- 1Medical Genetics Institute of Henan Province, Henan Provincial Key Laboratory of Genetic Diseases and Functional Genomics, Henan Provincial People’s Hospital, People’s Hospital of Zhengzhou University, Zhengzhou University, Zhengzhou, China
- 2National Health Commission Key Laboratory of Birth Defects Prevention, Zhengzhou, China
- 3Reproduction Medical Center, Henan Provincial People’s Hospital, Zhengzhou University People’s Hospital, Zhengzhou, China
The innovation in ultrasound has greatly promoted the prenatal diagnosis of ventricular septal defect. As a minor lesion of congenital heart disease, the prenatal genetic counseling of isolated ventricular septal defect faces some challenges, including the true genetic correlationship, selection of appropriated testing methods to identify deleterious mutations, and avoidance of overdiagnosis and overintervention. Researchers have explored the prenatal diagnosis efficiency of commonly used cytogenetic and molecular genetic technologies. Small insertions/deletions and monogenic variants with phenotypic heterogeneity play important role and contribute to the comprehend of pathogenesis. Isolated ventricular septal defect fetuses without genetic finding and extracardiac structural abnormality generally have good pregnancy outcome. Long-term follow-up data is needed to describe the comprehensive map, such as the potential missed diagnosis especially late-onset syndromes, the impact on the quality of life and life expectancy. When conducting prenatal genetic counseling, strict adherence to ethical principles is needed to ensure that the rights of all parties involved are fully protected. Clinicians should carefully evaluate the risks and benefits and provide parents with sufficient information and advice to enable them to make informed decisions.
1 Introduction
Congenital heart disease (CHD) is the most common birth defect and leading cause of global burden of disease (Authoranonmys, 2020; Roth et al., 2020). As the most frequent type of CHD, ventricular septal defect (VSD) has intricate etiology and Pathogenesis (Nora, 1968; Goor et al., 1970; Pierpont et al., 2007). Sonography is the primary imaging modality for diagnosing and monitoring VSDs. Over the past 30 years, the development and growth of fetal echocardiography has been driven by technical innovation (Rajiah et al., 2011; Maulik et al., 2017). The resolution improvement and operation standardization have pushed the boundaries of what can be seen and measured, and helped the advance period of diagnosis for small VSDs to fetal stage, resulted in the intense demand for prenatal counseling (van der Linde et al., 2011; Yagel et al., 2011; Boehme et al., 2022; Dawood et al., 2022). Genetics plays an important role in the pathogenesis of VSD, and this discovery benefits from the great technological progress in human genome research. The aim of this review is to explain the genetic association and clinical outcomes for fetuses with isolated VSD, so as to provide comprehensive basis for prenatal genetic counseling.
2 Search strategy and selection criteria
Articles referenced in this manuscript were identified by MEDLINE and EMBASE. The last electronic search was performed on 13 September 2024 utilizing combinations of the relevant medical subject heading (MeSH) terms, word variants, and keywords for “fetus, prenatal, isolated and ventricular septal defect”. The bibliography of high-impact articles were reviewed to identify additional relevant studies and was included in our references when appropriate.
Additional articles were included to evaluate the risk of genetic abnormalities and clinical outcomes of fetuses with isolated VSD if the following criteria were met (Figure 1): (1) fetuses were diagnosed as isolated VSD by prenatal ultrasound, (2) the study was conducted solely on human fetuses, and (3) the study contained information about genetic testing results or prognosis information. Cohort, retrospective, prospective, and longitudinal articles were included. Case reports, conference abstracts, comments and editorials were excluded. Pediatric, postnatal, adult and autopsybased studies were excluded. Articles in which isolated VSD category were included but could not be separated from others were excluded. To avoid duplication, the article with the longest study period or largest sample size was selected for final analysis in articles with overlapping locations and periods.
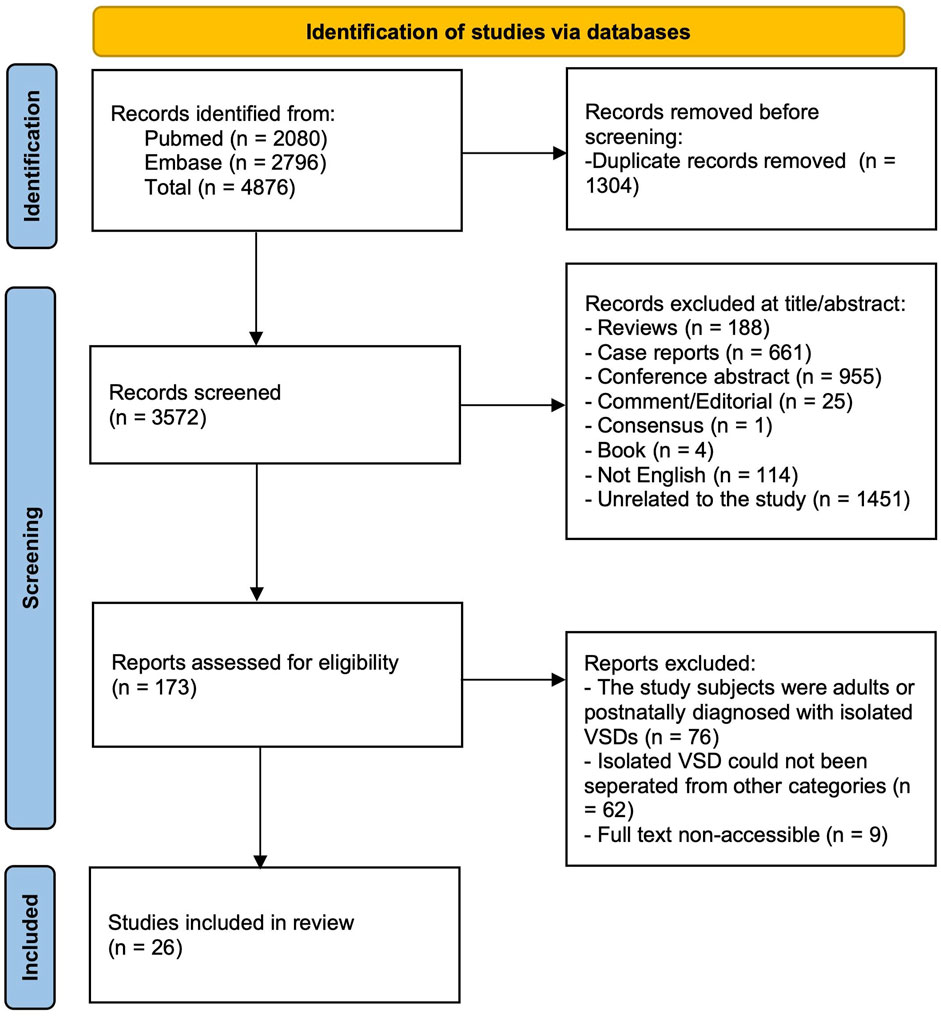
Figure 1. Flow chart of included articles to evaluate the risk of genetic abnormalities and clinical outcomes of fetuses with isolated VSD.
