliff (photo by Ross Henry). The climb is rated WI3, which indicates a sustained 70° angle, with short bulges and curtains approaching 90°.
In the early 1970s, modern ice climbing tools and techniques were introduced in the Mount Washington Valley (Waterman et al., 2018); today the region continues to be a hotbed for ice climbing activities, supporting a vibrant winter climbing community with at least 19 guiding companies (Personal communication with Mount Washington Valley Ice Festival coordinator, 2022).
One of the region's most the popular and frequently guided ice climbing areas, Frankenstein Cliff (457 m), boasts at least 55 ice and mixed climbing routes (mountainproject.com, n.d.). The best-known climb at Frankenstein Cliff is Standard Route, a broad, 91 m tall flow graded WI3, which indicates a sustained 70° angle, with short bulges approaching 90° (Figure 1c). According to the guidebook, Standard Route is the “most popular moderate climb at Frankenstein and likely in all of New Hampshire… It comes in early, stays late and is often crowded” (Lewis and Wilcox, 2002, p. 192). Area guides estimate 500 to 4000 individuals climb Standard Route annually depending on season length, ice quality, and outdoor participation (Personal communication between A. Hospers and M. Bowman, 2023). Given its importance to local ice climbing guides, we use this route as a reference for analyses in this study.
2.2. Methodology
Methodologies coupling natural and social scientific approaches are increasingly recognized as being foundational to the study of climate change vulnerability and adaptation (Turner et al., 2003; Adger, 2006; Smit and Wandel, 2006), including in mountain regions (Carey et al., 2017; McDowell and Koppes, 2017; McDowell et al., 2019). Accordingly, this study employed a mixed-methods approach to characterize the physical and human dimensions of changing ice conditions in the Mount Washington Valley, focusing specifically on archival analysis, climate modeling, and surveys and focus groups with local mountain guides.
Using Standard Route as our benchmark, this approach enabled us to reveal how ice conditions across seasons have changed and are likely to change in the future; how mountain guides observe, experience, and respond to such changes; and how winters in the Mount Washington Valley are likely to evolve into the future, including with what potential consequence for the local guiding community.
2.2.1. Archive and climatological record analysis
As part of a Mount Washington Valley ice climbing conditions report started in 2001, Standard Route has been photographed by a local mountain guide from a consistent vantage point on a weekly basis every winter season (available at NEClimbs.com, n.d.). Many of these photographs, which document evolving ice conditions over time, are supplemented by narrative reports of ice characteristics (n = 328 photographs, 44 written records). On average, 19 ± 4 conditions observations were captured during each season for a total of twenty seasons.
We established ice climbing season lengths from this archive by analyzing visible ice conditions in the photographs (Figure 2) and accompanying narrative reports. This assessment was undertaken by the lead author of this study, who was an ice climbing guide in the region from 2015 to 2017 and is familiar with Standard Route. Each photo was assigned an ordinal rating of 0–2, with “0” representing unclimbable conditions, “1” representing marginally climbable conditions, and “2” representing climbable conditions. “Climbability” is a subjective condition based on the presence of ice as well as the skill and risk tolerance of climbers. Unclimbable conditions are those in which there is no ice present or there are sections of the climb which have little to no ice present, and it is generally dangerous to climb regardless of climber skill level. Marginal conditions are those in which the ice may be climbable, but it is potentially unsafe (e.g., thin, detached) and may require a higher degree of skill or risk tolerance to climb. In marginal conditions the route may become unclimbable with melting temperatures (T > 0°C) or climbable with cold (T < 0°C). Climbable conditions are those in which there is sufficient ice present for safe passage (e.g., thick, bonded to the rock below). In climbable conditions, ice is likely to persist even with mild warming. For any weeks with missing photos, ratings were assigned based on written ice condition reports available at (NEClimbs.com, n.d.) If the last marginal or climbable record of a season was not followed by an explicit record detailing ice condition, the previous condition was assumed to persist for 1 week before the season ended with an unclimbable condition. Examples of these condition ratings are shown in Figure 2.
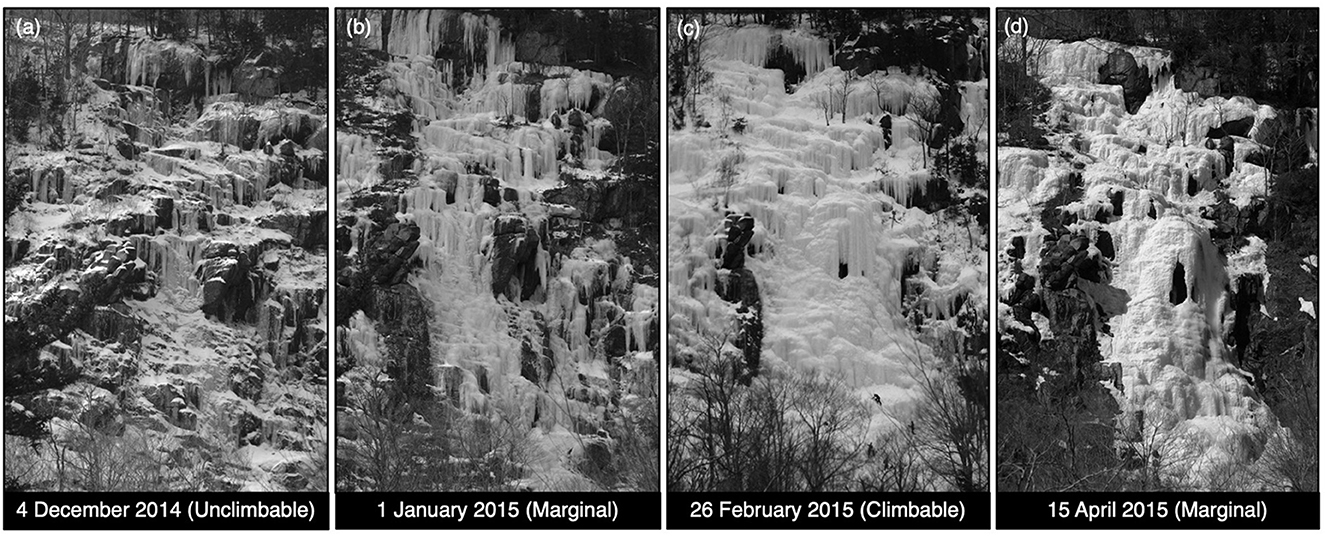
Figure 2. A typical pattern of ice growth and decay through a season, with assigned ratings in parentheses (photos by Al Hospers). (a) Ice begins building as temperatures drop in the early season. This was rated “Unclimbable” because the ice is thin with visible gaps, and the top of the climb is discontinuous. (b) Groundwater and thaw-freeze of snow provides water for climbs to continue building. As it runs over ice, channels form creating preferential flow paths. This was rated “Marginal” because while it is climbable, the volume of ice would likely be unclimbable after a week of warm temperatures. (c) Combined cold and water cause the ice to grow to substantial volumes by mid-season. Climber visible in bottom right of photograph. This was rated “Climbable” because the ice is thick and well filled-in. (d) As temperatures warm above freezing and solar radiation increases during the spring, ice climbs melt. This was rated “Marginal” because the ice, while thick enough to protect, shows substantial signs of decay. The potential for ice fall and collapse is higher during the spring, and climbers may face increased hazards if choosing to climb during this time. The decision to rate this “Marginal” vs. “Unclimbable” is an example of the subjective and experientially informed risk-based decision-making ice climbers engage with.
After each photo was assigned a rating, annual season length for each winter was determined using Equation 1 by calculating the time interval in days between the first photo and the next, multiplying the length of the interval (tn) by the condition variable (cn, ie: 0 to 2) for each interval, summing the results over each season, then multiplying the total by . This approach implies that the entire interval following a climbable condition and one-half of each interval following a marginal condition counted toward season length.
