- 1Department of Biomedical and Dental Sciences and Morphofunctional Imaging, University of Messina, Messina, Italy
- 2Unit of Statistical and Mathematical Sciences, Department of Economics, University of Messina, Messina, Italy
- 3Department of Cardiovascular Medicine, Fondazione Policlinico Universitario A. Gemelli-IRCCS, Rome, Italy
- 4Department of Biomedical Sciences and Morphological and Functional Imaging, University Hospital Messina, Messina, Italy
Introduction: Non-alcoholic fatty liver disease (NAFLD) is the most prevalent form of chronic liver disease in the world, and it is characterized by an excessive hepatic fat accumulation in more than 5% of hepatocytes documented by histology in the absence of alcohol consumption. It is a multifactorial pathology, where genetic component plays a fundamental role: the loss-of-function polymorphisms of genes coding for glutathione S-transferases would predispose to the pathology onset, also in the absence of other risk factors. The aim of the study was to evaluate the relation between the “NULL” GST-T1 and GST-M1 polymorphisms and the onset of NAFLD.
Methods: A group of 117 “apparently healthy” Caucasian volunteers, selected from a larger population through the analysis of previously administered short questionnaires, underwent both magnetic resonance imaging-proton density fat fraction (MRI-PDFF) and buccal swabs: the aim was to identify the possible presence of hepatic steatosis and of the aforementioned “NULL” polymorphisms of interest.
Results: A statistically significant association between the GST-T1 and GST-M1 “NULL” genotypes and the probability of developing NAFLD has been identified. In particular, the GST-T1 “NULL” genotype has been associated with a greater probability of developing steatosis in early age, while the GST-M1 “NULL” genotype seems to increase the risk of developing a higher grade of steatosis. No statistically significant correlations between the “NULL” genotype and sex have been detected.
Discussion: Among the numerous risk factors capable of predisposing to NAFLD onset and progression, the genetic factors seem to play an important role. In particular, GST-T1 and GST-M1 “NULL” polymorphisms would appear to acquire even greater importance, as their loss of function results in an increase of oxidative stress. At high concentrations, ROS can determine oxidative modifications of cellular macromolecules, such as lipids, determining their accumulation into hepatocytes. The study also highlighted the importance of MRI-PDFF for hepatic steatosis diagnosis: this method allows the acquisition of data comparable to those of conventional biopsy; however, it permits the entire liver parenchyma to be visualized.
Conclusion: A statistically significant correlation between the presence of GST-T1 and GST-M1 “NULL” genotypes and the presence of hepatic steatosis has been found.
1 Introduction
“The future is fatty”: so begins a 2013 editorial aimed at clarifying the challenges for an early diagnosis (lifestyle, genetic, imaging) of non-alcoholic fatty liver disease (NAFLD) (1). NAFLD is the most prevalent form of chronic liver disease in the world, frequently associated with obesity, type 2 diabetes mellitus, and insulin resistance (IR) (2).
NAFLD is characterized by an excessive hepatic fat accumulation, often responsible for the onset of IR, and it is defined by the presence of steatosis in >5% of hepatocytes (according to histological analysis), without ethanol consumption. In fact, the diagnosis of NAFLD requires the exclusion of both secondary causes and a daily alcohol consumption greater than P30 g for men and P20 g for women (3).
NAFLD is considered a multifactorial disease, a product of the numerous interactions between environmental factors, nutrition, and genetic factors. The interaction between all these factors is responsible for the disease phenotype and its progression (4, 5).
Given the growing interest of the scientific community on the genetic basis of NAFLD, in recent years, various associations have been described at the genomic level, particularly on some genes, which have improved our knowledge on the genetic basis of NAFLD (5). In fact, it seems that some genetic polymorphisms increase an individual’s susceptibility to environmental factors that, consequently, lead to the development of NAFLD, even in the case of an apparent lack of other risk factors (5). However, recently, the American Association for the Study of Liver Diseases (AASLD) in collaboration with other associations suggested a new nomenclature for steatotic liver disease, particularly the use of metabolic dysfunction-associated steatotic liver disease (MASLD) in place of NAFLD (6). This change has been introduced because several doubts were highlighted regarding the appropriateness of the term “non-alcoholic” that did not express accurately the etiology of the disease (for example, it is well known that there are overlapping biological processes that contribute to both NAFLD and ALD) (6). Moreover, the term “fatty” has been described as stigmatizing (6). Thus, the nomenclature MASLD, defined as the presence of hepatic steatosis associated with one cardiometabolic risk factor and no other discernible cause, has been widely accepted (6).
Since the first genome-wide association study (GWAS) was conducted to evaluate the genetic susceptibility of NAFLD, numerous other candidate gene studies have been conducted (7, 8).
The scientific literature has proposed several genes as both potentially responsible for the onset of this pathology and capable of influencing its natural history: among these, the membrane-bound O-acyl-transferase domain-containing 7 (MBOAT7), patatin-like phospholipase domain-containing 3 (PNPLA3), transmembrane 6 superfamily member 2 (TM6SF2), 17-beta hydroxysteroid dehydrogenase 13 (HSD17B13), and glucokinase regulator (GCKR) would appear to be those of greater interest (9). Further studies have focused on the possible association between increased oxidative stress and the onset of NAFLD (10).
In fact, there is a growing line of evidence that polymorphisms in genes encoding enzymes responsible for xenobiotic metabolism are among the key players in determining interindividual susceptibility to liver-related diseases (6). Oxidative stress and reactive oxygen species (ROS) production have also been widely implicated in the development of NAFLD. ROS production promotes lipid peroxidation, which leads to the formation of extremely reactive aldehyde compounds and the consequent damage at the intracellular level. At the same time, a decrease in antioxidant compounds is observed, such as GSTs, superoxide dismutase, and catalases (6).
Therefore, it can be stated that fatty liver is due to an interplay between genetic and environmental factors, with a major role played by oxidative stress.
In oxidative stress, many xenobiotic agents are metabolized by enzymes of both “phase I,” leading to the formation of procarcinogenic substances through oxidative reactions, and “phase II,” where metabolic intermediate products of the oxidative process are conjugated with endogenous mediators, leading to the production of hydrophilic products that can be easily excreted by the organism. GST is an example of a heterogeneous group of “phase II” enzymes (10).
GSTs are ubiquitous enzymes that catalyze the conjugation of reduced glutathione to electrophilic centers of various exogenous and endogenous substrates (xenobiotics, drugs, poisons, and ROS). Their importance in the human antioxidant system is confirmed by the significantly increased risk of oxidative stress‐related diseases in subjects bearing a “NULL” genotype for specific GST isoforms (i.e., homozygosity for genetic deletion), resulting in the lack of phenotypic expression of the corresponding enzyme (11, 12).
In humans, seven different isoforms of this enzyme have been found (α, ξ, μ, π, θ, σ, ω). Among these, GST-M1 falls into the μ class, while and GST-T1 falls into the θ class (13).
It is known that these genes can be affected by several mutations, capable of determining either a functional reduction or a complete absence of the related protein (14).
Numerous studies have also demonstrated that the functional alteration of the proteins encoded by the aforementioned genes renders their detoxification capacity ineffective, increasing oxidative stress (15). In fact, the “NULL” genotypes of GST-M1 and GST-T1 have been associated with an increased risk of developing several pathologies, including NAFLD, type 2 diabetes, and drug-induced hepatotoxicity (15).
Furthermore, literature data indicate geographic and ethnic variations in the frequency distribution of the “NULL” genotypes (12). Recent studies have documented that the percentage of individuals who do not express the enzyme encoded by the GST-M1 polymorphism is significantly higher in Caucasian and Asian populations than in the African population, while the frequency of the “NULL” genotype of the GST-T1 would be in the order of 60% among Asians, 40% among Africans, and approximately 20% among Caucasians (12, 16–18). The detected high frequency of the “NULL” genotype of these genetic polymorphisms (more than 50% for the GST-M1 polymorphism) has also been confirmed by a population study conducted in Eastern Sicily (11, 19).
The study aims to evaluate the relation between the GST-T1 and GST-M1 gene polymorphisms and the presence of NAFLD and to confirm the importance of magnetic resonance imaging-proton density fat fraction (MRI-PDFF) in quantifying liver fat fraction. MRI is considered a “state-of-the-art weapon” to quantify liver steatosis, considering that its reliability is by far better than ultrasonography, being comparable to biopsy.
