- Department of Biology, College of Natural and Computational Science, Mekdela Amba University, Tuluawlia, Ethiopia
Antimicrobial agents have significant effects on the ecological balance of the human microbiota through incomplete absorption (e.g., orally administered antimicrobial agents) or secretion (e.g., by the salivary glands, in the bile, or from the intestinal mucosa) of the agents. This study aimed to examine the effects of novel antimicrobial agents on the normal functioning of the intestinal microbiota. The articles, written in English, were recovered from PubMed, ScienceDirect, Web of Science, Google Scholar, and DOAJ, as well as from manual searches using a reference list. “Microbiota”, “Intestinal Microbiota”, “Eubiotic Microbiota”, “Ecological Impact”, “Antimicrobial Agents,”, “Antibiotics”, “Dysbiosis”, “Gut Microbiota”, and “Probiotics” were the search terms used to retrieve the articles. The PRISMA 2009 checklist was applied for article search strategy, article selection, data extraction, and result reporting for the review process. A total of eight original research articles were included from a total of 379 articles obtained in different search strategies. The eight new antimicrobial agents demonstrated significant impacts on the ecological balance of the human intestinal microbiota. Therefore, eubiosis is crucial in preventing the establishment of exogenous antimicrobial-resistant strains as well as their gene transfer.
Systematic review registration: [PRISMA], identifier [2009].
1 Introduction
Under normal conditions, the normal human microbiota is relatively stable in each ecological habitat and provides a myriad of benefits to the host’s health. The normal microbiota acts as a barrier against colonization by potentially pathogenic microorganisms as well as the overgrowth of already present microorganisms such as yeasts (e.g., Candida spp.) or Clostridioides difficile in the intestinal tract through competition for nutrients and adhesion sites, and by producing metabolites [e.g., short-chain fatty acids (SCFAs)] and bacteriocins. Controlling the growth of opportunistic microorganisms is known as “colonization resistance,” and it is maintained not only by normal microbiota but also by several anatomical and physiological factors such as peristalsis of the intestinal tract and the secretion of saliva, sweat, and gastric and bile acids (1–3).
Delivery methods, age, miRNAs, the host’s intestinal secretory function, geographical variation, food and diet habits, physical stress, disease, and antibiotics can affect the normal microbiota composition and result in disruptions of the human microbiota’s normal function (4–14). The frequent use of antimicrobial drugs can have several negative effects on the normal microbiota found in different parts of the body. For example, the length and frequency of antibiotic use increase the chance of infection by C. difficile by causing the loss of specific microbial populations from the microbiota, which deregulates the production of antimicrobial peptides (e.g., bacteriocins) or metabolites against pathogen colonization (15). The emergence of resistance among bacteria in the normal microbiota and the distribution of resistant genes by vertical or horizontal (conjugation, transformation, or transduction) gene transfer in the microbial community can contribute to increased loads of pathogenic and drug-resistant microbes. Furthermore, disturbed normal microbiota reduces colonization resistance, resulting in an overgrowth of already present microorganisms or exogenous pathogens (16, 17).
Administration of antimicrobial drugs significantly disturb the ecological equilibrium of the normal microbiota located within the human body. The spectrum of the drug, the dose, the method of administration, the pharmacokinetic and pharmacodynamic characteristics, and the in vivo inactivation of the agent determine the extent of disturbance of antimicrobial agents in the normal human microbiota. The intestinal microbiota can be affected by the incomplete absorption of oral medications and the secretion of an antimicrobial agent by the vaginal or intestinal mucosa, bile, salivary glands, or eccrine or apocrine sweat glands. As a result, antibiotic-resistant microbiota and their genes could consequently become more prevalent in different parts of the body (17, 18).
Antimicrobial resistance is a major global public health problem that poses a challenge to the treatment of diseases such as skin and soft tissue infections, C. difficile infection (CDI), endocarditis, meningitis, pneumonia, tuberculosis, malaria, and AIDS (19–24) due to the use of broad- or narrow-spectrum and ecologically non-favorable agents. Since, changes in the composition of the microbiota (through loss of diversity or loss of specific taxonomic groups) due to therapeutic agents, result in microbial imbalances with increased susceptibility to different conditions and comorbidities, i.e., gastrointestinal (GI) infections, diabetes, obesity, liver disease, colon cancer, and inflammatory bowel disease (IBD). Therefore, during prescription, it is crucial that clinicians understand how antimicrobial drugs affect the ecology of the human microbiota. Furthermore, to mitigate this problem, novel antimicrobial agents with activity against drug-resistant pathogens are urgently needed due to the rise in resistance in many Gram-positive and Gram-negative pathogenic microbes, such as methicillin-resistant Staphylococcus aureus (MRSA), pan-drug-resistant Gram-negative bacteria, carbapenem-resistant Enterobacteriaceae (CRE), extended-spectrum beta-lactamase-producing Enterobacteriaceae (ESBL-E) (25), and other extreme drug-resistant or pan-drug-resistant organisms such as Mycobacterium tuberculosis, vancomycin-resistant Enterococci (VRE), highly infectious and fluoroquinolone-resistant C. difficile, multidrug-resistant Streptococcus pneumoniae, and Neisseria gonorrhoeae (26–32). Using probiotics is another significant alternative to minimize antibiotic-associated complications and comorbidities.
2 Methodology
Several research articles concerning the effects of novel antimicrobials on intestinal microbiota were extensively searched and collected from different databases. Many published articles were available separately, and a detailed review was essential to combine all results to draw a conclusion and avoid any information conflicts, ambiguities, or misunderstandings. The review, which aimed to highlight the type of novel agents, the dose, drug administration, the number of subjects, the drug’s impact on human intestinal microbiota, and the means of drug elimination, was conducted according to systematic reviews, as recommended by Moher et al. (33) The PRISMA 2009 (i.e., Preferred Reporting Items for Systematic Reviews and Meta-Analyses) guidelines and checklist were strictly followed to document this review.
2.1 Formulation of research questions and problems
This systematic review was guided by the following question: “What are the ecological impacts of novel antimicrobial agents on the human intestinal microbiota?” The problem was formulated while searching and assessing the impacts of antimicrobial agents on human health. Due to their diverse impacts, the study focused on examining the impact of new antimicrobials on the intestinal microbiota. This question created a further interest in examining whether novel antimicrobial agents simultaneously affect the resident microbiota and human health or if these agents alternatively affect the transient microbiota.
2.2 Search engine for research articles
An extensive search of research articles was conducted in international electronic databases [PubMed, ScienceDirect, Web of Science, Google Scholar, and Directory of Open Access Journals (DOAJ)] and other sources (manual search using a reference list). The articles were searched using the following key terms and phrases taken from the title, abstract, and keywords in combination or separately using Boolean operators (“OR” or “AND”): “Microbiota”, “Intestinal Microbiota”, “Eubiotic Microbiota”, “Ecological Impact”, “Antimicrobial agents”, “Antibiotics”, “Dysbiosis”, “Gut Microbiota”, and “Probiotics”. The study was carried out from October 2022 to January 2023. The search process was presented in accordance with the PRISMA 2009 flow diagram (33) guidelines, together with the included and excluded items and reasons for exclusion (Figure 1).
2.3 Inclusion and exclusion criteria for included studies
In this systematic review, different studies conducted around the world were included. Articles collected through the searches were evaluated for inclusion in the systematic review based on the following criteria: (i) original articles on novel antimicrobial agents that address their impact on the ecological balance of the human intestinal microbiota; (ii) clinical studies; (iii) human studies only; (iv) only studies reported in English; (v) recent journals studied from 2006 to 2014; (vi) only intestinal microbiota; and (vii) articles published and available online. However, reports on the impacts of antimicrobials on other experimental animals, other microbiota, non-peer-reviewed articles, in vitro studies, other non-pharmaceutical agents, studies not published in English, review papers (comprehensive, scoping, systematic, meta-analysis, or other forms of review), duplicate publications (articles published in two journals with the same title, same first author, same study design, same sample size, and the same number of in-text citations or references), or extensions of analysis from original studies that were incompletely presented were excluded from the review process.
2.4 Data extraction
A data abstraction protocol was used to construct data from each of the included articles. The data extraction protocol consisted of the normal microbiota body sites; examples of the microbiota encountered and its functions are in Table 1. The type of new agents, the dose, drug administration, the method of drug receiving, the number of subjects, the drug’s impact on the human intestinal microbiota, the means of drug elimination, and references are in Table 2. Furthermore, type of patient, age, the type of probiotic species, probiotic dose (CFU) on a daily, probiotic therapy duration, and references are in Table 3. The selection of all
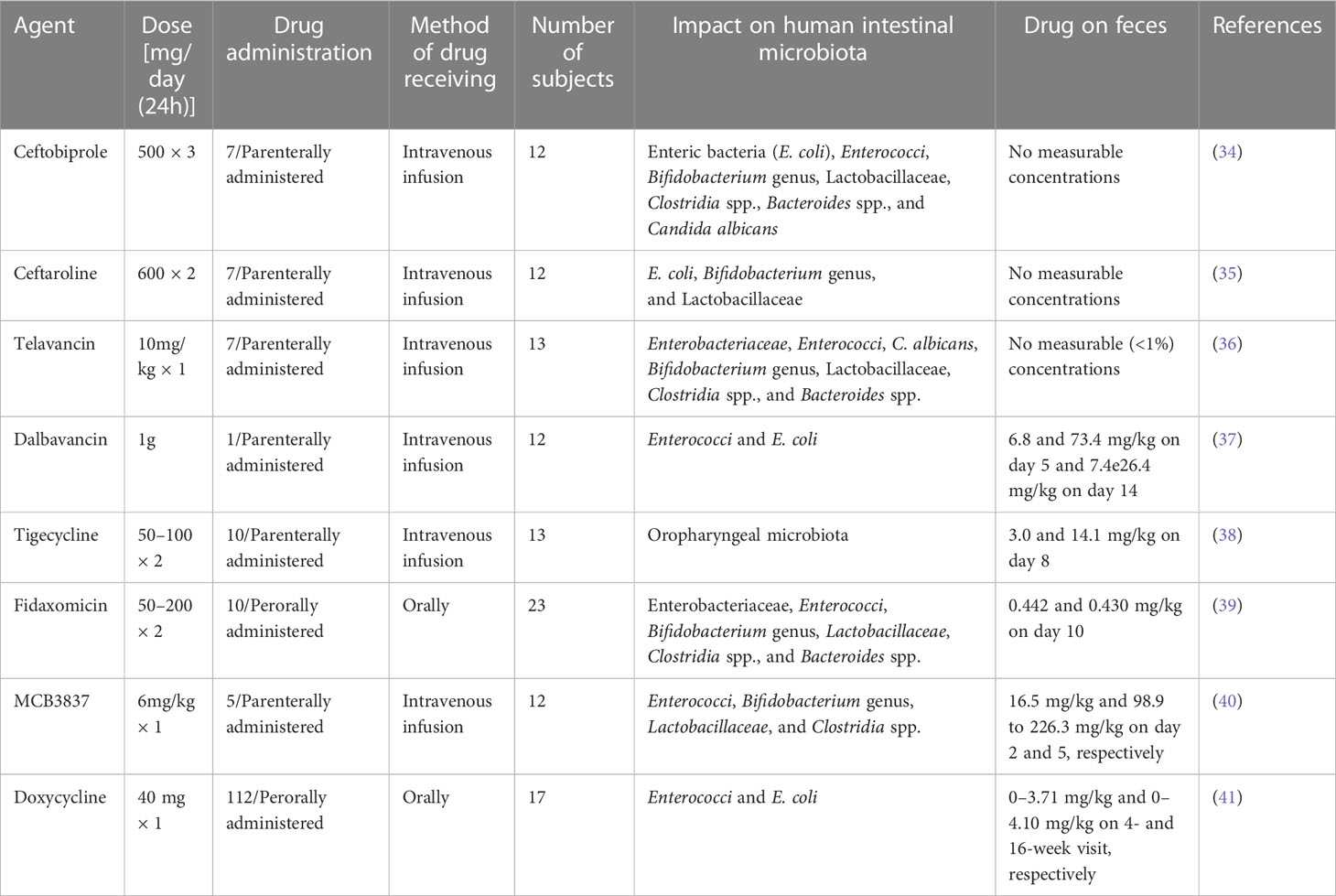
Table 2 The effect of novel antimicrobial agents on the ecological balance of human intestinal microbiota.