Ice forms when water drops below its freezing point (0°C), therefore, the potential for ice growth is indicated by sustained, below-zero temperatures (Montagnat et al., 2010; Gauthier et al., 2013, 2015). The simplest parameter indicating sustained cold is simply the total number of days below zero (Days0C) in the winter season, which we define as November 1st through April 30th in this study, consistent with Lewis and Wilcox (2002). Another parameter, accumulated freezing degree days (AFDD), is a measure of cold intensity which is commonly used to estimate the thickness of river and lake ice (Ashton, 1986), and which provides a secondary measure of conditions amenable to ice formation and persistence.
Based on these principles, we analyzed both Days0C and AFDD over each winter season based on historical temperature averages calculated from daily minimum and maximum temperatures observed at the Pinkham Notch weather station (GHCNd: USC00276818), which is located 14.2 km northeast of Standard Route at a similar elevation (617 m). Estimated season length was assessed vs. Days0C and AFDD using a linear regression analysis, where Days0C and AFDD were independent variables and estimated season length was the dependent variable.
2.2.2. Climate modeling
Global climate models (GCMs) simulate maximum and minimum temperatures across pixels with resolutions >100 km, which are too coarse to determine local-scale impacts and particularly topographic differences within pixels. Therefore, we used the Localized Constructed Analogs (LOCA) downscaled climate projections, which simulated daily minimum and maximum temperature for RCP 4.5 and RCP 8.5 over a much finer resolution (6 km) across 32 independent models in the Coupled Model Intercomparison Project Phase 5 (CMIP5) (Pierce et al., 2014). The LOCA projections were used as the basis for the United States Global Change Research Program Fourth National Climate Assessment (NCA4) Climate Science Special Report (USGCRP, 2017). The pathways selected are an intermediate (RCP 4.5) scenario consistent with significant greenhouse gas reductions and a very high (RCP 8.5) scenario which is considered to be a “worst-case” (IPCC, 2014). We selected the dataset associated with the grid cell collocated with Pinkham Notch, consistent with the location of the Pinkham Notch weather station. We assume that this grid cell is representative of projections for Standard Route, which we believe is reasonable given the similar elevation, location, and meteorological conditions at these sites.
Using the LOCA projections for Pinkham Notch, we calculated simulated average daily temperatures from simulated daily minimum and maximum temperature. We then calculated Days0C and AFDD for each winter season from 1981 through 2099 within each model, and computed summary statistics by season across the 29-model ensemble. We completed this post-processing using Python.
2.2.3. Survey and focus group
To complement and extend insights from photo archival and climate modeling work, we conducted an online survey and a focus group with local mountain guides in the Mount Washington Valley (University of New Hampshire IRB-FY2021-40). All social scientific research was conducted virtually due to the COVID pandemic.
A survey composed of both closed choice and open-ended response options (13 questions total; see Supplementary material) was conducted online (Qualtrics, Provo, UT) to investigate local mountain guides' experiences of changing winter conditions. Survey questions covered three broad thematic areas: Guide's biographical data and level of experience, the effects of changing winter conditions on guide's ability to deliver positive experience to guests; and guide's level of concern about current and future climate-related changes [this latter group of questions was informed by the Six Americas Super Short Survey (Chryst et al., 2018)]. Survey participation was limited to a list of guides that had been invited to participate in the 2021 Mountain Washington Valley Ice Festival (mwv-icefest.com, n.d.), an invitation reserved for accomplished guides who are knowledgeable about local ice climbing areas and conditions. These experienced guides were then contacted by the study team by email where they were invited to complete the study's online survey. In total, 20 guides completed the survey between November and December 2021, with respondents being heavily skewed toward male-identifying individuals (90%). The response bias is consistent with current gender disparities in mountain guiding work in the United States (AMGA, 2013). Survey summary statistics were computed using Microsoft Excel.
Following the survey, a focus group was conducted virtually on Zoom (Zoom Video Communications, San Jose, CA) with guides to elicit their observations of change, examine their vulnerability and adaptation to change, and understand their outlook for the future. Participants were recruited from a cohort which completed the initial survey. A subset of these individuals was selected by the study team, with an aim of including guides with a range of perspectives and career experience. To enable thoughtful conversation among participants and the study team, the number of focus group participants was limited to five individuals, including three male guides and two female guides. The focus group was conducted in February 2022. The event was video recorded and a transcription was made to support manifest (explicitly stated) and latent (implicit) content analysis (Payne and Payne, 2004; Bengtsson, 2016).
3. Results and discussion
3.1. Archive and climatological record analysis
Analysis of the photo archive for Standard Route yielded season lengths for 20 winters from 2001 through 2021. The complete analysis of seasons is shown in Figure 3. The record shows ice was typically climbable from early January through mid-March, with a greater occurrence of marginal conditions in January and March. February tends to have most consistently climbable ice conditions, whereas December and April only tend to have a few days of climbable conditions. The average estimated season length over the 20-year reference period was 99 ± 4 days.
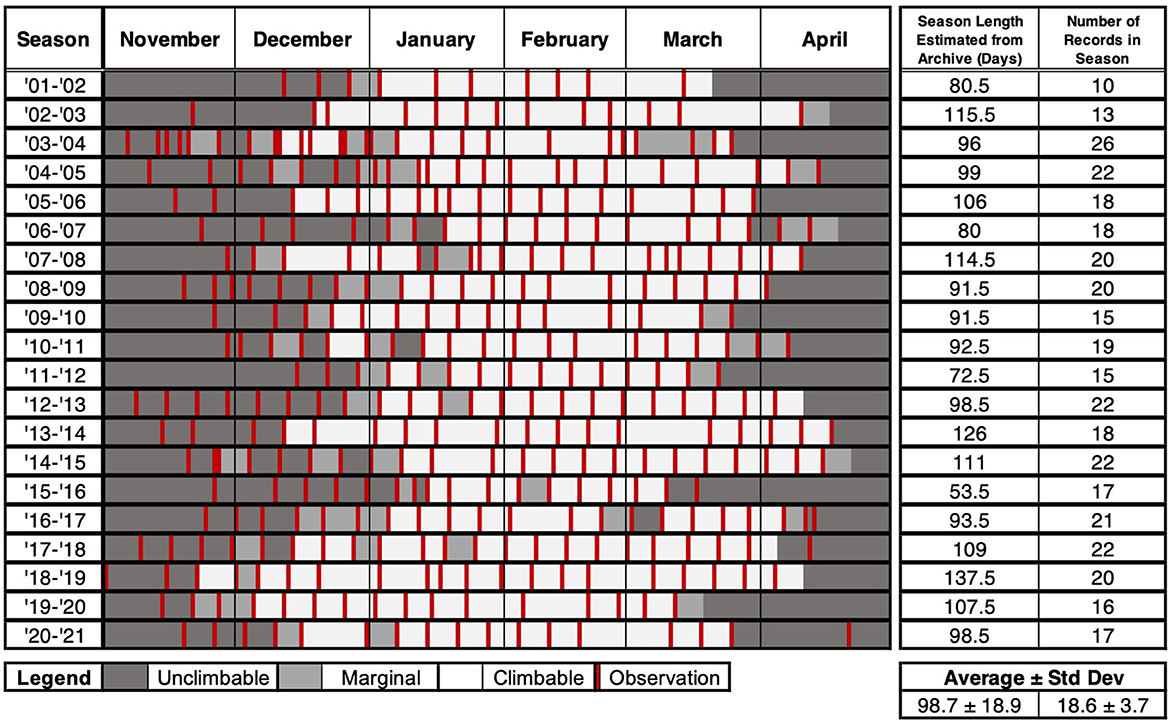
Figure 3. The map of quality across the 20-year archive record shows season length and quality vary annually. While each year did not have a consistent number of records, in most years roughly weekly reporting from December through late March captured ice quality observations through the season. The archive captures some novel behavior in recent years. While January thaws occurred through the period of record, significant February thaws only occurred in 2015/2016 and 2016/2017.