2 Methods
A group of 117 volunteers were selected from a larger population through the analysis of previously administered short questionnaires: these questionnaires had the aim of selecting “apparently healthy” subjects, i.e., apparently without any risk factors.
In fact, the questionnaire was aimed at obtaining information on eating habits, smoking, lifestyle, professional profile (near and remote pathological history, physiological history, work history), use of drugs, and/or presence of concomitant pathologies, including those with metabolic disorders, ascertained by negative blood tests performed within the previous 3 months. Therefore, all enrolled subjects have a body mass index (BMI) between 18.5 and 24.9 and declare that they do not take drugs for any pathologies. The questionnaire was administered to Caucasian individuals residing in northeastern Sicily.
Both authorizations for the processing of sensitive data and informed consent have been acquired. These subjects then underwent genetic analysis.
2.1 Genetic research
Each patient underwent sampling of buccal mucosa cells through sterile tamponade. DNA extraction was carried out according to the Chelex® 100 method, starting from the individual buccal swabs belonging to each subject of the group under study. Oligonucleotide sequences reported in the literature were used as the primer for the polymerase chain reaction (PCR) (16).
A multiplex has been set up, which is useful for the co-amplification of the loci GST-T1, GST-M1, and β-globin (the latter as a positive control of the reaction itself), in a final volume of 25 μl including 2.5 μl of extract (5–250 ng of DNA), 0.5 μM of each primer, 2.5 μl of Taq buffer (10× PCR Buffer II, Applied Biosystems, Waltham, Massachusetts, USA), 2 μl of MgCl 25 mM (Applied Biosystems, Waltham, Massachusetts, USA), 0.5 μl of dNTP mix (10 mM of PCR Nucleotide Mix, Promega, Madison, Wisconsin, USA), and 1 U of Taq polymerase (DyNAzyme II DNA polymerase, Finnzymes, Espoo, Finland).
The reaction was carried out with the use of a thermocycler (PCR Sprint, Hybaid, Teddington, United Kingdom) for a total of 30 amplification cycles: denaturation at 95°C for 1 min, annealing at 60°C for 1 min, and extension to 72°C for 1 min. The analysis of PCR products was executed with vertical electrophoresis on denaturing gel 6% polyacrylamide in ultrathin 7 M urea (0.4 mm) in TBE buffer 1×, using the following running conditions: 2,000 V, max mA, max W, for 150 min.
Migration bands related to the amplification products (480 bp for GST-T1 and 215 bp for GST-M1) were visualized by silver staining and identified by comparing the number of base pairs to a standard DNA ladder (DNA pGEM® marker, Promega, Madison, Wisconsin, USA). The following primers were used:
- GST-M1:
• forward primer 5′-GAACTCCCTGAAAAGCTAAAGC-3′,
• reverse primer 5′-GTTGGGCTCAAATATACGGTGG-3′;
- GST-T1:
• forward primer 5′-TTCCTTACTGGTCCTCACATCTC-3′,
• reverse primer 5′-TCACCGGATCATGGCCAGCA-3′.
2.2 Magnetic resonance imaging
Fat quantification (PDFF) was performed with a 1.5-Tesla scanner using a GE Quant T1 3D multi-echo sequence with the following parameters:
● repetition time (TR) 500 ms;
● six echoes with first echo time (ET) 1.5 ms and interecho spacing 1.3 ms;
● flip angle 5°;
● acquisition time 13 s.
Nowadays, hepatic PDFF is a standardized technique based on MRI. It represents a reproducible biomarker of liver fat content that has been applied as an endpoint in clinical trials and whose successful implementation into clinical care is an important goal. On the other hand, MR spectroscopy (MRS), an accepted non-invasive reference standard for PDFF measurement, is not widely available. Moreover, it is capable of sampling only small portions of the liver, leading to sampling variability. However, it allows an accurate in-vivo differentiation of the characteristic fat peaks which cannot be observed with PDFF (17).
MRI fat quantification methods use a low flip angle to minimize the spin-lattice (T1*) relaxation time, acquire multiple echoes to measure the difference in the characteristic relaxation for water and fat species when they relax in-phase and out-of-phase, and incorporate into their mathematical model the multifrequency interference effects of protons in fat (18). Several studies in adults have shown that MRI-estimated PDFF (MRI-PDFF) agrees closely with MRS-measured PDFF (MRS-PDFF) and biopsy, thus suggesting their future wide use for the quantification of hepatic PDFF in populations instead of MRS or biopsy (19).
According to the literature, steatosis value made it possible to divide the enrolled subjects into four classes: healthy individuals (steatosis value less than 5%), individuals with mild steatosis (6%–33%), individuals with moderate steatosis (34%–66%), and individuals with severe steatosis (>66%).
2.3 Statistical analysis
The numerical data were expressed as mean, standard deviation, median, and range (minimum and maximum), and the categorical variables as absolute frequencies and percentages.
The non-parametric approach was used since numerical variables were not normally distributed, verified by the Kolmogorov–Smirnov test.
The Spearman correlation test was applied in order to assess the possible correlation between the percentage of steatosis, age, and null positivity of genotypes T1 and M1.
In order to assess the existence of significant differences between categories of genotype (NULL vs. NO NULL), the Mann–Whitney test was applied with references to numerical parameters (age, percentage of steatosis), and the chi-square test was used for categorical variables (gender and grade of steatosis). Some boxplots were realized to better visualize the data.
Finally, a cumulative proportional odds model was estimated in order to identify significant predictive factors of the grade of steatosis, since response variables were ordinal on three ordered levels; the covariates were gender, age, GST-T1, and GST-M1. These models can be implemented through the Polytomous Universal Model (PLUM) procedure of the statistical software SPSS (Norušis, 2009).
Statistical analyses were performed using SPSS 27.0 for Windows package. A p-value lower than 0.05 was statistically significant.
3 Results
A population of 117 apparently healthy volunteers underwent both genetic test for the identification of GST-T1 and GST-M1 gene polymorphisms and MRI exams to evaluate the presence and grade of NAFLD. The subjects were between 27 and 69 years of age, with an average age of 49.98, consisting of 61 men (52.1%) and 56 women (47.9%).
After performing genetic analysis, the following results were obtained: 53 subjects (45.3%) had “NO NULL” GST-T1 polymorphism and 64 (54.7%) had “NULL” GST-T1 polymorphism. Sixty-six subjects (56.4%) had “NO NULL” GST-M1 polymorphism, and 51 (43.6%) had GST-M1 polymorphism (Figures 1A, B).