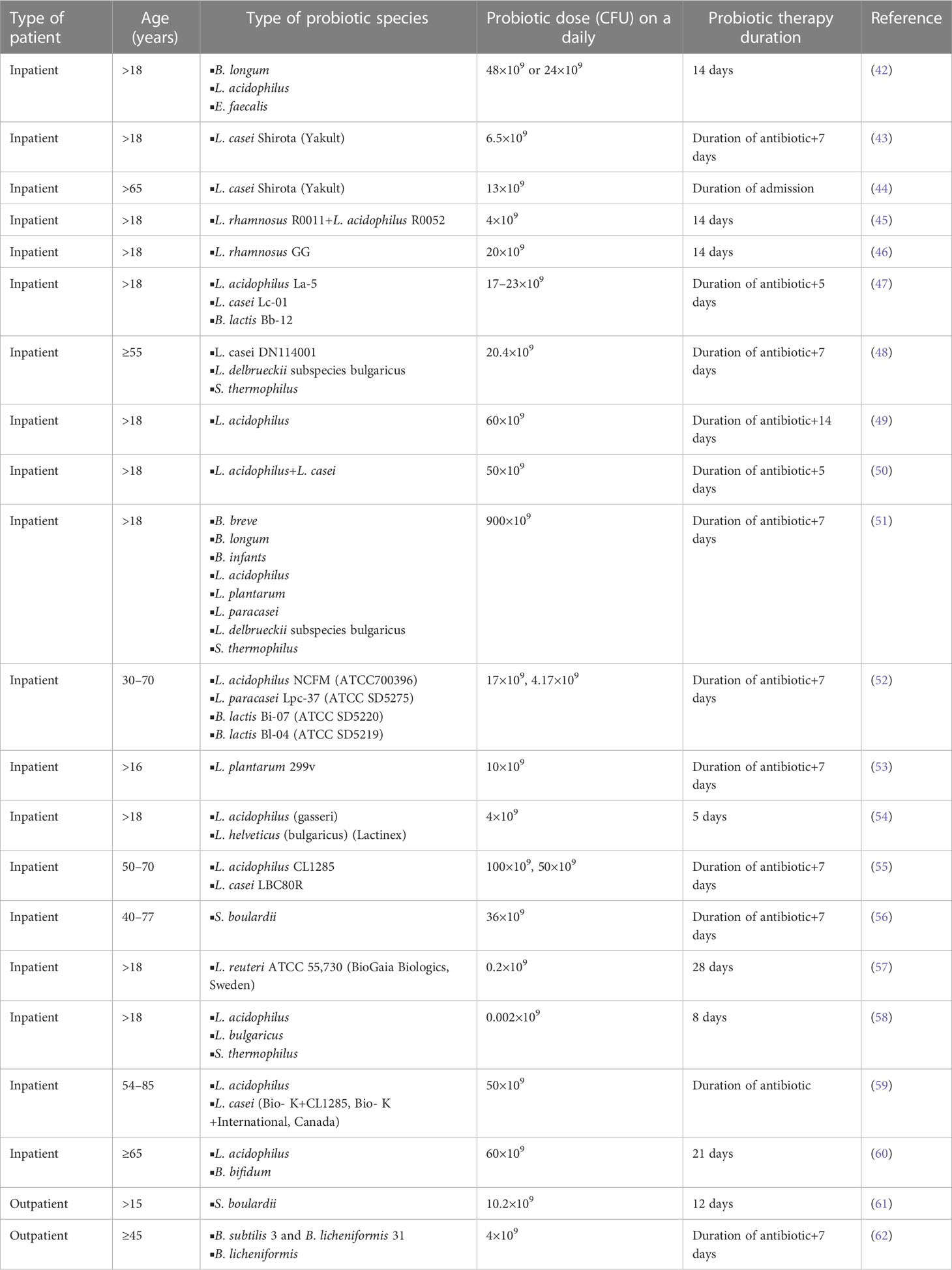
Table 3 The role of probiotics in decreasing antibiotic-associated diarrhea impacts among in- and out-patients.
recovered articles was carried out step by step by Abayeneh Girma, and finally the extracted data were combined and clearly presented in the table with key information and findings. The period from 1 November to 30 December 2022 was used for study selection, quality evaluation, and data extraction.
2.5 Quality assessment of each included study
For a systematic review, the PRISMA 2009 checklist (33) is the best tool to assess and examine the validity, reliability, and presentation quality of all extracted data from each included article. The choice and evaluation of the quality were performed by Abayeneh Girma as per the flow diagram presented in Figure 1. The articles were added after carefully checking their quality.
3 Results
In total, 379 articles on the ecological impacts of novel antimicrobial agents on human intestinal microbiota were recovered from across the world. One hundred and two of these articles were excluded due to duplicates. Of the remaining 277 articles, 97 were excluded after screening the titles. Of the remaining 180 articles, 92 were also excluded after abstract selection. Of the 88 articles, 80 were further excluded after observation and review due to the inclusion and exclusion criteria used. Therefore, only eight of the studies met the eligibility criteria and were included in the final systematic review (Figure 1). Of the included articles, six investigated the parenteral type of administration of the agents, while the remaining two articles tested by peroral type of administration (Table 2). Six studies used intravenous infusion and two studies used oral administration (Table 2). One of the included articles evaluated the impacts of antimicrobial agents on 23 subjects, and the other seven articles assessed fewer than 20 subjects (Table 2). Five of the included articles showed the presence of drugs on faces during excretion on different days, while the remaining three articles reported no measurable concentrations of drugs on feces (Table 2).
4 Human microbiota
The normal human microbiota (Table 1), also known as the normal microbiota, the indigenous microbial population, or microbiota, is a mixture of microorganisms that regularly inhabit any site in the human body and contribute to the regulation of a host’s health. The internal organs and systems, including the spleen, pancreas, liver, bladder, central nervous system, and blood, are sterile apart from occasional transient intruders. A healthy newborn was previously reported as being practically sterile when it is born but quick to acquire the typical microbiota from food and the environment, even from other people (63, 64). Recent findings, however, revealed that bacteria are present in the placenta, umbilical cord blood, fetal membranes, and amniotic fluid of healthy neonates who show no symptoms of illness or inflammation. The meconium (first stools of newborns) of premature infants with healthy mothers contains a particular microbiome, with the main phyla being Bacillota and a predominance of Staphylococcus spp., while the Pseudomonadota phyla are present in species such as Escherichia coli, Klebsiella pneumoniae, and Serratia marcescens (4, 63, 65, 66). In a healthy person, these microbes are generally harmless and even helpful through metabolic, defensive, and trophic functions (Figure 2). For all humans, the species found in the normal microbiota cannot be precisely defined because they differ from person to person due to physiological variances, dietary preferences, age, and geographical habitat (67).
5 Classification of the microbiota
Normal microbiota are clearly classified as resident (they permanently reside in a given body site, e.g., the skin, because they do not completely flush off from a given anatomical site and they have the potential to re-establish after disturbance, and this type of microbe does not associate with the transmission of disease because they are totally non-pathogenic) or transient (they reside temporarily, cannot re-establish after destruction, can flush off the body site, can be pathogenic or non-pathogenic, and this group is highly associated with disease transmission since they become opportunistic pathogen due to dysbiosis).
6 Beneficial effects of eubiotic microbiota
Eubiotic microbiota are important in the synthesis of different vitamins (e.g., vitamins K, B7, and B9), for the digestion and absorption of different nutrients, for improving lactose use in people with lactose intolerance and serum cholesterol concentration, for producing volatile fatty acids (e.g., acetic, propionic, and butyric acids) by anaerobic bacteria that are toxic to the growth of enterobacteria in the gut, for preventing the proliferation of disease-causing microbes by lowering the pH of the environment, for producing organic acids and substances (e.g., bacteriocins) that discourage colonization by exogenous microorganisms, for competition with pathogens for nutrients and space (attachment sites), for the inactivation of microbial toxins (e.g., bacteria or fungi) or metabolites, for stimulating non-specific immunity, and for increasing the brain development and behavior of the host (67) (Table 1). Generally, metabolic, defensive, and trophic effects are the main beneficial effects of eubiotic intestinal microbiota (15) (Figure 2).
7 The effect of antimicrobial agents on the intestinal microbiota and its resistance mechanisms
Antimicrobial agents have a great impact on the human body and its microbiota. For example, the phyla Firmicutes, Bacteroidetes, Actinobacteria, and Proteobacteria mainly dominate the human intestinal microbiota; however, they are highly sensitive to the agent’s deleterious effect. Administration of antimicrobial drugs is the most common and substantial cause of changes in the normal gut microbiota. The antimicrobial agents’ effect on the ecological balance of the human intestinal microbiota has direct and indirect impacts on the health of the host. When the normal microbiota of the intestine is reduced in species diversity during therapy, the resistance to colonization is significantly decreased, leading to the following outcomes: (i) The resistance of microorganisms to administered antimicrobial agents allows them to proliferate in large concentrations in the intestine. (ii) Allows the overgrowth of exogenous bacteria, yeast, and other microbes. (iii) Pathogenic microorganisms that are resistant to antimicrobial agents and colonies within the intestine can spread to different parts of the host. (iv) Overgrowth of pathogenic microorganisms in the intestine encourages the transfer and spread of resistant organisms and their genes either vertically or horizontally (Figure 3). (v) A lower contamination threshold dose results from a decrease in resistance to colonization (68). (vi) Reduces the diversity of species in the intestinal microbiota. (vii) Alters metabolic activity. (viii) Causes antibiotic-associated complications and comorbidities such as obesity, asthma, allergies, and IBD, especially in children (15, 69–71).