Figure 3 has no obvious year-on-year trend of shorter seasons. However, when we compared Days0C and AFDD observed at Pinkham Notch with the estimated season length for Standard Route, we see a clear relationship. Estimated season length was positively correlated with Days0C (R2 = 0.72) and AFDD (R2 = 0.49); that is, the greater the number of Days0C and the larger the AFDD, the greater the estimated season length.
Figure 4 shows the linear regression analysis of Days0C and estimated season length. Days0C was always less than estimated season length. This discrepancy likely a lag time required for ice to build to a climbable state (i.e., starting from no ice, multiple days of freezing temperatures are required to yield enough ice for climbing), an observation which is consistent with previous works indicating from several days and up to one month of subfreezing temperatures are required for ice to build to a climbable state (Montagnat et al., 2010; Gauthier et al., 2013). While relying on Days0Calone omits other factors pertinent to ice formation and persistence (e.g. precipitation regimes, groundwater dynamics, albedo, etc.), variations in winter precipitation and groundwater availability are historically not limiting factors for ice formation on Standard Route based on the archival photographs. Furthermore, winter precipitation is projected to increase under future climate scenarios (Burakowski et al., 2022). Thus, we interpret Days0C to be a simple and adequate predictor of season length for Standard Route.
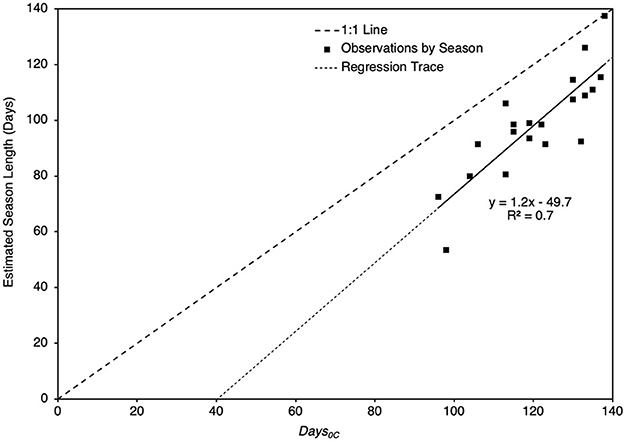
Figure 4. The comparison of Days0C and estimated season length. Days0C is always lower than the 1:1 line, suggesting the parameter is a “ceiling” variable for the presence of ice climbs.
3.2. Climate modeling
Across the ensemble of downscaled climate models, we simulated Days0C (Figure 5A) and AFDD (shown in Figure 1 of Supporting Information) from 1981 through 2099 under RCP 4.5 and 8.5. The results of RCP 4.5 and RCP 8.5 diverge around 2040 for both Days0C and AFDD.
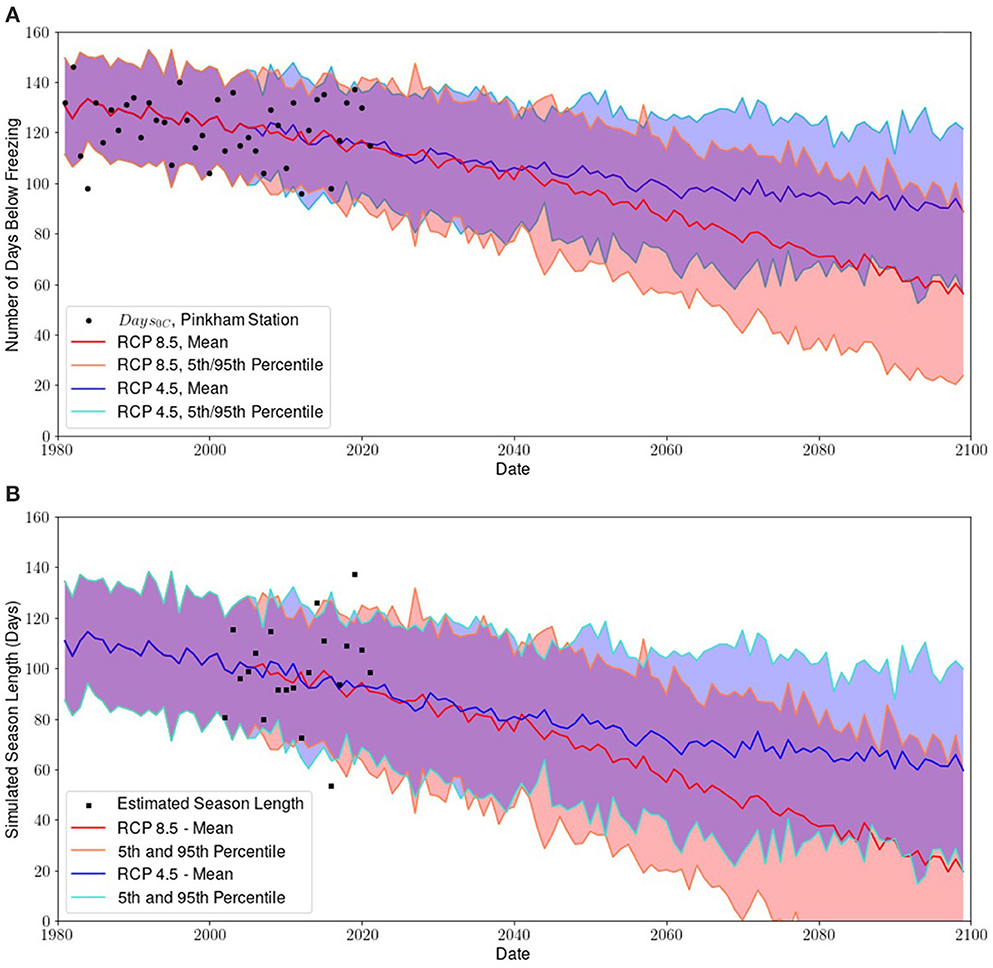
Figure 5. The results of climate modeling. (A) Simulation of Days0C at Pinkham Station under RCPs 4.5 and 8.5, with historical Days0C superimposed. (B) Simulation of season length at Pinkham Station under RCPs 4.5 and 8.5, with historical season length estimates superimposed. Simulation was conducted by transforming Days0C using the linear regression relationship between Days0C and estimated season length outlined in Figure 4. Significant declines in average season length are expected under both scenarios.
We used the linear regression relationship (Figure 4) and simulation of Days0C in the model scenarios (Figure 5A) to estimate future season length (Figure 5B). A summary of the season length estimates under different climate scenarios and periods are shown in Table 1. Under RCP 8.5, average season lengths are expected to decline from 97 days (2001 to 2021 reference period), to 70 days (72% of today's season) by midcentury (2041 to 2061) and 30 days (31% of today's season) by the end of the century (2079 to 2099). Under RCP 4.5, average season lengths are expected to decline to 76 days (78% of today's season) by midcentury and 64 days (66% of today's season) by the end of the century.
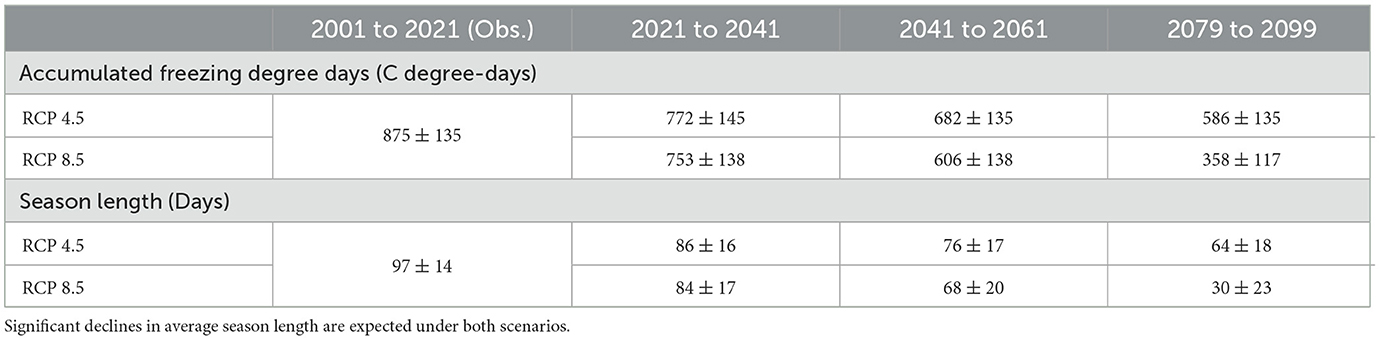
Table 1. Results of simulations for AFDD and Days0C transformed to season length at Pinkham Station under RCPs 4.5 and 8.5.