3 Prevalence
A meta-analysis of 260 studies that incorporated global data concluded that the reported birth prevalence of CHD globally continues to increase. Among the total CHD, the three mild lesions including VSD (occurred in 3.1 per 1,000 birth), atrial septal defect (ASD) and patent ductus arteriosus (PDA) together explained 93.4% of the increase prevalence (Liu et al., 2019). Although there are significant regional discrepancies in the incidence of VSD, this trend were also observed in the studies of Sweden and China, with a VSD birth prevalence of 7 per 1,000 and 1.4 per 1,000 respectively (Zhao et al., 2020a; Giang et al., 2023). Considering impact of the detection rate, intervention and spontaneous closure of the defect, prenatal statistics may vary from postnatal data. In a recent large-scale screening project of Chinese population, the incidence of isolated single VSD was 1.7 per 1,000 among pregnancies between 18 and 22 weeks’ gestational age nationwide, significantly higher than 0.1 per 1,000 of non-isolated VSD (Chen et al., 2023). Another register-based study from Denmark retreved nationwide data from routine ultrasound screening over a five-year period and found an incidence rate of isolated VSD of 0.5 per 1,000 low-risk pregnancies (Vedel et al., 2021).
4 Genetic association
The causes and pathological mechanisms of VSD serve as core information for assessing fetal prognosis, managing pregnancy, and parental decision-making. Clinicians should assess risk factors and discuss testing options in prenatal genetic counseling. Karyotype analysis is the gold standard for chromosomal abnormalities and the first-line method for prenatal diagnosis. However, it is controversial at present about the association between isolated VSD and the risk of chromosomal abnormality, thus puzzles the pre-test clinical counseling (Gómez et al., 2014; Shen et al., 2014; Lee et al., 2016; Svirsky et al., 2019; Vedel et al., 2021). The existence of additional risk indicators beyond isolated VSD may be the primary reason for the inconsistent genetic detection rates, as is shown in Tables 1, 2. In addition, geographical and socio-economic factors also lead to the deviation of the incidence. On the basis of conventional karyotype analysis, chromosomal microarray analysis (CMA) and exome sequencing (ES) have become more common in the prenatal setting in recent years. It also brings challenges for clinicians to choose approproate prenatal testing methods.
4.1 Chromosomal abnormality in isolated VSD
In early years, prenatal sonographic diagnosis of VSD was difficult. Large VSD was usually detected by prenatal ultrasound with cardiac and extracardiac abnormalities, and karyotype analysis was the most effective available detection method at that time. In the year of 2000 and 2006, Paladini et al. and Axt-fliedner et al. recruited fetal VSDs without associated cardiac defect and obtained high detection yields 26.67% and 32.89% of chromosomal abberation by karyotype analysis (Paladini et al., 2000; Axt-Fliedner et al., 2006). Trisomy 18 was the most common chromosomal aberration in fetal VSD without associated cardiac defect, followed by trisomy 21 and trisomy 13. Paladini et al. also reported a strong correlation between trisomy 21 and inlet VSD, as well as trisomy 18 and malalignment VSD (Paladini et al., 2000). Till the era of first-trimester screening, aneuploid was less prevalent in the cohort of isolated VSD without associated cardiac abnormalities. Li et al. from China performed karyotyping in 80 cases and identified 9 chromosomal anomalies, including 8 aneuploids and 1 balanced translocation (Li et al., 2016). Another study in East Asian population reported deleterious derivative chromosomes but no aneuploid in 4 fetuses (Cho et al., 2017). However, in these studies, the presence of extracardiac abnormalities has raised doubts about the association between chromosomal aberration and isolated VSD.
Some researchers concerned about the risk of fetal genetic abnormalities increased by isolated VSD itself and excluded VSD with extracardiac abnormalities from the prenatal cohort. During this period, sequential prenatal diagnostic method of cytogenetics and molecular genetics was widely adopted. Some authors believe that ultrasound soft markers woud not influence the postnatal cardiac management of VSD. Cheng et al. analyzed 170 invasive samples combining quantitative fluorescent polymerase chain reaction (QF-PCR), karyotyping analysis and CMA. Two aneuploids and seven copy number variations (CNVs) were identified, with detection rate of 1.18% for chromosomal aberration and 4.12% for CNV(Cheng et al., 2022). Interestingly, all of the positive variants were found in perimembranous VSD (Supplementary Table S1) (Cheng et al., 2022). Another large-scale study by Gómez et al. perfomed karyotyping and fluorescence in situ hybridization (FISH) in 119 amniotic fluid samples and clinically assessed karyotype postnatally in 129 cases, and obtained a total diagnostic yield of 3.13% in perimembranous VSD and 0.93% in muscular VSD. Gómez et al. concluded that perimembranous VSD was associated with a higher risk of chromosomal anomalies than muscular VSD and muscular VSD had a similar risk to those of normal pregnancies (Gómez et al., 2014). Qiao et al. also reported a total 16.66% detection rate of 17 aneuploides and CNVs in 102 isolated VSDs by CMA (Qiao et al., 2021).
Other researchers set rigorous inclusion criteria. In the cohort studies of isolated VSD without cardiac, extracardiac abnormalities or ultrasound soft markers, Fu et al. and Du et al. reported CMA diagnostic rates of 5.48% and 4.55% on the basis of normal karyotype, respectively (Du et al., 2016; Fu et al., 2017). These results also demonstrated the value of CMA in the prenatal diagnosis of isolated VSD (Du et al., 2016; Fu et al., 2017). However, such conclusion was questioned by Maya et al. (2020). In Israel, routinely reported CMA analysis supported by the Ministry of Health is performed for sonographic defect, allowing for a comparison among additional risk factors. In a cohort of 691 cases, 8 abnormal CMA results were found in 568 isolated VSD (1.4%), compared with 1 in 20 (5%) VSD with soft markers, 15 in 82 (18.3%) VSD with additional major anomalies and 2 in 21 (9.5%) VSD with growth or amniotic fluid index anomalies. Maya et al. suggested that the rate of abnormal CMA findings in isolated VSD was not different from pregnancies with normal ultrasound (Maya et al., 2020).
4.2 Sequence variant in isolated VSD
Chinese researchers have also made some attempts on the prenatal application of ES in isolated VSD. Qiao et al. conducted ES over CMA for fetuses with isolated VSD, resulted in an additional 5.6% diagnostic yield of sequence variant (SV). Among the 6 cases with SVs, 3 fetuses without additional abnormalities were detected to have genetic syndromes (Qiao et al., 2021). Wang et al. reported a likely pathogenic variation from 12 isolated VSD samples detected by ES (Wang et al., 2023c).
5 Prenatal genetic testing
5.1 Karyotyping analysis
In the overall prenatal diagnostic environment, karyotype analysis with high cost-effective ratio is often the first-line and irreplaceable choice for detection. For fetuses with VSD accompanied by other structural abnormalities, karyotype analysis is an appropriate option. For other clinical situations, it seems that additional indicators should be more considered in the risk assessment of chromosome abnormality, since isolated VSD itself does not increase or only slightly increases the risk of aneuploid. As a minor form of CHD, isolated VSD appears to be more associated with small genomic deletion and insertion, and monogenic disorders that have a insidious onset and non-fatal phenotype. However, A phenotypic shift has been reported in infants birth with Down syndrome from complex CHD to isolated VSD or ASD in recent years (Bergström et al., 2016). Chau et al. reported a trisomy 18 in isolated VSD fetus without any extracardiac defect or complications, diagnosed in the gestational age of 17+5 weeks (Chau et al., 2018). With the development of maternal-fetal medicine and improvement of prenatal care, it is possible that fetal phenotypes in the future may tend to be milder, and in this case, the proportion of chromosomal abnormalities may increase accordingly.