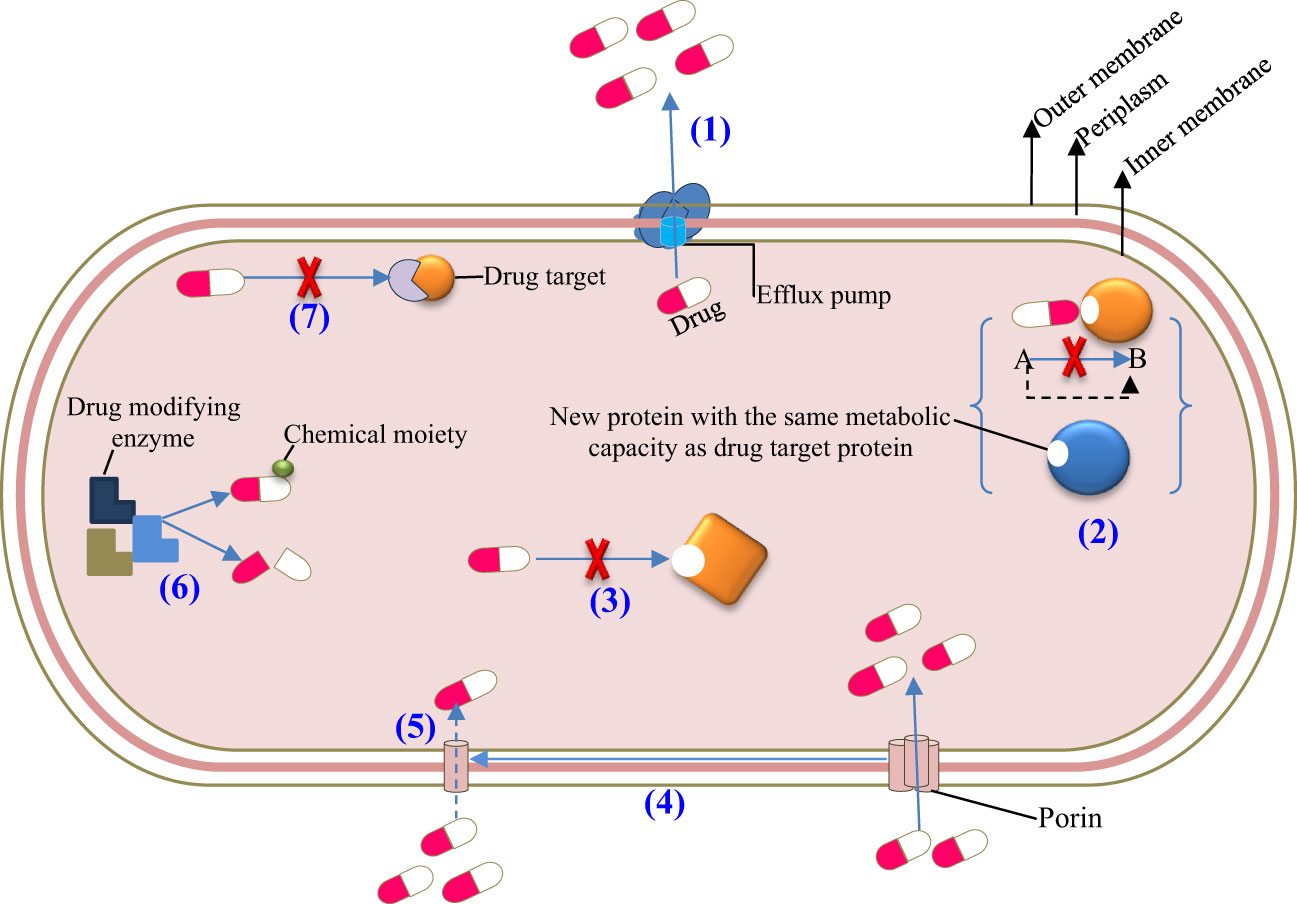
Figure 3 Resistance mechanisms of microbiota after frequent exposure to antimicrobial agents. (1) Active efflux, (2) Target bypass, (3) Target site modification, (4) Downregulation, (5) Decreased influx, (6) Drug inactivation, and (7) Target protection.
Furthermore, the frequency and duration of antimicrobial treatment affect the intestinal microbiota and make the area of the resistome favorable for antimicrobial-resistant pathogens and their genes. Resistomes are intestinal microbes that have numerous genes for antibiotic resistance. The resident resistome (commensal bacteria containing antibiotic-resistant genes) and the transitory resistome (antibiotic-resistant genes carried by bacteria periodically) are the two different types of resistomes found in the intestinal microbiota. The transient resistome has the potential to become a long-term component of the microbiota or pass on its resistance gene to commensal bacteria. Therefore, it is of high interest to determine the gut microbiota of people who have the antibiotic-resistant gene and understand how the gene can spread among various commensal organisms and opportunistic pathogens (Figure 3). Generally, the alteration of the intestinal microbiota composition due to antimicrobial agents results in dysbiosis or disease and affects the host’s health (71–74). Some of the antimicrobial agents that have been recently approved by the US Food and Drug Administration (FDA) for clinical use are listed in Table 2, and their brief effects on the intestinal microbiota are presented as follows:
7.1 Ceftobiprole
The new broad-spectrum cephem antibiotic known as “ceftobiprole” (Figure 4A and Table 2) is an effective antimicrobial agent against Gram-negative bacteria such as Enterobacteriaceae and Pseudomonas spp., as well as methicillin-resistant Staphylococcus aureus (MRSA) and vancomycin-resistant Enterococcus faecalis (75–77). Ceftobiprole is a preliminary cephalosporin that shows clinical efficacy in patients with MRSA-causing infections. It is a potential antibacterial drug for the treatment of pneumonia and severe skin infections. According to different reports, it is a well-accepted antimicrobial agent with good safety for the host and is excreted in urine. Furthermore, according to the reports of Bäckström et al. (34), this agent had no remarkable ecological effect on the human microbiota of the intestine. Bacteroides fragilis and Prevotella spp. are resistant to ceftobiprole, which implies that the agent is secreted by the intestinal mucosa or bile, affecting the normal functioning of the intestinal microbiota and causing resistance to antibiotics.
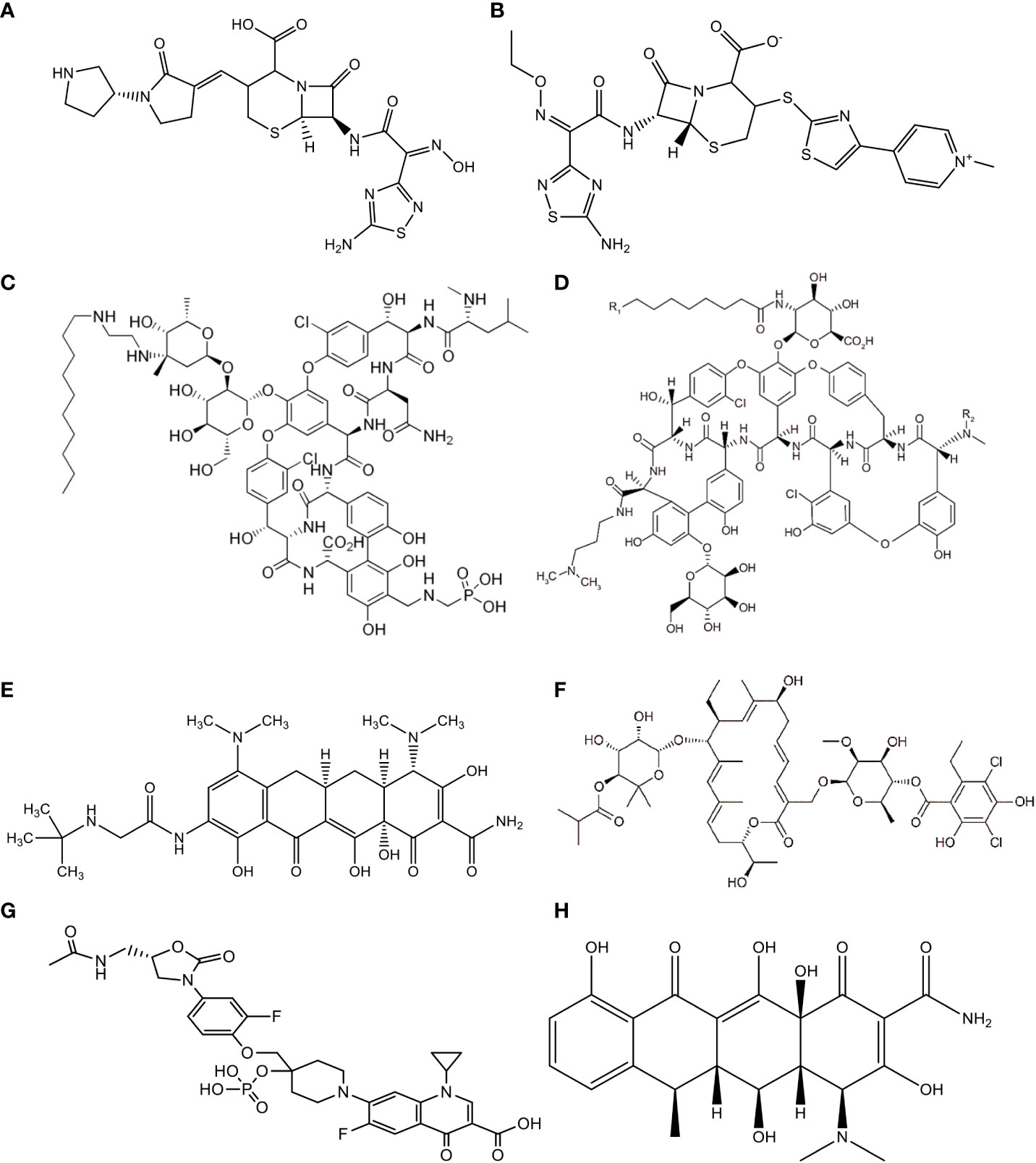
Figure 4 Chemical Structure of (A) Ceftobiprole, (B) Ceftaroline, (C) Telavancin, (D) Dalbavancin, (E) Tigecycline, (F) Fidaxomicin, (G) MCB3837, (H) Doxycycline.
7.2 Ceftaroline
A new semisynthetic parenteral cephalosporin antimicrobial agent with broad-spectrum action is called “ceftaroline” (Figure 4B and Table 2). Unlike other cephalosporins, ceftaroline continues to be active against MRSA, penicillin-resistant pneumococci, and Gram-negative infections. It is a potential antibacterial drug that can be used to treat complex skin and skin structure infections, as well as pneumonia caused by a bacterial community infection (78). According to reports, ceftaroline is a well-accepted and safe antimicrobial agent, and renal excretion is the means of drug elimination (79–81). However, B. fragilis and Prevotella species are resistant to this antibiotic. This might be due to the secretion of ceftaroline by intestinal mucosa or bile, which leads to antibiotic resistance. Furthermore, Panagiotidis et al. (35) reported that this antibiotic had a minor impact on the normal function of the human intestinal microbiota.
7.3 Telavancin
A novel and narrow-spectrum lipoglycopeptide antibiotic used for the treatment of Gram-positive aerobic and anaerobic bacterial pathogens is known as “telavancin” (Figure 4C and Table 2). Inhibition of bacterial cell wall synthesis and disruption of the plasma membrane are the main mechanisms of action of this antibiotic (82, 83). According to Saravolatz et al. (84), Stryjewski et al. (85), Rubinstein et al. (86), and Finegold et al. (87), telavancin is crucial for the treatment of severe skin and skin structure infections, bacterial infections, and pneumonia acquired in hospitals that are caused by MRSA, methicillin-susceptible Staphylococcus aureus (MSSA), S. pneumoniae, S. pyogenes, S. agalactiae, S. anginosus, S. intermedius, or S. constellatus, as well as vancomycin-susceptible E. faecalis. This antibiotic had higher activity against MRSA and Clostridium spp. than other antibiotics such as vancomycin. However, telavancin is not effective against aerobic and anaerobic bacteria due to the lipopolysaccharide found in these organisms, which makes the target inaccessible to this antimicrobial agent. Furthermore, according to different reports, telavancin is a safe and well-tolerated antibiotic, with 60% to 70% of the drug eliminated by renal excretion and <1% in feces (36, 88, 89).