While Days0C seems to be a good estimate of season length, it is not necessarily a determinant of ice quality. For example, the type and thickness of ice produced during a season that has many days just below freezing will be very different from ice quality in a winter with an equivalent number of very cold days.
To discern the range of effects of warmer winters on ice quality we must turn to analogs based on AFDD. Using the simulated AFDD averages for RCP 4.5 and RCP 8.5 (from 2079 to 2099), we identified periods within the archive with a similar AFDD, which we assume represent analogs for ice growth. In other words, AFDD of a similar magnitude in the future will generate ice volumes consistent with periods of AFDD we have observed in the past. In determining which previous AFDD observations to use, we excluded any periods with temperatures warmer than freezing. We consider three scenarios, though we note there are many potential variations on these.
• Scenario 1—multiple cold periods interspersed by warming events with ice collapse: An AFDD of 105 C degree-days occurred in December of the 2011/2012 season (Figure 6a) and Standard Route was rated ‘marginal' in this condition. If future seasons yield multiple cold periods with AFDD of ~100 C degree-days, interspersed by melting periods with total ice collapse, Standard Route may exist in marginal conditions periodically through the season.
• Scenario 2—one extended cold period under an average season under RCP 8.5: An AFDD of 335 C degree-days occurred in February of the 2015/2016 ice season (Figure 6b). This condition represents the maximum probable ice volume in an average season under RCP 8.5 if cold weather comes during one extended period.
• Scenario 3—one extended cold period under an average season under RCP 4.5: An AFDD of 553 C degree-days was observed during February of the 2009/2010 season (Figure 6c). This condition represents the maximum probable ice volume in an average season under RCP 4.5 if cold weather comes during one extended period.
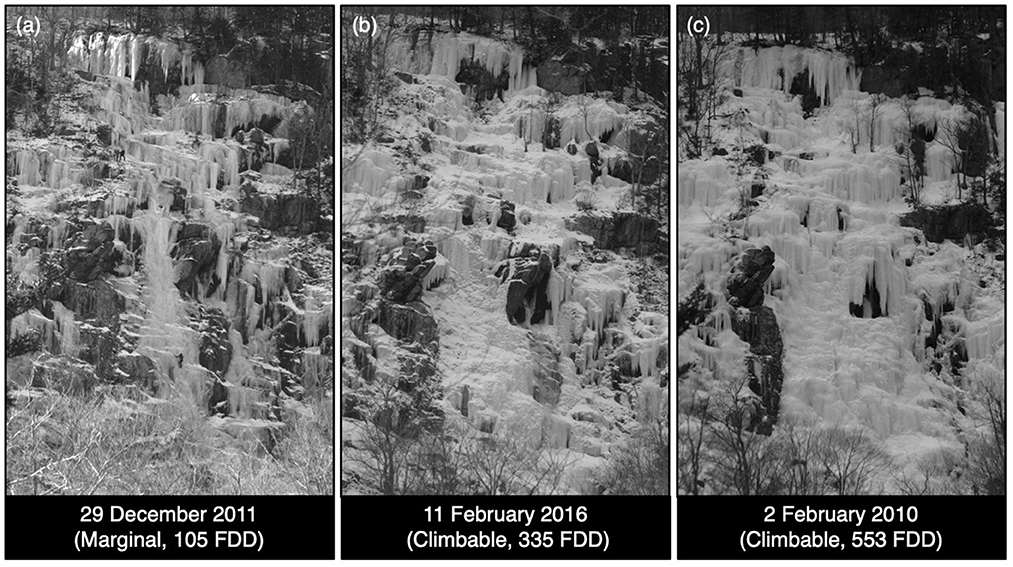
Figure 6. Analogs for ice formation between 2079 and 2099 under different scenarios based on AFDD values. (a) An AFDD of 105 C degree-days occurred in December of the 2011/2012 season and Standard Route was rated ‘marginal' in this condition. If future seasons yield multiple cold periods with AFDD of ~100 C degree-days, interspersed by melting periods with total ice collapse, Standard Route may exist in this condition throughout winter. (b) An AFDD of 335 C degree-days occurred in February of the 2015/2016 season. This condition is consistent with the median AFDD expected under RCP 8.5. (c) An AFDD of 553 C degree-days occurred in February of the 2009/2010 season. This condition is consistent with the median AFDD expected under RCP 4.5.
The analogs presented for RCP 8.5 (Figure 6c) and RCP 4.5 (Figure 6b) indicate the probable condition of Standard Route if all AFDD is concentrated during one cold spell. However, given the increasing prevalence of midwinter warm spells and rain, it is more likely that these AFDD would be spread out over the course of the season and interspersed with melting periods. This may induce ice diminishment, collapse, and/or weak bonding, leading to overall poorer ice conditions and reduced climbability (consistent with Figure 6a).
3.3. Survey
All 20 survey respondents had at least 7 years of ice climbing experience, with most having climbed ice for considerably longer (range 7–30 years, median 21 years). In terms of professional activities, respondents had an average of 14 years ice guiding experience in the Mount Washington Valley (range 4 to 30 years), and 90% of respondents guide ice in the Mount Washington Valley at least 3 months per season. Most respondents live in the Mount Washington Valley (65%), while others live nearby or travel frequently to the area for guiding work.
On a given day, several variables consistent with climate-related changes were reported as having negative effects on ice conditions (Figure 7A); namely, rainfall, water flow behind ice features, variable ice conditions, and warming temperatures. Likewise, unfrozen river crossings were cited as reducing guides' ability to deliver positive experiences to guests on a given day, a situation that will become more common based on modeling projections of temperature change in the Mount Washington Valley (Huntington, 2003; Thellman et al., 2021). The variable reported to have the most overall positive effect for guests' experience was plastic ice; that is, ice that is sufficiently soft to make placing ice tools easier, while also reducing the tendency of ice to shatter or “dinner plate” when struck. Such conditions tend to increase the enjoyment and success of new climbers. We note that at temperatures warmer than freezing, plastic ice may melt and create running water which may detract from climber enjoyment, though we did not inquire about the prevalence of this condition.