5.2 Chromosomal microarray analysis
CMA has been already confirmed as valuable tool and recommended to be used as the first-line genetic diagnostic testing for investigating the causes of fetal CHD (Thienpont et al., 2007; Southard et al., 2012; Mastromoro et al., 2022). Although authors disputed about the application of CMA in isolated VSD, CMA seems to be more efficient than karyotyping. Compared with karyotype analysis, CMA has high resolution and short turnaround, and was capable of identifying both chromosomal aneuploids and CNVs prenatally (Sukenik-Halevy et al., 2016; Zhu et al., 2016).
The deletion of 22q11.2 was the most common CNV identified in isolated VSD, while other CNVs occur in a sporadic form, such as 1q21.1 duplication and 16p11.2 duplication (Supplementary Table S2). With the features of reduced penetrance and pleiotropic effects, these CNVs in the genomic hotspots were reported to be enriched in CHD cohorts and affect dosage-sensitive genes that were required for cardiac development (Ehrlich and Prakash, 2022). Cheng et al. recommended CMA for fetal isolated VSD especially perimembranous subtype (Cheng et al., 2022). Besides, CMA also have clinical efficacy in exploring the etiology and pathogenesis of isolated VSD. Fu et al. identified potential candidate genes of VSD including FAT1 and ULK1 through prenatal testing by CMA (Fu et al., 2017). In some centers, array analysis has become a standard procedure for prenatal genetic analysis, and it is commonly preceded by rapid aneuploidy detection to exclude common aneuploidies, which can be an effective alternative to cytogenetics.
5.3 Exome sequencing
In recent years, ES has becoming a robust tool for prenatal diagnositic applications of CHD (Jin et al., 2017; Lord et al., 2019; Petrovski et al., 2019). Monogenic defects are known causitive factors for isolated VSD in the young (Pierpont et al., 2007). Pedigree studies have identified critical genes encoding important constituents of signaling pathways involved in controlling heart development. For these reasons, ES was also utilized in isolated VSD prenatally. In the two existing studies, desirable diagnostic increments were obtained by the application of ES (Qiao et al., 2021; Wang et al., 2023c). In addition, deleterious SVs in isolated VSD fetuses have been described in several CHD cohort studies (Fu et al., 2018; Li et al., 2020; Yi et al., 2023).
The interactions of multiple cardiac genes and their activation by up stream inductive signals maintain the cardiac phenotype (Chaithra et al., 2022). To date, researchers have mapped three gene loci (GATA4, OMIM#600576; CITED2, OMIM#602937; NKX2.5, OMIM#600584) related to the phenotype of VSD using sequencing technology (Table 3) (Sperling et al., 2005; Zhang et al., 2008; Peng et al., 2010). These gene variants lead to varying degrees of familial VSD with or without other cardiac abnormalities, such as ASD and pulmonary hypertension (Sperling et al., 2005; Chen et al., 2010; Wang et al., 2011a; Wang et al., 2011b; Yang et al., 2012). In addition, several genetic syndromes with varying severity and phenotypic heterogeneity, including Noonan syndrome, Kabuki syndrome, CHARGE syndrome and Holt-Oram syndrome, have been reported in fetal isolated VSD (Fu et al., 2018; Li et al., 2020; Qiao et al., 2021; Wang et al., 2023c; Yi et al., 2023). Due to the unintuitive physical characteristics of prenatal ultrasound and late onset phenotype of some syndromes, especially those with neurodevelpmental and psychiatric characteristics, isolated symptoms may be the only indication for those fetuses (Kabra and Gulati, 2003; Wang et al., 2020b).
As concluded by Wang et al., ES can be reccommended for fetuses with VSD without chromosome abnormalities and pathogenic CNV (Wang et al., 2023c). Objectively, ES may have the potential to replace CMA and as a first-line diagnostic tool. Methods for calling CNV from ES data have been widely developed and in the process of clinical optimization, which expand the clinical practice by ES (D'Aurizio et al., 2016; Zhao et al., 2020b; Testard et al., 2022; Babadi et al., 2023). As the decline in costs and accumulation of data, it was undisputed that ES would be applied extensively in fetal isolated VSD in the following years.
5.4 Prenatal genetic screening
Lee et al. have attempted to investigate the impact of abnormal maternal serum screening on the detection rate of chromosomal abnormalities in isolated VSD (Lee et al., 2016). Among the two isolated VSD with trisomy 21, one has a high risk of serum screening and the other has not reveived the test (Lee et al., 2016). A few studies have also involved the contribution of non-invasive prenatal testing (NIPT) in prenatal finding of isolated VSD. In a single-center cohort study, 9 cases with high risk of chromosomal abnormalities were identified by NIPT from a total of 125 fetuses with isolated VSD (Selhorst et al., 2024). Despite the lack of detailed corresponding genetic results, their results indicated the NIPT has promoted the early prevention of chromosome-related VSD (Selhorst et al., 2024). Zhao et al. discussed that fetuses with isolated VSD and low-risk of NIPT might not need invasive prenatal diagnosis because no genetic variant was found in 45 NIPT low-risk isolated VSD fetuses by karyotype and CNV-seq in their study (Zhao et al., 2024). However, a case of neonatal death due to metabolic disease has not been reasonably explained which might mean the potential missed diagnosis of monogenic disease. The exploration of NIPT for monogenic diseases is under way. Due to the variable severity and phenotypic heterogeneity, it is possible to discuss in the future whether VSD-related genes can be included in the NIPT for monogenic diseases.
6 Spontaneously closure, pregnancy outcome and long-term follow-up
Most of the isolated VSDs detected by fetal ultrasound could close spontaneously during pregnancy and infancy (Table 4). Size, site and maternal age are prognostic factors for the natural closure of the defect (Paladini et al., 2000; Cho et al., 2017; Cheng et al., 2022; Gordin Kopylov et al., 2022). The spontaneous closure mechanisms of muscular and perimembranous isolated VSD were different and have been well elaborated, as reviewed by Miyake (Miyake, 2020). For fetuses, authors regard muscular isolated VSD as benign finding, while perimembranous VSD tends to have larger size, lower closure rates, and more likely require treatment by medications, special care or surgery to thrive (Gómez et al., 2014; Li et al., 2016; Cho et al., 2017; Svirsky et al., 2019; Cheng et al., 2022; Raucher Sternfeld et al., 2022; Zhao et al., 2024). Li et al. also found a higher spontaneous closure rate in male than female (Li et al., 2016).
Remarkably, as evidenced by the findings of Selhorst et al., genetic variations manifested with a heightened prevalence in infants whose VSD underwent spontaneous closure in utero, compared to infants who exhibited persistent VSD (Selhorst et al., 2024). Genetic abnormalities and extracardiac abnormalities are the main reasons for termination of pregnancy (TOP) and adverse pregnancy outcomes (Table 5). Most of the other isolated VSD fetuses have a positive pregnancy outcome and no need for surgery. A few referral to pediatric surgical department usually have low operative mortality and good prognosis when treated in a timely manner (Nurmi et al., 2022). However, it has also been reported that unrepaired and surgically closed isolated VSD affected the long-term survival of patients and was prone to late complications (Eckerström et al., 2023). The recent advances in minimally invasive treatment options including periventricular approach and transcatheter techniques have improved patient outcomes, yet at the expense of higher residual rates (Adan et al., 2021). Right ventricular function and exercise capacity were found impaired in VSD patients, and post-surgical outcome in these patients may be less benign than presently assumed (Nederend et al., 2018; Eckerström et al., 2020; Adan et al., 2021).