7.4 Dalbavancin
A novel and narrow-spectrum lipoglycopeptide antibiotic effective in the laboratory against most Gram-positive bacterial pathogens is called “dalbavancin” (Figure 4D and Table 2); these pathogens include staphylococci, streptococci, Enterococci, corynebacteria, and anaerobic bacteria (90, 91). Its in vitro activity is also observed in MSSA, MRSA, and coagulase-negative staphylococci (CNS) such as S. epidermidis, S. haemolyticus, and others (30). Furthermore, it is highly effective against S. pneumoniae (either susceptible or resistant to penicillin), vancomycin-susceptible bacteria, and some classes of resistant Enterococci (30, 31). Different clinical studies around the world have confirmed that administration of this antibiotic once a week is highly effective in the treatment of Gram-positive bacterial pathogens. This agent is reported as a well-tolerated and safe antibiotic with no major ecological impact on the intestinal microbiota. The drug is excreted through both urine and feces (37, 92–94).
7.5 Tigecycline
A broad-spectrum and novel analog of minocycline antibiotic analogs that demonstrates activity against aerobic and anaerobic Gram-positive and Gram-negative pathogenic bacteria, including antimicrobial-resistant bacterial strains, is known as “tigecycline” (Figure 4E and Table 2) (95–99). This antibiotic is crucial for the treatment of severe skin and skin structure infections, as well as intra-abdominal infections. The reports also suggest that this antibiotic is tolerable and safe for the host. This drug is excreted through biliary elimination and, therefore, has a temporary impact on the ecological balance of the human intestinal microbiota (Enterococci, E. coli, Lactobacillaceae, and Bifidobacterium genus), apart from the oropharyngeal microbiota, due to the broad spectrum and high concentrations of tigecycline in the intestine (38, 100–102).
7.6 Fidaxomicin
A novel, narrow-spectrum and 18-membered-ring macrocyclic bactericidal agent developed primarily to treat CDI is called “fidaxomicin” (Figure 4F and Table 2). In vitro, this antibiotic was highly effective against CDIs compared with the other, poorly absorbed, oral antibiotic known as “vancomycin” (103, 104). Fidaxomicin is less effective against Gram-positive spore formation, such as Propionibacterium, Lactobacillaceae and Peptostreptococci, as well as being ineffective against aerobic and anaerobic Gram-negative bacilli such as Enterobacteriaceae, Pseudomonas spp., Campylobacter spp., Helicobacter spp., Haemophilus spp., Bacteroides spp., Fusobacterium, Porphyromonas spp., Prevotella spp., and Veillonella spp. This antibiotic prevents transcription through the inhibition of the RNA polymerase enzyme. According to different studies, as compared with vancomycin, it had a low ecological impact on the human intestinal microbiota. B. fragilis also resists this drug (39, 105).
7.7 MCB3837
A novel, fluoroquinolone-oxazolidinone, narrow-spectrum, and water-soluble synthetic antibiotic that targets only Gram-positive bacteria is called “MCB3837” (Figure 4G and Table 2), which belongs to a group of medicines known as “quinolone antibiotics”. After parenteral administration in vivo, oxaquin is rapidly converted to its active form called “MCB3681”. It is a bactericidal agent developed primarily to treat CDI (106–108). In addition, it is used to treat bacterial infections such as severe skin and soft tissue infections, genital tract infections, acute exacerbation of chronic obstructive pulmonary disease, community-acquired pneumonia (CAP), bronchitis, and mild to moderate pelvic inflammatory disease. The mechanism of action of MCB3681 is to bind to a DNA gyrase enzyme and block the DNA replication of the pathogen. The reports also suggest that this antibiotic is considered ecologically favorable for the host. This drug has a pronounced impact on the ecological balance of the human intestinal microbiota (Enterococci, Lactobacillaceae, Clostridia, and Bifidobacterium genus), apart from skin, nasal and oropharyngeal microbiota due to its spectrum, pharmacokinetic properties, and high concentrations of MCB3681 in the intestine without resistance development (40).
7.8 Doxycycline
A novel, broad-spectrum, and semi-synthetic antibiotic that belongs to the class “tetracyclines” and is effective against, aerobic, and anaerobic Gram positive and Gram-negative bacteria, spirochetes, and mycoplasma is called “Doxycycline” (Figure 4H and Table 2). It is primarily developed for the treatment of anthrax caused by Bacillus anthracis. Inhibition of protein synthesis by allosterically binding to the 30S bacterial ribosomal unit is the mechanism of action of doxycycline. It has a minor ecological effect on the oropharyngeal and intestinal microbiota. The drug is excreted through both urine and feces. Furthermore, the agent is secreted by the intestinal mucosa or bile and affects the normal functioning of the intestinal microbiota (Enterococci and E. coli) through inflammatory effects and causes resistance to antibiotics (41, 109, 110).
8 The effect of diet and medicines on microbiota diversity among Western and non-Western countries
It is generally known that a long-term diet affects the microbiome’s taxonomic composition and functional characteristics (Figure 5). The inverse association between Prevotella and Bacteroides, two members of the Bacteroidetes phylum, is a recurring pattern. Prevotella is associated with a diet high in plant-based foods, which is predominant in non-industrialized people, but Bacteroides is associated with a larger intake of animal fats and proteins, which is typical in industrialized populations (112–115).
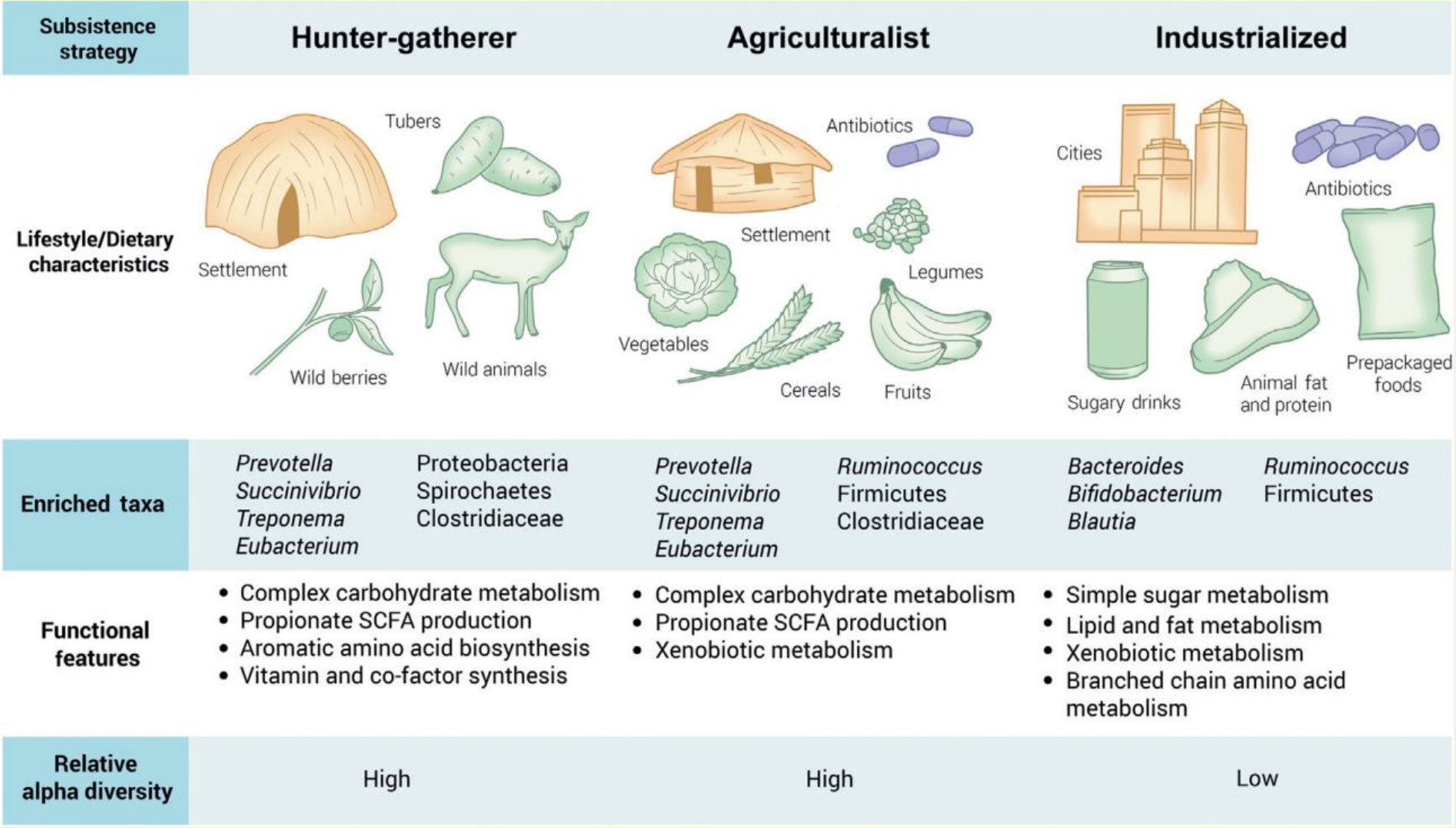
Figure 5 Effects of diet and medicines on microbiota diversity among Western and non-Western countries. Adapted from (111) with permission from Elsevier Ltd.
With regard to antibiotic utilization, hunter-gatherer nations did not use it, while agriculturalists utilized fewer antibiotics than industrialized countries, which utilized more drugs either separately or in combination with food or water (111). As a result, according to Brewster et al. (111), we can conclude that in Western or developed countries, the intestinal microbiota diversity and function are more highly affected by medicines and diet than in non-Western or developing countries. Similarly, Mosca et al. (116) reported that dysbiosis and a lack of microbial diversity in the gut microbiota are linked to the majority of human diseases that strike Westernized nations. This is because the widespread use of antibiotics and other environmental triggers in Western lifestyles means there may be fewer bacterial predators, which could result in less microbial diversity in the human gut. Furthermore, according to Nasiri et al. (117), the protective gut microbiota is significantly reduced in patients taking broad-spectrum antibiotics, which promote the growth of Clostridioides difficile, which can be present in certain people at low levels.
9 The role of probiotics against the side effects of antimicrobial agents
Probiotics are living microorganisms that, when taken in substantial amounts, confer host health. Lactobacillaceae, Bifidobacteria, and yeasts (e.g., Saccharomyces boulardii) are the most known, commercially exploited, and important probiotic organisms to human health. They increase the growth of beneficial intestinal microbiota, compete with intestinal pathogenic microbes (e.g., E. coli, C. difficile), produce antimicrobials (e.g., bacteriocins) that kill intestinal pathogens, and induce the immune response of the host (e.g., production of “reuterin” Limosilactobacillus reuteri) (Table 3). Furthermore, it is important in the control of antibiotic-associated diarrhea (e.g., European Societies for Paediatric Gastroenterology, Hepatology, and Nutrition guidelines recommend the use of Lacticaseibacillus rhamnosus and S. boulardii probiotic therapy to prevent the harmful effects of antibiotic-associated diarrhea). Furthermore, the Lactobacillaceae and Bifidobacteria probiotics in combination with H. pylori eradication therapy are more effective at eradicating H. pylori in the stomach than therapy without probiotics (15, 118–126).