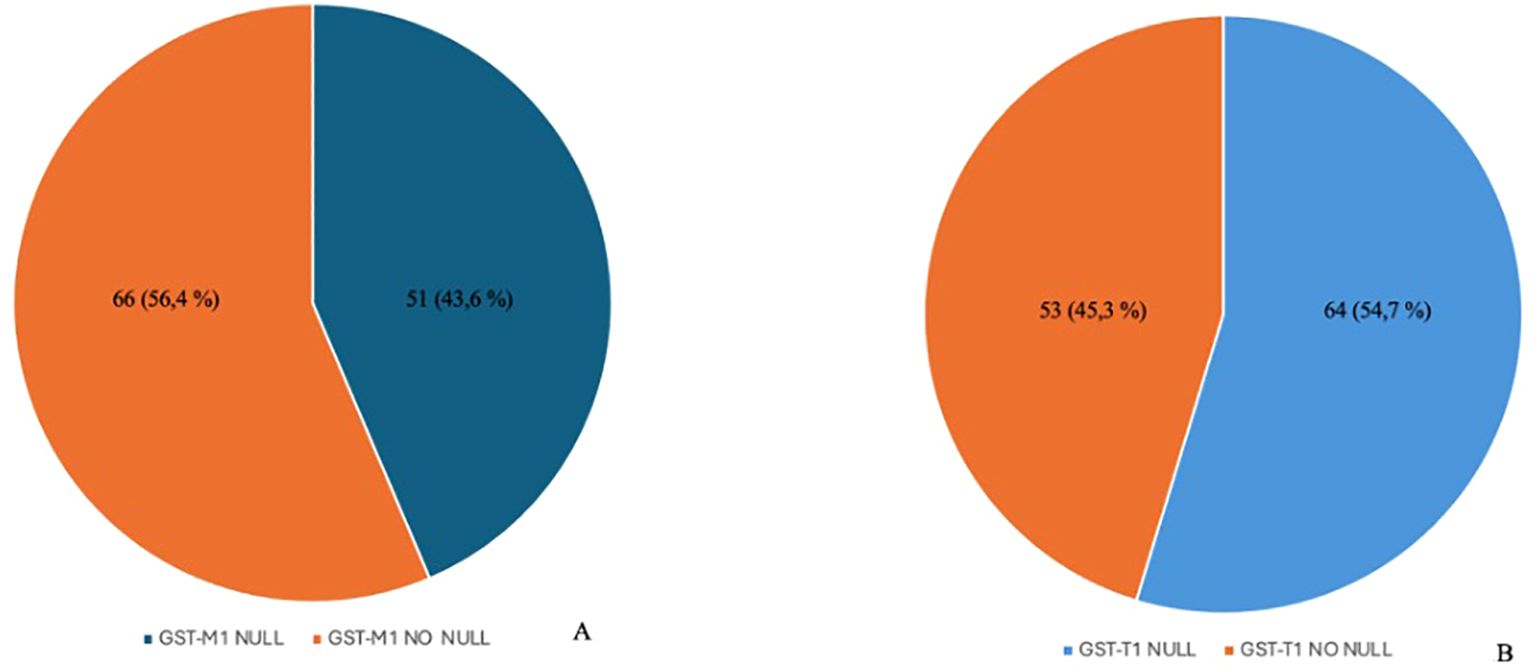
Figure 1. Distribution of polymorphisms in the enrolled population. The image shows a higher prevalence of the GST-M1 NO NULL genotype compared to the NULL genotype (A) and the prevalence of the GST-T1 NULL genotype compared to the NO NULL genotype (B). GST-M1, Glutathione S-transferases Mu 1; GST-T1, Glutathione S-Transferase Theta 1.
The volunteers subsequently underwent MRI: 28 subjects (23.9%) had no steatosis (steatosis percentage <5%), 64 (54.7%) had mild steatosis (steatosis percentage 6%–33%), and 25 (21.4%) had moderate steatosis (steatosis percentage 34%–66%). No subjects with severe steatosis (>66%) were identified (Table 1). GE T1-weighted in-phase images show loss of hepatic signal on opposed phase images, demonstrating the presence of steatosis (Figures 2A, B). However, a quantification of fat fraction is not possible. On the other hand, both spectroscopy and PDFF sequence allow a reliable evaluation of the liver fat fraction. The PDFF sequence allows to evaluate the whole liver (Figures 3, 4).
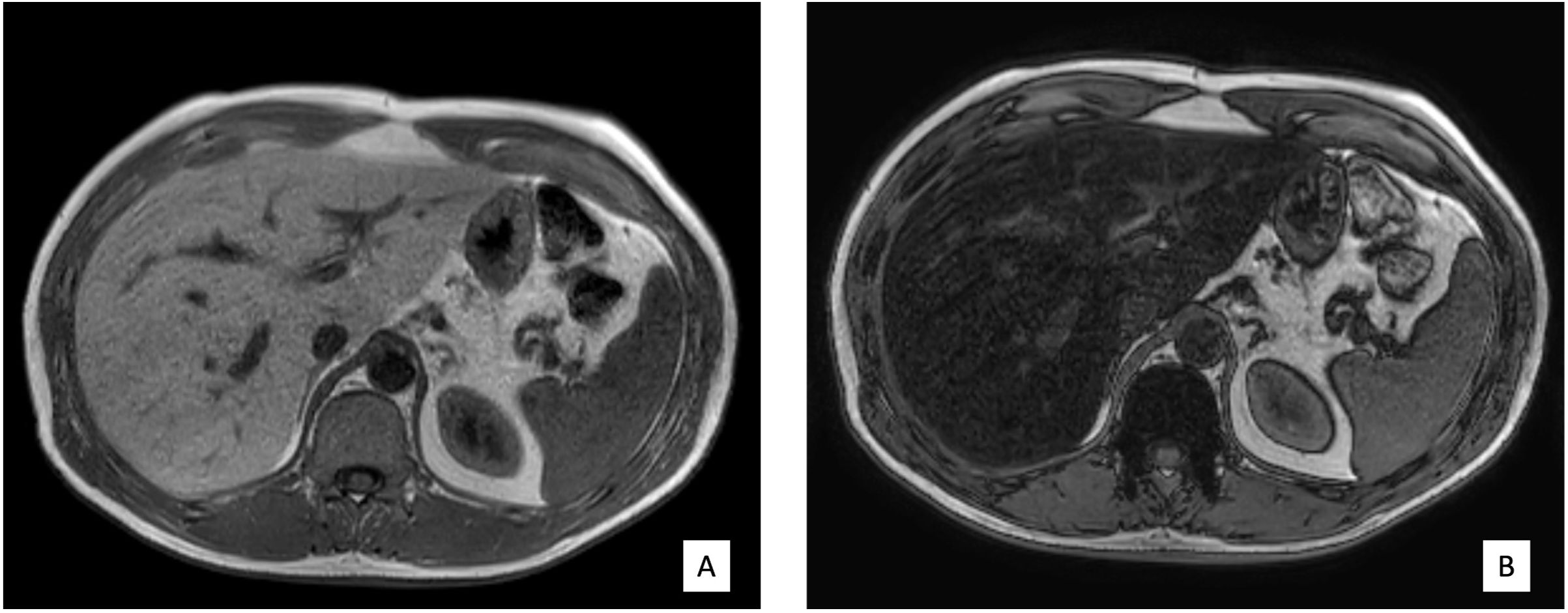
Figure 2. (A, B) Case 32: in-phase images showing loss of hepatic signal on opposed-phase images, demonstrating the presence of steatosis.
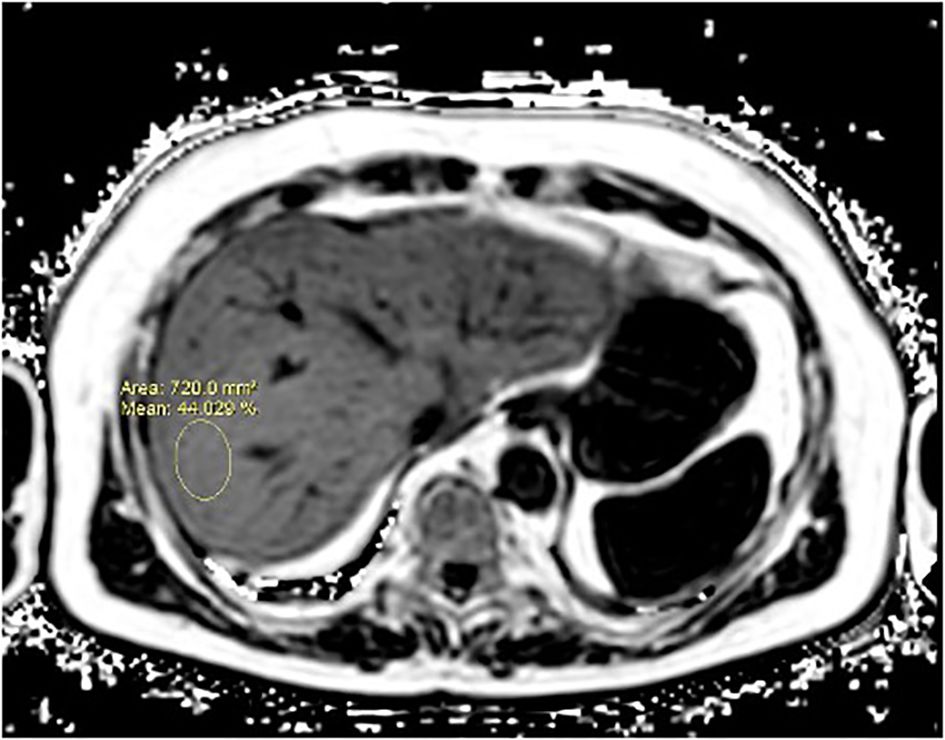
Figure 4. A case of NAFLD evaluated with PDFF. PDFF offers a precise, non-invasive quantification of liver fat content, covering the entire organ and providing consistent, reproducible results. It also correlates well with histopathological findings, making it a reliable alternative to biopsy for comprehensive liver fat evaluation.