Several individuals with isolated VSD have developed extra abnormalities during late pregnancy to infancy, such as esophageal atresia, coarctation of the aorta and pulmonary stenosis, which suggested that clinicians should monitor the associated abnormalities in the subsequent period (Bensemlali et al., 2017; Fu et al., 2017; Cheng et al., 2022; Raucher Sternfeld et al., 2022). A specific link between VSD and central nervous system anomalies has been described by Huang et al., but the detailed mechanisms underlying the breadth of co-occurring anomalies have yet to be delineated (Huang et al., 2023). Due to the limitation of follow-up time and retrospective study exclusion criteria, the results of this aspect have not been well-described in the prenatal cohort. Noncardiac anomalies are crucial for perioperative management and etiology study, and can also increase the risk of postoperative complications, such as respiratory complications with heterotaxy (Swisher et al., 2011; Harden et al., 2014). The complete record including mild and late-onset phenotypes, especially neuropsychiatric symptoms by long-term follow-up, was important for the forward evaluation of prognosis.
7 Prenatal counseling
The term “isolated VSD” was originally defined anatomically limited to the heart, referring to VSD without other cardiac defect (e.g. ASD) or as part of complex CHD (e.g. tetralogy of Fallot). However, in the prenatal setting, clinicians need to comprehensively evaluate the fetal prognosis based on the overall pregnancy to assess the risk. The risk brought by each indicator and the combined risk of multiple indicators should be deeply concerned.
The associated genetic abnormalities strongly influences the parent’s decision to choose postnatal compassionate care or TOP. CNV and SV with phenotypic heterogeneity and incomplete penetrance were more likely to cause fetal isolated VSD than chromosomal aberration. Clinicians should be aware of the benefits and implications of the responsible use of genomics. At the same time, issues raised by the prenatal detection of possible complex disorders from mild phenotypes should be taken into account:
- Balance of information disclosure. Clinicians should provide sufficient information to help parents understand the risk of disease, while ensuring that they do not unduly worry them, that is, balancing the completeness of the information with the parents’ ability to cope.
- Variants of unknown significance (VOUS). Clinicians should fully communicate with the parents to ensure that they understand the significance and limitations of the test results, while avoiding over-interpretation or misleading. It is necessary to set appropriate informed consent before testing. Researches are sometimes needed to verify the clinical significance, and it must follow the principles of medical ethics, ensuring it conforms to ethical standard and the informed consent from parents is obtained.
- Parental decision-making pressure. Clinicians should ensure that parents fully understand the genetic nature and associated risks of isolated VSD, so that they can make informed decisions. However, this may also place excessive decision-making pressure on parents, making it difficult to make a choice.
- Rights of the fetus. When considering the wishes and needs of the parents, it is also necessary to fully protect the rights of the fetuses, including their right to health and future autonomous decision-making.
- Overdiagnosis and overintervention. In some cases, parents may request excessive diagnosis or intervention, which may pose unnecessary risks to the fetus. Clinicians should carefully assess these risks and ensure that parents’ decisions are based on sufficient medical evidence.
- Resource allocation. As genetic counseling and testing may require significant medical resources and funding, clinicians need to ensure that these resources are allocated fairly and reasonably, so that all those need it can receive necessary services.
- Legal and ethical framework. Clinicians should understand and comply with relevant laws and ethical frameworks to ensure that their actions meet legal requirements and ethical standards. This includes respecting the rights of parents, protecting the rights of the fetus, and ensuring the confidentiality of information.
8 Future prospective
Advances in genomic technology reshape the practice of prenatal counseling in isolated VSD. Precision genome-wide detection will play a role in prenatal diagnosis, and further reveal the genetic mechanism of isolated VSD in the future. Seeking biomarkers for the prediction of fetal VSD is another research orientation in the assessment of health and disease. VSD-ralated specific IncRNAs and microRNAs have been authenticated in maternal serum and expected to serve as prenatal VSD diagnostic markers (Jin et al., 2021; Yang et al., 2022; Wang et al., 2023a; Wang et al., 2023b). Another differentially expressed protein CFHR4 was also identified as a promising biomarker (He et al., 2022). More omics analysis are expected to accurately predict the risk of VSD in fetuses, and thus providing basis for early intervention and treatment.
Author contributions
XC: Conceptualization, Data curation, Funding acquisition, Investigation, Methodology, Visualization, Writing–original draft. QZ: Data curation, Funding acquisition, Investigation, Writing–original draft. ML: Visualization, Writing–original draft. Qiuxia Feng: Project administration, Writing–original draft. LQ: Conceptualization, Methodology, Supervision, Writing–review and editing. SL: Conceptualization, Methodology, Supervision, Writing–review and editing.
Funding
The author(s) declare that financial support was received for the research, authorship, and/or publication of this article. This work was supported by the Open Source Project from the NHC Key Laboratory of Birth Defects Prevention (ZD202307), Science and Technology Research Project of Henan Province (232102310255) and Medical Science and Technology Research Project (LHGJ20190593).
Conflict of interest
The authors declare that the research was conducted in the absence of any commercial or financial relationships that could be construed as a potential conflict of interest.
Publisher’s note
All claims expressed in this article are solely those of the authors and do not necessarily represent those of their affiliated organizations, or those of the publisher, the editors and the reviewers. Any product that may be evaluated in this article, or claim that may be made by its manufacturer, is not guaranteed or endorsed by the publisher.
Supplementary material