10 Future approved novel antimicrobial agents under different developmental stages
Currently, novel antimicrobial agents are highly needed to reduce the increasing number of multidrug resistant (MDR) microbial pathogens and the impacts of dysbiosis on the intestinal microbiota. ACH-702 is a preclinical stage antimicrobial agent active against both Gram positive and Gram-negative bacterial pathogens including MRSA and M. tuberculosis (Figure 6). Plazomicin (active against Gram-positive and Gram-negative bacteria), delafloxacin (for the treatment of infections in low pH environments such as the skin and vaginal and urinary tracts), nemonoxacin (for the treatment of CAP and diabetic foot infections), radezolid (for the treatment of complicated skin and soft tissue infections, and CAP), sutezolid (for the treatment of extensive drug-resistant tuberculosis), razupenem (for the treatment of complicated skin and soft-tissue infections caused by MRSA, VRE), sulopenem (for the treatment of skin and soft-tissues infections, and CAP caused by various aerobic Gram-positive and Gram-negative organisms as well as anaerobes), solithromycin (for the treatment of CAP and other Gram-positive infections), TP-434 (active against Gram-positive and Gram-negative pathogens including MRSA, Streptococcus pyogenes, and E. coli), and BC-3781 (to treat serious skin and skin structure infections) are collectively grouped under the second phase of the developmental stage (Figure 6). Finally, finafloxacin (demonstrates activity against MRSA, VRE, anaerobes, and other drug-resistant strains), prulifloxacin (active against Gram-positive and Gram-negative bacteria causing urinary and respiratory tract infections), tedizolid (activates against Gram-positive bacterial pathogens, including linezolid-resistant strains), omadacycline (for the treatment of MRSA), oritavancin (for the treatment of Gram-positive bacterial pathogens including MRSA, VRSA, and VRE), dalbavancin (for the treatment of VRE), ramoplanin (for the treatment of local gastrointestinal infections caused by C. difficile), and iclaprim (for the treatment of complicated skin and soft-tissue infections caused by S. aureus and S. pneumoniae, H. influenzae, Moraxella catarrhalis, and Legionella pneumophila) are found under the third phase of the developmental stage (Figure 6) (127–138).
11 Conclusions and future perspectives
Antimicrobial agents can cause several adverse effects on the normal functioning of the human microbiota. Based on the spectrum of antibiotics, dose, administration route, pharmacokinetic and pharmacodynamic properties of antibiotics, and in vivo inactivation of the agent, the extent of disturbances can vary between clinical conditions, such as systemic infections in immunocompromised patients and antibiotic-associated diarrhea or colitis. Ceftobiprole, ceftaroline, telavancin, dalbavancin, tigecycline, fidaxomicin, MCB3681, and doxycycline have minor impacts on the ecological balance of the human intestinal microbiota. Generally, clinicians should consider the antibiotic interaction between the agent administered and the normal intestinal microbiota when they choose the agent to treat microbial infections. In the future, novel antimicrobial agents and probiotics are highly needed to reduce the increasing impact of MDR pathogens and dysbiosis on intestinal microbiota health.
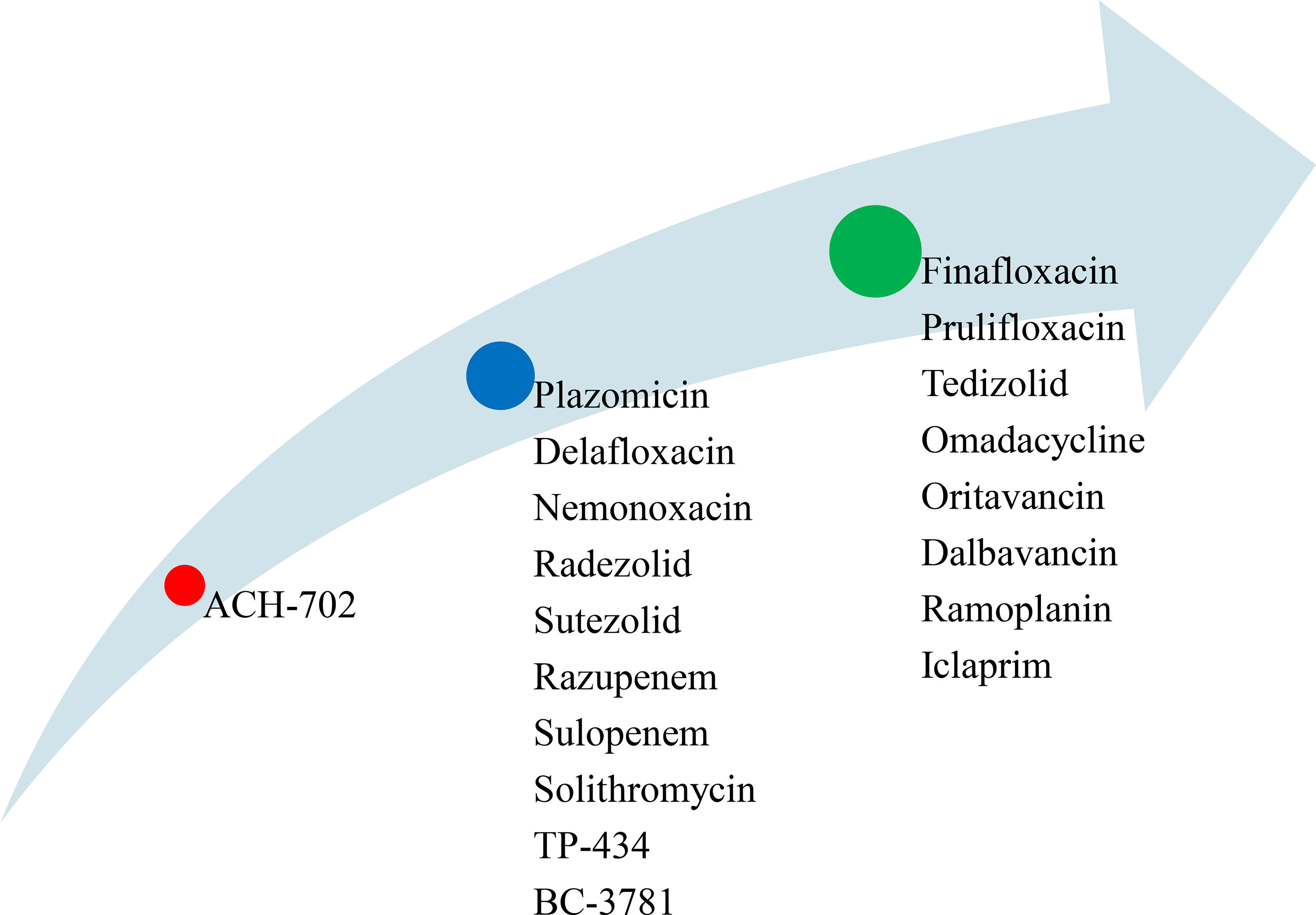
Figure 6 List of novel antimicrobial agents under different development stages (red=preclinical stage, blue= second phase, and green = third phase).
Author contributions
The author confirms being the sole contributor of this work and has approved it for publication.
Conflict of interest
The author declares that he has no conflicts of interest with respect to the publication of this study.
Publisher’s note
All claims expressed in this article are solely those of the authors and do not necessarily represent those of their affiliated organizations, or those of the publisher, the editors and the reviewers. Any product that may be evaluated in this article, or claim that may be made by its manufacturer, is not guaranteed or endorsed by the publisher.
References
1. Sullivan Å, Edlund C, Nord CE. Effect of antimicrobial agents on the ecological balance of human microflora. Lancet Infect diseases (2001) 1(2):101–14. doi: 10.1016/S1473-3099(01)00066-4
2. Sullivan Å, Edlund C, Nord CE. Interaction between antimicrobial agents and the oropharyngeal and intestinal normal microflora. Antimicrobial agents: antibacterials antifungals (2005), 1357–70. doi: 10.1128/9781555815929.ch56
3. Rashid M-U, Weintraub A, Nord CE. Effect of new antimicrobial agents on the ecological balance of human microflora. Anaerobe (2012) 18(2):249–53. doi: 10.1016/j.anaerobe.2011.11.005
4. Santacroce L, Man A, Charitos IA, Haxhirexha K, Topi S. Current knowledge about the connection between health status and gut microbiota from birth to elderly. A narrative review Front Bioscience-Landmark. (2021) 26(6):135–48. doi: 10.52586/4930
5. Marcobal A, Barboza M, Froehlich JW, Block DE, German JB, Lebrilla CB, et al. Consumption of human milk oligosaccharides by gut-related microbes. J Agric Food Chem (2010) 58(9):5334–40. doi: 10.1021/jf9044205
6. Gupta VK, Paul S, Dutta C. Geography, ethnicity or subsistence-specific variations in human microbiome composition and diversity. Front Microbiol (2017) 8:1162. doi: 10.3389/fmicb.2017.01162
7. Okada H, Kuhn C, Feillet H, Bach J-F. The ‘hygiene hypothesis’ for autoimmune and allergic diseases: an update. Clin Exp Immunol (2010) 160(1):1–9. doi: 10.1111/j.1365-2249.2010.04139.x
8. Muniz LR, Knosp C, Yeretssian G. Intestinal antimicrobial peptides during homeostasis, infection, and disease. Front Immunol (2012) 3:310. doi: 10.3389/fimmu.2012.00310
9. Pickard JM, Zeng MY, Caruso R, Núñez G. Gut microbiota: Role in pathogen colonization, immune responses, and inflammatory disease. Immunol Rev (2017) 279(1):70–89. doi: 10.1111/imr.12567
10. MacFarlane L-A, Murphy P R. MicroRNA: biogenesis, function and role in cancer. Curr Genomics (2010) 11(7):537–61. doi: 10.2174/138920210793175895
11. Yoon MY, Yoon SS. Disruption of the gut ecosystem by antibiotics. Yonsei Med J (2018) 59(1):4–12. doi: 10.3349/ymj.2018.59.1.4
12. Jernberg C, Löfmark S, Edlund C, Jansson JK. Long-term ecological impacts of antibiotic administration on the human intestinal microbiota. ISME J (2007) 1(1):56–66. doi: 10.1038/ismej.2007.3
13. Lewis BB, Buffie CG, Carter RA, Leiner I, Toussaint NC, Miller LC, et al. Loss of microbiota-mediated colonization resistance to Clostridium difficile infection with oral vancomycin compared with metronidazole. J Infect diseases (2015) 212(10):1656–65. doi: 10.1093/infdis/jiv256
14. Jakobsson HE, Jernberg C, Andersson AF, Sjölund-Karlsson M, Jansson JK, Engstrand L. Short-term antibiotic treatment has differing long-term impacts on the human throat and gut microbiome. PloS One (2010) 5(3):e9836. doi: 10.1371/journal.pone.0009836
15. Ramirez J, Guarner F, Bustos Fernandez L, Maruy A, Sdepanian VL, Cohen H. Antibiotics as major disruptors of gut microbiota. Front Cell infection Microbiol (2020) 10:572912. doi: 10.3389/fcimb.2020.572912
16. Davies J, Davies D. Origins and evolution of antibiotic resistance. Microbiol Mol Biol Rev (2010) 74(3):417–33. doi: 10.1128/MMBR.00016-10
17. Nord C, Edlund C. Impact of antimicrobial agents on human intestinal microflora. J Chemotherapy (1990) 2(4):218–37. doi: 10.1080/1120009X.1990.11739021
18. van der Waaij D, Nord CE. Development and persistence of multi-resistance to antibiotics in bacteria; an analysis and a new approach to this urgent problem. Int J antimicrobial agents. (2000) 16(3):191–7. doi: 10.1016/S0924-8579(00)00227-2
19. Shah D, Dang M-D, Hasbun R, Koo HL, Jiang Z-D, DuPont HL, et al. Clostridium difficile infection: update on emerging antibiotic treatment options and antibiotic resistance. Expert Rev anti-infective Ther (2010) 8(5):555–64. doi: 10.1586/eri.10.28
20. DeLeo FR, Otto M, Kreiswirth BN, Chambers HF. Community-associated meticillin-resistant Staphylococcus aureus. Lancet (2010) 375(9725):1557–68. doi: 10.1016/S0140-6736(09)61999-1
21. Milloy M, Wood E. Transmitted antiretroviral-resistant HIV: a coming anarchy? Lancet Infect Dis (2011) 11(5):336–7. doi: 10.1016/S1473-3099(11)70068-8
22. Miethke T. Increasing therapeutic challenges through multi-resistant bacteria in the hospital. Zentralblatt fur Chirurgie (2011) 137(3):279–83. doi: 10.1055/s-0031-1271348
23. Guinovart C, Navia M, Tanner M, Alonso P. Malaria: burden of disease. Curr Mol Med (2006) 6(2):137–40. doi: 10.2174/156652406776055131
24. McDonald LC, Killgore GE, Thompson A, Owens RC Jr., Kazakova SV, Sambol SP, et al. An epidemic, toxin gene–variant strain of Clostridium difficile. New Engl J Med (2005) 353(23):2433–41. doi: 10.1056/NEJMoa051590
25. Qiao N, Wittouck S, Mattarelli P, Zheng J, Lebeer S, Felis GE, et al. After the storm—Perspectives on the taxonomy of Lactobacillaceae. JDS Commun (2022) 3(3):222–227. doi: 10.3168/jdsc.2021-0183
26. Klevens RM, Morrison MA, Nadle J, Petit S, Gershman K, Ray S, et al. Invasive methicillin-resistant Staphylococcus aureus infections in the United States. Jama (2007) 298(15):1763–71. doi: 10.1001/jama.298.15.1763
27. Higgins PG, Dammhayn C, Hackel M, Seifert H. Global spread of carbapenem-resistant Acinetobacter baumannii. J Antimicrobial Chemotherapy (2010) 65(2):233–8. doi: 10.1093/jac/dkp428
28. Henrich TJ, Krakower D, Bitton A, Yokoe DS. Clinical risk factors for severe Clostridium difficile–associated disease. Emerging Infect diseases (2009) 15(3):415.