The association between the two polymorphism types detected was subsequently evaluated: 33 subjects (28.21%) had a “NULL” genotype for both GST-T1 and GST-M1, 33 (28.21%) had a “NULL” genotype for GST-T1 and “NO NULL” for GST-M1, 19 (16.24%) had a “NO NULL” genotype for GST-T1 and “NULL” for GST-M1, and 35 (29.91%) had a “NO NULL” genotype for both GST-T1 and GST-M1 (Table 2).
Both GST-T1 and GST-M1 “NULL” genotypes show a statistically significant association with the presence of steatosis: for GST-T1, r = 0.258, p = 0.005; for GST-M1, r = 0.260, p = 0.005 (Tables 3A, B). A high statistically significant association between the GST-M1 “NULL” genotype and a higher grade of steatosis (moderate steatosis, 34%–66%) (r = 0.362, p < 0.001) was found. Therefore, subjects with GST-M1 “NULL” have a greater probability of developing not only steatosis but also a higher steatosis grade. On the other hand, no statistically significant association between the presence of the “NULL” GST-T1 genotype and the steatosis grade was found. In fact, the ordinal logistic regression model allowed to highlight that both the GST-M1 “NULL” and the GST-T1 “NULL” genotypes were associated with a greater probability of developing steatosis: in particular, the GST-M1 “NULL” genotype was associated with a greater risk (p = <0.001) of developing a higher grade of steatosis than the GST-T1 “NULL” genotype (p = 0.039) (Figures 5A, B; Table 4).
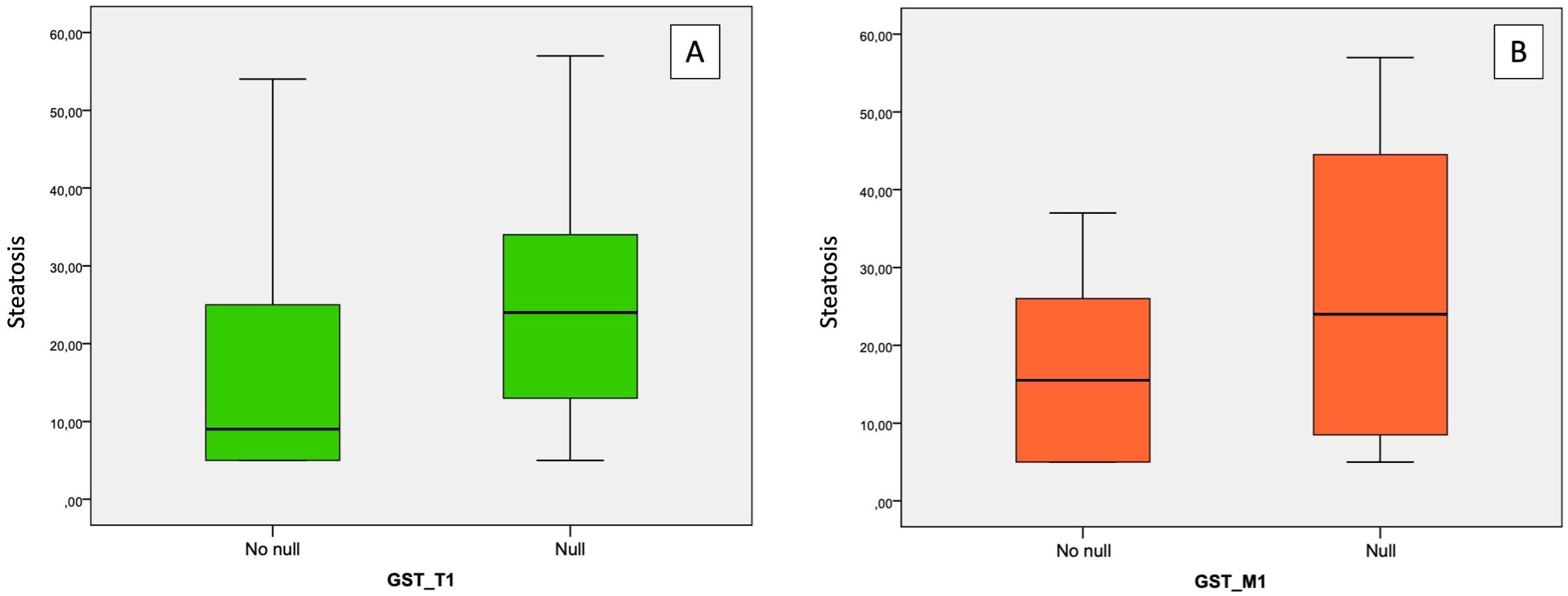
Figure 5. Association between “NULL” and “NO NULL” gene polymorphisms and hepatic steatosis grade. (A) correlation between GST-T1 and steatosis grade; (B) correlation between GST-M1 and steatosis grade. GST-M1, Glutathione S-transferases Mu 1; GST-T1, Glutathione S-Transferase Theta 1.
Age appeared inversely related to the GST-T1 “NULL” genotype (r = −0.216, p = 0.019) (Table 3B); therefore, the risk of developing hepatic steatosis in early age was higher in subjects with the GST-T1 “NULL” genotype (p = 0.019) than in those with the GST-M1 “NULL” genotype (p = 0.073), leading to consider it as a risk factor for the onset of NAFLD. No statistically significant correlations between the “NULL” genotype and sex were detected.
The results are to be considered expected given that the trend of the “NULL” polymorphism does not present any evidence of gender in the relevant literature. The particular distribution of the enzymatic polymorphism is a geographical one.
4 Discussion
NAFLD is considered a complex disease resulting from the interactions between the environment and the different genetic profiles of each individual, which makes them susceptible to develop the disease and to influence its progression. In recent years, we have witnessed multiple genome-wide associations, which have then enriched our knowledge on the genetic basis of NAFLD (6, 20, 21).
Furthermore, more recently, direct evaluation of hepatic fat fraction by MRI led to an estimated heritability of NAFLD in the general population at approximately 52% (22, 23).
In the conducted study, 117 healthy subjects with no risk factors and appropriately selected from the general population using specific questionnaires were enrolled. Genetic analysis was carried out to identify the presence of GST-T1 and GST-M1 “NULL” and “NO NULL” polymorphisms. Subsequent MRI examination to evaluate the grade of steatosis was performed. These analyses were performed to evaluate any correlation between the aforementioned gene polymorphism and NAFLD.
In recent years, in the literature, increasing importance in the possible pathogenesis of NAFLD is attributed to the loss of function of some genes, including those encoding for both phase I and phase II metabolic enzymes, as GST families (24–26). In this perspective, oxidative stress is considered to play a key role in determining liver injury, leading to cell damage and then progression in NAFLD (27). Oxidative stress is due to the production of ROS during metabolic processes: ROS accumulation is usually prevented by the action of antioxidant systems; therefore, their malfunction can lead to its progressive increase (28, 29). At high concentrations, ROS can determine oxidative modifications of cellular macromolecules, such as lipids, resulting in their accumulation into hepatocytes. ROS promotes lipid peroxidation, causing the formation of extremely reactive aldehyde compounds and damage at the intracellular level (30). Thus, one of the mechanisms by which ROS could lead to NAFLD onset and progression may be related to indiscriminate oxidative biomolecular damage although specific molecular pathways are not yet clearly defined (31).
Despite that, various other mechanisms have been reported to cause lipid peroxidation: pro-oxidant systems, such as cytochrome P450, lipoxygenase, and cyclooxygenase, have been solely or synergistically implicated in the emergence of OxS in NAFLD (26).
Since GST enzymes intervene in ROS catabolism and detoxification processes, a reduction in their functionality can determine their increase, potentially predisposing to the onset of NAFLD (26).
MRI is considered a non-invasive method for the evaluation of intrahepatic fat accumulation and then steatosis grade (32, 33).