The Supplementary Material for this article can be found online at: https://www.frontiersin.org/articles/10.3389/fgene.2024.1447216/full#supplementary-material
References
Adan, A., Eleyan, L., Zaidi, M., Ashry, A., Dhannapuneni, R., and Harky, A. (2021). Ventricular septal defect: diagnosis and treatments in the neonates: a systematic review. Cardiol. Young 31 (5), 756–761. doi:10.1017/S1047951120004576
Axt-Fliedner, R., Schwarze, A., Smrcek, J., Germer, U., Krapp, M., and Gembruch, U. (2006). Isolated ventricular septal defects detected by color Doppler imaging: evolution during fetal and first year of postnatal life. Ultrasound Obstetrics & Gynecol. Official J. Int. Soc. Ultrasound Obstetrics Gynecol. 27 (3), 266–273. doi:10.1002/uog.2716
Babadi, M., Fu, J. M., Lee, S. K., Smirnov, A. N., Gauthier, L. D., Walker, M., et al. (2023). GATK-gCNV enables the discovery of rare copy number variants from exome sequencing data. Nat. Genet. 55 (9), 1589–1597. doi:10.1038/s41588-023-01449-0
Baban, A., Olivini, N., Lepri, F. R., Calì, F., Mucciolo, M., Digilio, M. C., et al. (2019). SOS1 mutations in Noonan syndrome: cardiomyopathies and not only congenital heart defects! Report of six patients including two novel variants and literature review. Am. J. Med. Genet. Part A 179 (10), 2083–2090. doi:10.1002/ajmg.a.61312
Bahtiyar, M. O., Dulay, A. T., Weeks, B. P., Friedman, A. H., and Copel, J. A. (2008). Prenatal course of isolated muscular ventricular septal defects diagnosed only by color Doppler sonography: single-institution experience. J. Ultrasound Med. Official J. Am. Inst. Ultrasound Med. 27 (5), 715–720. doi:10.7863/jum.2008.27.5.715
Bensemlali, M., Bajolle, F., Laux, D., Parisot, P., Ladouceur, M., Fermont, L., et al. (2017). Neonatal management and outcomes of prenatally diagnosed CHDs. Cardiol. Young 27 (2), 344–353. doi:10.1017/S1047951116000639
Bergström, S., Carr, H., Petersson, G., Stephansson, O., Bonamy, A.-K. E., Dahlström, A., et al. (2016). Trends in congenital heart defects in infants with Down syndrome. Pediatrics 138 (1), e20160123. doi:10.1542/peds.2016-0123
Boehme, C., Fruitman, D., Eckersley, L., Low, R., Bennett, J., McBrien, A., et al. (2022). The diagnostic yield of fetal echocardiography indications in the current era. J. Am. Soc. Echocardiogr. 35 (2), 217–227.e1. doi:10.1016/j.echo.2021.09.005
Cai, M., Huang, H., Su, L., Lin, N., Wu, X., Xie, X., et al. (2018). Chromosomal abnormalities and copy number variations in fetal ventricular septal defects. Mol. Cytogenet. 11, 58. doi:10.1186/s13039-018-0408-y
Chaithra, S., Agarwala, S., and Ramachandra, N. B. (2022). High-risk genes involved in common septal defects of congenital heart disease. Gene 840, 146745. doi:10.1016/j.gene.2022.146745
Chau, A. C., Jones, A., Sutherland, M., Lilje, C., Sernich, S., Hagan, J., et al. (2018). Characteristics of isolated ventricular septal defects less likely to close in utero. J. Ultrasound Med. Official J. Am. Inst. Ultrasound Med. 37 (8), 1891–1898. doi:10.1002/jum.14535
Chen, X., Zhao, S., Dong, X., Liu, J., Guo, Y., Ju, W., et al. (2023). Incidence, distribution, disease spectrum, and genetic deficits of congenital heart defects in China: implementation of prenatal ultrasound screening identified 18,171 affected fetuses from 2,452,249 pregnancies. Cell & Biosci. 13 (1), 229. doi:10.1186/s13578-023-01172-7
Chen, Y., Mao, J., Sun, Y., Zhang, Q., Cheng, H.-B., Yan, W.-H., et al. (2010). A novel mutation of GATA4 in a familial atrial septal defect. Clin. Chimica Acta; Int. J. Clin. Chem. 411 (21-22), 1741–1745. doi:10.1016/j.cca.2010.07.021
Cheng, K., Zhou, H., Fu, F., Lei, T., Li, F., Huang, R., et al. (2022). Should prenatal chromosomal microarray analysis be offered for isolated ventricular septal defect? A single-center retrospective study from China. Front. Cardiovasc. Med. 9, 988438. doi:10.3389/fcvm.2022.988438
Cho, Y.-S., Park, S. E., Hong, S.-K., Jeong, N.-Y., and Choi, E.-Y. (2017). The natural history of fetal diagnosed isolated ventricular septal defect. Prenat. Diagn. 37 (9), 889–893. doi:10.1002/pd.5100
D'Aurizio, R., Pippucci, T., Tattini, L., Giusti, B., Pellegrini, M., and Magi, A. (2016). Enhanced copy number variants detection from whole-exome sequencing data using EXCAVATOR2. Nucleic Acids Res. 44 (20), e154. doi:10.1093/nar/gkw695
Dawood, Y., Buijtendijk, M. F. J., Shah, H., Smit, J. A., Jacobs, K., Hagoort, J., et al. (2022). Imaging fetal anatomy. Seminars Cell & Dev. Biol. 131, 78–92. doi:10.1016/j.semcdb.2022.02.023
Du, L., Xie, H.-N., Huang, L.-H., Xie, Y.-J., and Wu, L.-H. (2016). Prenatal diagnosis of submicroscopic chromosomal aberrations in fetuses with ventricular septal defects by chromosomal microarray-based analysis. Prenat. Diagn. 36 (13), 1178–1184. doi:10.1002/pd.4953
Eckerström, F., Nyboe, C., Maagaard, M., Redington, A., and Hjortdal, V. E. (2023). Survival of patients with congenital ventricular septal defect. Eur. Heart J. 44 (1), 54–61. doi:10.1093/eurheartj/ehac618
Eckerström, F., Rex, C. E., Maagaard, M., Heiberg, J., Rubak, S., Redington, A., et al. (2020). Cardiopulmonary dysfunction in adults with a small, unrepaired ventricular septal defect: a long-term follow-up. Int. J. Cardiol. 306, 168–174. doi:10.1016/j.ijcard.2020.02.069
Ehrlich, L., and Prakash, S. K. (2022). Copy-number variation in congenital heart disease. Curr. Opin. Genet. & Dev. 77, 101986. doi:10.1016/j.gde.2022.101986
Erol, O., Sevket, O., Keskin, S., Yazıcıoğlu, H. F., and Gül, A. (2014). Natural history of prenatal isolated muscular ventricular septal defects. J. Turkish Ger. Gynecol. Assoc. 15 (2), 96–99. doi:10.5152/jtgga.2014.0012
Fu, F., Deng, Q., Lei, T.-Y., Li, R., Jing, X.-Y., Yang, X., et al. (2017). Clinical application of SNP array analysis in fetuses with ventricular septal defects and normal karyotypes. Archives Gynecol. Obstetrics 296 (5), 929–940. doi:10.1007/s00404-017-4518-2
Fu, F., Li, R., Li, Y., Nie, Z. Q., Lei, T., Wang, D., et al. (2018). Whole exome sequencing as a diagnostic adjunct to clinical testing in fetuses with structural abnormalities. Ultrasound Obstetrics & Gynecol. Official J. Int. Soc. Ultrasound Obstetrics Gynecol. 51 (4), 493–502. doi:10.1002/uog.18915
Gallagher, D., Voronova, A., Zander, M. A., Cancino, G. I., Bramall, A., Krause, M. P., et al. (2015). Ankrd11 is a chromatin regulator involved in autism that is essential for neural development. Dev. Cell 32 (1), 31–42. doi:10.1016/j.devcel.2014.11.031
Gedikbaşı, A., Oztarhan, K., Yıldırım, G., Gül, A., Asar-Canaz, E., and Ceylan, Y. (2010). Atrioventricular and ventricular septal defects; topographical analysis and impact of associated cardiac and extracardiac findings and postpartum outcome. J. Turkish Ger. Gynecol. Assoc. 11 (1), 8–15.