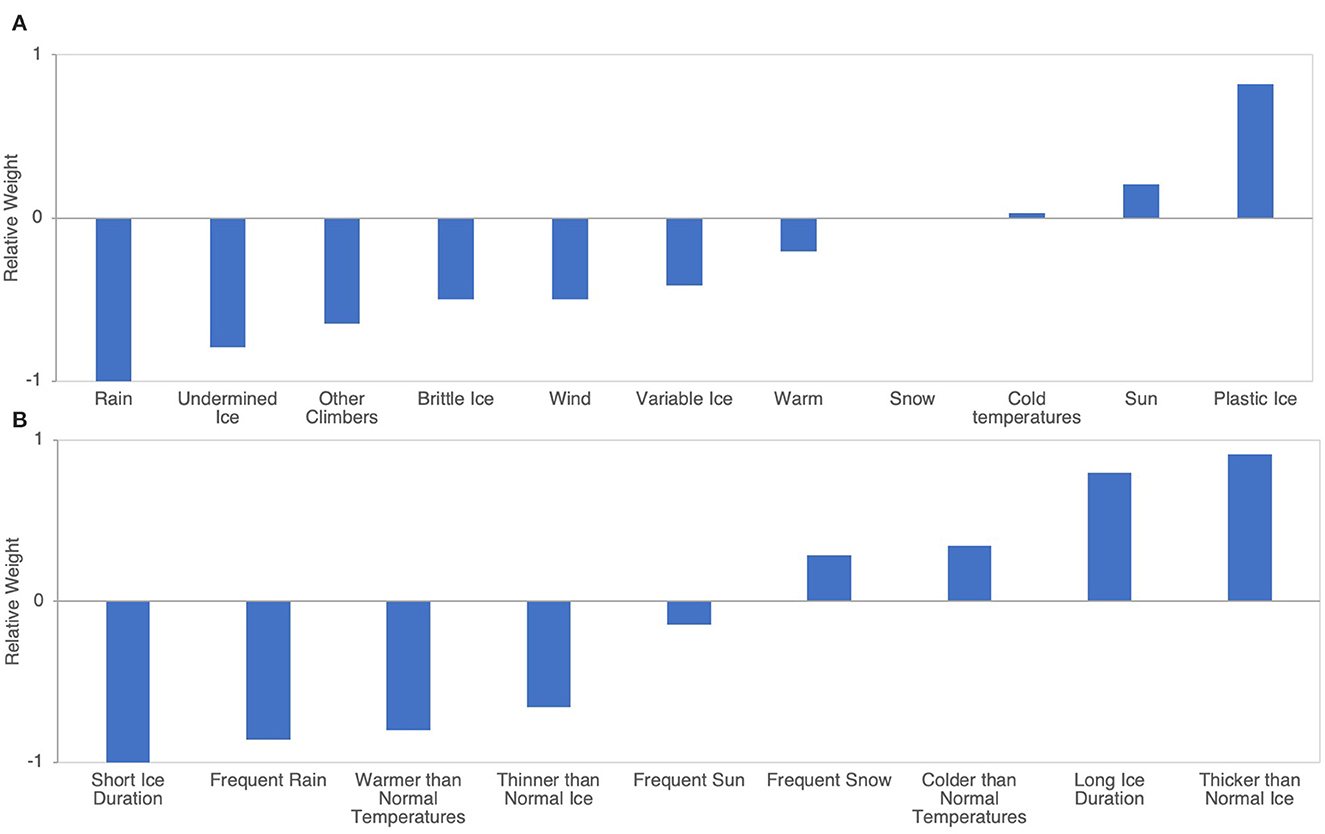
Figure 7. Variable affecting guides' ability to deliver positive experiences to guests (A) over the course of a given day and (B) over the course of a season. Negative values indicate parameters reduce guides abilities to deliver positive experiences; positive values indicate parameters improve guides abilities to deliver positive experiences.
Over the course of an ice climbing season, there are several climate-sensitive variables that have important effects on guiding activities (Figure 7B). Variables reported as having negative impacts on guiding include a short season of climbable ice (fewer opportunities for guides to deliver positive experience to guests and consequently less income), frequent rain events (which drive melt, ice instability, and generally unpleasant winter conditions), warmer than normal temperatures (which drives melt and ice instability), and thinner than normal ice (which can be more challenging and intimidating for novice ice climbers). Variables reported to have positive effects on guiding are essentially the converse of these negative variables (e.g., colder than normal temperatures, which supports ice growth and persistence; longer ice climbing season, which increases ice climbing opportunities).
Those variables having a negative impact on guiding in the Mount Washington Valley on a given day or over the course of a season are likely to occur more frequently with regional climate warming; those having a positive impact are likely to occur less frequently. However, modest climate warming might enhance certain variables that enhance guides' ability to deliver rewarding experiences to guests in a positive direction in the short-term. For example, plastic ice might become more common (and brittle ice less common) with modest climate warming. Nevertheless, guides expect climate warming to have overall adverse impacts on cold dependent activities like ice climbing (e.g., plastic ice turning to melting ice, shorter ice climbing seasons, or ice never forming in the first place).
Such observations are consistent with guides' level of concern about climate change, where 70% of respondents indicated that they are “alarmed” and 30% of respondents indicated they are “concerned” about climate change, which is markedly different than the rest of the United States population where 26% of respondents were “alarmed” and 29% were “concerned” (Chryst et al., 2018). Moreover, in our study, 85% of guide respondents believe they will experience “a moderate amount” or “a great deal” of adverse effects due to climate change, highlighting the sensitivity of ice climbing guides to climate change.
3.4. Focus group
The focus group began with guide participants discussing their observations of changing ice climbing conditions in the Mount Washington Valley. These “bottom-up” insights were consistent with “top-down” modeling and survey results, but added nuance and specificity. For example, when discussing changes in season length, Participant #5 reflected: “There have been intermittent times in the 2000s when the ice climbing was excellent, but the longevity is waning. If you look at pictures of routes in the 60's, it's amazing the size of the routes back then. Now, I feel that once it starts to get cold, I better get active because it's not gonna last.” Similarly, Participant #4 observed that: “Overall the ice, particularly at Frankenstein, never gets as fat as it used to.” Participants unanimously associated such changes with increases in winter temperature, but Participant #5 clarified that: “we also have less snow than before and therefore less water falls into the seeps and the cracks that build ice climbs.”
Interestingly, many guide participants recognized a discrepancy between climate-related changes in the occurrence of reliable ice and perceptions of changes in season length. Changes in technical standards, particularly the advent of mixed climbing, have seen more climbers on ice when climbs are in conditions that might have been considered “unclimbable” 30 years ago. According to Participant #5: “Ice climbing was about climbing something that was thick, fat, and large in the old days. Then in the 1990s Jeff Lowe introduced mixed climbing and you were able to climb earlier or later because of changes in the sport.” This subjective change in perceptions about what constitutes climbable conditions has the effect of masking objective changes in season length, a kind of shifting baseline phenomenon.
Another important insight offered by guide participants was that changes in the length of reliable ice can be highly localized, even route specific, according to the nexus of distal (i.e., climate change) and proximate factors (e.g., groundwater dynamics, autumn rainfall). Although the exception, for some routes the climbable season might increase due to near-term climate change, especially those in very cold areas (higher altitude, north facing) where an intensification of melt/freeze dynamics might increase ice mass. Conversely, lower, south facing routes can be impacted dramatically by even minor warming. Proximate variables such as changes in groundwater flow can attenuate or reverse climate driven changes. And these determinants of ice formation can vary from year to year: “Different cliffs will have good years” (Participant #1). Such nuances are a natural part of ice climbing: “That's the game, finding good quality ice. But the opportunity is less than before” (Participant #1).
Alongside these observations of downward trends in the length of ice climbing seasons, respondents provided specific examples of how climate related changes are affecting their guiding activities. A commonly cited concern was an increase in ice and rockfall hazards. For example, Participant #1 indicated that there had been a dangerous occurrence on Standard Route in the middle of the 2016/2017 season (Figure 8): “…there was a large rain event and then it didn't get cold enough to reform properly. Bonds between ice and the wall had been eroded, and when it refroze, it didn't rebond. It wasn't as stable as it appeared and there was a spontaneous ice fall event.” This guide also expressed concern about spontaneous rock fall events, which along with icefall events, “could result in massive tragedy”. Participant #3 agreed: “If it isn't cold it can be really dangerous.”