Steatosis is typically graded on a 0–3 scale based on the number of cells with intracellular vacuoles of fat: grade 0 (normal) = up to 5% of cells affected; grade 1 (mild) = 5% to 33% of cells affected; grade 2 (moderate) = 34% to 66% of cells affected; and grade 3 (severe) = 67% or greater of cells affected (34). MRI exploits the difference in the resonance frequencies between water and fat proton signals. By acquiring the images at echo times in which water and fat signals are approximately in-phase (W+F) and opposed phase (W−F), volumetric liver fat detection is possible according to the relative signal loss on opposed-phase (also known as “out-of-phase”) images (35). Echo times for in-phase and opposed-phase imaging are based on the relative chemical shift between water and the methylene peak (–CH2) of fat (35). At 1.5 T and normal body temperature, this peak resonates approximately at −217 Hz, being slower than water (−434 Hz at 3.0 T) (35).
Unlike CT, where the pixel value directly reflects X-ray attenuation (in Hounsfield units), the signal intensity in MR images is arbitrary and depends on the receiver gain and the sensitivity of received RF coils. Fat signal fraction (η) can be calculated as
where W and F are the signal contributions from water and fat (35).
An attractive approach to create a PDFF map is to use a chemical shift based on the “water–fat separation method” that allows to separate the signal of both water and fat into water-only and fat-only images (36). Both the accurate separation of the signal of fat mobile protons from other mobile protons (i.e., water) and the correction for all those factors that influence MR signal intensity permit the calculation of PDFF: PDFF is defined as the density of hydrogen protons attributable to fat. To provide an accurate estimation of PDFF, the following five confounders must be evaluated: −T1 bias, −T2* decay, −spectral complexity of fat, −noise bias, and −eddy currents (36). After running the fix for the above five confounding factors, the fat-signal fraction and the PDFF are equivalent.
The right fat quantification techniques can be distinguished into two categories:
● “Magnitude-based”: This technique is easier to implement and uses two or more (six echo times) in-phase and opposed-phase images or magnitude images (18, 37); this method provides an estimate of the fat fraction, with a dynamic range of 0%–50% fat signal, which is probably sufficient to estimate the range of liver fat concentrations clinically encountered although it may not apply to all patients.
● “Complex-based”: This technique uses both magnitude and phase information from three or more images (37); this method provides estimates of fat fraction with a dynamic range of 0%–100% (38).
Although it is not possible to identify a threshold that allows to distinguish normal conditions from pathological ones, today, a reference threshold value of the fat fraction of 5.56% is commonly used (39).
The use of MRI as a quantitative biomarker of intracellular liver fat has shown tremendous progress in recent years and holds great promise to provide a cost-effective, accessible, and accurate evaluation of such a widespread disease (40, 41). Furthermore, MRI allows the acquisition of data comparable to those of conventional biopsy; however, it enables the entire liver parenchyma to be visualized, whereas only a small part is taken for histological examination.
In this study, MRI-PDFF has been used to detect the presence of hepatic steatosis in healthy subjects, apparently without any risk factors. Genetic analyses were conducted to identify “NULL” mutations of the GST-T1 and GST-M1 genes, evaluating their possible role in the genesis of NAFLD. As mentioned, these enzymes intervene in the catabolism processes of ROS; therefore, their loss of function (“NULL” genotype) is able to determine an increase in oxidative stress: ROS has been reported to cause lipid peroxidation, predisposing to the onset of NAFLD itself (41).
Four groups of cytological GSTs have been identified in humans, classified with the Greek letters alpha (α), mu (µ), pi (π), and theta (θ), and defined based on their isoelectric point (40). Four gene sequences were investigated for these enzymes: GSTA (GSTα), located on chromosome 6; GSTM (GSTµ), located on chromosome 1; GSTP (GSTπ), located on chromosome 11; and GSTT (GSTθ), located on chromosome 22. Several studies have described the specific structure, enzymology, and affinity of the tissue and gender of GST-M and GST-T polymorphisms (42). The enzymatic chains encoded by GST-M1 polymorphism are expressed by hepatic, gastric, and nervous systems, while the enzymatic chains encoded by GST-M2 and GST-M5 polymorphisms are expressed by extrahepatic tissues and synthesized by other cell lines (43).
The genetic polymorphisms GST-T1 and GST-T2 are located in the same region on chromosome 22, and the related enzyme chains are mainly expressed in the liver (44).
The genetic polymorphism GST-M1 can be typed by highlighting three allelic forms: GST-M1*0, GST-M1*A, and GST-M1*B. The GST-M1*0 allele (homozygous null) indicates a total deletion of the gene tract with a consequent lack of expression, at the cellular level, of the related enzyme chains (44). Alleles GST-M1*A and GST-M1*B differ from each other for a single base at the level of exon 7 and encode for protein monomers that constitute the functionally active isoform of the enzyme. It is also important to highlight that approximately 50% of the Caucasian population does not express the enzyme in relation to the gene deletion which, in homozygosity, gives rise to the GST-M1*0/*0 genotype (45). Another significant deletion is expressed at the level of the GST-T1 polymorphism; the null genotype of GST-T1 is expressed in approximately 15% of the Caucasian population and in approximately 60% of the Chinese and Korean populations (46).
According to the role played by these enzymes in the biotransformation of endogenous and exogenous toxicants, including carcinogens (for example, polycyclic aromatic hydrocarbons, PAHs), pollutants, alcohol, drugs, and other xenobiotic agents, it is probable that they play an important role even on the onset of pathologies widely spread in the general population, such as NAFLD (45, 46). The main mechanism appears to be related to the increase in oxidative stress and its consequences (46).
The “NULL” GST-T1 polymorphism has been associated with higher TNF-α concentration when compared with the “NO NULL” polymorphism; thus, it could indicate activation of the proinflammatory segment of the cytokine profile and inflammatory processes (47). Noteworthily, TNF-α is considered to play a pivotal role in those mechanisms leading to IR, inflammation, and apoptosis in the case of NAFLD; thus, its elevated level has been considered a predictive factor of NAFLD progression (48). Alterations in the adipokine profile were instead detected in subjects with the GST-M1 “NULL” genotype: leptin plasma levels were significantly higher than in patients with the “NO NULL” genotype. The high leptin level could be related to a high TNF-α concentration, which is capable of stimulating leptin production (49).
Prysyazhnyuk et al. (50) also documented a reduction of restored glutathione in patients with NAFLD and GST-T1 and GST-M1 gene “NULL” polymorphisms. A higher level of reaction products of thiobarbituric acid has also been detected in subjects with the zero genotype of the GST-M1 gene than in those with the functional allele of the gene. Furthermore, Kassab et al. (51) claimed that the “NULL” genotypes of GST-T1 and GST-M1 genes could determine an activity reduction of sulfhydryl binding and then a loss of detoxification body capacity. Despite this, some authors maintained that the presence of the “NULL” polymorphism alone cannot determine a relevant lack of GST isoenzyme synthesis to determine a greater susceptibility to genetic damage (52). In the literature, several studies have evaluated the different distribution of GST-T1 and GST-M1 polymorphisms between subjects with NAFLD and the rest of the general population. Hori et al. (53) conducted a study involving the Japanese population, reporting a higher frequency of the GST-M1 null genotype in NAFLD patients as compared to the control group. Similar results were obtained in the study conducted by Kordi-Tamandani et al. (54). On the other hand, Hori et al. (53) found liver function alterations in the presence of “NULL” GST-T1, GST-P1, and GST-M1 polymorphisms, with the synthesis, detoxification, and excretion parameters altered. No alterations in the lipid profile were found either (53). Different data have been obtained by Maciel et al. (55), who found hypertriglyceridemia in the presence of the aforementioned “NULL” polymorphisms. Furthermore, in agreement with the study of Hori et al., Prysyazhnyuk et al. (56) in a study conducted in Ukraine found a higher prevalence of “NULL” polymorphisms among NAFLD patients than in healthy individuals. Zhu et al. (40) conducted six studies including 700 NAFLD patients and 1,317 controls, aiming at identifying the frequency of GST-M1 gene polymorphism in subjects with NAFLD: it was revealed that GST-M1 is appreciably connected with NAFLD. Similar results have been obtained for GST-T1 “NULL” polymorphism distribution: five studies involving 620 NAFLD and 1,237 healthy subjects found a noticeable association between the SNP of GST-T1 and NAFLD vulnerability. Therefore, these data suggest a significant correlation between GST-M1/T1 “NULL” polymorphisms and the onset of NAFLD. These data are in agreement with the results of our study. Both GST-M1 and GST-T1 showed a higher prevalence in subjects with NAFLD although GST-M1 showed a greater strength of association. Furthermore, the GST-M1 NULL genotype is related to the onset of a higher grade of steatosis. Damavandi et al. (12) analyzed 242 NAFLD patients and 324 healthy controls in the Iranian population, reporting a higher prevalence of the GST-M1 and GST-T1 “NULL” genotypes in the first group. Subjects with those polymorphisms were then considered at a higher risk of developing NAFLD. Another study conducted by Hashemi et al. (6) in the Iranian population on 83 patients with NAFLD and 93 healthy subjects confirmed an increased risk of developing NAFLD in subjects with the GST-M1 “NULL” genotype. On the other hand, the GST-T1 “NULL” polymorphism distribution was not significantly different between NAFLD and control groups. Therefore, this study showed that GST-M1, but not GST-T1, can be considered a risk factor for developing NAFLD. These data disagree with what has been obtained in our study: in fact, although a stronger statistical association was identified between NAFLD and “NULL” GST-M1 polymorphism, also the “NULL” GST-T1 genotype has a statistically significant correlation. Therefore, it could also be considered an NAFLD genetic predisposing factor. The main data are summarized in Table 5.