Giang, K. W., Mandalenakis, Z., Fedchenko, M., Eriksson, P., Rosengren, A., Norman, M., et al. (2023). Congenital heart disease: changes in recorded birth prevalence and cardiac interventions over the past half-century in Sweden. Eur. J. Prev. Cardiol. 30 (2), 169–176. doi:10.1093/eurjpc/zwac227
Authoranonmys (2020). Global burden of 369 diseases and injuries in 204 countries and territories, 1990-2019: a systematic analysis for the Global Burden of Disease Study 2019. Lancet London, Engl. 396 (10258), 1204–1222. doi:10.1016/S0140-6736(20)30925-9
Gómez, O., Martínez, J. M., Olivella, A., Bennasar, M., Crispi, F., Masoller, N., et al. (2014). Isolated ventricular septal defects in the era of advanced fetal echocardiography: risk of chromosomal anomalies and spontaneous closure rate from diagnosis to age of 1 year. Ultrasound Obstetrics & Gynecol. Official J. Int. Soc. Ultrasound Obstetrics Gynecol. 43 (1), 65–71. doi:10.1002/uog.12527
Goor, D. A., Edwards, J. E., and Lillehei, C. W. (1970). The development of the interventricular septum of the human heart; correlative morphogenetic study. Chest 58 (5), 453–467. doi:10.1378/chest.58.5.453
Gordin Kopylov, L., Dekel, N., Maymon, R., Feldman, N., Zimmerman, A., Hadas, D., et al. (2022). Prenatally diagnosed isolated perimembranous ventricular septal defect: genetic and clinical implications. Prenat. Diagn. 42 (4), 461–468. doi:10.1002/pd.6128
Gremer, L., Merbitz-Zahradnik, T., Dvorsky, R., Cirstea, I. C., Kratz, C. P., Zenker, M., et al. (2011). Germline KRAS mutations cause aberrant biochemical and physical properties leading to developmental disorders. Hum. Mutat. 32 (1), 33–43. doi:10.1002/humu.21377
Harden, B., Tian, X., Giese, R., Nakhleh, N., Kureshi, S., Francis, R., et al. (2014). Increased postoperative respiratory complications in heterotaxy congenital heart disease patients with respiratory ciliary dysfunction. J. Thorac. Cardiovasc. Surg. 147 (4), 1291–1298. doi:10.1016/j.jtcvs.2013.06.018
He, J., Xie, L., Yu, L., Liu, L., Xu, H., Wang, T., et al. (2022). Maternal serum CFHR4 protein as a potential non-invasive marker of ventricular septal defects in offspring: evidence from a comparative proteomics study. Clin. Proteomics 19 (1), 17. doi:10.1186/s12014-022-09356-y
Huang, X., Gao, Y., Chen, W., Sheng, W., and Huang, G. (2023). Noncardiac anomalies in children with congenital heart disease. Front. Cardiovasc. Med. 10, 1293210. doi:10.3389/fcvm.2023.1293210
Jin, S. C., Homsy, J., Zaidi, S., Lu, Q., Morton, S., DePalma, S. R., et al. (2017). Contribution of rare inherited and de novo variants in 2,871 congenital heart disease probands. Nat. Genet. 49 (11), 1593–1601. doi:10.1038/ng.3970
Jin, Y., Ai, L., Chai, X., Tang, P., Zhang, W., Yang, L., et al. (2021). Maternal circulating exosomal miRNAs as non-invasive biomarkers for the prediction of fetal ventricular septal defect. Front. Genet. 12, 717208. doi:10.3389/fgene.2021.717208
Kabra, M., and Gulati, S. (2003). Mental retardation. Indian J. Pediatr. 70 (2), 153–158. doi:10.1007/BF02723745
Kim, J.-H., Shinde, D. N., Reijnders, M. R. F., Hauser, N. S., Belmonte, R. L., Wilson, G. R., et al. (2016). De novo mutations in SON disrupt RNA splicing of genes essential for brain development and metabolism, causing an intellectual-disability syndrome. Am. J. Hum. Genet. 99 (3), 711–719. doi:10.1016/j.ajhg.2016.06.029
Lee, G., Fong, S. X., Salpekar, M., Satterwhite, C. L., and Weiner, C. (2016). Is an isolated ventricular septal defect detected before 24 weeks on ultrasound associated with fetal aneuploidy? J. Maternal-fetal & Neonatal Med. Official J. Eur. Assoc. Perinat. Med. Fed. Asia Ocean. Perinat. Soc. Int. Soc. Perinat. Obstetricians 29 (10), 1687–1690. doi:10.3109/14767058.2015.1059810
Li, R., Fu, F., Yu, Q., Wang, D., Jing, X., Zhang, Y., et al. (2020). Prenatal exome sequencing in fetuses with congenital heart defects. Clin. Genet. 98 (3), 215–230. doi:10.1111/cge.13774
Li, X., Song, G.-X., Wu, L.-J., Chen, Y.-M., Fan, Y., Wu, Y., et al. (2016). Prediction of spontaneous closure of isolated ventricular septal defects in utero and postnatal life. BMC Pediatr. 16 (1), 207. doi:10.1186/s12887-016-0735-2
Liu, Y., Chen, S., Zühlke, L., Black, G. C., Choy, M.-K., Li, N., et al. (2019). Global birth prevalence of congenital heart defects 1970-2017: updated systematic review and meta-analysis of 260 studies. Int. J. Epidemiol. 48 (2), 455–463. doi:10.1093/ije/dyz009
Lord, J., McMullan, D. J., Eberhardt, R. Y., Rinck, G., Hamilton, S. J., Quinlan-Jones, E., et al. (2019). Prenatal exome sequencing analysis in fetal structural anomalies detected by ultrasonography (PAGE): a cohort study. Lancet London, Engl. 393 (10173), 747–757. doi:10.1016/S0140-6736(18)31940-8
Lu, Q., Luo, L., Zeng, B., Luo, H., Wang, X., Qiu, L., et al. (2024). Prenatal chromosomal microarray analysis in a large Chinese cohort of fetuses with congenital heart defects: a single center study. Orphanet J. Rare Dis. 19 (1), 307. doi:10.1186/s13023-024-03317-4
Mastromoro, G., Khaleghi Hashemian, N., Guadagnolo, D., Giuffrida, M. G., Torres, B., Bernardini, L., et al. (2022). Chromosomal microarray analysis in fetuses detected with isolated cardiovascular malformation: a multicenter study, systematic review of the literature and meta-analysis. Diagn. Basel, Switz. 12 (6), 1328. doi:10.3390/diagnostics12061328
Maulik, D., Nanda, N. C., Maulik, D., and Vilchez, G. (2017). A brief history of fetal echocardiography and its impact on the management of congenital heart disease. Echocardiogr. Mt. Kisco, N.Y. 34 (12), 1760–1767. doi:10.1111/echo.13713
Maya, I., Singer, A., Yonath, H., Reches, A., Rienstein, S., Zeligson, S., et al. (2020). What have we learned from 691 prenatal chromosomal microarrays for ventricular septal defects? Acta Obstetricia Gynecol. Scand. 99 (6), 757–764. doi:10.1111/aogs.13708
Meisner, J. K., and Martin, D. M. (2020). Congenital heart defects in CHARGE: the molecular role of CHD7 and effects on cardiac phenotype and clinical outcomes. Am. J. Med. Genet. Part C, Seminars Med. Genet. 184 (1), 81–89. doi:10.1002/ajmg.c.31761
Miyake, T. (2020). A review of isolated muscular ventricular septal defect. World J. Pediatr. WJP 16 (2), 120–128. doi:10.1007/s12519-019-00289-5
Mosimann, B., Zidere, V., Simpson, J. M., and Allan, L. D. (2014). Outcome and requirement for surgical repair following prenatal diagnosis of ventricular septal defect. Ultrasound Obstetrics & Gynecol. Official J. Int. Soc. Ultrasound Obstetrics Gynecol. 44 (1), 76–81. doi:10.1002/uog.13284