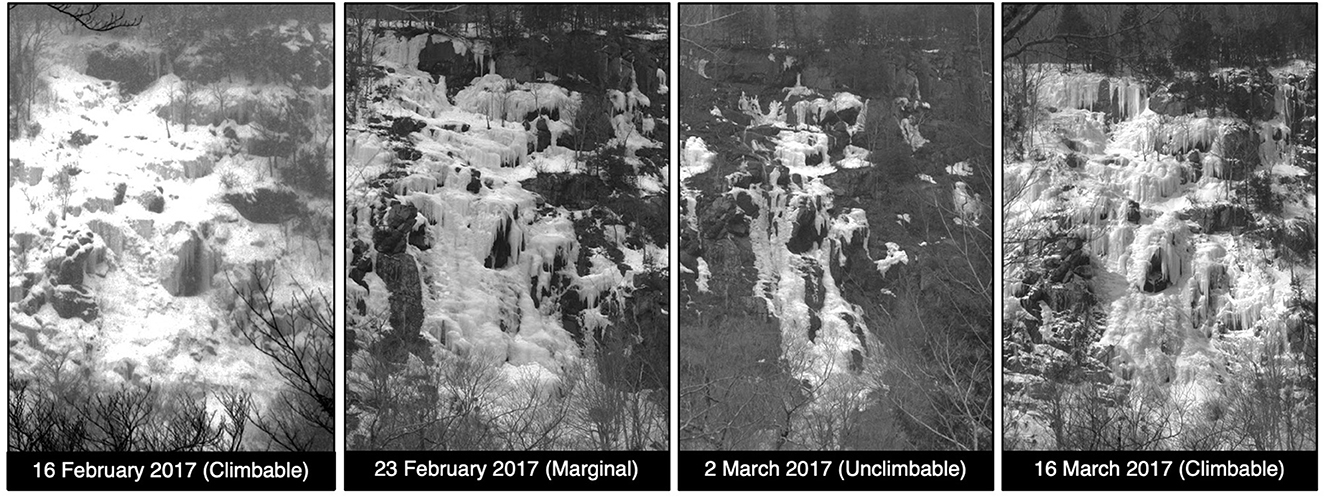
Figure 8. Course of an icefall event during a midwinter warm up during the 2016/2017 ice season, as referred to during the focus group session.
Compounding potential changes in the frequency and intensity of ice and rock fall events, crowding issues are making it more difficult to provide rewarding and safe experiences for guests. The drivers behind increasing crowding were threefold: climate change reducing the number of reliable ice climbs; social media leading to increased interest in visiting ice climbs, especially those that are reliable; and, more recently, the COVID pandemic leading to an increase in outdoor recreation, including ice climbing. “We're not able to spread out to those secondary routes anymore. That puts more folks in one place. Everyone goes to the more reliable routes. And as soon as something is posted on social media then the gravitational pull shifts to those routes” (Participant # 1). In addition, “COVID has pushed a lot of folks outdoors” (Participant #2), an insight consistent with findings from other studies examining the effects of the pandemic on participation in outdoor recreation (Taff et al., 2021; Outdoor Industry Association, 2022). And because some of those venturing into ice climbing areas without guides have little or no experience, their ability to perceive potentially hazardous conditions is low, increasing the likelihood of an accident related to an ice or rock fall event. This can increase stress for guides who are often the most experienced individuals on scene, and who must account for increasing numbers of individuals who might pose a threat to themselves or their guests.
Ultimately, the cumulative pressures of changes in season length, hazards, and crowding are making it difficult for guides to practice their craft: “You want truth in advertising. You want to schedule a date in advance and give a good experience. If [guests] have an idea in their mind of what it's gonna be like you want to show them that. When that pressure is in your control it's reasonable but when it isn't it's super difficult. It's becoming more difficult than ever before to stand behind what you offer and then deliver on that” (Participant #1).
Guides are adapting to counteract these emerging difficulties. For example, several guides mentioned seeking out alternate ice climbing routes across the Mount Washington Valley as a common response to reliability, hazard, and crowding issues. While this response can be effective, it also entails several challenges, including those related to guest satisfaction (e.g., not getting to climb the “classic” routes they hoped to) and guide comfort (e.g., taking guests to areas that may be both less familiar for guides and less forgiving for guests). Perhaps the most significant disadvantage of this adaptive response; however, is that guides often “have to spend more time scoping out alternate climbing routes” (Participant #5), adding a major increase to the time required to provide rewarding and safe experiences for guests. This need to scope out routes in advance–a major task for more remote routes–was noted as even more challenging due to limited snow cover in recent years; a thin snowpack makes approach trails slick and unpleasant. Moreover, the capacity to mobilize this adaptive response is highly dependent on guides' level of knowledge about potential viable alternate routes, suggesting that more experienced guides will have greater adaptive capacity with respect to this adaptation option: “I lean heavily on past experiences to understand how things will set in” (Participant # 1).
Another key adaptation is changing activities, particularly from ice climbing to mixed climbing (climbing rock and ice with ice tools) or dry tooling (climbing rock with ice tools). This option makes sense in the context of thinner and less reliable ice, and can provide new opportunities for local guides. However, “showing up and providing a good day no matter the conditions requires a much broader skillset as a guide” (Participant #1). Moreover, “If you're a guide that likes to do mixed routes then you can keep your season long but if your [guests] don't want to mixed climb then your season is much shorter” (Participant #5). However, mixed climbing and dry tooling are generally considered more demanding and technical forms of winter climbing and fewer climbers have the necessary skills. Relatedly, such a response is not feasible for bread-and-butter activities such as “Introduction to Ice Climbing” courses where guests are explicitly interested in cultivating basic skills on ice. For guests without the requisite skills or that want to climb ice exclusively, seeking out mixed and dry tooling options is not a viable adaptation option for guides. In general, participants felt that changing activities is more feasible for independent guides with experienced guests; guide services running large introductory courses “are less nimble” (Participant #1).
Given these challenges, adaptation to changing ice conditions by selecting alternate routes or changing activities was not considered by participants to be no or low-cost options. Instead, all mentioned growing demands on their knowledge and time as well as concerns about less guest demand for mixed and dry tooling objectives. Moreover, the dynamic nature of adaptation options, makes scheduling trips with guests more difficult: “[Guests] want to schedule way ahead of time but now we can't do that as often” (Participant #4). Although the knowledgeable guides from the focus group are finding ways to navigate changing winter conditions in the Mount Washington Valley, ultimately, “we're losing reliable ice and that hits the pocketbook” (Participant #3). Looking forward: “I think climbers will be a part of the first group that notices it's hard to adapt…at some point will we cross a line [with climate change] that changes everything” (Participant # 4). Such insights portend potentially transformative changes for ice climbing activities and associated livelihoods in the Mountain Washington Valley. This is consistent with our projections of declining ice season length and reliability for the region.
Adaptation strategies are also emerging amongst guiding communities elsewhere. Consistent with the strategies of ice climbing guides in the Mount Washington Valley, French Alpine guides adapt to climate-related changes by selecting alternate routes and changing activities, though one study identified changing seasonality (i.e., approaching routes at different times of the year in order find optimal conditions) as their most common adaptation strategy (Mourey et al., 2020). This makes sense considering the glaciated nature, significantly higher relief, and substantial elevation of the French Alps (Mont Blanc; 4,807 m) vs. the low-lying, temperate nature of the White Mountains (Mt. Washington; 1,917 m). Ultimately, adaptation strategies for guides are not “one-size-fits-all” and will depend on regionally and environmentally specific risks and opportunities presented by climate change.
4. Conclusions
To date there have been few studies examining the impacts of climate change for ice climbing, though warmer winters imply potentially significant consequences for the persistence of seasonal ice flows. In response, this study developed a novel mixed-methods approach combining archival analysis, climate modeling, and surveys and focus groups with local mountain guides to examine how climate change is affecting ice climbing activities in the Mount Washington Valley, New Hampshire. Our results reveal significant impacts on season length and the reliability of ice climbs, with attendant impacts for local guides:
• Today, the Mount Washington Valley's typical ice climbing season is both shorter and more variable than historical seasons, a conclusion supported by archival analysis, meteorological records, and evidence from interviews with experienced ice climbers in the region. Overall, climate change is likely to reduce the number of days with climbable ice each winter, in an increasingly patchy manner.