Furthermore, the correlation between GST-T1 and GST-M1 “NULL” polymorphisms and the etiopathogenesis of hepatic steatosis can be considered a challenge for forensic genetic laboratories since screening assumes the significance of biomarker of individual susceptibility in genetic predispositions to metabolic diseases especially in those subjects professionally exposed to xenobiotic substances. Genetic analysis could help us to understand some pathological findings in forensic cases in ascertaining the cause of steatosis even in the absence of specific dietary and/or environmental risk factors. Further forensic applications include the study of polymorphisms in association with liver function for those cases in which it is necessary to interpret the degree of drug biotransformation in postmortem toxicological investigations as a contribution to molecular autopsy. Therefore, the identification of genetic biomarkers linked to hepatic steatosis can support more accurate forensic and toxicological evaluations. The biomolecular interest in identifying the causes of hepatic steatosis cannot be overlooked, as this is a pathology of significant social interest for the resulting social security and tertiary protection aspects. Based on what has been described, the analysis of the correlation between the GST-M1 and GST-T1 NULL genotypes and hepatic steatosis would seem promising, and there are several future perspectives related to this area of research: i) the analysis of the role of both oxidative stress and lipid metabolism, ii) the interactions with environmental factors and other polymorphisms, iii) the potential role of GST NULL genotypes as risk factors and early biomarkers for the identification of hepatic steatosis, iv) the possibility of developing tailored prevention interventions based on genetic risk and thus identifying paths for the development of new strategies, and v) reconsidering social security and public protection strategies and the allocation of resources are certainly among the most relevant.
4.1 Limitations and risk of bias
Despite the analyses performed, some possible limitations of the study must be reported. Although the enrolled subjects have a BMI within the limits of normality and declare they are not affected by any pathology, the blood analyses only allow to exclude dysmetabolic pathologies (diabetes mellitus, dyslipidemia, etc.), but it was not possible to ascertain the real absence of other pathologies. In addition, the information regarding eating habits generally refers to the number of meals during the day, the consumption of fruits and vegetables, the quantity of alcohol consumed daily, etc. Therefore, for instance, no specific information has been acquired on the medium daily caloric intake.
5 Conclusion
In conclusion, the study conducted by this research group aimed to demonstrate a correlation between the presence of the GST-T1 and GST-M1 “NULL” genotypes and the presence of steatosis. Thanks to the analysis of the collected data, it was possible to identify a statistically significant correlation between the presence of the GST-T1 and GST-M1 “NULL” genotypes and the presence of hepatic steatosis, which is higher for GST-M1 “NULL.” Furthermore, a high statistically significant association between the GST-M1 “NULL” genotype and a higher grade of steatosis was found. In contrast, no statistically significant association between the GST-T1 “NULL” genotype and steatosis grade was identified. It was also possible to underline the importance of MRI-estimated PDFF in the diagnosis of hepatic steatosis: this method allows the acquisition of data comparable to those of conventional biopsy; however, it permits the entire liver parenchyma to be visualized and only a small part is taken for histological examination. Despite this, considering both the stringent exclusion criteria that led to the healthy subjects without risk factors enrolled and the small number of the population analyzed, further studies are required to confirm the data obtained.
Data availability statement
The original contributions presented in the study are included in the article/supplementary material, further inquiries can be directed to the corresponding authors.
Ethics statement
Ethical approval was not required for the studies involving humans because the enrolled sample consists of 117 healthy subjects who voluntarily underwent screening by signing an informed consent. The identity of each subject remained anonymous by assigning a numerical ID. Therefore, neither approval from the ethics committee nor ethical approval is necessary. The studies were conducted in accordance with the local legislation and institutional requirements. The participants provided their written informed consent to participate in this study.
Author contributions
VC: Conceptualization, Data curation, Methodology, Supervision, Writing – original draft. CM: Data curation, Investigation, Writing – original draft. GB: Investigation, Writing – original draft. GS: Data curation, Supervision, Writing – review & editing. AAl: Formal analysis, Methodology, Writing – original draft. ACi: Conceptualization, Writing – original draft. ACr: Formal analysis, Writing – original draft. PG: Writing – review & editing. AAs: Writing – review & editing. MG: Investigation, Writing – review & editing. CG: Writing – original draft. DS: Formal analysis, Methodology, Supervision, Writing – original draft.
Funding
The author(s) declare that no financial support was received for the research, authorship, and/or publication of this article.
Conflict of interest
The authors declare that the research was conducted in the absence of any commercial or financial relationships that could be construed as a potential conflict of interest.
Publisher’s note
All claims expressed in this article are solely those of the authors and do not necessarily represent those of their affiliated organizations, or those of the publisher, the editors and the reviewers. Any product that may be evaluated in this article, or claim that may be made by its manufacturer, is not guaranteed or endorsed by the publisher.
References
1. Ray K. NAFLD-the next global epidemic. Nat Rev Gastroenterol Hepatol. (2013) 10:621. doi: 10.1038/nrgastro.2013.197
2. Riazi K, Azhari H, Charette JH, Underwood FE, King JA, Afshar EE, et al. The prevalence and incidence of NAFLD worldwide: a systematic review and meta-analysis. Lancet Gastroenterol Hepatol. (2022) 7:851–61. doi: 10.1016/S2468-1253(22)00165-0
3. Yoshiji H, Nagoshi S, Akahane T, Asaoka Y, Ueno Y, Ogawa K, et al. Evidence-based clinical practice guidelines for Liver Cirrhosis. J Gastroenterol. (2021) 56:593–619. doi: 10.1007/s00535-021-01788-x
4. Brunt EM, Wong VW, Nobili V, Day CP, Sookoian S, Maher JJ, et al. Nonalcoholic fatty liver disease. Nat Rev Dis Primers. (2015) 1:15080. doi: 10.1038/nrdp.2015.80
5. Eslam M, Valenti L, Romeo S. Genetics and epigenetics of NAFLD and NASH: Clinical impact. J Hepatol. (2018) 68:268–79. doi: 10.1155/2020/6638306
6. Hashemi M, Eskandari-Nasab E, Fazaeli A, Bahari A, Hashemzehi NA, Shafieipour S, et al. Association of genetic polymorphisms of glutathione-S-transferase genes (GSTT1, GSTM1, and GSTP1) and susceptibility to nonalcoholic fatty liver disease in Zahedan, Southeast Iran. DNA Cell Biol. (2012) 31:672–7. doi: 10.1089/dna.2011.1343
7. Romeo S, Kozlitina J, Xing C, Pertsemlidis A, Cox D, Pennacchio LA, et al. Genetic variation in PNPLA3 confers susceptibility to nonalcoholic fatty liver disease. Nat Genet. (2008) 40:1461–5. doi: 10.1038/ng.257
8. Juanola O, Martínez-López S, Francés R, Gómez-Hurtado I. Non-alcoholic fatty liver disease: metabolic, genetic, epigenetic and environmental risk factors. Int J Environ Res Public Health. (2021) 18:5227. doi: 10.3390/ijerph18105227
9. Trépo E, Valenti L. Update on NAFLD genetics: From new variants to the clinic. J Hepatol. (2020) 72:1196–209. doi: 10.1016/j.jhep.2020.02.020
10. Sapienza D, Asmundo A, Gualniera P, Sole G, Bambara M, Spatari G. Polimorfismi genetici (GSTT1 e GSTM1) ed escrezione urinaria di acido t,t-muconico in lavoratori di una raffineria [Genetic polymorphisms (GSTT1 e GSTM1) and urinary excretion of t,t-muconic acid among refinery workers. G Ital Med Lav Ergon. (2007) 29:541–2.