Nederend, I., de Geus, E. J. C., Blom, N. A., and Ten Harkel, A. D. J. (2018). Long-term follow-up after ventricular septal defect repair in children: cardiac autonomic control, cardiac function and exercise capacity. Eur. J. Cardio-thoracic Surg. Official J. Eur. Assoc. For Cardio-thoracic Surg. 53 (5), 1082–1088. doi:10.1093/ejcts/ezx438
Nora, J. J. (1968). Multifactorial inheritance hypothesis for the etiology of congenital heart diseases. The genetic-environmental interaction. Circulation 38 (3), 604–617. doi:10.1161/01.cir.38.3.604
Nurmi, M. O., Pitkänen-Argillander, O., Räsänen, J., and Sarkola, T. (2022). Accuracy of fetal echocardiography diagnosis and anticipated perinatal and early postnatal care in congenital heart disease in mid-gestation. Acta Obstetricia Gynecol. Scand. 101 (10), 1112–1119. doi:10.1111/aogs.14423
Paladini, D., Palmieri, S., Lamberti, A., Teodoro, A., Martinelli, P., and Nappi, C. (2000). Characterization and natural history of ventricular septal defects in the fetus. Ultrasound Obstetrics & Gynecol. Official J. Int. Soc. Ultrasound Obstetrics Gynecol. 16 (2), 118–122. doi:10.1046/j.1469-0705.2000.00202.x
Pannone, L., Bocchinfuso, G., Flex, E., Rossi, C., Baldassarre, G., Lissewski, C., et al. (2017). Structural, functional, and clinical characterization of a novel PTPN11 mutation cluster underlying noonan syndrome. Hum. Mutat. 38 (4), 451–459. doi:10.1002/humu.23175
Peng, T., Wang, L., Zhou, S.-F., and Li, X. (2010). Mutations of the GATA4 and NKX2.5 genes in Chinese pediatric patients with non-familial congenital heart disease. Genetica 138 (11-12), 1231–1240. doi:10.1007/s10709-010-9522-4
Perrot, A., and Rickert-Sperling, S. (2024). Human genetics of ventricular septal defect. Adv. Exp. Med. Biol. 1441, 505–534. doi:10.1007/978-3-031-44087-8_27
Petrovski, S., Aggarwal, V., Giordano, J. L., Stosic, M., Wou, K., Bier, L., et al. (2019). Whole-exome sequencing in the evaluation of fetal structural anomalies: a prospective cohort study. Lancet London, Engl. 393 (10173), 758–767. doi:10.1016/S0140-6736(18)32042-7
Pierpont, M. E., Basson, C. T., Benson, D. W., Gelb, B. D., Giglia, T. M., Goldmuntz, E., et al. (2007). Genetic basis for congenital heart defects: current knowledge: a scientific statement from the American heart association congenital cardiac defects committee, council on cardiovascular disease in the young: endorsed by the American academy of pediatrics. Circulation 115 (23), 3015–3038. doi:10.1161/CIRCULATIONAHA.106.183056
Qiao, F., Wang, Y., Zhang, C., Zhou, R., Wu, Y., Wang, C., et al. (2021). Comprehensive evaluation of genetic variants using chromosomal microarray analysis and exome sequencing in fetuses with congenital heart defect. Ultrasound Obstetrics & Gynecol. Official J. Int. Soc. Ultrasound Obstetrics Gynecol. 58 (3), 377–387. doi:10.1002/uog.23532
Rajiah, P., Mak, C., Dubinksy, T. J., and Dighe, M. (2011). Ultrasound of fetal cardiac anomalies. AJR. Am. J. Roentgenol. 197 (4), W747–W760. doi:10.2214/AJR.10.7287
Raucher Sternfeld, A., Sheffy, A., Tamir, A., Mizrachi, Y., Assa, S., Shohat, M., et al. (2022). Isolated ventricular septal defects demonstrated by fetal echocardiography: prenatal course and postnatal outcome. J. Maternal-fetal & Neonatal Med. Official J. Eur. Assoc. Perinat. Med. Fed. Asia Ocean. Perinat. Soc. Int. Soc. Perinat. Obstetricians 35 (1), 129–133. doi:10.1080/14767058.2020.1712710
Roth, G. A., Mensah, G. A., Johnson, C. O., Addolorato, G., Ammirati, E., Baddour, L. M., et al. (2020). Global burden of cardiovascular diseases and risk factors, 1990-2019: update from the GBD 2019 study. J. Am. Coll. Cardiol. 76 (25), 2982–3021. doi:10.1016/j.jacc.2020.11.010
Selhorst, S., Habib, P. A., Chaves, A. H., and Turan, S. (2024). Early clinical outcomes in infants with prenatally diagnosed perimembranous and muscular ventricular septal defects (VSDs). Pediatr. Cardiol. 45 (3), 491–499. doi:10.1007/s00246-023-03390-9
Shen, O., Lieberman, S., Farber, B., Terner, D., Lahad, A., and Levy-Lahad, E. (2014). Prenatal isolated ventricular septal defect may not Be associated with trisomy 21. J. Clin. Med. 3 (2), 432–439. doi:10.3390/jcm3020432
Smemo, S., Campos, L. C., Moskowitz, I. P., Krieger, J. E., Pereira, A. C., and Nobrega, M. A. (2012). Regulatory variation in a TBX5 enhancer leads to isolated congenital heart disease. Hum. Mol. Genet. 21 (14), 3255–3263. doi:10.1093/hmg/dds165
Southard, A. E., Edelmann, L. J., and Gelb, B. D. (2012). Role of copy number variants in structural birth defects. Pediatrics 129 (4), 755–763. doi:10.1542/peds.2011-2337
Sperling, S., Grimm, C. H., Dunkel, I., Mebus, S., Sperling, H.-P., Ebner, A., et al. (2005). Identification and functional analysis of CITED2 mutations in patients with congenital heart defects. Hum. Mutat. 26 (6), 575–582. doi:10.1002/humu.20262
Su, D., Song, J.-X., Gao, Q., Guan, L., Li, Q., Shi, C., et al. (2016). Cited2 participates in cardiomyocyte apoptosis and maternal diabetes-induced congenital heart abnormality. Biochem. Biophysical Res. Commun. 479 (4), 887–892. doi:10.1016/j.bbrc.2016.09.101
Sukenik-Halevy, R., Sukenik, S., Koifman, A., Alpert, Y., Hershkovitz, R., Levi, A., et al. (2016). Clinical aspects of prenatally detected congenital heart malformations and the yield of chromosomal microarray analysis. Prenat. Diagn. 36 (13), 1185–1191. doi:10.1002/pd.4954
Svirsky, R., Brabbing-Goldstein, D., Rozovski, U., Kapusta, L., Reches, A., and Yaron, Y. (2019). The genetic and clinical outcome of isolated fetal muscular ventricular septal defect (VSD). J. Maternal-fetal & Neonatal Med. Official J. Eur. Assoc. Perinat. Med. Fed. Asia Ocean. Perinat. Soc. Int. Soc. Perinat. Obstetricians 32 (17), 2837–2841. doi:10.1080/14767058.2018.1449829
Swisher, M., Jonas, R., Tian, X., Lee, E. S., Lo, C. W., and Leatherbury, L. (2011). Increased postoperative and respiratory complications in patients with congenital heart disease associated with heterotaxy. J. Thorac. Cardiovasc. Surg. 141 (3), 637–644. doi:10.1016/j.jtcvs.2010.07.082
Testard, Q., Vanhoye, X., Yauy, K., Naud, M.-E., Vieville, G., Rousseau, F., et al. (2022). Exome sequencing as a first-tier test for copy number variant detection: retrospective evaluation and prospective screening in 2418 cases. J. Med. Genet. 59 (12), 1234–1240. doi:10.1136/jmg-2022-108439
Thienpont, B., Mertens, L., de Ravel, T., Eyskens, B., Boshoff, D., Maas, N., et al. (2007). Submicroscopic chromosomal imbalances detected by array-CGH are a frequent cause of congenital heart defects in selected patients. Eur. Heart J. 28 (22), 2778–2784. doi:10.1093/eurheartj/ehl560