• Climate-related changes have led to less reliable ice characteristics as well as more hazardous climbing conditions, including enhanced ice and rock fall relative to historical conditions. When combined with growing pressure from crowding related to an increase in outdoor participation and social media, such dynamics are making guides' work increasingly more difficult and dangerous. To address these risks, climbers and guides could consider the actions outlined in Table 2 when planning itineraries.
• Guides are adapting to changes in season length, hazards, and crowding by drawing on their local expert knowledge and diverse climbing skills. However, sensitivity and adaptive capacity to changes amongst the guiding community is differentiated according to guides' level of knowledge and skill, their guests' ability and interests, and their respective business model (independent guide vs. guide service). All adaptations inflate operating costs by increasing demands on guides' time and effort. Limits to adaptation may exist when shortening ice seasons and increasing costs and/or risks render guiding activities infeasible.
• In the future, the season length and reliability of established ice climbs will be further diminished by climate change. Average season length could decrease from ~100 days to 65 days (RCP 4.5) or 30 days (RCP 8.5) by the end of the century (2079 to 2099), suggesting a substantial reduction in the quantity of climbable ice in the Mount Washington Valley. The magnitude of these changes threaten guides' ability to adapt effectively; this uncertainty is tied to society's realized decarbonization pathway.
• Ice climbing has a significant imprint on the culture and economy of the Mount Washington Valley. Projected changes in winter conditions and potential limits to adaptation calls the future of regional festivals, businesses, and guiding companies into question.
This first-of-its-kind study advances understanding of the relationship between warming winters, ice conditions, and guiding activities. Meaningful policies taken to mitigate global warming will help preserve the meteorological conditions necessary for ice climbing, with more aggressive action generally preserving more favorable conditions. However, even with aggressive mitigation some level of climate impact will occur. In this context, there is a need to institutionalize awareness of climate impacts and potential adaptation strategies within guide training methodologies, such as those offered by the American Mountain Guides Association and other certifying bodies. Future research examining the physical and human dimensions of warming winters for ice climbing is needed to inform these initiatives. The mixed-methods approach developed in this study, including its emphasis on collaboration between guides and researchers, can be further developed to address remaining knowledge gaps for other ice climbing areas within the United States and beyond.
Data availability statement
The raw data supporting the physical science in this article will be made available by the authors, without undue reservation. Data from work with human subjects cannot be shared due to confidentiality considerations.
Ethics statement
The studies involving human participants were reviewed and approved by Institutional Review Board for the Protection of Human Subjects in Research at the University of New Hampshire (#IRB-FY2021-40). The patients/participants provided their written informed consent to participate in this study. Written informed consent was obtained from the individual(s) for the publication of any potentially identifiable images or data included in this article.
Author contributions
JV contributed to writing, archive analysis, climate modeling, surveys, the focus group, and project management. GM contributed to writing, surveys, and focus group. EB contributed to writing, climate modeling, and surveys. TL contributed to the focus group and project management. All authors contributed to the article and approved the submitted version.
Funding
Funding for this research was provided by the American Alpine Club, the Petzl Foundation, and Black Diamond Equipment. Additionally, JV was supported by a National Geographic Young Explorer's grant, GM was supported by a Banting Postdoctoral Fellowship, and EB was supported by NSF #1832959.
Conflict of interest
The authors declare that the research was conducted in the absence of any commercial or financial relationships that could be construed as a potential conflict of interest.
Publisher's note
All claims expressed in this article are solely those of the authors and do not necessarily represent those of their affiliated organizations, or those of the publisher, the editors and the reviewers. Any product that may be evaluated in this article, or claim that may be made by its manufacturer, is not guaranteed or endorsed by the publisher.
Supplementary material
The Supplementary Material for this article can be found online at: https://www.frontiersin.org/articles/10.3389/fhumd.2023.1097414/full#supplementary-material
Supplementary Figure 1. Simulation of AFDD at Pinkham Station under RCPs 4.5 and 8.5, with historical AFDD superimposed.
References
Adger, W. N. (2006). Vulnerability. Glob. Environ. Change 16, 268–281. doi: 10.1016/j.gloenvcha.2006.02.006
Beck, H. E., Zimmermann, N. E., McVicar, T. R., Vergopolan, N., Berg, A., Wood, E. F., et al. (2018). Present and future Köppen-Geiger climate classification maps at 1-km resolution. Sci. Data 5, 180214. doi: 10.1038/sdata.2018.214
Bengtsson, M. (2016). How to plan and perform a qualitative study using content analysis. NursingPlus Open 2, 8–14. doi: 10.1016/j.npls.2016.01.001
Burakowski, E. A., Contosta, A. R., Grogan, D., Nelson, S. J., Garlick, S., Casson, N., et al. (2022). Future of winter in Northeastern North America: climate indicators portray warming and snow loss that will impact ecosystems and communities. Northeast. Nat. 28, 180–207. doi: 10.1656/045.028.s1112
Campbell, J. L., Ollinger, S. V., Flerchinger, G. N., Wicklein, H., Hayhoe, K., Bailey, A. S., et al. (2010). Past and projected future changes in snowpack and soil frost at the Hubbard Brook Experimental Forest, New Hampshire, USA. Hydrol. Process. 24, 2465–2480. doi: 10.1002/hyp.7666
Carey, M., Molden, O. C., Rasmussen, M. B., Jackson, M., Nolin, A. W., Mark, B. G., et al. (2017). Impacts of glacier recession and declining meltwater on mountain societies. Ann. Am. Assoc. Geogr. 107, 350–359. doi: 10.1080/24694452.2016.1243039
Chryst, B., Marlon, J., van der Linden, S., Leiserowitz, A., Maibach, E., Roser-Renouf, C., et al. (2018). Global warming's “six Americas short survey”: Audience segmentation of climate change views using a four question instrument. Environ. Commun. 12, 1109–1122. doi: 10.1080/17524032.2018.1508047
Coe, J. A., Bessette-Kirton, E. K., and Geertsema, M. (2018). Increasing rock-avalanche size and mobility in Glacier Bay National Park and preserve, Alaska detected from 1984 to 2016 Landsat imagery. Landslides 15, 393–407. doi: 10.1007/s10346-017-0879-7
Eusden, J. D., Thompson, W., Fowler, B., Davis, P. T., Bothner, W., Boisvert, R., et al. (2013). The geology of New Hampshire's white mountains. Fac. Scholarsh. Durand Press.
Gauthier, F., Allard, M., and Hétu, B. (2015). Ice Wall Growth and Decay: Meteorological Analysis and Modelling. Permafr. Periglac. Process. 26, 84–102. doi: 10.1002/ppp.1835
Gauthier, F., Montagnat, M., Weiss, J., Allard, M., and Hétu, B. (2013). Ice cascade growth and decay: a thermodynamic approach. J. Glaciol. 59, 507–523. doi: 10.3189/2013JoG12J206
Gruber, S., and Haeberli, W. (2009). “Mountain Permafrost,” in R. Margesin, eds Permafrost Soils Soil Biology (Berlin, Heidelberg: Springer). p. 33–44. doi: 10.1007/978-3-540-69371-0_3
Hayhoe, K., Wake, C. P., Huntington, T. G., Luo, L., Schwartz, M. D., Sheffield, J., et al. (2007). Past and future changes in climate and hydrological indicators in the US Northeast. Clim. Dyn. 28, 381–407. doi: 10.1007/s00382-006-0187-8
Huntington, T. G. (2003). Climate warming could reduce runoff significantly in New England, USA. Agric. For. Meteorol. 117, 193–201. doi: 10.1016/S0168-1923(03)00063-7
IPCC (2014). “Long-term Climate Change: Projections, Commitments and Irreversibility Pages 1029 to 1076,” in Climate Change 2013—The Physical Science Basis: Working Group I Contribution to the Fifth Assessment Report of the Intergovernmental Panel on Climate Change (Cambridge: Cambridge University Press), 1029–1136. doi: 10.1017/CBO9781107415324.024
IPCC (2021). Climate Change 2021: The Physical Science Basis. Contribution of Working Group I to the Assessment Report of the Intergovernmental Panel on Climate Change. Cambridge, United Kingdom and New York, NY, USA: Cambridge University Press
Karmalkar, A. V., and Bradley, R. S. (2017). Consequences of global warming of 1.5°C and 2°C for regional temperature and precipitation changes in the contiguous United States. PLOS ONE 12, e0168697. doi: 10.1371/journal.pone.0168697
Lewis, S. P., and Wilcox, R. (2002). An Ice Climber's Guide to Northern New England. TMC Books L.L.C.