11. Carrieri M, Spatari G, Tranfo G, Sapienza D, Scapellato ML, Bartolucci GB, et al. Biological monitoring of low level exposure to benzene in an oil refinery: Effect of modulating factors. Toxicol Lett. (2018) 298:70–5. doi: 10.1016/j.toxlet.2018.08.001
12. Damavandi N, Zeinali S. Association of xenobiotic-metabolizing enzymes (GSTM1 and GSTT 1), and pro-inflammatory cytokines (TNF-α and IL-6) genetic polymorphisms with non-alcoholic fatty liver disease. Mol Biol Rep. (2021) 48:1225–123. doi: 10.1007/s11033-021-06142-1
13. Rinella ME, Lazarus JV, Ratziu V, Francque SM, Sanyal AJ, Kanwal F, et al. A multisociety Delphi consensus statement on new fatty liver disease nomenclature. ” J Hepatol. (2023) 79:1542–56. doi: 10.1016/j.jhep.2023.06.003
14. Bolt HM, Thier R. Relevance of the deletion polymorphisms of the glutathione S-transferases GSTT1 and GSTM1 in pharmacology and toxicology. Curr Drug Metab. (2006) 7:613–28. doi: 10.2174/138920006778017786
15. Ghobadloo SM, Yaghmaei B, Bakayev V, Goudarzi H, Noorinayer B, Rad FH, et al. GSTP1, GSTM1, and GSTT1 genetic polymorphisms in patients with cryptogenic liver cirrhosis. J Gastrointest Surg. (2004) 8:423–7. doi: 10.1016/j.gassur.2004.02.005
16. Pavanello S, Simioli P, Lupi S, Gregorio P, Clonfero E. Exposure levels and cytochrome P450 1A2 activity, but not N-acetyltransferase, glutathione S-transferase (GST) M1 and T1, influence urinary mutagen excretion in smokers. Cancer Epidemiol Biomarkers Prev. (2002) 11:998–1003.
17. Springer F, Machann J, Claussen CD, Schick F, Schwenzer NF. Liver fat content determined by magnetic resonance imaging and spectroscopy. World J Gastroenterol. (2010) 16:1560–6. doi: 10.3748/wjg.v16.i13.1560
18. Zand KA, Shah A, Heba E, Wolfson T, Hamilton G, Lam J, et al. Accuracy of multiecho magnitude-based MRI (M-MRI) for estimation of hepatic proton density fat fraction (PDFF) in children. J Magn Reson Imaging. (2015) 42:1223–32. doi: 10.1002/jmri.24888
19. Kim JW, Lee CH, Yang Z, Kim BH, Lee YS, Kim KA. The spectrum of magnetic resonance imaging proton density fat fraction (MRI-PDFF), magnetic resonance spectroscopy (MRS), and two different histopathologic methods (artificial intelligence vs. pathologist) in quantifying hepatic steatosis. Quant Imaging Med Surg. (2022) 12:5251–62. doi: 10.21037/qims-22-393
20. Rossini A, Rapozo DC, Amorim LM, Macedo JM, Medina R, Neto JF, et al. Frequencies of GSTM1, GSTT1, and GSTP1 polymorphisms in a Brazilian population. Genet Mol Res. (2002) 1:233–40.
21. Powell EE, Wong VW, Rinella M. Non-alcoholic fatty liver disease. Lancet. (2021) 397:2212–24. doi: 10.1016/S0140-6736(20)32511-3
22. Loomba R, Schork N, Chen CH, Bettencourt R, Bhatt A, Ang B, et al. Genetics of NAFLD in twins consortium. Heritability of hepatic fibrosis and steatosis based on a prospective twin study. Gastroenterology. (2015) 149:1784–93. doi: 10.1053/j.gastro.2015.08.011
23. Leung H, Long X, Ni Y, Qian L, Nychas E, Siliceo SL, et al. Risk assessment with gut microbiome and metabolite markers in NAFLD development. Sci Transl Med. (2022) 14:eabk0855. doi: 10.1126/scitranslmed.abk0855
24. Duval C, Teixeira-Clerc F, Leblanc AF, Touch S, Emond C, Guerre-Millo M, et al. Chronic exposure to low doses of dioxin promotes liver fibrosis development in the C57BL/6J diet-induced obesity mouse model. Environ Health Perspect. (2017) 125:428–36. doi: 10.1289/EHP316
25. Cave MC, Clair HB, Hardesty JE, Falkner KC, Feng W, Clark BJ, et al. Nuclear receptors and nonalcoholic fatty liver disease. Biochim Biophys Acta. (2016) 1859:1083–99. doi: 10.1016/j.bbagrm.2016.03.002
26. Chen Z, Tian R, She Z, Cai J, Li H. Role of oxidative stress in the pathogenesis of nonalcoholic fatty liver disease. Free Radic Biol Med. (2020) 152:116–41. doi: 10.1016/j.freeradbiomed.2020.02.025
27. Friedman SL, Neuschwander-Tetri BA, Rinella M, Sanyal AJ. Mechanisms of NAFLD development and therapeutic strategies. Nat Med. (2018) 24:908–22. doi: 10.1038/s41591-018-0104-9
28. Takaki A, Kawai D, Yamamoto K. Multiple hits, including oxidative stress, as pathogenesis and treatment target in non-alcoholic steatohepatitis (NASH). Int J Mol Sci. (2013) 14:20704–28. doi: 10.3390/ijms141020704
29. Masarone M, Rosato V, Dallio M, Gravina AG, Aglitti A, Loguercio C, et al. Role of oxidative stress in pathophysiology of nonalcoholic fatty liver disease. Oxid Med Cell Longev. (2018) 2018:9547613. doi: 10.1155/2018/9547613
30. Rolo AP, Teodoro JS, Palmeira CM. Role of oxidative stress in the pathogenesis of nonalcoholic steatohepatitis. Free Radic Biol Med. (2012) 52:59–69. doi: 10.1016/j.freeradbiomed.2011.10.003
31. Mansouri A, Gattolliat CH, Asselah T. Mitochondrial dysfunction and signaling in chronic liver diseases. Gastroenterology. (2018) 155:629–47. doi: 10.1053/j.gastro.2018.06.083
32. Ajmera V, Loomba R. Imaging biomarkers of NAFLD, NASH, and fibrosis. Mol Metab. (2021) 50:101167. doi: 10.1016/j.molmet.2021.101167
33. Ferraioli G, Soares Monteiro LB. Ultrasound-based techniques for the diagnosis of liver steatosis. World J Gastroenterol. (2019) 25:6053–62. doi: 10.3748/wjg.v25.i40.6053
34. Mamidipalli A, Fowler KJ, Hamilton G, Wolfson T, Covarrubias Y, Tran C, et al. Prospective comparison of longitudinal change in hepatic proton density fat fraction (PDFF) estimated by magnitude-based MRI (MRI-M) and complex-based MRI (MRI-C). Eur Radiol. (2020) 30:5120–9. doi: 10.1007/s00330-020-06858-x
35. Yu H, Shimakawa A, Hines CD, McKenzie CA, Hamilton G, Sirlin CB, et al. Combination of complex-based and magnitude-based multiecho water-fat separation for accurate quantification of fat-fraction. Magn Reson Med. (2011) 66:199–206. doi: 10.1002/mrm.22840
36. Hui SCN, So HK, Chan DFY, Wong SKH, Yeung DKW, Ng EKW, et al. Validation of water-fat MRI and proton MRS in assessment of hepatic fat and the heterogeneous distribution of hepatic fat and iron in subjects with non-alcoholic fatty liver disease. Eur J Radiol. (2018) 107:7–13. doi: 10.1016/j.ejrad.2018.08.008
37. Eddowes PJ, McDonald N, Davies N, Semple SIK, Kendall TJ, Hodson J, et al. Utility and cost evaluation of multiparametric magnetic resonance imaging for the assessment of non-alcoholic fatty liver disease. Aliment Pharmacol Ther. (2018) 47:631–44. doi: 10.1111/apt.14469