Välimäki, M. J., Tölli, M. A., Kinnunen, S. M., Aro, J., Serpi, R., Pohjolainen, L., et al. (2017). Discovery of small molecules targeting the synergy of cardiac transcription factors GATA4 and NKX2-5. J. Med. Chem. 60 (18), 7781–7798. doi:10.1021/acs.jmedchem.7b00816
van der Linde, D., Konings, E. E. M., Slager, M. A., Witsenburg, M., Helbing, W. A., Takkenberg, J. J. M., et al. (2011). Birth prevalence of congenital heart disease worldwide: a systematic review and meta-analysis. J. Am. Coll. Cardiol. 58 (21), 2241–2247. doi:10.1016/j.jacc.2011.08.025
Vedel, C., Rode, L., Jørgensen, F. S., Petersen, O. B., Sundberg, K., Tabor, A., et al. (2021). Prenatally detected isolated ventricular septum defects and the association with chromosomal aberrations-A nationwide register-based study from Denmark. Prenat. Diagn. 41 (3), 347–353. doi:10.1002/pd.5853
Wang, H., Lin, X., Wang, X., Liu, X., He, S., and Lyu, G. (2023a). Maternal body fluid lncRNAs serve as biomarkers to diagnose ventricular septal defect: from amniotic fluid to plasma. Front. Genet. 14, 1254829. doi:10.3389/fgene.2023.1254829
Wang, H., Lin, X., Wang, Z., He, S., Dong, B., and Lyu, G. (2023b). Differential lncRNA/mRNA expression profiling and ceRNA network analyses in amniotic fluid from foetuses with ventricular septal defects. PeerJ 11, e14962. doi:10.7717/peerj.14962
Wang, H., Liu, Y., Han, S., Zi, Y., Zhang, Y., Kong, R., et al. (2020a). Nkx2-5 regulates the proliferation and migration of H9c2 cells. Med. Sci. Monit. Int. Med. J. Exp. Clin. Res. 26, e925388. doi:10.12659/MSM.925388
Wang, J., Fang, M., Liu, X.-Y., Xin, Y.-F., Liu, Z.-M., Chen, X.-Z., et al. (2011a). A novel GATA4 mutation responsible for congenital ventricular septal defects. Int. J. Mol. Med. 28 (4), 557–564. doi:10.3892/ijmm.2011.715
Wang, J., Xin, Y.-F., Liu, X.-Y., Liu, Z.-M., Wang, X.-Z., and Yang, Y.-Q. (2011b). A novel NKX2-5 mutation in familial ventricular septal defect. Int. J. Mol. Med. 27 (3), 369–375. doi:10.3892/ijmm.2010.585
Wang, Y., Li, R., Fu, F., Huang, R., Li, D., and Liao, C. (2023c). Prenatal genetic diagnosis associated with fetal ventricular septal defect: an assessment based on chromosomal microarray analysis and exome sequencing. Front. Genet. 14, 1260995. doi:10.3389/fgene.2023.1260995
Wang, Y., Zhang, J., Feng, W., Cao, H., He, Q., Xu, J., et al. (2020b). Description of misdiagnosis and missed diagnosis of fetal complex heart malformations by prenatal echocardiography combined with postnatal cardiovascular casting. Prenat. Diagn. 40 (7), 792–802. doi:10.1002/pd.5689
Xie, G., Lee, J.-E., Senft, A. D., Park, Y.-K., Jang, Y., Chakraborty, S., et al. (2023). MLL3/MLL4 methyltransferase activities control early embryonic development and embryonic stem cell differentiation in a lineage-selective manner. Nat. Genet. 55 (4), 693–705. doi:10.1038/s41588-023-01356-4
Yagel, S., Cohen, S. M., Rosenak, D., Messing, B., Lipschuetz, M., Shen, O., et al. (2011). Added value of three-/four-dimensional ultrasound in offline analysis and diagnosis of congenital heart disease. Ultrasound Obstetrics & Gynecol. Official J. Int. Soc. Ultrasound Obstetrics Gynecol. 37 (4), 432–437. doi:10.1002/uog.8867
Yang, Y., Yang, H., Lian, X., Yang, S., Shen, H., Wu, S., et al. (2022). Circulating microRNA: myocardium-derived prenatal biomarker of ventricular septal defects. Front. Genet. 13, 899034. doi:10.3389/fgene.2022.899034
Yang, Y.-Q., Li, L., Wang, J., Liu, X.-Y., Chen, X.-Z., Zhang, W., et al. (2012). A novel GATA4 loss-of-function mutation associated with congenital ventricular septal defect. Pediatr. Cardiol. 33 (4), 539–546. doi:10.1007/s00246-011-0146-y
Yi, T., Hao, X., Sun, H., Zhang, Y., Han, J., Gu, X., et al. (2023). Genetic aetiology distribution of 398 foetuses with congenital heart disease in the prenatal setting. Esc. Heart Fail. 10 (2), 917–930. doi:10.1002/ehf2.14209
Zhang, W., Li, X., Shen, A., Jiao, W., Guan, X., and Li, Z. (2008). GATA4 mutations in 486 Chinese patients with congenital heart disease. Eur. J. Med. Genet. 51 (6), 527–535. doi:10.1016/j.ejmg.2008.06.005
Zhao, L., Chen, L., Yang, T., Wang, T., Zhang, S., Chen, L., et al. (2020a). Birth prevalence of congenital heart disease in China, 1980-2019: a systematic review and meta-analysis of 617 studies. Eur. J. Epidemiol. 35 (7), 631–642. doi:10.1007/s10654-020-00653-0
Zhao, L., Liu, H., Yuan, X., Gao, K., and Duan, J. (2020b). Comparative study of whole exome sequencing-based copy number variation detection tools. BMC Bioinforma. 21 (1), 97. doi:10.1186/s12859-020-3421-1
Zhao, X., Shen, Y., Kong, D., Li, W., Yao, L., Li, S., et al. (2024). Correlation between types of ventricular septal defect and chromosomal abnormalities in low-risk non-invasive prenatal testing. Archives Gynecol. Obstetrics 310 (3), 1517–1523. doi:10.1007/s00404-024-07566-3
Zhu, X., Li, J., Ru, T., Wang, Y., Xu, Y., Yang, Y., et al. (2016). Identification of copy number variations associated with congenital heart disease by chromosomal microarray analysis and next-generation sequencing. Prenat. Diagn. 36 (4), 321–327. doi:10.1002/pd.4782
Keywords: isolated ventricular septal defect, prenatal diagnosis, genetic counseling, pregnancy outcome, molecular genetic technologies
Citation: Chen X, Zhang Q, Lu M, Feng Q, Qin L and Liao S (2024) Prenatal finding of isolated ventricular septal defect: genetic association, outcomes and counseling. Front. Genet. 15:1447216. doi: 10.3389/fgene.2024.1447216
Received: 11 June 2024; Accepted: 20 September 2024;
Published: 02 October 2024.
Edited by:
Wei Xu, Texas A&M University Corpus Christi, United StatesReviewed by:
Emine Ikbal Atli, Trakya University, TürkiyeJingbin Yan, Shanghai Children’s Hospital, China
Copyright © 2024 Chen, Zhang, Lu, Feng, Qin and Liao. This is an open-access article distributed under the terms of the Creative Commons Attribution License (CC BY). The use, distribution or reproduction in other forums is permitted, provided the original author(s) and the copyright owner(s) are credited and that the original publication in this journal is cited, in accordance with accepted academic practice. No use, distribution or reproduction is permitted which does not comply with these terms.
*Correspondence: Shixiu Liao, eWNoc2xzaHhAenp1LmVkdS5jbg==; Litao Qin, bGl0YW9fcWluQHp6dS5lZHUuY24=
†These authors have contributed equally to this work