29. Richards T. Alliance pledges new cheap TB drug by 2010. BMJ (2000) 321(7267):981. doi: 10.1136/bmj.321.7267.981
30. Spellberg B, Guidos R, Gilbert D, Bradley J, Boucher HW, Scheld WM, et al. The epidemic of antibiotic-resistant infections: a call to action for the medical community from the Infectious Diseases Society of America. Clin Infect diseases (2008) 46(2):155–64. doi: 10.1086/524891
31. Woerther P-L, Angebault C, Lescat M, Ruppé E, Skurnik D, El Mniai A, et al. Emergence and dissemination of extended-spectrum β-lactamase-producing Escherichia coli in the community: lessons from the study of a remote and controlled population. J Infect diseases (2010) 202(4):515–23. doi: 10.1086/654883
32. Tomasz A. Multiple-antibiotic-resistant pathogenic bacteria–a report on the Rockefeller University workshop. New Engl J Med (1994) 330(17):1247–51. doi: 10.1056/NEJM199404283301725
33. Moher D, Liberati A, Tetzlaff J, Altman DG, PRISMA Group* t. Preferred reporting items for systematic reviews and meta-analyses: the PRISMA statement. Ann Internal Med (2009) 151(4):264–9. doi: 10.7326/0003-4819-151-4-200908180-00135
34. Bäckström T, Panagiotidis G, Beck O, Asker-Hagelberg C, Rashid M-U, Weintraub A, et al. Effect of ceftobiprole on the normal human intestinal microflora. Int J antimicrobial agents. (2010) 36(6):537–41. doi: 10.1016/j.ijantimicag.2010.07.021
35. Panagiotidis G, Baückstroüm T, Asker-Hagelberg C, Jandourek A, Weintraub A, Nord CE. Effect of ceftaroline on normal human intestinal microflora. Antimicrobial Agents chemotherapy (2010) 54(5):1811–4. doi: 10.1128/AAC.01716-09I
36. Rashid M-U, Weintraub A, Nord CE. Effect of telavancin on human intestinal microflora. Int J antimicrobial agents. (2011) 38(6):474–9. doi: 10.1016/j.ijantimicag.2011.08.012
37. Nord CE, Rasmanis G, Wahlund E. Effect of dalbavancin on the normal intestinal microflora. J antimicrobial chemotherapy (2006) 58(3):627–31. doi: 10.1093/jac/dkl281
38. Nord CE, Sillerstroüm E, Wahlund E. Effect of tigecycline on normal oropharyngeal and intestinal microflora. Antimicrobial Agents chemotherapy (2006) 50(10):3375–80. doi: 10.1128/AAC.00373-06
39. Tannock GW, Munro K, Taylor C, Lawley B, Young W, Byrne B, et al. A new macrocyclic antibiotic, fidaxomicin (OPT-80), causes less alteration to the bowel microbiota of Clostridium difficile-infected patients than does vancomycin. Microbiology (2010) 156(11):3354–9. doi: 10.1099/mic.0.042010-0
40. Rashid M-U, Dalhoff A, Bäckström T, Björkhem-Bergman L, Panagiotidis G, Weintraub A, et al. Ecological impact of MCB3837 on the normal human microbiota. Int J antimicrobial agents. (2014) 44(2):125–30. doi: 10.1016/j.ijantimicag.2014.03.016
41. Rashid M-U, Panagiotidis G, Bäckström T, Weintraub A, Nord CE. Ecological impact of doxycycline at low dose on normal oropharyngeal and intestinal microflora. Int J antimicrobial agents. (2013) 41(4):352–7. doi: 10.1016/j.ijantimicag.2012.11.014
42. Wu J, Gan T, Zhang Y, Xia G, Deng S, Lv X, et al. The prophylactic effects of BIFICO on the antibiotic-induced gut dysbiosis and gut microbiota. Gut Pathogens (2020) 12:1–11. doi: 10.1186/s13099-020-00379-0
43. Wong S, Jamous A, O'Driscoll J, Sekhar R, Weldon M, Yau CY, et al. A Lactobacillus casei Shirota probiotic drink reduces antibiotic-associated diarrhoea in patients with spinal cord injuries: a randomised controlled trial. Br J Nutr (2014) 111(4):672–8. doi: 10.1017/S0007114513002973
44. Wright K, Wright H, Murray M. Probiotic treatment for the prevention of antibiotic-associated diarrhoea in geriatric patients: A multicentre randomised controlled pilot study. Australas J Ageing (2015) 34(1):38–42. doi: 10.1111/ajag.12116
45. Song HJ, Kim J-Y, Jung S-A, Kim S-E, Park H-S, Jeong Y, et al. Effect of probiotic Lactobacillus (Lacidofil® cap) for the prevention of antibiotic-associated diarrhea: a prospective, randomized, double-blind, multicenter study. J Korean Med science (2010) 25(12):1784–91. doi: 10.3346/jkms.2010.25.12.1784
46. Thomas MR, Litin SC, Osmon DR, Corr AP, Weaver AL, Lohse CM. Lack of effect of Lactobacillus GG on antibiotic-associated diarrhea: a randomized, placebo-controlled trial. Mayo Clinic Proc (2001) 76(9):883–889. doi: 10.4065/76.9.883
47. Velasco M, Requena T, Delgado-Iribarren A, Peláez C, Guijarro C. Probiotic yogurt for the prevention of antibiotic-associated diarrhea in adults. J Clin Gastroenterology. (2019) 53(10):717–23. doi: 10.1097/MCG.0000000000001131
48. Rajkumar C, Wilks M, Islam J, Ali K, Raftery J, Davies K, et al. Do probiotics prevent antibiotic-associated diarrhoea? Results of a multicentre randomized placebo-controlled trial. J Hosp Infection (2020) 105(2):280–8. doi: 10.1016/j.jhin.2020.01.018
49. Safdar N, Barigala R, Said A, McKinley L. Feasibility and tolerability of probiotics for prevention of antibiotic-associated diarrhoea in hospitalized US military veterans. J Clin Pharm Ther (2008) 33(6):663–8. doi: 10.1111/j.1365-2710.2008.00980.x
50. Psaradellis E, Sampalis J. Efficacy of BIO K+ CL1285® in the reduction of antibiotic-associated diarrhea–a placebo controlled double-blind randomized, multi-center study. Arch Med Science (2010) 6(1):56–64. doi: 10.5114/aoms.2010.13508
51. Selinger C, Bell A, Cairns A, Lockett M, Sebastian S, Haslam N. Probiotic VSL# 3 prevents antibiotic-associated diarrhoea in a double-blind, randomized, placebo-controlled clinical trial. J Hosp Infection (2013) 84(2):159–65. doi: 10.1016/j.jhin.2013.02.019
52. Ouwehand AC, DongLian C, Weijian X, Stewart M, Ni J, Stewart T, et al. Probiotics reduce symptoms of antibiotic use in a hospital setting: a randomized dose response study. Vaccine (2014) 32(4):458–63. doi: 10.1016/j.vaccine.2013.11.053
53. Lönnermark E, Friman V, Lappas G, Sandberg T, Berggren A, Adlerberth I. Intake of Lactobacillus plantarum reduces certain gastrointestinal symptoms during treatment with antibiotics. J Clin gastroenterology (2010) 44(2):106–12. doi: 10.1097/MCG.0b013e3181b2683f
54. Gotz V, ROmankiewicz JA, Moss J, Murray HW. Prophylaxis against ampicillin-associated diarrhea with a lactobacillus preparation. Am J Hosp Pharmacy (1979) 36(6):754–7. doi: 10.1093/ajhp/36.6.754
55. Gao XW, Mubasher M, Fang CY, Reifer C, Miller LE. Dose–Response Efficacy of a Proprietary Probiotic Formula ofLactobacillus acidophilusCL1285 andLactobacillus caseiLBC80R for Antibiotic-Associated Diarrhea andClostridium difficile-Associated Diarrhea Prophylaxis in Adult Patients. Off J Am Coll Gastroenterology| ACG. (2010) 105(7):1636–41. doi: 10.1038/ajg.2010.11
56. Ehrhardt S, Guo N, Hinz R, Schoppen S, May J, Reiser M, et al. Saccharomyces boulardii to prevent antibiotic-associated diarrhea: a randomized, double-masked, placebo-controlled trial. Open Forum Infect Dis (2016) 3(1):ofw011. doi: 10.1093/ofid/ofw011
57. Cimperman L, Bayless G, Best K, Diligente A, Mordarski B, Oster M, et al. A randomized, double-blind, placebo-controlled pilot study of Lactobacillus reuteri ATCC 55730 for the prevention of antibiotic-associated diarrhea in hospitalized adults. J Clin gastroenterology (2011) 45(9):785–9. doi: 10.1097/MCG.0b013e3182166a42
58. Beniwal RS, Arena VC, Thomas L, Narla S, Imperiale TF, Chaudhry RA, et al. A randomized trial of yogurt for prevention of antibiotic-associated diarrhea. Digestive Dis Sci (2003) 48:2077–82. doi: 10.1023/A:1021711204498
59. Beausoleil M, Fortier N, Guénette S, L’Ecuyer A, Savoie M, Franco M, et al. Effect of a fermented milk combining Lactobacillus acidophilus Cl1285 and Lactobacillus casei in the prevention of antibiotic-associated diarrhea: a randomized, double-blind, placebo-controlled trial. Can J Gastroenterol Hepatology (2007) 21:732–6. doi: 10.1155/2007/720205
60. Allen SJ, Wareham K, Wang D, Bradley C, Hutchings H, Harris W, et al. Lactobacilli and bifidobacteria in the prevention of antibiotic-associated diarrhoea and Clostridium difficile diarrhoea in older inpatients (PLACIDE): a randomised, double-blind, placebo-controlled, multicentre trial. Lancet (2013) 382(9900):1249–57. doi: 10.1016/S0140-6736(13)61218-0
61. Bravo MV, Bunout D, Leiva L, de la Maza MP, Barrera G, de la Maza J, et al. Efecto del probiótico Saccharomyces boulardii en la prevención de la diarrea asociada con antibióticos en adultos ambulatorios en tratamiento con amoxicilina. Rev médica Chile (2008) 136(8):981–8. doi: 10.4067/S0034-98872008000800004
62. Horosheva TV, Vodyanoy V, Sorokulova I. Efficacy of Bacillus probiotics in prevention of antibiotic-associated diarrhoea: a randomized, double-blind, placebo-controlled clinical trial. JMM Case Rep (2014) 1(3):e004036. doi: 10.1099/jmmcr.0.004036
63. Aagaard K, Ma J, Antony KM, Ganu R, Petrosino J, Versalovic J. The placenta harbors a unique microbiome. Sci Trans Med (2014) 6(237):237ra65–ra65. doi: 10.1126/scitranslmed.3008599
64. Stinson LF, Boyce MC, Payne MS, Keelan JA. The not-so-sterile womb: evidence that the human fetus is exposed to bacteria prior to birth. Front Microbiol (2019) 1124:1124. doi: 10.3389/fmicb.2019.01124