38. Sen P, Qadri S, Luukkonen PK, Ragnarsdottir O, McGlinchey A, Jäntti S, et al. Exposure to environmental contaminants is associated with altered hepatic lipid metabolism in non-alcoholic fatty liver disease. J Hepatol. (2022) 76:283–93. doi: 10.1016/j.jhep.2021.09.039
39. Prysyazhnyuk V, Sydorchuk L, Sydorchuk R, Prysiazhniuk I, Bobkovych K, Buzdugan I, et al. Glutathione-S-transferases genes-promising predictors of hepatic dysfunction. World J Hepatol. (2021) 13:620–33. doi: 10.4254/wjh.v13.i6.620
40. Zhu Y, Yang JH, Hu JP, Qiao M. Association of glutathione S-transferases (GSTT1, GSTM1 and GSTP1) genes polymorphisms with nonalcoholic fatty liver disease susceptibility: A PRISMA-compliant systematic review and meta-analysis. Med (Baltimore). (2022) 101:e30803. doi: 10.1097/MD.0000000000030803
41. Uno Y, Murayama N, Kato M, Tanaka S, Ohkoshi T, Yamazaki H. Genetic variants of glutathione S-transferase GSTT1 and GSTT2 in cynomolgus macaques: identification of GSTT substrates and functionally relevant alleles. Chem Res Toxicol. (2018) 31:1086–91. doi: 10.1021/acs.chemrestox.8b00198
42. Chbili C, Fathallah N, Laadhari C, Ouni B, Saguem S, Ben Fredj M, et al. Glutathione-S-transferase genetic polymorphism and risk of hepatotoxicity to antitubercular drugs in a North-African population: A case-control study. Gene. (2022) 809:146019. doi: 10.1016/j.gene.2021.146019
43. Tan KL, Webb GC, Baker RT, Board PG. Molecular cloning of a cDNA and chromosomal localization of a human theta-class glutathione S-transferase gene (GSTT2) to chromosome 22. Genomics. (1995) 25:381–7. doi: 10.1016/0888-7543(95)80037-m
44. Idilman IS, Ozdeniz I, Karcaaltincaba M. Hepatic steatosis: etiology, patterns, and quantification. Semin Ultrasound CT MR. (2016) 37:501–10. doi: 10.1053/j.sult.2016.08.003
45. Dioguardi Burgio M, Ronot M, Reizine E, Rautou PE, Castera L, Paradis V, et al. Quantification of hepatic steatosis with ultrasound: promising role of attenuation imaging coefficient in a biopsy-proven cohort. Eur Radiol. (2020) 30:2293–301. doi: 10.1007/s00330-019-06480-6
46. Reeder SB, Robson PM, Yu H, Shimakawa A, Hines CD, McKenzie CA, et al. Quantification of hepatic steatosis with MRI: the effects of accurate fat spectral modeling. J Magn Reson Imaging. (2009) 29:1332–9. doi: 10.1002/jmri.21751
47. Prysyazhnyuk V, Voloshyn O, Prysiazhniuk I, Ilashchuk T, Sydorchuk L, Prysyazhnyuk P. Glutathione S-transferase T1 and M1 null genotype distribution among non-alcoholic fatty liver disease patients and its association with cytokine and adipokine profiles. Clin Exp Hepatol. (2020) 6:142–9. doi: 10.5114/ceh.2020.95678
48. Polyzos SA, Kountouras J, Zavos Ch. The multi-hit process and the antagonistic roles of tumor necrosis factor-alpha and adiponectin in non alcoholic fatty liver disease. Hippokratia. (2009) 13:127–8.
49. Paniagua JA. Nutrition, insulin resistance and dysfunctional adipose tissue determine the different components of metabolic syndrome. World J Diab. (2016) 7:483–514. doi: 10.4239/wjd.v7.i19.483
50. Prysyazhnyuk VP, Sydorchuk LP. The relationship between GSTT1 and GSTM1 deletion gene polymorphisms with biochemical blood parameters, indicators of pro- and antioxidant systems in patients with nonalcoholic fatty liver disease. Gastro. (2015) 5:40–7.
51. Kassab A, Msolly A, Lakhdar R, Gharbi O, Miled A. Polymorphisms of glutathi- one-S-transferases M1, T1, P1 and susceptibility to colorectal cancer in a sample of the Tunisian population. Med Oncol. (2013) 31:1–6. doi: 10.1007/s12032-013-0760-z
52. Ghorbel R, Ben Salah G, Ghorbel R, Ben Mahmoud A, Chamkha I, Mkaouar-Rebai E, et al. Do GSTM1 and GSTT1 polymorphisms infuence the risk of developing mitochondrial diseases in a Tunisian population. Environ Sci pollut Res. (2018) 25:1–9. doi: 10.1007/s11356-017-0775-7
53. Hori M, Oniki K, Nakagawa T, Takata K, Mihara S, MArubayashi T, et al. Association between combinations of glutathione-S-transferase M1, T1 and P1 genotypes and non-alcoholic fatty liver disease. Liver Int. (2009) 29:164–8. doi: 10.1111/j.1478-3231.2008.01794.x
54. Kordi-Tamandani DM, Hashemi M, Birjandian E, Bahari A, Valizadeh J, Torkamanzehi A. Lack of association of GSTT1 and GSTP1 genes methylation and their expression profiles with risk of NAFLD in a sample of Iranian patients. Clin Res Hepatol Gas. (2011) 35:387–92. doi: 10.1016/j.clinre.2011.01.015
55. Maciel SS, Pereira Ada C, Silva GJ, Rodrigues MV, Mill JG, Krieger JE. Association between glutathione S-transferase polymorphisms and triglycerides and HDL-cholesterol. Atherosclerosis. (2009) 206:204–8. doi: 10.1016/j.atherosclerosis.2009.02.011
56. Prysyazhnyuk VP, Rossokha ZI, Gorovenko NG. Variation in particular biochemical indicators, cytokine and adipokine profiles of the blood, and the structural and functional parameters of the liver in patients with nonalcoholic fatty liver disease and different genotypes by the polymorphic locus A313G of the GSTP1 gene. Cytol Genet. (2017) 6:50–7. doi: 10.3103/S0095452717060111
Keywords: steatosis, NAFLD, GSTs, oxidative stress, magnetic resonance imaging, PDFF, forensic genetics, environmental exposure
Citation: Cianci V, Mondello C, Baldino G, Spatari G, Alibrandi A, Cianci A, Cracò A, Gualniera P, Asmundo A, Gaeta M, Giorgianni C and Sapienza D (2024) Potential contribution of GST-T1 and GST-M1 polymorphisms in the onset of hepatic steatosis: from radiological to molecular and medico-legal analyses. Front. Gastroenterol. 3:1393282. doi: 10.3389/fgstr.2024.1393282
Received: 11 March 2024; Accepted: 28 August 2024;
Published: 20 September 2024.
Edited by:
Rasheed Ahmad, Dasman Diabetes Institute, KuwaitReviewed by:
Francesco Sessa, University of Catania, ItalyAngelo Montana, University of Catania, Italy
Copyright © 2024 Cianci, Mondello, Baldino, Spatari, Alibrandi, Cianci, Cracò, Gualniera, Asmundo, Gaeta, Giorgianni and Sapienza. This is an open-access article distributed under the terms of the Creative Commons Attribution License (CC BY). The use, distribution or reproduction in other forums is permitted, provided the original author(s) and the copyright owner(s) are credited and that the original publication in this journal is cited, in accordance with accepted academic practice. No use, distribution or reproduction is permitted which does not comply with these terms.
*Correspondence: Vincenzo Cianci, ZW56b2NpYW5jaS4xOTk3QGdtYWlsLmNvbQ==; Daniela Sapienza, ZGFuaWVsYS5zYXBpZW56YUB1bmltZS5pdA==
†These authors have contributed equally to this work and share last authorship