McDowell, G., Huggel, C., Frey, H., Wang, F. M., Cramer, K., Ricciardi, V., et al. (2019). Adaptation action and research in glaciated mountain systems: are they enough to meet the challenge of climate change? Glob. Environ. Change 54, 19–30. doi: 10.1016/j.gloenvcha.2018.10.012
McDowell, G., and Koppes, M. (2017). Robust adaptation research in high mountains: integrating the scientific, social, and ecological dimensions of glacio-hydrological change. Water 9, 739. doi: 10.3390/w9100739
Montagnat, M., Weiss, J., Cinquin-Lapierre, B., Labory, P. A., Moreau, L., Damilano, F., et al. (2010). Waterfall ice: formation, structure and evolution. J. Glaciol. 56, 225–234. doi: 10.3189/002214310791968412
mountainproject.com (n.d.). Mt. Proj. Available online at: https://www.mountainproject.com/area/106099658/nh-ice-and-mixed (accessed on October 14 2022).
Mourey, J., Lacroix, P., Duvillard, P-. A., Marsy, G., Marcer, M., Malet, E., et al. (2022a). Multi-method monitoring of rockfall activity along the classic route up Mont Blanc (4809 a.s.l.) to encourage adaptation by mountaineers. Nat. Hazards Earth Syst. Sci. 22, 445–460. doi: 10.5194/nhess-22-445-2022
Mourey, J., Marcuzzi, M., Ravanel, L., and Pallandre, F. (2019). Effects of climate change on high Alpine mountain environments: evolution of mountaineering routes in the Mont Blanc massif (Western Alps) over half a century. Arct. Antarct. Alp. Res. 51, 176–189. doi: 10.1080/15230430.2019.1612216
Mourey, J., Perrin-Malterre, C., and Ravanel, L. (2020). Strategies used by French Alpine guides to adapt to the effects of climate change. J. Outdoor Recreat. Tour. 29, 100278. doi: 10.1016/j.jort.2020.100278
Mourey, J., Ravanel, L., and Lambiel, C. (2022b). Climate change related processes affecting mountaineering itineraries, mapping and application to the Valais Alps (Switzerland). Geogr. Ann. Ser. Phys. Geogr. 104, 109–126. doi: 10.1080/04353676.2022.2064651
Murray, G. L. D., Colgan, A. M., Nelson, S. J., Kelsey, E. P., and Kimball, K. D. (2021). Climate Trends on the Highest Peak of the Northeast: Mount Washington, NH. Northeast. Nat. 28:64–82. doi: 10.1656/045.028.s1105
mwv-icefest.com (n.d.). Mt. Wash. Val. Ice Festiv. Available online at: https://www.mwv-icefest.com/ (accessed on October 14, 2022).
NEClimbs.com (n.d.). NEClimbs. Available online at: http://neclimbs.com/ (accessed on October 13, 2022).
Ning, L., and Bradley, R. S. (2015). Snow occurrence changes over the central and eastern United States under future warming scenarios. Sci. Rep. 5, 17073. doi: 10.1038/srep17073
Outdoor Industry Association (2022). Outdoor participation trends report. Outdoor Ind. Assoc. Available online at: https://outdoorindustry.org/resource/2022-outdoor-participation-trends-report/ (accessed on January 23, 2023).
Payne, G., and Payne, J. (2004). Key Concepts in Social Research. 1 Oliver's Yard, 55 City Road, London England EC1Y 1SP United Kingdom: SAGE Publications, Ltd doi: 10.4135/9781849209397
Pierce, D. W., Cayan, D. R., and Thrasher, B. L. (2014). Statistical downscaling using localized constructed analogs (LOCA). J. Hydrometeorol. 15, 2558–2585. doi: 10.1175/JHM-D-14-0082.1
Rawlins, M. A., Bradley, R. S., Diaz, H. F., Kimball, J. S., and Robinson, D. A. (2016). Future decreases in freezing days across North America. J. Clim. 29, 6923–6935. doi: 10.1175/JCLI-D-15-0802.1
Salim, E., Ravanel, L., Deline, P., and Gauchon, C. (2021). A review of melting ice adaptation strategies in the glacier tourism context. Scand. J. Hosp. Tour. 21, 229–246. doi: 10.1080/15022250.2021.1879670
Scott, D., Steiger, R., Knowles, N., and Fang, Y. (2020). Regional ski tourism risk to climate change: an inter-comparison of Eastern Canada and US Northeast markets. J. Sustain. Tour. 28, 568–586. doi: 10.1080/09669582.2019.1684932
Smit, B., and Wandel, J. (2006). Adaptation, adaptive capacity and vulnerability. Glob. Environ. Change 16, 282–292. doi: 10.1016/j.gloenvcha.2006.03.008
Taff, B. D., Rice, W. L., Lawhon, B., and Newman, P. (2021). Who started, stopped, and continued participating in outdoor recreation during the COVID-19 pandemic in the United States? results from a national panel study. Land 10, 1396. doi: 10.3390/land10121396
Thellman, A., Jankowski, K. J., Hayden, B., Yang, X., Dolan, W., Smits, A. P., et al. (2021). The ecology of river ice. J. Geophys. Res. Biogeosci. 126, e2021JG.006275. doi: 10.1029/2021JG006275
Turner, B. L., Kasperson, R. E., Matson, P. A., McCarthy, J. J., Corell, R. W., Christensen, L., et al. (2003). A framework for vulnerability analysis in sustainability science. Proc. Natl. Acad. Sci. 100, 8074–8079. doi: 10.1073/pnas.1231335100
USGCRP (2017). Climate Science Special Report: Fourth National Climate Assessment, Volume I. Washington, DC, USA: U.S. Global Change Research Program
Waterman, L., Waterman, G., Garlick, S., and Wejchert, M. (2018). Yankee Rock and Ice: A History of Climbing in the Northeastern United States. Stackpole Books.
Keywords: climate change, vulnerability, adaptation, ice climbing, winter sports, guides, recreation, New Hampshire
Citation: Voorhis J, McDowell G, Burakowski E and Luneau T (2023) The implications of warmer winters for ice climbing: A case study of the Mount Washington Valley, New Hampshire, USA. Front. Hum. Dyn. 5:1097414. doi: 10.3389/fhumd.2023.1097414
Received: 13 November 2022; Accepted: 03 February 2023;
Published: 02 March 2023.
Edited by:
Emmanuel Salim, Université de Lausanne, SwitzerlandReviewed by:
Ludovic Ravanel, UMR5204 Environnements, Dynamiques et Territoires de la Montagne (EDYTEM), FranceShah Md Atiqul Haq, Shahjalal University of Science and Technology, Bangladesh
Copyright © 2023 Voorhis, McDowell, Burakowski and Luneau. This is an open-access article distributed under the terms of the Creative Commons Attribution License (CC BY). The use, distribution or reproduction in other forums is permitted, provided the original author(s) and the copyright owner(s) are credited and that the original publication in this journal is cited, in accordance with accepted academic practice. No use, distribution or reproduction is permitted which does not comply with these terms.
*Correspondence: Jimmy Voorhis, amFtZXN2b29yaGlzQGdtYWlsLmNvbQ==