65. Milani C, Duranti S, Bottacini F, Casey E, Turroni F, Mahony J, et al. The first microbial colonizers of the human gut: composition, activities, and health implications of the infant gut microbiota. Microbiol Mol Biol Rev (2017) 81(4):10–1128. doi: 10.1128/MMBR.00036-17IF:13.044Q1
66. Di Domenico M, Ballini A, Boccellino M, Scacco S, Lovero R, Charitos IA, et al. The intestinal microbiota may be a potential theranostic tool for personalized medicine. J Personalized Med (2022) 12(4):523. doi: 10.3390/jpm12040523
67. Dekaboruah E, Suryavanshi MV, Chettri D, Verma AK. Human microbiome: an academic update on human body site specific surveillance and its possible role. Arch Microbiol (2020) 202:2147–67. doi: 10.1007/s00203-020-01931-x
68. Nord CE, Kager L, Heimdahl A. Impact of antimicrobial agents on the gastrointestinal microflora and the risk of infections. Am J Med (1984) 76(5):99–106. doi: 10.1016/0002-9343(84)90250-X
69. Bokulich NA, Chung J, Battaglia T, Henderson N, Jay M, Li H, et al. Antibiotics, birth mode, and diet shape microbiome maturation during early life. Sci Trans Med (2016) 8(343):343ra82–ra82. doi: 10.1126/scitranslmed.aad7121
70. Kronman MP, Zaoutis TE, Haynes K, Feng R, Coffin SE. Antibiotic exposure and IBD development among children: a population-based cohort study. Pediatrics (2012) 130(4):e794–803. doi: 10.1542/peds.2011-3886
71. Shah T, Baloch Z, Shah Z, Cui X, Xia X. The intestinal microbiota: impacts of antibiotics therapy, colonization resistance, and diseases. Int J Mol Sci (2021) 22(12):6597. doi: 10.3390/ijms22126597
72. Casals-Pascual C, Vergara A, Vila J. Intestinal microbiota and antibiotic resistance: Perspectives and solutions. Hum Microbiome J (2018) 9:11–5. doi: 10.1016/j.humic.2018.05.002
73. Baron SA, Diene SM, Rolain J-M. Human microbiomes and antibiotic resistance. Hum Microbiome J (2018) 10:43–52. doi: 10.1016/j.humic.2018.08.005
74. Penders J, Stobberingh EE, Savelkoul PH, Wolffs PF. The human microbiome as a reservoir of antimicrobial resistance. Front Microbiol (2013) 4:87. doi: 10.3389/fmicb.2013.00087
75. Schirmer PL, Deresinski SC. Ceftobiprole: a new cephalosporin for the treatment of skin and skin structure infections. Expert Rev anti-infective Ther (2009) 7(7):777–91. doi: 10.1586/eri.09.54
76. Barbour A, Schmidt S, Rand KH, Derendorf H. Ceftobiprole: a novel cephalosporin with activity against Gram-positive and Gram-negative pathogens, including methicillin-resistant Staphylococcus aureus (MRSA). Int J antimicrobial agents. (2009) 34(1):1–7. doi: 10.1016/j.ijantimicag.2008.12.012
77. Obot I, Obi-Egbedi N. Adsorption properties and inhibition of mild steel corrosion in sulphuric acid solution by ketoconazole: experimental and theoretical investigation. Corrosion Science (2010) 52(1):198–204. doi: 10.1016/j.corsci.2009.09.002
78. Ge Y, Biek D, Talbot GH, Sahm DF. In vitro profiling of ceftaroline against a collection of recent bacterial clinical isolates from across the United States. Antimicrobial Agents chemotherapy (2008) 52(9):3398–407. doi: 10.1128/AAC.00149-08
79. Zhanel GG, Sniezek G, Schweizer F, Zelenitsky S, Lagace-Wiens PR, Rubinstein E, et al. Ceftaroline: a novel broad-spectrum cephalosporin with activity against meticillin-resistant Staphylococcus aureus. Drugs (2009) 69:809–31. doi: 10.2165/00003495-200969070-00003
80. Talbot GH, Thye D, Das A, Ge Y. Phase 2 study of ceftaroline versus standard therapy in treatment of complicated skin and skin structure infections. Antimicrobial Agents chemotherapy (2007) 51(10):3612–6. doi: 10.1128/AAC.00590-07
81. Suma BV. Newly validated stability-indicating ultra-performance liquid chromatography-tandem mass spectrometry method for the estimation of Ceftaroline Fosamil by using a quadrupole mass detector. J Appl Pharm Science (2022) 12(6):215–23. doi: 10.7324/JAPS.2022.120621
82. Higgins DL, Chang R, Debabov DV, Leung J, Wu T, Krause KM, et al. Telavancin, a multifunctional lipoglycopeptide, disrupts both cell wall synthesis and cell membrane integrity in methicillin-resistant Staphylococcus aureus. Antimicrobial Agents chemotherapy (2005) 49(3):1127–34. doi: 10.1128/AAC.49.3.1127-1134.2005
83. Lunde CS, Hartouni SR, Janc JW, Mammen M, Humphrey PP, Benton BM. Telavancin disrupts the functional integrity of the bacterial membrane through targeted interaction with the cell wall precursor lipid II. Antimicrobial Agents chemotherapy (2009) 53(8):3375–83. doi: 10.1128/AAC.01710-08
84. Saravolatz LD, Stein GE, Johnson LB. Telavancin: a novel lipoglycopeptide. Clin Infect diseases (2009) 49(12):1908–14. doi: 10.1086/648438
85. Stryjewski ME, Graham DR, Wilson SE, O'Riordan W, Young D, Lentnek A, et al. Telavancin versus vancomycin for the treatment of complicated skin and skin-structure infections caused by gram-positive organisms. Clin Infect diseases (2008) 46(11):1683–93. doi: 10.1086/587896
86. Rubinstein E, Lalani T, Corey GR, Kanafani ZA, Nannini EC, Rocha MG, et al. Telavancin versus vancomycin for hospital-acquired pneumonia due to gram-positive pathogens. Clin Infect Diseases (2011) 52(1):31–40. doi: 10.1093/cid/ciq031
87. Finegold SM, Bolanos M, Sumannen PH, Molitoris DR. In vitro activities of telavancin and six comparator agents against anaerobic bacterial isolates. Antimicrobial Agents chemotherapy (2009) 53(9):3996–4001. doi: 10.1128/AAC.00908-08
88. Wong SL, Barriere SL, Kitt MM, Goldberg MR. Multiple-dose pharmacokinetics of intravenous telavancin in healthy male and female subjects. J antimicrobial chemotherapy (2008) 62(4):780–3. doi: 10.1093/jac/dkn273
89. Corey GR, Rubinstein E, Stryjewski ME, Bassetti M, Barriere SL. Potential role for telavancin in bacteremic infections due to gram-positive pathogens: focus on Staphylococcus aureus. Clin Infect Diseases (2015) 60(5):787–96. doi: 10.1093/cid/ciu971
90. Goldstein EJ, Citron DM, Merriam CV, Warren Y, Tyrrell K, Fernandez HT. In vitro activities of dalbavancin and nine comparator agents against anaerobic gram-positive species and corynebacteria. Antimicrobial Agents chemotherapy (2003) 47(6):1968–71. doi: 10.1128/AAC.47.6.1968-1971.2003
91. Streit JM, Fritsche TR, Sader HS, Jones RN. Worldwide assessment of dalbavancin activity and spectrum against over 6,000 clinical isolates. Diagn Microbiol Infect disease (2004) 48(2):137–43. doi: 10.1016/j.diagmicrobio.2003.09.004
92. Seltzer E, Dorr MB, Goldstein BP, Perry M, Dowell JA, Henkel T, et al. Once-weekly dalbavancin versus standard-of-care antimicrobial regimens for treatment of skin and soft-tissue infections. Clin Infect diseases (2003) 37(10):1298–303. doi: 10.1086/379015
93. Raad I, Darouiche R, Vazquez J, Lentnek A, Hachem R, Hanna H, et al. Efficacy and safety of weekly dalbavancin therapy for catheter-related bloodstream infection caused by gram-positive pathogens. Clin Infect diseases (2005) 40(3):374–80. doi: 10.1086/427283
94. Leuthner KD, Buechler KA, Kogan D, Saguros A, Lee HS. Clinical efficacy of dalbavancin for the treatment of acute bacterial skin and skin structure infections (ABSSSI). Ther Clin Risk management (2016) 12:931–40. doi: 10.2147/TCRM.S86330
95. Boucher HW, Wennersten CB, Eliopoulos GM. In vitro activities of the glycylcycline GAR-936 against gram-positive bacteria. Antimicrobial Agents chemotherapy (2000) 44(8):2225–9. doi: 10.1128/AAC.44.8.2225-2229.2000
96. Gales AC, Jones RN. Antimicrobial activity and spectrum of the new glycylcycline, GAR-936 tested against 1,203 recent clinical bacterial isolates. Diagn Microbiol Infect disease (2000) 36(1):19–36. doi: 10.1016/S0732-8893(99)00092-9
97. Jacobus N, McDermott L, Ruthazer R, Snydman D. In vitro activities of tigecycline against the Bacteroides fragilis group. Antimicrobial Agents chemotherapy (2004) 48(3):1034–6. doi: 10.1128/AAC.48.3.1034-1036.2004
98. Petersen PJ, Jacobus N, Weiss W, Sum P, Testa R. In vitro and in vivo antibacterial activities of a novel glycylcycline, the 9-t-butylglycylamido derivative of minocycline (GAR-936). Antimicrobial Agents chemotherapy (1999) 43(4):738–44. doi: 10.1128/AAC.43.4.738
99. Edlund C, Nord C. In-vitro susceptibility of anaerobic bacteria to GAR-936, a new glycylcycline. Clin Microbiol infection (2000) 6(3):158. doi: 10.1046/j.1469-0691.2000.00034-6.x
100. Babinchak T, Grosse EE, Dartois N, Rose GM, Loh E, , Tigecycline 301 and 306 Study Groups. The efficacy and safety of tigecycline for the treatment of complicated intra-abdominal infections: analysis of pooled clinical trial data. Clin Infect Diseases (2005) 41(Supplement_5):S354–S67. doi: 10.1086/431676
101. Grosse EE, Babinchak T, Dartois N, Rose G, Loh E, , Tigecycline 301 and 306 Study Groups, et al. The efficacy and safety of tigecycline in the treatment of skin and skin-structure infections: results of 2 double-blind phase 3 comparison studies with vancomycin-aztreonam. Clin Infect diseases (2005) 41(Supplement_5):S341–S53. doi: 10.1086/431675
102. Da Silva LM, Salgado HRN. Validation of a stability-indicating RP-LC method for the determination of tigecycline in lyophilized powder. J chromatographic science (2013) 51(2):192–9. doi: 10.1093/chromsci/bms126
103. Karlowsky JA, Laing NM, Zhanel GG. In vitro activity of OPT-80 tested against clinical isolates of toxin-producing Clostridium difficile. Antimicrobial Agents chemotherapy (2008) 52(11):4163–5. doi: 10.1128/AAC.00476-08
104. Shue Y, Sears P, Shangle S, Walsh R, Lee C, Gorbach S, et al. Safety, tolerance, and pharmacokinetic studies of OPT-80 in healthy volunteers following single and multiple oral doses. Antimicrobial Agents chemotherapy (2008) 52(4):1391–5. doi: 10.1128/AAC.01045-07
105. Zhanel GG, Walkty AJ, Karlowsky JA. Fidaxomicin: a novel agent for the treatment of Clostridium difficile infection. Can J Infect Dis Med Microbiol (2015) 26(6):305–12. doi: 10.1155/2015/934594
106. Dalhoff A, Rashid M-U, Kapsner T, Panagiotidis G, Weintraub A, Nord C. Analysis of effects of MCB3681, the antibacterially active substance of prodrug MCB3837, on human resident microflora as proof of principle. Clin Microbiol Infection (2015) 21(8):767. e1–. e4. doi: 10.1016/j.cmi.2015.05.025
107. Rashid M-U, Dalhoff A, Weintraub A, Nord CE. In vitro activity of MCB3681 against Clostridium difficile strains. Anaerobe (2014) 28:216–9. doi: 10.1016/j.anaerobe.2014.07.001
108. Dieterle MG, Rao K, Young VB. Novel therapies and preventative strategies for primary and recurrent Clostridium difficile infections. Ann New York Acad Sci (2019) 1435(1):110–38. doi: 10.1111/nyas.13958
109. Cunha BA, Sibley CM, Ristuccia AM. Doxycycline. Ther Drug monitoring (1982) 4(2):115. doi: 10.1097/00007691-198206000-00001
110. Holmes NE, Charles PG. Safety and efficacy review of doxycycline. Clin Med Ther (2009) 1:CMT-S2035. doi: 10.4137/CMT.S2035
111. Brewster R, Tamburini FB, Asiimwe E, Oduaran O, Hazelhurst S, Bhatt AS. Surveying gut microbiome research in Africans: toward improved diversity and representation. Trends Microbiol (2019) 27(10):824–35. doi: 10.1016/j.tim.2019.05.006
112. De Filippo C, Cavalieri D, Di Paola M, Ramazzotti M, Poullet JB, Massart S, et al. Impact of diet in shaping gut microbiota revealed by a comparative study in children from Europe and rural Africa. Proc Natl Acad Sci (2010) 107(33):14691–6. doi: 10.1073/pnas.1005963107
113. Schnorr SL, Candela M, Rampelli S, Centanni M, Consolandi C, Basaglia G, et al. Gut microbiome of the Hadza hunter-gatherers. Nat Commun (2014) 5(1):3654. doi: 10.1038/ncomms4654
114. De Filippo C, Di Paola M, Ramazzotti M, Albanese D, Pieraccini G, Banci E, et al. Diet, environments, and gut microbiota. A preliminary investigation in children living in rural and urban Burkina Faso and Italy. Front Microbiol (2017) 8:1979. doi: 10.3389/fmicb.2017.01979
115. Ayeni FA, Biagi E, Rampelli S, Fiori J, Soverini M, Audu HJ, et al. Infant and adult gut microbiome and metabolome in rural Bassa and urban settlers from Nigeria. Cell Rep (2018) 23(10):3056–67. doi: 10.1016/j.celrep.2018.05.018
116. Mosca A, Leclerc M, Hugot JP. Gut microbiota diversity and human diseases: should we reintroduce key predators in our ecosystem? Front Microbiol (2016) 7:455. doi: 10.3389/fmicb.2016.00455
117. Nasiri MJ, Goudarzi M, Hajikhani B, Ghazi M, Goudarzi H, PourIran R. Clostridioides (Clostridium) difficile infection in hospitalized patients with antibiotic-associated diarrhea: A systematic review and meta-analysis. Anaerobe (2018) 50:32–7. doi: 10.1016/j.anaerobe.2018.01.011
118. Santacroce L, Inchingolo F, Topi S, Del Prete R, Di Cosola M, Charitos IA, et al. Potential beneficial role of probiotics on the outcome of COVID-19 patients: An evolving perspective. Diabetes Metab Syndrome: Clin Res Rev (2021) 15(1):295–301. doi: 10.1016/j.dsx.2020.12.040
119. Dinleyici EC, Eren M, Ozen M, Yargic ZA, Vandenplas Y. Effectiveness and safety of Saccharomyces boulardii for acute infectious diarrhea. Expert Opin Biol Ther (2012) 12(4):395–410. doi: 10.1517/14712598.2012.664129
120. Servin AL. Antagonistic activities of lactobacilli and bifidobacteria against microbial pathogens. FEMS Microbiol Rev (2004) 28(4):405–40. doi: 10.1016/j.femsre.2004.01.003
121. Akbari V, Hendijani F. Effects of probiotic supplementation in patients with type 2 diabetes: systematic review and meta-analysis. Nutr Rev (2016) 74(12):774–84. doi: 10.1093/nutrit/nuw039
122. McFarland LV. Antibiotic-associated diarrhea: epidemiology, trends and treatment. Future Microbiology (2008) 3(5):563–578. doi: 10.2217/17460913.3.5.563
123. Cleusix V, Lacroix C, Vollenweider S, Duboux M, Le Blay G. Inhibitory activity spectrum of reuterin produced by Lactobacillus reuteri against intestinal bacteria. BMC Microbiol (2007) 7(1):1–9. doi: 10.1186/1471-2180-7-101
124. Beaugerie L, Flahault A, Barbut F, Atlan P, Lalande V, Cousin P, et al. Antibiotic-associated diarrhoea and Clostridium difficile> in the community. Alimentary Pharmacol Ther (2003) 17(7):905–12. doi: 10.1046/j.1365-2036.2003.01531.x
125. Szajewska H, Canani RB, Guarino A, Hojsak I, Indrio F, Kolacek S, et al. Probiotics for the prevention of antibiotic-associated diarrhea in children. J Pediatr Gastroenterol Nutr (2016) 62(3):495–506. doi: 10.1097/MPG.0000000000001081
126. Neut C, Mahieux S, Dubreuil L. Antibiotic susceptibility of probiotic strains: Is it reasonable to combine probiotics with antibiotics? Medecine maladies infectieuses (2017) 47(7):477–83. doi: 10.1016/j.medmal.2017.07.001
127. Armstrong ES, Miller GH. Combating evolution with intelligent design: the neoglycoside ACHN-490. Curr Opin Microbiol (2010) 13(5):565–73. doi: 10.1016/j.mib.2010.09.004
128. Landman D, Kelly P, Bäcker M, Babu E, Shah N, Bratu S, et al. Antimicrobial activity of a novel aminoglycoside, ACHN-490, against Acinetobacter baumannii and Pseudomonas aeruginosa from New York City. J Antimicrobial Chemotherapy (2011) 66(2):332–4. doi: 10.1093/jac/dkq459
129. Cass RT, Brooks CD, Havrilla NA, Tack KJ, Borin MT, Young D, et al. Pharmacokinetics and safety of single and multiple doses of ACHN-490 injection administered intravenously in healthy subjects. Antimicrobial Agents chemotherapy (2011) 55(12):5874–80. doi: 10.1128/AAC.00624-11
130. Abbanat D, Morrow B, Bush K. New agents in development for the treatment of bacterial infections. Curr Opin Pharmacol (2008) 8(5):582–92. doi: 10.1016/j.coph.2008.08.001
131. Paknikar SS, Narayana S. Newer antibacterials in therapy and clinical trials. North Am J Med Sci (2012) 4(11):537. doi: 10.4103/1947-2714.103312
132. van Rensburg DJ, Perng R-P, Mitha IH, Bester AJ, Kasumba J, Wu R-G, et al. Efficacy and safety of nemonoxacin versus levofloxacin for community-acquired pneumonia. Antimicrobial Agents chemotherapy (2010) 54(10):4098–106. doi: 10.1128/AAC.00295-10
133. Podos SD, Thanassi JA, Leggio M, Pucci MJ. Bactericidal activity of ACH-702 against nondividing and biofilm Staphylococci. Antimicrobial Agents chemotherapy (2012) 56(7):3812–8. doi: 10.1128/AAC.00092-12
134. Devasahayam G, Scheld WM, Hoffman PS. Newer antibacterial drugs for a new century. Expert Opin investigational Drugs (2010) 19(2):215–34. doi: 10.1517/13543780903505092
135. Betriu C, Morales G, Rodríguez-Avial I, Culebras E, Gómez M, López-Fabal F, et al. Comparative activities of TR-700 (torezolid) against staphylococcal blood isolates collected in Spain. Antimicrobial Agents chemotherapy (2010) 54(5):2212–5. doi: 10.1128/AAC.01653-09
136. Dunbar LM, Tang DM, Manausa RM. A review of telavancin in the treatment of complicated skin and skin structure infections (cSSSI). Ther Clin Risk Management (2008) 4(1):235–44. doi: 10.2147/TCRM.S1843
137. Guskey MT, Tsuji BT. A comparative review of the lipoglycopeptides: oritavancin, dalbavancin, and telavancin. Pharmacotherapy: J Hum Pharmacol Drug Ther (2010) 30(1):80–94. doi: 10.1592/phco.30.1.80
Keywords: antimicrobial agents, intestinal microbiota, gut microbiota, eubiosis, dysbiosis, probiotics, systematic review
Citation: Girma A (2023) The effect of novel antimicrobial agents on the normal functioning of human intestinal microbiota: a systematic review. Front. Gastroenterol. 2:1159352. doi: 10.3389/fgstr.2023.1159352
Received: 05 February 2023; Accepted: 25 July 2023;
Published: 16 August 2023.
Edited by:
Santanu Chattopadhyay, Rajiv Gandhi Center for Biotechnology, IndiaCopyright © 2023 Girma. This is an open-access article distributed under the terms of the Creative Commons Attribution License (CC BY). The use, distribution or reproduction in other forums is permitted, provided the original author(s) and the copyright owner(s) are credited and that the original publication in this journal is cited, in accordance with accepted academic practice. No use, distribution or reproduction is permitted which does not comply with these terms.
*Correspondence: Abayeneh Girma, Z2FiYXllbmVoMjAxM0BnbWFpbC5jb20=